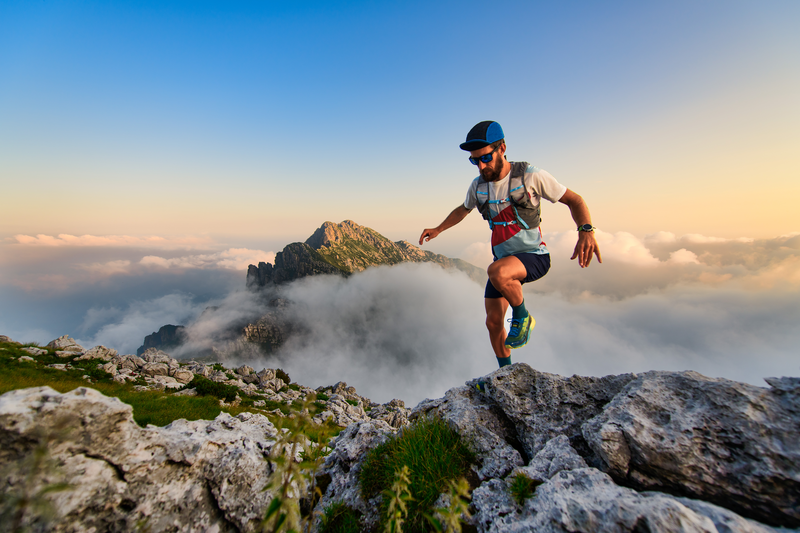
95% of researchers rate our articles as excellent or good
Learn more about the work of our research integrity team to safeguard the quality of each article we publish.
Find out more
REVIEW article
Front. Endocrinol. , 11 November 2022
Sec. Cellular Endocrinology
Volume 13 - 2022 | https://doi.org/10.3389/fendo.2022.1027237
This article is part of the Research Topic Hormonal Imbalance-Associated Oxidative Stress and Protective Benefits of Nutritional Antioxidants View all 13 articles
Type 2 diabetes mellitus is a metabolic disorder resulting from impaired insulin secretion and resistance. Dipeptidyl peptidase (DPP)-IV is an enzyme known to trigger the catalysis of insulinotropic hormones, further abating the endogenous insulin levels and elevating the glucose levels in blood plasma. In the field of drug development, DPP-IV inhibitors have opened up numerous opportunities for leveraging this target to generate compounds as hypoglycemic agents by regulating incretin activity and subsequently decreasing blood glucose levels. However, the practice of synthetic drugs is an apparent choice but poses a great pharmacovigilance issue due to their incessant undesirable effects. The ideology was set to inventively look upon different ethnomedicinal plants for their anti-diabetic properties to address these issues. To date, myriads of phytochemicals are characterized, eliciting an anti-diabetic response by targeting various enzymes and augmenting glucose homeostasis. Antioxidants have played a crucial role in alleviating the symptoms of diabetes by scavenging free radicals or treating the underlying causes of metabolic disorders and reducing free radical formation. Plant-based DPP-IV inhibitors, including alkaloids, phenolic acid, flavonoids, quercetin, and coumarin, also possess antioxidant capabilities, providing anti-diabetic and antioxidative protection. This review article provides a new gateway for exploring the ability of plant-based DPP-IV inhibitors to withstand oxidative stress under pathological conditions related to diabetes and for reforming the strategic role of ethnomedicinal plants as potent DPP-IV inhibitors through the development of polyherbal formulations and nanophytomedicines to regulate incretin activity.
Diabetes mellitus (DM) is a chronic hyperglycemic metabolic condition caused by decreased insulin production, peripheral insulin resistance, or both. According to a WHO study, diabetes was the ninth biggest cause of death in 2019, directly responsible for almost 1.5 million fatalities. Type 2 diabetes mellitus (T2DM) affects the majority of people with diabetes, accounting for more than 90% of those with diabetes, and is characterized by insulin secretion defects in pancreatic β-cells and insulin resistance, whereas type 1 DM is caused by autoreactive T cell-mediated destruction of β-cells (Figure 1) (1, 2). In reaction to food consumption, pancreatic β-cells secrete the hormone insulin. The primary function of insulin is to regulate blood glucose levels by inducing muscles, liver, and fat cells to absorb accumulated glucose from the bloodstream and store it as an energy source. T2DM, also called non-insulin DM, plays a cardinal role in the humongous populace and is triggered by an interplay of environmental and genetic factors (3). It is hallmarked by hyperglycemia and characterized by the impairment of the inositol triphosphate kinase (PI3K-Akt) pathway (metabolic arm) of insulin signaling, failing to transport glucose and synthesize glycogen and thus further leading to compensatory hyperinsulinemia to maintain euglycemia which ultimately causes insulin resistance (4, 5).
Figure 1 An overview of the impact of dipeptidyl peptidase (DPP)-IV inhibitors on the kidney and the insulin signaling pathways. The key aspects of the insulin signaling pathway, such as insulin binding, signal cascade, exocytosis, and glucose entry, are presented. DPP-IV inhibitors (DPP-IVis) are non-insulin glucose-lowering agents that are members of the incretin family. They are able to improve glycemic control while maintaining an acceptable level of safety. In individuals with chronic kidney disease, these medications are associated with a minimal risk of hypoglycemia, no weight gain, and good overall tolerability. The results of clinical trials are still up for debate, even though several experimental and clinical research point to the possibility that DPP-IVis may exert considerable pleiotropic effects, particularly on the kidneys. The figure was created with BioRender.com.
Consequently, this results in the redundant stimulation of the unaffected RAS-MAPK pathway (mitogenic arm) of insulin signaling, contributing to cardiovascular dysfunction and endothelial injury and advancing myriads of chronic diabetic complications by significantly compromising the quality of life (5). The previous studies show a growing prevalence of DM in India, triggering a shift in the onset age of DM from adult to adolescence (6). This invokes a constant need for a promising treatment to mitigate DM and the progression of chronic symptoms.
The insulinotropic effects of incretin hormones are the reason why the body produces more insulin in response to meals when glucose is ingested orally rather than when it is administered intravenously, even when the plasma glucose levels are the same. This phenomenon is referred to as the incretin effect (7). The previous studies demonstrated that gut extracts possess a hormone that tightly regulates the secretion of pancreas and was named as glucose-lowering element or incretin (INtestine seCRETtion Insulin). It was verified that diabetic patients unveil a total loss of the incretin effect. Henceforth, a hypothesis suggesting that an impaired incretin function contributes to the pathogenesis of T2DM was formulated. The two most potent incretin hormones released in response to oral glucose are glucagon-like peptide-1 (GLP-1) (Figure 1) and gastric inhibitory peptide (GIP) (7–9).
GIP is a peptide of 42 amino acids belonging to the glucagon secretin family of peptides secreted from the K-cells of the upper intestine was earlier known to inhibit gastric acid secretion, so it was called gastric inhibitory peptide, but then later, it also showcased its efficacy on the pancreas by stimulating insulin secretion glucose dependently and was renamed as glucose-dependent insulinotropic peptide (7–9). GLP1 is the second most potent incretin hormone, a peptide of 31 amino acids known to be one of the enteroglucagons synthesized from proglucagon genes while secreted from both pancreatic alpha cells as well as L cells of the lower intestine and colon, which are known to stimulate the islets of the pancreas and secrete insulin (8).
Both GLP-1 and GIP validate their functional role by binding to their specific receptors GLP-1R and GIPR, which belong to the G protein-coupled receptor family triggering adenylate cyclase activity and elevating the levels of intracellular cyclic adenosine monophosphate (C-AMP) in pancreatic β-cells with the activation of protein kinase A (PKA) and exchange protein activated by C-AMP2 (EPAC2) involved in a broad range of intracellular actions such as altered ion channel activity, elevated cytosolic calcium levels which facilitate the fusion of insulin granules to the plasma membrane, and enhanced exocytosis of insulin-containing granules, contributing to the enhancement of insulin secretion in a glucose-dependent manner (8–10). Both GLP-1 and GIP induce insulin secretion in response to oral glucose consistently with their functional role as incretins. Based on data from several studies previously, both incretins share a few common insulinotropic actions, like increasing insulin secretion, enhancing resistance to apoptosis, and increased β-cell proliferation, but also differ in a few biological attributes, like GIP stimulates the pancreas by increasing glucagon secretion and acts on the gastric tract by inhibiting gastric acid secretion, whereas GLP 1 decreases glucagon secretion and acts on the gastrointestinal (GI) tract by decreasing gastric emptying and postprandial blood glucose (8, 10).
The human gene dipeptidyl peptidase (DPP)-IV is located on chromosome 2 and encodes dipeptidyl peptidase IV, a serine protease, an enzyme located on epithelial as well as endothelial cells found to be expressed in varied tissues including the liver, gut, placenta, and kidney, causing the catalytic degradation and reduction in the half-lives of GLP-1 and GIP levels and further ensuing the perturbation of glucose homeostasis (Figure 2). From the recent investigations done, it was reported that 75% of GLP-1 metabolism by DPP-IV and elimination occurs in the gut and liver, respectively, permitting only 10–15% of GLP-1 circulation in the blood (8, 10). In order to augment the insulinotropic activity in blood plasma, various synthetic and herbal DPP-IV inhibitors have gained much focus as oral hypoglycemic agents with minimal side effects (7, 11, 12).
Figure 2 Model of the mode of action of dipeptidyl peptidase (DPP)-IV inhibitors for augmenting incretin activity. In healthy individuals, food intake aids the secretion of incretin hormones from the alimentary canal, which further favors the synthesis and release of insulin and additionally arrests the synthesis and release of glucagon, subsequently maintaining the glucose levels in blood plasma. However, type 2 diabetes mellitus patients incur a loss of incretin activity by the catalytic action of the DPP-IV enzyme, which is abated by DPP-IV inhibitors in order to escalate the incretin levels for regulating glucose homeostasis. .
Various naturally available plant-based compounds are investigated as DPP-IV inhibitors. Different plant parts such as leaves, seeds, flowers, buds, and bulbs are analyzed to identify their inhibitory capacity against DPP-IV activity. Parts of the plants were found to have different IC50 values against DPP-IV. The distinction arises from the fact that diverse phytochemicals, such as glycosides, flavonoids and phenols, terpenoids, and stilbenoids, are located in different parts of various plants. Numerous bioactive peptides derived from various plant components are likewise highly efficient against DPP-IV activity (Figure 3).
Figure 3 Levels of dipeptidyl peptidase (DPP)-IV activity inhibition concentration of bioactive compounds in certain plants studied. Different inhibitory concentrations of the plant products are found for the inhibition of DPP-IV (13–28). In (A), the inhibitory concentration is evaluated in μM, while in (B) the inhibition concentrations are emulated in μg/ml.
Many phytochemicals, including some that specifically target the DPP-IV enzyme, can be found in the leaves of plants. The leaves contain the most DPP-IV inhibitory compounds compared with other parts of the plant. To identify DPP-IV inhibitory compounds, the leaves of various plant orders, including Myrtales, Fabales, Sapindales, Gentianales, Urticales, Ericales, and Liliales, have been studied (Figure 3)—for example, the leaves of different plants from the order Myrtales, such as Psidium guajava (29), Eucalyptus globulus, Eucalyptus citriodora, and Eucalyptus camaldulensis (30), have IC50 values against DPP-IV activity of 380, 3.098, 6.138, and 3.99 μg ml-1, respectively. Similarly, the IC50 value against DPP-IV activity in the leaves of Cicer arietinum, Pterocarpus marsupium, and Punica Granatum belonging to order Fabales are 0.09 (31), 273.73 (29), and 0.19 μg ml-1 (32), respectively. Aegle marmelos, Mangifera indica, and Melicope glabra, which belong to the order Sapindales, have an IC50 value against DPP-IV activity of 790, 182.7, and 169.40 μg ml-1 (33–35), respectively. The IC50 value was 96.8 µM (36) and 773.22 μg ml-1 (16) in a few other plants such as Apocynum cannabinum and Gymnema sylvestre from the order Gentianales. Similarly, for several other plants such as Morus alba and Pilea microphylla (order Urticales), Aloe vera (order Liliales), Camellia sinensis (order: Ericales), and Urena lobate (order: Malvales), the recorded IC50 value against DPP-IV activity was 480 (37), 520.4, 2.716, 227, and 1,654.64 μg ml-1, respectively (37–39). These values show that the leaves of many plants have a very important role in inhibiting DPP-IV activity.
The leaves of Fagopyrum esculentum and Rheum palmatum (order: Chenopodiales) containing rutin and emodin were found to have IC50 values of 485 µM (27) and 5.76 µM (40), respectively. Fan et al. (2013) (41) documented that the leaves of Camellia sinensis from the order Ericales have gallic acid and epigallocatechin gallate having IC50 values of 4.65 and 10.21 µM, respectively. Against DPP-IV activity, the leaves of Psoralea corylifolia (order: Fabales) contain genistein, Coffea arabica (order: Gentinales) contains caffeic acid, and Brassica oleracea (order: Brassicales) contains luteolin with IC50 values of 0.48, 3.37, and 0.12 M, respectively (41). Similarly, Bower et al. (2014) (14) observed that the leaves of Rosmarinus officinalis and Lippia graveolens (order: Lamiales) have cirsimaritin with IC50 value of 0.43 and 10.9 µM, respectively, which can be used for inhibition of DPP-IV activity.
The seeds of various plants belonging to different orders, such as Castanospermum austral, Cicer arietinum, Trigonella foenum graceum (order: Fabales), Avena sativa, Hordeum vulgare var. trifurcatum (order: Graminales), Amaranthus hypochondriacus (Order: Chenopodiales) Eugenia jambolana (order: Myrtales), Fagopyrum esculentum (Order: Polygonales), Ferula assa-foetida (order: Apiales), and Prunus amygdalus (order: Rosales), were documented to have IC50 concentration against DPP-IV activity of 13.96 (42), 0.09, 0.03 (31), 0.99, 1.83 (43), 1.1 (44), 278.94 (32), 1.98 (43), 24.5 (45), and 162.9 μg ml-1 (46), respectively. Kim et al. (2018) (47) also examined that the seeds of Lens culinaris (order: Fabales) have different compounds such as kaempferol-3-O-βgulcopyranosyl-(1→2)-βgalactopyranosyl-7-Oαrhamnopyranoside, kaempferol-3-O-βgulcopyranosyl-(1→2)-[αrhamnopyranosyl(1→6)]-βgalactopyranosyl-7-O-αrhamnopyranoside, robinin, and kaempferol with IC50 value against DPP-IV activity of 27.89, 36.52, 37.01, and 51.9 µM, respectively.
Several additional plant parts have been identified to have compounds with DPP-IV activity-inhibiting properties. The aerial parts of Hedera nepalensis (order: Apiales), Fagonia cretica (order: Zygophyllales), and Desmodium gangeticum (order: Fabales) have IC50 value against DPP-IV activity of 17.2 (19), 38.1 (19), and 255.5 μg ml-1, respectively. Similarly, the IC50 values for Helichrysum arenarium (order: Asterales) flowers, Berberis aristate (order: Ranunculales) bark, and Anogeissus latifolia (order: Myrtales) were reported as 41.2, 14.4, and 754 μg ml-1 (34, 48), respectively, for DPP-IV inhibition. The bulb of Allium sativum (Order: Asparagales) was likewise documented with an IC50 value of 70.9 μg ml-1 (49), while the fruits of Schisandra chinensis (Order: Austrobaileyales) and Punica granatum (Order: Fabales) were recorded with an IC50 value of about 10.8 μg ml-1 (31, 32) against DPP-IV activity.
Saleem et al. (2014) and Kalhotra et al. (2018) (19, 49) noticed that lupeol, present in the aerial parts of Hedera nepalensis (Order: Apiales), has an IC50 value of 31.6 µM, but malvidin, found in the aerial parts of Anagallis monellin (Order: Ericales), has an IC50 value of 1.41 µM against DPP-IV activity. The stems and roots of Coptis chinensis (Order: Ranunculales) contain berberine, which exhibits DPP-IV activity with an IC50 value of 14.4 μg ml-1 (13). The whole Pilea microphylla (Order: Rosales) plant contains the active ingredient isoquercetin, which has an IC50 value of 96.8 µM against DPP-IV activity (50). Lin et al. (2015) (28) identified hopeaphenol, vitisin A, and vitisin B in the stems and leaves of Vitis thunbergii that inhibited DPP-IV activity with IC50 values of 401, 90.75, and 15.3 µM, respectively. Similarly, Fan et al. (2013) (41) found apigenin in the stems and pods of Acacia auriculiformis (order: Fabales), which had an IC50 value of 0.14 µM, in the fruits of Citrus aurantium, Citrus limon, and Citrus maxima belonging to order Sapindales having hesperetin (with IC50 value of 0.28 µM), eriocitrin (IC50 value of 10.36 µM), and naringenin (IC50 value of 0.24 µM), respectively. They also recorded that the fruits of Rubus fruticosus belonging to order Rosales have cyanidin (IC50 value 1.41 µM) that acts as a DPP-IV inhibitor. The compound cyanidin-3-glucoside present in Vaccinum corymbosum (order: Ericales) has an IC50 value of 125.1 µM against DPP-IV activity (26).
It has been demonstrated that several bioactive peptide sequences found in a wide variety of plants are effective against DPP-IV activity. In Phaseolus vulgaris, Mojica et al. (2017) (18) identified peptide sequences such as KTYGL, KKSSG, GGGLHK, and CPGNK, each of which had an IC50 value of 0.03, 0.64, 0.61, and 0.87 mg DW ml-1, respectively. Similarly, Lammi et al. (2016) (17) reported that the IC50 value for the peptide AVPTGVA in Glycine max and LTFPGSAED in Lupinus albus was 106 and 228 µM, respectively. Wang et al. (2015) (43) determined that the peptide LQAFEPLR present in Avena sativa has an IC50 value of 103.5 µM. Harnedy et al. (2015) (15) found that ILAP and LLAP present in Palmaria palmata had IC50 values of 43.40 and 53.67 µM, respectively. The details about these plants are specified in Section 9.
With an ideology to work on the crux of the matter, DPP-IV to manage diabetes, orally active small molecules called DPP-IV inhibitors have already been introduced in the market since 2006 (51). From previous investigations, a therapeutic dosage of different authorized DPP-IV inhibitors led to a two- to threefold raise in endogenous GLP-1 concentration without any inherent hypoglycemia risk and a favorable safety profile (Figure 2). Synthetic authorized drugs in the market as DPP-IV inhibitors are known as gliptins such as sitagliptin, linagliptin, and saxagliptin, usually substantiated to be efficient competitive inhibitors. In order to address the crisis imparted by DPP-IV, sitagliptin (Merck) was the initial lead to embark on the journey towards the upregulation of GLUT-4 expressions in the skeletal muscles of spontaneously hypertensive rats by controlling the postprandial glucose concentration and glycated hemoglobin (52). With an appropriate drug administration, gliptins can prove their efficacy for 24 h (53). Moreover, from the pharmacovigilance studies done priorly, gliptins were able to curtail the risk of hypoglycemia and weight loss and additionally also had the potential to proliferate β-cell mass.
Variously targeted (GLP-1) and off-targeted substrates lead to DPP-IV inhibition, contributing to managing incretin activity and maintaining the basal blood glucose level (54). Lately, these oral hypoglycemic agents, because of their insulinotropic effect, have progressively replaced sulfonylurea as second-line therapy and are also endorsed in the guidelines in triple therapies along with metformin and SGLT-2 inhibitors (55–57). For attaining the benchmark among all the other synthetic drugs, structural backbones ranging from cyanopyrrolidines, triazopiperazine amides, and pyrrolidines highly influenced the pharmacodynamics of each commercialized gliptins (58). The pharmacodynamics of each commercially authorized gliptin has been summarized in Table 1 (54, 59).
Data are partially retrieved from the approved list of DPP inhibitors by the US Food and Drug Administration (FDA) (60). FDA has underlined the adverse effects of DPP-IV inhibitor drugs such as Januvia, Janumet, Janumet XR, Onglyza, Kombiglyze XR, Tradjenta, Glyxambi, Jentadueto, Nesina, Kazano, and Oseni with active compounds sitagliptin, sitagliptin and metformin, sitagliptin and metformin extended release, saxagliptin, saxagliptin and metformin extended release, linagliptin, linagliptin and empagliflozin, linagliptin and metformin, alogliptin, alogliptin and metformin andalogliptin, and pioglitazone, respectively (60).
The previous studies revealed the most common adverse effects in patients, including infections in the respiratory passage, nasopharyngitis, headache, and bladder infections associated with sitagliptin and saxagliptin, respectively (61, 62). Moreover, cases of hypoglycemia were also encountered with the synergistic intake of sitagliptin and saxagliptin. From analyzing the pharmacovigilance report and data supported by FDA, it was proclaimed that, on intake of DPP-IV inhibitors, several patients were diagnosed with hypersensitivity and joint pain issues, and in some cases, these drugs have proven to be fatal for patients with a history of pancreatitis (7, 56, 61, 63).
On a wider scale, strong aversions were laid against incretin-based therapy regarding its cost-effectiveness and the increasing risk of morbidity in patients with cardiovascular issues (64). With an ideology to surpass the discredits, investigators have inclined toward plant origin to discover innovative therapies to combat glycemia and the emergence of severe morbidities associated with the same.
Since antiquity, many traditional practitioners across the globe have assertively exploited natural flora, which has proven superlatively therapeutic over synthetic agents to regulate several pathological conditions. Botanical leads, being a repository of bioactive compounds, fostered the strategic role of pharmacognosy (65). Metformin, a commercially effective anti-diabetic medicine, was recently identified in the field of drug discovery by adapting the concept of pharmacognosy and establishing the foundation for formulating herbal products to inhibit DPP-IV and regulate incretin activity (7, 66, 67).
Oxidative stress (OS) has been linked to the onset and progression of diabetes and its many consequences resulting from insulin insufficiency or insulin resistance (68). Several clinical studies have demonstrated that T2DM reduces antioxidant status and free radical scavenging activity due to the decreased activities of superoxide dismutase (SOD), catalase (CAT), and glutathione peroxidase (GPx) and ascorbate and vitamin E levels, increasing the likelihood of diabetic patients acquiring chronic OS (68). Several different molecular mechanisms such as protein modifications, including oxidant-induced modifications in phosphorylation state, alterations in gene regulation including transcriptomic and transcriptional modifications with the affected proteins being either direct insulin signaling molecules or oxidant-sensitive signaling pathways that interfere with the insulin signaling cascade, have been postulated to contribute to reactive oxygen species (ROS)-induced insulin resistance (69). Excessive levels of ROS inhibit insulin gene expression and insulin production as well as damage islet tissue. Anti-diabetes effects can be enhanced using plant-based DPP-IV inhibitors, such as alkaloids, phenolic acid, flavonoids, quercetin, and coumarin, possessing antioxidant characteristics (70, 71).
Antioxidants derived from plants, such as kinsenosides and flavonoids, exhibit anti-diabetic and antioxidant properties and assist in maintaining the function of pancreatic β-cells in vivo (71, 72). The majority of studies examining the role of plant-derived antioxidants in protecting β-cells focus on flavonoids. The administration of flavonoids to diabetic animals boosts the antioxidant potential of β-cells by increasing both enzymatic and non-enzymatic antioxidants, consequently limiting ROS generation and lipid peroxidation in β-cells and protecting them against autophagy, apoptosis, or necroptosis (73, 74). Diabetes is associated with an increase in the expression of pro-apoptotic genes (e.g., caspases) and a decrease in the expression of anti-apoptotic genes (e.g., Bcl-2 proteins) in β-cells. Flavonoids have been proven to protect β-cell viability by limiting these gene expression changes (Figure 4) (71, 75).
Figure 4 Role of dipeptidyl peptidase inhibitors with antioxidant properties in protecting pancreatic β-cells and treating diabetes. GLP-1, glucagon-like peptide-1; GIP, glucose-dependent intestinal polypeptide; OS, oxidative stress.
Flavonoids, which include flavanols, flavonols, flavones, flavanones, isoflavones, and anthocyanins, also possess DPP-4 inhibitory activity—for instance, the flavonoid-rich fraction of Pilea microphylla displayed antidiabetic efficacy in rats with diabetes generated by a high-fat diet and streptozotocin by lowering DPP-IV. This was accomplished while simultaneously increasing the endogenous antioxidant status in the liver of mice (38). Similarly, supplements derived from citrus bioflavonoids suppress the DPP-4 enzyme and also have higher free radical scavenging capabilities (27, 76). It has also been reported that an ethanolic extract of the leaves of Psidium guajava contains seven major flavonol-glycosides, all of which inhibit DPP-IV in a dose-dependent manner (29). Additionally, it was demonstrated through an in vitro bioassay that three flavonol glycosides extracted from the seeds of Lens culinaris possessed DPP-IV inhibitory activity in a concentration-dependent manner (47). Antioxidants derived from plants with antidiabetic qualities, such as acting as DPP-4 inhibitors, are regarded to be the most effective strategy for keeping a normal β-cell physiology and treating diabetes (77). The following sections address plants that have DPP-4 inhibitor actions, either with or without known antioxidant properties.
The effectiveness of the DPP-IV inhibition offered by a variety of tropical plants is being evaluated. In addition, in prior studies, a wide variety of organic solvents, plant parts, and extraction procedures were employed to isolate different bioactive components. The percentage yield of the extracts and the number of bioactive compounds are profoundly influenced by the polarity of the organic solvents (39, 78).
Recent studies on the root and the aqueous leaf extract of U. lobata reported its anti-diabetic competency in streptozotocin-induced diabetic rats, in compliance with the fact that the polarity of the organic solvent affects the solubility of bioactive compounds and its antioxidant property, which is supported by in vitro and in vivo generated results (79, 80). As a result of the research performed in vitro, the findings of the alcoholic extract portrayed its inhibition efficacy by expressing an IC50 value of 1,654.64 µg/ml. This value demonstrated that the alcoholic extract was four times more effective than the aqueous extract, which had an IC50 value of 6,489.88 µg/ml. However, the in vitro inhibitory activity of standard vildagliptin was superior to both extracts (IC50 = 57.44 µg/ml) (39, 78). In vivo, however, pharmacokinetic indices indicated that the aqueous extract was more effective than the alcoholic extract because water positively influences the absorption of active compounds in the serum by synergistically forming a complex and enhancing the inhibition efficacy against DPP-IV as well as the bioavailability of GLP-1 in the serum (81). Because the extracts contain sterols, which are known to alter the conformational structure of active compounds and subsequently reduce the bioactivity within the gastrointestinal (GI) tract, the alcoholic extract displays a lower level of in vivo inhibitory activity (39, 82, 83).
From the in vivo studies undertaken previously using P. tuberose methanolic root extract (PTME) as a potential herbal treatment on the liver homogenates of alloxan-induced diabetic rats, it was reported that PTME possessed an abundance of flavonoids and significantly reduced DPP-IV activity in a time-dependent manner from 16 DPP-IV activity in a time-dependent manner fromto 4 μM/unit as compared with alloxan control (17.5 μM/unit) within a period of 40 days, which proved that PTME was a promising candidate to cure DPP-IV-induced liver disorders (84). From another investigation done, the aqueous extract was found to be potent enough to upregulate antioxidant SOD levels and downregulate DPP-IV mRNA expression, which apparently led to a concomitant reduction in stress, changes in the structural complexity of villi, increase of the intestinal patch, subsequent increase of the rate of nutrient absorption, and augmentation of GLP-1 and GIP secretion by causing an enhanced intestinotrophic effect (85). Besides this, in vitro results also supported the data as a potential DPP-IV inhibitor by exhibiting a considerable IC50 value of 17.4 mg/ml. In addition, when in vivo studies were undertaken on glucose-fed rats administered with the aqueous extract, the plasma glucose concentration during 60 min was reduced by 27.68%, and plasma DPP-IV activity was reduced by approximately onefold as compared with untreated rats. Additionally, a sharp rise of 1.2-fold was also observed in the plasma GLP-1 concentration compared with the untreated control (84).
As one of the prospective candidates, W. coagulans was chosen, and its effectiveness was checked using root, leaf, and fruits with 100 and 80% methanol as an organic solvent. Among all the plant parts, a root extract of the mature plant with 100% methanol exerted the maximum 50% inhibition efficacy against DPP-IV at a concentration of 8.76 µg/ml, whereas 80% methanol expressed its 50% inhibition at a concentration of 21.03 µg/ml. However, both extracts showed lesser efficacy than diprotin, the standard drug expressing its 50% inhibition efficacy at a concentration of 4.13 µg/ml (86).
Recent findings have unveiled that the alcoholic extract of C. mukul gum resin with an abundance of antioxidants significantly proclaimed its antihyperglycemic activity by modulating the key glucose-metabolizing enzymes of the liver and kidney and further promoting normal blood glucose homeostasis (87–89).
With an ideology to get the maximum yield, optimization of procedure was an essential need which was undertaken by microwave-assisted extraction (MAE) method and conventional Soxhlet extraction (CSE) method resulting from giving a yield of 2.5%–3% and 2%, respectively, by using ethyl acetate as the solvent of interest (90). MAE proved to show a better yield than the Soxhlet extraction method because it was found to be less laborious, required less solvent, with improved quality of extract, and more economical (91). Moreover, by taking Hydro alcohol (HA) extract into practice for obtaining in vitro results, the maximum inhibitory activity imparted by C. mukul was 92%, with an IC50 value of 17 µM.
When experiments were undertaken by choosing F. asafoetida to assess DPP-IV inhibition activity using methanol, ethanol, water, and methanol–ethanol as organic solvents, it was observed that, among all the fractions, ethanol and ethanol–methanol fractions showed a maximum inhibitory effect with 24% and 22%, respectively (45).
By choosing Desmodium gangeticum as one of the potential DPP-IV inhibitors, it was noted that the aqueous extract exhibited 73.21 % inhibition at 1,000 µg/ml concentration with an IC50 value of 255.5 µg/ml, which was inferior concerning its efficacy when compared with the standard drug diprotin expressing IC50 =10 µg/ml by 78.3% (92).
According to the data, the leaf extract of Moringa oleifera was considered one of the potential candidates with significant antihyperglycemic activity, particularly in the ethanol and ethyl acetate extracts (93). Recent findings suggested that the extracts successfully reduced the blood glucose levels, increased the insulin levels, decreased the inflammatory cytokines and HOMA-R values, and improved the PPAR gamma levels. Nevertheless, the extract failed to prove its inhibition efficacy against DPP-IV when a set of in vivo experiments was performed (94). However, by undergoing ADMET analysis to evaluate the pharmacokinetic indices, myriads of different compounds from the extract were screened. Seven out of all could make it through in silico analysis by undergoing molecular docking, and just one compound with a specific conformation that exhibited the highest inhibitory activity with maximum binding energy was selected. Its in vitro evaluation was also undertaken, where it responded with an IC50 value of 798 nM (95).
Recent data has also reported Morus alba (also called white mulberry) as a potential DPP-IV inhibitor. MAE was used to obtain the extract from dried stem bark using ethanol with and without hydrolyzed acid. In addition, it was observed that the proportion of bioactive compounds in the Morus alba ethanolic extract without acid hydrolysis was only 0.04% but that the percentage of these compounds increased to 0.16% when the extraction was carried out with acid hydrolysis (96). Furthermore, previous research findings suggested that the compounds from M. alba root bark and fruits have also been reported to have anti-diabetic effects on STZ-induced mice by stimulating insulin secretion but lack the bioactive compounds which could exert DPP-IV Inhibition (97–100). The experiments were conducted to check the efficacy of stem bark as a DPP-IV inhibitor, wherein it showed a considerable inhibitory activity of 23%, which was 0.33 times the inhibitory activity of the standard drug sitagliptin (96).
Pterocarpus marsupium was selected to treat hypoglycemia by utilizing various parts of the plant, and the DPP-IV inhibitor activity of the plant was investigated in both in vitro and in vivo conditions. Based on the findings, it was possible to determine an IC50 value of 273 µg/ml. In addition, the experimental conditions impacted the inhibitory half-life of the enzyme, which was 462.3 min, whereas the in vivo studies also reported that the extract could successfully increase the GLP-1 levels compared with the control group; the highest peak for GLP-1 was detected within 2 h (32).
As one of the potential options for combating hyperglycemia and hyperinsulinemia, the Hypoxidaceae family member C. latifolia has been selected. The root and the fruit extracts were prepared using the subcritical water extraction method. Both extracts expressed inhibitory potential against DPP-IV, and from the results, it was portrayed that the root extract (66.15%) exhibited better inhibition against DPP-IV than the fruit extract (42.79%).
In the latest studies, the anti-diabetic and antioxidant potential of M. latifolia has been demonstrated, and the bark extract obtained from the plant has been investigated using various solvents. Methanol showed the highest yield out of all the evaluated extracts. Moreover, the in vitro results depicted that chloroform extract depicted the maximum inhibitory strength against DPP-IV (IC50 value = 221.58 µg/ml), whereas the hexane extract expressed the minimum inhibitory potential.
Poor pharmacokinetic characteristics are a common reason many drugs never make it to the market (101) (Table 2). Therefore, it is crucial to develop lead compounds that are easily absorbed orally, efficiently delivered to the site of action, not readily converted into harmful metabolic products en route to the site of action, and rapidly excreted from the body. The acronym ADMET is commonly used to describe the aforementioned characteristics (absorption, distribution, metabolism, excretion, and toxicity). Although Lipinski's “rule of five" (106) is an early version of an integrated approach, its parameters should be used for guidance rather than as strict cutoffs. This mnemonic predicts that poor absorption or permeation is more likely when there are more than five hydrogen-bond donors and more than 10 hydrogen-bond acceptors, the molecular weight is greater than 500, and the calculated partition coefficient (logP) is greater than five. The same holds for the methods used to deal with problems caused by specific ADMET attributes (107).
Table 2 Inhibitory role of different tropical plant extracts against dipeptidyl peptidase-IV enzyme.
A broad variety of bioactive compounds have been identified through the use of various plants and various ways of extraction. In addition, over the course of time, certain novel bioactive compounds have been explored for their potential as DPP-IV inhibitors through the use of in silico analysis (Figure 5). With the aid of advanced computational biotechnological studies, scientists can foretell the potential drug candidates in the pharmaceutical industry. New ligands are needed for the targets of known structure, wherein the potential compounds are screened for their adequate efficacy against a target of interest, and also the therapeutic dosage approval is possible by analyzing the ADMET properties to be able to abide by the Lipinski rule and investigate the drug likeliness of the compound (108, 109). The score of the free binding energy is directly proportional to the binding affinity. The binding energy with a lower value reinforced the synthesis of a strong binding molecule by exhibiting potential biological activity and binding orientation field (109, 110).
Figure 5 General mechanism of molecular docking. With a view to check the inhibition efficacy of different phytocompounds against the dipeptidyl peptidase (DPP)-IV putative binding site, in silico analysis can be performed by exploring various software, and in accordance with the same, different binding scores and orientations can be known, which are further projected towards molecular dynamics simulation. The ribbon structure of the human DPP-IV and the molecular structure of the ligand were downloaded from the protein data bank of PubMed and PubChem, respectively. The molecular interaction figure is recited after Kim et al. (2018) (47) under creative common license attribution.
By generating an ensemble of ligand conformers that are subsequently firmly docked into the binding site, fast rigid exhaustive docking (FRED; part of the OpenEye Scientific Software) enables ligand conformational flexibility. Fast computations are possible with FRED, which is useful when screening libraries containing hundreds of thousands of molecules (111). A FRED docking study indicated that inhibition of DPP-IV is one of the mechanisms by which berberine exerts its hypoglycemic impact (13). Amini et al. (2016) performed molecular docking experiments and modeled the DPP-IV inhibitory activities of a variety of new aminomethyl-piperidones using a quantitative structure–activity relationship (QSAR) technique (112). This paper demonstrates the utility of a hybrid docking-QSAR approach to the study of ligand–protein interactions. In another study, three of the sugars found in fructooligosaccharides (i.e., 1-kestose, nystose, and 1-β-fructofuranosyl nystose) are powerful inhibitors of dipeptidyl peptidase-IV as determined by molecular docking with Gold and Glide software (113). Furthermore, with the results of the isobologram and combination index analysis, the synergistic effects were also evaluated showing nystose and panose combinations having the greatest synergistic effects (114). In a recent report, the interaction of flavonoids with DPP-IV has been studied elaborately (115). Among a panel of 70 structurally different flavonoids that were tested for their inhibitory properties, myricetin, hyperoside, narcissoside, cyanidin 3-O-glucoside, and isoliquiritigenin exhibited concentration-dependent stronger inhibitory effects against DPP-4. Furthermore, fluorescence quenching studies revealed that these five flavonoid molecules could efficiently suppress the intrinsic fluorescence of DPP-4 by forming an unstable complex through spontaneous binding. While myricetin's complex with DPP-4 was mostly stabilized by hydrogen bonds and van der Waals forces, electrostatic forces may play a significant role in maintaining the complexes of the other four flavonoids with DPP-4. AutoDock 4.2 software was used to perform the molecular docking simulation, which further confirmed the binding interactions between DPP-4 and the five flavonoids chosen. The binding sites of hyperoside, narcissoside, cyaniding 3-O-glucoside, and isoliquiritigenin were found within the active site cavity of DPP-4, while myricetin's binding site was found in a minor cavity close to the active pockets (115).
Most of the flavonoids and terpenoids in the herbs have potency as antioxidants (116), antiseptics, and anti-inflammatory, and sterols are known for their anti-inflammatory property, but the pharmacological influence of alkaloids is difficult to predict because of the presence of many biological activities. Anti-diabetic herbs have many active compounds which could either work synergistically or antagonistically (117).
To reduce the pathogenesis of diabetes and its complications, flavonoids and polyphenolics have played an extremely significant role by potentiating GLUT-4 (Figure 1) isomer expression and uptake (118). Flavonoids portray their characteristic antidiabetic potency by promoting carbohydrate digestion, insulin signaling, secretion, glucose uptake, and adipose deposition (119). From the previous findings, a strong notion was developed that the phytocompounds have a potency to regulate GLUT-4 translocation through insulin signaling pathways, namely, the PI3K-Akt pathway and AMPK-dependent pathway (120). Moreover, even the phenols trigger the secretion of GLP-1 from the L cells of the large intestine, causing the elevation of the half-life of GLP-1 and the inhibition of DPP-IV enzymatic activity (121). Synergistically, many phytocompounds also showcased their antioxidant and anti-inflammatory effect by suppressing oxidation and inflammatory signaling pathways and further preventing the risk of developing diabetes-related complications.
The flavonoids obtained from the water and ethanolic leaf extract of U. lobata were chrysoeriol and gossypetin. Chrysoeriol is a flavone exhibiting anti-inflammatory properties, found to be soluble in water and alkali solution, and after undergoing liquid chromatography (LC)–mass spectrometry (MS), this bioactive compound was found to be present in both the ethanolic and aqueous leaf extracts, which were further explored by undergoing molecular docking to check its inhibition activity as a ligand against the enzyme DPP-IV, and its efficacy was proven by portraying the binding energy of -4.6 kcal/mol (78). Besides this, gossypetin was the other prominent flavone identified in the aqueous and ethanolic leaf extracts of U. lobata by undergoing LC–MS screening, and the inhibition activity of this bioactive compound was investigated by calculating the free binding energy, which was -5.20 kcal/mol (78).
Two of the most potent flavonoids, puererone and robinin, were obtained by undergoing HPLC–MS analysis from the aqueous root extract of P. tuberose, which was exploited through computational studies to investigate their potency as DPP-IV inhibitors. Both flavones identified were competitive inhibitors of DPP-IV as they docked directly into all the three putative active sites of DPP-IV by exhibiting direct interaction, hydrogen bonding, and pi–pi interaction to substantiate the strong binding affinity and inhibition activity towards DPP-IV by possessing free binding energies of 7.543 and 7.376 kcal/mol, respectively, which further validated the efficacy of PTWE (84).
To select a potent phytochemical for the development of a plant-based DPP-IV inhibitor, the fruit extract of W. coagulans was screened and subjected to computational analysis, and from the analysis, hydrogen bonding was analyzed for various bioactive compounds against the target enzyme. In accordance with the same, even the binding energies of the competent compounds were detected to range from -7.2 to -9.8 kcal/mol, further inhibiting the target enzyme irreversibly. Among all the phytocompounds screened, sitoindoside IX showed the maximum inhibitory activity (-9.8 kcal/mol), which was even higher than the standard drug sitagliptin (103).
To analyze the DPP-IV inhibitory activity of Ferrula asafoetida methanolic seed extract, 12 different bioactive compounds were isolated and characterized by undergoing gas chromatography (GC)–MS analysis; they were further screened to examine their inhibitory role by going through docking studies using AUTODOCK software. Among the 12 different bioactive compounds, four bioactive compounds—namely, hexadecanoic acid, methyl tetradecanoate, ethoxy-disilane, and 9,12-octadecadienoic acid—were found to be potent enough and can be used for in vivo studies (122).
Among different plants, even the seeds of Moringa oleifera could show its efficacy as a DPP-IV inhibitor when being addressed to different docking programs. This resulted in generating 23 different competent compounds as ligands to develop compatible configurations. This investigation revealed that seven out of 23 candidate compounds had higher LibDock scores than the standard vildagliptin. These seven were further subjected to CDOCKER program, and three of them showed the best potency as DPP-IV inhibitors by possessing binding energy better than vildagliptin. Among the three, compound 1, a unique urethane O-ethyl-4-[L-rhamnosyloxy) benzyl] carbamate, possessed the highest binding energy (-84.99 kcal/mol) (95).
Five different bioactive compounds were detected from the methanolic leaf extract of Gynura bicolor, which were characterized using HPLC and LC–MS analysis and subjected to docking to identify the compounds with strong binding efficacy. Among the evaluated bioactive compounds, 3-caffeoyl quinic acid showed the highest binding energy (-29.07 kJ/mol), which was higher than sitagliptin (-25.04 kJ/mol), linagliptin (-27.57 kJ/mol), and saxagliptin (-23.45 kJ/mol) and subsequently proved to be a promising inhibitor of the enzyme to regulate incretin activity (123).
By evaluating the in vitro activity of Curcligo latifolia, fractionation was performed, and five different bioactive compounds were obtained, which were then analyzed for their drug-like properties; phlorizin exhibited the highest binding energy (-10.9 kcal/mol), which was higher than that of the reference drug sitagliptin (-8.6 kcal/mol) (104), whereas the bioactive compounds of Melicope latifolia were isolated from chloroform extract by undergoing LC–MS. It was observed that, among the four different compounds, p-coumarate featured the maximum binding efficacy towards the target molecule (-5.7 kcal/mol) (105).
It has been discovered that several mechanisms in the treatment of diabetes effectively control the progress as well as alter the deteriorating condition of patients. Conventional medicines have been discouraged due to their adverse effects and withdrawal symptoms. In lieu of getting diabetes control, several herbal mixtures have been estimated and analyzed for treating diabetic patients. A traditional therapeutic herbal strategy has been practiced for the past many years. Furthermore, this strategy also confers its maximum effectiveness due to the synergistic action of several herbs to achieve better potency as compared with that of an individual herb, and this gave rise to the mechanistic concept of polyherbalism or polypharmacy, which could be looked at as one of the comprehensive approaches to address diabetes and its emerging complications (85, 124–126).
Moreover, nanoformulations could be used to address the limitations of herbal medicinal products, such as low stability and restricted absorption, which hinder their development as medicinal agents (127). Extracts from plants and isolated phytochemicals have been nanoformulated in a variety of ways to improve their therapeutic efficacy as nanoformulations and are proven to possess superior characteristics compared with the respective plant extracts or isolated phytochemicals. Prior research on peptides obtained from oat globulin revealed potent DPP4-inhibiting activity (128). Solid lipid nanoparticle-embedded oat globulin peptides were reported by Su et al. (2020) as stable and being able to maintain their capacity to inhibit DPP4, while non-embedded peptides suffered secondary hydrolysis by proteases in gastrointestinal fluids and lost their inhibitory effects (129). In a glucose-induced diabetic zebrafish model, Eysenhardtia polystachya (EP)-loaded silver nanoparticles (AgNPs) were likewise reported to boost pancreatic β-cell survival and insulin secretion-enhanced hyperglycemia. In addition, EP-AgNPs restored insulin production in insulinoma cell line (INS-1; an established model for studying the function of pancreatic islet β-cells) cells induced by H2O2, indicating that this may be due to cytoprotection against oxidative damage (130). Bark methanol/water extract from EP contains bioactive components including chalcones, flavonoids, and dihydrochalcones, which play a determining role in the phytofabrication of AgNPs (130).
Compared to the control Chang liver cells, glucose absorption was enhanced by the Leonotis leonurus extract-nanostructured lipid carriers (NLCs) (131). Under hyperglycemic conditions, INS-1 cells exposed to the NLCs released more insulin than the control untreated cells and the unformulated-extract-treated cells (131). A rise in the ATP/ADP ratio results from glucose metabolism in β-cells, which triggers insulin release. Increased intracellular Ca2+ stimulates insulin secretion by closing ATP-sensitive K+-channels, depolarizing the cell membrane, and activating voltage-gated Ca2+-channels (132). It has been demonstrated that a component derived from the Leonotis sibiricus plant can boost insulin production in INS-1E cells while simultaneously promoting cell proliferation (133). It is possible that the rise in insulin release caused by the extract NLC is attributable to the formulation's small particle size and high bioavailability (134), both of which lead to efficient cellular absorption, which is necessary for the effect to be achieved.
Paul et al. (2014) described an α-eleostearic acid (ESA)-rich nanoemulsion (NE) formulation and its anti-diabetic and antioxidative effects (135). Bitter gourd seed oil (BGO) contains 30%–50% ESA (136), whose antioxidative activity in scavenging ROS has been described (137). In plasma and liver homogenate fractions, the antioxidative defense system of rats with alloxan-induced T2DM was considerably impaired compared with the normal control group. Evidently, the administration of synthesized BGO-NE alleviated the oxidative stress state by activating the antioxidative defense mechanism (as demonstrated by an improvement in GPx, SOD, and CAT activities) (135).
For decades now, it has been established that endothelial dysfunction and, ultimately, atherosclerosis are directly linked to the increase in oxidative stress that occurs during the progression of diabetic hyperglycemia. According to prior research, plasma antioxidants, including tocopherol, carotene, lutein, lycopene, retinol, and ascorbic acid, decline significantly in diabetes (138). Many phytochemicals are utilized to treat various diseases, and they play a crucial role in the fight against free radicals by targeting myriads of enzymes in several metabolic pathways.
Recent studies show that quercetin and resveratrol are used to increase insulin sensitivity, whereas beta-glucans and basic acids have been clinically used to stimulate glycogen synthesis and gluconeogenesis. Scirupsin B and myricetin are clinically proven for efficacy by targeting amylase from the salivary gland. Curcumin and turmerin have been recorded to inhibit glucosidase in the small intestine. Additionally, chicoric acid and lupanine acids are administered for insulin secretion from β-cells. Even berberine and pectin imparted their efficacy for reducing fasting blood glucose, postprandial blood glucose, and hemoglobin A1c (HbA1c) (139). The previous studies also showed that the seed extract of Castanospermum australe possesses very strong DPP-IV inhibitory alkaloids, including castanospermine, 7-deoxy-6-epi-castanospermine, and australine, and these are depicted as their efficacy in the management of hyperglycemia in rats (42). Recent studies have also been undertaken with garlic bulb, which exhibited a substantial DPP-IV inhibition efficacy (IC50 = 70.88 μg/ml), and this was because of the presence of caffeic acid 3-glucoside, malonylgenistin, and calenduloside E which have very high binding energy Table 3 (49). The recent in silico studies performed on the plant Amberboa ramose determined that the compound 5-hydroxy-7,8 dimethoxyflavone can be considered a promising candidate for serving well for all the purposes of drug likeliness by following the Lipinski’s rule (140). The phytochemicals extracted from Commiphora mukul are guggulsterone E, guggulsterone Z, and glucogallin, and these compounds have potent DPP-IV inhibitor activity compared with vildagliptin (standard plant drug). Additionally, phytochemicals like pzrogallol, beta-glucogallin, and gallic acid purified from the plant Phyllanthus emblica possess considerable inhibitory activity against the DPP-IV target compound (141).
Among many other medicinal plants, Terminalia arjuna is a natural DPP-IV inhibitor with significant cardio-protective effects owing to the presence of some active ingredients, including arjungenin, ellagic acid, and arjunic acid. These active compounds show superior DPP-IV inhibitory activity compared with synthetic inhibitors (64). Nowadays, combinations of ethnomedicine are used, and their efficacy is enhanced by adding bioactivity-determining related ingredients—for example, the efficacy of some of the tea extracts is enhanced by adding bioactive compounds like epigallocatechin-3-O-gallate, kaempferol rutinoside, myricetin-3-0-glucoside, and theogallin. The ethanolic extract of Eucalyptus citriodora has active compounds such as rhodomyrtosone B, rhodomyrtosone E, and quercitroside. These compounds have remarkable in vivo and in vitro effects on managing hyperglycemia. These biochemical compounds enhance insulin functionality in 3T3-L1 cells by improving plasma insulin, glucose tolerance in the high-fat-diet-fed obese rats, and attenuating plasma DPP-IV with a concomitant rise in GLP-1 levels (142). Moreover, a hot water extract from Heritiera fomes possesses some bioactive compounds similar to quercetin. These compounds exhibit antidiabetic action, proved using BRIN-BD11 cells and high-fat-fed rats. A significant improvement in glucose tolerance and plasma DPP-IV was observed in rats fed with the abovementioned compounds. It also led to a decrease in intestinal disaccharidase activity while increasing the GI tract motility and transit time (143). In one of the experiments done on the quinine tree (Rauvolfia caffra sond), the efficacy of crude extract was found to be exterminated because of the dwindling alliances between alkaloids and saponins. Assessing fractions containing saponins and alkaloids revealed decreased antioxidant and DPP-IV activity. Based on these results, alkaloids and saponins should be kept separate during drug formulation (144).
Using bioactive compounds alone or in combination for their synergistic effects is thoroughly studied for their effectiveness against free radicals. The synergism and antagonism of two potential compounds of the crude extract are well known to be strongly influenced by the structure and spatial conformation of the antioxidants involved. It has been stated recently that several compounds can have synergistic effects in particular ratios, as at a particular concentration one bioactive compound can enhance the bioavailability of the other bioactive compound. With an increase of weaker antioxidants like hydroquinone and resveratrol, synergism decreases. Maximum synergism was observed when rutin hydrate was paired with resveratrol in 3:1 proportion, whereas maximum antagonism was observed when rutin hydrate was paired with hydroquinone in 1:3 proportion (145, 146). Moreover, studies have demonstrated that the efficacy of different combinations is also influenced by molar ratio, and the externalization of synergy strongly depends on the inflow of free radicals (147). In one of the studies, the combination of Terminalia arjuna and Commiphora mukul exhibited synergism and augmented antioxidant activity as measured by the normalization of superoxide radicals and nitric oxide levels (148).
Moreover, several polyherbal formulations like BGR-34 (blood glucose regulator) were marketed by Aimil Pharmaceuticals, which was approved by the AYUSH ministry, Government of India. It was named after 34 different active phytoconstituents formulated with a consortium of six different plant extracts, including the stem extract of Berberis aristata, Pterocarpus marsupium, and Tinospora cardiofolia, the leaf extract of Gymnema sylvestre, and the seed extract of Trigonella foenum graecum. It has exhibited DPP-IV action along with its cardioprotective effects, reduced glycated Hb level, enhanced antioxidant action, and regulated glucose homeostasis (124, 149, 150). Similarly, Insulin Management Expert-9, which was developed by the Central Council of Ayurvedic Sciences (CCRAS), is a consortium of five different plant extracts, including the seed extract of Mangifera indica and Syzigium cumini, the fruit extract of Momordica charantia, the leaf extract of Gymnae sylvestre, and the exudates of Asphaltum punjabinum. Its effects led to the regeneration of pancreatic β-cells, stimulation of insulin production, decrease in insulin resistance, delayed insulin resistance, delayed intestinal absorption, and reduced sugar cravings (124, 149, 150).
Flavonoids (Figure 6) are the most abundant plant polyphenolics that are consumed on a regular basis by humans, which can modulate DPP-4 activity, thus exerting their anti-diabetic effects (115). Whether key flavonoid subclasses (anthocyanins, flavones, flavonols, flavanones, and flavan-3-ols) in the diet are connected with the incidence of T2DM has been the subject of a number of research. Several randomized clinical trials (RCTs) have demonstrated the importance of flavonoids in T2DM, especially in long-term trials, but short-term therapies had no discernible impact on blood glucose levels or insulin resistance. An 8-week RCT by Hall et al. (2006) (151) and a 6-month RCT using soy protein and isoflavones did not find a beneficial effect on the plasma concentrations of insulin and glucose in postmenopausal women (152). In another study, green tea extracts containing 456 mg of catechins (a type of flavonoid) daily did not have noticeable effects on blood glucose levels or insulin resistance in an RCT lasting for 2 months (153). However, the long-term randomized, double-blind, placebo-controlled clinical investigation indicated the benefit of green tea extract on patients with T2DM and lipid abnormalities. For the duration of the 16-week research, the therapeutic group took 500 mg of green tea extract three times daily, whereas the control group received cellulose at the same dose and frequency. An increase in GLP-1, likely by inhibiting DPP-4, and a decrease in triglycerides and insulin resistance were noticed in the therapeutic group who received green tea extract (154). Additionally, Curtis et al. (2012) reported that postmenopausal women with T2DM who took flavan-3-ols and isoflavones for a year exhibited significant reductions in estimated peripheral insulin resistance and persistent improvements in lipid profile and insulin sensitivity (155). Similarly, a meta-analysis of 24 RCTs by Shrime et al. (2011) concluded that consuming flavonoid-rich cocoa significantly improved insulin resistance (156), and a meta-analysis of the effects of cocoa, chocolate, and flavan-3-ols from 42 RCTs was summarized by Hooper et al. (2012), who noticed significant reductions in insulin resistance and fasting serum insulin after cocoa or chocolate interventions (157). Notably, flavan-3-ols are among the most abundant bioactive components and account for 82.5% of the total flavonoid consumption among adults in the United States (158).
Figure 6 Flavonoids exhibiting an association with a reduced risk of type 2 diabetes mellitus in clinical studies.
While the consumption of anthocyanin-rich foods, notably blueberries and apples/pears, was related to a decreased risk of T2DM in US men and women, no significant relationships were reported between total flavonoid intake and other flavonoid subclasses and T2DM risk (159). Dietary intakes of the flavonoids quercetin (found in fruits and vegetables) and myricetin (found in tea, berries, fruits, vegetables, and medicinal herbs) were found to be marginally associated with a reduced risk of T2DM in a study involving 10,054 Finnish men and women (160). Because of the contradictory results, well-designed, long-term studies are required to determine whether flavonoids offer protection against T2DM.
Since antiquity, natural product leads have been enriched with bioactive compounds encompassing a huge arena compared with small synthetic molecules (161). Myriads of bioactive compounds have made an incredible contribution to pharmacotherapy. They are far superior to structural leads based on diversified chemical properties. The presence of structural complexity and scaffold diversity is the most distinguishing and prominent feature which has characterized natural drugs to be better and more advantageous than synthetic drugs (162, 163). The existence of higher molecular mass, a larger number of sp3 carbon atoms, more oxygen atoms, and lesser nitrogen and halogen atoms serve to be hydrogen donors and acceptors with greater molecular rigidity over synthetic compounds. Earlier synthetic drugs were preferred over herbal ones because of some shortcomings. To isolate and identify bioactive compounds from plant origin is a challenging task as plants can have a varied chemical composition influenced by various parameters like environmental conditions and choice of extraction methods (164) which can create obstructions during isolation. Henceforth, new advanced technologies have been introduced to reduce the risk of degradation of plant extracts. Microwave, ultrasonic-assisted, and accelerated solvent extraction have outcompeted and flourished over conventional techniques. Currently, the application of ionic liquids, also known as designer solvents, have been used for MAE as well as UAE, have been strongly preferred over other classical solvents for their novel properties, and have replaced several volatile organic compounds in solvent extraction (165). Recent findings have also suggested that adapting the supercritical fluid extraction methodology for extracting various natural products results in better yield (164, 166). As a deduction, herbal leads are perpetuated with a humongous repository of compounds to delineate various pharmacognostic products. With evolving times, people are getting more inclined towards adopting a healthy lifestyle and are required to get nutritionally enriched products with more antioxidants and bioactive compounds; sometimes, these are lost while undergoing processing. Hence, improvement of physicochemical properties is needed along with retention of phenolic compounds by maintaining all the organoleptic characteristics in the final product. Therefore, many emerging treatments are needed to preserve the characteristics of the food product, and by following the block freeze crystallization (BFC) technique, the entire solution is subjected to a freezing process, which is further thawed by microwave heating with separation and extraction of cryoconcentrated solution by centrifugation and vacuum from the frozen matrix. Maintaining concentration is the major trump card of this technique. An advancement of adding a filter to centrifugal BFC is to increase solute efficiency and solute yield. While undergoing experiments with green tea extract, the number of phenolics and antioxidant concentrations was increased by following the BFC technique (167, 168). Moreover, it is well established that obtaining a powder form of pomegranate fruit by performing BFC and spray drying resulted in greater retention of antioxidants as well as significant increases in physical parameters such as solubility index, bulk density, and hygroscopicity (169). The previous studies conducted on fruits of Citrus sinensis demonstrated that freeze drying technique expressed a higher content of phenol and also antioxidants. Flavonoids (hesperidin) were found to be decreased during heat drying (170). Due to the heat-sensitive nature of phenol compounds and the activation of polyphenol oxidase and peroxidase during the thermal drying process, phenolic compounds are destroyed. In contrast, polyphenol oxidase enzyme activity was reduced in freeze drying, which was conducted at a lower temperature. So, instead of using a heat drying process, freeze drying is the best alternative for preserving and quality extraction of bioactive compounds (170). A study on the preservation of antioxidant capacity and health-promoting components in frozen baby mustard also found that blanching before freezing diminishes antioxidant capacity levels as well as the contents of high-content glucosinolates and ascorbic acid (171).
With the recent progression in advanced drug discovery, several in silico approaches have been re-strategized for testing and synthesizing new phytochemicals as anti-diabetic compounds (172). Moreover, in terms of pharmacological and pharmacokinetic synergism, polyherbal formulation offers a better therapeutic effect compared with a single multi-component drug; hence, this strategy produced maximum inhibition with minimal adverse effects (124). Although phytochemicals have gained much interest as therapeutic negotiators, a gap exists between the in vitro examination and in vivo outcomes, eventually impeding clinical performance. To compensate for its efficacy in this regard, the application of nanotechnology has proven to be the best approach by turning all the odds associated with their pharmacokinetic profile. In accordance with the same from recent findings, nanocarrier-assembled nanoparticles fabricated with bioactive compounds act as hypoglycemia agents, which, in turn, strongly influence the effectiveness of the anti-diabetic agents to improve drug penetration within the GI tract against the specific target, perpetuating the hypoglycemic effect with minimal side effects (173). In line with the discussion above about DPP-IV inhibition by the methanolic and aqueous extract of U. lobata, it was understood that methanolic extract expressed better in vitro results than aqueous extract. However, on the contrary, aqueous extract expressed better in vivo results, but not methanolic extract because of lack of solubility and bioavailability within the GI tract, and to improvise the efficacy, the methanolic extract could be fabricated with the nanoformulations for enhancing the bioavailability within the GI tract.
In summary, the DPP-IV inhibitory properties of phytochemicals have sparked an interest in their potential role in glycemia management. Results from in vitro and animal research highlight the potential of these natural inhibitors as plant-based constituents to complement pharmacotherapy in the regulation of blood glucose levels; however, human studies on these compounds are limited. The next stage in researching plant-derived DPP-IV inhibitors is to gather crucial data on the bioaccessibility and bioavailability of these bioactive compounds as well as clinical proof of their usefulness in humans and probable Table 4 interactions with presently prescribed medicines. DPP-IV inhibitors derived from plants may be developed as dietary supplements to minimize the risk of developing hyperglycemia or they may be used with anti-diabetic medications currently approved for glycemic management in T2DM; however, additional research is required to determine the strategies that will result in economically producible levels of these inhibitors.
Table 4 Different marketed polyherbal drugs with their composition and mechanism of action as dipeptidyl peptidase-IV inhibitors.
At the molecular level, DPP-IV inhibitors have proved their functional role by holding an important position in drug discovery by hampering the deterioration of incretin hormones and further preserving their endogenous and blood glucose levels. Additionally, it also aids in the regeneration of pancreatic β-cell mass and enhances skeletal muscle cell proliferation. Nowadays, pharmacognosy has flourished enough to design herbal drugs far more reliable and superior to synthetic drugs with the least adverse effects. This review article aims to shed some light on the potency of different isolated bioactive compounds, polyherbal formulations, nanophytomedicines, and their functional role in efficacious treatment for T2DM. With anticipation of promoting pharmacognosy and phytochemistry to young researchers, this review is entailed with all the information which will be a steppingstone to designing and developing an herbal drug against the target enzyme to maintain endogenous incretin levels.
Original concept, writing the original draft, editing, and methodology: SC. Writing the original draft, editing, and methodology: SM. Writing the original draft, editing, and methodology: SV. Writing the original draft, editing, methodology, and proofreading: DS. Writing the original draft, methodology, and proofreading: PM. Writing the original draft, editing, methodology, and proofreading: BP. All authors contributed to the article and approved the submitted version.
Schemes [number ECR/2016/001984 by SERB, DST, Government of India, and 1188/ST, Bhubaneswar, dated 01.03.17, ST-(Bio)-02/2017, DST, Government of Odisha, India] to BP are acknowledged.
The support from Iowa State University, President Science College, and St. Xavier’s College, Gujurat, India, and from Odisha University of Agriculture and Technology, respectively, are highly acknowledged.
The authors declare that the research was conducted in the absence of any commercial or financial relationships that could be construed as a potential conflict of interest.
All claims expressed in this article are solely those of the authors and do not necessarily represent those of their affiliated organizations, or those of the publisher, the editors and the reviewers. Any product that may be evaluated in this article, or claim that may be made by its manufacturer, is not guaranteed or endorsed by the publisher.
1. Roden M, Shulman GI. The integrative biology of type 2 diabetes. Nature (2019) 576:51–60. doi: 10.1038/S41586-019-1797-8
2. Olokoba AB, Obateru OA, Olokoba LB. Type 2 diabetes mellitus: a review of current trends. Oman Med J (2012) 27:269–73. doi: 10.5001/OMJ.2012.68
3. Murea M, Ma L, Freedman BI. Genetic and environmental factors associated with type 2 diabetes and diabetic vascular complications. Rev Diabetes Stud (2012) 9:6. doi: 10.1900/RDS.2012.9.6
4. Huang X, Liu G, Guo J, Su ZQ. The PI3K/AKT pathway in obesity and type 2 diabetes. Int J Biol Sci (2018) 14:1483. doi: 10.7150/IJBS.27173
5. Henquin JC. Triggering and amplifying pathways of regulation of insulin secretion by glucose. Diabetes (2000) 49:1751–60. doi: 10.2337/DIABETES.49.11.1751
6. Ramachandran A, Snehalatha C, Shetty AS, Nanditha A. Trends in prevalence of diabetes in Asian countries. World J Diabetes (2012) 3:110. doi: 10.4239/WJD.V3.I6.110
7. Gamage K, Wasana P, Attanayake AP, Perera A, Jayatilaka W, Weerarathna TP. Natural drug leads as novel DPP-IV inhibitors targeting the management of type 2 diabetes mellitus. J Of Complementary Med Res (2020) 11:43–53. doi: 10.5455/jcmr.2020.11.01.06
8. Campbell JE, Drucker DJ. Pharmacology, physiology, and mechanisms of incretin hormone action. Cell Metab (2013) 17:819–37. doi: 10.1016/J.CMET.2013.04.008
9. Hussain MA, Laimon-Thomson E, Mustafa SM, Deck A, Song B. Detour ahead: Incretin hormone signaling alters its intracellular path as β-cell failure progresses during diabetes. Front Endocrinol (Lausanne) (2021) 12:665345/BIBTEX. doi: 10.3389/FENDO.2021.665345/BIBTEX
10. Müller TD, Finan B, Bloom SR, D’Alessio D, Drucker DJ, Flatt PR, et al. Glucagon-like peptide 1 (GLP-1). Mol Metab (2019) 30:72–130. doi: 10.1016/J.MOLMET.2019.09.010
11. Pratley RE, Salsali A. Inhibition of DPP-4: a new therapeutic approach for the treatment of type 2 diabetes. Curr Med Res Opin (2007) 23:919–31. doi: 10.1185/030079906X162746
12. Röhrborn D, Wronkowitz N, Eckel J. DPP4 in diabetes. Front Immunol (2015) 6:386/BIBTEX. doi: 10.3389/FIMMU.2015.00386/BIBTEX
13. Al-Masri IM, Mohammad MK, Tahaa MO. Inhibition of dipeptidyl peptidase IV (DPP IV) is one of the mechanisms explaining the hypoglycemic effect of berberine. J Enzy Inhibit Med Chem (2009) 24:1061–6. doi: 10.1080/14756360802610761
14. Bower AM, Real Hernandez LM, Berhow MA, de Mejia EG. Bioactive compounds from culinary herbs inhibit a molecular target for type 2 diabetes management, dipeptidyl peptidase IV. J Agric Food Chem (2014) 62:6147–58. doi: 10.1021/JF500639F
15. Harnedy PA, O’Keeffe MB, Fitzgerald RJ. Purification and identification of dipeptidyl peptidase (DPP) IV inhibitory peptides from the macroalga palmaria palmata. Food Chem (2015) 172:400–6. doi: 10.1016/J.FOODCHEM.2014.09.083
16. Kalhotra P, Chittepu VCSR, Osorio-Revilla G, Gallardo-Velázquez T. Structure–Activity relationship and molecular docking of natural product library reveal chrysin as a novel dipeptidyl peptidase-4 (DPP-4) inhibitor: An integrated in silico and In vitro study. Molecules (2018) 23. doi: 10.3390/MOLECULES23061368
17. Lammi C, Zanoni C, Arnoldi A, Vistoli G. Peptides derived from soy and lupin protein as dipeptidyl-peptidase IV inhibitors: In vitro biochemical screening and in silico molecular modeling study. J Agric Food Chem (2016) 64:9601–6. doi: 10.1021/ACS.JAFC.6B04041
18. Mojica L, Luna-Vital DA, González de Mejía E. Characterization of peptides from common bean protein isolates and their potential to inhibit markers of type-2 diabetes, hypertension and oxidative stress. J Sci Food Agric (2017) 97:2401–10. doi: 10.1002/JSFA.8053
19. Saleem S, Jafri L, Haq IU, Chang LC, Calderwood D, Green BD, et al. Plants fagonia cretica l. and hedera nepalensis k. Koch contain natural compounds with potent dipeptidyl peptidase-4 (DPP-4) inhibitory activity. J Ethnopharmacol (2014) 156:26–32. doi: 10.1016/J.JEP.2014.08.017
20. Ayachi H, Merad M, Ghalem S. Study of interaction between dipeptidyl peptidase-4 and products extracted from the stevia plant by molecular modeling. Int J Pharm Sci Rev Res (2013) 23(1):87–90.
21. Singh AK, Jatwa R, Joshi J. Cytoprotective and dipeptidyl peptidase- IV (DPP-IV/CD26) inhibitory roles of ocimum sanctum and momordica charantia extract. Asian J Pharm Clin Res (2014) 7:115–20.
22. Ekayanti M, Sauriasari R, Elya B. Dipeptidyl peptidase IV inhibitory activity of fraction from white tea ethanolic extract (Camellia sinensis (L.) kuntze) ex vivo. Pharmacogn J (2018) 10:190–3. doi: 10.5530/pj.2018.1.32
23. Riyanti S, Suganda AG, Sukandar EY. Dipeptidyl peptidase –IV inhibitory activity of some Indonesian medicinal plants. Asian J Pharm Clin Res (2016) 9:375–7.
24. Bisht R, Bhattacharya S, Jaliwala YA. Evaluating the use of desmodium gangeticum as alpha glucosidase and DPPIV inhibitor for type-II diabetes. Am J Phytomed Clin Ther (2014) 2(4):530–9.
25. Borde MK, Mohanty I, Suman R, Deshmukh Y. Dipeptidyl peptidase-IV inhibitory activities of medicinal plants: Terminalia arjuna, commiphora mukul, gymnema sylvestre, morinda citrifolia, emblica officinalis. Asian J Pharm Clin Res (2016) 9:180–2. Available at: https://innovareacademics.in/journals/index.php/ajpcr/article/view/10894
26. Cásedas G, Les F, González-Burgos E, Gómez-Serranillos MP, Smith C, López V. Cyanidin-3-O-glucoside inhibits different enzymes involved in central nervous system pathologies and type-2 diabetes. South Afr J Bot (2019) 120:241–6. doi: 10.1016/J.SAJB.2018.07.001
27. Gupta A, Jacobson GA, Burgess JR, Jelinek HF, Nichols DS, Narkowicz CK, et al. Citrus bioflavonoids dipeptidyl peptidase-4 inhibition compared with gliptin antidiabetic medications. Biochem Biophys Res Commun (2018) 503:21–5. doi: 10.1016/J.BBRC.2018.04.156
28. Lin YS, Chen CR, Wu WH, Wen CL, Chang CI, Hou WC. Anti-α-glucosidase and anti-dipeptidyl peptidase-IV activities of extracts and purified compounds from vitis thunbergii var. taiwaniana. J Agric Food Chem (2015) 63:6393–401. doi: 10.1021/ACS.JAFC.5B02069/SUPPL_FILE/JF5B02069_SI_001.PDF
29. Eidenberger T, Selg M, Krennhuber K. Inhibition of dipeptidyl peptidase activity by flavonol glycosides of guava (Psidium guajava l.): A key to the beneficial effects of guava in type II diabetes mellitus. Fitoterapia (2013) 89:74–9. doi: 10.1016/J.FITOTE.2013.05.015
30. Dey B, Mitra A, Katakam P, Singla RK. Exploration of natural enzyme inhibitors with hypoglycemic potentials amongst eucalyptus spp. by in vitro assays. World J Diabetes (2014) 5:209. doi: 10.4239/WJD.V5.I2.209
31. Martinez G. Comprehensive medicinal chemistry. volume 1, general perspective - the future of drug discovery. Martinez A, Gil C, editors. Madrid, Spain: Centro de Investigaciones Biologicas-CSIC (2017).
32. Kosaraju J, Dubala A, Chinni S, Khatwal RB, Satish Kumar MN, Basavan D. A molecular connection of pterocarpus marsupium, Eugenia jambolana and gymnema sylvestre with dipeptidyl peptidase-4 in the treatment of diabetes. Pharm Biol (2014) 52:268–71. doi: 10.3109/13880209.2013.823550
33. Yogisha S, Raveesha KA. Dipeptidyl peptidase IV inhibitory activity of mangifera indica. J Nat Prod (2010) 3:76–9.
34. Ansari P, Hannon-Fletcher MP, Flatt PR, Abdel-Wahab YHA. Effects of 22 traditional anti-diabetic medicinal plants on DPP-IV enzyme activity and glucose homeostasis in high-fat fed obese diabetic rats. Biosci Rep (2021) 41. doi: 10.1042/BSR20203824
35. Quek A, Kassim NK, Ismail A, Latif MAM, Shaari K, Tan DC, et al. Identification of dipeptidyl peptidase-4 and α-amylase inhibitors from melicope glabra (Blume) t. g. Hartley (Rutaceae) using liquid chromatography tandem mass spectrometry, In vitro and in silico methods. Molecules (2020) 26:1–35. doi: 10.3390/MOLECULES26010001
36. Gao Y, Zhang Y, Zhu J, Li B, Li Z, Zhu W, et al. Recent progress in natural products as DPP-4 inhibitors. Future Med Chem (2015) 7:1079–89. doi: 10.4155/FMC.15.49
37. Wang HJ, Chiang BH. Anti-diabetic effect of a traditional Chinese medicine formula. Food Funct (2012) 3:1161–9. doi: 10.1039/C2FO30139C
38. Bansal P, Paul P, Mudgal J, G. Nayak P, Thomas Pannakal S, Priyadarsini KI, et al. Antidiabetic, antihyperlipidemic and antioxidant effects of the flavonoid rich fraction of pilea microphylla (L.) in high fat diet/streptozotocin-induced diabetes in mice. Exp Toxicol Pathol (2012) 64:651–8. doi: 10.1016/J.ETP.2010.12.009
39. Purnomo Y, Soeatmadji DW, Sumitro SB, Widodo MA. Inhibitory activity of urena lobata leaf extract on dipeptidyl peptidase-4 (DPP-4): Is it different in vitro and in vivo? Medi Plants (2018) 10:99–105. doi: 10.5958/0975-6892.2018.00016.3
40. Wang Z, Yang L, Fan H, Wu P, Zhang F, Zhang C, et al. Screening of a natural compound library identifies emodin, a natural compound from rheum palmatum linn that inhibits DPP4. PeerJ (2017) 1–14. doi: 10.7717/PEERJ.3283/SUPP-5
41. Fan J, Johnson MH, Lila MA, Yousef G, de Mejia EG. Berry and citrus phenolic compounds inhibit dipeptidyl peptidase IV: Implications in diabetes management. Evid Based Complement Alternat Med (2013) 2013:1–13. doi: 10.1155/2013/479505
42. Bharti SK, Krishnan S, Kumar A, Rajak KK, Murari K, Bharti BK, et al. Antihyperglycemic activity with DPP-IV inhibition of alkaloids from seed extract of castanospermum australe: Investigation by experimental validation and molecular docking. Phytomedicine (2012) 20:24–31. doi: 10.1016/J.PHYMED.2012.09.009
43. Wang F, Yu G, Zhang Y, Zhang B, Fan J. Dipeptidyl peptidase IV inhibitory peptides derived from oat (Avena sativa l.), buckwheat (Fagopyrum esculentum), and highland barley (Hordeum vulgare trifurcatum (L.) trofim) proteins. J Agric Food Chem (2015) 63:9543–9. doi: 10.1021/ACS.JAFC.5B04016
44. Velarde-Salcedo AJ, Barrera-Pacheco A, Lara-González S, Montero-Morán GM, Díaz-Gois A, González De Mejia E, et al. In vitro inhibition of dipeptidyl peptidase IV by peptides derived from the hydrolysis of amaranth (Amaranthus hypochondriacus l.) proteins. Food Chem (2013) 136:758–64. doi: 10.1016/J.FOODCHEM.2012.08.032
45. Yarizade A, Kumleh HH, Niazi A. In vitro antidiabetic effects of ferula assa-foetida extracts through dipeptidyl peptidase IV and α-glucosidase inhibitory activity. Asian J Pharm Clin Res (2017) 10:357–60. doi: 10.22159/AJPCR.2017.V10I5.16648
46. Kumar V, Sachan R, Rahman M, Sharma K, Al-Abbasi FA, Anwar F. Prunus amygdalus extract exert antidiabetic effect via inhibition of DPP-IV: in-silico and in-vivo approaches. J Biomol Struct Dyn (2021) 39:4160–74. doi: 10.1080/07391102.2020.1775124
47. Kim BR, Kim HY, Choi I, Kim JB, Jin CH, Han AR. DPP-IV inhibitory potentials of flavonol glycosides isolated from the seeds of lens culinaris: In vitro and molecular docking analyses. Molecules (2018) 23:1–10. doi: 10.3390/MOLECULES23081998
48. Chakrabarti R, Bhavtaran S, Narendra P, Varghese N, Vanchhawng L, Sham Shihabudeen MH, et al. Dipeptidyl peptidase-IV inhibitory activity of berberis aristata. J Nat Prod (2011) 4:158–63.
49. Kalhotra P, Chittepu VCSR, Osorio-Revilla G, Gallardo-Velazquez T. Phytochemicals in garlic extract inhibit therapeutic enzyme DPP-4 and induce skeletal muscle cell proliferation: A possible mechanism of action to benefit the treatment of diabetes mellitus. Biomolecules (2020) 10:1–16. doi: 10.3390/BIOM10020305
50. Zhang L, Zhang ST, Yin YC, Xing S, Li WN, Fu XQ. Hypoglycemic effect and mechanism of isoquercitrin as an inhibitor of dipeptidyl peptidase-4 in type 2 diabetic mice. RSC Adv (2018) 8:14967–74. doi: 10.1039/C8RA00675J
51. Gallwitz B. Clinical use of DPP-4 inhibitors. Front Endocrinol (Lausanne) (2019) 10:389/BIBTEX. doi: 10.3389/FENDO.2019.00389/BIBTEX
52. Biftu T, Sinha-Roy R, Chen P, Qian X, Feng D, Kuethe JT, et al. Omarigliptin (MK-3102): a novel long-acting DPP-4 inhibitor for once-weekly treatment of type 2 diabetes. J Med Chem (2014) 57:3205–12. doi: 10.1021/JM401992E
53. Barnett A. DPP-4 inhibitors and their potential role in the management of type 2 diabetes. Int J Clin Pract (2006) 60:1454–70. doi: 10.1111/J.1742-1241.2006.01178.X
54. Deacon CF. Dipeptidyl peptidase-4 inhibitors in the treatment of type 2 diabetes: a comparative review. Diabetes Obes Metab (2011) 13:7–18. doi: 10.1111/J.1463-1326.2010.01306.X
55. del Prato S, Rosenstock J, Garcia-Sanchez R, Iqbal N, Hansen L, Johnsson E, et al. Safety and tolerability of dapagliflozin, saxagliptin and metformin in combination: Post-hoc analysis of concomitant add-on versus sequential add-on to metformin and of triple versus dual therapy with metformin. Diabetes Obes Metab (2018) 20:1542–6. doi: 10.1111/DOM.13258
56. Scheen AJ. The safety of gliptins : updated data in 2018. Expert Opin Drug Saf (2018) 17:387–405. doi: 10.1080/14740338.2018.1444027
57. Goldenberg RM. Choosing dipeptidyl peptidase-4 inhibitors, sodium-glucose cotransporter-2 inhibitors, or both, as add-ons to metformin: Patient baseline characteristics are crucial. Clin Ther (2017) 39:2438–47. doi: 10.1016/J.CLINTHERA.2017.10.016
58. Kumar S, Mittal A, Mittal A. A review upon medicinal perspective and designing rationale of DPP-4 inhibitors. Bioorg Med Chem (2021) 46. doi: 10.1016/J.BMC.2021.116354
59. Scheen AJ. A review of gliptins in 2011. Expert Opin Pharmacother (2012) 13:81–99. doi: 10.1517/14656566.2012.642866
60. FDA Drug Safety Communication. FDA Warns that DPP-4 inhibitors for type 2 diabetes may cause severe joint pain (2016). Available at: https://www.fda.gov/drugs/drug-safety-and-availability/fda-drug-safety-communication-fda-warns-dpp-4-inhibitors-type-2-diabetes-may-cause-severe-joint-pain (Accessed October 4, 2022).
61. Gupta V, Kalra S. Choosing a gliptin. Indian J Endocrinol Metab (2011) 15:298. doi: 10.4103/2230-8210.85583
62. Pathak R, Bridgeman MB. Dipeptidyl peptidase-4 (DPP-4) inhibitors in the management of diabetes. Pharm Ther (2010) 35:509.
63. Chahal H, Chowdhury TA. Gliptins: a new class of oral hypoglycaemic agent. QJM: Int J Med (2007) 100:671–7. doi: 10.1093/QJMED/HCM081
64. Mohanty IR, Borde M, Kumar CS, Maheshwari U. Dipeptidyl peptidase IV inhibitory activity of terminalia arjuna attributes to its cardioprotective effects in experimental diabetes: In silico, in vitro and in vivo analyses. Phytomedicine (2019) 57:158–65. doi: 10.1016/J.PHYMED.2018.09.195
65. Johansen JS, Harris AK, Rychly DJ, Ergul A. Oxidative stress and the use of antioxidants in diabetes: Linking basic science to clinical practice. Cardiovasc Diabetol (2005) 4:5. doi: 10.1186/1475-2840-4-5
66. Chan SMH, Ye JM. Strategies for the discovery and development of anti-diabetic drugs from the natural products of traditional medicines. J Pharm Sci (2013) 16:207–16. doi: 10.18433/J3T60G
67. Esmail Al-Snafi A, Majid WJ, Ali Talab T, Al-Battat HA, Author C. Medicinal plants with antidiabetic effects-an overview (Part 1). IOSR J Pharm (2019) 9:9–46.
68. Chainy GBN, Sahoo DK. Hormones and oxidative stress: an overview. Free Radic Res (2020) 54:1–21. doi: 10.1080/10715762.2019.1702656
69. Bashan N, Kovsan J, Kachko I, Ovadia H, Rudich A. Positive and negative regulation of insulin signaling by reactive oxygen and nitrogen species. Physiol Rev (2009) 89:27–71. doi: 10.1152/PHYSREV.00014.2008/ASSET/IMAGES/LARGE/Z9J0010924990009.JPEG
70. Sharifi-Rad J, Cruz-Martins N, López-Jornet P, Lopez EPF, Harun N, Yeskaliyeva B, et al. Natural coumarins: Exploring the pharmacological complexity and underlying molecular mechanisms. Oxid Med Cell Longev (2021) 2021. doi: 10.1155/2021/6492346
71. Ghorbani A, Rashidi R, Shafiee-Nick R. Flavonoids for preserving pancreatic beta cell survival and function: A mechanistic review. Biomed Pharmacother (2019) 111:947–57. doi: 10.1016/J.BIOPHA.2018.12.127
72. Oh YS. Plant-derived compounds targeting pancreatic beta cells for the treatment of diabetes. Evid Based Complement Alternat Med (2015) 2015. doi: 10.1155/2015/629863
73. Annadurai T, Muralidharan AR, Joseph T, Hsu MJ, Thomas PA, Geraldine P. Antihyperglycemic and antioxidant effects of a flavanone, naringenin, in streptozotocin–nicotinamide-induced experimental diabetic rats. J Physiol Biochem (2012) 68:307–18. doi: 10.1007/S13105-011-0142-Y
74. Coskun O, Kanter M, Korkmaz A, Oter S. Quercetin, a flavonoid antioxidant, prevents and protects streptozotocin-induced oxidative stress and β-cell damage in rat pancreas. Pharmacol Res (2005) 51:117–23. doi: 10.1016/J.PHRS.2004.06.002
75. Lee D, Kim KH, Lee J, Hwang GS, Lee HL, Hahm DH, et al. Protective effect of cirsimaritin against streptozotocin-induced apoptosis in pancreatic beta cells. J Pharm Pharmacol (2017) 69:875–83. doi: 10.1111/JPHP.12719
76. He JZ, Shao P, Liu JH, Ru QM. Supercritical carbon dioxide extraction of flavonoids from pomelo (Citrus grandis (L.) osbeck) peel and their antioxidant activity. Int J Mol Sci (2012) 13:13065–78. doi: 10.3390/IJMS131013065
77. Singh AK, Yadav D, Sharma N, Jin JO. Dipeptidyl peptidase (DPP)-IV inhibitors with antioxidant potential isolated from natural sources: A novel approach for the management of diabetes. Pharmaceuticals (2021) 14. doi: 10.3390/PH14060586
78. Purnomo Y, Soeatmadji DW, Sumitro SB, Widodo MA. Original article. Asian Pac J Trop BioMed (2015) 8:645–9. doi: 10.1016/J.APJTB.2015.05.014
79. Omonkhua AA, Onoagbe IO. Evaluation of the long-term effects of urena lobata root extracts on blood glucose and hepatic function of normal rabbits. J Toxicol Environ Health Sci (2011) 3:204–13.
80. Sekendar Ali M, Omar Faruq K, Aziz Abdur Rahman M, Aslam Hossain M. Antioxidant and cytotoxic activities of methanol extract of urena lobata (L) leaves. Pharma Innovation-Journal (2013) 2.
81. Goodman LS, Louis S, Gilman A, Hardman JG, Gilman AG, Limbird LE. Goodman & gilman’s the pharmacological basis of therapeutics. (New York: McGraw-Hill, Medical Publishing Division). (1996). 1905.
82. Stevens MM, Honerkamp-Smith AR, Keller SL. Solubility limits of cholesterol, lanosterol, ergosterol, stigmasterol, and β-sitosterol in electroformed lipid vesicles. Soft Matter (2010) 6:5882–90. doi: 10.1039/C0SM00373E
83. Morris GM, Lim-Wilby M. Molecular docking. Methods Mol Biol (2008) 443:365–82. doi: 10.1007/978-1-59745-177-2_19/TABLES/1
84. Srivastava S, Yadav D, Bhusan Tripathi Y. DPP-IV inhibitory potential of methanolic extract of pueraria tuberosa in liver of alloxan induced diabetic model. Biosci Biotechnol Res Asia (2018) 15:01–4. doi: 10.13005/BBRA/2602
85. Choudhury H, Pandey M, Hua CK, Mun CS, Jing JK, Kong L, et al. An update on natural compounds in the remedy of diabetes mellitus: A systematic review. J Tradit Complement Med (2018) 8:361. doi: 10.1016/J.JTCME.2017.08.012
86. Kempegowda PK, Zameer F, Narasimashetty CK, Kollur SP, Murari SK. Inhibitory potency of Withania somnifera extracts against DPP-4: an in vitro evaluation. Afr J Traditional Complementary Altern Medicines (2018) 15:11–25. doi: 10.4314/ajtcam.v15i1
87. Bellamkonda R, Rasineni K, Singareddy SR, Kasetti RB, Pasurla R, Chippada AR, et al. Antihyperglycemic and antioxidant activities of alcoholic extract of commiphora mukul gum resin in streptozotocin induced diabetic rats. Pathophysiology (2011) 18:255–61. doi: 10.1016/J.PATHOPHYS.2010.10.002
88. Ramesh B, Karuna R, Sreenivasa Reddy S, Sudhakara G, Saralakumari D. Ethanolic extract of commiphora mukul gum resin attenuates streptozotocin-induced alterations in carbohydrate and lipid metabolism in rats. EXCLI J (2013) 12:556.
89. Ramesh B, Saralakumari D. Antihyperglycemic, hypolipidemic and antioxidant activities of ethanolic extract of commiphora mukul gum resin in fructose-fed male wistar rats. J Physiol Biochem (2012) 68:573–82. doi: 10.1007/S13105-012-0175-X
90. Asghari J, Ondruschka B, Mazaheritehrani M. Extraction of bioactive chemical compounds from the medicinal Asian plants by microwave irradiation. J Medi Plants Res (2011) 5:495–506.
91. Mubarik S, Aftab M, Tariq A. Issue 2 article 4 part of the biodiversity commons, and the biology commons recommended citation recommended citation javad. J Bioresource Manage (2016) 3. doi: 10.35691/JBM.6102.0051
92. Bisht R, Bhattacharya S. Effect of various extracts of desmodium gangeticum on streptozotocin-nicotinamide induced type-2 diabetes. Asian J Plant Sci Res (2013) 3:28–34.
93. Nwakulite A, Obeagu EI, Nwanjo HU, Nwosu DC, Nnatuanya IN, Eze R, et al. Studies on molecular docking of moringa oleifera leaf phytochemical constituents on alpha glucosidase, alpha amylase and dipeptidyl peptidase. J Pharm Res Int (2021) 33:239–45. doi: 10.9734/JPRI/2021/V33I28A31527
94. Anwer T, Safhi MM, Makeen HA, Alshahrani S, Siddiqui R, Sivakumar SM, et al. Antidiabetic potential of moringa oleifera lam. leaf extract in type 2 diabetic rats, and its mechanism of action. Trop J Pharm Res (2021) 20:97–104. doi: 10.4314/tjpr.v20i1.15
95. Yang Y, Shi CY, Xie J, Dai JH, He SL, Tian Y. Identification of potential dipeptidyl peptidase (DPP)-IV inhibitors among moringa oleifera phytochemicals by virtual screening, molecular docking analysis, ADME/T-based prediction, and In vitro analyses. Molecules (2020) 25:1–12. doi: 10.3390/MOLECULES25010189
96. Agusfina M, Saputri FC, Sakti AS, Mun’im A. Difference of acidic adding effect in ethanol extraction of white mulberry stem bark (Morus alba) and DPP-4 inhibiting activity screening for identifying its antidiabetic potential. Pharmacogn J (2019) 11:790–5. doi: 10.5530/pj.2019.11.126
97. Guo C, Li R, Zheng N, Xu L, Liang T, He Q. Anti-diabetic effect of ramulus mori polysaccharides, isolated from morus alba l., on STZ-diabetic mice through blocking inflammatory response and attenuating oxidative stress. Int Immunopharmacol (2013) 16:93–9. doi: 10.1016/J.INTIMP.2013.03.029
98. Jiao Y, Wang X, Jiang X, Kong F, Wang S, Yan C. Antidiabetic effects of morus alba fruit polysaccharides on high-fat diet- and streptozotocin-induced type 2 diabetes in rats. J Ethnopharmacol (2017) 199:119–27. doi: 10.1016/J.JEP.2017.02.003
99. Kim SB, Chang BY, Hwang BY, Kim SY, Lee MK. Pyrrole alkaloids from the fruits of morus alba. Bioorg Med Chem Lett (2014) 24:5656–9. doi: 10.1016/J.BMCL.2014.10.073
100. Zhang Y, Ren C, Lu G, Cui W, Mu Z, Gao H, et al. Purification, characterization and anti-diabetic activity of a polysaccharide from mulberry leaf. Regul Toxicol Pharmacol (2014) 70:687–95. doi: 10.1016/J.YRTPH.2014.10.006
101. Darvas F, Keseru G, Papp A, Dorman G, Urge L, Krajcsi P. In silico and ex silico ADME approaches for drug discovery. Curr Top Med Chem (2002) 2:1287–304. doi: 10.2174/1568026023392841
102. Bharti R, Chopra BS, Raut S, Khatri N. Pueraria tuberosa: A review on traditional uses, pharmacology, and phytochemistry. Front Pharmacol (2020) 11:582506. doi: 10.3389/FPHAR.2020.582506
103. Ram H, Kumar P, Purohit A, Kashyap P, Kumar S, Kumar S, et al. Improvements in HOMA indices and pancreatic endocrinal tissues in type 2-diabetic rats by DPP-4 inhibition and antioxidant potential of an ethanol fruit extract of withania coagulans. Nutr Metab (Lond) (2021) 18:1–17. doi: 10.1186/S12986-021-00547-2/TABLES/4
104. Zabidi NA, Ishak NA, Hamid M, Ashari SE, Mohammad Latif MA. Inhibitory evaluation of curculigo latifolia on α-glucosidase, DPP (IV) and in vitro studies in antidiabetic with molecular docking relevance to type 2 diabetes mellitus. J Enzyme Inhib Med Chem (2021) 36:109–21. doi: 10.1080/14756366.2020.1844680
105. Quek A, Kassim NK, Lim PC, Tan DC, Mohammad Latif MA, Ismail A, et al. α-amylase and dipeptidyl peptidase-4 (DPP-4) inhibitory effects of melicope latifolia bark extracts and identification of bioactive constituents using in vitro and in silico approaches. Pharm Biol (2021) 59:964–73. doi: 10.1080/13880209.2021.1948065
106. Lipinski CA, Lombardo F, Dominy BW, Feeney PJ. Experimental and computational approaches to estimate solubility and permeability in drug discovery and development settings. Adv Drug Delivery Rev (1997) 23:3–25. doi: 10.1016/S0169-409X(96)00423-1
108. Meng X-Y, Zhang H-X, Mezei M, Cui M. Molecular docking: a powerful approach for structure-based drug discovery. Curr Comput Aided Drug Des (2011) 7:146–57. doi: 10.2174/157340911795677602
109. Ferreira LG, dos Santos RN, Oliva G, Andricopulo AD. Molecular docking and structure-based drug design strategies. Molecules (2015) 20:13384–421. doi: 10.3390/MOLECULES200713384
110. Bikadi Z, Hazai E. Application of the PM6 semi-empirical method to modeling proteins enhances docking accuracy of AutoDock. J Cheminform (2009) 1:1–16. doi: 10.1186/1758-2946-1-15/FIGURES/3
111. Lape M, Elam C, Paula S. Comparison of current docking tools for the simulation of inhibitor binding by the transmembrane domain of the sarco/endoplasmic reticulum calcium ATPase. Biophys Chem (2010) 150:88. doi: 10.1016/J.BPC.2010.01.011
112. Amini Z, Fatemi MH, Gharaghani S. Hybrid docking-QSAR studies of DPP-IV inhibition activities of a series of aminomethyl-piperidones. Comput Biol Chem (2016) 64:335–45. doi: 10.1016/J.COMPBIOLCHEM.2016.08.003
113. Bharti SK, Krishnan S, Kumar A, Rajak KK, Murari K, Bharti BK, et al. Antidiabetic activity and molecular docking of fructooligosaccharides produced by aureobasidium pullulans in poloxamer-407-induced T2DM rats. Food Chem (2013) 136:813–21. doi: 10.1016/J.FOODCHEM.2012.08.083
114. Bharti SK, Krishnan S, Kumar A, Gupta AK, Ghosh AK, Kumar A. Mechanism-based antidiabetic activity of fructo- and isomalto-oligosaccharides: Validation by in vivo, in silico and in vitro interaction potential. Process Biochem (2015) 50:317–27. doi: 10.1016/J.PROCBIO.2014.10.014
115. Pan J, Zhang Q, Zhang C, Yang W, Liu H, Lv Z, et al. Inhibition of dipeptidyl peptidase-4 by flavonoids: Structure–activity relationship, kinetics and interaction mechanism. Front Nutr (2022) 9:892426. doi: 10.3389/FNUT.2022.892426
116. Ilango S, Sahoo DK, Paital B, Kathirvel K, Gabriel JI, Subramaniam K, et al. A review on annona muricata and its anticancer activity. Cancers (2022) 14:4539. doi: 10.3390/CANCERS14184539
118. Gannon NP, Conn CA, Vaughan RA. Dietary stimulators of GLUT4 expression and translocation in skeletal muscle: a mini-review. Mol Nutr Food Res (2015) 59:48–64. doi: 10.1002/MNFR.201400414
119. Vinayagam R, Xu B. Antidiabetic properties of dietary flavonoids: a cellular mechanism review. Nutr Metab (2015) 12:1–20. doi: 10.1186/S12986-015-0057-7
120. Md Sayem AS, Arya A, Karimian H, Krishnasamy N, Hasamnis AA, Hossain CF. Action of phytochemicals on insulin signaling pathways accelerating glucose transporter (GLUT4) protein translocation. Molecules (2018) 23. doi: 10.3390/MOLECULES23020258
121. Domínguez Avila JA, Rodrigo García J, González Aguilar GA, de la Rosa LA. The antidiabetic mechanisms of polyphenols related to increased glucagon-like peptide-1 (GLP1) and insulin signaling. Molecules (2017) 22. doi: 10.3390/MOLECULES22060903
122. Vijaya Nagini D, Krishna MSR, Karthikeyan S. Identification of novel dipeptidyl peptidase -IV inhibitors from ferula asafoetida through GC-MS and molecular docking studies. Res J Pharm Technol (2020) 13:5072–6. doi: 10.5958/0974-360X.2020.00888.4
123. Rozano L, Abdullah Zawawi MR, Ahmad MA, Jaganath IB. Computational analysis of gynura bicolor bioactive compounds as dipeptidyl peptidase-IV inhibitor. Adv Bioinf (2017) 2017:1–17. doi: 10.1155/2017/5124165
124. Karole S, Shrivastava S, Thomas S, Soni B, Khan S, Dubey J, et al. Polyherbal formulation concept for synergic action: A review. J Drug Delivery Ther (2019) 9:453–66. doi: 10.22270/JDDT.V9I1-S.2339
125. Gordon A, Buch Z, Baute V, Coeytaux R. Use of ayurveda in the treatment of type 2 diabetes mellitus. Glob Adv Health Med (2019) 8. doi: 10.1177/2164956119861094
126. Parasuraman S, Thing GS, Dhanaraj SA. Polyherbal formulation: Concept of ayurveda. Pharmacogn Rev (2014) 8:73–80. doi: 10.4103/0973-7847.134229
127. Wickramasinghe ASD, Kalansuriya P, Attanayake AP. Nanoformulation of plant-based natural products for type 2 diabetes mellitus: From formulation design to therapeutic applications. Curr Ther Res Clin Exp (2022) 96:100672. doi: 10.1016/J.CURTHERES.2022.100672
128. Wang F, Zhang Y, Yu T, He J, Cui J, Wang J, et al. Oat globulin peptides regulate antidiabetic drug targets and glucose transporters in caco-2 cells. J Funct Foods (2018) 42:12–20. doi: 10.1016/J.JFF.2017.12.061
129. Su L, Zhou F, Yu M, Ge R, He J, Zhang B, et al. Solid lipid nanoparticles enhance the resistance of oat-derived peptides that inhibit dipeptidyl peptidase IV in simulated gastrointestinal fluids. J Funct Foods (2020) 65:103773. doi: 10.1016/J.JFF.2019.103773
130. Garcia Campoy AH, Pérez Gutiérrez RM, Manriquez-Alvirde G, Muñiz Ramirez A. Protection of silver nanoparticles using eysenhardtia polystachya in peroxide-induced pancreatic β-cell damage and their antidiabetic properties in zebrafish. Int J Nanomed (2018) 13:2601. doi: 10.2147/IJN.S163714
131. Odei-Addo F, Shegokar R, Müller RH, Levendal R-A, Frost C. Nanoformulation of leonotis leonurus to improve its bioavailability as a potential antidiabetic drug. 3 Biotech (2017) 7:344. doi: 10.1007/S13205-017-0986-0
132. Ashcroft FM, Proks P, Smith PA, Ämmälä C, Bokvist K, Rorsman P. Stimulus-secretion coupling in pancreatic beta cells. J Cell Biochem (1994) 55 Suppl:54–65. doi: 10.1002/JCB.240550007
133. Schmidt S, Jakab M, Jav S, Streif D, Pitschmann A, Zehl M, et al. Extracts from leonurus sibiricus l. increase insulin secretion and proliferation of rat INS-1E insulinoma cells. J Ethnopharmacol (2013) 150:85–94. doi: 10.1016/J.JEP.2013.08.013
134. Jin X, Zhang ZH, Li SL, Sun E, Tan XB, Song J, et al. A nanostructured liquid crystalline formulation of 20(S)-protopanaxadiol with improved oral absorption. Fitoterapia (2013) 84:64–71. doi: 10.1016/J.FITOTE.2012.09.013
135. Paul D, Dey TK, Mukherjee S, Ghosh M, Dhar P. Comparative prophylactic effects of α-eleostearic acid rich nano and conventional emulsions in induced diabetic rats. J Food Sci Technol (2014) 51:1724. doi: 10.1007/S13197-014-1257-2
136. Saha SS, Ghosh M. Ameliorative role of conjugated linolenic acid isomers against oxidative DNA damage induced by sodium arsenite in rat model. Food Chem Toxicol (2010) 48:3398–405. doi: 10.1016/J.FCT.2010.09.011
137. Dhar P, Ghosh S, Bhattacharyya DK. Dietary effects of conjugated octadecatrienoic fatty acid (9 cis, 11 trans, 13 trans) levels on blood lipids and nonenzymatic in vitro lipid peroxidation in rats. Lipids (1999) 34:109–14. doi: 10.1007/S11745-999-0343-2
138. Bharti SK, Krishnan S, Kumar A, Kumar A. Antidiabetic phytoconstituents and their mode of action on metabolic pathways. Ther Adv Endocrinol Metab (2018) 9:81–100. doi: 10.1177/2042018818755019
139. Alam S, Sarker MMR, Sultana TN, Chowdhury MNR, Rashid MA, Chaity NI, et al. Antidiabetic phytochemicals from medicinal plants: Prospective candidates for new drug discovery and development. Front Endocrinol (Lausanne) (2022) 13:800714. doi: 10.3389/FENDO.2022.800714
140. Paul RK, Ahmad I, Patel H, Kumar V, Raza K. Phytochemicals from amberboa ramosa as potential DPP-IV inhibitors for the management of type-II diabetes mellitus: Inferences from in-silico investigations. J Mol Struct (2023) 1271:134045. doi: 10.1016/J.MOLSTRUC.2022.134045
141. Mohanty I, Kumar C, Borde M. Antidiabetic activity of commiphora mukul and phyllanthus emblica and computational analysis for the identification of active principles with dipeptidyl peptidase IV inhibitory activity. Indian J Pharmacol (2021) 53:384–7. doi: 10.4103/IJP.IJP_69_19
142. Ansari P, Choudhury ST, Abdel-Wahab YHA. Insulin secretory actions of ethanol extract of eucalyptus citriodora leaf, including plasma DPP-IV and GLP-1 levels in high-Fat-Fed rats, as well as characterization of biologically effective phytoconstituents. Metabolites (2022) 12:757. doi: 10.3390/METABO12080757
143. Ansari P, Hannan JA, Abdel-Wahab YHA, Flatt PR. Antidiabetic and insulinotropic properties of bark of heritiera fomes: inhibits starch digestion, protein glycation, DPP-IV activity, and glucose absorption in gut. Planta Med (2021) 87:YRW9. doi: 10.1055/S-0041-1736789
144. Milugo TK, Omosa LK, Ochanda JO, Owuor BO, Wamunyokoli FA, Oyugi JO, et al. Antagonistic effect of alkaloids and saponins on bioactivity in the quinine tree (Rauvolfia caffra sond.): further evidence to support biotechnology in traditional medicinal plants. BMC Complement Altern Med (2013) 13. doi: 10.1186/1472-6882-13-285
145. Joshi T, Deepa PR, Sharma PK. Effect of different proportions of phenolics on antioxidant potential: Pointers for bioactive Synergy/Antagonism in foods and nutraceuticals. Proc Natl Acad Sci India Sect B Biol Sci (2022). doi: 10.1007/S40011-022-01396-6
146. Roell KR, Reif DM, Motsinger-Reif AA. An introduction to terminology and methodology of chemical synergy-perspectives from across disciplines. Front Pharmacol (2017) 8:158/BIBTEX. doi: 10.3389/FPHAR.2017.00158/BIBTEX
147. Ilyasov I, Beloborodov V, Antonov D, Dubrovskaya A, Terekhov R, Zhevlakova A, et al. Flavonoids with glutathione antioxidant synergy: Influence of free radicals inflow. Antioxidants (2020) 9:1–20. doi: 10.3390/ANTIOX9080695
148. Rizvanov AA, Kumar B, Padma S, Editors G. Lecture notes in bioengineering. advances in biomedical engineering and technology select proceedings of ICBEST (2018). Available at: http://www.springer.com/series/11564 (Accessed September 30, 2022).
149. Gajarmal DA, Kumar Rath D. Review on promotions of ayurveda product, bgr-34 through multimedia. J Drug Res (2016) 5:16–29.
150. Gupta BP, Sharma I, Kohli N, Sharma S, Rathi A, Sharma AK. Preliminary clinical assessment and non- toxicity evaluation of an ayurvedic formulation BGR-34 in NIDDM. J Tradit Complement Med (2018) 8:506. doi: 10.1016/J.JTCME.2017.11.004
151. Hall WL, Vafeiadou K, Hallund J, Bugel S, Reimann M, Koebnick C, et al. Soy-isoflavone-enriched foods and markers of lipid and glucose metabolism in postmenopausal women: interactions with genotype and equol production. Am J Clin Nutr (2006) 83:592–600. doi: 10.1093/AJCN.83.3.592
152. Liu YJ, Zhan J, Liu XL, Wang Y, Ji J, He QQ. Dietary flavonoids intake and risk of type 2 diabetes: A meta-analysis of prospective cohort studies. Clin Nutr (2014) 33:59–63. doi: 10.1016/J.CLNU.2013.03.011
153. Fukino Y, Shimbo M, Aoki N, Okubo T, Iso H. Randomized controlled trial for an effect of green tea consumption on insulin resistance and inflammation markers. J Nutr Sci Vitaminol (Tokyo) (2005) 51:335–42. doi: 10.3177/JNSV.51.335
154. Liu CY, Huang CJ, Huang LH, Chen IJ, Chiu JP, Hsu CH. Effects of green tea extract on insulin resistance and glucagon-like peptide 1 in patients with type 2 diabetes and lipid abnormalities: A randomized, double-blinded, and placebo-controlled trial. PloS One (2014) 9:e91163. doi: 10.1371/JOURNAL.PONE.0091163
155. Curtis PJ, Dhatariya K, Sampson M, Kroon PA, Potter J, Cassidy A. Chronic ingestion of flavan-3-ols and isoflavones improves insulin sensitivity and lipoprotein status and attenuates estimated 10-year CVD risk in medicated postmenopausal women with type 2 DiabetesA 1-year, double-blind, randomized, controlled trial. Diabetes Care (2012) 35:226–32. doi: 10.2337/DC11-1443
156. Shrime MG, Bauer SR, McDonald AC, Chowdhury NH, Coltart CEM, Ding EL. Flavonoid-rich cocoa consumption affects multiple cardiovascular risk factors in a meta-analysis of short-term studies. J Nutr (2011) 141:1982–8. doi: 10.3945/JN.111.145482
157. Hooper L, Kay C, Abdelhamid A, Kroon PA, Cohn JS, Rimm EB, et al. Effects of chocolate, cocoa, and flavan-3-ols on cardiovascular health: a systematic review and meta-analysis of randomized trials. Am J Clin Nutr (2012) 95:740–51. doi: 10.3945/AJCN.111.023457
158. Ock KC, Sang JC, Song WO. Estimated dietary flavonoid intake and major food sources of U.S. adults. J Nutr (2007) 137:1244–52. doi: 10.1093/JN/137.5.1244
159. Wedick NM, Pan A, Cassidy A, Rimm EB, Sampson L, Rosner B, et al. Dietary flavonoid intakes and risk of type 2 diabetes in US men and women. Am J Clin Nutr (2012) 95:925–33. doi: 10.3945/AJCN.111.028894
160. Knekt P, Kumpulainen J, Järvinen R, Rissanen H, Heliövaara M, Reunanen A, et al. Flavonoid intake and risk of chronic diseases. Am J Clin Nutr (2002) 76:560–8. doi: 10.1093/AJCN/76.3.560
161. Lachance H, Wetzel S, Kumar K, Waldmann H. Charting, navigating, and populating natural product chemical space for drug discovery. J Med Chem (2012) 55:5989–6001. doi: 10.1021/JM300288G
162. Atanasov AG, Zotchev SB, Dirsch VM, Orhan IE, Banach M, Rollinger JM, et al. Natural products in drug discovery: advances and opportunities. Nat Rev Drug Discovery (2021) 20:200–16. doi: 10.1038/s41573-020-00114-z
163. Feher M, Schmidt JM. Property distributions: differences between drugs, natural products, and molecules from combinatorial chemistry. J Chem Inf Comput Sci (2003) 43:218–27. doi: 10.1021/CI0200467
164. Bucar F, Wube A, Schmid M. Natural product isolation–how to get from biological material to pure compounds. Nat Prod Rep (2013) 30:525–45. doi: 10.1039/C3NP20106F
165. Delazar A, Nahar L, Hamedeyazdan S, Sarker SD. Microwave-assisted extraction in natural products isolation. Methods Mol Biol (2012) 864:89–115. doi: 10.1007/978-1-61779-624-1_5
166. Sarker SD, Nahar L. An introduction to natural products isolation. Methods Mol Biol (2012) 864:1–25. doi: 10.1007/978-1-61779-624-1_1
167. da Silva Haas IC, de Espindola JS, de Liz GR, Luna AS, Bordignon-Luiz MT, Prudêncio ES, et al. Gravitational assisted three-stage block freeze concentration process for producing enriched concentrated orange juice (Citrus sinensis l.): Multi-elemental profiling and polyphenolic bioactives. J Food Eng (2022) 315:110802. doi: 10.1016/J.JFOODENG.2021.110802
168. Meneses DL, Ruiz Y, Hernandez E, Moreno FL. Multi-stage block freeze-concentration of green tea (Camellia sinensis) extract. J Food Eng (2021) 293:110381. doi: 10.1016/J.JFOODENG.2020.110381
169. Orellana-Palma P, Guerra-Valle M, Gianelli MP, Petzold G. Evaluation of freeze crystallization on pomegranate juice quality in comparison with conventional thermal processing. Food Biosci (2021) 41. doi: 10.1016/J.FBIO.2021.101106
170. Kumar D, Ladaniya MS, Gurjar M, Kumar S. Impact of drying methods on natural antioxidants, phenols and flavanones of immature dropped citrus sinensis l. osbeck fruits. Sci Rep (2022) 12:1–12. doi: 10.1038/s41598-022-10661-7
171. Zhang F, Zhang J, Di H, Xia P, Zhang C, Wang Z, et al. Effect of long-term frozen storage on health-promoting compounds and antioxidant capacity in baby mustard. Front Nutr (2021) 8:665482/BIBTEX. doi: 10.3389/FNUT.2021.665482/BIBTEX
172. Pinzi L, Rastelli G. Molecular docking: Shifting paradigms in drug discovery. Int J Mol Sci (2019) 20. doi: 10.3390/IJMS20184331
173. Dewanjee S, Chakraborty P, Mukherjee B, de Feo V. Plant-based antidiabetic nanoformulations: The emerging paradigm for effective therapy. Int J Mol Sci (2020) 21. doi: 10.3390/IJMS21062217
174. Ganesan P, Ramalingam P, Karthivashan G, Ko YT, Choi DK. Recent developments in solid lipid nanoparticle and surface-modified solid lipid nanoparticle delivery systems for oral delivery of phyto-bioactive compounds in various chronic diseases. Int J Nanomed (2018) 13:1569–83. doi: 10.2147/IJN.S155593
175. Saratale GD, Saratale RG, Benelli G, Kumar G, Pugazhendhi A, Kim DS, et al. Anti-diabetic potential of silver nanoparticles synthesized with argyreia nervosa leaf extract high synergistic antibacterial activity with standard antibiotics against foodborne bacteria. J Clust Sci (2017) 28:1709–27. doi: 10.1007/S10876-017-1179-Z
Keywords: dipeptidyl peptidase-IV, insulin resistance, incretin, polyherbal formulations, antioxidants, hormonal disorder
Citation: Chhabria S, Mathur S, Vadakan S, Sahoo DK, Mishra P and Paital B (2022) A review on phytochemical and pharmacological facets of tropical ethnomedicinal plants as reformed DPP-IV inhibitors to regulate incretin activity. Front. Endocrinol. 13:1027237. doi: 10.3389/fendo.2022.1027237
Received: 24 August 2022; Accepted: 18 October 2022;
Published: 11 November 2022.
Edited by:
Brian Yee Hong Lam, University of Cambridge, United KingdomReviewed by:
Sudhanshu Kumar Bharti, Patna University, IndiaCopyright © 2022 Chhabria, Mathur, Vadakan, Sahoo, Mishra and Paital. This is an open-access article distributed under the terms of the Creative Commons Attribution License (CC BY). The use, distribution or reproduction in other forums is permitted, provided the original author(s) and the copyright owner(s) are credited and that the original publication in this journal is cited, in accordance with accepted academic practice. No use, distribution or reproduction is permitted which does not comply with these terms.
*Correspondence: Biswaranjan Paital, Ymlzd2FyYW5qYW5wYWl0YWxAZ21haWwuY29t; Dipak Kumar Sahoo, ZHNhaG9vQGlhc3RhdGUuZWR1; ZGlwYWtzYWhvbzExQGdtYWlsLmNvbQ==
Disclaimer: All claims expressed in this article are solely those of the authors and do not necessarily represent those of their affiliated organizations, or those of the publisher, the editors and the reviewers. Any product that may be evaluated in this article or claim that may be made by its manufacturer is not guaranteed or endorsed by the publisher.
Research integrity at Frontiers
Learn more about the work of our research integrity team to safeguard the quality of each article we publish.