- 1Centre Hospitalier Universitaire de Québec - Research Centre and Department of Obstetrics, Gynecology and Reproduction, Faculty of Medicine, Université Laval, Québec, QC, Canada
- 2Nephrology Division, Department of Medicine, Massachusetts General Hospital, Harvard Medical School, Boston, MA, United States
Purinergic receptors are ubiquitously expressed throughout the body and they participate in the autocrine and paracrine regulation of cell function during normal physiological and pathophysiological conditions. Extracellular nucleotides activate several types of plasma membrane purinergic receptors that form three distinct families: P1 receptors are activated by adenosine, P2X receptors are activated by ATP, and P2Y receptors are activated by nucleotides including ATP, ADP, UTP, UDP, and UDP-glucose. These specific pharmacological fingerprints and the distinct intracellular signaling pathways they trigger govern a large variety of cellular responses in an organ-specific manner. As such, purinergic signaling regulates several physiological cell functions, including cell proliferation, differentiation and death, smooth muscle contraction, vasodilatation, and transepithelial transport of water, solute, and protons, as well as pathological pathways such as inflammation. While purinergic signaling was first discovered more than 90 years ago, we are just starting to understand how deleterious signals mediated through purinergic receptors may be involved in male infertility. A large fraction of male infertility remains unexplained illustrating our poor understanding of male reproductive health. Purinergic signaling plays a variety of physiological and pathophysiological roles in the male reproductive system, but our knowledge in this context remains limited. This review focuses on the distribution of purinergic receptors in the testis, epididymis, and vas deferens, and their role in the establishment and maintenance of male fertility.
Introduction
Purinergic signaling was first described in 1929 by Drury and Szent-Gyorgyi who showed extracellular adenosine-induced transient effects on the mammalian heart (1). The term “purinergic” was then proposed by Geoffrey Burnstock who demonstrated in 1970 that adenosine 5’-triphosphate (ATP) acts as an extracellular mediator in the gut (2). It is now known that, through evolutionarily conserved autocrine and paracrine communication mechanisms, extracellular adenosine, ATP, and other types of nucleotides activate members of the membrane-bound purinoceptor family (3–8). Receptors that bind adenosine were named P1 purinergic receptors, and those that bind ATP were named P2 purinergic receptors (9) (Figure 1). P1 receptors are G protein-coupled receptors (GPCRs) activated by adenosine. The P2 family was later subdivided into P2X ionotropic receptors and P2Y metabotropic receptors. P2X receptors are ligand-gated non-selective cation channels activated by ATP, and P2Y receptors are GPCRs activated by adenine and several uracil nucleotides (ATP, ADP, UTP, UDP and UDP-glucose (6, 10–19) (Figure 1).
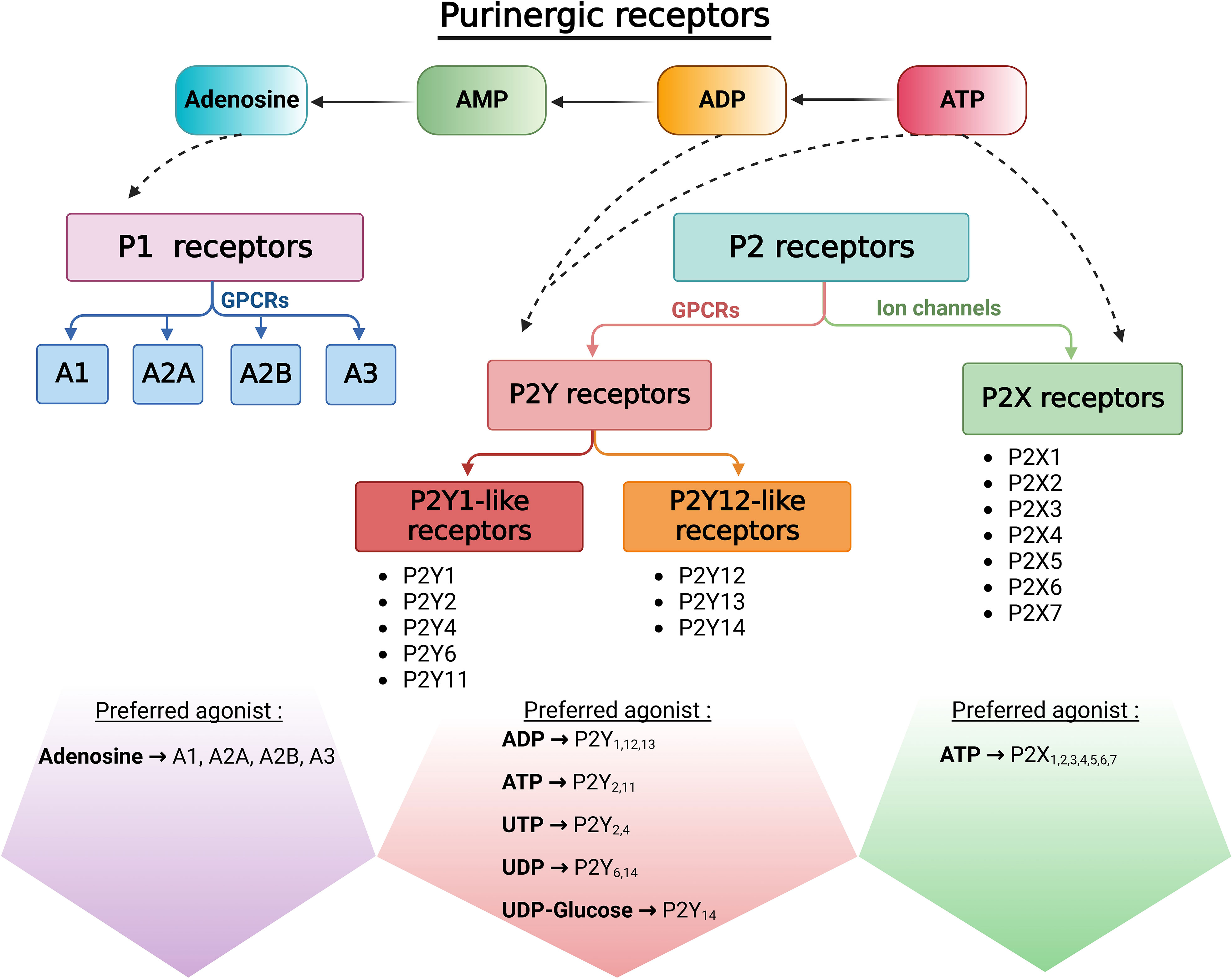
Figure 1 Classification of purinergic receptors. Once released into the extracellular space, ATP is rapidly hydrolyzed by ectonucleotidases to generate ADP, AMP, and adenosine. Adenosine activates P1 receptors, a family of G-protein coupled receptors (GPCRs). P2X receptors are non-selective cation channels that open in response to ATP by their only endogenous ligand. In addition to ATP and ADP, P2Y metabotropic receptors can mediate cellular responses to uracil nucleotides and nucleotides sugars.
Purinergic receptors are ubiquitously expressed throughout the body and they participate in intercellular communication during normal physiological and pathophysiological conditions. This review focuses on the distribution of purinergic receptors in the male reproductive tract, and their role in the establishment and maintenance of male fertility.
The purinergic receptor family
P1, P2X, and P2Y receptors are expressed by virtually all cell types, and they participate in several cell functions, including cell proliferation, differentiation, and death, through the modulation of a variety of intracellular signaling pathways. Other physiological functions regulated by these receptors include smooth muscle contraction, vasodilatation, and transepithelial transport of water, solute, and protons, as well as pathological pathways including inflammation.
The P2X receptor family includes seven homotrimeric (P2X1–7) and several heterotrimeric isoforms (e.g. P2X2, 4, 6) (Figure 2). These isoforms can be distinguished through their ligand affinities, activation and desensitization kinetics, as well as specific pharmacological fingerprints (11, 20). P2X receptors are formed by two transmembrane domains separated by a large extracellular domain and have both their N- and C-termini domains located on the cytoplasmic side of the plasma membrane (21). Activation of P2X receptors by ATP triggers conformational changes that lead to the influx of calcium and sodium, or the efflux of potassium via the opening of a non-selective cation-permeable channel pore (22). The P2Y receptors consist of seven transmembrane domains with an extracellular N-terminus domain and intracellular C-terminus domain (Figure 2). This family is formed by eight isoforms (P2Y1,2,4,6,11,12,13,14) that are coupled to different G proteins and activate distinct intracellular signaling pathways (11, 21). The missing numbers in the P2Y receptor series indicate receptors that are unresponsive to nucleotides. The gene coding for the P2Y11 receptor is absent in the rodent genome. The P2Y1-like receptors P2Y1,2,4,6 activate Gq and phospholipase C (PLC). PLC hydrolyzes phosphatidylinositol-4,5-bisphosphate to form inositol1,4,5-trisphosphate (IP3) and diacylglycerol (DAG), leading to the release of Ca2+ from intracellular calcium storage organelles and activation of protein kinase C (PKC), respectively. The P2Y11 receptor couples to both Gq and Gs, and its activation thus increases intracellular Ca2+ and cAMP. The P2Y12-like receptors, P2Y12,13,14 are coupled to Gi and reduce intracellular cAMP concentration through inhibition of adenylyl cyclase (Figure 2). P2Y receptors were later shown to modulate additional intracellular signaling pathways, including the recruitment of the Gβγ subunit followed by activation of phosphatidylinositol-4,5-biphosphate 3-kinase (PI3K), PLC-β2 and PLC-β3, GPCR kinase 2 and 3, Rho, and MAPKs (23). Different affinities of the P2X and P2Y receptors for ATP and nucleotides confer functional specificity and physiological flexibility to various cellular functions. As such, the P2 receptor expression profile of a given cell underlies its unique response phenotype upon ATP stimulation. In addition, the degradation of extracellular ATP into ADP or UDP modulates the cellular response via activation of different P2X and P2Y receptors (24, 25). On the other hand, ATP is rapidly hydrolyzed into adenosine by extracellular ectonucleotidases (26–29). Adenosine binds to P1 receptors and triggers opposite effects in cells by either inducing a decrease in intracellular cAMP, through ADORA1 (A1) and ADORA3 (A3) receptors that are coupled to Gi/o, or a cAMP increase through ADORA2A (A2A) or ADORA2B (A2B) receptors that are coupled to Gs (Figure 2).
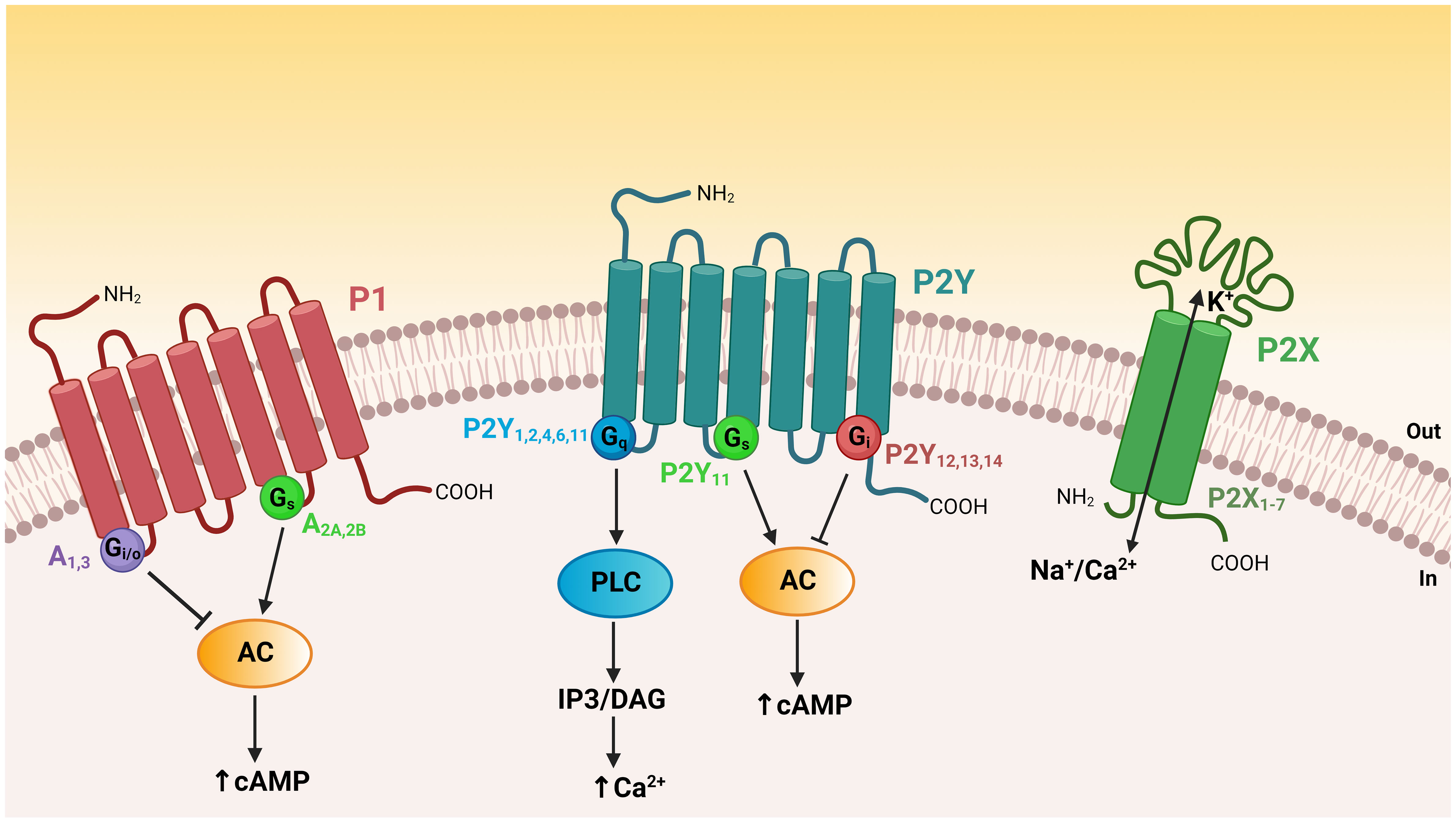
Figure 2 Topology of purinergic receptors and intracellular signaling pathways. The P2X receptors have two transmembrane domains with both their N- and C-termini on the cytoplasmic side of the plasma membrane. Activation of P2X receptors by extracellular ATP opens a non-selective cation channel, resulting in calcium and sodium influx or potassium efflux. P1 and P2Y receptors are GPCRs possessing seven transmembrane domains with an extracellular N-terminus domain and an intracellular C-terminus domain. P2Y1-like receptors (P2Y1,2,4,6,11) are coupled to Gq and their activation leads to phospholipase C (PLC) activation, generation of IP3 and DAG, and intracellular Ca2+ release. The P2Y11 receptor couples to both Gq and Gs to increase intracellular Ca2+ and cAMP. P2Y12-like receptors (P2Y12,13,14) are coupled to Gi and thus reduce intracellular cAMP levels through inhibition of adenylyl cyclase (AC). Adenosine P1 receptors differentially regulate the intracellular concentration of cAMP. A1 and A3 receptors are coupled to Gi/o and induce a diminution in cAMP levels, whereas A2A and A2B receptors are coupled to Gs and their activation leads to an elevation in intracellular cAMP.
Physiological and pathophysiological roles of purinergic receptors
Extracellular nucleotides are released in the extracellular fluid during cell lysis, exocytosis, or by efflux through transport proteins located in the cell membranes (30). These nucleotides act in an autocrine or paracrine manner to regulate cellular activity in physiological and pathophysiological conditions via purinergic signaling. Adenosine has been administered clinically since the 1940s to treat supraventricular tachycardia (31, 32), and P2Y12 antagonists are widely used as anti-thrombotic agents (19). In general, adenosine is considered to be tissue protective and anti-inflammatory, in contrast to ATP and UDP-glucose, which are damage-associated molecular pattern (DAMP) molecules that have pro-inflammatory effects (31, 33, 34). In trauma, ischemia, stroke, etc., high levels of extracellular ATP are related to massive inflammatory response and cell death (35). Activation of P2Y1, P2Y2, P2Y6, P2X1 and P2X7 triggers the production of chemokines in monocytes and dendritic cells, and promotes the recruitment of inflammatory cells to inflamed areas (36, 37). Tissue-resident macrophages express P2X4, which mediates Ca2+-dependent PLA2 and COX signaling (36). In addition, ATP acts as a “find-me” signal to promote the effective clearance of apoptotic cells by phagocytes through activation of P2Y2 (38). ATP and adenosine drive neutrophil migration through P2X1, P2Y2 and A3 receptors in an autocrine manner (37, 39).
Several other purinergic modulators are now being considered to treat central nervous system (CNS), kidney, heart, and lung diseases, as well as diabetes, gouty arthritis, obesity, and cancer (6, 19, 31, 33, 34, 40–47). In the CNS, P2X and P2Y receptors regulate synaptic transmission (48). The P2Y13 receptor, for example, participates in pain transmission and neuroprotection (49). P2X3,4,7 and P2Y12 and P2Y14 are involved in maladaptive pain neuroplasticity and their antagonists have been proposed as therapeutic agents to reduce chronic or inflammatory neuropathic pain (44, 50). P2Y1 and P2Y13 are involved in neuronal differentiation and axonal elongation (51, 52), and the participation of P2Y1 is down-regulated by the activation of P2Y13 by ADP or the activation of P2X7 (52). During neurogenesis and early brain development, ectonucleotidases can lead to the downregulation of purinergic signaling, which controls progenitor cell growth and helps neuronal differentiation (53). P2Y12 located in microglial cells directs the extension of microglial processes and participates in the rapid closure of the blood-brain barrier following CNS injury (54). Microglial P2Y12 can also participate in neuron-glia intercellular communication following its activation by ATP secreted by neurons. This pathway further contributes to providing a neuroprotective mechanism after acute brain injury (55). The neurological action of purinergic receptors is important to male reproductive function, where neuro-muscular interactions play a key role in muscle contractions in the vas deferens and epididymis (discussed below). In addition, ATP participates in penile erection, through its action in the corpus cavernosum (56–58).
Purinergic receptors also participate in the regulation of the cardiovascular system (59). In the heart, adenosine, acting mainly on the A1 receptor, influences the cardiac pacemakers causing a negative chronotropic effect (60). In addition, following a decrease in oxygen concentration, erythrocytes release ATP that binds P2Y1 and P2Y2 receptors in blood vessels, leading to an increase in intracellular Ca2+ and production of nitric oxide (NO) that results in vasodilatation (61, 62). In the testis, purinergic signaling is also involved in circulatory disorders through an increase in NO production in spermatic veins and seminal plasma in patients with varicocele (63).
In addition, luminal nucleotides and adenosine are potent modulators of transepithelial transport in nearly all tubular organs. Several P2X, P2Y, and all P1 receptors are differentially expressed throughout the kidney and contribute to the regulation of renal function (33). A particularity of several purinergic receptors is their apical localization in epithelial cells (4). ATP release is activated by mechanical stimulation such as cell swelling and shear stress, and ATP together with its hydrolysis product adenosine are part of local intrarenal mechanisms that help regulate glomerular filtration rate (GFR) (64, 65), and water and electrolyte transport in collecting duct principal cells (33, 66). Renal disease is often associated with acidosis and intra-renal adenosine has a protective effect following kidney injury (43, 67–69). Extracellular adenosine stimulates proton secretion in the collecting duct, via activation of A2A and A2B receptors located on the apical membrane of intercalated cells (70). This would contribute to reducing acidosis, thus providing additional evidence of the protective effect of adenosine in the kidney. In contrast, activation of P2Y14 by UDP-glucose triggers sterile inflammation in the kidney, through the production of pro-inflammatory chemokines in intercalated cells, and infiltration of neutrophils and monocytes, which contribute to renal tubule damage following renal ischemia-reperfusion injury (45). ATP can also have a pro-inflammatory role in the kidney via activation of P2X7. While this receptor is barely detectable in healthy adult kidneys, its expression is increased in rodent models of kidney disease (33). Interestingly, P2X7 KO mice do not develop kidney fibrosis following ureteric obstruction (71).
Activation of purinergic receptors by ATP is now proposed to be part of a non-TLR4-mediated mechanism that protects against bacterial infections in the urinary tract and kidney (33). Bacterial infections can induce host- or bacteria-related ATP local signaling. Exposure of human uroepithelial A498 cells to Escherichia coli (E. coli) induces the release of ATP and P2Y12 activation leading to IL-8 release. Interestingly, the E. coli toxin α-hemolysin (HlyA), forms a large ATP pore in the infected cells that contributes to the inflammatory response (72). HlyA is a stronger inducer of IL-1 production compared to lipopolysaccharide (LPS) (72).
P2X7 is one of the best characterized purinergic receptors in the context of cancer (73), including ovarian (74), mesothelioma (75), pancreatic cell (76), osteosarcoma cell (77), endometrial (78) and skin cancer (79, 80). Activation of P2X7 by ATP leads to a decrease in intracellular potassium levels, activation of inflammasomes followed by cell death by necrosis or apoptosis, and mediates an inflammatory response by causing the release of interleukin IL-1β and IL-18 (33, 36, 72, 81). This pathway confers an anti-tumor role of P2X7 that contributes to the immunogenic death of tumor cells. In addition, P2Y2 was shown to inhibit cell proliferation in several cancers, such as human colorectal carcinoma (82), human oesophageal cancer (83), nasopharyngeal carcinoma (84), endometrial carcinoma (85). Some antitumor therapies, such as chemotherapy and radiation therapy, induce tissue damage and cell death, leading to the release of many DAMP molecules – including ATP (86). These nucleotides in the tumor microenvironment serve as the interface of interaction with the immune system (87).
In the respiratory system, ATP participates in the secretion of mucin through activation of P2Y2 (88) and it regulates the respiratory rhythm (89). UDP-glucose acting on P2Y14 is involved in airway eosinophilia associated with asthma (46). In that study, mice treated with P2Y14 antagonists as well as mice lacking P2Y14 had decreased eosinophilia and airway hyperresponsiveness indicating that this receptor could be targeted to treat asthma exacerbations and glucocorticoid-resistant forms of asthma. P2Y12 also plays a role in bronchial asthma (90), where it is involved in the migration of platelets to the lung tissue and their subsequent activation following allergen exposure (91, 92).
Purinergic receptors in the male reproductive tract and maturing spermatozoa
Purinergic regulation of male reproductive organs was first described more than 20 years ago in studies showing the physiological action of extracellular ATP and adenosine in the vas deferens (93, 94). Years later, the role of P2X receptors in fertility gained particular interest when P2X1 knockout mice were proven infertile, due to a reduction in vas deferens contractions leading to impaired sperm delivery in the ejaculate (95, 96). An increasing number of purinergic receptors are now being described all along the male reproductive tract. Here we focus on the role of purinergic signaling in selected male organs, including the testis, the epididymis, and vas deferens, as well as in spermatozoa. Readers interested in organs located more distally in the male reproductive tract are referred to other excellent reviews (97–99).
Testis
The testis is composed of seminiferous tubules - where spermatogenesis takes place – that are surrounded by an interstitial space that includes various cell types such as Leydig cells and peritubular myoid cells. Sertoli cells in the seminiferous tubules are responsible for the differentiation of germ cells located at the base of the tubule. These maturing cells migrate along the side of Sertoli cells and become spermatozoa, which are delivered into the tubule lumen (100–103).
Purinergic signaling is a critical component of testicular autocrine and paracrine communication (104–111). Different sets of purinergic receptors are expressed in the testis in a cell-specific manner, including Leydig cells (112, 113), Sertoli cells (108, 114), testicular peritubular cells (109), and pre- and post-meiotic germ cells (104, 115) (Figure 3). Several P2X receptors were initially described in the testis, including P2X1,2,3,5,7, while P2X4 and P2X6 were not detected in this organ (115). However, in a previous paper, P2rx4 mRNA expression had been described in mouse seminiferous tubules (116). In later studies, P2X2, 4, 6, and 7 were detected in Leydig cells (103, 112), and electrophysiological experiments suggested that P2X2, 4, and 6 form heteromeric channels (112).
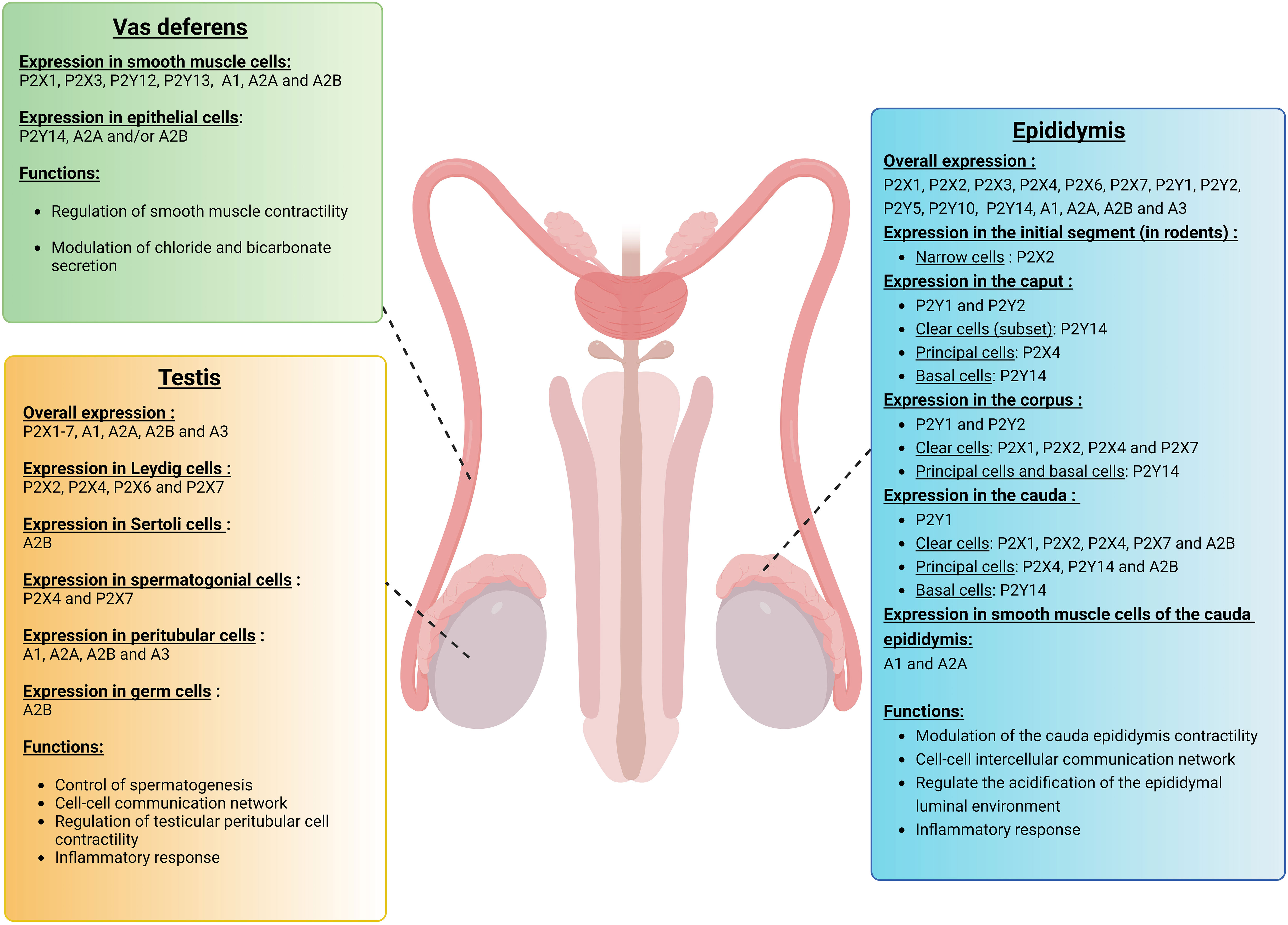
Figure 3 Expression of purinergic receptors in vas deferens, testis, and epididymis. Different sets of purinergic receptors belonging to the P1, P2X, and P2Y families are expressed in the vas deferens, testis, and epididymis. These receptors are involved in various cell functions, including smooth muscle contraction, vasodilatation, transepithelial transport as well as inflammation, and thus play a role in the establishment of male fertility. In the vas deferens, purinergic signaling mostly participates in the regulation of smooth muscle contraction and quality of the semen. In the testis, various purinergic receptors are expressed in a cell-specific manner, including Leydig cells, Sertoli cells, spermatogonial cells, testicular peritubular cells, and germ cells. These purinergic receptors play a critical role in testicular autocrine and paracrine communication. In the epididymis, different subtypes of purinergic receptors are expressed in a cell-specific and segment-specific manner. Purinergic signaling in the epididymis is part of a complex intercellular communication network that leads to the establishment of an optimal acidic extracellular environment.
Regarding the roles of P1 receptors in the testis, the A1 receptor was first detected in rat testes (117, 118). In a separate study, adenosine receptors were detected in Sertoli cells by evaluating the binding of the adenosine analog cyclohexyladenosine in testicular cell extracts from immature rats (119). More recently, mRNA transcripts coding for all P1 receptors, A1, A2A, A2B, and A3, were observed in mouse testicular peritubular cells, while only A1, A2A, and A2B mRNAs were found in human peritubular cells (120). In the same study, A2B protein was localized by immunocytochemistry in human Sertoli cells, germ cells, and peritubular cells. In addition, adenosine and the A2B agonist BAY60-6583 increased IL6 and CXCL8 mRNA levels in isolated human testicular peritubular cells, showing a pro-inflammatory role for adenosine in the testis.
In Leydig and Sertoli cells purinergic signaling controls gonadotrophin responses (106, 107, 121–124). In Sertoli cells, ATP induced the release of calcium from intracellular stores, as well as the influx of calcium and sodium, indicating the participation of both P2X and P2Y receptors (105). ATP-induced sodium-dependent membrane depolarization in these cells triggered estradiol secretion (125). ATP also increased intracellular Ca2+ in Leydig cells triggering the secretion of testosterone. Moreover, in the mouse testis, Sertoli cells were shown to release ATP and adenosine, while germ cells and myoid peritubular cells could release adenosine (106, 122). The secretion of these extracellular mediators is under the control of follicle-stimulating hormone (FSH) and vitamin A (retinol), and participates in the paracrine regulation of testicular function (122, 126). Activation of both P2X and P2Y receptors by ATP in testicular peritubular cells induced their contractions, which triggered directional sperm movement within the mouse seminiferous tubules (127). Collectively, these studies showed that ATP participates in the autocrine regulation of Sertoli cells, as well as in a Sertoli cell to peritubular cell communication mechanism for the transport of spermatozoa in the testis.
Another example of testicular intercellular communication mediated by ATP was illustrated in an elegant study published by Fleck et al. in 2016 (104). Intimate interactions between Sertoli cells and the maturing germ cells are crucial for spermatogenesis. In this study, ATP secreted by Sertoli cells was proposed to activate P2X4 and P2X7 located in spermatogonial cells to modulate synchronized sperm development and release. Interestingly, ATP-induced ATP release was observed, indicating the presence of a mechanism that could increase the paracrine radius of locally generated signals. The testis is an immune-privileged organ whose luminal compartment is separated from the blood circulation by Sertoli cells that form the blood-testicular barrier. Thus, a local cell-cell intercellular communication network mediated by luminal factors such as ATP and adenosine would provide an ideal mechanism through which spermatogenesis could be performed in a synchronized manner while being protected from the peripheral immunological environment.
Epididymis
At their exit from the testis, spermatozoa have acquired their overall morphology characterized by their long flagellum and head that contains densely packed DNA, but they remain immature cells. They acquire their fertilization capacity as they transit in the epididymis, a process called epididymal maturation (128–133). Another role of the epididymis is to protect sperm from the immune system while ensuring protection against ascending and blood pathogens (131, 134–138). Sperm maturation, protection, and storage occur in a unique epididymal luminal environment that has a high osmolarity, high potassium, low sodium, and low bicarbonate concentrations, and an acidic pH compared to blood (139–141). Communication networks between the different epithelial cell types that line the epididymal lumen, as well as between epithelial cells and spermatozoa, ensure tight regulation of this optimal environment (131, 142, 143). In addition, transcriptionally inactive spermatozoa acquire several new proteins that are essential for their fertilization ability as they transit along the epididymal tubule. This process occurs via the production of extracellular vesicles called epididymosomes, which are produced by epithelial cells and fuse with the sperm membrane (144–149).
In rodents, the epididymis is separated from the testis by the efferent ducts (150), and it is segmented into four main regions, the initial segment (IS), the caput, the corpus, and the cauda (151). In humans, efferent ducts occupy a large portion of the proximal epididymis, which is then divided into the caput, corpus, and cauda (152). To our knowledge, very little is known about purinergic signaling in the efferent ductules, except that the pro-inflammatory receptor P2Y14 is expressed in ciliated cells in the proximal regions and unidentified epithelial cells in the distal regions of the human efferent ducts (153). However, elaborate paracrine and autocrine purinergic signaling networks are present in the epididymis. The epididymal epithelium is lined by several cell types including narrow cells (located exclusively in the rodent IS), and clear cells (CC), principal cells (PC), and basal cells (BC).
As mentioned above, several purinergic receptors are located on the luminal side of transporting epithelia (4). The epididymis was the first intact epithelial organ where apical P2 receptors were shown to modulate transepithelial transport (154). Ten years later, the role of A1 and A2A receptors in the contractility of the cauda epididymis was demonstrated (155). Various purinergic receptors belonging to the P1, P2X, and P2Y families, and numerous ectonucleotidases are expressed in the epididymis in a cell type-specific and segment-specific manner (Figure 3) (7, 95, 98, 156–163).
In the cauda epididymis perfused in vivo, the addition of adenosine or ATP to the luminal perfusate induced the redistribution of the proton pumping V-ATPase from intracellular vesicles to the apical membrane in CC in rats (158) and mice (164) (Figure 4). Luminal acidification in this organ is essential for the maintenance of sperm in a quiescent state during their storage and favors the transfer of proteins from epithelial cells to spermatozoa via epididymosomes (140, 141, 148, 165–168). V-ATPase apical accumulation in CC depends upon elevations of intracellular cAMP and calcium (169–171). In the rat epididymis, the apical accumulation of V-ATPase elicited by luminal adenosine was inhibited by myristoylated protein kinase A inhibitor, indicating the contribution of either A2A or A2B receptors (158). Because only A1, A2B, and A3 were detected in epithelial cells isolated by laser-cut microdissection, and because A1 and A3 induce a decrease in cAMP, it was postulated that A2B was responsible for the adenosine action. This was further confirmed in the mouse epididymis, where A2B was located in the apical membrane of CC and where its specific agonist, BAY60-6583, induced V-ATPase apical accumulation in CC (164). A2B was also localized in the apical membrane of PC and its role in the regulation of these cells is currently being evaluated in our laboratory. V-ATPase-dependent proton secretion is activated at luminal alkaline pH (70, 164, 172). However, how these cells can sense and respond to variations in their extracellular environment remains incompletely understood. Modulation of purinergic signaling by extracellular pH would provide such a pH sensory mechanism. Indeed, some P2X receptors such as P2X4, as well as ectonucleotidases are activated at an alkaline pH (173–175). Increased hydrolysis of luminal ATP into adenosine by ectonucleotidases at alkaline pH versus acidic pH was shown to participate in V-ATPase dependent proton secretion (164). On the other hand, activation of P2X4 by ATP could provide an additional mechanism through which V-ATPase-dependent proton secretion would be activated at alkaline pH.
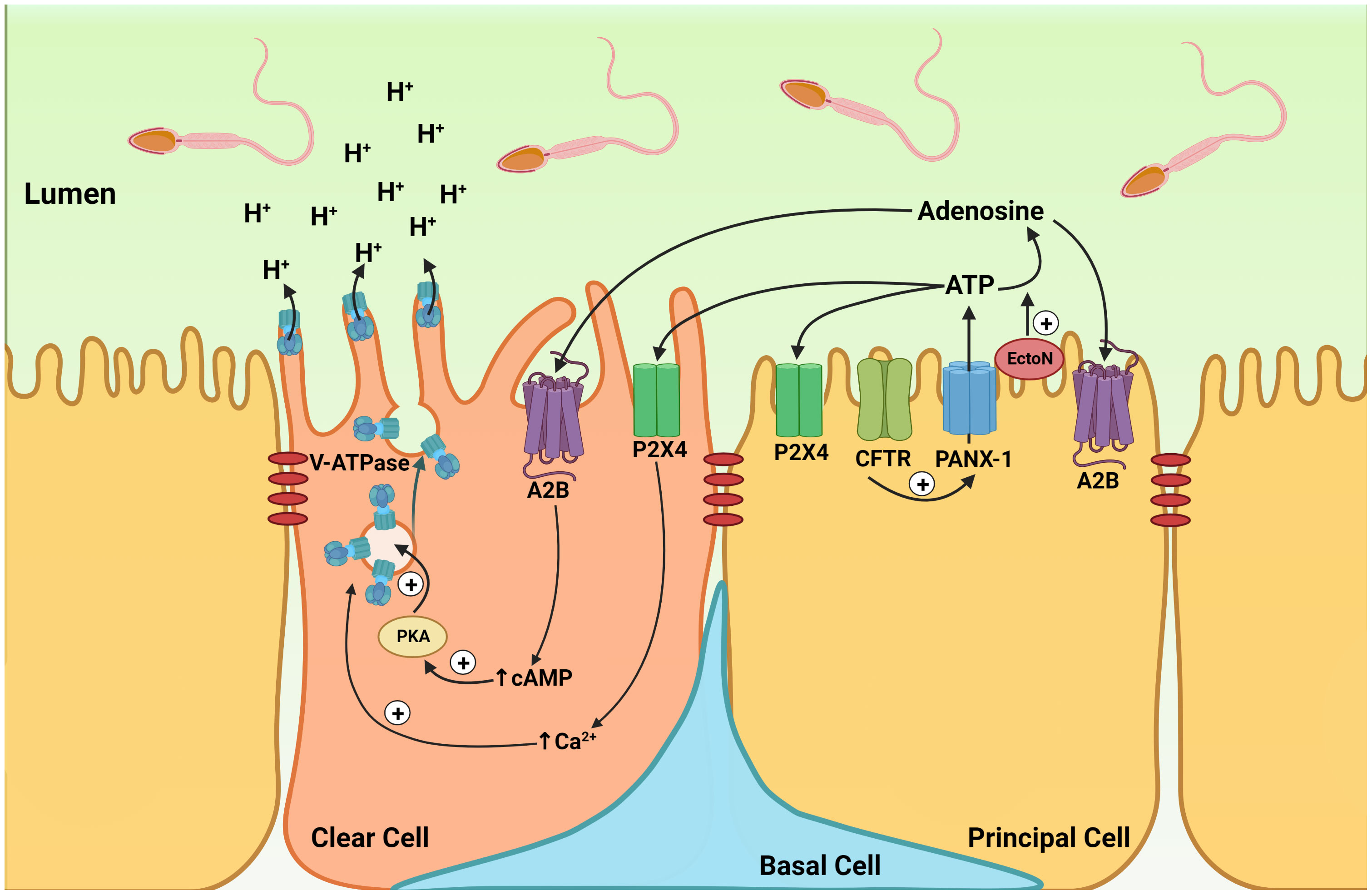
Figure 4 Cell-cell crosstalk model of purinergic regulation in the epididymis. Model showing the participation of purinergic regulation in the coordinated interaction between principal cells and clear cells, leading to the establishment of an optimal acidic luminal environment in the epididymis. Located on the apical membrane of PCs, CFTR activation stimulates ATP secretion by the pannexin-1 channel (PANX-1). Extracellular ATP is then hydrolyzed into adenosine by ectonucleotidases (EctoN) that are present on the apical surface of the epithelium. Luminal ATP activates the P2X4 receptor, and adenosine activates the A2B receptors located on the apical membrane of CC. Activation of A2B by adenosine leads to an elevation in intracellular cAMP. Activation of the cAMP/PKA pathway then induces V-ATPase apical membrane accumulation. Intracellular calcium also facilitates V-ATPase membrane accumulation in CCs, following P2X4 receptor activation by luminal ATP. The redistribution of V-ATPase from intracellular vesicles to the apical membrane results in the formation and elongation of V-ATPase-enriched microplicae in CCs, which stimulates proton secretion. The P2X4 and A2B receptors are also located on the apical membrane of PC, and their role in PC function is currently being evaluated.
Significant ATP concentration was measured in the luminal perfusate collected in the vas deferens following luminal perfusion of the cauda epididymis indicating that epididymal epithelial cells secrete ATP (176). The cell line (DC2) representing epididymal PC (177) also secretes ATP (176). In the perfused cauda epididymis, the pannexin inhibitor carbenoxolone (CBX) partially prevented luminal pH recovery from an alkaline pH and inhibited alkaline pH-induced V-ATPase apical accumulation (164). Altogether, these results showed that ATP secretion by PC, followed by its rapid hydrolysis to produce adenosine, participates in the pH-induced activation of V-ATPase-dependent proton secretion by CC (164). This indicates the presence of a PC-CC crosstalk to activate proton secretion in CC (Figure 4). Interestingly, Cystic Fibrosis Transmembrane Regulator (CFTR), the gene mutated in cystic fibrosis also participates in ATP secretion by PC (176). Cystic fibrosis is associated with male infertility, and it will be interesting to determine whether dysfunction in ATP secretion might be involved in this medical condition.
In addition to its physiological role, purinergic signaling in the epididymis might also play a pro-inflammatory role. Epididymitis is a relatively common disorder compared to orchitis in outpatient clinics (178). ATP and UDP-glucose are DAMPs that could potentially trigger sterile inflammation by activating purinergic receptors in the epididymis. Pro-inflammatory purinergic signaling in the epididymis was recently indicated by the increase in CXCL10 expression induced in epithelial cells following exposure to damaged male germ cells (179). Although not investigated, damaged germ cells might release DAMPs, such as ATP and UDP-glucose, and activate purinergic receptors located on the apical membrane of epididymal epithelial cells. Interestingly, UDP-glucose which activates P2Y14 might participate in inflammatory disorders of the epididymis. In particular, accumulation of spermatozoa in the epididymis following vasectomy may induce congestive epididymitis and subsequent tissue structural and functional alterations. P2Y14 is expressed by BC in all epididymal regions of non-vasectomized men, as well as in PC located in the corpus and cauda regions (153) (Figure 5). A subpopulation of CC located in the caput region also showed strong P2Y14 labeling. In vasectomized men, an increase in P2RY14 mRNA was observed in the corpus and cauda, and stronger apical labeling was detected in PC in the corpus region (153). Interestingly, the interstitium of the corpus and cauda epididymis of vasectomized men was characterized by the presence of numerous CD45+ leukocytes, compared to non-vasectomized men. Several CD45+ leukocytes were also observed in the corpus lumen following a vasectomy, where they appeared to have internalized spermatozoa. Thus, this study highlighted the potential role of P2Y14 in the generation of an inflamed-prone environment in the epididymis following vasectomy. In addition, UDP-glucose is involved in the homeostasis of the bacterial envelope, and bacteria themselves could modulate ATP secretion by epithelial cells (33, 72). Future studies will be required to test the role of purinergic signaling in pathogen-induced epididymitis.
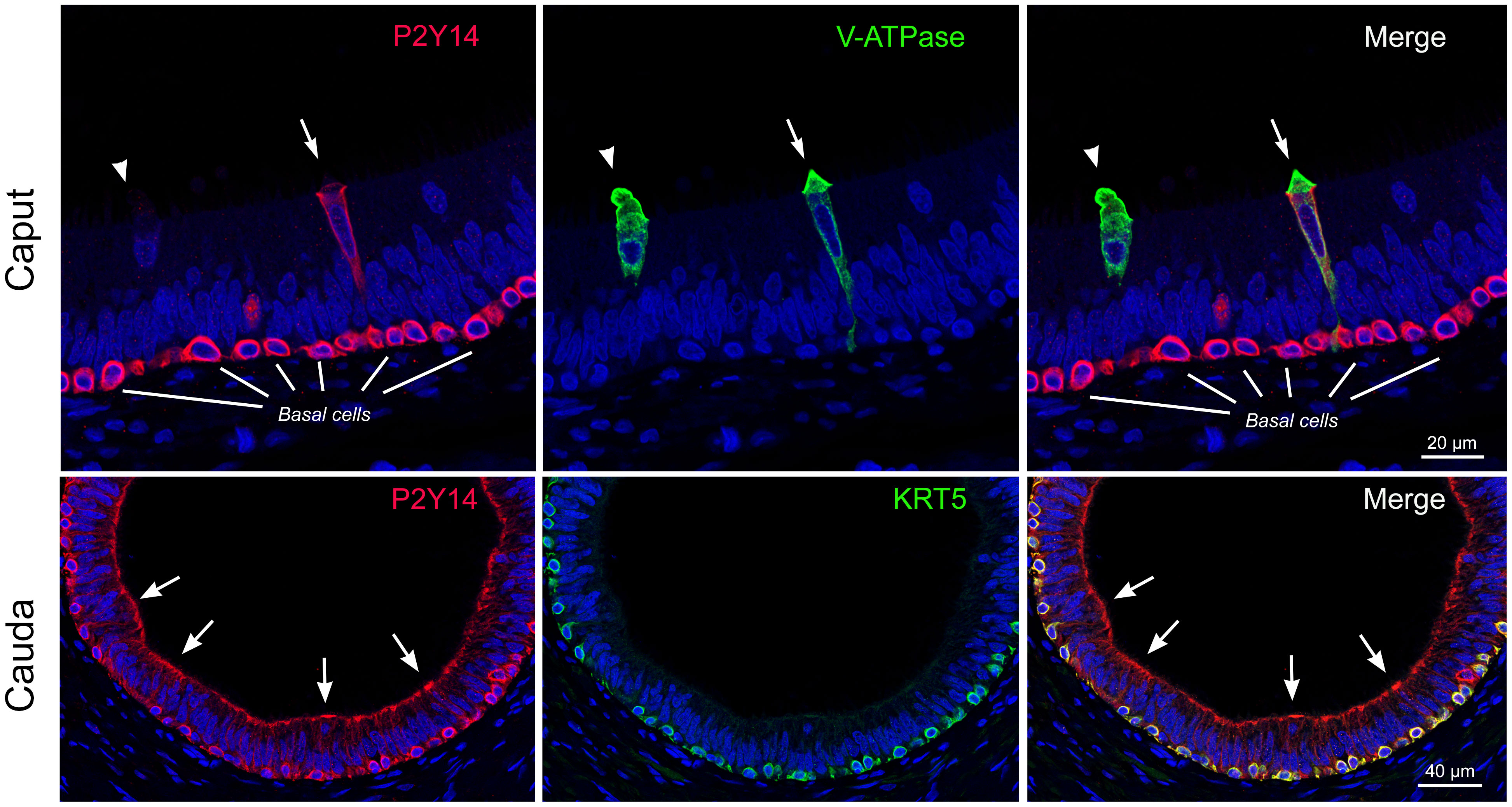
Figure 5 Localization of the pro-inflammatory P2Y14 receptor in the human epididymis. Top panels: Double immunofluorescence labeling for P2Y14 (red) and the B1 subunit of the V-ATPase (V-ATPase: green) in the human caput epididymis. Two V-ATPase-positive clear cells are shown (arrow and arrowhead). Only one clear cell is labeled for P2Y14 (arrow). Basal cells show strong labeling for P2Y14. Scale bar = 20 µm. Bottom panels: Double immunofluorescence labeling for P2Y14 (red) and cytokeratin 5 (KRT5: green) in the human cauda epididymis. Principal cells show strong apical labeling for P2Y14 (arrows). P2Y14 is also detected in KRT5-positive basal cells. Scale bar = 40 µm. Modified from Belardin et al. Andrology 2022.
In spermatozoa isolated from the mouse epididymis, P2X2 participates in an ATP-mediated intracellular Ca2+ increase (180). While spermatozoa from P2rx2 KO mice have normal functional parameters, the fertility of P2rx2-/- males rapidly declines with frequent mating. It was suggested that P2X2 confers a selection advantage by enhancing the continuous production of functional sperm under conditions of high demand. ATP is also involved in acrosomal exocytosis, a process that releases enzymes essential for sperm penetration into the egg (181).
Vas deferens
While several purinergic receptors are located in the vas deferens (Figure 3), purinergic signaling in this tissue is best known for its action on smooth muscle contraction and semen quality, which has been demonstrated in several species including humans (93, 95, 97, 182–185). Vas deferens smooth muscle contraction is initiated by motor nerve stimulation and is mediated by ATP and noradrenaline, which act as co-transmitters of the motor response in several species (185–187). In rats, guinea pigs, and rabbits, vas deferens muscle contraction is biphasic, and ATP acts through P2X receptors to stimulate the initial fast phase of contraction (186, 188–190). In later studies, ATP was shown to play opposite roles through inhibition of noradrenaline release mediated by P2Y12 or P2Y13 receptors, and the increase in noradrenaline release mediated by homomeric P2X1, P2X3 or heteromeric P2X2/P2X3 receptors (187). Confirmation of the role of the P2X1 receptor located on the smooth muscle membrane in the contractile response elicited by ATP was demonstrated in several additional studies (95, 183, 184). In support of this, null-mice for the P2X1 receptor have a 90% fertility reduction caused by reduced nerve-induced vas deferens smooth muscle contraction (95). Of note, no effect on spermatozoa was observed in these mice.
In addition to ATP, adenosine is also a key mediator of smooth muscle contraction in the vas deferens (93) and the epididymis (191). The A1 and A2 receptors were shown to enhance or inhibit contractions, respectively (187, 192, 193). A2B inhibited contractions in both the prostatic and epididymal regions of the vas deferens, while A2A was involved in the prostatic region only (193, 194). Inhibition of contractility by A2B was later shown to be mediated via the release of noradrenaline and activation of potassium channels, which involved the participation of both protein kinase A and G (195).
Regarding the purinergic modulation of transepithelial transport in the vas deferens, luminal but not basolateral adenosine stimulates anion secretion in cultured epithelial cells isolated from porcine and human vas deferens (196, 197). Adenosine also rapidly increased anion secretion in freshly excised human vas deferens (196). ATPγS, the non-hydrolysable form of ATP, was without effect, excluding the role of ATP receptors in the modulation of anion transport. Significant increase of cAMP was elicited by luminal adenosine indicating the role of A2A or A2B receptors (196). Extensive purinergic signaling that operates on the luminal side of the epithelium was proposed to modulate the luminal environment in which spermatozoa transit during ejaculation. Similar to the epididymis, adenosine may be produced in the vas deferens following ATP secretion and hydrolysis by ectonucleotidases. Of note, in the kidney ATP secretion is activated by flow (33), and it is possible that bicarbonate secretion would be increased in the vas deferens following ejaculation-induced flow. Bicarbonate induces a cAMP increase in spermatozoa through direct activation of bicarbonate-activated adenylyl cyclase (sAC; ADCY10) (198). This process is essential to initiate sperm motility and capacitation. Thus, activation of bicarbonate secretion in the vas deferens would help prime spermatozoa while they start their long journey to reach and fertilize the egg. Finally, P2Y14 is expressed in BC and in the apical membrane of PC in the human vas deferens, indicating its potential role in immune responses triggered by the DAMP molecule UDP-glucose in this organ (153).
Conclusion and perspective
Infertility is a major public health issue that affects nearly 1 in 7 couples in North America, and male infertility is the culprit in up to half of these infertility cases (199, 200). Importantly, 40-50% of male infertility is still labeled idiopathic (201), illustrating our incomplete knowledge about male reproduction health. Male infertility is caused by defective spermatogenesis and/or by sperm that have reduced fertilization functions, and the establishment of male reproductive health depends on several physiological processes that take place in the testis and the post-testicular reproductive tract. Purinergic signaling plays a variety of physiological and pathophysiological roles in the male reproductive system, but our knowledge in this context remains limited. While several purinergic receptors are expressed in the testicular and post-testicular tract, only P2X1 was shown to be indispensable for the establishment of male fertility. The vast array of purinergic receptors located all along the male reproductive tract could certainly provide several compensatory mechanisms, which would mask the crucial role of each receptor. When and how these individual receptors become dysfunctional and drive specific disease processes in the male reproductive tract is just starting to be elucidated. Future progress in our understanding of purinergic signaling in male reproduction will depend on increasing information regarding the molecular structures of P1, P2Y, and P2X receptors, the generation of novel knock-out mice, and the development of specific receptor agonists and antagonists.
Author contributions
LB: Drafting and revising the manuscript; and final approval of the manuscript to be submitted for publication. KB: Drafting and revising the manuscript; and final approval of the manuscript to be submitted for publication. CL: Revising the manuscript; and final approval of the manuscript to be submitted for publication. MB: Revising the manuscript; and final approval of the manuscript to be submitted for publication. SB: Writing and revising the manuscript; and final approval of the version to be submitted for publication. All authors contributed to the article and approved the submitted version.
Funding
This study was supported by the Canada Research Chair in Epithelial Dynamics of Kidney and Reproductive Organs (to SB), the Foundation of the Centre Hospitalier Universitaire de Québec (to SB), NIH grant HD040793 (to SB), by Canadian Institutes of Health Research (CIHR) grant 183711(to. SB), by the Fonds de Recherche du Québec en Santé (FRQS) (to LB.) and by NIH grant HD104672-01 (to MB).
Conflict of interest
S.B. is the scientific co-founder of Kantum Pharma.
The remaining authors declare that the research was conducted in the absence of any commercial or financial relationships that could be construed as a potential conflict of interest.
Publisher’s note
All claims expressed in this article are solely those of the authors and do not necessarily represent those of their affiliated organizations, or those of the publisher, the editors and the reviewers. Any product that may be evaluated in this article, or claim that may be made by its manufacturer, is not guaranteed or endorsed by the publisher.
References
1. Drury AN, Szent-Gyorgyi A. The physiological activity of adenine compounds with especial reference to their action upon the mammalian heart. J Physiol (1929) 68:213–37. doi: 10.1113/jphysiol.1929.sp002608
2. Burnstock G, Campbell G, Satchell D, Smythe A. Evidence that adenosine triphosphate or a related nucleotide is the transmitter substance released by non-adrenergic inhibitory nerves in the gut. Br J Pharmacol (1970) 40:668–88. doi: 10.1111/j.1476-5381.1970.tb10646.x
3. Burnstock G. Overview. Purinergic mechanisms. Ann N Y Acad Sci (1990) 603:1–17; discussion 18. doi: 10.1111/j.1749-6632.1990.tb37657.x
4. Leipziger J. Control of epithelial transport via luminal P2 receptors. Am J Physiol Renal Physiol (2003) 284:F419–32. doi: 10.1152/ajprenal.00075.2002
5. Yegutkin GG. Nucleotide- and nucleoside-converting ectoenzymes: Important modulators of purinergic signalling cascade. Biochim Biophys Acta (2008) 1783:673–94. doi: 10.1016/j.bbamcr.2008.01.024
6. Praetorius HA, Leipziger J. Intrarenal purinergic signaling in the control of renal tubular transport. Annu Rev Physiol (2010) 72:377–93. doi: 10.1146/annurev-physiol-021909-135825
7. Burnstock G. Purinergic signalling: from discovery to current developments. Exp Physiol (2014) 99:16–34. doi: 10.1113/expphysiol.2013.071951
8. Gallagher JA. ATP P2 receptors and regulation of bone effector cells. J Musculoskelet Neuronal Interact (2004) 4:125–7.
9. Burnstock G. A basis for distinguishing two types of purinergic receptor. In: Straub RW, Sods L, editor. Cell membrane receptors for drugs and hormones: A multidisciplinary approach, vol. pp. New York: Raven Press (1978). p. 107–18.
10. Burnstock G, Kennedy C. Is there a basis for distinguishing two types of P2-purinoceptor? Gen Pharmacol (1985) 16:433–40. doi: 10.1016/0306-3623(85)90001-1
11. Jain S, Jacobson KA. Purinergic signaling in diabetes and metabolism. Biochem Pharmacol (2021) 187:114393. doi: 10.1016/j.bcp.2020.114393
12. Rieg T, Vallon V. ATP and adenosine in the local regulation of water transport and homeostasis by the kidney. Am J Physiol Regul Integr Comp Physiol (2009) 296:R419–27. doi: 10.1152/ajpregu.90784.2008
13. Vallon V, Stockand J, Rieg T. P2Y receptors and kidney function. Wiley Interdiscip Rev Membr Transp Signal (2012) 1:731–42. doi: 10.1002/wmts.61
14. Bean BP. Pharmacology and electrophysiology of ATP-activated ion channels. Trends Pharmacol Sci (1992) 13:87–90. doi: 10.1016/0165-6147(92)90032-2
15. Bean BP, Friel DD. ATP-activated channels in excitable cells. Ion Channels (1990) 2:169–203. doi: 10.1007/978-1-4615-7305-0_5
16. Barnard EA, Burnstock G, Webb TE. G Protein-coupled receptors for ATP and other nucleotides: a new receptor family. Trends Pharmacol Sci (1994) 15:67–70. doi: 10.1016/0165-6147(94)90280-1
17. Abbracchio MP, Ceruti S. Roles of P2 receptors in glial cells: focus on astrocytes. Purinergic Signal (2006) 2:595–604. doi: 10.1007/s11302-006-9016-0
18. Khakh BS, Burnstock G, Kennedy C, King BF, North RA, Seguela P, et al. International union of pharmacology. XXIV. current status of the nomenclature and properties of P2X receptors and their subunits. Pharmacol Rev (2001) 53:107–18.
19. Jacobson KA, Delicado EG, Gachet C, Kennedy C, von Kugelgen I, Li B, et al. Update of P2Y receptor pharmacology: IUPHAR review 27. Br J Pharmacol (2020) 177:2413–33. doi: 10.1111/bph.15005
20. North RA. Molecular physiology of P2X receptors. Physiol Rev (2002) 82:1013–67. doi: 10.1152/physrev.00015.2002
21. Vultaggio-Poma V, Falzoni S, Salvi G, Giuliani AL, Di Virgilio F. Signalling by extracellular nucleotides in health and disease. Biochim Biophys Acta Mol Cell Res (2022) 1869:119237. doi: 10.1016/j.bbamcr.2022.119237
22. Thomas SA, Hume RI. Permeation of both cations and anions through a single class of ATP-activated ion channels in developing chick skeletal muscle. J Gen Physiol (1990) 95:569–90. doi: 10.1085/jgp.95.4.569
23. Erb L, Weisman GA. Coupling of P2Y receptors to G proteins and other signaling pathways. Wiley Interdiscip Rev Membr Transp Signal (2012) 1:789–803. doi: 10.1002/wmts.62
24. Burnstock G. Physiology and pathophysiology of purinergic neurotransmission. Physiol Rev (2007) 87:659–797. doi: 10.1152/physrev.00043.2006
26. Burnstock G. Purine and pyrimidine receptors. Cell Mol Life Sci (2007) 64:1471–83. doi: 10.1007/s00018-007-6497-0
27. Lopez V, Schakel L, Schuh HJM, Schmidt MS, Mirza S, Renn C, et al. Sulfated polysaccharides from macroalgae are potent dual inhibitors of human ATP-hydrolyzing ectonucleotidases NPP1 and CD39. Mar Drugs 19 (2021) 19(2):1–13. doi: 10.3390/md19020051
28. Cardoso AM, Schetinger MR, Correia-de-Sa P, Sevigny J. Impact of ectonucleotidases in autonomic nervous functions. Auton Neurosci (2015) 191:25–38. doi: 10.1016/j.autneu.2015.04.014
29. Shirley DG, Vekaria RM, Sevigny J. Ectonucleotidases in the kidney. Purinergic Signal (2009) 5:501–11. doi: 10.1007/s11302-009-9152-4
30. Dubyak GR, Moatassim Ce-. Signal transduction via P2-purinergic receptors for extracellular ATP and other nucleotides. Am J Physiol (1993) 265:C577–606. doi: 10.1152/ajpcell.1993.265.3.C577
31. Jacobson KA, Muller CE. Medicinal chemistry of adenosine, P2Y and P2X receptors. Neuropharmacology (2016) 104:31–49. doi: 10.1016/j.neuropharm.2015.12.001
32. Delacretaz E. Clinical practice. supraventricular tachycardia. New Engl J Med (2006) 354:1039–51. doi: 10.1056/NEJMcp051145
33. Vallon V, Unwin R, Inscho EW, Leipziger J, Kishore BK. Extracellular nucleotides and P2 receptors in renal function. Physiol Rev (2020) 100:211–69. doi: 10.1152/physrev.00038.2018
34. Jain S, Jacobson KA. Adipocyte purinergic receptors activated by uracil nucleotides as obesity and type 2 diabetes targets. Curr Opin Pharmacol (2022) 63:102190. doi: 10.1016/j.coph.2022.102190
35. Draganov D, Gopalakrishna-Pillai S, Chen YR, Zuckerman N, Moeller S, Wang C, et al. Modulation of P2X4/P2X7/Pannexin-1 sensitivity to extracellular ATP via ivermectin induces a non-apoptotic and inflammatory form of cancer cell death. Sci Rep (2015) 5:16222. doi: 10.1038/srep16222
36. Alarcon-Vila C, Pizzuto M, Pelegrin P. Purinergic receptors and the inflammatory response mediated by lipids. Curr Opin Pharmacol (2019) 47:90–6. doi: 10.1016/j.coph.2019.02.004
37. Hechler B, Gachet C. Purinergic receptors in thrombosis and inflammation. Arterioscler Thromb Vasc Biol (2015) 35:2307–15. doi: 10.1161/ATVBAHA.115.303395
38. Elliott MR, Chekeni FB, Trampont PC, Lazarowski ER, Kadl A, Walk SF, et al. Nucleotides released by apoptotic cells act as a find-me signal to promote phagocytic clearance. Nature (2009) 461:282–6. doi: 10.1038/nature08296
39. Chen Y, Corriden R, Inoue Y, Yip L, Hashiguchi N, Zinkernagel A, et al. ATP release guides neutrophil chemotaxis via P2Y2 and A3 receptors. Science (2006) 314:1792–5. doi: 10.1126/science.1132559
40. Allard B, Longhi MS, Robson SC, Stagg J. The ectonucleotidases CD39 and CD73: Novel checkpoint inhibitor targets. Immunol Rev (2017) 276:121–44. doi: 10.1111/imr.12528
41. Li H, Jiang W, Ye S, Zhou M, Liu C, Yang X, et al. P2Y14 receptor has a critical role in acute gouty arthritis by regulating pyroptosis of macrophages. Cell Death Dis (2020) 11:394. doi: 10.1038/s41419-020-2609-7
42. Menzies RI, Tam FW, Unwin RJ, Bailey MA. Purinergic signaling in kidney disease. Kidney Int (2017) 91:315–23. doi: 10.1016/j.kint.2016.08.029
43. Oyarzun C, Garrido W, Alarcon S, Yanez A, Sobrevia L, Quezada C, et al. Adenosine contribution to normal renal physiology and chronic kidney disease. Mol aspects Med (2017) 55:75–89. doi: 10.1016/j.mam.2017.01.004
44. Mufti F, Jung YH, Giancotti LA, Yu J, Chen Z, Phung NB, et al. P2Y14 receptor antagonists reverse chronic neuropathic pain in a mouse model. ACS Med Chem Lett (2020) 11:1281–6. doi: 10.1021/acsmedchemlett.0c00115
45. Battistone MA, Mendelsohn AC, Spallanzani RG, Allegretti AS, Liberman RN, Sesma J, et al. Proinflammatory P2Y14 receptor inhibition protects against ischemic acute kidney injury in mice. J Clin Invest (2020) 130:3734–49. doi: 10.1172/JCI134791
46. Karcz TP, Whitehead GS, Nakano K, Nakano H, Grimm SA, Williams JG, et al. UDP-Glucose and P2Y14 receptor amplify allergen-induced airway eosinophilia. J Clin Invest (2021) 131:140709. doi: 10.1172/JCI140709
47. Quintana FJ, Yeste A, Mascanfroni ID. Role and therapeutic value of dendritic cells in central nervous system autoimmunity. Cell Death Differ (2015) 22:215–24. doi: 10.1038/cdd.2014.125
48. Tozaki-Saitoh H, Tsuda M, Inoue K. Role of purinergic receptors in CNS function and neuroprotection. Adv Pharmacol (2011) 61:495–528. doi: 10.1016/B978-0-12-385526-8.00015-1
49. Perez-Sen R, Gomez-Villafuertes R, Ortega F, Gualix J, Delicado EG, Miras-Portugal MT. An update on P2Y13 receptor signalling and function. Adv Exp Med Biol (2017) 1051:139–68. doi: 10.1007/5584_2017_91
50. Jacobson KA, Giancotti LA, Lauro F, Mufti F, Salvemini D. Treatment of chronic neuropathic pain: purine receptor modulation. Pain (2020) 161:1425–41. doi: 10.1097/j.pain.0000000000001857
51. Perez-Sen R, Queipo MJ, Morente V, Ortega F, Delicado EG, Miras-Portugal MT. Neuroprotection mediated by P2Y13 nucleotide receptors in neurons. Comput Struct Biotechnol J (2015) 13:160–8. doi: 10.1016/j.csbj.2015.02.002
52. del Puerto A, Diaz-Hernandez JI, Tapia M, Gomez-Villafuertes R, Benitez MJ, Zhang J, et al. Adenylate cyclase 5 coordinates the action of ADP, P2Y1, P2Y13 and ATP-gated P2X7 receptors on axonal elongation. J Cell Sci (2012) 125:176–88. doi: 10.1242/jcs.091736
53. Del Puerto A, Wandosell F, Garrido JJ. Neuronal and glial purinergic receptors functions in neuron development and brain disease. Front Cell Neurosci (2013) 7:197. doi: 10.3389/fncel.2013.00197
54. Lou N, Takano T, Pei Y, Xavier AL, Goldman SA, Nedergaard M. Purinergic receptor P2RY12-dependent microglial closure of the injured blood-brain barrier. Proc Natl Acad Sci USA (2016) 113:1074–9. doi: 10.1073/pnas.1520398113
55. Cserep C, Posfai B, Lenart N, Fekete R, Laszlo ZI, Lele Z, et al. Microglia monitor and protect neuronal function through specialized somatic purinergic junctions. Science (2020) 367:528–37. doi: 10.1126/science.aax6752
56. Klinge E, Sjostrand NO. Comparative study of some isolated mammalian smooth muscle effectors of penile erection. Acta Physiol Scand (1977) 100:354–67. doi: 10.1111/j.1748-1716.1977.tb05961.x
57. Tong YC, Broderick G, Hypolite J, Levin RM. Correlations of purinergic, cholinergic and adrenergic functions in rabbit corporal cavernosal tissue. Pharmacology (1992) 45:241–9. doi: 10.1159/000139007
58. Levin RM, Hypolite JA, Broderick GA. Comparison of the pharmacological response of human corpus cavernosal tissue with the response of rabbit cavernosal tissue. Gen Pharmacol (1995) 26:1107–11. doi: 10.1016/0306-3623(94)00260-T
59. McIntosh VJ, Lasley RD. Adenosine receptor-mediated cardioprotection: are all 4 subtypes required or redundant? J Cardiovasc Pharmacol Ther (2012) 17:21–33. doi: 10.1177/1074248410396877
60. Mustafa SJ, Morrison RR, Teng B, Pelleg A. Adenosine receptors and the heart: role in regulation of coronary blood flow and cardiac electrophysiology. Handb Exp Pharmacol (2009) 193:161–88. doi: 10.1007/978-3-540-89615-9_6
61. Lohman AW, Billaud M, Isakson BE. Mechanisms of ATP release and signalling in the blood vessel wall. Cardiovasc Res (2012) 95:269–80. doi: 10.1093/cvr/cvs187
62. Dahl G, Muller KJ. Innexin and pannexin channels and their signaling. FEBS Lett (2014) 588:1396–402. doi: 10.1016/j.febslet.2014.03.007
63. Keyhan H, Dadvar A, Ansari M, Rafiee K. Comparison of before and after varicocelectomy levels of nitric oxide in seminal fluid of infertile men. Nephrourol Mon (2012) 4:629–32. doi: 10.5812/numonthly.4696
64. Arulkumaran N, Turner CM, Sixma ML, Singer M, Unwin R, Tam FW. Purinergic signaling in inflammatory renal disease. Front Physiol (2013) 4:194. doi: 10.3389/fphys.2013.00194
65. Ren Y, Garvin JL, Liu R, Carretero OA. Role of macula densa adenosine triphosphate (ATP) in tubuloglomerular feedback. Kidney Int (2004) 66:1479–85. doi: 10.1111/j.1523-1755.2004.00911.x
66. Leipziger J, Praetorius H. Renal autocrine and paracrine signaling: A story of self-protection. Physiol Rev (2020) 100:1229–89. doi: 10.1152/physrev.00014.2019
67. Bauerle JD, Grenz A, Kim JH, Lee HT, Eltzschig HK. Adenosine generation and signaling during acute kidney injury. J Am Soc Nephrol (2011) 22:14–20. doi: 10.1681/ASN.2009121217
68. Poth JM, Brodsky K, Ehrentraut H, Grenz A, Eltzschig HK. Transcriptional control of adenosine signaling by hypoxia-inducible transcription factors during ischemic or inflammatory disease. J Mol Med (Berl) (2013) 91:183–93. doi: 10.1007/s00109-012-0988-7
69. Awad AS, Rouse M, Liu L, Vergis AL, Rosin DL, Linden J, et al. Activation of adenosine 2A receptors preserves structure and function of podocytes. J Am Soc Nephrol (2008) 19:59–68. doi: 10.1681/ASN.2007030276
70. Battistone MA, Nair AV, Barton CR, Liberman RN, Peralta MA, Capen DE, et al. Extracellular adenosine stimulates vacuolar ATPase-dependent proton secretion in medullary intercalated cells. J Am Soc Nephrol (2018) 29:545–56. doi: 10.1681/ASN.2017060643
71. Goncalves RG, Gabrich L, Rosario A Jr., Takiya CM, Ferreira ML, Chiarini LB, et al. The role of purinergic P2X7 receptors in the inflammation and fibrosis of unilateral ureteral obstruction in mice. Kidney Int (2006) 70:1599–606. doi: 10.1038/sj.ki.5001804
72. Praetorius H. The bacteria and the host: a story of purinergic signaling in urinary tract infections. Am J Physiol Cell Physiol 321 (2021) 321(1):C134–46. doi: 10.1152/ajpcell.00054.2021
73. Coutinho-Silva R, Persechini PM, Bisaggio RD, Perfettini JL, Neto AC, Kanellopoulos JM, et al. P2Z/P2X7 receptor-dependent apoptosis of dendritic cells. Am J Physiol 276 (1999) 276(5):C1139–47. doi: 10.1152/ajpcell.1999.276.5.C1139
74. Vazquez-Cuevas FG, Martinez-Ramirez AS, Robles-Martinez L, Garay E, Garcia-Carranca A, Perez-Montiel D, et al. Paracrine stimulation of P2X7 receptor by ATP activates a proliferative pathway in ovarian carcinoma cells. J Cell Biochem (2014) 115:1955–66. doi: 10.1002/jcb.24867
75. Amoroso F, Salaro E, Falzoni S, Chiozzi P, Giuliani AL, Cavallesco G, et al. P2X7 targeting inhibits growth of human mesothelioma. Oncotarget (2016) 7:49664–76. doi: 10.18632/oncotarget.10430
76. Giannuzzo A, Pedersen SF, Novak I. The P2X7 receptor regulates cell survival, migration and invasion of pancreatic ductal adenocarcinoma cells. Mol Cancer (2015) 14:203. doi: 10.1186/s12943-015-0472-4
77. Zhang Y, Cheng H, Li W, Wu H, Yang Y. Highly-expressed P2X7 receptor promotes growth and metastasis of human HOS/MNNG osteosarcoma cells via PI3K/Akt/GSK3beta/beta-catenin and mTOR/HIF1alpha/VEGF signaling. Int J Cancer (2019) 145:1068–82. doi: 10.1002/ijc.32207
78. Li X, Qi X, Zhou L, Catera D, Rote NS, Potashkin J, et al. Decreased expression of P2X7 in endometrial epithelial pre-cancerous and cancer cells. Gynecol Oncol (2007) 106:233–43. doi: 10.1016/j.ygyno.2007.03.032
79. Fu W, McCormick T, Qi X, Luo L, Zhou L, Li X, et al. Activation of P2X(7)-mediated apoptosis inhibits DMBA/TPA-induced formation of skin papillomas and cancer in mice. BMC Cancer (2009) 9:114. doi: 10.1186/1471-2407-9-114
80. Greig AV, Linge C, Healy V, Lim P, Clayton E, Rustin MH, et al. Expression of purinergic receptors in non-melanoma skin cancers and their functional roles in A431 cells. J Invest Dermatol (2003) 121:315–27. doi: 10.1046/j.1523-1747.2003.12379.x
81. Cai X, Yao Y, Teng F, Li Y, Wu L, Yan W, et al. The role of P2X7 receptor in infection and metabolism: Based on inflammation and immunity. Int Immunopharmacol (2021) 101:108297. doi: 10.1016/j.intimp.2021.108297
82. Hopfner M, Maaser K, Barthel B, von Lampe B, Hanski C, Riecken EO, et al. Growth inhibition and apoptosis induced by P2Y2 receptors in human colorectal carcinoma cells: involvement of intracellular calcium and cyclic adenosine monophosphate. Int J Colorectal Dis (2001) 16:154–66. doi: 10.1007/s003840100302
83. Maaser K, Hopfner M, Kap H, Sutter AP, Barthel B, von Lampe B, et al. Extracellular nucleotides inhibit growth of human oesophageal cancer cells via P2Y(2)-receptors. Br J Cancer (2002) 86:636–44. doi: 10.1038/sj.bjc.6600100
84. Yang G, Zhang S, Zhang Y, Zhou Q, Peng S, Zhang T, et al. The inhibitory effects of extracellular ATP on the growth of nasopharyngeal carcinoma cells via P2Y2 receptor and osteopontin. J Exp Clin Cancer Res (2014) 33:53. doi: 10.1186/1756-9966-33-53
85. Katzur AC, Koshimizu T, Tomic M, Schultze-Mosgau A, Ortmann O, Stojilkovic SS. Expression and responsiveness of P2Y2 receptors in human endometrial cancer cell lines. J Clin Endocrinol Metab (1999) 84:4085–91. doi: 10.1210/jcem.84.11.6119
86. de Leve S, Wirsdorfer F, Jendrossek V. Targeting the immunomodulatory CD73/Adenosine system to improve the therapeutic gain of radiotherapy. Front Immunol (2019) 10:698. doi: 10.3389/fimmu.2019.00698
87. de Andrade Mello P, Coutinho-Silva R, Savio LEB. Multifaceted effects of extracellular adenosine triphosphate and adenosine in the tumor-host interaction and therapeutic perspectives. Front Immunol (2017) 8:1526. doi: 10.3389/fimmu.2017.01526
88. Lazarowski ER, Boucher RC. Purinergic receptors in airway epithelia. Curr Opin Pharmacol (2009) 9:262–7. doi: 10.1016/j.coph.2009.02.004
89. Housley GD. Recent insights into the regulation of breathing. Auton Neurosci (2011) 164:3–5. doi: 10.1016/j.autneu.2011.08.002
90. von Kugelgen I. Structure, pharmacology and roles in physiology of the P2Y12 receptor. Adv Exp Med Biol (2017) 1051:123–38. doi: 10.1007/5584_2017_98
91. Knauer KA, Fish JE, Adkinson NF Jr., Lichtenstein LM, Peters SP, Newball HH. Platelet activation in antigen-induced bronchoconstriction. N Engl J Med (1981) 305:892–3. doi: 10.1056/NEJM198110083051513
92. Pitchford SC, Momi S, Baglioni S, Casali L, Giannini S, Rossi R, et al. Allergen induces the migration of platelets to lung tissue in allergic asthma. Am J Respir Crit Care Med (2008) 177:604–12. doi: 10.1164/rccm.200702-214OC
93. Sakai KK, Hymson DL, Shapiro R. The effects of adenosine and adenine nucleotides on the guinea-pig vas deferens: evidence for existence of purinergic receptors. Life Sci (1979) 24:1299–308. doi: 10.1016/0024-3205(79)90149-8
94. Westfall DP, Stitzel RE, Rowe JN. The postjunctional effects and neural release of purine compounds in the guinea-pig vas deferens. Eur J Pharmacol (1978) 50:27–38. doi: 10.1016/0014-2999(78)90250-9
95. Mulryan K, Gitterman DP, Lewis CJ, Vial C, Leckie BJ, Cobb AL, et al. Reduced vas deferens contraction and male infertility in mice lacking P2X1 receptors. Nature (2000) 403:86–9. doi: 10.1038/47495
97. Burnstock G. Purinergic signalling in the reproductive system in health and disease. Purinergic Signal (2014) 10:157–87. doi: 10.1007/s11302-013-9399-7
98. Gorodeski GI. Purinergic signalling in the reproductive system. Auton Neurosci (2015) 191:82–101. doi: 10.1016/j.autneu.2015.04.008
99. Mundt N, Kenzler L, Spehr M. Purinergic signaling in spermatogenesis. Front Endocrinol (Lausanne) (2022) 13:867011. doi: 10.3389/fendo.2022.867011
100. Paniagua R, Nistal M. Morphological and histometric study of human spermatogonia from birth to the onset of puberty. J Anat (1984) 139(Pt 3):535–52.
101. de Kretser DM, Loveland KL, Meinhardt A, Simorangkir D, Wreford N. Spermatogenesis. Hum Reprod (1998) 13 Suppl 1:1–8. doi: 10.1093/humrep/13.suppl_1.1
102. Neto FT, Bach PV, Najari BB, Li PS, Goldstein M. Spermatogenesis in humans and its affecting factors. Semin Cell Dev Biol (2016) 59:10–26. doi: 10.1016/j.semcdb.2016.04.009
103. Wang RS, Yeh S, Tzeng CR, Chang C. Androgen receptor roles in spermatogenesis and fertility: lessons from testicular cell-specific androgen receptor knockout mice. Endocr Rev (2009) 30:119–32. doi: 10.1210/er.2008-0025
104. Fleck D, Mundt N, Bruentgens F, Geilenkirchen P, Machado PA, Veitinger T, et al. Distinct purinergic signaling pathways in prepubescent mouse spermatogonia. J Gen Physiol (2016) 148:253–71. doi: 10.1085/jgp.201611636
105. Foresta C, Rossato M, Bordon P, Di Virgilio F. Extracellular ATP activates different signalling pathways in rat sertoli cells. Biochem J (1995) 311(Pt 1):269–74. doi: 10.1042/bj3110269
106. Gelain DP, de Souza LF, Bernard EA. Extracellular purines from cells of seminiferous tubules. Mol Cell Biochem (2003) 245:1–9. doi: 10.1023/A:1022857608849
107. Poletto Chaves LA, Pontelli EP, Varanda WA. P2X receptors in mouse leydig cells. Am J Physiol Cell Physiol 290 (2006) 290(4):C1009–17. doi: 10.1152/ajpcell.00506.2005
108. Veitinger S, Veitinger T, Cainarca S, Fluegge D, Engelhardt CH, Lohmer S, et al. Purinergic signalling mobilizes mitochondrial Ca(2)(+) in mouse sertoli cells. J Physiol (2011) 589:5033–55. doi: 10.1113/jphysiol.2011.216309
109. Walenta L, Fleck D, Frohlich T, von Eysmondt H, Arnold GJ, Spehr J, et al. ATP-mediated events in peritubular cells contribute to sterile testicular inflammation. Sci Rep (2018) 8:1431. doi: 10.1038/s41598-018-19624-3
110. Rudge SA, Hughes PJ, Brown GR, Michell RH, Kirk CJ. Inositol lipid-mediated signalling in response to endothelin and ATP in the mammalian testis. Mol Cell Biochem (1995) 149-150:161–74. doi: 10.1007/BF01076574
111. Bjorkgren I, Lishko PV. Purinergic signaling in testes revealed. J Gen Physiol (2016) 148:207–11. doi: 10.1085/jgp.201611676
112. Antonio LS, Costa RR, Gomes MD, Varanda WA. Mouse leydig cells express multiple P2X receptor subunits. Purinergic Signal (2009) 5:277–87. doi: 10.1007/s11302-008-9128-9
113. Foresta C, Rossato M, Nogara A, Gottardello F, Bordon P, Di Virgilio F. Role of P2-purinergic receptors in rat leydig cell steroidogenesis. Biochem J (1996) 320(Pt 2):499–504. doi: 10.1042/bj3200499
114. Ko WH, Au CL, Yip CY. Multiple purinergic receptors lead to intracellular calcium increases in cultured rat sertoli cells. Life Sci (2003) 72:1519–35. doi: 10.1016/S0024-3205(02)02410-4
115. Glass R, Bardini M, Robson T, Burnstock G. Expression of nucleotide P2X receptor subtypes during spermatogenesis in the adult rat testis. Cells Tissues Organs (2001) 169:377–87. doi: 10.1159/000047905
116. Tanaka J, Murate M, Wang CZ, Seino S, Iwanaga T. Cellular distribution of the P2X4 ATP receptor mRNA in the brain and non-neuronal organs of rats. Arch Histol Cytol (1996) 59:485–90. doi: 10.1679/aohc.59.485
117. Stiles GL, Pierson G, Sunay S, Parsons WJ. The rat testicular A1 adenosine receptor-adenylate cyclase system. Endocrinology (1986) 119:1845–51. doi: 10.1210/endo-119-4-1845
118. Murphy KM, Snyder SH. Adenosine receptors in rat testes: labeling with 3H-cyclohexyladenosine. Life Sci (1981) 28:917–20. doi: 10.1016/0024-3205(81)90054-0
119. Monaco L, Conti M. Localization of adenosine receptors in rat testicular cells. Biol Reprod (1986) 35:258–66. doi: 10.1095/biolreprod35.2.258
120. Missel A, Walenta L, Eubler K, Mundt N, Heikela H, Pickl U, et al. Testicular adenosine acts as a pro-inflammatory molecule: role of testicular peritubular cells. Mol Hum Reprod (2021) 27:1–13. doi: 10.1093/molehr/gaab037
121. Filippini A, Riccioli A, De Cesaris P, Paniccia R, Teti A, Stefanini M, et al. Activation of inositol phospholipid turnover and calcium signaling in rat sertoli cells by P2-purinergic receptors: modulation of follicle-stimulating hormone responses. Endocrinology (1994) 134:1537–45. doi: 10.1210/endo.134.3.8119196
122. Gelain DP, Casali EA, de Oliveira RB, de Souza LF, Barreto F, Dal-Pizzol F, et al. Effects of follicle-stimulating hormone and vitamin a upon purinergic secretion by rat sertoli cells. Mol Cell Biochem (2005) 278:185–94. doi: 10.1007/s11010-005-7500-4
123. Lalevee N, Rogier C, Becq F, Joffre M. Acute effects of adenosine triphosphates, cyclic 3',5'-adenosine monophosphates, and follicle-stimulating hormone on cytosolic calcium level in cultured immature rat sertoli cells. Biol Reprod (1999) 61:343–52. doi: 10.1095/biolreprod61.2.343
124. Meroni SB, Canepa DF, Pellizzari EH, Schteingart HF, Cigorraga SB. Effects of purinergic agonists on aromatase and gamma-glutamyl transpeptidase activities and on transferrin secretion in cultured sertoli cells. J Endocrinol (1998) 157:275–83. doi: 10.1677/joe.0.1570275
125. Rossato M, Merico M, Bettella A, Bordon P, Foresta C. Extracellular ATP stimulates estradiol secretion in rat sertoli cells in vitro: modulation by external sodium. Mol Cell Endocrinol (2001) 178:181–7. doi: 10.1016/S0303-7207(01)00426-9
126. Lalevee N, Rogier C, Becq F, Joffre M. Acute effects of adenosine triphosphates, cyclic 3',5'-adenosine monophosphates, and follicle-stimulating hormone on cytosolic calcium level in cultured immature rat ssertoli cells. Biol Reprod (1999) 61:343–52. doi: 10.1095/biolreprod61.2.343
127. Fleck D, Kenzler L, Mundt N, Strauch M, Uesaka N, Moosmann R, et al. ATP activation of peritubular cells drives testicular sperm transport. Elife (2021) 10:1–30. doi: 10.7554/eLife.62885
128. Belleannée C, Calvo E, Thimon V, Cyr DG, Légaré C, Garneau L, et al. Role of microRNAs in controlling gene expression in different segments of the human epididymis. PloS One (2012) 7:e34996. doi: 10.1371/journal.pone.0034996
129. Sullivan R, Mieusset R. The human epididymis: its function in sperm maturation. Hum Reprod Update (2016) 22:574–87. doi: 10.1093/humupd/dmw015
130. Machado-Neves M. Effect of heavy metals on epididymal morphology and function: An integrative review. Chemosphere (2022) 291:133020. doi: 10.1016/j.chemosphere.2021.133020
131. Breton S, Nair AV, Battistone MA. Epithelial dynamics in the epididymis: role in the maturation, protection, and storage of spermatozoa. Andrology (2019) 7:631–43. doi: 10.1111/andr.12632
132. Robaire B HB. The epididymis. In: Knobil and neill’s physiology of reproduction. Cambridge, Massachusetts: Elsevier (2015). p. 691–771.
133. Sullivan R, Belleannée C. Role of the epididymis in sperm maturation. In: C.D.J.C. B, editor. The sperm cell: Production, maturation, fertilization, regeneration. Cambridge: Cambridge University Press (2017). p. 73–87.
134. Da Silva N, Barton CR. Macrophages and dendritic cells in the post-testicular environment. Cell Tissue Res (2016) 363:97–104. doi: 10.1007/s00441-015-2270-0
135. Fijak M, Pilatz A, Hedger MP, Nicolas N, Bhushan S, Michel V, et al. Infectious, inflammatory and 'autoimmune' male factor infertility: how do rodent models inform clinical practice? Hum Reprod Update (2018) 24:416–41. doi: 10.1093/humupd/dmy009
136. Voisin A, Whitfield M, Damon-Soubeyrand C, Goubely C, Henry-Berger J, Saez F, et al. Comprehensive overview of murine epididymal mononuclear phagocytes and lymphocytes: Unexpected populations arise. J Reprod Immunol (2018) 126:11–7. doi: 10.1016/j.jri.2018.01.003
137. Hasan H, Bhushan S, Fijak M, Meinhardt A. Mechanism of inflammatory associated impairment of sperm function, spermatogenesis and steroidogenesis. Front Endocrinol (Lausanne) (2022) 13:897029. doi: 10.3389/fendo.2022.897029
138. Pleuger C, Silva EJR, Pilatz A, Bhushan S, Meinhardt A. Differential immune response to infection and acute inflammation along the epididymis. Front Immunol 0 (2020) 11. doi: 10.3389/fimmu.2020.599594
139. Zhou W, De Iuliis GN, Dun MD, Nixon B. Characteristics of the epididymal luminal environment responsible for sperm maturation and storage. Front Endocrinol (Lausanne) (2018) 9:59. doi: 10.3389/fendo.2018.00059
140. Levine N, Kelly H. Measurement of pH in the rat epididymis in vivo. J Reprod Fertil (1978) 52:333–5. doi: 10.1530/jrf.0.0520333
141. Levine N, Marsh DJ. Micropuncture studies of the electrochemical aspects of fluid and electrolyte transport in individual seminiferous tubules, the epididymis and the vas deferens in rats. J Physiol (1971) 213:557–70. doi: 10.1113/jphysiol.1971.sp009400
142. Gregory M, Cyr DG. The blood-epididymis barrier and inflammation. Spermatogenesis (2014) 4:e979619. doi: 10.4161/21565562.2014.979619
143. Mital P, Hinton BT, Dufour JM. The blood-testis and blood-epididymis barriers are more than just their tight junctions. Biol Reprod (2011) 84:851–8. doi: 10.1095/biolreprod.110.087452
144. Frenette G, Girouard J, D'Amours O, Allard N, Tessier L, Sullivan R. Characterization of two distinct populations of epididymosomes collected in the intraluminal compartment of the bovine cauda epididymis. Biol Reprod (2010) 83:473–80. doi: 10.1095/biolreprod.109.082438
145. Labas V, Spina L, Belleannee C, Teixeira-Gomes AP, Gargaros A, Dacheux F, et al. Analysis of epididymal sperm maturation by MALDI profiling and top-down mass spectrometry. J Proteomics (2015) 113:226–43. doi: 10.1016/j.jprot.2014.09.031
146. Sullivan R. Epididymosomes: a heterogeneous population of microvesicles with multiple functions in sperm maturation and storage. Asian J Androl (2015) 17:726–9. doi: 10.4103/1008-682X.155255
147. Belleannee C, Calvo E, Caballero J, Sullivan R. Epididymosomes convey different repertoires of microRNAs throughout the bovine epididymis. Biol Reprod (2013) 89:30. doi: 10.1095/biolreprod.113.110486
148. Sullivan R, Saez F. Epididymosomes, prostasomes, and liposomes: their roles in mammalian male reproductive physiology. Reproduction (2013) 146:R21–35. doi: 10.1530/REP-13-0058
149. Barrachina F, Battistone MA, Castillo J, Mallofre C, Jodar M, Breton S, et al. Sperm acquire epididymis-derived proteins through epididymosomes. Hum Reprod (2022) 37:651–68. doi: 10.1093/humrep/deac015
150. Avellar MC HB. Epididymis. In: Ilpo Huhtaniemi LM, editor. Encyclopedia of endocrine diseases, vol. pp . Cambridge, Massachusetts: Academic Press (2019). p. 807–13.
151. Robaire BH, Hinton. BT, Orgebin-Crist M. The epididymis. In: Neill JD, editor. Knobil and neill’s physiology of reproduction. Cambridge, New York: Academic Press (2006). p. 1071–147.
152. Sullivan R, Légaré C, Lamontagne-Proulx J, Breton S, Soulet D. Revisiting structure/functions of the human epididymis. Andrology (2019) 7:748–57. doi: 10.1111/andr.12633
153. Belardin LB, Legare C, Sullivan R, Belleannee C, Breton S. Expression of the pro-inflammatory P2Y14 receptor in the non-vasectomized and vasectomized human epididymis. Andrology (2022) 10(8):1522–39. doi: 10.1111/andr.13284
154. Wong PY. Control of anion and fluid secretion by apical P2-purinoceptors in the rat epididymis. Br J Pharmacol (1988) 95:1315–21. doi: 10.1111/j.1476-5381.1988.tb11770.x
155. Haynes JM, Alexander SP, Hill SJ. A1 and A2 adenosine receptor modulation of contractility in the cauda epididymis of the guinea-pig. Br J Pharmacol (1998) 125:570–6. doi: 10.1038/sj.bjp.0702095
156. Shariatmadari R, Sipila P, Vierula M, Tornquist K, Huhtaniemi I, Poutanen M. Adenosine triphosphate induces Ca2+ signal in epithelial cells of the mouse caput epididymis through activation of P2X and P2Y purinergic receptors. Biol Reprod (2003) 68:1185–92. doi: 10.1095/biolreprod.102.007419
157. Banks FC, Calvert RC, Burnstock G. Changing P2X receptor localization on maturing sperm in the epididymides of mice, hamsters, rats, and humans: a preliminary study. Fertil Steril (2010) 93:1415–20. doi: 10.1016/j.fertnstert.2009.02.061
158. Belleannée C, Da Silva N, Shum WWC, Brown D, Breton S. Role of purinergic signaling pathways in V-ATPase recruitment to apical membrane of acidifying epididymal clear cells. Am J Physiol Cell Physiol (2010) 298:C817–830. doi: 10.1152/ajpcell.00460.2009
159. Battistone MA, Spallanzani RG, Mendelsohn AC, Capen D, Nair AV, Brown D, et al. Novel role of proton-secreting epithelial cells in sperm maturation and mucosal immunity. J Cell Sci (2019) 133:jcs233239. doi: 10.1242/jcs.233239
160. Kauffenstein G, Pelletier J, Lavoie EG, Kukulski F, Martin-Satue M, Dufresne SS, et al. Nucleoside triphosphate diphosphohydrolase-1 ectonucleotidase is required for normal vas deferens contraction and male fertility through maintaining P2X1 receptor function. J Biol Chem (2014) 289:28629–39. doi: 10.1074/jbc.M114.604082
161. Martin-Satue M, Lavoie EG, Pelletier J, Fausther M, Csizmadia E, Guckelberger O, et al. Localization of plasma membrane bound NTPDases in the murine reproductive tract. Histochem Cell Biol (2009) 131:615–28. doi: 10.1007/s00418-008-0551-3
162. Martin-Satue M, Lavoie EG, Fausther M, Lecka J, Aliagas E, Kukulski F, et al. High expression and activity of ecto-5'-nucleotidase/CD73 in the male murine reproductive tract. Histochem Cell Biol (2010) 133:659–68. doi: 10.1007/s00418-010-0704-z
163. Robson SC, Sevigny J, Zimmermann H. The e-NTPDase family of ectonucleotidases: Structure function relationships and pathophysiological significance. Purinergic Signal (2006) 2:409–30. doi: 10.1007/s11302-006-9003-5
164. Battistone MA, Merkulova M, Park YJ, Peralta MA, Gombar F, Brown D, et al. Unravelling purinergic regulation in the epididymis: activation of V-ATPase-dependent acidification by luminal ATP and adenosine. J Physiol (2019) 597:1957–73. doi: 10.1113/JP277565
165. Acott TS, Carr DW. Inhibition of bovine spermatozoa by caudal epididymal fluid: II. interaction of pH and a quiescence factor. Biol Reprod (1984) 30:926–35. doi: 10.1095/biolreprod30.4.926
166. Carr DW, Usselman MC, Acott TS. Effects of pH, lactate, and viscoelastic drag on sperm motility: a species comparison. Biol Reprod (1985) 33:588–95. doi: 10.1095/biolreprod33.3.588
167. Kirichok Y, Navarro B, Clapham DE. Whole-cell patch-clamp measurements of spermatozoa reveal an alkaline-activated Ca2+ channel. Nature (2006) 439:737–40. doi: 10.1038/nature04417
168. Orgebin-Crist MC. Studies on the function of the epididymis. Biol Reprod 1 (1969) Suppl 1:155–75. doi: 10.1095/biolreprod1.Supplement_1.155
169. Beaulieu V, Da Silva N, Pastor-Soler N, Brown CR, Smith PJ, Brown D, et al. Modulation of the actin cytoskeleton via gelsolin regulates vacuolar h+-ATPase recycling. J Biol Chem (2005) 280:8452–63. doi: 10.1074/jbc.M412750200
170. Pastor-Soler N, Beaulieu V, Litvin TN, Da Silva N, Chen Y, Brown D, et al. Bicarbonate-regulated adenylyl cyclase (sAC) is a sensor that regulates pH-dependent V-ATPase recycling. J Biol Chem (2003) 278:49523–9. doi: 10.1074/jbc.M309543200
171. Pastor-Soler NM, Hallows KR, Smolak C, Gong F, Brown D, Breton S. Alkaline pH- and cAMP-induced V-ATPase membrane accumulation is mediated by protein kinase a in epididymal clear cells. Am J Physiol Cell Physiol (2008) 294:C488–94. doi: 10.1152/ajpcell.00537.2007
172. Park Y-J, Battistone MA, Kim B, Breton S. Relative contribution of clear cells and principal cells to luminal pH in the mouse epididymis. Biol Reprod (2017) 96:366–75. doi: 10.1095/biolreprod.116.144857
173. Clarke CE, Benham CD, Bridges A, George AR, Meadows HJ. Mutation of histidine 286 of the human P2X4 purinoceptor removes extracellular pH sensitivity. J Physiol (2000) 523 Pt 3:697–703. doi: 10.1111/j.1469-7793.2000.00697.x
174. Holzer P. Acid-sensitive ion channels in gastrointestinal function. Curr Opin Pharmacol (2003) 3:618–25. doi: 10.1016/j.coph.2003.06.008
175. Huang P, Zou Y, Zhong XZ, Cao Q, Zhao K, Zhu MX, et al. P2X4 forms functional ATP-activated cation channels on lysosomal membranes regulated by luminal pH. J Biol Chem (2014) 289:17658–67. doi: 10.1074/jbc.M114.552158
176. Ruan YC, Shum WWC, Belleannée C, Da Silva N, Breton S. ATP secretion in the male reproductive tract: essential role of CFTR. J Physiol (Lond.) (2012) 590:4209–22. doi: 10.1113/jphysiol.2012.230581
177. Araki Y, Suzuki K, Matusik RJ, Obinata M, Orgebin-Crist MC. Immortalized epididymal cell lines from transgenic mice overexpressing temperature-sensitive simian virus 40 large T-antigen gene. J Androl (2002) 23:854–69.
178. Trojian TH, Lishnak TS, Heiman D. Epididymitis and orchitis: an overview. Am Fam Physician (2009) 79:583–7.
179. Liu WH, Wang F, Yu XQ, Wu H, Gong ML, Chen R, et al. Damaged male germ cells induce epididymitis in mice. Asian J Androl (2020) 22:472–80. doi: 10.4103/aja.aja_116_19
180. Navarro B, Miki K, Clapham DE. ATP-activated P2X2 current in mouse spermatozoa. Proc Natl Acad Sci USA (2011) 108:14342–7. doi: 10.1073/pnas.1111695108
181. Luria A, Rubinstein S, Lax Y, Breitbart H. Extracellular adenosine triphosphate stimulates acrosomal exocytosis in bovine spermatozoa via P2 purinoceptor. Biol Reprod (2002) 66:429–37. doi: 10.1095/biolreprod66.2.429
182. Head RJ, O'Donnell JP, Hogaboom GK, Fedan JS. Cell surface localization of P2-purinergic receptors in vas deferens. Biochem Pharmacol (1983) 32:563–5. doi: 10.1016/0006-2952(83)90539-7
183. Lee HY, Bardini M, Burnstock G. P2X receptor immunoreactivity in the male genital organs of the rat. Cell Tissue Res (2000) 300:321–30. doi: 10.1007/s004410000207
184. Vulchanova L, Arvidsson U, Riedl M, Wang J, Buell G, Surprenant A, et al. Differential distribution of two ATP-gated channels (P2X receptors) determined by immunocytochemistry. Proc Natl Acad Sci U.S.A. (1996) 93:8063–7. doi: 10.1073/pnas.93.15.8063
185. Banks FC, Knight GE, Calvert RC, Thompson CS, Morgan RJ, Burnstock G. The purinergic component of human vas deferens contraction. Fertil Steril (2006) 85:932–9. doi: 10.1016/j.fertnstert.2005.09.024
186. Sneddon P, Westfall DP, Fedan JS. Cotransmitters in the motor nerves of the guinea pig vas deferens: electrophysiological evidence. Science (1982) 218:693–5. doi: 10.1126/science.6291151
187. Queiroz G, Talaia C, Goncalves J. ATP modulates noradrenaline release by activation of inhibitory P2Y receptors and facilitatory P2X receptors in the rat vas deferens. J Pharmacol Exp Ther (2003) 307:809–15. doi: 10.1124/jpet.103.054809
188. Sneddon P, Westfall DP, Colby J, Fedan JS. A pharmacological investigation of the biphasic nature of the contractile response of rabbit and rat vas deferens to field stimulation. Life Sci (1984) 35:1903–12. doi: 10.1016/0024-3205(84)90470-3
189. Meldrum LA, Burnstock G. Evidence that ATP acts as a co-transmitter with noradrenaline in sympathetic nerves supplying the guinea-pig vas deferens. Eur J Pharmacol (1983) 92:161–3. doi: 10.1016/0014-2999(83)90126-7
190. Fedan JS, Hogaboom GK, O'Donnell JP, Colby J, Westfall DP. Contribution by purines to the neurogenic response of the vas deferens of the guinea pig. Eur J Pharmacol (1981) 69:41–53. doi: 10.1016/0014-2999(81)90600-2
191. Haynes JM. A(2A) adenosine receptor mediated potassium channel activation in rat epididymal smooth muscle. Br J Pharmacol (2000) 130:685–91. doi: 10.1038/sj.bjp.0703323
192. Dehpour AR, Vazifehshenas F. Rabbit isolated vas deferens possess A1 and A2 adenosine receptors. Gen Pharmacol (1992) 23:631–5. doi: 10.1016/0306-3623(92)90139-B
193. Brownhill VR, Hourani SM, Kitchen I. Differential distribution of adenosine A2 receptors in the epididymal and prostatic portions of the rat vas deferens. Eur J Pharmacol (1996) 303:87–90. doi: 10.1016/0014-2999(96)00077-5
194. Diniz C, Leal S, Goncalves J. Regional differences in the adenosine A(2) receptor-mediated modulation of contractions in rat vas deferens. Eur J Pharmacol (2003) 460:191–9. doi: 10.1016/S0014-2999(02)02926-6
195. Queiroz G, Quintas C, Talaia C, Goncalves J. Coupling to protein kinases a and c of adenosine A2B receptors involved in the facilitation of noradrenaline release in the prostatic portion of rat vas deferens. Neuropharmacology (2004) 47:216–24. doi: 10.1016/j.neuropharm.2004.03.015
196. Carlin RW, Lee JH, Marcus DC, Schultz BD. Adenosine stimulates anion secretion across cultured and native adult human vas deferens epithelia. Biol Reprod (2003) 68:1027–34. doi: 10.1095/biolreprod.102.009381
197. Sedlacek RL, Carlin RW, Singh AK, Schultz BD. Neurotransmitter-stimulated ion transport by cultured porcine vas deferens epithelium. Am J Physiol Renal Physiol 281 (2001) 281(3):F557–70. doi: 10.1152/ajprenal.2001.281.3.F557
198. Ferreira J, Levin LR, Buck J. Strategies to safely target widely expressed soluble adenylyl cyclase for contraception. Front Pharmacol (2022) 13:953903. doi: 10.3389/fphar.2022.953903
199. E. NIH, CHandHD. Infertility and fertility: Condition information (2012). Available at: https://www.nichd.nih.gov/health/topics/infertility/conditioninfo.
200. Clinic M. Male Infertility (2022). Available at: https://www.mayoclinic.org/diseases-conditions/male-infertility/symptoms-causes/syc-20374773.
Keywords: epididymis, testis, vas deferens, ATP, adenosine, inflammation, purinergic receptors, sperm
Citation: Belardin LB, Brochu K, Légaré C, Battistone MA and Breton S (2022) Purinergic signaling in the male reproductive tract. Front. Endocrinol. 13:1049511. doi: 10.3389/fendo.2022.1049511
Received: 20 September 2022; Accepted: 21 October 2022;
Published: 07 November 2022.
Edited by:
Ye Chun Ruan, Hong Kong Polytechnic University, Hong Kong SAR, ChinaCopyright © 2022 Belardin, Brochu, Légaré, Battistone and Breton. This is an open-access article distributed under the terms of the Creative Commons Attribution License (CC BY). The use, distribution or reproduction in other forums is permitted, provided the original author(s) and the copyright owner(s) are credited and that the original publication in this journal is cited, in accordance with accepted academic practice. No use, distribution or reproduction is permitted which does not comply with these terms.
*Correspondence: Sylvie Breton, c3lsdmllLmJyZXRvbkBjcmNodWRlcXVlYmVjLnVsYXZhbC5jYQ==