- 1Department of Traumatic Orthopedics, Changhai Hospital, Naval Medical University, Shanghai, China
- 2National Key Laboratory of Medical Immunology and Institute of Immunology, Institute of Immunology, Naval Medical University, Shanghai, China
- 3Department of Traumatic Orthopedics, the First Affiliated Hospital of Naval Medical University, Naval Medical University, Shanghai, China
Osteoporosis, characterized by bone mineral density reduction, bone mass loss, increased bone fragility, and propensity to fractures, is a common disease in older individuals and one of the most serious health problems worldwide. The imbalance between osteoblasts and osteoclasts results in the predominance of bone resorption and decreased bone formation. In recent years, it has been found that regular and proper exercise not only helps prevent the occurrence of osteoporosis but also adds benefits to osteoporosis therapy; accordingly, bone homeostasis is closely associated with mechanical stress and the intricate crosstalk between osteoblasts and osteoclasts. In this review, we summarize the mechanisms of exercise on osteoporosis and provide new proposals for the prevention and treatment of osteoporosis.
1. Introduction
Osteoporosis is a metabolic bone disease characterized by the loss of bone mineral density and the deterioration of bone quality via microarchitectural and biomechanical alterations. The bone mass reduction in osteoporosis results in skeletal fragility and increases the fracture risk. Approximately 1.5 million new fractures are reported worldwide each year, resulting in a huge economic burden for the affected families and countries (1).
The cellular and molecular mechanisms underlying osteoporosis are multifactorial, reflecting the complex interactions of the diverse cells in the bone marrow microenvironment. Osteoporosis is considered to be a direct result of the reduction of bone mass and imbalance in bone remodeling. Human bone mass is determined by the intricate bone microenvironment led by the dynamic balance of osteoblasts, osteoclasts, and other cells. Bone remodeling imbalance is responsible for several pathological conditions, including osteosclerosis, osteopetrosis, and osteoporosis. The imbalance within the bone microenvironment is not only closely related to menopause and aging, but is also associated with the occurrence and development of inflammation.
Depending on its primary cause, osteoporosis is divided to primary osteoporosis and secondary osteoporosis. Primary osteoporosis includes postmenopausal osteoporosis, senile osteoporosis, and juvenile osteoporosis, while secondary osteoporosis occurs due to drugs, such as long-term glucocorticoid administration in large doses, long-term limb disuse, or in other diseases.
Current solutions to reduce the incidence of osteoporosis include identifying persons at risk, prescribing long-term pharmaceutical treatment, and reducing the risk of falls. In addition, its prevention strategies include sufficient calcium and vitamin D intake, cessation of tobacco or alcohol consumption, and being physically active at young age to increase and maintain peak bone mass (2). However, many researchers have recently found that exercise is beneficial for preventing and treating osteoporosis (3–5).
2. Bone remodeling and bone microenvironment
Osteoblasts, osteoclasts, and osteocytes are the fundamental cell types in the bone microenvironment, which interact and cooperate with each other on the basis of extracellular communication to coordinate bone remodeling (6). The precursors of osteoblasts originate from bone marrow mesenchymal stem cells (BMSCs) (7, 8). In the period of bone formation, osteoblast precursors on the bone surface generate calcified matrix, also called mineralized matrix. When osteoclast precursors, which differentiate from bone marrow hematopoietic stem cells, contact with the mineralized matrix, they polarize and fuse to multinucleated giant cells, giving rise to osteoclasts. Osteoclasts form specific domains, including the ruffled border, the sealing zone, and the functional secretory domain, which are important for the function of bone resorption reabsorption (9, 10). Bone resorption is the precondition for the formation of resorption lacuna. Osteoblasts express and secrete two necessary cytokines, including macrophage colony-stimulating factor M-CSF and the ligand for the receptor activator of nuclear factor kB (RANKL). Under the stimulation by the two cytokines, hematopoietic cells differentiate to osteoclasts. Likewise, osteoclasts generate MMP-2, 4, 6, and 7, which are important for recruiting osteoblasts and their subsequent activation (11). Osteocytes differentiate from osteoblasts. Mature osteocytes are rooted in the bone matrix and are connected to each other by stretched dendritic cell extensions through a network formed by canaliculi, called lacunocanalicular network (LCN).
3. Mechanisms of exercise on bone health
Mechanical strain, such as during exercise, is an important physiological factor that regulates bone formation in living organisms (12). The mechanism is based on the function of osteocytes, which perceive, transform, and transmit mechanical loading. Osteocytes are the main bone cells capable of transforming mechanical stimuli into intercellular signals through the exceedingly specialized LCN. In the state of exercise, osteocytes are able to sense variation in mechanical stress, respond and send signals to the surrounding cells, and regulate functions of osteoblasts, osteoclasts, and extracellular matrix (ECM) of the bone microenvironment (13). Transduction of the mechanical signals to other cells in the microenvironment leads to the upregulation of bone turnover and subsequent bone deposition (14). Other cells, such as osteoblasts, can also sense mechanical stimuli (15, 16).
Osteocytes are able to respond to physical stimulation directly by making use of various mechanosensors, such as Wnt signaling components (17), integrins sensing extracellular matrix stress (18, 19), connexins forming intercellular junctions (20, 21), and purinergic receptors (22). The function of osteocytes in response to mechanical stimulation from exercise is also regulated indirectly by extracellular cytokines ranging from RANKL/OPG to sclerostin (23).
Here, we gathered and curated information related to mechanical signaling mechanisms (Figure 1).
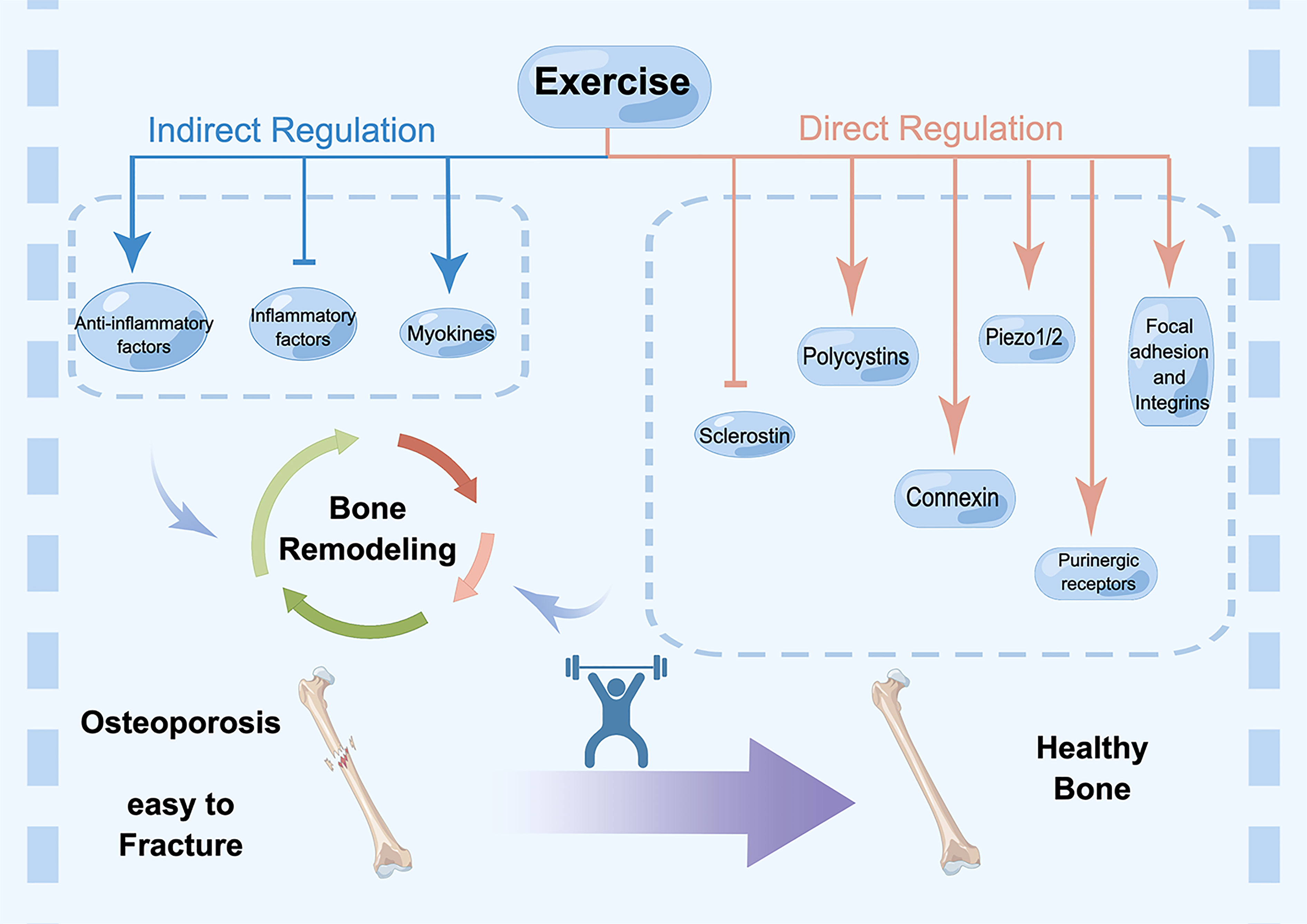
Figure 1 Direct and indirect regulation mechanisms of exercise for osteoporosis. Cell surface receptors are the starting point for understanding the regulatory effects of mechanical loading, which include focal adhesions, integrins, purinergic receptors, connexin, polycystins, Piezos, Wnt signaling and sclerostin. In addition, exercise-induced regulation of bone environment also occurs through cytokines, including inflammatory factors and myokines, such as irisin. These factors affect bone remodeling collectively. Exercise promotes bone mass and sustain healthy bone, while lacking exercise results in osteoporosis and fracture.
3.1. Direct regulation of cell surface receptors
Cell surface receptors are vital signal‐sensing molecules that respond to extracellular environmental changes. This phenomenon is the starting point for understanding the regulatory effects of mechanical loading. Many proteins on the cell surface have been reported to act as mechanical transducers in bone cells, which include focal adhesions (24), integrins (25), purinergic receptors (22), connexin (20, 21), polycystins (26, 27), Piezos (28, 29), Wnt signaling and sclerostin.
3.1.1. Focal adhesions and integrins
Focal adhesion complex is the most important component of integrin-based adhesion complex, which transduces mechanical stress from ECM into cytoplasm (18). Integrin proteins include α and β subunits, where integrin α senses different signals out of the cells, while integrin β delivers signal pathways in the cells (19). The integrin family consists of 24 members, which were discovered in the past two decades. Different tissues express different types of integrin, and proteins in the ECM help determine which type of integrin these tissues express. Integrins are expressed in various bone cells, such as osteoblasts, osteocytes, and osteoclasts.
During exercise, focal adhesion enables osteocytes to sense mechanical forces. With the help from other different adhesion proteins, osteocytes respond to different stimuli in the context of exercise (19). Osteocytes express β1 and β3 subunits of integrins. Integrin β1 is related to α1 to α5 subunits, while integrin β3 only corresponds to αv. Using an Itga5 conditional gene knockout mouse model, it has been shown that integrin α5 plays an important role in regulating the anabolic response of bone to mechanical loading. Integrin α5 inhibits osteocytes apoptosis, increases endosteal osteoblasts and bone formation, and reduces osteoclasts and bone resorption. Moreover, integrin α5 increases load-induced Cx43 function and decreases the production and release of PGE2, which results in the upregulation of sclerostin and reduction of β-catenin. For bone loss, integrin α5 has the potential of becoming a new therapeutic target, especially in older individuals with reduced mechanical sensitivity (30).
3.1.2. Connexins and gap junctions
In addition to integrins and focal adhesion, gap junctions and hemichannels, which are formed by connexins, play important roles in regulating cell-to-cell communication in the bone microenvironment (21). Six connexin molecules, either same or different types, compose a connexon, and two connexons form a gap junction on the surface of adjacent osteocytes. When connexons are only found on one side of a cell, they form hemichannels. Hemichannels and gap junctions are necessary in cell-to-cell messenger exchange (21). Gap junctions are most abundant in osteoblasts and osteocytes, and because connexin forms gap junctions, the expression of connexin family members, including Cx37, Cx40, Cx43, and Cx45, is regulated by the bone microenvironment (21). Under mechanical stimulus of exercise, Cx43 is expressed in osteocytes to orchestrate bone turnover and remodeling (21).
A study in mice with dominant-negative Cx43 mutants in osteocytes has demonstrated that osteocytic Cx43 is essential for anabolic function of bone in response to mechanical loading (31). Mechanical loading of tibial bone upregulated cortical bone mass and mechanical properties in control mice through increased PGE2, endosteal osteoblast activity, and reduced sclerostin. In contrast, in gap junction–impaired Δ130–136 mice, these bone anabolic responses were all impeded and endosteal osteoclast activity was enhanced (31).
3.1.3. Purinergic receptors
Purinergic receptors also participate in the regulation of bone homeostasis by exercise because they can be activated by ATP as well as ADP, which are produced in various types of exercise. It has been reported that ATP is concentrated inside the cell, while extracellular ATP levels are only upregulated during cell lysis or in response to robust mechanical stimuli such as in high-intensity exercise (32). By using the MLO-Y4 osteocyte model, it has been demonstrated that osteocytes secrete ATP in response to mechanical stimulation of exercise in a dose-dependent manner (32).
Purinergic receptors were first classified into three types: G-protein–coupled receptors (GPCRs) adenosine receptors (P1), P2X ion channels, and P2Y G-protein–coupled receptors (22, 33). P2X and P2Y receptors have been detected in osteoblasts, osteoclasts, and osteocytes (20). In regulation of the bone environment in exercise, ATP is released, and P2 receptor and its downstream signaling are vital modulators. It has been reported that P2X7 receptor activation increases mineralization of osteoblasts (34). Furthermore, P2X7 knockout mice express bone mass reduction (34), and defective osteogenesis, which leads to wider calvarial sutures (35).
3.1.4. Polycystins (PCs)
Polycystin-1 (PC-1) and polycystin-2 (PC-2) were first identified in renal primary cilia cells. By conjugating transiently, they form mechanosensitive receptor channel complexes, TRPP channels, and act as mechanosensitive channels. It is known that defects in PC-1 and PC-2 result in autosomal dominant polycystic kidney disease. However, the full spectrum of PC1 and PC2 effects has been difficult to establish (26, 27, 36–39).
Recently, it has been found that mice with the conditional deletion of PC1 in bone marrow mesenchymal stem cells (BMSCs), osteoblasts, and osteocytes display noteworthy decreases in bone mineral density as a result of the reduced osteoblast-related bone formation in vivo (37, 38). In the same way, the conditional deletion of PC2 in osteoblasts leads to a decrease in trabecular bone volume, bone mineral density, and cortical thickness in vivo (39). PC1, PC2, and TAZ react to the flow shear force, characterized in collaboration to each other, and accelerate osteoblast maturation by activating Runx2 and repressing PPARγ activities (40). Furthermore, human genome-wide association studies (GWAS) have suggested the relationship between PC2 and osteoporosis (41). At present, there is an agonist called triptolide, which can bind to PC2 and rescue Ca2+ signaling to diminish whole cyst formation in renal epithelial cells in vitro (42). Hence, triptolide seems promising in providing a new option for the treatment of osteoporosis, although additional experiments are needed to confirm its effects.
The effects of exercise on PC1/3 have rarely been investigated. Moderate-intensity exercise (20 m/min, 1 h) increased the quantity of PC1/3 in the pituitary gland (43), indicating that PC1/3 might be a possible regulator of bone response to exercise.
3.1.5. Piezo1/2
Piezo proteins (Piezo1 and Piezo2), discovered in 2010, form mechanosensitive cation channels (44). Piezo channels are mechanosensitive ion channels located in the cell membrane and function as key cellular mechanotransducers for converting mechanical stimuli into electrochemical signals. Under mechanical stimuli, these channels are opened to allow cations to cross the membrane, which promotes cellular mechanotransduction to adapt to the microenvironment (45). Recently, some researchers have suggested that those Piezo proteins are important mechanosensitive channels in the bone microenvironment.
PIEZO1 and PIEZO2 show robust expression in primary articular chondrocytes. GsMTx4, a PIEZO-blocking peptide, reduces chondrocyte death by regulating the Ca2+ signaling pathway after mechanical injury, shedding light on cartilage injury therapy (28). Moreover, the Piezo channels are involved in bone disease and play pivotal roles in the transduction of mechanical forces in other bone cells (29, 46).
It has been reported that the Piezo1–YAP1–collagen pathway in osteoblasts is important for the regulation of bone mass in vivo and in vitro (47). Deficiency of Piezo1 in osteoblasts resulted in osteoporosis. In tail suspension assays, the hind limb of mice was unloaded for 8 weeks. The bone mass of these mice was reduced, supporting the hypothesis that PIEZO channels directly sense mechanical loading in skeletal cells. Piezo1 increased collagen II and collagen IX expression by promoting YAP nuclear translocation in osteoblasts. In contrast, in osteoclasts, Piezo1 knockout did not affect bone loss (47).
Li et al. verified the vital role of Piezo1 in sustaining bone homeostasis by regulating the sensitivity of osteocytes and osteoblasts to mechanical loading in vivo and in vitro (46). An in vitro study demonstrated that the activation of Piezo1 by Yoda1 mirrored the raise of Ca2+ influx outcomes of fluid shear stress on osteocytes. In line with the in vitro results, Yoda1 increased the bone mass of the mice (46).
3.1.6. Wnt signaling and sclerostin
Osteocytes sense mechanical loading transmitted to bone and downregulate the expression and secretion of sclerostin (25). Sclerostin competitively binds to the extracellular binding site LRP5/6, conveying an antagonistic effect on the Wnt/β-catenin pathway (48) (Figure 2).
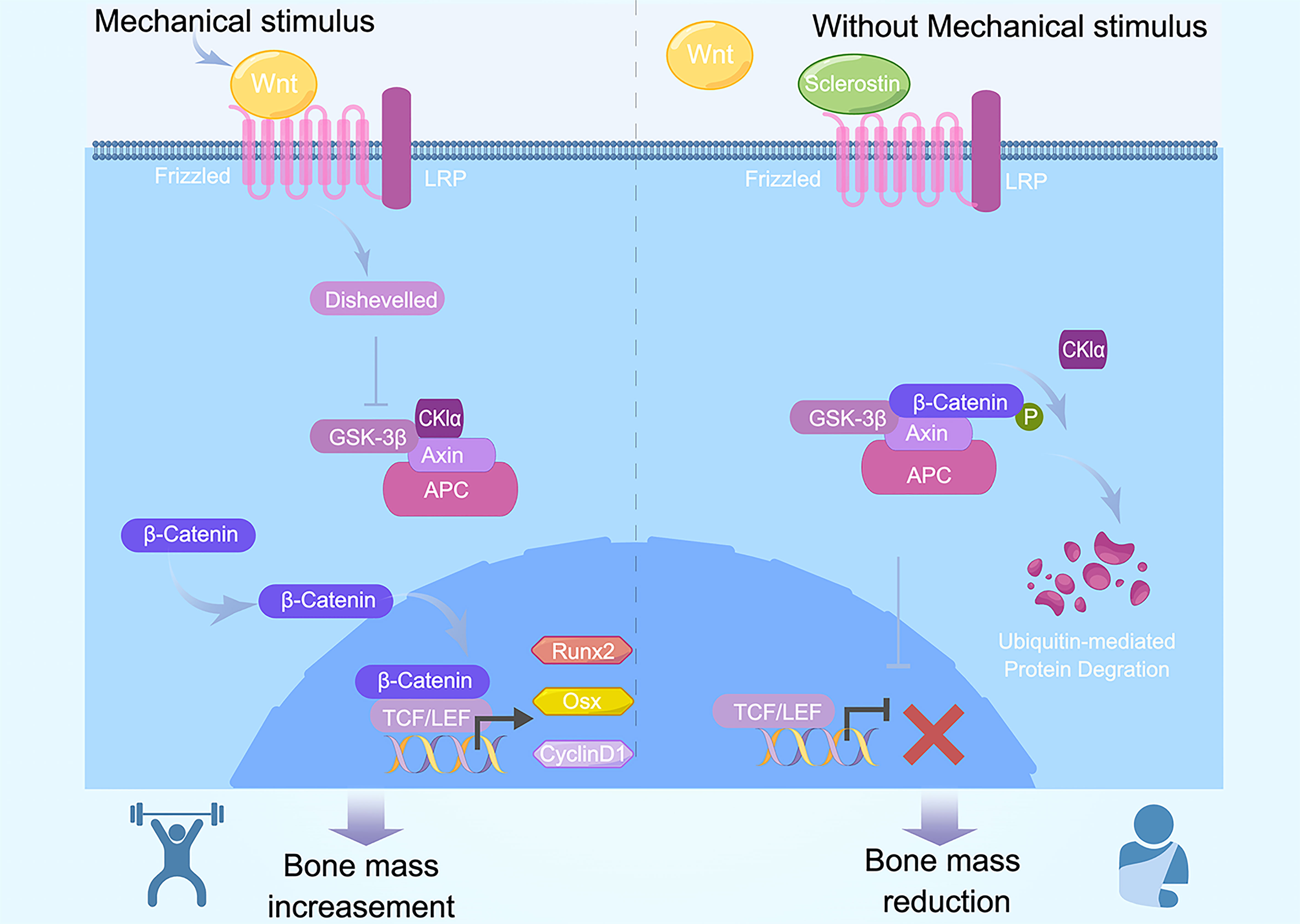
Figure 2 Canonical Wnt signaling and sclerostin in mechanical regulation of exercise. Exercises produce mechanical stimulus and increase Wnt1. Wnt1 binds with LRP5/6, subsequently activate intercellular Dvl, resulting in release of free β-catenin and translocation into nuclei. β-cateniin binds with TEF/LEF, contributes to induction and activation of Runx2, Osx, and cyclinD1. Osteoblast differentiation and bone mass increase is activated by Wnt and inhibited by the Wnt antagonists Frizzled and sclerostin. When without mechanical stimulus bring by exercise, Wnt1 decreased and β-catenin is ubiquitinated and degraded, inducing downregulation in the expression of downstream target gene, bone mass subsequently decreased.
The Wnt/β-catenin pathway is conservative in various organisms. It is important for cell differentiation, growth, apoptosis, and biological functions. It is also necessary for organism growth and development. So far, there are three types of Wnt signaling, including canonical Wnt signaling, non-canonical Wnt/Ca+ signaling, and Wnt/PCP signaling pathway (17). Canonical Wnt signaling is the most important regulator of differentiation from stem cells to osteoblasts and osteocytes.
Wnt signaling components, such as Wnt, β-catenin, GSK-3β, and Axin, play a pivotal role in bone metastasis regulation (17). Wnt1 binds with frizzled family member FZD and LDL receptor–related protein (LRP5/6). Subsequently, they activate intercellular Dvl (Dishevelled), thus forming a complex that destroys another complex that is composed with APC, Axin, GSK-3β, and β-catenin. As a result, released free β-catenin translocates into nuclei and binds with the transcriptional factor T cell factor/lymphoid enhancer factor (TCF/LEF). At last, expression of downstream target genes Runx2, Osx, and cyclinD1 increases. In contrast, without Wnt1, the β-catenin complex is ubiquitinated and degraded, inducing a decrease in the expression of downstream target gene (17). Wnt signaling activation results in bone mass increase (49).
A recent study has reported a new vibration-induced bone-enhancing protein, R-spondin 1 (50). As a Wnt pathway modulator, R-spondin 1 is secreted by young mesenchymal progenitor cells and promotes bone formation in osteoporosis in response to vibration (50).
It has been shown that the Wnt3a/β-catenin pathway in mice with type 2 diabetes mellitus is upregulated after downhill running. Subsequently, osteoblasts differentiation, osteogenic capacity, and bone morphological structure are enhanced. In a word, downhill running reverses osteoporosis by activating Wnt signaling in osteoblasts (51).
In another study, mechanical loading of the ulna decreased Sost transcriptional levels in wild-type mice, while HLU enhanced sclerostin expression (23). The phenomenon was in parallel with the increased level of sclerostin detected in human serum after prolonged bed rest and immobilization.
In summary, these findings suggest that mechanically responsive activation of receptors and iron channels on the surface of osteocytes, osteoblasts, and osteoclasts, ranging from integrins, connexin, purinergic receptors, Piezo1/2, and PCs to Wnt signaling pathway, is closely related to osteoporosis. In particular, Piezo1, PC1, and PC2 are innovative therapeutic targets for osteoporosis, and their activators may be able to treat osteoporosis. They may also be used to evaluate recovery after orthopedic surgery by detecting the levels of each target.
3.2. Indirect regulation by extracellular signaling molecules
Independent of the receptors on cell surface, exercise-induced regulation of bone environment may also occur through cytokines. Here, we summarize the common cytokines, such as inflammatory factors. Moreover, we address more promising myokines, such as irisin and myostatin.
3.2.1. Cytokines
3.2.1.1. Inflammatory factors
Cells sense and respond to mechanical strain fluctuations by adhesion structures such as integrins. Mechanical signals are then transferred into biochemical responses, regulating various processes from cytoskeleton change to cytokines secretion (52). This may lead to a disbalance between bone resorption and bone modeling (53).
The majority of proinflammatory cytokines such as IL-6, TNF-α, IL-1, and IFN-γ play a pivotal role in the treatment of osteoporosis through exercise (54–56). Exercise may improve inflammation by upregulating anti-inflammatory factors, which may have an effect on bone metabolism (57). It has been reported that high-intensity, low-impact, interphase exercise is an effective stimulus for producing proinflammatory cytokines such as IL-1, IL-6, and TNF-α, which shows a relationship between exercise, immunity, and the bone microenvironment (57).
Genetic variants of IL-6 are one of the risk factors for osteoporosis. It can increase the number of S1PR2 receptors on the surface of osteoclast precursors and accelerate the transformation from bone marrow-derived macrophage (BMDM) to mature osteoclasts (58). Zhou et al. reported that the beneficial effects of proper exercise on bone damage recovery were mediated through inflammation-inducing factors (59). The authors studied the osteoblast genesis mechanism in mice that continued exercising. At week 8, proinflammatory factors such as TNF-α and IL-6 were reduced through running or weight-bearing running, and the bone and bone marrow formation were promoted by weight-bearing exercise. Running or weight-bearing running may also be helpful for the recovery of patients with osteoporotic fractures. Zou et al. measured the levels of IL-1β, IL-6, and Cox-2 in serum and bone marrow; they found that ovariectomy led to a sharp increase in IL-1β in the bone marrow and IL-6 and Cox-2 in tibias, while exercise reduced the levels of IL-1, IL-6, and Cox-2 (60). That study suggested exercise as a robust method for the improvement of osteoporosis in postmenopausal osteoporosis rat models through inhibiting bone resorption and promoting bone formation, particularly in trabecular bone (60). Similarly, another study reported that after 6 weeks of moderate exercise training with estradiol therapy of ovariectomized (OVX) rats, serum analysis showed a significant increase in bone resorption markers and bone formation markers, from osteocalcin (OCN) and bone-specific alkaline phosphatase (ALP) to proinflammatory cytokines such as TNF-α and IL-6 (55). Hence, moderate exercise training improved osteoporotic alterations in OVX rats by inflammatory change and showed a potential to be an effective therapeutic strategy for postmenopausal osteoporosis (55). Mert et al. found a relationship between IL-6 levels and hip fracture in the study group after surgery, but TNF-α and IL-6 levels were not related to the occurrence of death and walking capability after the surgery. By measuring the levels of TNF-α and IL-6, they analyzed the relationship between inflammatory markers and postoperative mortality as well as walking capability. However, further clinical and functional confirmation is needed.
IFN-γ belongs to type-II interferons. The last developmental phases of osteoclasts can fuse to multinucleated osteoclasts through IFN-γ activation. IFN-γ induces the expression of NFATc1 and then the transcription of c-FOS (61). RANKL is a well-known messenger that starts the differentiation of osteoclasts. By increasing the quantity of MHC-II, IFN-γ activated T cells, and then the T cells secreted more RANKL (61). Inflammatory bone loss in the OVX mice associated with IFN-γ was rescued by wheel-running exercise. After that, CD8 T cells were activated and then secreted IFN-γ, which induced the NF-κB/MAPK pathway activation and inhibited osteoclastogenesis (56). After HA/β-TCP biomaterials were implanted into the mice, IFN-γ levels increased, which helped to treat osteoporotic fractures (59).
However, another study showed that running inhibited bone metabolism and levels of proinflammatory factors in male aged rats (62). In older rats on running training, the levels of TNF-α and IFN-γ from CD8(+) T cells were upregulated, and the number and activity of osteoblasts from the running rats were decreased (62). Inflammatory factors, such as TNF-α, IL-6, and IFN-γ, secreted by different cells in bone microenvironment. Their activities and effects depend on environmental heterogeneity. As a result, more robust studies are needed for this topic and further work will be necessary to allow for more complex and powerful experimental designs. Hence, it seems that we need more delicate settings to investigate the role of exercise in fighting osteoporosis by inflammatory regulation.
In addition to inflammatory cytokines, other factors associated with bone microenvironment, such as osteoprotegerin (OPG), can respond to mechanical stimuli. OPG is a decoy receptor for RANKL, and also influences bone remodeling. A prior study showed that moderate intensity endurance and vibration exercise upregulated OPG expression and downregulated the level of RANKL, thereby delaying osteoclast maturation and activity (63).
Overall, these findings indicate that cytokines, especially inflammatory factors, eventually modify the microenvironment in response to exercise or external mechanical stimuli, which changes cellular functions, influences bone homeostasis, and facilitates bone remodeling.
3.2.2. Myokines
Exercise promotes the activity of different types of muscle, especially skeletal muscle and cardiac muscle. Thus, muscles can be considered organs that can release signal factors. These factors, such as irisin, cathepsin B, and lactic acid, are important messengers in the muscle–organ crosstalk. Recently, it has been shown that in response to exercise, many myokines have effects on mitochondrial metabolism and are beneficial for cognitive behavioral therapy (64). Moreover, some myokines such as myostatin (MSTN) and irisin could work in the bone environment and have an effect on bone remodeling. Their role in the muscle–bone crosstalk is essential for osteoporosis regulation under various types of exercise.
3.2.2.1. Irisin
Irisin is a myokine that is a transcript of FNDC5 (65). The level of irisin expression is upregulated when people tremble in cold environment, which promotes brown adipocytes to burn energy and produce heat, as mitochondria are abundant in brown adipocytes (65).
Irisin not only allows for the crosstalk with adipose tissue, but also with bone, which are closely related to muscle in time and space. It has been shown in humans that after exercise, the levels of irisin produced from skeletal muscle and released to serum are increased (65, 66). Compared with aerobic exercise, endurance exercise can stimulate skeletal muscle to produce more irisin. Given that endurance exercise resembles trembling, the incretion of irisin is upregulated in response to both resistance exercise and trembling in cold conditions. However, in another study, after 8 weeks of training, the resistance exercise group and irisin injection group were more likely to have an increase in bone strength compared with the control group, but not compared with the endurance exercise group (67). This showed that resistance exercise can prevent bone mass loss and osteoporosis, although the relationship of endurance exercise and irisin secretion needs more investigation.
Under simulated microgravity, differentiation of osteoblasts can be increased by irisin (68). Meanwhile, irisin promotes osteoblastogenesis in the bony callus and accelerates the repair and growth of bone tissue after osteoporosis-related fracture. It is clear that irisin has a positive effect on osteoblasts. However, the effects and action mechanisms of irisin on osteoclasts are still unclear. Hu et al. found that irisin both rescued Ti-particle–impaired osteogenesis and mitigated the increase of wear-particle–induced RANKL from osteoblasts, thereby reducing bone resorption (69). In addition, irisin may lead to the inhibition of osteoclastogenesis by activating reactive oxygen species (ROS) signaling (69). This study revealed the mechanism of irisin in maintaining bone mass when binding to wear particles (69). But in another study, irisin activated downstream signaling pathways in cementoblasts by binding to the receptor integrin αV, and then to cells secreted RANKL and IL-6, which communicated with osteoclasts and promoted bone resorption (70).
Thus, it may be a novel pharmacologic modulator for the therapy of fracture and related complications of disuse osteoporosis. Moreover, moderate-intensity treadmill exercise promotes the circulation of irisin, so it is not difficult to deduce that after fracture, moderate-intensity treadmill exercise as soon as the body conditions allow may stimulate bone healing and recovery (71).
3.2.2.2. Myostatin
Myostatin (MSTN), also known as growth differentiation factor 8 (GCD-8), belongs to the transforming growth factor-β family. It is also a negative regulator of muscle growth.
MSTN is secreted as a precursor protein; then, an N-terminal propeptide and a C-terminal growth factor dimer are generated, and an inactive latent complex is subsequently formed. Under the regulation by bone morphogenetic protein 1/tolloid proteases, the complex liberates mature myostatin (72, 73).
Organisms with MSTN knockout show BMD upregulation. A recent study in ovariectomized rats has demonstrated that after 10-weeks weight-bearing training, MSTN circulation increased, and bone loss was attenuated (74). In that study, the rats were ovariectomized and exposed to weight-bearing training. Specifically, the rats had to bear 35% of the body weight and run at 20 m/min, for 6 days/week in total. After 10 weeks, the BMD of the rats’ total femur and trabecular bone increased, compared with the control group; mechanically, Wnt1 and β-catenin mRNA and protein levels were upregulated (74).
3.2.2.3. Others
In addition to MSTN and irisin, other myokines such as kynurenic acid (Kyna) also show important regulatory function in bone metabolism in osteoporosis (75). In the serum of ovariectomized mice, the concentrations of serum Kyna and kynurenine aminotransferases (Kats) were lower than in the control group. After treadmill-running exercise, the muscle levels of KATs and the serum concentration of Kyna increased. Meanwhile, the bone loss was reversed due to inhibiting osteoclast maturation through the Kyna/Gpr35/NFκB p65/Nfatc1 pathway and enhancing osteoblast proliferation (75).
Postmenopausal osteoporosis due to decreased estrogen levels and ovariectomized models show the characteristics of reduced estrogen levels (76). A recent study has demonstrated that estrogen signaling regulates expression and secretion of myokines that modulate osteoclast differentiation and activity. The authors used conditioned media that were collected from ovariectomized and skeletal muscle deficient in estrogen receptor α expression mice, with cytokine array, and found that estrogen deficiency altered myokines levels (76).
In summary, myokines, especially irisin and myostatin, can protect bone mass by acting on osteoblasts and osteoclasts, and by regulating the crosstalk in the bone microenvironment.
4. Types of osteoporosis and effects of exercise
A patient with osteoporosis has a low bone mass and deteriorated osseous microarchitecture, which results in weakened bone strength and an increased risk of fragility fractures. The Ministry of Health in the UK estimated 137 million women and 21 million men have a high osteoporotic fracture risk globally, and its prevalence is expected to double in the coming 40 years (1). Exercise is important in preventing and treating osteoporotic fractures. In older adults with higher levels of physical exercise, the incidence rate of hip fractures is 30%–50% lower than that in age-matched individuals with less exercise (77). In a prospective Epidemiology of Osteoporosis (EPIDOS) study, after following 6901 White women (≥75 years old) for 3.6 years, it was shown that low-intensity physical exercise increased the risk for proximal humerus fractures by more than two-fold (77).
Choice of exercise types requires careful consideration of risks and benefits of each manner of activity, as well as modern health status and physical fitness intensity. Physical exercise programs appear to have varying impacts on osteoporosis depending on their frequency, duration, and intensity (78). For patients with depression and osteoporosis, resistance training is a more universal and more reasonable choice than aerobic exercise, with evidence suggesting only benefits for depression (79). High-velocity, high-impact activity, such as jumping, is suitable for osteoporosis patients if they are able to tolerate the intensity. However, if patients present various clinical manifestations of osteoarthritis, it is better to avoid such activities. Moreover, considering that resistance training acts as a local stimulus to the bone microenvironment, to expand training into the whole-body skeletal strength, balance training should be encouraged to prevent falls (2).
Similarly, the most recent national guidelines from UK also recommend individuals with osteoporosis to undergo resistance and effective exercise programs to increase bone strength. Moreover, by taking part in physical activities, the risk of falls is reduced with the improvement of bone strength and balance. Vertebral fractures and falls caused pain. To reduce the pain, we should improve posture by undertaking spinal extension exercise (80).
4.1. Primary osteoporosis
Primary osteoporosis is most common in older population and postmenopausal women. There are two main forms of primary osteoporosis, including postmenopausal osteoporosis (type 1) and senile osteoporosis (type 2) (81).
4.1.1. Postmenopausal osteoporosis
Due to reduced estrogen levels in postmenopausal women, osteoporosis and osteoporotic fractures are common (82). It has been reported that 80% of individuals with osteoporosis are postmenopausal women (83), and approximately half of all postmenopausal women sustain a fracture due to osteoporosis (84). Early studies have shown that estrogen and mechanical stimulation corporately inhibit osteoclast (OC) generation (85), suggesting that they may work better against postmenopausal osteoporosis.
Recent research has demonstrated that effective exercises as indicated intervention strategies can prevent and control postmenopausal osteoporosis (86). By measuring and comparing the BMD of postmenopausal athletes with different levels of physical activity, Yang et al. concluded that long-term exercise effectively prevented bone loss in female athletes and improved their musculoskeletal fitness (87). In addition, wheel-running exercise prevented bone loss in OVX mice (56). RNA-sequencing technology in the peripheral blood mononuclear cells (PBMCs) of OVX rats was also used to investigate the effect of moderate-intensity treadmill exercise on the sensitive genes related to bone mass (88). It was found that moderate-intensity treadmill exercise improved bone mass by increasing CCL2 and other genes in PBMCs. Thus, PBMCs can be a useful tool in the peripheral blood for supervising the exercise effect on bone strength in postmenopausal osteoporosis (88).
Although exercise is regarded as an effective method to deter bone loss triggered by estrogen deficiency in postmenopausal women, the optimal training system for peak bone growth has not yet been outlined. A previous study has found that interval training is not better than endurance training regarding OVX mice bone growth (89). However, since the authors did not give a clear regime to support the training methods (89), further experiments investigating the duration and frequency of the training schedule are needed.
4.1.2. Senile osteoporosis
Age-related bone loss is associated with accelerated bone resorption and lower bone formation, resulting in osteoporosis and related fractures. Resembling other types of osteoporosis conditions, age-related bone loss is associated with a systemic bone loss related to trabecular and cortical compartments, osteopenia, osteoporosis, and subsequently high fracture risk (90). The number of patients who suffer from age-related bone fracture has substantially increased because of the global aging. Long-term care after fracture has also increased, which dramatically adds to the economic and societal burden. The effects of osteoporotic fractures on the health of older adults are also cataclysmic.
Due to widespread aging-induced changes in cellular function, tissues and organs change phenotypically, including those in the bone microenvironment. In elderly people, the production of mesenchymal stem cells (MSCs) and hematopoietic stem cells (HSC) is exhausted, and the regulation of cellular signaling is changed, resulting in the upregulation of pro-adipogenic, pro-osteoclastogenic, and anti-osteogenic factors. These are all systemic and bone-localized cytokines that affect the cellular crosstalk in the bone microenvironment (91). Impaired autophagy (92), excess ROS (93), DNA damage (94) and cellular senescence (95) may be potential therapeutic targets in treating osteoporosis.
Physical activity is considered an effective support for improving bone health in older people (90). The number of HSCs is upregulated by physical activity or exercise (96, 97). Exercise training increases the survival rate of bone marrow transplantation mice from 25% to 82% (98). In aged OVX mice, resistance exercise conducted by voluntary tower climbing reduces the number of osteoclasts compared to the control groups (99). It has also been reported that vibrations of the ankle muscles at a low intensity improve balance in elderly people at a high risk of falls (100), which may help prevent age-related osteoporosis complications.
In conclusion, the beneficial effects of exercise against aging-related osteoporosis have been reported in many studies. However, there are still many unsolved issues, such as improvement of its clinical efficacy and reduction of time spent on exercise by elderly patients.
4.2. Secondary osteoporosis
Osteoporosis can occur secondary to other traumatic conditions and metabolic diseases, such as long-term and large-dose drug intake (101, 102), hyperparathyroidism (103), and rheumatoid arthritis (104). Here, we address the two most common types of secondary osteoporosis.
4.2.1. Disuse osteoporosis
Immobilization and weightlessness have important effects on body physiology; because they involve a lack of mechanical loading, they lead to the reduction in skeletal integrity. As a result, disuse is one of the major risk factors for osteoporosis (105). Disuse osteoporosis has become a common clinical problem that impairs physical function. Many diseases contribute to disuse osteoporosis, such as multiple sclerosis (106) and neurological disorders (107); however, unloading is the least uncommon factor in skeletal deterioration. The affected limb of a patient who undergoes amputation tends to lose bone mineral density more quickly than the fit limb (108). It has been demonstrated in patients with spinal cord injury that bone loss reaches a peak after three months (L. 109, 110). The greatest bone mass reduction always occurs below the level of injury, but it also occurs throughout the body (L 109). Disuse conditions favor a combination of high bone resorption and low bone formation (111), leading to immediate bone loss and eventually to osteoporosis with increased falling and fracture risk.
Exercise increases osteoblast collagen expression and mechanical strain affects collagen arrangement, which have an effect on new bone formation, thereby strengthening the skeleton. Bed rest or weightlessness, without physical activity, negatively regulates bone by affecting the activities of osteoblasts and osteoclasts (112). It has been reported that fluid flow upregulates Runx2 expression by both the classical and nonclassical Wnt pathways in mouse BMSCs, and promotes BMSCs differentiation to osteoblasts, while mechanical unloading inhibits β-catenin expression and suppresses osteoblast proliferation (113).
In recent years, there has been an increased concern about mechanoresponsive sensitivity in human fracture recovery to help patients’ fast and robust return to normal life. Qian et al. found that, for bedridden patients, increased miR-138-5p levels in bone specimens correlated with a lower degree of bone formation (114). They found that under different mechanical conditions, miR-138-5p directly targeted MACF1 to inhibit osteoblast maturation (114). By bone-targeted inhibition of miR-138-5p, the bone anabolic response to mechanical loading became sensitized in miR-138-5p transgenic mice, and bone formation was accelerated. They identified miR-138-5p as a mechanoresponsive miRNA, and inhibition of miR-138-5p in osteoblasts may be a novel bone anabolic sensitization strategy for improving disuse osteoporosis (114).
Altogether, physical exercise is an effective therapeutic strategy to help patients with immobilization and improve their quality of life. Improvement of sensitivity to mechanical stimuli is a feasible approach for accelerating patients’ recovery.
4.2.2. Glucocorticoid-related osteoporosis
Glucocorticoids are broadly used to suppress inflammation in many immune diseases, such as rheumatic diseases and those arising after organ transplantation (101). Glucocorticoid-related osteoporosis is the most critical complication induced by glucocorticoids (101).
Early studies have found that glucocorticoids regulate differentiated bone cell functions and osteoclastogenesis to regulate the balance between bone resorption and bone formation (115). Glucocorticoid-related osteoporosis is a predominant form of secondary osteoporosis (116). Its major cause is likely the impairment in bone formation (116). Normally, BMSCs can differentiate into osteogenic, adipogenic, and chondrogenic cells, which is known as tri-lineage differentiation potential (117). However, in glucocorticoid-related osteoporosis, the quantity and activity of osteoblasts decreases, while those of fat cells increases (118). However, because of other side effects and off-target effects, current drug treatments are a long way from achieving satisfactory effects (102).
Glucocorticoid overdose and unsatisfied treatments lead to detrimental side effects on bone as well as on muscle, cartilage, and fat mass. Healthy diet and regular physical exercise might mitigate these conditions (119). As for physical exercises, the BBC guidelines on postmenopausal osteoporosis recommend focusing on impact training, body balance, strength, and resistance training (120). It is also suggested that regular exercise is an effective therapeutic and preventive strategy with few side effects in glucocorticoid-related osteoporosis in adults (119).
In conclusion, exercise has a positive effect on the treatment of glucocorticoid-related osteoporosis, although more studies are needed to investigate the physiological and pathological mechanisms involved.
5. Conclusions and perspective
In summary, mechanically speaking, the exercise-related regulation of osteoporosis is complex and likely involves multiple pathways, including direct and indirect mechanisms. This includes mechanical signaling transduction from receptors on the cell surface, such as PC-1/2 and Piezo1/2, to cytokines in the bone microenvironment, ranging from inflammatory factors to myokines. The changes in biochemical markers of bone turnover and consequently the change in bone mass might be seen as a manifestation of direct or indirect exercise effects, but more studies are needed to further clarify fine regulation.
Considering the advantages of exercise in the regulation of osteoporosis, such as low costs, easy performance, and few adverse effects, exercise has a huge potential in the future in osteoporosis treatment and prevention. Hence, exercise has become increasingly appreciated by doctors and researchers (Table 1). Exercise effects in multiple classes of osteoporosis have also been well documented. Recent UK guidelines also provide dedicated recommendations (80). We need further studies to determine the mechanisms by which exercise regulates different types of osteoporosis, reduce the exercise sensitive threshold of patients, increase exercise regulation efficiency, and prevent harm from hypermobility. All of these aspects would lessen the pain and fracture risk of osteoporosis patients.
Author contributions
All authors were involved in conceptualizing the paper. XC led the drafting of the manuscript. SX and HZ reviewed, edited, and approved the final paper. All authors contributed to the article and approved the submitted version.
Funding
This work was also supported by Science Fund of the National Natural Science Foundation of China (No. 31870910 and No. 82071789).
Conflict of interest
The authors declare that the research was conducted in the absence of any commercial or financial relationships that could be construed as a potential conflict of interest.
Publisher’s note
All claims expressed in this article are solely those of the authors and do not necessarily represent those of their affiliated organizations, or those of the publisher, the editors and the reviewers. Any product that may be evaluated in this article, or claim that may be made by its manufacturer, is not guaranteed or endorsed by the publisher.
References
1. Odén A, McCloskey EV, Kanis JA, Harvey NC, Johansson H. Burden of high fracture probability worldwide: Secular increases 2010–2040. Osteoporosis Int (2015) 26(9):2243–48. doi: 10.1007/s00198-015-3154-6
2. Compston J, Cooper A, Cooper C, Gittoes N, Gregson C, Harvey N, et al. UK Clinical guideline for the prevention and treatment of osteoporosis. Arch Osteoporosis (2017) 12(1):43. doi: 10.1007/s11657-017-0324-5
3. Braith RW, Conner JA, Fulton MN, Lisor CF, Casey DP, Howe KS, et al. Comparison of alendronate vs alendronate plus mechanical loading as prophylaxis for osteoporosis in lung transplant recipients: A pilot study. J Heart Lung Transpl: Off Publ Int Soc Heart Transplant (2007) 26(2):132–37. doi: 10.1016/j.healun.2006.11.004
4. Li X, Liu D, Li J, Yang S, Xu J, Yokota H, et al. Wnt3a involved in the mechanical loading on improvement of bone remodeling and angiogenesis in a postmenopausal osteoporosis mouse model. FASEB J (2019) 33(8):8913–24. doi: 10.1096/fj.201802711R
5. Montgomery G, Abt G, Dobson C, Smith T, Evans W, Ditroilo M. The mechanical loading and muscle activation of four common exercises used in osteoporosis prevention for early postmenopausal women. J Electromyography Kinesiology (2019) 44:124–31. doi: 10.1016/j.jelekin.2018.12.004
6. Olsen BR, Reginato AM, Wang W. Bone development. Annu Rev Cell Dev Biol (2000) 16(1):191–220. doi: 10.1146/annurev.cellbio.16.1.191
7. Ponte AL, Marais EE, Gallay N, Langonné A, Delorme B, Hérault O, et al. The in vitro migration capacity of human bone marrow mesenchymal stem cells: Comparison of chemokine and growth factor chemotactic activities. Stem Cells (2007) 25(7):1737–45. doi: 10.1634/stemcells.2007-0054
8. Garg P, Mazur MM, Buck AC, Wandtke ME, Liu J, Ebraheim NA. Prospective review of mesenchymal stem cells differentiation into osteoblasts. Orthopaedic Surg (2017) 9(1):13–9. doi: 10.1111/os.12304
9. Crockett JC, Rogers MJ, Coxon FP, Hocking LJ, Helfrich MH. Bone remodelling at a glance. J Cell Sci (2011) 124(7):991–98. doi: 10.1242/jcs.063032
10. Martin JT, Sims NA. Osteoclast-derived activity in the coupling of bone formation to resorption. Trends Mol Med (2005) 11(2):76–81. doi: 10.1016/j.molmed.2004.12.004
11. Garimella R, Tague SE, Zhang J, Belibi F, Nahar N, Sun BH, et al. Expression and synthesis of bone morphogenetic proteins by osteoclasts: A possible path to anabolic bone remodeling. J Histochem Cytochem (2008) 56(6):569–77. doi: 10.1369/jhc.2008.950394
12. Klein-Nulend J, Bakker AD, Bacabac RG, Vatsa A, Weinbaum S. Mechanosensation and transduction in osteocytes. Bone (2013) 54(2):182–90. doi: 10.1016/j.bone.2012.10.013
13. Hemmatian H, Bakker AD, Klein-Nulend J, van Lenthe GH. Aging, osteocytes, and mechanotransduction. Curr Osteoporosis Rep (2017) 15(5):401–11. doi: 10.1007/s11914-017-0402-z
14. Troy KL, Mancuso ME, Butler TA, Johnson JE. Exercise early and often: Effects of physical activity and exercise on women’s bone health. Int J Environ Res Public Health (2018) 15(5):878. doi: 10.3390/ijerph15050878
15. Styner M, Pagnotti GM, McGrath C, Wu X, Sen B, Uzer G, et al. Exercise decreases marrow adipose tissue through SS-oxidation in obese running mice. J Bone Mineral Res: Off J Am Soc Bone Mineral Res (2017) 32(8):1692–702. doi: 10.1002/jbmr.3159
16. Suniaga S, Rolvien T, Scheidt Av, Fiedler IAK, Bale HA, Huysseune A, et al. Increased mechanical loading through controlled swimming exercise induces bone formation and mineralization in adult zebrafish. Sci Rep (2018) 8(1):3646. doi: 10.1038/s41598-018-21776-1
17. Glass IIDA, Karsenty G. In vivo analysis of wnt signaling in bone. Endocrinology (2007) 148(6):2630–34. doi: 10.1210/en.2006-1372
18. Geiger B, Spatz JP, Bershadsky AD. Environmental sensing through focal adhesions. Nat Rev Mol Cell Biol (2009) 10(1):21–33. doi: 10.1038/nrm2593
19. Barczyk M, Carracedo S, Gullberg D. Integrins. Cell Tissue Res (2010) 339(1):269–80. doi: 10.1007/s00441-009-0834-6
20. Plotkin LI, Davis HM, Cisterna BA, Sáez JC. Connexins and pannexins in bone and skeletal muscle. Curr Osteoporosis Rep (2017) 15(4):326–34. doi: 10.1007/s11914-017-0374-z
21. Buo AM, Stains JP. Gap junctional regulation of signal transduction in bone cells. FEBS Lett (2014) 588:1315–21. doi: 10.1016/j.febslet.2014.01.025
22. Burnstock G. Purinergic signalling: Therapeutic developments. Front Pharmacol (2017) 8. doi: 10.3389/fphar.2017.00661
23. Robling AG, Niziolek PJ, Baldridge LA, Condon KW, Allen MR, Alam I, et al. Mechanical stimulation of bone in vivo reduces osteocyte expression of Sost/Sclerostin *. J Biol Chem (2008) 283(9):5866–75. doi: 10.1074/jbc.M705092200
24. Rubin J, Rubin C, Jacobs CR. Molecular pathways mediating mechanical signaling in bone. Gene (2006) 367(1–2):1–16. doi: 10.1016/j.gene.2005.10.028
25. Qin L, Liu W, Cao H, Xiao G. Molecular mechanosensors in osteocytes. Bone Res (2020) 8(1):23. doi: 10.1038/s41413-020-0099-y
26. Wang H, Sun W, Ma J, Pan Y, Wang L, Zhang W. Polycystin-1 mediates mechanical strain-induced osteoblastic mechanoresponses via potentiation of intracellular calcium and akt/β-catenin pathway. PloS One (2014) 9(3):e91730–0. doi: 10.1371/journal.pone.0091730
27. Xiao Z, Zhang S, Mahlios J, Zhou G, Magenheimer BS, Guo D, et al. Cilia-like structures and polycystin-1 in Osteoblasts/Osteocytes and associated abnormalities in skeletogenesis and Runx2 expression. J Biol Chem (2006) 281(41):30884–95. doi: 10.1074/jbc.M604772200
28. Lee W, Leddy HA, Chen Y, Lee SH, Zelenski NA, McNulty AL, et al. Synergy between Piezo1 and Piezo2 channels confers high-strain mechanosensitivity to articular cartilage. Proc Natl Acad Sci USA (2014) 111(47):E5114–22. doi: 10.1073/pnas.1414298111
29. Zhou T, Gao B, Fan Y, Liu Y, Feng S, Cong Q, et al. Piezo1/2 mediate mechanotransduction essential for bone formation through concerted activation of NFAT-YAP1-ß-Catenin. ELife (2020) 9:e52779. doi: 10.7554/eLife.52779
30. Zhao D, Hua R, Riquelme MA, Cheng H, Guda T, Xu H, et al. Osteocytes regulate bone anabolic response to mechanical loading in Male mice via activation of integrin A5. Bone Res (2022) 10(1):49. doi: 10.1038/s41413-022-00222-z
31. Zhao D, Riquelme MA, Guda T, Tu C, Xu H, Gu S, et al. Connexin hemichannels with prostaglandin release in anabolic function of bone to mechanical loading. ELife (2022) 11:e74365. doi: 10.7554/ELIFE.74365
32. Kringelbach TM, Aslan D, Novak I, Ellegaard M, Syberg S, Andersen CKB, et al. Fine-tuned ATP signals are acute mediators in osteocyte mechanotransduction. Cell Signalling (2015) 27(12):2401–9. doi: 10.1016/j.cellsig.2015.08.016
33. Menzies RI, Tam FW, Unwin RJ, Bailey MA. Purinergic signaling in kidney disease. Kidney Int (2017) 91(2):315–23. doi: 10.1016/j.kint.2016.08.029
34. Li J, Liu D, Ke HZ, Duncan RL, Turner CH. The P2X7 nucleotide receptor mediates skeletal mechanotransduction. J Biol Chem (2005) 280(52):42952–59. doi: 10.1074/jbc.M506415200
35. Manaka S, Tanabe N, Kariya T, Naito M, Takayama T, Nagao M, et al. Low-intensity pulsed ultrasound-induced ATP increases bone formation via the P2X7 receptor in osteoblast-like MC3T3-E1 cells. FEBS Lett (2015) 589(3):310–18. doi: 10.1016/j.febslet.2014.12.013
36. Dalagiorgou G, Piperi C, Georgopoulou U, Adamopoulos C, Basdra EK, Papavassiliou AG. Mechanical stimulation of polycystin-1 induces human osteoblastic gene expression via potentiation of the Calcineurin/NFAT signaling axis. Cell Mol Life Sciences: CMLS (2013) 70(1):167–80. doi: 10.1007/s00018-012-1164-5
37. Xiao Z, Zhang S, Cao L, Qiu N, David V, Quarles LD. Conditional disruption of Pkd1 in osteoblasts results in osteopenia due to direct impairment of bone formation. J Biol Chem (2010) 285(2):1177–87. doi: 10.1074/jbc.M109.050906
38. Xiao Z, Dallas M, Qiu N, Nicolella D, Cao L, Johnson M, et al. Conditional deletion of Pkd1 in osteocytes disrupts skeletal mechanosensing in mice. FASEB J: Off Publ Fed Am Societies Exp Biol (2011) 25(7):2418–32. doi: 10.1096/fj.10-180299
39. Xiao Z, Cao L, Liang Y, Huang J, Stern AR, Dallas M, et al. Osteoblast-specific deletion of Pkd2 leads to low-turnover osteopenia and reduced bone marrow adiposity. PloS One (2014) 9(12):e114198–e114198. doi: 10.1371/journal.pone.0114198
40. Xiao Z, Quarles LD. Physiological mechanisms and therapeutic potential of bone mechanosensing. Rev Endocr Metab Disord (2015) 16(2):115–29. doi: 10.1007/s11154-015-9313-4
41. Mesner LD, Ray B, Hsu Y-H, Manichaikul A, Lum E, Bryda EC, et al. Bicc1 is a genetic determinant of osteoblastogenesis and bone mineral density. J Clin Invest (2014) 124(6):2736–49. doi: 10.1172/JCI73072
42. Leuenroth SJ, Okuhara D, Shotwell JD, Markowitz GS, Yu Z, Somlo S, et al. Triptolide is a traditional Chinese medicine-derived inhibitor of polycystic kidney disease. Proc Natl Acad Sci USA (2007) 104(11):4389–94. doi: 10.1073/pnas.0700499104
43. Hiramoto K, Kobayashi H, Sekiyama AE, Sato F, Tsuruta D, Ishii M, et al. Mild exercise suppresses exacerbation of dermatitis by increasing cleavage of the βendorphin from proopiomelanocortin in NC/Nga mice. J Clin Biochem Nutr (2013) 52(1):58–63. doi: 10.3164/jcbn.12251
44. Poole K, Herget R, Lapatsina L, Ngo HD, Lewin GR. Tuning piezo ion channels to detect molecular-scale movements relevant for fine touch. Nat Commun (2014) 5:3520. doi: 10.1038/ncomms4520
45. Zhao Q, Wu K, Geng J, Chi S, Wang Y, Zhi P, et al. Ion permeation and mechanotransduction mechanisms of mechanosensitive piezo channels. Neuron (2016) 89(6):1248–63. doi: 10.1016/j.neuron.2016.01.046
46. Li X, Han L, Nookaew I, Mannen E, Silva MJ, Almeida M, et al. Stimulation of Piezo1 by mechanical signals promotes bone anabolism. eLife (2019) 8:e49631. doi: 10.7554/eLife.49631.001
47. Wang L, You X, Lotinun S, Zhang L, Wu N, Zou W. Mechanical sensing protein PIEZO1 regulates bone homeostasis via osteoblast-osteoclast crosstalk. Nat Commun (2020) 11(1):282. doi: 10.1038/s41467-019-14146-6
48. Manolagas SC. Wnt signaling and osteoporosis. Maturitas (2014) 78(3):233–37. doi: 10.1016/j.maturitas.2014.04.013
49. Liao HW, Huang TH, Chang YH, Liou HH, Chou YH, Sue YM, et al. Exercise alleviates osteoporosis in rats with mild chronic kidney disease by decreasing sclerostin production. Int J Mol Sci (2019) 20(8):2044. doi: 10.3390/ijms20082044
50. Wang H, Brennan TA, Russell E, Kim JH, Egan KP, Chen Q, et al. R-spondin 1 promotes vibration-induced bone formation in mouse models of osteoporosis. J Mol Med (2013) 91(12):1421–29. doi: 10.1007/s00109-013-1068-3
51. Chen X, Yang K, Sun P, Zhao R, Liu B, Lu P. Exercise improves bone formation by upregulating the Wnt3a/β-catenin signalling pathway in type 2 diabetic mice. Diabetol Metab Syndrome (2021) 13(1):116. doi: 10.1186/s13098-021-00732-6
52. Khan RB, Goult BT. Adhesions assemble!–autoinhibition as a major regulatory mechanism of integrin-mediated adhesion. Front Mol Biosci (2019) 6. doi: 10.3389/fmolb.2019.00144
53. Grässel S, Zaucke F, Madry H. Osteoarthritis: Novel molecular mechanisms increase our understanding of the disease pathology. J Clin Med (2021) 10(9):1938. doi: 10.3390/jcm10091938
54. Walsh MC, Takegahara N, Kim H, Choi Y. Updating osteoimmunology: Regulation of bone cells by innate and adaptive immunity. Nat Rev Rheumatol (2018) 14(3):146–56. doi: 10.1038/nrrheum.2017.213
55. Abdelfattah Abulfadle K, Atia RRA, Mohammed HO, Ramadan RS, Mohammed NA. The potential anti-osteoporotic effect of exercise–induced increased preptin level in ovariectomized rats. Anatomical Sci Int (2022) 10.1007/s12565-022-00666-7. doi: 10.1007/s12565-022-00666-7
56. Shen H, He J, Ling X, Liu C, Wang Y, Zhang X, et al. Wheel-running exercise protects ovariectomized mice from bone loss via IFN-γ-Mediated suppression of the NF-κB and MAPK pathways. Oxid Med Cell Longevity (2022) 2022:1–17. doi: 10.1155/2022/2030818
57. Mezil YA, Allison D, Kish K, Ditor D, Ward WE, Tsiani E, et al. Response of bone turnover markers and cytokines to high-intensity low-impact exercise. Med Sci Sports Exercise (2015) 47(7):1495–502. doi: 10.1249/MSS.0000000000000555
58. Tanaka K, Hashizume M, Mihara M, Yoshida H, Suzuki M, Matsumoto Y. Anti-Interleukin-6 receptor antibody prevents systemic bone mass loss via reducing the number of osteoclast precursors in bone marrow in a collagen-induced arthritis model. Clin Exp Immunol (2014) 175(2):172–80. doi: 10.1111/cei.12201
59. Cheng L, Khalaf AT, Lin T, Ran L, Shi Z, Wan J, et al. Exercise promotes the osteoinduction of HA/B-TCP biomaterials via the wnt signaling pathway. Metabolites (2020) 10(3):90. doi: 10.3390/metabo10030090
60. Li L, Chen X, Lv S, Dong M, Zhang L, Tu J, et al. Influence of exercise on bone remodeling-related hormones and cytokines in ovariectomized rats: A model of postmenopausal osteoporosis. PloS One (2014) 9(11):e112845. doi: 10.1371/journal.pone.0112845
61. Tang M, Tian L, Luo G, Yu X. Interferon-Gamma-Mediated osteoimmunology. Front Immunol (2018) 9(JUN). doi: 10.3389/fimmu.2018.01508
62. Sipos W, Rauner M, Skalicky M, Viidik A, Hofbauer G, Schett G, et al. Running has a negative effect on bone metabolism and proinflammatory status in Male aged rats. Exp Gerontol (2008) 43(6):578–83. doi: 10.1016/j.exger.2008.03.008
63. Notomi T, Karasaki I, Okazaki Y, Okimoto N, Kato Y, Ohura K, et al. Insulinogenic sucrose+amino acid mixture ingestion immediately after resistance exercise has an anabolic effect on bone compared with non-insulinogenic fructose+amino acid mixture in growing rats. Bone (2014) 65:42–8. doi: 10.1016/j.bone.2014.05.002
64. Guo P, Jin Z, Wang J, Sang A, Wu H. Irisin rescues blood-brain barrier permeability following traumatic brain injury and contributes to the neuroprotection of exercise in traumatic brain injury. Oxid Med Cell Longevity (2021) 2021:1118981. doi: 10.1155/2021/1118981
65. Albrecht E, Schering L, Buck F, Vlach K, Schober HC, Drevon CA, et al. Irisin: Still chasing shadows. Mol Metab (2020) 34:124–35. doi: 10.1016/j.molmet.2020.01.016
66. Reza MM, Subramaniyam N, Sim CM, Ge X, Sathiakumar D, McFarlane C, et al. Irisin is a pro-myogenic factor that induces skeletal muscle hypertrophy and rescues denervation-induced atrophy. Nat Commun (2017) 8(1):1104. doi: 10.1038/s41467-017-01131-0
67. Shahabi S, Esfarjani F, Reisi J, Momenzadeh S, Jami M-S, Zamani S. The effects of 8-week resistance and endurance trainings on bone strength compared to irisin injection protocol in mice. Adv Biomed Res (2021) 10(1):40. doi: 10.4103/abr.abr_220_20
68. Chen Z, Zhang Y, Zhao F, Yin C, Yang C, Wang X, et al. Recombinant irisin prevents the reduction of osteoblast differentiation induced by stimulated microgravity through increasing β-catenin expression. Int J Mol Sci (2020) 21(4):1259. doi: 10.3390/ijms21041259
69. Hu S, Xue Y, He J, Chen C, Sun J, Jin Y, et al. Irisin recouples osteogenesis and osteoclastogenesis to protect Wear-Particle-Induced osteolysis by suppressing oxidative stress and RANKL production. Biomater Sci (2021) 9(17):5791–801. doi: 10.1039/d1bm00563d
70. Zhao C, Wang Y, Cao Z, Zhu J, He H. Effect of irisin on the expression of osteoclast-related genes in cementoblasts. Eur J Orthodontics (2022) 40(4):420–6. doi: 10.1093/ejo/cjac010
71. Colucci SC, Buccoliero C, Sanesi L, Errede M, Colaianni G, Annese T, et al. Systemic administration of recombinant irisin accelerates fracture healing in mice. Int J Mol Sci (2021) 22(19):10863. doi: 10.3390/ijms221910863
72. Lee SJ, McPherron AC. Regulation of myostatin activity and muscle growth. Proc Nat Acad Sci USA (2001) 98(6):9306–11. doi: 10.1073/pnas.151270098
73. Wolfman NM, Mcpherron AC, Pappano WN, Davies MV, Song K, Tomkinson KN, et al. Activation of latent myostatin by the BMP-1tolloid family of metalloproteinases. Proc Nat Acad Sci USA (2003) 100(26):15842–6. doi: 10.1073/pnas.2534946100
74. Tang L, Zhao T, Kang Y, An S, Fan X, Sun L. MSTN is an important myokine for weight-bearing training to attenuate bone loss in ovariectomized rats. J Physiol Biochem (2022) 78(1):61–72. doi: 10.1007/s13105-021-00838-5
75. Shi T, Shi Y, Gao H, Ma Y, Wang Q, Shen S, et al. Exercised accelerated the production of muscle-derived kynurenic acid in skeletal muscle and alleviated the postmenopausal osteoporosis through the Gpr35/NFκB P65 pathway. J Orthopaedic Translation (2022) 35:1–12. doi: 10.1016/j.jot.2022.03.003
76. Norton A, Thieu K, Baumann CW, Lowe DA, Mansky KC. Estrogen regulation of myokines that enhance osteoclast differentiation and activity. Sci Rep (2022) 12(1):15900. doi: 10.1038/s41598-022-19438-4
77. Lee SH, Dargent-Molina P, Bréart G. Risk factors for fractures of the proximal humerus: Results from the EPIDOS prospective study. J Bone Mineral Res (2002) 17(5):817–25. doi: 10.1359/jbmr.2002.17.5.817
78. Rossini M, Adami S, Bertoldo F, Diacinti D, Gatti D, Giannini S, et al. Guidelines for the diagnosis, prevention and management of osteoporosis, Reumatismo (2016) 68(1):1–39.
79. Izquierdo M, Duque G, Morley JE. Physical activity guidelines for older people: Knowledge gaps and future directions. Lancet Healthy Longevity (2021) 2(6):e380–83. doi: 10.1016/S2666-7568(21)00079-9
80. Brooke-Wavell K, Skelton DA, Barker KL, Clark EM, de Biase S, Arnold S, et al. Strong, steady and straight: UK consensus statement on physical activity and exercise for osteoporosis. Br J Sports Med (2022) 56(15):837–46. doi: 10.1136/bjsports-2021-104634
81. Walallawita US, Wolber FM, Ziv-Gal A, Kruger MC, Heyes JA. Potential role of lycopene in the prevention of postmenopausal bone loss: Evidence from molecular to clinical studies. Int J Mol Sci (2020) 21(19):1–21. doi: 10.3390/ijms21197119
82. Massart F, Reginster JY, Brandi ML. Genetics of menopause-associated diseases. Maturitas (2001) 40(2):103–16. doi: 10.1016/S0378-5122(01)00283-3
83. Lotz EM, Cohen DJ, Ellis RA, Wayne JS, Schwartz Z, Boyan BD. Ibandronate treatment before and after implant insertion impairs osseointegration in aged rats with ovariectomy induced osteoporosis. JBMR Plus (2019) 3(7):e10184. doi: 10.1002/jbm4.10184
84. Hansen KE, Johnson RE, Chambers KR, Johnson MG, Lemon CC, Vo TNT, et al. Treatment of vitamin d insufficiency in postmenopausal women: A randomized clinical trial. JAMA Internal Med (2015) 175(10):1612–21. doi: 10.1001/jamainternmed.2015.3874
85. Allison H, Mcnamara LM. Inhibition of osteoclastogenesis by mechanically stimulated osteoblasts is attenuated During1 deficiency. Cell Physiol (2019) 317(5):C969–82. doi: 10.1152/ajpcell.00168.2019
86. Iwamoto J, Takeda T, Ichimura S. Effect of exercise training and detraining on bone mineral density in postmenopausal women with osteoporosis. J Orthopaedic Sci (2001) 6(2):128–32. doi: 10.1007/s007760100059
87. Chen K-T, Yang R-S. Effects of exercise on lipid metabolism and musculoskeletal fitness in female athletes. World J Gastroenterol (2004) 10(1):122–26. doi: 10.3748/wjg.v10.i1.122
88. Gao L, Li Y, Yang YJ, Zhang DY. The effect of moderate-intensity treadmill exercise on bone mass and the transcription of peripheral blood mononuclear cells in ovariectomized rats. Front Physiol (2021) 12:729910. doi: 10.3389/fphys.2021.729910
89. Latza J, Otte M, Lindner T, Fischer DC, Bruhn S, Hollinski R, et al. Interval training is not superior to endurance training with respect to bone accrual of ovariectomized mice. Front Physiol (2020) 11:1096. doi: 10.3389/fphys.2020.01096
90. Yi L, Ju Y, He Y, Yin X, Xu Y, Weng T. Intraperitoneal injection of desferal® alleviated the age-related bone loss and senescence of bone marrow stromal cells in rats. Stem Cell Res Ther (2021) 12(1):45. doi: 10.1186/s13287-020-02112-9
91. Raisz LG, Rodan GA. Pathogenesis of osteoporosis. Endocrinol Metab Clinics North America (2003) 32(1):15–24. doi: 10.1016/S0889-8529(02)00055-5
92. Yuan Y, Fang Y, Zhu L, Gu Y, Li L, Qian J, et al. Deterioration of hematopoietic autophagy is linked to osteoporosis. Aging Cell (2020) 19(5):e13114. doi: 10.1111/acel.13114
93. Ishii K, Fumoto T, Iwai K, Takeshita S, Ito M, Shimohata N, et al. Coordination of PGC-1β and iron uptake in mitochondrial biogenesis and osteoclast activation. Nat Med (2009) 15(3):259–66. doi: 10.1038/nm.1910
94. Kim HN, Chang J, Shao L, Han L, Iyer S, Manolagas SC, et al. DNA Damage and senescence in osteoprogenitors expressing Osx1 may cause their decrease with age. Aging Cell (2017) 16(4):693–703. doi: 10.1111/acel.12597
95. Piemontese M, Almeida M, Robling AG, Kim HN, Xiong J, Thostenson JD, et al. Old age causes de Novo intracortical bone remodeling and porosity in mice. JCI Insight (2017) 2(17):e93771. doi: 10.1172/JCI.INSIGHT.93771
96. Emmons R, Niemiro GM, de Lisio M. Exercise as an adjuvant therapy for hematopoietic stem cell mobilization. Stem Cells Int (2016) 2016: 7131359. doi: 10.1155/2016/7131359
97. Frodermann V, Rohde D, Courties G, Severe N, Schloss MJ, Amatullah H, et al. Exercise reduces inflammatory cell production and cardiovascular inflammation via instruction of hematopoietic progenitor cells. Nat Med (2019) 25(11):1761–71. doi: 10.1038/s41591-019-0633-x
98. de Lisio M, Baker JM, Parise G. Exercise promotes bone marrow cell survival and recipient reconstitution post-bone marrow transplantation, which is associated with increased survival. Exp Hematol (2013) 41(2):143–54. doi: 10.1016/j.exphem.2012.10.003
99. Notomi T, Okimoto N, Okazaki Y, Nakamura T, Suzuki M. Tower climbing exercise started 3 months after ovariectomy recovers bone strength of the femur and lumbar vertebrae in aged osteopenic rats. J Bone Mineral Res: Official J Am Soc Bone Mineral Res (2003) 18(1):140–9. doi: 10.1359/jbmr.2003.18.1.140
100. Toosizadeh N, Mohler J, Marlinski V. Low intensity vibration of ankle muscles improves balance in elderly persons at high risk of falling. PloS One (2018) 13(3):e0194720. doi: 10.1371/journal.pone.0194720
101. Cain DW, Cidlowski JA. Specificity and sensitivity of glucocorticoid signaling in health and disease. Best Pract Res: Clin Endocrinol Metab (2015) 29(4):545–56. doi: 10.1016/j.beem.2015.04.007
102. Chotiyarnwong P, McCloskey EV. Pathogenesis of glucocorticoid-induced osteoporosis and options for treatment. Nat Rev Endocrinol (2020) 16(8):437–47. doi: 10.1038/s41574-020-0341-0
103. Walker MD, Silverberg SJ. Primary hyperparathyroidism. Nat Rev Endocrinol (2018) 14(2):115–25. doi: 10.1038/nrendo.2017.104
104. Sparks JA. In the clinic® rheumatoid arthritis. Ann Internal Med (2019) 170(1):ITC1–15. doi: 10.7326/AITC201901010
105. Rachner TD, Khosla S, Hofbauer LC. Osteoporosis: Now and the future. Lancet (London England) (2011) 377(9773):1276–87. doi: 10.1016/S0140-6736(10)62349-5
106. Terzi T, Terzi M, Tander B, Cantürk F, Onar M. Changes in bone mineral density and bone metabolism markers in premenopausal women with multiple sclerosis and the relationship to clinical variables. J Clin Neurosci (2010) 17(10):1260–64. doi: 10.1016/j.jocn.2010.01.044
107. Morse LR, Battaglino RA, Stolzmann KL, Hallett LD, Waddimba A, Gagnon D, et al. Osteoporotic fractures and hospitalization risk in chronic spinal cord injury. Osteoporosis Int (2009) 20(3):385–92. doi: 10.1007/s00198-008-0671-6
108. Cunningham HC, West DWD, Baehr LM, Tarke FD, Baar K, Bodine SC, et al. Age-dependent bone loss and recovery during hindlimb unloading and subsequent reloading in rats. BMC Musculoskeletal Disord (2018) 19(1):223. doi: 10.1186/s12891-018-2156-x
109. Maïmoun L, Couret I, Mariano-Goulart D, Dupuy AM, Micallef JP, Peruchon E, et al. Changes in Osteoprotegerin/RANKL system, bone mineral density, and bone biochemicals markers in patients with recent spinal cord injury. Calcif Tissue Int (2005) 76(6):404–11. doi: 10.1007/s00223-004-0048-6
110. Roberts D, Lee W, Cuneo RC, Wittmann J, Ward G, Flatman R, et al. Longitudinal study of bone turnover after acute spinal cord injury. J Clin Endrocrinol Metab (1998) 83(2):415–22. doi: 10.1210/jc.83.2.415
111. Gaudio A, Pennisi P, Bratengeier C, Torrisi V, Lindner B, Mangiafico RA, et al. Increased sclerostin serum levels associated with bone formation and resorption markers in patients with immobilization-induced bone loss. J Clin Endocrinol Metab (2010) 95(5):2248–53. doi: 10.1210/jc.2010-0067
112. Armbrecht G, Belavý DL, Backström M, Beller G, Alexandre C, Rizzoli R, et al. Trabecular and cortical bone density and architecture in women after 60 days of bed rest using high-resolution PQCT: WISE 2005. J Bone Mineral Res (2011) 26(10):2399–410. doi: 10.1002/jbmr.482
113. Yin C, Zhang Y, Hu L, Tian Y, Chen Z, Li D, et al. Mechanical unloading reduces microtubule actin crosslinking factor 1 expression to inhibit β-catenin signaling and osteoblast proliferation. J Cell Physiol (2018) 233(7):5405–19. doi: 10.1002/jcp.26374
114. Chen Z, Zhao F, Liang C, Hu L, Li D, Zhang Y, et al. Silencing of MiR-138-5p sensitizes bone anabolic action to mechanical stimuli. Theranostics (2020) 10(26):12263–78. doi: 10.7150/thno.53009
115. Börcsök I, Schairer HU, Sommer U, Wakley GK, Schneider U, Geiger F, et al. Glucocorticoids regulate the expression of the human osteoblastic endothelin a receptor gene. J Exp Med (1998) 188(9):1563–73. doi: 10.1084/jem.188.9.1563
116. Yu D, Mu S, Zhao D, Wang G, Chen Z, Ren H, et al. Puerarin attenuates glucocorticoid-induced apoptosis of HFOB1.19 cells through the JNK- and akt-mediated mitochondrial apoptotic pathways. Int J Mol Med (2015) 36(2):345–54. doi: 10.3892/ijmm.2015.2258
117. Watanabe M, Takahashi H, Saeki Y, Ozaki T, Itoh S, Suzuki M, et al. The E3 ubiquitin ligase TRIM23 regulates adipocyte differentiation via stabilization of the adipogenic activator PPARγ. ELife (2015) 2015(4):1–26. doi: 10.7554/eLife.05615.001
118. Zhang Y, Chen Y, Sun H, Zhang W, Zhang L, Li H, et al. SENP3-mediated PPARγ2 DeSUMOylation in BM-MSCs potentiates glucocorticoid-induced osteoporosis by promoting adipogenesis and weakening osteogenesis. Front Cell Dev Biol (2021) 9:693079. doi: 10.3389/fcell.2021.693079
119. Devogelaer JP, Goemaere S, Boonen S, Body JJ, Kaufman JM, Reginster JY, et al. Evidence-based guidelines for the prevention and treatment of glucocorticoid-induced osteoporosis: A consensus document of the Belgian bone club. Osteoporosis Int (2006) 17(1):8–19. doi: 10.1007/s00198-005-2032-z
120. Sanchez-Rodriguez D, Bergmann P, Body JJ, Cavalier E, Gielen E, Goemaere S, et al. The Belgian bone club 2020 guidelines for the management of osteoporosis in postmenopausal women. Maturitas (2020) 139:69–89. doi: 10.1016/j.maturitas.2020.05.006
Keywords: osteoporosis, bone mass, exercise, cytokines, irisin, Piezo1, PCs
Citation: Chang X, Xu S and Zhang H (2022) Regulation of bone health through physical exercise: Mechanisms and types. Front. Endocrinol. 13:1029475. doi: 10.3389/fendo.2022.1029475
Received: 03 September 2022; Accepted: 15 November 2022;
Published: 07 December 2022.
Edited by:
Kok Yong Chin, National University of Malaysia, MalaysiaReviewed by:
Md. Abdul Alim Al-Bari, University of Rajshahi, BangladeshCopyright © 2022 Chang, Xu and Zhang. This is an open-access article distributed under the terms of the Creative Commons Attribution License (CC BY). The use, distribution or reproduction in other forums is permitted, provided the original author(s) and the copyright owner(s) are credited and that the original publication in this journal is cited, in accordance with accepted academic practice. No use, distribution or reproduction is permitted which does not comply with these terms.
*Correspondence: Xinyu Chang, changxinyu1230@foxmail.com; Sheng Xu, x.xusheng@163.com; Hao Zhang, zhanghsmmu@126.com
†These authors have contributed equally to this work