- 1Department of Orthopaedics, Peking University Third Hospital, Beijing, China
- 2Engineering Research Center of Bone and Joint Precision Medicine, Beijing, China
- 3Beijing Key Laboratory of Spinal Disease Research, Beijing Municipal Science & Technology Commission, Beijing, China
CTGF is a multifunctional protein and plays different roles in different cells and under different conditions. Pamrevlumab, a monoclonal antibody against CTGF, is an FDA approved drug for idiopathic pulmonary fibrosis (IPF) and Duchenne muscular dystrophy (DMD). Recent studies have shown that CTGF antibodies may potentially serve as a new drug for osteoarthritis (OA). Expression of CTGF is significantly higher in OA joints than in healthy counterparts. Increasing attention has been attracted due to its interesting roles in joint homeostasis. Joint homeostasis relies on normal cellular functions and cell-cell interactions. CTGF is essential for physiological activities of chondrocytes. Abnormal CTGF expression may cause cartilage degeneration. In this review, the physiological functions of CTGF in chondrocytes and related mechanisms are summarized. Changes in the related signaling pathways due to abnormal CTGF are discussed, which are contributing factors to inflammation, cartilage degeneration and synovial fibrosis in OA. The possibility of CTGF as a potential therapeutic target for OA treatment are reviewed.
Introduction
Connective tissue growth factor (CTGF), also named as HCS24, Ecogenin, Regenerin and CCN2, is a member of the CCN family (CTGF, Cyr61, NOV). CTGF is a multifunctional secretory protein in a variety of fibrous tissues, and involved in numerous physiological and pathophysiological processes including embryonic development, tumor formation and osteoarthritis (OA) progression (1).
CTGF, 38 kDa, consists of 4 domains, including insulin-like growth factor binding protein domains (IGFBP), von Willbrand factor C-type repeat domains (VWC), thrombospondin type-1 repeat domains (TSP-1) and cysteine knot-containing domains (CT) (2). IGFBP and VWC constitute the N-terminus of CTGF. TSP-1 and CT constitute the C-terminus of CTGF. Non-conserved protease-regulating hinge connects the N-terminal and C-terminal. All the domains have distinctive functions (3). The distictive structure provides CTGF the capability to participate in various biological processes (4).
OA is a common joint disease, mainly featured as cartilage degeneration, subchondral sclerosis and synovial inflammation (5). CTGF is involved in chondrogenesis and OA processes (6). The roles of CTGF in OA are complicated (7–9). This review summarizes the current understanding of the physiological roles of CTGF in chondrocytes and the related mechanisms for OA. The potential of CTGF as a therapeutic target for OA treatment is also briefly reviewed.
Under the physiological condition
Under the normal physiological condition, CTGF can mediate chondrocyte activities and cartilage matrix synthesis, sense environmental stimulation, and promote celluar communication. The roles and related mechanisms of CTGF in chondrocytes under the normal physiological condition better illustrate its multifunctional attributes.
Involvement in chondrocyte activities
CTGF can boost chondrocyte proliferation. Overexpression of Ctgf or treatment with recombinant CTGF (rCTGF) leads to increased proliferation of HCS-2/8 chondrocytes (10, 11). The similar results were observed in rabbit chondrocytes (12). Proliferation cell nuclear antigen (PCNA) is closely related to DNA synthesis and is a specific proliferation marker (13). A large number of PCNA positive chondrocytes were observed in the epiphyseal proliferation zone of Col2a1-ccn2 transgenic mice (14). CTGF was considered to regulate chondrocyte proliferation via MAPK and PI3K/Akt signaling pathways (15–18). The main branches of the MAPK signaling pathway include the JNK, ERK and p38MAPK pathways (19). CTGF stimulates proliferation of HCS-2/8 chondrocytes and rabbit growth plate chondrocytes through ERK (15, 16). CTGF was reported to activate the ERK signaling pathway through PKC and MEK1/2 (16). On the other hand, CTGF can form complexes by combining with cytokines directly, such as BMP2 and FGF2 (20–22). It is worth noting that responses of chondrocytes change with different complexes with FGF2. The heterocomplex formed by CTGF and FGF2 can promote proliferation of chondrocytes due to the ability to interact with FGFR1. However, the heterocomplex formed by CT module and FGF2 inhibits proliferation of chondrocytes possibly due to the inability of the CT module to bind to FGFR1 (22).
CTGF participates in several essential processes including mesenchymal cell (MSC) aggregation and terminal differentiation. In vitro culture of C3H10T1/2 cells showed that CTGF could induce MSC aggregation and upregulate expression of Sox9, a marker of chondrogenic differentiation (23). The roles of CTGF in chondrocyte differentiation depend on the specific types of cells. Under stimulation with rCTGF, COL-X and ALP, the two markers of terminal differentiation, were detected in the growth plate chondrocytes, but not in the articular chondrocytes (11). Hypertrophic differentiation of chondrocytes is highly related with endochondral osteogenesis. CTGF was proven to be an “ecogenin” (endochondral ossification genetic factor) (1, 10, 11). The expression of ALP can be increased by CTGF through p38MAPK pathway and PI3K pathway, thereby promoting endochondral ossification (15).
Sensing environmental stimulation
Chondrocytes are regulated by mechanical stimuli, which are eventually converted into biological effects by inhibiting or activating signaling pathways (24). Abnormal mechanical loading causes imbalance of chondrocyte homeostasis and promotes the occurrence and development of OA 25. CTGF is one of the “mechanical stimulation receptors” of chondrocytes (7). Significant differences were observed in the distribution and expression of CTGF at different stages of OA and in different regions of cartilage which reflected different loading conditions (26). The expression of CTGF in articular cartilage was inhibited after 4 h of cyclic loading 27. Tensile stress can induce significantly higher expression of CTGF than hydrostatic pressure (28). Different intensities of pulsed ultrasound have various effects on the production of CTGF (29).
In chondrocytes, CTGF not only senses mechanical stimulation, but also participates in transferring mechanical signals into biochemical signals in chondrocytes. CTGF transmits mechanical signals through molecules which are distributed in the cell membrane, thereby activating or inhibiting downstream signaling pathways (30–32). CTGF, as a ligand of integrins, also regulates cellular activities induced by mechanical loading through integrin pathways (7). Another way for CTGF to convert mechanical cues into biochemical signals is to activate Smad2/3 signaling in articular cartilage (33).
Expression of CTGF in chondrocytes demonstrates rhythmic changes, possibly responsible for the circadian rhythm of chondrocytes (34). During the diurnal cycle, cartilage and chondrocytes experience cyclic high- and low-intensity loadings, which is reflected by the diurnal variation in cartilage thickness at the distal femur 35. Rhythmic changes were also observed in pain and in the expressions of chondrocyte-related markers such as ACAN, COL-II, COMP (36). Proteomic sequencing of mouse articular cartilage showed that, among the 146 proteins, only expression of CTGF fluctuated with a cycle of 16 hours (34).
Mediating cartilage matrix synthesis
Cartilage is in a dynamic balance between extracellular matrix (ECM) synthesis and decomposition. Under the normal physiological condition, CTGF is a matrix protein involved in extracellular matrix protein synthesis (37). By knocking out Ctgf in chondrocytes, synthesis of COL-II and proteoglycan (PG) in ECM was reduced (10). Several researches reported that CTGF can combine with different molecules to directly or indirectly regulate synthesis and degradation of cartilage ECM. The CTGF and MMP family can synergistically function in cartilage ECM (38). Cotransfection of Ctgf and Timp-1 (MMP-specific inhibitory gene) can significantly promote the expression of PG and COL-II (39). By cutting the hinge between VWC and TSP1 in CTGF, MMPs release C-terminal fragments, which can activate Akt and ERK pathways (40, 41). CTGF, the downstream of the TGF-β signaling pathway, can also interact with the TGF-β family to influence cartilage ECM synthesis (42).
CTGF can regulate ECM formation by directly binding to ECM proteins or through cellular energy metabolism. Destruction of the interaction between CTGF and ACAN can lead to inhibition of chondrogenesis (43). The IGFBP-VWC region of CTGF promotes the synthesis and deposition of ACAN by connecting to the ACAN G3 domain (44). However, binding with the CT domain might be independent of ECM protein synthesis (45). ECM protein synthesis requires energy consumption. Adenosine triphosphate, also known as ATP, is a characteristic molecule representing energy in cells. Since cartilage is an avascular tissue, its main type of metabolism is glycolysis (46). ATP in chondrocytes can be effected by glycolysis and availability of free amino acids. In Ctgf-null chondrocytes, the free amino acid can not be captured by CTGF and glycolysis is impared, causing ATP shortage and energy production decrease (47, 48). Metabolomics and transcriptome sequencing of Ctgf-null chondrocytes also revealed low levels of ATP, ENO1 (one of the glycolysis enzymes) and ACAN (47).
Regulating communication among cells
CTGF is also known as cellular communication network factor 2 (CCN2), which acts as a signal conductor for communication among chondrocytes by intercellular junctions between chondrocytes (49). Under rCTGF intervention, the migration efficiency of chondrocytes increased in a concentration-dependent manner, and the formation of intercellular junctions of chondrocytes were significantly increased. Cx43, as the main connexin of chondrocytes, is one of the semichannel structures of gap junctions (50). CTGF can induce Cx43 opening to promote the exchange of miRNA, ATP and Ga+, through the PI3K/Akt signaling pathway (49).
CTGF can also mediate communication between chondrocytes and other types of cells, for example, osteoclast, osteoblast, and endotheliocyte (1, 51, 52). Increased secretion of CTGF in OUMS-27 cells (a human chondrocyte cell line) due to TNF-α treatment has a synergistic effect on osteoclastogenesis in coordination with M-CSF/RANKL. The possible mechanism was proposed that CTGF from chondrocytes can activate FAK and ERK1/2 through binding to αVβ3 on osteoclasts (53). CTGF secreted by chondrocytes can also act on osteoblasts close to the hypertrophic zone of cartilage in a paracrine manner during endochondral osteogenesis (54).
Under the OA condition
OA is a common chondro-related degenerative disease. Chondrocytes are the main cell in cartilage. Phenotypic changes of chondrocytes are the important characteristics of OA. CTGF is gradually recognized as an important mediator of chondrocyte activities. There are growing interests in identifying the role of CTGF in OA recently. Several studies have reported an abnormal increase in CTGF expression in OA cartilage (55) and OA synovium (6). The role of CTGF in OA is still in debate. The functions and pathways of CTGF in OA development are summarized in Figure 1.
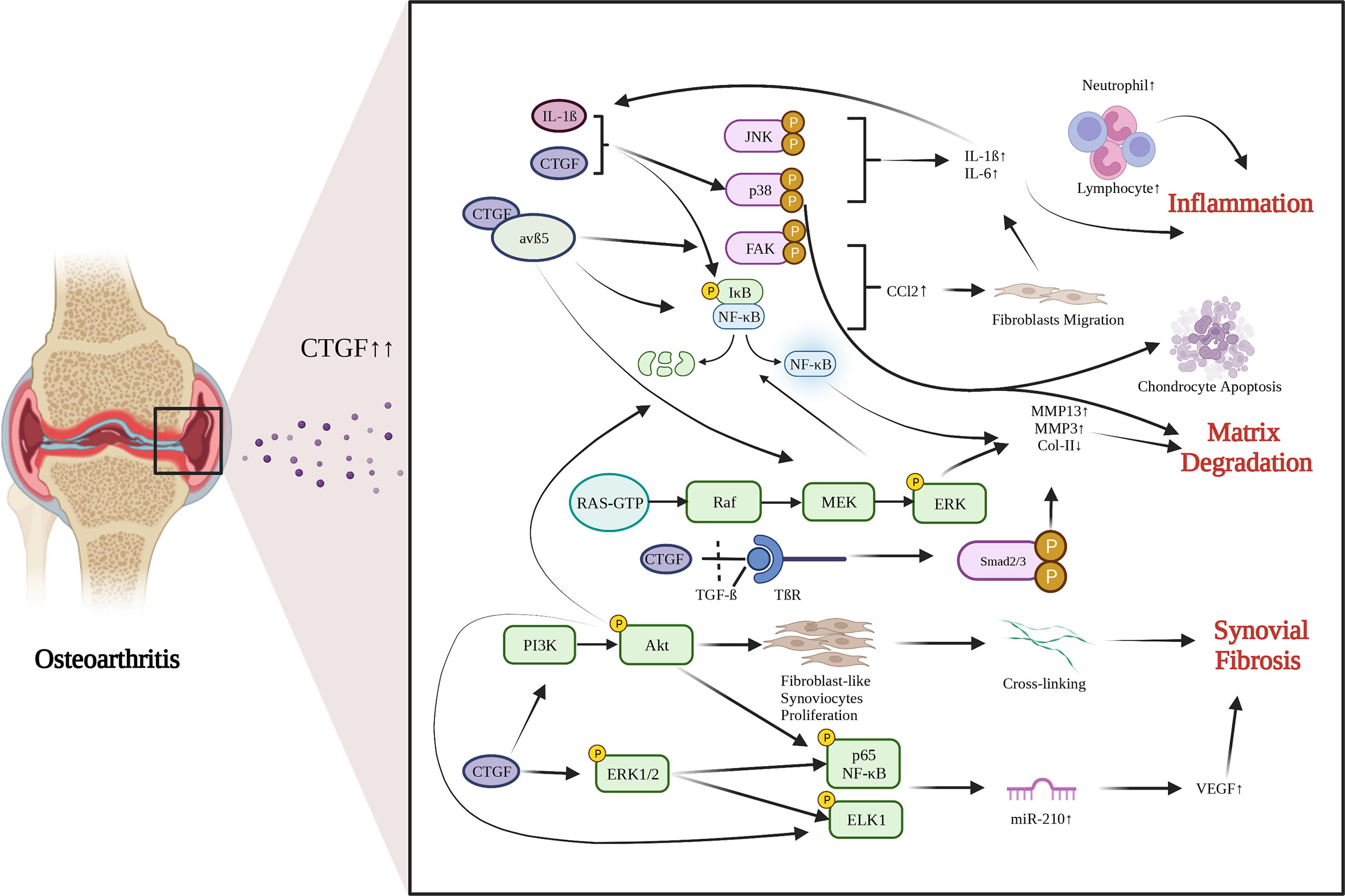
Figure 1 Functions and pathways of CTGF in OA development. CTGF is involved in promoting inflammation, matrix degradation and synovial fibrosis in articular joints. Created with biorender.com.
Cartilage degeneration
As mentioned above, CTGF mediates cartilage ECM synthesis through multiple pathways. However, whether CTGF can repair or aggravate cartilage degeneration is still unclear. The different opinions were based on in vivo studies and their designs are summarized in Table 1. The inconsistent conclusions might result from differences in types of techniques for CTGF deletion, OA models, animal species/ages/genders, experimental schedules, and OA scoring systems used in those studies. CTGF are widely distributed in other joint tissues such as synovium and bone (63). Selective deletion of CTGF might have different effects from global deletion (56). Different types of OA models represent different pathologies which might affect the microenvironment of CTGF. Proliferative activity of chondrocyte varies with different stages and status (64, 65). Thus, the ages of animal and the schedule of intervention may influence how CTGF functions. CTGF might be sensitive to microenvironment determined by multiple factors.
CTGF is essentially a multifunctional molecule that can combine with different cytokines and receptors. CTGF can combine with TGF-β to form a complex through disulfide bonds in chondrocytes (57). After abnormal mechanical stimulation, the complex binds to the TGF-β receptor, activates Smad2/3, and releases CTGF from the complex. When Ctgf is specifically knocked out, the progression of OA will be inhibited. Tang et al. mentioned that CTGF release may be related to sodium ions, which was proven in Keppies experiment (66). CTGF also controls ECM synthesis by interacting with other molecules, such as Rab14 (67) and Wif-1 (68).
Synovial fibrosis
Synovial fibrosis is one of the characteristics of OA. CTGF is a profibrotic protein, and is considered to be the main driver of many fibrotic diseases (69). Liao have proposed that CTGF is a highly discriminatory biomarker of synovial fibrosis in OA (70). Adenovirus-Ctgf was transfected into the mouse knee synovium, which exacerbated synovial fibrosis (39). Currently, the regulatory mechanisms by which CTGF induces synovial fibrosis in OA remain largely unrevealed. Remst found that TGF-β induces Lysyl hydroxylase 2b in fibroblasts via ALK5 signaling (71). CTGF may associated with TGF-β-mediated synovial fibrosis (70). CTGF can induce vascular endothelial growth factor (VEGF) production and angiogenesis in synovial fibroblasts by raising miR-210 expression via PI3K, AKT, ERK and NF-κB/ELK1 pathways, leading to aggravated synovial fibrosis (72).
Inflammation
OA has been considered a low-grade inflammatory disease. Synovial inflammation is realized to play an important role in the development of OA. CTGF, as an inflammatory mediator, is involved in synovial inflammation, inducing synovial cells to produce inflammatory cytokines (73). After treating synovial cells with 0.1 mg/mL sodium hyaluronate, the expression of CTGF was significantly decreased, and the degradation of cartilage was significantly inhibited (74). Monocyte migration can also stimulate production of inflammatory factors. CTGF can induce monocyte migration in OA by activating the downstream FAK, MEK, ERK, and NF-κB signaling pathways through interaction with integrin avβ5, and by enhancing generation and activation of CCL2, a promotor for monocyte migration (75). When synovial fibroblasts are treated with rCTGF, CTGF and integrin avβ5 jointly activate the p38 MAPK and JNK pathways, and increase the entry of p65 NF-κB and C-Jun into the nucleus to induce IL-6 expression (76). CTGF can also activate the ERK1/2, p38 MAPK and p65 NF-κB signaling pathways and increase the production of inflammatory factors and chondrocyte catabolic markers through synergistic interaction with IL-1β (77). These findings imply that CTGF acts as an inducer of inflammatory cytokines and promoter of inflammation in OA.
Current therapeutic strategy targeting CTGF
CTGF plays a critical role in the physiological and pathological states of cartilage, indicating it may be a therapeutic target for OA. Although some scholars have considered rCTGF as a cartilage regeneration strategy (78, 79), a large amount of recent data have proven that suppressing CTGF expression and antagonizing CTGF activities are effective in combatting OA. Antibodies, natural compounds, gene knockout and other interventions were used to target CTGF for OA treatment.
At the protein level, the therapeutic effects of targeting CTGF are most focused on CTGF antibody. Pamrevluma (FG-3019), a monoclonal antibody against CTGF, has already been in phase II clinical trials for treatment of PIF and DMD (80). Its application to OA treatment has not yet been reported. Miniato developed CTGF antibodies against four different modules of CTGF. Among them, IGFBP, VWC and CT module-specific antibodies can promote PG synthesis (81). Other inhibitors of OA that target CTGF include sodium hyaluronate (73), PGE2 (Prostaglandin E2); (82), AP-1 inhibitor (Curcumin), IL-1β inhibitor Berberine (59), CTGF upstream inhibitor ROCK inhibitor (Y27632) and CTGF downstream inhibitors, such as avβ5 integrin-neutralizing antibody and NF-κB inhibitor IKK (76). Berberine, is the main anti-inflammatory component of the Chinese herb Rhizoma coptidis (Huanglian). Thirty-day injection of Berberine was able to inhibit the expression of IL-1β and cartilage degeneration induced by the collagenase (59).
Great advancements in gene therapy for OA has been achieved through preclinical and clinical studies (83). Target CTGF for OA treatment at the gene level were also investigated recently. Caos study showed that miR-296-5p could inhibit the abnormal increase in CTGF expression, preventing chondrocyte apoptosis and cartilage matrix degradation in IL-1β induced OA (77). LncRNA Pvt1 knockdown can effectively improve collagen degradation caused by abnormally elevated CTGF in diabetes-mediated OA (84). Knocking out Ctgf by genome editing technology can significantly inhibit the OA process (57). Gene therapy targeting CTGF will be a promising strategy for OA.
Conclusion
CTGF is a multifunctional molecule that has great potential as a diagnostic marker and therapeutic target in OA. As an inflammatory factor, although CTGF plays an irreplaceable role in promoting chondrogenesis, it also serves as a driving factor for pathological changes in OA. It is far from clear why CTGF functions differently and how it works. Other than exploring the complex signal transduction and the different microenvironment, investigating different binding targets and different distribution of binding sites probably can explain varying effects of CTGF on chondrocytes, and shed more lights on how can we target CTGF as a treatment of OA.
Author contributions
ZY took responsibility for drafting the manuscript. ZY, WL, and CS participated in the collection, sorting, and analysis of documents. HL gave critical direction on the review and revised the manuscript. All authors contributed to the article and approved the submitted version.
Funding
This work was supported by the National Natural Science Foundation of China (Grant number: 12172011, 11872076, 11472017).
Conflict of interest
The authors declare that the research was conducted in the absence of any commercial or financial relationships that could be construed as a potential conflict of interest.
Publisher’s note
All claims expressed in this article are solely those of the authors and do not necessarily represent those of their affiliated organizations, or those of the publisher, the editors and the reviewers. Any product that may be evaluated in this article, or claim that may be made by its manufacturer, is not guaranteed or endorsed by the publisher.
References
1. Masaharu T, Takigawa M. An early history of CCN2/CTGF research: the road to CCN2 via hcs24, ctgf, ecogenin, and regenerin. J Cell communication Signaling (2018) 12(1):253–64. doi: 10.1007/S12079-017-0414-6
2. Perbal B. CCN proteins: multifunctional signalling regulators. Lancet (London England) (2004) 363(9402):62–4. doi: 10.1016/S0140-6736(03)15172-0
3. Zaykov V, Chaqour B. The CCN2/CTGF interactome: an approach to understanding the versatility of CCN2/CTGF molecular activities. J Cell communication Signaling (2021) 15(4):567–80. doi: 10.1007/S12079-021-00650-2
4. Shen YW, Zhou YD, Chen HZ, Luan X, Zhang WD. Targeting CTGF in cancer: An emerging therapeutic opportunity. Trends Cancer (2021) 7(6):511–24. doi: 10.1016/J.TRECAN.2020.12.001
5. Sharma L. Osteoarthritis of the knee. New Engl J Med (2021) 384(1):51–9. doi: 10.1056/NEJMCP1903768
6. Tu M, Yao Y, Qiao FH, Wang L. The pathogenic role of connective tissue growth factor in osteoarthritis. Bioscience Rep (2019) 39(7):1–10. doi: 10.1042/bsr20191374
7. Nishida T. Roles of CCN2 as a mechano-sensing regulator of chondrocyte differentiation. Japanese Dental Sci Rev (2020) 56(1):119–26. doi: 10.1016/J.JDSR.2020.07.001
8. Giusti V, Scotlandi K. CCN proteins in the musculoskeletal system: current understanding and challenges in physiology and pathology. J Cell Communication Signaling (2021) 15(4):545–66. doi: 10.1007/s12079-021-00631-5
9. Macdonald IJ, Huang CC, Liu SC, Lin YY, Tang CH. Targeting CCN proteins in rheumatoid arthritis and osteoarthritis. Int J Mol Sci (2021) 22(9):4340. doi: 10.3390/IJMS22094340
10. Nakanishi T, Nishida T, Shimo T, Kobayashi K, Kubo T, Tamatani T, et al. Effects of CTGF/Hcs24, a product of a hypertrophic chondrocyte-specific gene, on the proliferation and differentiation of chondrocytes in culture. Endocrinology (2000) 141(1):264–73. doi: 10.1210/ENDO.141.1.7267
11. Nishida T, Kubota S, Nakanishi T, Kuboki T, Yosimichi G, Kondo S, et al. CTGF/Hcs24, a hypertrophic chondrocyte-specific gene product, stimulates proliferation and differentiation, but not hypertrophy of cultured articular chondrocytes. J Cell Physiol (2002) 192(1):55–63. doi: 10.1002/JCP.10113
12. Fujisawa T, Hattori T, Ono M, Uehara J, Kubota S, Kuboki T, et al. CCN family 2/connective tissue growth factor (CCN2/CTGF) stimulates proliferation and differentiation of auricular chondrocytes. Osteoarthritis Cartilage (2008) 16(7):787–95. doi: 10.1016/J.JOCA.2007.11.001
13. Choe KN, Moldovan GL. Forging ahead through darkness: PCNA, still the principal conductor at the replication fork. Mol Cell (2017) 65(3):380–92. doi: 10.1016/J.MOLCEL.2016.12.020
14. Tomita N, Hattori T, Itoh S, Aoyama E, Yao M, Yamashiro T, et al. Cartilage-specific over-expression of CCN family member 2/Connective tissue growth factor (CCN2/CTGF) stimulates insulin-like growth factor expression and bone growth. PloS One (2013) 8(3):e59226. doi: 10.1371/journal.pone.0059226
15. Yosimichi G, Nakanishi T, Nishida T, Hattori T, Takano-Yamamoto T, Takigawa M. CTGF/Hcs24 induces chondrocyte differentiation through a p38 mitogen-activated protein kinase (p38MAPK), and proliferation through a p44/42 MAPK/extracellular-signal regulated kinase (ERK). Eur J Biochem (2001) 268(23):6058–65. doi: 10.1046/J.0014-2956.2001.02553.X
16. Yosimichi G, Kubota S, Nishida T, Kondo S, Yanagita T, Nakao K, et al. Roles of PKC, PI3K and JNK in multiple transduction of CCN2/CTGF signals in chondrocytes. Bone (2006) 38(6):853–63. doi: 10.1016/J.BONE.2005.11.016
17. Hori A, Nishida T, Takashiba S, Kubota S, Takigawa M. Regulatory mechanism of CCN2 production by serotonin (5-HT) via 5-HT2A and 5-HT2B receptors in chondrocytes. PLoS One (2017) 12(11):e0188014. doi: 10.1371/JOURNAL.PONE.0188014
18. Xing X, Li ZZ, Yu Z, Cheng G, Li D, Li ZZ. Effects of connective tissue growth factor (CTGF/CCN2) on condylar chondrocyte proliferation, migration, maturation, differentiation and signalling pathway. (2018) 495(1):1447–53. doi: 10.1016/J.BBRC.2017.11.190
19. Fang JY, Richardson BC. The MAPK signalling pathways and colorectal cancer. Lancet Oncol (2005) 6(5):322–7. doi: 10.1016/S1470-2045(05)70168-6
20. Nishida T, Kondo S, Maeda A, Kubota S, Lyons KM, Takigawa M. CCN family 2/connective tissue growth factor (CCN2/CTGF) regulates the expression of vegf through hif-1α expression in a chondrocytic cell line, HCS-2/8, under hypoxic condition. Bone (2009) 44(1):24–31. doi: 10.1016/J.BONE.2008.08.125
21. Maeda S. An impact of CCN2-BMP-2 complex upon chondrocyte biology: Evoking a signalling pathway bypasses ERK and smads? J Biochem (2011) 150(3):219–21. doi: 10.1093/JB/MVR089
22. Aoyama E, Kubota S, Takigawa M. CCN2/CTGF binds to fibroblast growth factor receptor 2 and modulates its signaling. FEBS Lett (2012) 586(24):4270–5. doi: 10.1016/J.FEBSLET.2012.10.038
23. Song JJ, Aswad R, Kanaan RA, Rico MC, Owen TA, Barbe MF, et al. Connective tissue growth factor (CTGF) acts as a downstream mediator of TGF-beta1 to induce mesenchymal cell condensation. J Cell Physiol (2007) 210(2):398–410. doi: 10.1002/JCP.20850
24. Qin L, He T, Chen S, Yang D, Yi W, Cao H, et al. Roles of mechanosensitive channel Piezo1/2 proteins in skeleton and other tissues. Bone Res 2021 9:1 (2021) 9(1):1–17. doi: 10.1038/s41413-021-00168-8
25. Yang Y, Wang Y, Kong Y, Zhang X, Zhang H, Feng X, et al. Moderate mechanical stimulation protects rats against osteoarthritis through the regulation of TRAIL via the NF- κ B/NLRP3 pathway. Oxid Med Cell Longevity (2020) 2020. doi: 10.1155/2020/6196398
26. Omoto S, Nishida K, Yamaai Y, Shibahara M, Nishida T, Doi T, et al. Expression and localization of connective tissue growth factor (CTGF/Hcs24/CCN2) in osteoarthritic cartilage. Osteoarthritis Cartilage (2004) 12(10):771–8. doi: 10.1016/J.JOCA.2004.06.009
27. Lomas C, Tang XD, Chanalaris A, Saklatvala J, Vincent TL. Cyclic mechanical load causes global translational arrest in articular chondrocytes: a process which is partially dependent upon PKR phosphorylation. Eur Cells materials (2011) 22:178–89. doi: 10.22203/ecm.v022a14
28. Wong M, Siegrist M, Goodwin K. Cyclic tensile strain and cyclic hydrostatic pressure differentially regulate expression of hypertrophic markers in primary chondrocytes. Bone (2003) 33(4):685–93. doi: 10.1016/S8756-3282(03)00242-4
29. Kamatsuki Y, Aoyama E, Furumatsu T, Miyazawa S, Maehara A, Yamanaka N, et al. Possible reparative effect of low-intensity pulsed ultrasound (LIPUS) on injured meniscus. J Cell Communication Signaling (2019) 13(2):193–207. doi: 10.1007/S12079-018-0496-9
30. Hall-Glenn F, Aivazi A, Akopyan L, Ong JR, Baxter RR, Benya PD, et al. CCN2/CTGF is required for matrix organization and to protect growth plate chondrocytes from cellular stress. J Cell Communication Signaling (2013) 7(3):219–30. doi: 10.1007/S12079-013-0201-Y
31. Nishida T, Kubota S, Aoyama E, Yamanaka N, Lyons KM, Takigawa M. Low-intensity pulsed ultrasound (LIPUS) treatment of cultured chondrocytes stimulates production of CCN family protein 2 (CCN2), a protein involved in the regeneration of articular cartilage: mechanism underlying this stimulation. Osteoarthritis cartilage (2017) 25(5):759–69. doi: 10.1016/J.JOCA.2016.10.003
32. Dieterle MP, Husari A, Rolauffs B, Steinberg T, Tomakidi P. Integrins, cadherins and channels in cartilage mechanotransduction: perspectives for future regeneration strategies. Expert Rev Mol Med (2021) 23:1–16. doi: 10.1017/ERM.2021.16
33. Madej W, van Caam A, Blaney Davidson EN, Hannink G, Buma P, van der Kraan PM. Ageing is associated with reduction of mechanically-induced activation of Smad2/3P signaling in articular cartilage. Osteoarthritis cartilage (2016) 24(1):146–57. doi: 10.1016/J.JOCA.2015.07.018
34. Dudek M, Angelucci C, Pathiranage D, Wang P, Mallikarjun V, Lawless C, et al. Circadian time series proteomics reveals daily dynamics in cartilage physiology. Osteoarthritis cartilage (2021) 29(5):739–49. doi: 10.1016/J.JOCA.2021.02.008
35. Kilic E, Kilic G, Ozgocmen S. Diurnal variation in distal femoral cartilage thickness. J Rheumatol (2015) 42(11):2215–5. doi: 10.3899/JRHEUM.150418
36. Yang N, Meng QJ. Circadian clocks in articular cartilage and bone: A compass in the sea of matrices. J Biol Rhythms (2016) 31(5):415–27. doi: 10.1177/0748730416662748
37. Choi H, Tessier S, Silagi ES, Kyada R, Yousefi F, Pleshko N, et al. A novel mouse model of intervertebral disc degeneration shows altered cell fate and matrix homeostasis. Matrix Biol (2018) 70:102–22. doi: 10.1016/J.MATBIO.2018.03.019
38. Eguchi T, Kubota S, Kawata K, Mukudai Y, Uehara J, Ohgawara T, et al. Novel transcription factor-like function of human matrix metalloproteinase 3 regulating the CTGF/CCN2 gene. Mol Cell Biol (2008) 28(7):2391–413. doi: 10.1128/MCB.01288-07
39. Davidson ENB, Vitters EL, Mooren FM, Oliver N, Van Den Berg WB, Van Der Kraan PM. Connective tissue growth factor/CCN2 overexpression in mouse synovial lining results in transient fibrosis and cartilage damage. (2006) 54(5):1653–61. doi: 10.1002/ART.21795
40. Hashimoto G, Inoki I, Fujii Y, Aoki T, Ikeda E, Okada Y. Matrix metalloproteinases cleave connective tissue growth factor and reactivate angiogenic activity of vascular endothelial growth factor 165. J Biol Chem (2002) 277(39):36288–95. doi: 10.1074/JBC.M201674200
41. Kaasbøll OJ, Gadicherla AK, Wang JH, Monsen VT, Hagelin EMV, Dong MQ, et al. Connective tissue growth factor (CCN2) is a matricellular preproprotein controlled by proteolytic activation. J Biol Chem (2018) 293(46):17953–70. doi: 10.1074/JBC.RA118.004559
42. Shimo T, Kanyama M, Wu C, Sugito H, Billings PC, Abrams WR, et al. Expression and roles of connective tissue growth factor in meckels cartilage development. Dev Dynamics (2004) 231(1):136–47. doi: 10.1002/DVDY.20109
43. Ivkovic S, Yoon BS, Popoff SN, Safadi FF, Libuda DE, Stephenson RC, et al. Connective tissue growth factor coordinates chondrogenesis and angiogenesis during skeletal development. Development (2003) 130(12):2779–91. doi: 10.1242/DEV.00505
44. Aoyama E, Hattori T, Hoshijima M, Araki D, Nishida T, Kubota S, et al. N-terminal domains of CCN family 2/connective tissue growth factor bind to aggrecan. (2009) 420(3):413–20. doi: 10.1042/BJ20081991
45. Hoshijima M, Hattori T, Inoue M, Araki D, Hanagata H, Miyauchi A, et al. CT domain of CCN2/CTGF directly interacts with fibronectin and enhances cell adhesion of chondrocytes through integrin alpha5beta1. FEBS Lett (2006) 580(5):1376–82. doi: 10.1016/J.FEBSLET.2006.01.061
46. Zheng L, Zhang Z, Sheng P, Mobasheri A. The role of metabolism in chondrocyte dysfunction and the progression of osteoarthritis. Ageing Res Rev (2021) 66:101249. doi: 10.1016/J.ARR.2020.101249
47. Maeda-Uematsu A, Kubota S, Kawaki H, Kawata K, Miyake Y, Hattori T, et al. CCN2 as a novel molecule supporting energy metabolism of chondrocytes. (2014) 115(5):854–65. doi: 10.1002/JCB.24728
48. Murase Y, Hattori T, Aoyama E, Nishida T, Maeda-Uematsu A, Kawaki H, et al. Role of CCN2 in amino acid metabolism of chondrocytes. (2016) 117(4):927–37. doi: 10.1002/JCB.25377
49. Wu Z, Zhou C, Yuan Q, Zhang D, Xie J, Zou S. CTGF facilitates cell-cell communication in chondrocytes via PI3K/Akt signalling pathway. Cell Proliferation (2021) 54(3). doi: 10.1111/CPR.13001
50. Mayan MD, Gago-Fuentes R, Carpintero-Fernandez P, Fernandez-Puente P, Filgueira-Fernandez P, Goyanes N, et al. Articular chondrocyte network mediated by gap junctions: role in metabolic cartilage homeostasis. Ann rheumatic Dis (2015) 74(1):275–84. doi: 10.1136/ANNRHEUMDIS-2013-204244
51. Aoyama E, Kubota S, Khattab HM, Nishida T, Takigawa M. CCN2 enhances RANKL-induced osteoclast differentiation via direct binding to RANK and OPG. Bone (2015) 73:242–8. doi: 10.1016/J.BONE.2014.12.058
52. He H, Luo H, Liu L, Shangguan Y, Xie X, Wen Y, et al. Prenatal caffeine exposure caused h-type blood vessel-related long bone dysplasia via miR375/CTGF signaling. FASEB journal : Off Publ Fed Am Societies Exp Biol (2021) 35(2):e21370. doi: 10.1096/FJ.202002230R
53. Nozawa K, Fujishiro M, Kawasaki M, Kaneko H, Iwabuchi K, Yanagida M, et al. Connective tissue growth factor promotes articular damage by increased osteoclastogenesis in patients with rheumatoid arthritis. Arthritis Res Ther (2009) 11(6):R174. doi: 10.1186/AR2863
54. Takigawa M, Nakanishi T, Kubota S, Nishida T. Role of CTGF/HCS24/ecogenin in skeletal growth control. (2003) 194(3):256–66. doi: 10.1002/JCP.10206
55. Honsawek S, Yuktanandana P, Tanavalee A, Chirathaworn C, Anomasiri W, Udomsinprasert W, et al. Plasma and synovial fluid connective tissue growth factor levels are correlated with disease severity in patients with knee osteoarthritis. Biomarkers : Biochem Indic exposure response susceptibility to chemicals (2012) 17(4):303–8. doi: 10.3109/1354750X.2012.666676
56. Keenan CM, Ramos-Mucci L, Kanakis I, Milner PI, Leask A, Abraham D, et al. Post-traumatic osteoarthritis development is not modified by postnatal chondrocyte deletion of Ccn2. DMM Dis Models Mech (2020) 13(7):dmm044719. doi: 10.1242/dmm.044719
57. Tang X, Muhammad H, McLean C, Miotla-Zarebska J, Fleming J, Didangelos A, et al. Connective tissue growth factor contributes to joint homeostasis and osteoarthritis severity by controlling the matrix sequestration and activation of latent TGFβ. Ann Rheumatic Dis (2018) 77(9):1372–80. doi: 10.1136/annrheumdis-2018-212964
58. Wei J, Fu W, Hettinghouse A, He W, Lipson KE, Liu C, et al. Role of ADAMTS-12 in protecting against inflammatory arthritis in mice by interacting with and inactivating proinflammatory connective tissue growth factor. Arthritis Rheumatol (Hoboken N.J.) (2018) 70(11):1745–56. doi: 10.1002/ART.40552
59. Liu SC, Lee HP, Hung CY, Tsai CH, Li TM, Tang CH. Berberine attenuates CCN2-induced IL-1β expression and prevents cartilage degradation in a rat model of osteoarthritis. Toxicol Appl Pharmacol (2015) 289(1):20–9. doi: 10.1016/J.TAAP.2015.08.020
60. Abd El Kader T, Kubota S, Nishida T, Hattori T, Aoyama E, Janune D, et al. The regenerative effects of CCN2 independent modules on chondrocytes in vitro and osteoarthritis models in vivo. Bone (2014) 59:180–8. doi: 10.1016/J.BONE.2013.11.010
61. Nishida T, Kubota S, Kojima S, Kuboki T, Nakao K, Kushibiki T, et al. Regeneration of defects in articular cartilage in rat knee joints by CCN2 (connective tissue growth factor). J Bone Mineral Res (2004) 19(8):1308–19. doi: 10.1359/JBMR.040322
62. Itoh S, Hattori T, Tomita N, Aoyama E, Yutani Y, Yamashiro T, et al. CCN family member 2/Connective tissue growth factor (CCN2/CTGF) has anti-aging effects that protect articular cartilage from age-related degenerative changes. PloS One (2013) 8(8):e71156. doi: 10.1371/JOURNAL.PONE.0071156
63. Kubota S, Takigawa M. Cellular and molecular actions of CCN2/CTGF and its role under physiological and pathological conditions. Clin Sci (2014) 128(3):181–96. doi: 10.1042/CS20140264
64. Sandell LJ, Aigner T. Articular cartilage and changes in arthritis an introduction: Cell biology of osteoarthritis. Arthritis Res (2001) 3(2):107–13. doi: 10.1186/AR148/FIGURES/1
65. Jiang Y, Tuan RS. Origin and function of cartilage stem/progenitor cells in osteoarthritis. Nat Rev Rheumatol (2015) 11(4):206–12. doi: 10.1038/NRRHEUM.2014.200
66. Keppie SJ, Mansfield JC, Tang X, Philp CJ, Graham HK, Önnerfjord P, et al. Matrix-bound growth factors are released upon cartilage compression by an aggrecan-dependent sodium flux that is lost in osteoarthritis. Funct (Oxford England) (2021) 2(5):zqab037. doi: 10.1093/FUNCTION/ZQAB037
67. Hoshijima M, Hattori T, Aoyama E, Nishida T, Kubota S, Kamioka H, et al. Roles of interaction between ccn2 and rab14 in aggrecan production by chondrocytes. Int J Mol Sci (2020) 21(8):2769. doi: 10.3390/ijms21082769
68. Surmann-Schmitt C, Sasaki T, Hattori T, Eitzinger N, Schett G, von der Mark K, et al. The wnt antagonist wif-1 interacts with CTGF and inhibits CTGF activity. J Cell Physiol (2012) 227(5):2207–16. doi: 10.1002/JCP.22957
69. Richeldi L, Fernández Pérez ER, Costabel U, Albera C, Lederer DJ, Flaherty KR, et al. Pamrevlumab, an anti-connective tissue growth factor therapy, for idiopathic pulmonary fibrosis (PRAISE): a phase 2, randomised, double-blind, placebo-controlled trial. Lancet Respir Med (2020) 8(1):25–33. doi: 10.1016/S2213-2600(19)30262-0
70. Liao B, Guan M, Tan Q, Wang G, Zhang R, Huang J, et al. Low-intensity pulsed ultrasound inhibits fibroblast-like synoviocyte proliferation and reduces synovial fibrosis by regulating wnt/β-catenin signaling. J orthopaedic translation (2021) 30:41–50. doi: 10.1016/J.JOT.2021.08.002
71. Remst DFG, Blaney Davidson EN, Vitters EL, Bank RA, Van Den Berg WB, Van Der Kraan PM. TGF-ß induces lysyl hydroxylase 2b in human synovial osteoarthritic fibroblasts through ALK5 signaling. Cell Tissue Res (2014) 355(1):163–71. doi: 10.1007/S00441-013-1740-5
72. Liu SC, Chuang SM, Hsu CJ, Tsai CH, Wang SW, Tang CH. CTGF increases vascular endothelial growth factor-dependent angiogenesis in human synovial fibroblasts by increasing miR-210 expression. Cell Death Dis (2014) 5(10):e1485–5. doi: 10.1038/cddis.2014.453
73. Lee YT, Shao HJ, Wang JH, Liu HC, Hou SM, Young TH. Hyaluronic acid modulates gene expression of connective tissue growth factor (CTGF), transforming growth factor-beta1 (TGF-beta1), and vascular endothelial growth factor (VEGF) in human fibroblast-like synovial cells from advanced-stage osteoarthritis in vitro. J orthopaedic research : Off Publ Orthopaedic Res Soc (2010) 28(4):492–6. doi: 10.1002/JOR.21029
74. Jones IA, Togashi R, Wilson ML, Heckmann N, Vangsness CT. Intra-articular treatment options for knee osteoarthritis. Nat Rev Rheumatol (2019) 15(2):77–90. doi: 10.1038/S41584-018-0123-4
75. Liu SC, Hsu CJ, Fong YC, Chuang SM, Tang CH. CTGF induces monocyte chemoattractant protein-1 expression to enhance monocyte migration in human synovial fibroblasts. Biochim Biophys Acta (2013) 1833(5):1114–24. doi: 10.1016/J.BBAMCR.2012.12.014
76. Liu SC, Hsu CJ, Chen H, Tsou HK, Chuang SM, Tang CH. CTGF increases IL-6 expression in human synovial fibroblasts through integrin-dependent signaling pathway. PloS One (2012) 7(12):e51097. doi: 10.1371/JOURNAL.PONE.0051097
77. Cao Z, Liu W, Qu X, Bi H, Sun X, Yu Q, et al. miR-296-5p inhibits IL-1β-induced apoptosis and cartilage degradation in human chondrocytes by directly targeting TGF-β1/CTGF/p38MAPK pathway. Cell Cycle (2020) 19(12):1443–53. doi: 10.1080/15384101.2020.1750813
78. Djouad F, Delorme B, Maurice M, Bony C, Apparailly F, Louis-Plence P, et al. Microenvironmental changes during differentiation of mesenchymal stem cells towards chondrocytes. Arthritis Res Ther (2007) 9(2):R33. doi: 10.1186/AR2153
79. Hara ES, Ono M, Kubota S, Sonoyama W, Oida Y, Hattori T, et al. Novel chondrogenic and chondroprotective effects of the natural compound harmine. Biochimie (2013) 95(2):374–81. doi: 10.1016/J.BIOCHI.2012.10.016
80. Wells AU. Pamrevlumab in idiopathic pulmonary fibrosis. Lancet Respir Med (2020) 8(1):2–3. doi: 10.1016/S2213-2600(19)30339-X
81. Minato M, Kubota S, Kawaki H, Nishida T, Miyauchi A, Hanagata H, et al. Module-specific antibodies against human connective tissue growth factor: utility for structural and functional analysis of the factor as related to chondrocytes. J Biochem (2004) 135(3):347–54. doi: 10.1093/JB/MVH042
82. Masuko K, Murata M, Yudoh K, Shimizu H, Beppu M, Nakamura H, et al. Prostaglandin E2 regulates the expression of connective tissue growth factor (CTGF/CCN2) in human osteoarthritic chondrocytes via the EP4 receptor. BMC Res Notes (2010) 3(5). doi: 10.1186/1756-0500-3-5
83. Bellavia D, Veronesi F, Carina V, Costa V, Raimondi L, De Luca A, et al. Gene therapy for chondral and osteochondral regeneration: is the future now? Cell Mol Life sciences : CMLS (2018) 75(4):649–67. doi: 10.1007/S00018-017-2637-3
84. Ding L, Li Y, Liu GY, Li TH, Li F, Guan J, et al. Long non-coding RNA PVT1, a molecular sponge of miR-26b, is involved in the progression of hyperglycemia-induced collagen degradation in human chondrocytes by targeting CTGF/TGF- β signal ways. Innate Immun (2020) 26(3):204–14. doi: 10.1177/1753425919881778
Keywords: CTGF, chondrocyte, cartilage, degeneration, osteoarthritis treatment
Citation: Yang Z, Li W, Song C and Leng H (2022) CTGF as a multifunctional molecule for cartilage and a potential drug for osteoarthritis. Front. Endocrinol. 13:1040526. doi: 10.3389/fendo.2022.1040526
Received: 09 September 2022; Accepted: 29 September 2022;
Published: 17 October 2022.
Edited by:
Yichuan Pang, Tongji University, ChinaReviewed by:
Yuki Matsushita, Nagasaki University, JapanCopyright © 2022 Yang, Li, Song and Leng. This is an open-access article distributed under the terms of the Creative Commons Attribution License (CC BY). The use, distribution or reproduction in other forums is permitted, provided the original author(s) and the copyright owner(s) are credited and that the original publication in this journal is cited, in accordance with accepted academic practice. No use, distribution or reproduction is permitted which does not comply with these terms.
*Correspondence: Huijie Leng, lenghj@bjmu.edu.cn