- 1Department of Endocrinology and Metabolism, Affiliated Hospital of Southwest Medical University, Luzhou, China
- 2Metabolic Vascular Disease Key Laboratory of Sichuan Province, Luzhou, China
- 3Sichuan Clinical Research Center for Nephropathy, Luzhou, China
- 4Cardiovascular and Metabolic Diseases Key Laboratory of Luzhou, Luzhou, China
Background: This study aimed to evaluate the relationship between thyroid-related hormones and vascular complications in type 2 diabetes mellitus (T2DM) patients with euthyroidism.
Methods: We enrolled 849 patients with T2DM after screening out the ineligible. Multivariate logistic regression was used to analyze the relationship between fT3, fT4, the fT3/fT4 ratio, thyroid-stimulating hormone, and diabetic vascular complications. Spearman correlation analysis was used to determine the correlation between thyroid-related hormones and vascular complications.
Results: In this cross-sectional study of T2DM, 538 patients with carotid atherosclerosis (CA) and 299 patients with diabetic peripheral neuropathy (DPN). The prevalence of DPN was negatively correlated with fT3 and the fT3/fT4 ratio but positively correlated with fT4 (all P<0.01). At the same time, the odds ratio for DPN decreased with increasing fT3 (T1: reference; T2: OR: 0.689, 95%CI: 0.477, 0.993; T3: OR: 0.426, 95% CI: 0.286, 0.633, all P<0.05) and fT3/fT4 ratio (T1: reference; T2: OR: 0.528, 95% CI: 0.365, 0.763; T3: OR: 0.413, 95% CI: 0.278, 0.613, all P<0.001). In terms of sensitivity and specificity, fT4 was found to be 39.5% and 71.4% accurate, respectively, with a 95% CI of 0.531-0.611.
Conclusions: We found a negative correlation between fT3 and fT3/fT4 ratio and the number of individuals with DPN, and a positive correlation between fT4 and the prevalence of DPN.
Introduction
One of the most frequent endocrine illnesses is diabetes mellitus (1). According to a recent report from the International Diabetes Federation (version 2019), the global prevalence of diabetes is expected to increase from 9.3% in 2019 to 10.9% in 2045 (2). More than 90% of people with diabetes have type 2 diabetes mellitus (T2DM) (3–5). The worldwide pandemic of type 2 diabetes indicates that more people are suffering from the psychological and physical burden of diabetes (6–8). T2DM can cause many vascular complications, such as coronary heart disease, renal disease, retinopathy, and diabetic peripheral neuropathy (DPN) (9, 10). Cardiovascular problems are the primary cause of death in T2DM patients (4). Therefore, other modifiable risk factors need to be investigation to prevent cardiovascular complications.
Like T2DM, thyroid diseases are also common endocrine system disorders (11). A growing number of studies suggest that abnormal thyroid function may impact glucose metabolism, insulin sensitivity, and the development of chronic complications of diabetes (12, 13). This indicates that T2DM and its long-term consequences may be associated with abnormal thyroid function (14, 15). Recent studies have shown that free triiodothyronine (fT3), free thyroxine (fT4) and thyroid-stimulating hormone (TSH) levels have been linked to insulin resistance and chronic complications of diabetes such as carotid atherosclerosis (CA) and DPN (16–19). However, patients with T2DM who have normal thyroid function have rarely been found to have a link between thyroid-related hormones and vascular problems like DPN and CA.
Therefore, the current study aimed to evaluate the association between fT3, fT4, fT3/fT4, TSH and DPN, CA in T2DM patients with euthyroidism.
Methods
Study subjects
A retrospective cross-sectional study was performed by the Department of Endocrinology and Metabolism at the Affiliated Hospital of Southwest Medical University on T2DM patients hospitalized in the department from 2018 to 2020. Inclusion criteria: (1) age > 18 years old; (2) T2DM diagnosis according to the American Diabetes Association “Standards of Medical Care in Diabetes” diagnostic criteria (2019 version (20).; (3) Normal thyroid function was defined as: fT3: 1.80–3.80 pg/mL, fT4: 0.78–1.86 ng/dL, TSH: 0.38–5.57 mIU/L. Exclusion criteria: (1) abnormal thyroid function test index; (2) a history of thyroid disease or thyroid surgery; (3) use of thyroxine preparations, amiodarone, and other drugs affecting thyroid function. Information for the study was collected by health professionals using a traditional questionnaire and validated equipment. Each participant provided demographic information and medical record information. We divided smokers into current smokers and nonsmokers. Similarly, alcohol drinkers were divided into current drinkers and non-drinkers. Mean arterial blood pressure was derived from three right arm arterial blood pressure measurements taken continuously for at least 30 min during a physical examination.
The study complied with the ethical standards of the Declaration of Helsinki (2013) and was approved by the Ethics Committee of the Affiliated Hospital of Southwest Medical University (ethical approval code: 2018017) (21). All enrolled participants signed the informed consent form.
FT3/fT4 ratio assessment
FT3/fT4 ratio was determined as follows: fT3 (pg/mL)/fT4 (ng/dL). For further analysis, the fT3/fT4 ratio was transformed into categorical variables: T1: <1.88, T2: 1.88–2.24, T3: >2.24.
Diagnosis of diabetic vascular complications
All subjects were scanned bilaterally in the carotid arteries by the same color Doppler ultrasound diagnostic instrument under the guidance of an ultrasound operator. During data collection, measurements were performed by a single operator. The criteria for atherosclerotic plaque formation were local carotid intima-media thickness (CIMT) ≥1.5 mm or local CIMT greater than 50% of the peripheral area. The diagnosis of carotid atherosclerosis was defined as an increase in CIMT ≥1.0 mm and/or the presence of carotid plaque (22–25). At least one symptom and/or one nerve conduction study neuropathy and aberrant signs are needed to diagnose DPN, as defined by the Toronto International Consensus for DPN (26).
Statistics analysis
Descriptive statistics were used to compare baseline clinical characteristics of patients according to sex. For comparison between groups, one-way analysis of variance (ANOVA) (normally distributed continuous variables), Kruskal-Wallis H test (non-normally distributed continuous variables), and χ2 test (categorical variables) were used. Logistic regression analysis models were used to detect variables affecting diabetic complications. The relationship between thyroid-related hormones and vascular complications was identified by Spearman correlation analysis. We examined the anticipated accuracy of thyroid-related hormones using receiver operating characteristic (ROC) curves. All hypotheses were tested bilaterally at an α level of 0.05. The weighting of each risk factor was according to the values of odds ratio (OR). GraphPad Prism (version 9.0) was used to generate forest plots. SPSS (version 26.0) was used for all data analyses.
Results
In this study, 869 patients with T2DM participated. There were 468 men and 401 women in the group. The mean age was 55.23 ± 11.14 years in men and 59.30 ± 10.23 years in women. and an average of 88.83 ± 73.43 months of diabetes on record. There were 538 patients with CA and 299 patients with DPN. The detailed demographic information and biochemical indicators of the participants are shown in Table 1. Compared with women, men had higher values of height, weight, body mass index (BMI), waist circumference (WC), diastolic blood pressure (DBP), alanine aminotransferase (ALT), FT3, FT3/FT4, visceral fat area (VFA), current smoking rates, current alcohol consumption, and use of antihypertensive medication (all P<0.05). Conversely, women had higher values for mean age, systolic blood pressure (SBP), high-density lipoprotein cholesterol (HDL), TSH, subcutaneous fat area (SFA), use of hypoglycemic drugs, and duration of diabetes (all P<0.05).
Table 2 demonstrates the distribution of CA and DPN in the fT3, fT4, fT3/fT4 ratio, and TSH tertiles. The prevalence of CA in the fT3/fT4 tertiles was 66.2%, 56.0%, and 63.5%, respectively. Moreover, the prevalence of DPN negatively correlated with fT3 (42.7%, 33.6%, 26.8%, P<0.05) and fT3/fT4 ratio (46.9%, 30.9%, 25.3%, P<0.001), but positively correlated with fT4 (29.4%, 32.3%, 42.0%). Importantly, the prevalence of CA and DPN in all tertiles of TSH were virtually identical.
Multivariate regression models were used to calculate the odds ratios for DPN (Table 3). A higher fT3/fT4 ratio was associated with a lower odds ratio for DPN after adjustment for age and sex in model 2 (T1: reference; T2: OR: 0.482, 95% CI: 0.342, 0.680; T3: OR: 0.369, 95% CI: 0.258, 0.528; all P<0.001). This trend remained unchanged after adjustment for the remaining confounders in model 3 (T1: reference; T2: OR: 0.518, 95% CI: 0.352, 0.760; T3: OR: 0.356, 95% CI: 0.234, 0.542; all P<0.001). FT3 and the fT3/fT4 ratio were negatively related to DPN risk, whereas fT4 had the opposite effect. Unfortunately, we did not find a correlation between TSH and DPN prevalence.
As shown in Table 4, the association between thyroid-related hormones and vascular complications in T2DM patients with euthyroidism was determined by Spearman correlation analysis. The results revealed a negative correlation between fT3 (rs=-0.144, P<0.001) and fT3/fT4 (rs=-0.193, P<0.001) and DPN, and a positive correlation between fT4 (rs=0.117, P=0.001) and DPN. However, we did not find any relationship between thyroid-related hormones and CA, and the relationship between TSH and DPN.
Furthermore, we used fT3 tertiles to separate the DPN groups by, sex and age (Figure 1). The results showed that the overall prevalence was higher in men than in women. Meanwhile, all DPN subgroups, except those aged > 65 years, showed a declining prevalence with increasing fT3 (P<0.05).
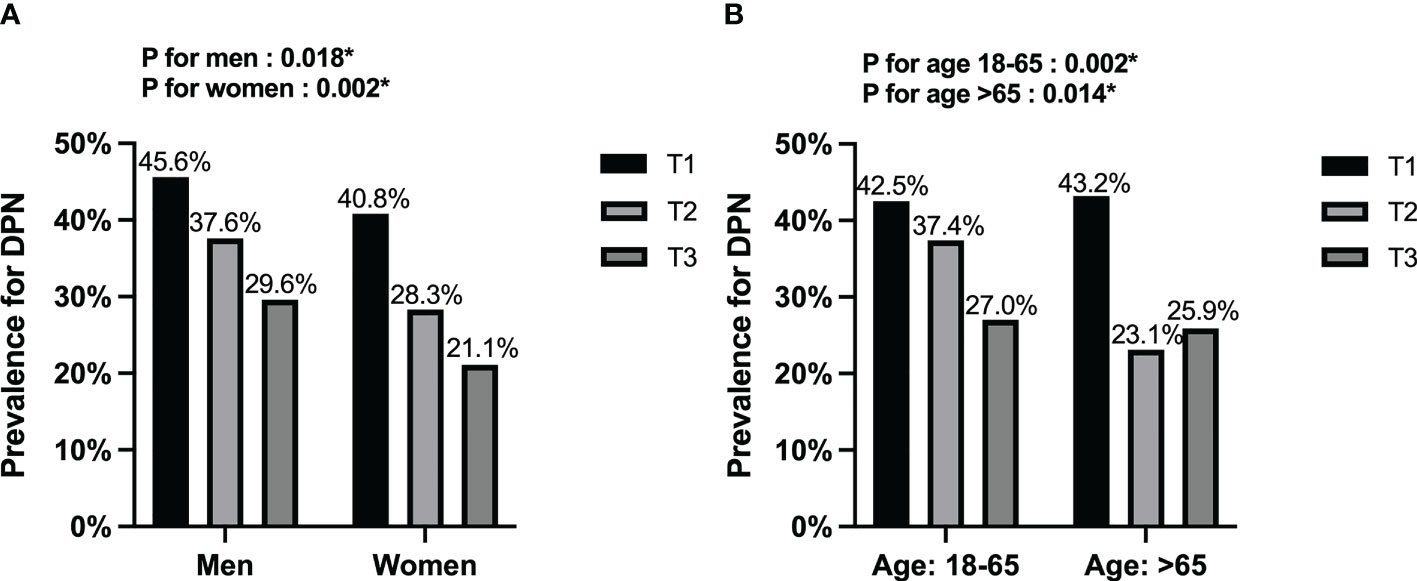
Figure 1 Stratification analysis of DPN among the fT3 tertiles. (A) Stratified by sex; (B) Stratified by age. DPN, diabetic peripheral neuropathy. *P<0.05.
As illustrated in Figure 2, we performed multivariate regression analysis for variables independently associated with diabetic CA or DPN. CA risk factors were age (OR: 1.073, 95% CI: 1.054, 1.091) and current smoking (OR: 1.893, 95% CI: 1.244, 2.878) (all P<0.01). And the forest plot revealed that HbA1c (OR: 1.104, 95% CI: 1.027, 1.187), DBP (OR: 1.032, 95%CI: 1.017,1.047), low-density lipoprotein cholesterol (LDL) (OR: 1.182, 95% CI: 1.022, 1.367), fT4 (OR: 3.736, 95%CI: 1.691,8.256), duration of diabetes (OR: 1.004, 95% CI: 1.002, 1.007), and insulin use (OR: 2.021, 95% CI: 1.380, 2.959) were risk factors for DPN (all P <0.05). Moreover, age (OR: 0.977, 95% CI: 0.963, 0.993) and antihypertensive drugs use (OR: 0.537, 95% CI: 0.354, 0.814) were protected factors for DPN (all P <0.01).
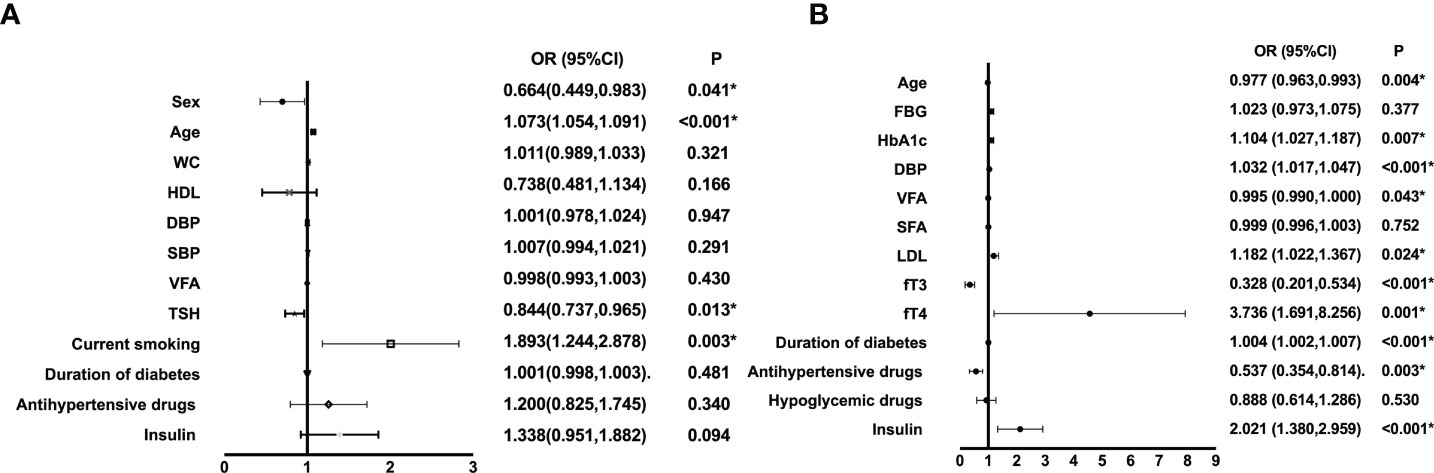
Figure 2 Multiple regression analysis of variables independently associated with CA (A) or DPN (B) in all participants. WC, waist circumference; SBP, systolic blood pressure; HDL, high-density lipoprotein cholesterol; FBG, fasting blood glucose; HbA1c, hemoglobin A1c; DBP, diastolic blood pressure; VFA, visceral fat area; SFA, subcutaneous fat area; LDL, low-density lipoprotein cholesterol; fT3, free triiodothyronine1; fT4, free thyroxine; TSH, thyroid stimulating hormone; CA, carotid atherosclerosis; DPN, diabetic peripheral neuropathy. *P<0.05.
Finally, we assessed the diagnostic value of thyroid-related hormones for DPN using a ROC curve (Figure 3). With an under the curve (AUC) of 0.571 (95% CI: 0.531, 0.611, P<0.001), fT4 was found to be the most accurate, followed by TSH (AUC: 0.485, 95%CI: 0.444, 0.525, P=0.455), fT3 (AUC: 0.412, 95%CI: 0.373, 0.452, P<0.01), fT3/fT4 ratio (AUC: 0.383, 95%CI: 0.343, 0.422, P<0.001). By calculating the Jorden index, the optimum cutoff value of fT4 was 1.325. The sensitivity of fT4 was 39.5%, and the specificity was 71.4%.
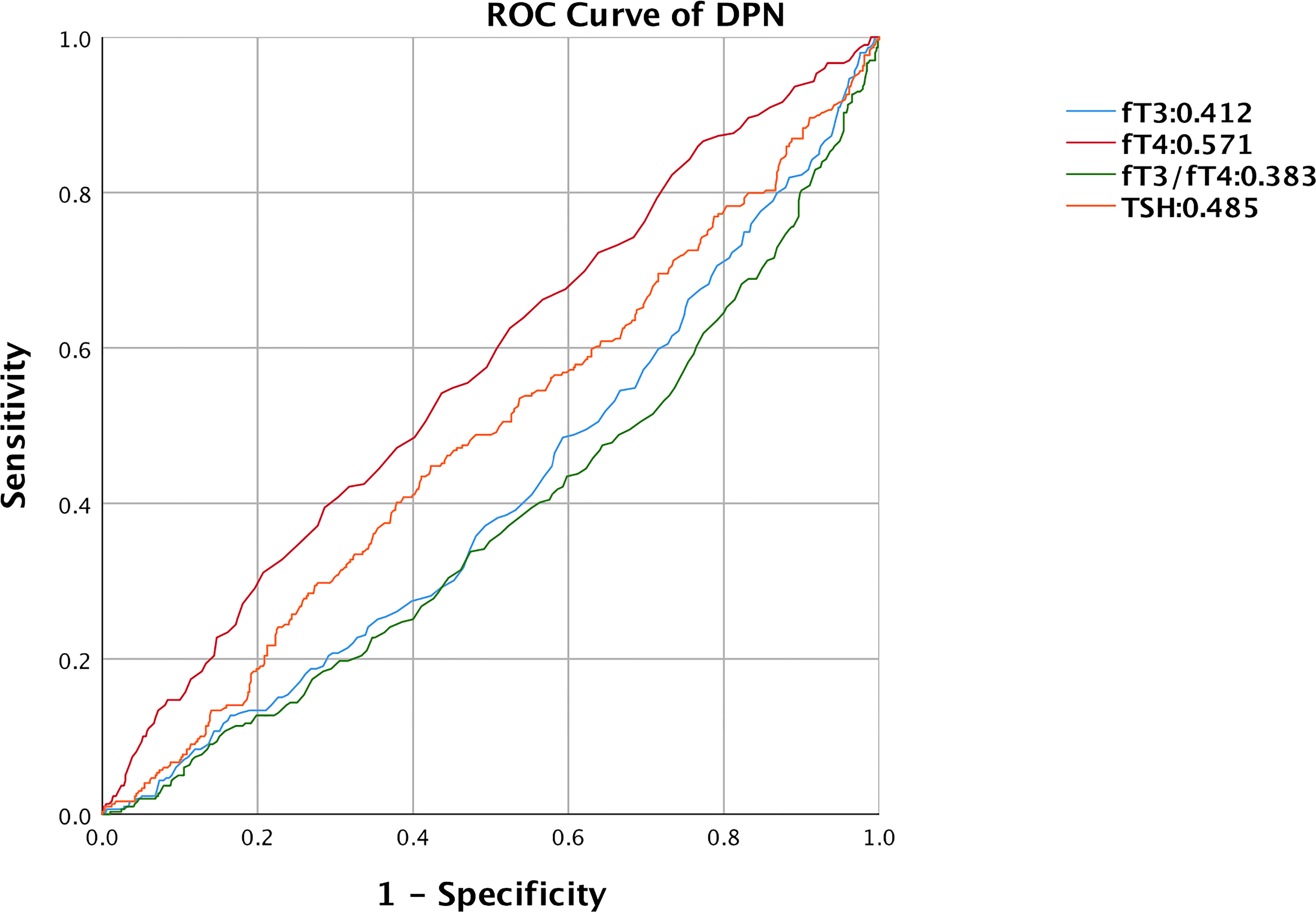
Figure 3 ROC curve of thyroid-related hormones predicting DPN in T2DM with euthyroid. ROC, receiver operating characteristic; DPN, diabetic peripheral neuropathy; fT3, free triiodothyronine1; fT4, free thyroxine; TSH, thyroid stimulating hormone.
Discussion
This cross-sectional study included 869 T2DM patients. We explored the correlation between the prevalence of DPN and CA and fT3, fT4, fT3/fT4, and TSH in T2DM patients with normal thyroid function. Significantly fewer people in the higher fT3 or fT3/fT4 tertiles had DPN (P<0.001). However, the prevalence of DPN increased with increasing fT4 tertiles (P<0.01). After adjustment for confounding factors, fT3 and the fT3/fT4 ratio was negatively associated with DPN (P < 0.05). Overall, fT3 performed the best in assessing the correlation of thyroid hormones with the prevalence of DPN, followed by fT3/fT4 ratio and fT4. Interestingly, we found DPN is more associated with thyroid hormone (TH) levels than with TSH levels.
DPN is a common microvascular complication of diabetes leading to lower limb amputations, neuropathic pain, and increased mortality (27). Both T2DM and obesity exacerbate the inflammatory response, leading to increased serum concentrations of inflammatory biomarkers such as C-reactive protein (28–30). These inflammatory biomarkers diminish the autonomic function and promote the development and progression of neuropathy (31). This may explain why LDL-c is a risk factor for DPN. Yang et al. demonstrated a linear relationship between T2DM and patients with HbA1c > 7.0% and an increased risk of DPN (32). This is because hyperglycemia and insulin resistance are essential for the development of DPN (27, 33, 34). In this study, DBP was found to be independently associated with the risk of developing DPN. The exact mechanism is unclear, and possibly due to a combination of reduced neural blood flow, slow nerve conduction, axonal atrophy, and thinning of myelinated fibers as a result of elevated blood pressure (35–37). The use of antihypertensive drugs may reverse these effects, leading to the annihilation of the risk of DPN. Several studies have shown that the prevalence of DPN increases with the duration of disease in T2DM, suggesting that we should screen for DPN early in the T2DM population (38–40). Patients with poor glycemic control may develop insulin neuritis characterized by acute severe distal limb pain, peripheral nerve fiber damage, and autonomic dysfunction (41, 42). Although rare, these patients are more likely to develop pain or autonomic neuropathy (42).
CA manifests as plaque formation and stenosis of the carotid artery and is a common macrovascular complication of T2DM, the prevention and treatment of which are of critical importance because of its high lethality (43). Some studies have demonstrated that smoking promotes CA by increasing the intima-medial wall thickness of the carotid artery (44). In addition, diabetics are at high risk for arterial atherosclerosis (45) because the arterial walls become less pliable and even harden with age. Plaque composed of cholesterol, fat, calcium, and fibrous tissue accumulates, causing blood vessels to narrow and promoting atherosclerosis (46, 47). Zhou et al. identified a negative correlation between fT3 and carotid intima-media thickness (17). Our study provides evidence to confirm their findings.
The mechanisms concerning fT3 and DPN pathogenesis are unclear. Some investigators have suggested that degeneration of microvascular endothelial cells and peripheral endothelial cells is one of the characteristic changes of DPN (48–50). In addition, the decrease in NO, which can relax blood vessels, is associated with endothelial dysfunction (51, 52). Some studies have reported that T3 mediates the production of endothelial NO (53). Therefore, T3 may reduce the occurrence of DPN by protecting the endothelial cells of microvessels. In addition, the natural metabolite 3,5-diiodothyronine, generated by the deiodination pathway of T3, could reverse the process of DPN by regulating the expression of Sirtuin 1 protein (54, 55). Previous studies have shown that fT3 level was negatively associated with risk of abnormal nerve conduction (56). Meanwhile, Li et al. demonstrated a negative association of fT3 with DPN in T2DM patients with normal thyroid function (57). This is consistent with our findings.
FT4 is deiodinated by three deiodinases to form the more active fT3, which exerts potent biological effects on target organs and tissues (58). Type II deiodinase (DIO2) is the most potent in converting T3, and fT3/fT4 is an indicator of DIO2 activity (59). Intracellular activation of thyroid hormone via DIO2 attenuates cellular dependence on aerobic glycolysis, decreases ROS production and thus minimizes oxidative stress (60). Therefore, decreased DIO2 activity and fT3 conversion lead to increased oxidative stress, which in turn promotes an inflammatory response. Further, prolonged exposure to a hyperglycemic environment promotes oxidative stress (61). This suggests that T2DM patients have decreased DIO2 activity, increased fT4, decreased fT3 production, and enhanced oxidative stress and inflammatory responses, which promote the development of DPN (61–63). Foremost, fT4 level was an independent risk factor for DPN (OR:3.376, 95% CI: 1.691,8.256) in our study. Hu et al. showed that fT4 was negatively correlated with DPN (OR:0.800) (64). This is contrary to our findings. The possible reasons include their smaller sample size, only containing 248 patients with T2DM, and group variability, living environment may have played a role.
When subclinical hypothyroidism occurs, it can exacerbate abnormalities in glucolipid metabolism and significantly exacerbate DPN due to TSH receptor palmitoylation-induced oxidative stress and apoptosis in Schwann’s cells (65). A cross-sectional study from China also demonstrated that TSH levels were independently associated with DPN in the T2DM population in patients with subclinical hypothyroidism (19). However, our study found that this correlation disappeared when the patient’s thyroid function was at normal levels. Does this mean that we should control TSH levels in patients with DPN? In addition, TSH can exacerbate atherosclerosis by promoting macrophage inflammation in plaques (66). Several studies have found a positive correlation between TSH and carotid intima-media thickness in patients with normal thyroid function (67, 68). Interestingly, we did not find this correlation in patients with T2DM. As we all know, hyperglycemia, obesity, and dyslipidemia can promote inflammation and exacerbate CA. The exact mechanism is unclear, and more animal studies are needed to explore it.
As we all know, early detection, early diagnosis, and early treatment can stop and delay the development of the disease and help patients return to health as soon as possible. If the high-risk group of DPN can be detected in advance to help patients diagnose as well as treat them earlier, the development of organic diseases such as gangrene can be reduced or avoided. For people with T2DM, regular monitoring of glycosylated hemoglobin to regulate blood glucose is essential. We can have the treating physician screen the patient for glycosylated hemoglobin along with thyroid function. Reducing the incidence of DPN by controlling fT4 at low normal levels or fT3 at high normal levels after thyroid disease has been ruled out as the cause of the abnormal thyroid function.
In this study, several thyroid-related hormones (fT3, fT4, fT3/fT4, TSH) were evaluated for their association with DPN or CA. Previous research often examined only one of these hormones in relation to the thyroid. One limitation of our study was its a small sample size, which could have led to some errors in the results. Furthermore, it was a single-center cross-sectional study, so the findings cannot be directly attributed to a specific cause. Therefore, further multicenter cohort studies are urgently required to support our conclusions.
Conclusions
Individuals with a low fT3 level and fT3/fT4 ratio in T2DM with normal thyroid function and those with high fT4 levels were more likely to develop DPN. Considering that elevated fT4 is a risk factor for DPN, even in the euthyroid range, early control of fT4 levels or redefinition of its reference range (0.78-1.15 ng/dL) in the T2DM population is beneficial for the prevention of DPN. And fT3 should be early controlled at high normal levels (2.64-3.78 pg/mL).
Data availability statement
The original contributions presented in the study are included in the article/supplementary material. Further inquiries can be directed to the corresponding author/s.
Ethics statement
The studies involving human participants were reviewed and approved by the ethics committee of the Affiliated Hospital of Southwest Medical University (ethical approval code: 2018017). The patients/participants provided their written informed consent to participate in this study.
Author contributions
JL, XX, and YQ made equal contributions to this work, performing the statistical analysis, interpreting the results, and writing the paper. JG reviewed the data, and QW proposed the ideas. All authors contributed to the article and approved the submitted version.
Funding
The Ministry of Science and Technology of China provided funding for this study through grants 2016YFC0901200.
Acknowledgments
Thanks to Yao, Liu, Linlin, Hang and Hongya, Wang for their help in clinical data analysis.
Conflict of interest
The authors declare that the research was conducted in the absence of any commercial or financial relationships that could be construed as a potential conflict of interest.
Publisher’s note
All claims expressed in this article are solely those of the authors and do not necessarily represent those of their affiliated organizations, or those of the publisher, the editors and the reviewers. Any product that may be evaluated in this article, or claim that may be made by its manufacturer, is not guaranteed or endorsed by the publisher.
References
1. Mukhtar Ya’U YU, Galalain AM. A modern overview on diabetes mellitus: A chronic endocrine disorder. European Journal of Biology (2020) 5:1–14. doi: 10.47672/ejb.409
2. Sun H, Saeedi P, Karuranga S, Pinkepank M, Ogurtsova K, Duncan BB, et al. Idf diabetes atlas: Global, regional and country-level diabetes prevalence estimates for 2021 and projections for 2045. Diabetes Res Clin Pract (2021) 183:109119. doi: 10.1016/j.diabres.2021.109119
3. World Health Organization. Diabetes. (2021). Available at: https://www.who.int/news-room/fact-sheets/detail/diabetes
4. Zheng Y, Ley SH, Hu FB. Global aetiology and epidemiology of type 2 diabetes mellitus and its complications. Nat Rev Endocrinol (2018) 14(2):88–98. doi: 10.1038/nrendo.2017.151
5. Chatterjee S, Khunti K, Davies MJ. Type 2 diabetes. Lancet (2017) 389(10085):2239–51. doi: 10.1016/S0140-6736(17)30058-2
6. Adili F, Larijani B, Haghighatpanah M. Diabetic patients: Psychological aspects. Ann New York Acad Sci (2006) 1084:329–49. doi: 10.1196/annals.1372.016
7. Polonsky WH. Emotional and quality-of-Life aspects of diabetes management. Curr Diabetes Rep (2002) 2(2):153–9. doi: 10.1007/s11892-002-0075-5
8. Bhat NA, M KP, Chaturvedi SK. Psychological aspects of diabetes. EMJ Diabetes (2020) 8(1):90–8. doi: 10.33590/emjdiabet/20-00174
9. Rahman S, Rahman T, Ismail AA-S, Rashid ARA. Diabetes-associated macrovasculopathy: Pathophysiology and pathogenesis. Diabetes Obes Metab (2007) 9(6):767–80. doi: 10.1111/j.1463-1326.2006.00655.x
10. Sobel BE, Schneider DJ. Cardiovascular complications in diabetes mellitus. Curr Opin In Pharmacol (2005) 5(2):143–8. doi: 10.1016/j.coph.2005.01.002
11. Biondi B, Kahaly GJ, Robertson RP. Thyroid dysfunction and diabetes mellitus: Two closely associated disorders. Endoc Rev (2019) 40(3):789–824. doi: 10.1210/er.2018-00163
12. Wang C. The relationship between type 2 diabetes mellitus and related thyroid diseases. J Diabetes Res (2013) 2013:390534. doi: 10.1155/2013/390534
13. Spira D, Buchmann N, Dörr M, Markus MRP, Nauck M, Schipf S, et al. Association of thyroid function with insulin resistance: Data from two population-based studies. Eur Thyroid J (2022) 11(2). doi: 10.1530/ETJ-21-0063
14. Rong F, Dai H, Wu Y, Li J, Liu G, Chen H, et al. Association between thyroid dysfunction and type 2 diabetes: A meta-analysis of prospective observational studies. BMC Med (2021) 19(1):257. doi: 10.1186/s12916-021-02121-2
15. Moura Neto A, Parisi MCR, Tambascia MA, Pavin EJ, Alegre SM, Zantut-Wittmann DE. Relationship of thyroid hormone levels and cardiovascular events in patients with type 2 diabetes. Endocrine (2014) 45(1):84–91. doi: 10.1007/s12020-013-9938-6
16. Tatar E, Kircelli F, Asci G, Carrero JJ, Gungor O, Demirci MS, et al. Associations of triiodothyronine levels with carotid atherosclerosis and arterial stiffness in hemodialysis patients. Clin J Am Soc Nephrol CJASN (2011) 6(9):2240–6. doi: 10.2215/CJN.02540311
17. Zhou Y, Zhao L, Wang T, Hong J, Zhang J, Xu B, et al. Free triiodothyronine concentrations are inversely associated with elevated carotid intima-media thickness in middle-aged and elderly Chinese population. J Atheroscl Thromb (2016) 23(2):216–24. doi: 10.5551/jat.30338
18. Chen Y, Zhang W, Wang N, Wang Y, Wang C, Wan H, et al. Thyroid parameters and kidney disorder in type 2 diabetes: Results from the metal study. J Diabetes Res (2020) 2020:4798947. doi: 10.1155/2020/4798947
19. Zhao W, Zeng H, Zhang X, Liu F, Pan J, Zhao J, et al. A high thyroid stimulating hormone level is associated with diabetic peripheral neuropathy in type 2 diabetes patients. Diabetes Res Clin Pract (2016) 115:122–9. doi: 10.1016/j.diabres.2016.01.018
20. American Diabetes Association. 2. classification and diagnosis of diabetes. Diabetes Care (2019) 42(Suppl 1):S13–28. doi: 10.2337/dc19-S002
21. World Medical Association. World medical association declaration of Helsinki: Ethical principles for medical research involving human subjects. JAMA (2013) 310(20):2191–4. doi: 10.1001/jama.2013.281053
22. Katakami N, Matsuoka T-A, Shimomura I. Clinical utility of carotid ultrasonography: Application for the management of patients with diabetes. J Diabetes Invest (2019) 10(4):883–98. doi: 10.1111/jdi.13042
23. Scoutt LM, Gunabushanam G. Carotid ultrasound. Radiol Clinics North America (2019) 57(3):501–18. doi: 10.1016/j.rcl.2019.01.008
24. Stein JH, Korcarz CE, Hurst RT, Lonn E, Kendall CB, Mohler ER, et al. Use of carotid ultrasound to identify subclinical vascular disease and evaluate cardiovascular disease risk: A consensus statement from the American society of echocardiography carotid intima-media thickness task force. endorsed by the society for vascular medicine. J Am Soc Echocardiogr Off Publ Am Soc Echocardiogr (2008) 21(2):93–111. doi: 10.1016/j.echo.2007.11.011
25. Johri AM, Nambi V, Naqvi TZ, Feinstein SB, Kim ESH, Park MM, et al. Recommendations for the assessment of carotid arterial plaque by ultrasound for the characterization of atherosclerosis and evaluation of cardiovascular risk: From the American society of echocardiography. J Am Soc Echocardiogr Off Publ Am Soc Echocardiogr (2020) 33(8):917–33. doi: 10.1016/j.echo.2020.04.021
26. Tesfaye S, Boulton AJM, Dyck PJ, Freeman R, Horowitz M, Kempler P, et al. Diabetic neuropathies: Update on definitions, diagnostic criteria, estimation of severity, and treatments. Diabetes Care (2010) 33(10):2285–93. doi: 10.2337/dc10-1303
27. Sloan G, Selvarajah D, Tesfaye S. Pathogenesis, diagnosis and clinical management of diabetic sensorimotor peripheral neuropathy. Nat Rev Endocrinol (2021) 17(7):400–20. doi: 10.1038/s41574-021-00496-z
28. Cruz NG, Sousa LP, Sousa MO, Pietrani NT, Fernandes AP, Gomes KB. The linkage between inflammation and type 2 diabetes mellitus. Diabetes Res Clin Pract (2013) 99(2):85–92. doi: 10.1016/j.diabres.2012.09.003
29. Pradhan AD, Manson JE, Rifai N, Buring JE, Ridker PM. C-reactive protein, interleukin 6, and risk of developing type 2 diabetes mellitus. JAMA (2001) 286(3):327–34. doi: 10.1001/jama.286.3.327
30. Duncan BB, Schmidt MI, Pankow JS, Ballantyne CM, Couper D, Vigo A, et al. Low-grade systemic inflammation and the development of type 2 diabetes: The atherosclerosis risk in communities study. Diabetes (2003) 52(7):1799–805. doi: 10.2337/diabetes.52.7.1799
31. Herder C, Kannenberg JM, Huth C, Carstensen-Kirberg M, Rathmann W, Koenig W, et al. Proinflammatory cytokines predict the incidence and progression of distal sensorimotor polyneuropathy: Kora F4/Ff4 study. Diabetes Care (2017) 40(4):569–76. doi: 10.2337/dc16-2259
32. Yang C-P, Lin C-C, Li C-I, Liu C-S, Lin W-Y, Hwang K-L, et al. Cardiovascular risk factors increase the risks of diabetic peripheral neuropathy in patients with type 2 diabetes mellitus: The Taiwan diabetes study. Medicine (2015) 94(42):e1783. doi: 10.1097/MD.0000000000001783
33. Van Dam PS, Cotter MA, Bravenboer B, Cameron NE. Pathogenesis of diabetic neuropathy: Focus on neurovascular mechanisms. Eur J Pharmacol (2013) 719(1-3):180–6. doi: 10.1016/j.ejphar.2013.07.017
34. Stino AM, Rumora AE, Kim B, Feldman EL. Evolving concepts on the role of dyslipidemia, bioenergetics, and inflammation in the pathogenesis and treatment of diabetic peripheral neuropathy. J Peripheral Nervous System JPNS (2020) 25(2):76–84. doi: 10.1111/jns.12387
35. Braffett BH, Gubitosi-Klug RA, Albers JW, Feldman EL, Martin CL, White NH, et al. Risk factors for diabetic peripheral neuropathy and cardiovascular autonomic neuropathy in the diabetes control and complications Trial/Epidemiology of diabetes interventions and complications (Dcct/Edic) study. Diabetes (2020) 69(5):1000–10. doi: 10.2337/db19-1046
36. Li L, Yang Y, Bai J, Zhang Y, Yang H, Zhang Y, et al. Impaired vascular endothelial function is associated with peripheral neuropathy in patients with type 2 diabetes. Diabetes Metab Syndrome Obes Targets Ther (2022) 15:1437–49. doi: 10.2147/DMSO.S352316
37. Gregory JA, Jolivalt CG, Goor J, Mizisin AP, Calcutt NA. Hypertension-induced peripheral neuropathy and the combined effects of hypertension and diabetes on nerve structure and function in rats. Acta Neuropathol (2012) 124(4):561–73. doi: 10.1007/s00401-012-1012-6
38. Cabezas-Cerrato J. The prevalence of clinical diabetic polyneuropathy in Spain: A study in primary care and hospital clinic groups. neuropathy Spanish study group of the Spanish diabetes society (Sds). Diabetologia (1998) 41(11):1263–9. doi: 10.1007/s001250051063
39. Bansal D, Gudala K, Muthyala H, Esam HP, Nayakallu R, Bhansali A. Prevalence and risk factors of development of peripheral diabetic neuropathy in type 2 diabetes mellitus in a tertiary care setting. J Diabetes Invest (2014) 5(6):714–21. doi: 10.1111/jdi.12223
40. Shehab D, Al-Jarallah K, Mojiminiyi OA, Al Mohamedy H, Abdella NA. Does vitamin d deficiency play a role in peripheral neuropathy in type 2 diabetes? Diabetic Med J Br Diabetic Assoc (2012) 29(1):43–9. doi: 10.1111/j.1464-5491.2011.03510.x
41. Knopp M, Srikantha M, Rajabally YA. Insulin neuritis and diabetic cachectic neuropathy: A review. Curr Diabetes Rev (2013) 9(3):267–74. doi: 10.2174/1573399811309030007
42. Gibbons CH, Freeman R. Treatment-induced neuropathy of diabetes: An acute, iatrogenic complication of diabetes. Brain J Neurol (2015) 138(Pt 1):43–52. doi: 10.1093/brain/awu307
43. Klimontov VV, Koroleva EA, Khapaev RS, Korbut AI, Lykov AP. Carotid artery disease in subjects with type 2 diabetes: Risk factors and biomarkers. J Clin Med (2021) 11(1):72–91. doi: 10.3390/jcm11010072
44. Diez-Roux AV, Nieto FJ, Comstock GW, Howard G, Szklo M. The relationship of active and passive smoking to carotid atherosclerosis 12-14 years later. Prev Med (1995) 24(1):48–55. doi: 10.1006/pmed.1995.1007
45. Howard G, Wagenknecht LE, Burke GL, Diez-Roux A, Evans GW, McGovern P, et al. Cigarette smoking and progression of atherosclerosis: The atherosclerosis risk in communities (Aric) study. JAMA (1998) 279(2):119–24. doi: 10.1001/jama.279.2.119
46. Kohn JC, Lampi MC, Reinhart-King CA. Age-related vascular stiffening: Causes and consequences. Front In Genet (2015) 6:112. doi: 10.3389/fgene.2015.00112
48. Giannini C, Dyck PJ. Ultrastructural morphometric abnormalities of sural nerve endoneurial microvessels in diabetes mellitus. Ann Neurol (1994) 36(3):408–15. doi: 10.1002/ana.410360312
49. Malik RA, Newrick PG, Sharma AK, Jennings A, Ah-See AK, Mayhew TM, et al. Microangiopathy in human diabetic neuropathy: Relationship between capillary abnormalities and the severity of neuropathy. Diabetologia (1989) 32(2):92–102. doi: 10.1007/BF00505180
50. Horton WB, Barrett EJ. Microvascular dysfunction in diabetes mellitus and cardiometabolic disease. Endoc Rev (2021) 42(1):29–55. doi: 10.1210/endrev/bnaa025
51. Michel JB. [Role of endothelial nitric oxide in the regulation of the vasomotor system]. Pathologie-biologie (1998) 46(3):181–9.
52. Cyr AR, Huckaby LV, Shiva SS, Zuckerbraun BS. Nitric oxide and endothelial dysfunction. Crit Care Clinics (2020) 36(2):307–21. doi: 10.1016/j.ccc.2019.12.009
53. Gaynullina DK, Schubert R, Tarasova OS. Changes in endothelial nitric oxide production in systemic vessels during early ontogenesis-a key mechanism for the perinatal adaptation of the circulatory system. Int J Mol Sci (2019) 20(6):1421–33. doi: 10.3390/ijms20061421
54. Shang G, Gao P, Zhao Z, Chen Q, Jiang T, Zhang N, et al. 3,5-Diiodo-L-Thyronine ameliorates diabetic nephropathy in streptozotocin-induced diabetic rats. Biochim Et Biophys Acta (2013) 1832(5):674–84. doi: 10.1016/j.bbadis.2013.01.023
55. Chandrasekaran K, Salimian M, Konduru SR, Choi J, Kumar P, Long A, et al. Overexpression of sirtuin 1 protein in neurons prevents and reverses experimental diabetic neuropathy. Brain J Neurol (2019) 142(12):3737–52. doi: 10.1093/brain/awz324
56. Zhu F-F, Yang L-Z. The association between the levels of thyroid hormones and peripheral nerve conduction in patients with type 2 diabetes mellitus. Exp Clin Endocrinol Diabetes Off Journal German Soc Endocrinol [and] German Diabetes Assoc (2018) 126(8):493–504. doi: 10.1055/a-0635-0826
57. Li M-F, Ke J-F, Li S, Wang J-W, Zhu Z-H, Li J-B. Serum free triiodothyronine is inversely associated with diabetic peripheral neuropathy but not with carotid atherosclerotic lesions in euthyroid patients with type 2 diabetes. Diabetol Metab Syndrome (2021) 13(1):142. doi: 10.1186/s13098-021-00760-2
58. Köhrle J. The deiodinase family: Selenoenzymes regulating thyroid hormone availability and action. Cell Mol Life Sci CMLS (2000) 57(13-14):1853–63. doi: 10.1007/PL00000667
59. Brent GA. Mechanisms of thyroid hormone action. J Clin Invest (2012) 122(9):3035–43. doi: 10.1172/JCI60047
60. Sagliocchi S, Cicatiello AG, Di Cicco E, Ambrosio R, Miro C, Di Girolamo D, et al. The thyroid hormone activating enzyme, type 2 deiodinase, induces myogenic differentiation by regulating mitochondrial metabolism and reducing oxidative stress. Redox Biol (2019) 24:101228. doi: 10.1016/j.redox.2019.101228
61. Brownlee M. The pathobiology of diabetic complications: A unifying mechanism. Diabetes (2005) 54(6):1615–25. doi: 10.2337/diabetes.54.6.1615
62. Albers JW, Pop-Busui R. Diabetic neuropathy: Mechanisms, emerging treatments, and subtypes. Curr Neurol Neurosci Rep (2014) 14(8):473. doi: 10.1007/s11910-014-0473-5
63. Prasad K. Pathophysiology and medical treatment of carotid artery stenosis. Int J Angiol Off Publ Int Coll Angiol Inc (2015) 24(3):158–72. doi: 10.1055/s-0035-1554911
64. Hu Y, Hu Z, Tang W, Liu W, Wu X, Pan C. Association of thyroid hormone levels with microvascular complications in euthyroid type 2 diabetes mellitus patients. Diabetes Metab Syndrome Obes Targets Ther (2022) 15:2467–77. doi: 10.2147/DMSO.S354872
65. Fan J, Pan Q, Gao Q, Li W, Xiao F, Guo L. Tsh combined with tshr aggravates diabetic peripheral neuropathy by promoting oxidative stress and apoptosis in schwann cells. Oxid Med Cell Longevity (2021) 2021:2482453. doi: 10.1155/2021/2482453
66. Yang C, Lu M, Chen W, He Z, Hou X, Feng M, et al. Thyrotropin aggravates atherosclerosis by promoting macrophage inflammation in plaques. J Exp Med (2019) 216(5):1182–98. doi: 10.1084/jem.20181473
67. Takamura N, Akilzhanova A, Hayashida N, Kadota K, Yamasaki H, Usa T, et al. Thyroid function is associated with carotid intima-media thickness in euthyroid subjects. Atherosclerosis (2009) 204(2):e77–81. doi: 10.1016/j.atherosclerosis.2008.09.022
68. Sakamaki K, Tsunekawa K, Ishiyama N, Kudo M, Ando K, Akuzawa M, et al. Association between high normal-range thyrotropin concentration and carotid intima-media thickness in euthyroid premenopausal, perimenopausal and postmenopausal women. Maturitas (2021) 144:29–36. doi: 10.1016/j.maturitas.2020.10.022
Keywords: free triiodothyronine, free thyroxine, thyroid-stimulating hormone, type 2 diabetes mellitus, diabetic peripheral neuropathy, carotid atherosclerosis
Citation: Lin J, Xiang X, Qin Y, Gui J and Wan Q (2022) Correlation of thyroid-related hormones with vascular complications in type 2 diabetes patients with euthyroid. Front. Endocrinol. 13:1037969. doi: 10.3389/fendo.2022.1037969
Received: 06 September 2022; Accepted: 26 October 2022;
Published: 18 November 2022.
Edited by:
Silvia Martina Ferrari, University of Pisa, ItalyReviewed by:
Armando Patrizio, University of Pisa, ItalyValeria Mazzi, Università degli Studi di Pisa, Italy
Copyright © 2022 Lin, Xiang, Qin, Gui and Wan. This is an open-access article distributed under the terms of the Creative Commons Attribution License (CC BY). The use, distribution or reproduction in other forums is permitted, provided the original author(s) and the copyright owner(s) are credited and that the original publication in this journal is cited, in accordance with accepted academic practice. No use, distribution or reproduction is permitted which does not comply with these terms.
*Correspondence: Qin Wan, d2FucWluMzYwQHN3bXUuZWR1LmNu
†These authors have contributed equally to this work and share the first authorship