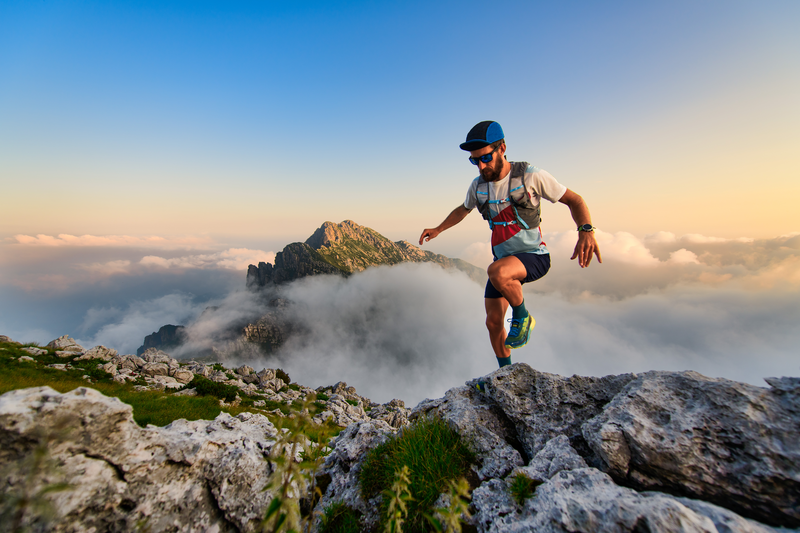
94% of researchers rate our articles as excellent or good
Learn more about the work of our research integrity team to safeguard the quality of each article we publish.
Find out more
SYSTEMATIC REVIEW article
Front. Endocrinol. , 11 October 2022
Sec. Clinical Diabetes
Volume 13 - 2022 | https://doi.org/10.3389/fendo.2022.1022989
This article is part of the Research Topic Endocrine Disruptors, Epigenetics, and Diabetes: An Intricate Relationship View all 7 articles
Background: Diabetes-induced reproductive complications can lead to subfertility and infertility, raising the need to protect reproductive organs. There are limited medications used to improve reproductive health in diabetic patients. Melatonin, mainly produced by the pineal gland, may improve diabetes-associated reproductive complications through various mechanisms and may be a preferred candidate to protect the reproductive system. The present review aims to elucidate the underlying mechanisms of melatonin’s effect on the reproductive system adversely affected by diabetes mellitus (DM).
Methods: A comprehensive systematic literature electronic search was done using the PRISMA guidelines. Web of Science, PubMed, Embase, and Scopus were searched for publications up to June 2022. Search terms were selected based on the study purpose and were explored in titles and abstracts. After screening, out of a total of 169 articles, 14 pertinent articles were included based on our inclusion and exclusion criteria.
Results: The results of studies using rats and mice suggest that DM adversely affects reproductive tissues, including testes and epididymis, prostate, corpus cavernosum, and ovary leading to alterations in histological and biochemical parameters compared to the normal groups. Treatment with melatonin improves oxidative stress, blocks apoptosis induced by endoplasmic reticulum stress and caspase activation, reduces pro-inflammation cytokines, and enhances steroidogenesis.
Conclusion: Melatonin exerted a protective action on the impaired reproductive system in in-vivo and in-vitro models of DM. The topic has to be followed up in human pregnancy cases that will need more time to be collected and approved.
Diabetes mellitus (DM), a severe metabolic disorder, results from impaired insulin production or dysfunction (1). The vast majority of people with type I diabetes are diagnosed before they reach the age of 30. According to a recent report, type I diabetes is increasing by 3% each year in European children, and an alarming number of children and young adolescents are being diagnosed with type II diabetes (2). DM is one of the most significant stresses in current public health due to its consequences caused by persistent hyperglycemia, including cardiovascular disease, nephropathy, retinopathy, neuropathy, and male and female reproductive injury (3–5). New research concerning the fertility rate in modern communities shows that an increase in the frequency of DM is linked to a decrease in birth and fertility rates. Similar results were also obtained in animal models (6). Recent studies have discovered that DM can be associated with various male reproductive complications, including decreased libido and impotence, erectile dysfunction, abnormal sperm motility and morphology, degenerative and apoptotic alterations in testis, and changes in the levels of hormones, including luteal hormone (LH), follicular stimulating hormone (FSH), and testosterone leading to subfertility and infertility (7). Although the prevalence of reproductive dysfunction in women is lower than in men, some studies have shown a decreased birth rate in diabetic women and reproductive malfunction due to ovarian dysfunction (8).
Moreover, in diabetic women, abnormalities in the menstrual cycle, delayed puberty, amenorrhea, and subsequent infertility have been documented (8–10). The exact mechanisms involved in reproductive dysfunction do not entirely elucidate. The biochemical mechanism underlying the changes in male reproductive capacity caused by DM has been the subject of several clinical and animal research. Endocrine problems, neuropathy, elevated oxidative stress and increased level of advanced glycation end products (AGE) are among them (11, 12). As the studies show, diabetes causes considerable oxidative stress in females, which leads to DNA damage. DNA damage causes cell cycle arrest and cell death, preventing the development of oocytes (13). Hyperglycemia is regarded as one of the major causes of DM-induced complications by activating a range of damaging pathways that appear to be initiated by mitochondrial superoxide overproduction. Transient episodes of hyperglycemia produce tissue damage through processes requiring recurrent acute alterations in cellular metabolism (14). It is well acknowledged that proper diabetes management, namely glycemic control, is critical in minimizing, preventing, or mitigating diabetic complications (15). Nevertheless, routine approved treatments do not reduce long-term issues, and few specific reproductive medications are available for diabetic patients (16, 17). According to recent research, antioxidant therapy lowers the glycemic index, minimizes diabetes complications, and protects against oxidative stress caused by free radicals. It may be a suitable therapeutic option for DM-induced complications (17, 18).
Melatonin (N-acetyl-5-methoxytryptamine) is an indoleamine neurohormone mainly secreted by the pineal gland and locally by several tissues (19, 20). This neurohormone with hydrophilic and lipophilic structure can easily cross all biological barriers and reach a high concentration in intracellular components, including mitochondria, the leading centers for reactive oxygen species (ROS) generation (21, 22). Melatonin exerts several physiological functions such as maintaining the body’s homeostasis, modulation of circadian rhythm, control of neuroendocrine axis, broad-spectrum antioxidant action, reproductive function regulation by affecting steroidogenesis and testicular development, rule of endoplasmic reticulum stress, anti-inflammatory, anti-tumor, anti-aging effect, etc. (23–28).
Oxidative stress induced by many diseases like DM has a prominent role in triggering many complications that can probably be alleviated by the antioxidative effect of melatonin (29). So, this molecule can be a potential candidate for preventing and mitigating DM complications (27).
This review focuses on the role of melatonin in diabetes-induced reproductive injury. Some points include diabetes’ impact on the reproductive system, melatonin’s potential effect on diabetic reproductive dysfunction, and the main mechanisms through which melatonin exerts the possible protective effect or prevents diabetes-induced reproductive dysfunction. So, we will discuss the resulting findings from non-clinical studies using melatonin on the reproductive components in diabetes.
The present systematic review was based on the previous guidelines (30).
A comprehensive literature search was performed in the following electronic databases, including Web of Sciences, PubMed, Scopus, and Embase, with the search terms up to June 2022 without restriction on publication year. The keywords used in this manuscript are in the supplementary file. After eliminating the duplicated studies, firstly, we screened all the studies in the title and abstract according to the abovementioned terms. The study’s full text was included in the second step while meeting our inclusion and exclusion criteria. In the present study, inclusion criteria included 1) the studies based on our aims, 2) the studies with adequate data, 3) there was no limitation in in-vitro and in-vivo studies, and 4) the studies in the English language. Furthermore, we excluded the studies, which included the following exclusion criteria; 1) case reports, 2) review articles, 3) oral communications, 4) not available articles, 5) letters to the editor, and 6) book chapters. Three reviewers independently performed all the search and screen processes (MA, BB, and HHA). The reviewers excluded the studies that did not meet the present study’s aims.
Until June 2022, 281 articles were found after a thorough search of electronic databases. After removing duplicated articles (n=112), 169 articles were screened in titles and abstracts, and 124 were eliminated. For further evaluation of their full text, 45 articles met the (inclusion and exclusion) criteria. In the end, 14 papers were eligible for inclusion in the systematic review. Figure 1 depicts the process of conducting a literature search and screening.
The data of each article includes 1) The first author and year of publication, 2) Models (in-vitro or in-vivo) and duration, 3) Diabetes induced agent (dosage) and route of administration, 4) Outcomes of reproductive system impairment, 5) Melatonin dosage & route of administration/duration of administration, 6) Melatonin administration outcomes, were independently extracted and organized by MA and BB, entered in Table 1 and checked by HHA.
The results of the studies indicated that experimental DM significantly reduced the epididymis weights or masses (31, 32, 37). In comparison, melatonin administration could increase the weight of epididymis (31, 32). Induction of DM caused a mild increase in glutathione peroxidase (GPx) activity than the control group that was not affected by melatonin treatment (37). Also, DM induced degeneration of germ cells in the ductal lumen, induced atrophy of epididymal cauda, and disturbed the epididymal duct wall (3, 31). The diabetic subjects treated with melatonin showed minor histological changes (3).
According to the results, although DM did not meaningfully affect testes’ weights in most reports (3, 31, 35, 37), it significantly reduced testis weights in two studies (3, 32). Treatment with melatonin increased testes weight in a study (36). Evaluation of the results showed that DM significantly increased the levels of thiobarbituric acid reactive substances (TBARs), malondialdehyde (MDA), 8-hydroxydeoxyguanosine (8-OHdG), as well as (glutathione-S-transferase) GST activity, reduced glutathione (GSH) levels, and catalase (CAT) activity, and caused an imbalance (elevation or reduction) in superoxide dismutase (SOD) activity (33, 35–37). In comparison, melatonin treatment could balance the parameters mentioned above in diabetic subjects (33, 35, 36). DM-induced hyperglycemia significantly up-regulated 78-KD glucose-regulated protein (Grp78), C/EBP homologous protein (CHOP), caspase-12, P53, and caspase-3 genes, increased protein expressions of Grp78, CHOP, apoptotic proteins such as P53 and caspase-3, elevated tumor necrosis factor-alpha (TNF-α), and interleukin-6 (IL-6) also reduced ratio of Bcl-2/Bax. Treatment with melatonin could mainly normalize these altered parameters (3, 36). Histological examination in the studies showed various abnormalities in testis tissue, such as congestion, loss of Leydig cells and spermatogonial stem cells (SSCs), increased seminiferous epithelium and Leydig cells volume with nuclear atrophy, reduction in the tubular diameter and the height of the seminiferous epithelium, the elevation of the Seminiferous Tubules Basement Membrane (STBM) thickness, and disorganization and depletion of germ cells and vacuolization of Sertoli cells in the diabetic group (3, 31, 33, 34, 36). The diabetic subjects treated with melatonin showed less histological injury in testis tissue (3, 31, 33, 34, 36).
Evaluation of the results showed a reduction in sperm concentration or density, the number of progressively motile spermatozoa, and transit time in the caudal segment of the epididymis, as well as an increase in sperm abnormalities, premature acrosome reaction rates, and immotile sperms in un-treated diabetic groups (3, 31, 33). However, treatment with melatonin was associated with increased sperm count and transit time and improvement of sperm abnormalities and motility (3, 31).
The results indicated decreased prostate weight in diabetic groups compared to the control group. Melatonin treatment reversed prostate weight to normal (37, 38). Also, experimental DM caused elevation in GPx, CAT, GST, and MDA content balanced by melatonin treatment (37). Moreover, DM caused various histological abnormalities indicated by the reduced absolute frequency of epithelium, thinner and interrupted smooth muscle cell (SMC) layers, increased apoptotic cells, neoplastic lesions, and metaplasia. Melatonin administration mainly improved the alterations above (37, 38).
Hyperglycemia causes alterations in various parameters in the cavernosal tissue. It significantly increased MDA, 8-OHdG levels, and caspase-3 activity while reducing GSH content, cyclic guanosine monophosphate (cGMP) level, sirtuin-1 (SIRT-1), and endothelial nitric oxide synthase (e-NOS) protein expression in diabetic rats. In contrast, melatonin administration markedly balanced the changes in these parameters (40, 41). The assessment of pathological changes in the tissue represented a reduction of endothelial density and smooth muscle ratio to collagen, cytoplasmic vacuolization in the endothelial cells, and vascular congestion mitigated by co-treatment with melatonin (39, 41).
Based on our search strategy, just one study was found in this area.
The present investigation demonstrated that untreated DM caused increased MDA level, decreased SOD and CAT activity, imbalanced total oxidant and antioxidant status, and elevated Nuclear Factor Kappa B (NF-κB) and caspase-3 levels. The results indicated that melatonin could reverse the alterations in the parameters mentioned above. Moreover, histological findings represented stromal fibrosis, increased follicular degeneration, and hemorrhage normalized by melatonin co-treatment (42).
Hyperglycemic rats were reported to have altered steroidogenesis, exhibiting decreased serum testosterone levels (31, 36, 37, 41). Melatonin treatment prevented the drop in circulating levels of testosterone due to DM (31, 36, 37, 41).
Diabetes induction resulted in decreased androgen receptors (AR) in the testis. In contrast, melatonin could moderately increase the value (36). Based on another study, the rate of ARs elevated in the untreated DM group was non-significantly reduced after melatonin treatment, while after eight weeks, melatonin increased ARs in testis (31). In a study, the AR rate in the prostate decreased in the short period of DM (1 week), whereas it significantly increased in long-term DM (8 weeks). Melatonin abolished the increase of ARs in long-term DM (37).
The present review evaluates the mechanisms involved in DM-induced damage to reproductive organs and the effect of melatonin administration on the reproductive system affected by hyperglycemia. The included studies used STZ or alloxan, leading to type 1 diabetes. Some of the crucial mechanisms involved in DM-induced reproductive injury are illustrated in the Figure 2.
Figure 2 The noticed mechanisms involved in diabetes-related cellular damage. Hyperglycemia triggers excessive ROS production leading to the cell membrane and DNA damage, induces apoptosis signaling pathways, and causes inflammation. ↑increased by hyperglycemia; ROS, reactive oxygen species; MDA, malondialdehyde; TBARs, thiobarbituric acid reactive substances; NF-κB, nuclear factor-kappa-β; IL-6, interleukin 6; TNF-α, tumor necrosis factor-alpha; GRP78, 78-KD glucose-regulated protein; CHOP, C/EBP homologous protein; Cyt c, cytochrome C.
Based on the evidence, DM can adversely affect various organs, among which reproductive injury has currently attracted much attention (43). Based on several findings, melatonin has roles in testicular function, the self-renewal of mouse SSCs, spermatogenesis, and oogenesis, demonstrating its beneficial effect on reproduction activity. Furthermore, different models evaluated melatonin as a potent antioxidant and anti-inflammatory molecule in mitigating reproductive damage (44, 45). In the following parts, we will focus on the mechanisms of melatonin on the reproductive system malfunction during the diabetic condition.
In a physiological state, free radicals, as products of cell physiological processes, are maintained at equilibrium by antioxidant system activity (46). While in pathological conditions, accumulating large amounts of free radicals can exceed the antioxidant defense capacity, leading to oxidative stress (47, 48). A large body of evidence has demonstrated that an increased amount of ROS and weakened antioxidant defense caused by hyperglycemia result in oxidative stress causing damage to various cell macromolecules such as proteins, lipids, and nucleic acids (8, 49). Hyperglycemia causes severe oxidative stress through glucose auto-oxidation, multiple proteins glycation, and polyol pathway induction (46). In this condition, mitochondria are the primary oxidative stress sources. Indeed, during mitochondrial oxidative metabolism, the excess oxygen is converted to oxygen free radical (O-), known as the main ROS that is transformed to other reactive species such as superoxide anion , hydroxyl (OH-), hydrogen peroxide (H2O2), and peroxynitrite (ONOO-) contributing to oxidative or nitrosative stress progress (50, 51). DM-related oxidative stress can induce malfunction in genital organs and subsequent infertility (31). The detrimental effect of excessive ROS on various reproductive tissues, including testis, prostate, and corpus cavernosum, is associated with lipid peroxidation characterized by elevated TBARS and MDA levels leading to cell membrane disruption (36, 37, 41).
Moreover, severe oxidative stress potentially contributes to DNA damage indicated by increased cellular 8-oxo, 2’-deoxyguanosine (8-oxodG), as a sensitive biomarker (52). Oxidative stress in sperm may interfere with membrane function, damage DNA, and negatively affect motility (33). GSH, a tripeptide molecule, potentially protects the cell against reactive species. Oxidative stress caused by hyperglycemia can deplete testis tissue GSH levels due to its oxidation to GSH disulfide (GSSG) (33, 36). Also, the alterations in the intracellular antioxidant enzymes (CAT, SOD, GPx, GST) involved in tissue protection may occur in response to oxidative stress resulting in tissue damage (33, 35, 37). Findings of a study have demonstrated that oxidative stress induced by experimental DM can make an antioxidant system response in male reproductive organs, particularly in prostate tissue that shows more vulnerability to hyperglycemia than the testis and epididymis, possibly due to different histopathologic characteristics and patterns of antioxidant enzymes expression (37). However, these findings contrasted with other research that found substantial alterations in testicular antioxidant enzymes in diabetic rats (33, 35). While the exact reason for these discrepancies is unclear, it may partially be explained by differences in the type and dosage of the chemicals used for induction of diabetes, the experiments’ methodology, and length.
Melatonin is a neurohormone with potent antioxidant action that readily crosses the cell membranes and biological barriers such as the brain and testis blood barriers (27, 34). By route, melatonin administration causes a fast rise in blood melatonin concentrations. It can reach a high level in mitochondria, the leading source of free radicals. Melatonin’s extensive subcellular distribution may minimize oxidative damage in the cell’s lipid and aqueous environments. Melatonin has an advantage over other antioxidants since it more slowly infiltrates cells (21). The antioxidant effect of melatonin is due to its direct and indirect antioxidant action. This molecule and its metabolites scavenge and detoxify various free radicals like superoxide anion, singlet oxygen, peroxyl radicals, and peroxynitrite anion. It also mitigates oxidative stress by enhancing antioxidative defense capacity, and regulating the expression and activity of multiple antioxidant enzymes and GSH content (53). Melatonin through melatonin receptor 1 (MT1) and interaction with calmodulin can inactivate the nuclear ROR-alpha melatonin receptor, a nuclear receptor, resulting in a modulation of antioxidant enzyme gene expression (54). So, melatonin can normalize the altered activity of SOD as a crucial enzyme in converting the superoxide anion into H2O2 and molecular oxygen.
Moreover, it can balance the hyperglycemia-induced changes in CAT and GPx as the enzymes neutralize H2O2, without which the high level of H2O2 will damage lipid membranes, causing the production of excessive MDA. This antioxidant molecule can normalize GST activity as an enzyme catalyzing the conjugation of GSH to various electrophilic molecules and possibly a diabetes-induced specific marker of prostate oxidative stress (37). GSH is regenerated by reducing GSSG due to the glutathione reductase (GR) enzyme’s function via nicotinamide adenine dinucleotide phosphate (NADPH) as a co-factor. Melatonin, possibly through its antioxidant action, up-regulation of cell GSH, and increased g-glutamylcysteine, can improve cellular GSH content (33, 36, 40). The antioxidant ability of melatonin was demonstrated when used to inhibit lipid peroxidation in testis tissue of diabetic rats. This decrease in lipid peroxidation is most likely due to the direct neutralization of free radicals and the indirect antioxidant effect through modulating the GSH levels and activity of CAT and SOD, resulting in less testis histopathological damage (33, 35, 36). Melatonin can restore sperm parameters and motility (3, 31). Although the indicators of oxidative stress in the diabetic rat epididymis did not alter (37), it is plausible that melatonin has a direct antioxidant effect on spermatozoa (55).
Also, melatonin restores the activity of antioxidant enzymes (CAT, GPx, GST) and reduces LPO in the prostate of diabetic rats, demonstrating its potent antioxidant action in this organ (37). Noteworthy, melatonin protects cavernosal tissue in type 1 diabetic rats by increasing GSH levels, reducing oxidative tissue damage and the harmful impact of free radicals on nitric oxide activity, contributing to corporal function improvement (40, 41).
Apoptosis is a controlled cell death playing a crucial role in maintaining cellular homeostasis (56, 57). Any disturbances regulating this pathway lead to cellular abnormalities and diseases (58, 59). Apoptosis can initiate in three main ways: extrinsic, intrinsic mitochondrial, and intrinsic endoplasmic reticulum pathways. Evidence suggests that glucose autooxidation-induced ROS production may be a driving force for activating the mitochondria-dependent path (60, 61). Indeed, the imbalance between anti-apoptotic proteins such as Bcl-2, B-cell lymphoma-extra-large (Bcl-xL), and also pro-apoptotic agents, including Bcl-2 associated agonist of cell death (Bad), BH3 interacting-domain death agonist (Bid), and Bcl-2-associated X (Bax) may occur due to activation of p53 induced by the high level of ROS (62, 63). These alterations lead to disruption in the outer mitochondrial membrane and release of cytochrome c involved in the apoptosome formation, a complex consisting of apoptotic protease activating factor-1 (Apaf1) and procaspase-9, which cleave procaspase-9 into caspase-9. Caspase-9 can finally activate caspase-3, which starts DNase and degrades DNA (64, 65). Based on the evidence, melatonin, through affecting its receptors, induces cellular signal transduction, leading to Bcl-2 up-regulation and re-localization to mitochondria, where Bax acts as a pro-apoptotic protein (66, 67). Notably, it prevents hyperglycemia-induced ovarian apoptosis by inducing antioxidant enzymes and inhibiting the caspase-3 pathway involved in the pathophysiology of ovarian injury (42). In cavernosal tissue, reduced caspase-3 activity reveals that melatonin might delay apoptosis by minimizing oxidative stress and improving tissue integrity (41).
The endoplasmic reticulum (ER), an organelle responsible for protein organization, sensitively reacts to homeostasis disturbance (68). Various stimuli, including nutrient restriction, altered glycosylation of proteins, and oxidative stress, are linked to the interference of proper protein folding, resulting in the accumulation of misfolded proteins and ER stress (ERS). ERS stimulates a group of signaling pathways recognized as the unfolded protein response (UPR). There are three main sensor proteins involved in UPR, including inositol-requiring protein-1 (IRE1), induced activation of transcription factor 6 (ATF6), and protein kinase RNA (PKR)-like ER kinase (PERK) that respond to the accumulation of misfolded proteins in the ER lumen and protect ER function by a reduction in protein translation rate (69). GRP78, an ER chaperone, is the primary regulator of UPR that prevents the activation of sensor proteins in resting cells (70, 71). Nevertheless, GRP78 is liberated from these transmembrane proteins in stressed cells, allowing them to activate their UPR pathways (70). A high glucose environment can shift the UPR pathway to an apoptotic program and promote the expression of the nuclear transcription factor CHOP and caspase-12 in Leydig cells (3). CHOP suppresses the production of Bcl-2, making cells more susceptible to apoptosis (72). Moreover, activated caspase-12 translocates to the cytoplasm and activates caspase-9 and, subsequently, caspase-3 playing a leading role in cellular death (73).
In diabetic conditions, melatonin can reduce the apoptosis rate induced by ERS by inhibiting Grp78, CHOP, caspase-12, and their downstream cascades. This effect of melatonin provides a more intact niche for spermatogonial stem cells to self-renew, maintaining sperm healthier for fertility (3).
Inflammation is an immune system reaction to harmful factors that account for the induction of several cellular signals. Excessive generation of various pro-inflammatory cytokines during the process worsens this response (74, 75). Studies have demonstrated that DM can impair reproductive organs by inducing inflammation. In this regard, experimental DM-induced oxidative stress triggers up-regulation of nuclear factor kappa B (NF-κB), a primary regulator of inflammation (76). Indeed, oxidative stress activates IκB kinase (IKK) that is responsible for phosphorylation of inhibitor of nuclear factor kappa B (IκB-α) and activation of NF-κB, resulting in up-regulation of pro-inflammatory cytokines such as TNF-α, IL-6, interleukin 1 beta (IL1-β), and cyclooxygenase-2 (COX-2) (54, 77). According to several studies, melatonin can decrease chronic and acute inflammation. It has been shown that melatonin reduces NF-κB translocation and downregulates inflammatory cytokines by blocking the activation of IKK and IκB-α, leading to an anti-inflammatory and anti-apoptotic action (77, 78). Melatonin benefits spermatogenesis in diabetic rats by reducing inflammatory cytokines such as TNF-α and IL6 (36). This molecule mitigates the detrimental impact of diabetes-induced oxidative stress on the ovaries via the NF-κB pathway, leading to reduced tissue injury such as fibrosis of the stroma and follicular degeneration (42).
In a well-functioning hypothalamic pituitary gonadal (HPG) axis, pulses of gonadotropin-releasing hormone (GnRH) produced by the hypothalamus induce secretion of follicle-stimulating hormone (FSH) and luteinizing hormone (LH) from the pituitary gland (16). FSH and LH stimulate spermatogenesis by acting on the Sertoli and Leydig cells. FSH binds to its receptor, expressed only by Sertoli cells, increasing cyclic adenosine monophosphate (cAMP) levels and stimulating the synthesis of androgen-binding protein (ABP) and release of LH, which controls testosterone production in Leydig cells (79, 80). Spermatogenesis fails to go through the meiosis stage when testosterone or androgen receptors are absent (81). The detrimental effect of DM on testosterone production and spermatogenesis may be related to the disturbed production and release of gonadotropins due to the link between the neurological and endocrine systems, causing structural alterations in Sertoli and Leydig cells (16, 82). Besides, the marked testosterone decrease in experimental diabetes can be linked to the lack of insulin because insulin can affect Leydig cells as a paracrine factor (36). Notably, melatonin modulates androgen release in Leydig cells via binding to a melatonin membrane receptor. In this regard, steroid production is accelerated when phosphorylated cyclic AMP response element-binding protein (CREB) binds to the steroidogenic acute regulatory protein (StAR) promoter’s cAMP response element (83). Some investigations have shown that rising androgen levels are predominantly due to melatonin’s stimulatory impact on the steroidogenic enzyme 3-hydroxysteroid dehydrogenase, an enzyme in the adrenal gland that catalyzes the synthesis of androstenedione from dehydroepiandrosterone (DHEA) (84). Melatonin can also affect the activity of the HPG axis by influencing GnRH production via binding sites in the suprachiasmatic nucleus, hypothalamic premammillary, and mediobasal hypothalamus (85). Besides, it has been manifested that melatonin improves spermatogenesis by improving glucose metabolism, facilitating glucose transport activity, and stimulating the Krebs cycle in Sertoli cells (86). The metabolism of Leydig cells may be similarly enhanced by exogenous melatonin in the Type 1 DM model, leading to the improvement of steroidogenesis. However, melatonin’s effect on Leydig cells must be verified (31). Melatonin ingestion increases AR expression in the testes, suggesting altering androgen signaling. The improvement in testosterone production might be explained by the enhanced AR expression generated by melatonin therapy since experiments with null animals for this receptor revealed decreased levels of 17β- hydroxysteroid dehydrogenase and 3β-hydroxysteroid dehydrogenase expression (31, 87).
Persistent hyperglycemia due to diabetes is accompanied by long-term injury, malfunction, and failure of various organs (88). Diabetes is also associated with reproductive impairment in both genders. Its negative effect on reproduction can be substantial, as seen by decreased fertility and reproductive failure (89). The studies show that precise glycemic control combined with antioxidant treatment can help reduce the risk of harmful diabetic outcomes (2). Studies have implied melatonin’s role in modulating several signaling pathways and indicated its preventive and therapeutic effects in various diseases, such as DM. Many animal and human research studies have shown the anti-diabetic effect of melatonin and its leading role in reducing DM-related complications, including cardiomyopathy, nephropathy, retinopathy, and neuropathy (27, 90). The present study demonstrated that melatonin administration could be associated with declining oxidative stress, blocking the apoptosis signaling pathway, modulating endoplasmic reticulum function, and reducing inflammation in various reproductive components (3, 32, 33, 35–38). Also, according to most of the findings, melatonin can positively affect androgen levels in diabetic male subjects (31, 36, 37, 41).
Nevertheless, It should be noticed that in experimental studies, the factors including disease length, glycemic levels, and the direct destructive effect of the chemicals used to induce diabetes on various tissues may conceal the true impact of diabetes on fertility during the investigation. The use of the induced type 1 diabetic animal models in the present studies may not accurately mimic the pathologic processes of the other form of diabetes. Indeed, type I and type II diabetic studies differ in animal models used in various aspects, including insulin resistance and body mass index. Besides, Type I diabetes is less common than type II diabetes in the community. Additionally, the included studies do not explain the interaction between DM and melatonin on females’ sexual hormones, and this aspect of the story remains unexplored. Therefore, further research on mechanisms exerted by melatonin in diabetic reproductive dysfunction in multiple elements of pre- and clinical studies will improve our insights.
Overall, DM is responsible for abnormalities in male and female reproductive system components, including ovary, corpus cavernosum, testis, epididymis, prostate, sperm concentration and motility, and testosterone level through several mechanisms. During the hyperglycemic condition, oxidative stress, apoptosis, inflammation, histological injury, and disturbance in androgen hormone will affect normal cells of the reproductive system leading to fertility disorders. Melatonin supplementation positively affects the reproductive tissues through several mechanisms. Melatonin neutralizes reactive species, indirectly modulates antioxidant enzymes, balances apoptotic and anti-apoptotic agents, downregulates the factors related to endoplasmic reticulum stress, inhibits pro-inflammatory cytokines, and normalizes testosterone levels. According to the present results, melatonin protects the reproductive organs during DM-induced hyperglycemia in non-clinical models, which must be proven in a clinical setting.
The original contributions presented in the study are included in the article/Supplementary Material. Further inquiries can be directed to the corresponding authors.
MAr gave the idea, extracted data, and drafted some parts of the manuscript. BB did the literature search and screening, extracted data, and drafted some parts of the manuscript. HH-A did the literature search and screening, prepared figures, and supervised the study. RF, MAt, SH, MS, and MHS drafted some parts of the manuscript and provided comprehensive revision and editing. MAb conceived the study, comprehensively edited the manuscript, and supervised the process. All authors read and approved the final version.
The authors wish to thank Tehran University of Medical Sciences and Ardabil University of Medical Sciences for providing the full text of the articles needed. The authors wish to thank INSF for the general Seat Award directed to MAb.
The authors declare that the research was conducted in the absence of any commercial or financial relationships that could be construed as a potential conflict of interest.
All claims expressed in this article are solely those of the authors and do not necessarily represent those of their affiliated organizations, or those of the publisher, the editors and the reviewers. Any product that may be evaluated in this article, or claim that may be made by its manufacturer, is not guaranteed or endorsed by the publisher.
The Supplementary Material for this article can be found online at: https://www.frontiersin.org/articles/10.3389/fendo.2022.1022989/full#supplementary-material
1. Vlad I, Popa AR. Epidemiology of diabetes mellitus: a current review. Romanian J Diabetes Nutr Metab Dis (2012) 19(4):433–40. doi: 10.2478/v10255-012-0050-0
2. Shi G-J, Li Z-M, Zheng J, Chen J, Han X-X, Wu J, et al. Diabetes associated with male reproductive system damages: Onset of presentation, pathophysiological mechanisms and drug intervention. Biomed pharmacother (2017) 90:562–74. doi: 10.1016/j.biopha.2017.03.074
3. Du Z, Xu S, Hu S, Yang H, Zhou Z, Sidhu K, et al. Melatonin attenuates detrimental effects of diabetes on the niche of mouse spermatogonial stem cells by maintaining leydig cells. Cell Death Dis (2018) 9(10):1–15. doi: 10.1038/s41419-018-0956-4
4. Kotian SR, Kumar A, Mallik SB, Bhat NP, Souza AD, Pandey AK. Effect of diabetes on the male reproductive system–a histomorphological study. J Morphol Sci (2019) 36(01):017–23. doi: 10.1055/s-0039-1683405
5. Rezaee MR, Amiri AA, Hashemi-Soteh MB, Daneshvar F, Emady-Jamaly R, Jafari R, et al. Aldose reductase c-106T gene polymorphism in type 2 diabetics with microangiopathy in Iranian individuals. Indian J Endocrinol Metab (2015) 19(1):95–9. doi: 10.4103/2230-8210.131762
6. Dinulovic D, Radonjic G. Diabetes mellitus/male infertility. Arch androl (1990) 25(3):277–93. doi: 10.3109/01485019008987617
7. Alves M, Martins A, Rato L, Moreira P, Socorro S, Oliveira P. Molecular mechanisms beyond glucose transport in diabetes-related male infertility. Biochim Biophys Acta (BBA)-Molecular Basis Dis (2013) 1832(5):626–35. doi: 10.1016/j.bbadis.2013.01.011
8. Amaral S, Oliveira PJ, Ramalho-Santos J. Diabetes and the impairment of reproductive function: possible role of mitochondria and reactive oxygen species. Curr Diabetes Rev (2008) 4(1):46–54. doi: 10.2174/157339908783502398
9. Yapca OE, Turan MI, Borekci B, Akcay F, Suleyman H. Bilateral ovarian ischemia/reperfusion injury and treatment options in rats with an induced model of diabetes. Iranian J Basic Med Sci (2014) 17(4):294.
10. Yeshaya A, Orvieto R, Dicker D, Karp M, Ben-Rafael Z. Menstrual characteristics of women suffering from insulin-dependent diabetes mellitus. Int J fertility menopausal Stud (1995) 40(5):269.
11. Barkabi-Zanjani S, Ghorbanzadeh V, Aslani M, Ghalibafsabbaghi A, Chodari L. Diabetes mellitus and the impairment of male reproductive function: Possible signaling pathways. Diabetes Metab Syndrome: Clin Res Rev (2020) 14(5):1307–14. doi: 10.1016/j.dsx.2020.07.031
12. He Z, Yin G, Li QQ, Zeng Q, Duan J. Diabetes mellitus causes male reproductive dysfunction: A review of the evidence and mechanisms. In Vivo (2021) 35(5):2503–11. doi: 10.21873/invivo.12531
13. Wu Y, Li Y, Liao X, Wang Z, Li R, Zou S, et al. Diabetes induces abnormal ovarian function via triggering apoptosis of granulosa cells and suppressing ovarian angiogenesis. Int J Biol Sci (2017) 13(10):1297. doi: 10.7150/ijbs.21172
14. Tavares R, Escada-Rebelo S, Silva A, Sousa M, Ramalho-Santos J, Amaral S. Antidiabetic therapies and male reproductive function: where do we stand? Reproduction (2018) 155(1):R13–37. doi: 10.1530/REP-17-0390
15. Lo H-C, Wasser SP. Medicinal mushrooms for glycemic control in diabetes mellitus: history, current status, future perspectives, and unsolved problems. Int J med mushrooms (2011) 13(5):401–26. doi: 10.1615/intjmedmushr.v13.i5.10
16. Schoeller EL, Schon S, Moley KH. The effects of type 1 diabetes on the hypothalamic, pituitary and testes axis. Cell Tissue Res (2012) 349(3):839–47. doi: 10.1007/s00441-012-1387-7
17. Rezvanfar MR, Heshmati G, Chehrei A, Haghverdi F, Rafiee F, Rezvanfar F. Effect of bedtime melatonin consumption on diabetes control and lipid profile. Int J Diabetes Developing Countries (2017) 37(1):74–7. doi: 10.1007/s13410-016-0497-2
18. Thakur P, Kumar A, Kumar A. Targeting oxidative stress through antioxidants in diabetes mellitus. J Drug Targeting (2018) 26(9):766–76. doi: 10.1080/1061186X.2017.1419478
19. Najafi M, Hooshangi Shayesteh MR, Mortezaee K, Farhood B, Haghi-Aminjan H. The role of melatonin on doxorubicin-induced cardiotoxicity: A systematic review. Life Sci (2020) 241:117173. doi: 10.1016/j.lfs.2019.117173
20. Nasrabadi NN, Sargazi F, Shokrzadeh M, Abediankenari S, Hoseini SV, Najafi M, et al. Expression of MT1 receptor in patients with gastric adenocarcinoma and its relationship with clinicopathological features. Neuro Endocrinol Lett (2018) 39(2):111–8.
21. Haghi-Aminjan H, Asghari MH, Farhood B, Rahimifard M, Hashemi Goradel N, Abdollahi M. The role of melatonin on chemotherapy-induced reproductive toxicity. J Pharm Pharmacol (2018) 70(3):291–306. doi: 10.1111/jphp.12855
22. Hajam YA, Rai S, Ghosh H, Basheer M. Combined administration of exogenous melatonin and insulin ameliorates streptozotocin induced toxic alteration on hematological parameters in diabetic male wistar rats. Toxicol Rep (2020) 7:353–9. doi: 10.1016/j.toxrep.2020.01.020
23. Sun FY, Lin X, Mao LZ, Ge WH, Zhang LM, Huang YL, et al. Neuroprotection by melatonin against ischemic neuronal injury associated with modulation of DNA damage and repair in the rat following a transient cerebral ischemia. J pineal Res (2002) 33(1):48–56. doi: 10.1034/j.1600-079X.2002.01891.x
24. Lampiao F, Du Plessis SS. New developments of the effect of melatonin on reproduction. World J Obstetrics Gynecol (2013) 2(2):8–15. doi: 10.5317/wjog.v2.i2.8
25. Farhood B, Mortezaee K, Haghi-Aminjan H, Khanlarkhani N, Salehi E, Nashtaei MS, et al. A systematic review of radiation-induced testicular toxicities following radiotherapy for prostate cancer. J Cell Physiol (2019) 234(9):14828–37. doi: 10.1002/jcp.28283
26. Sharma S, Singh H, Ahmad N, Mishra P, Tiwari A. The role of melatonin in diabetes: therapeutic implications. Arch Endocrinol Metab (2015) 59(5):391–9. doi: 10.1590/2359-3997000000098
27. Pourhanifeh MH, Hosseinzadeh A, Dehdashtian E, Hemati K, Mehrzadi S. Melatonin: new insights on its therapeutic properties in diabetic complications. Diabetol Metab Syndrome (2020) 12:1–20. doi: 10.1186/s13098-020-00537-z
28. Tarocco A, Caroccia N, Morciano G, Wieckowski MR, Ancora G, Garani G, et al. Melatonin as a master regulator of cell death and inflammation: molecular mechanisms and clinical implications for newborn care. Cell Death Dis (2019) 10(4):1–12. doi: 10.1038/s41419-019-1556-7
29. Nobakht-Haghighi N, Rahimifard M, Baeeri M, Rezvanfar MA, Moini Nodeh S, Haghi-Aminjan H, et al. Regulation of aging and oxidative stress pathways in aged pancreatic islets using alpha-lipoic acid. Mol Cell Biochem (2018) 449(1-2):267–76. doi: 10.1007/s11010-018-3363-3
30. Moher D, Liberati A, Tetzlaff J, Altman DG. Preferred reporting items for systematic reviews and meta-analyses: the PRISMA statement. PloS Med (2009) 6(7):e1000097. doi: 10.1371/journal.pmed.1000097
31. da Costa C, Gobbo M, Taboga S, Pinto-Fochi M, Góes RM. Melatonin intake since weaning ameliorates steroidogenic function and sperm motility of streptozotocin-induced diabetic rats. Andrology (2016) 4(3):526–41. doi: 10.1111/andr.12158
32. de Oliveira AC, Andreotti S, Sertie RAL, Campana AB, de Proenca ARG, Vasconcelos RP, et al. Combined treatment with melatonin and insulin improves glycemic control, white adipose tissue metabolism and reproductive axis of diabetic male rats. Life Sci (2018) 199:158–66. doi: 10.1016/j.lfs.2018.02.040
33. Akman O, Şengül E, Özkanlar S, Can S, Koc A, Karadeniz A, et al. The melatonin attenuates alloxan induced post-diabetic testicular damage and oxidative effects in rats. Kafkas Univ Vet Fak Derg (2015) 21(5):719–28. doi: 10.9775/kvfd.2015.13249
34. Guneli E, Tugyan K, Ozturk H, Gumustekin M, Cilaker S, Uysal N. Effect of melatonin on testicular damage in streptozotocin-induced diabetes rats. Eur Surg Res (2008) 40(4):354–60. doi: 10.1159/000118032
35. Armagan A, Uz E, Yilmaz HR, Soyupek S, Oksay T, Ozcelik N. Effects of melatonin on lipid peroxidation and antioxidant enzymes in streptozotocin-induced diabetic rat testis. Asian J Androl (2006) 8(5):595–600. doi: 10.1111/j.1745-7262.2006.00177.x
36. Alves ÉR, Ferreira CGM, da Silva MV, Vieira Filho LD, da Silva Junior VA, de Melo IMF, et al. Protective action of melatonin on diabetic rat testis at cellular, hormonal and immunohistochemical levels. Acta Histochem (2020) 122(5):151559. doi: 10.1016/j.acthis.2020.151559
37. Gobbo MG, Costa CFP, Silva DGH, de Almeida EA, Goes RM. Effect of melatonin intake on oxidative stress biomarkers in Male reproductive organs of rats under experimental diabetes. Oxid Med Cell Longevity (2015) 2015:614579. doi: 10.1155/2015/614579
38. Gobbo MG, Tamarindo GH, Ribeiro DL, de Campos SGP, Taboga SR, Goes RM. Pathological lesions and global DNA methylation in rat prostate under streptozotocin-induced diabetes and melatonin supplementation. Cell Biol Int (2018) 42(4):470–87. doi: 10.1002/cbin.10920
39. Qiu XF, Li XX, Chen Y, Lin HC, Yu W, Wang R, et al. Mobilisation of endothelial progenitor cells: one of the possible mechanisms involved in the chronic administration of melatonin preventing erectile dysfunction in diabetic rats. Asian J Androl (2012) 14(3):481–6. doi: 10.1038/aja.2011.161
40. Paskaloglu K, ?ener G, Ayanğolu-Dülger G. Melatonin treatment protects against diabetes-induced functional and biochemical changes in rat aorta and corpus cavernosum. Eur J Pharmacol (2004) 499(3):345–54. doi: 10.1016/j.ejphar.2004.08.002
41. Sahan A, Akbal C, Tavukcu HH, Cevik O, Cetinel S, Sekerci CA, et al. Melatonin prevents deterioration of erectile function in streptozotocin-induced diabetic rats via sirtuin-1 expression. Andrologia (2020) 52(9):9:e1363. doi: 10.1111/and.13639
42. Nayki U, Onk D, Balci G, Nayki C, Onk A, Cankaya M, et al. The effect of melatonin on oxidative stress and apoptosis in experimental diabetes mellitus-related ovarian injury. Gynecol Endocrinol (2016) 32(5):421–6. doi: 10.3109/09513590.2015.1126819
43. Gandhi J, Dagur G, Warren K, Smith N, Sheynkin YR, Zumbo A. The role of sexual dysfunction and infertility on reproductive health in diabetics: pathogenesis, evaluation, and management. Curr Diabetes Rev (2017) 13(6):573–81. doi: 10.2174/1573399813666161122124017
44. Olcese JM. Melatonin and female reproduction: an expanding universe. Front Endocrinol (2020) 11:85. doi: 10.3389/fendo.2020.00085
45. Sun T-C, Li H-Y, Li X-Y, Yu K, Deng S-L, Tian L. Protective effects of melatonin on male fertility preservation and reproductive system. Cryobiology (2020) 95:1–8. doi: 10.1016/j.cryobiol.2020.01.018
46. Maiese K, Daniela Morhan S, Zhong Chong Z. Oxidative stress biology and cell injury during type 1 and type 2 diabetes mellitus. Curr neurovascular Res (2007) 4(1):63–71. doi: 10.2174/156720207779940653
47. Hassani S, Maqbool F, Salek-Maghsoudi A, Rahmani S, Shadboorestan A, Nili-Ahmadabadi A, et al. Alteration of hepatocellular antioxidant gene expression pattern and biomarkers of oxidative damage in diazinon-induced acute toxicity in wistar rat: A time-course mechanistic study. EXCLI J (2018) 17:57–71. doi: 10.17179/excli2017-760
48. Moeini-Nodeh S, Rahimifard M, Baeeri M, Abdollahi M. Functional improvement in rats’ pancreatic islets using magnesium oxide nanoparticles through antiapoptotic and antioxidant pathways. Biol Trace element Res (2017) 175(1):146–55. doi: 10.1007/s12011-016-0754-8
49. Rahimifard M, Navaei-Nigjeh M, Mahroui N, Mirzaei S, Siahpoosh Z, Nili-Ahmadabadi A, et al. Improvement in the function of isolated rat pancreatic islets through reduction of oxidative stress using traditional Iranian medicine. Cell J (2014) 16(2):147–63.
50. Asmat U, Abad K, Ismail K. Diabetes mellitus and oxidative stress–a concise review. Saudi Pharm J (2016) 24(5):547–53. doi: 10.1016/j.jsps.2015.03.013
51. Sadeghiyan Galeshkalami N, Abdollahi M, Najafi R, Baeeri M, Jamshidzade A, Falak R, et al. Alpha-lipoic acid and coenzyme Q10 combination ameliorates experimental diabetic neuropathy by modulating oxidative stress and apoptosis. Life Sci (2019) 216:101–10. doi: 10.1016/j.lfs.2018.10.055
52. Shaikh H, Shrivastava VK, Amir M. Diabetes mellitus and impairment of male reproductive function: role of hypothalamus pituitary testicular axis and reactive oxygen species. Iranian J Diabetes Obes (2016) 8(1):41–50.
53. Hacışevki A, Baba B. An overview of melatonin as an antioxidant molecule: a biochemical approach. Melatonin Mol biol Clin Pharm approaches (2018) 5:59–85. doi: 10.5772/intechopen.79421
54. Tomás-Zapico C, Coto-Montes A. A proposed mechanism to explain the stimulatory effect of melatonin on antioxidative enzymes. J pineal Res (2005) 39(2):99–104. doi: 10.1111/j.1600-079X.2005.00248.x
55. Du Plessis S, Hagenaar K, Lampiao F. The in vitro effects of melatonin on human sperm function and its scavenging activities on NO and ROS. Andrologia (2010) 42(2):112–6. doi: 10.1111/j.1439-0272.2009.00964.x
56. Bameri B, Armandeh M, Baeeri M, Haghi-Aminjan H, Rahimifard M, Hassani S, et al. Electrocardiographic, hemodynamic, and biochemical evidence on the protective effects of exenatide against phosphine-induced cardiotoxicity in rat model. Hum Exp Toxicol (2021) 40(12_suppl):S381–96. doi: 10.1177/09603271211040819
57. Nejati K, Rastegar M, Fathi F, Dadashpour M, Arabzadeh A. Nanoparticle-based drug delivery systems to overcome gastric cancer drug resistance. JoDDS Technol (2022) 70:103231. doi: 10.1016/j.jddst.2022.103231
58. Haghi-Aminjan H, Asghari MH, Goharbari MH, Abdollahi M. A systematic review on potential mechanisms of minocycline in kidney diseases. Pharmacol reports: PR (2017) 69(4):602–9. doi: 10.1016/j.pharep.2017.02.001
59. Najafi M, Mortezaee K, Rahimifard M, Farhood B, Haghi-Aminjan H. The role of curcumin/curcuminoids during gastric cancer chemotherapy: A systematic review of non-clinical study. Life Sci (2020) 257:118051. doi: 10.1016/j.lfs.2020.118051
60. Samadi M, Haghi-Aminjan H, Sattari M, Shayesteh MRH, Bameri B, Armandeh M, et al. The role of taurine on chemotherapy-induced cardiotoxicity: A systematic review of non-clinical study. Life Sci (2020) 265:118813. doi: 10.1016/j.lfs.2020.118813
61. Zohre S, Kazem N-K, Abolfazl A, Mohammad R-Y, Aliakbar M, Effat A, et al. Trichostatin a-induced apoptosis is mediated by krüppel-like factor 4 in ovarian and lung cancer. Asian Pac J Cancer Prev (2014) 15(16):6581–6. doi: 10.7314/apjcp.2014.15.16.6581
62. Krijnen PA, Simsek S, Niessen HW. Apoptosis in diabetes. Apoptosis (2009) 14(12):1387–8. doi: 10.1007/s10495-009-0419-6
63. Adlravan E, Nejati K, Karimi MA, Mousazadeh H, Abbasi A, Dadashpour M, et al. Potential activity of free and PLGA/PEG nanoencapsulated nasturtium officinale extract in inducing cytotoxicity and apoptosis in human lung carcinoma A549 cells. JoDDS (2021) 61:102256. doi: 10.1016/j.jddst.2020.102256
64. Demirtas L, Guclu A, Erdur FM, Akbas EM, Ozcicek A, Onk D, et al. Apoptosis, autophagy & endoplasmic reticulum stress in diabetes mellitus. Indian J Med Res (2016) 144(4):515. doi: 10.4103/0971-5916.200887
65. Dadashpour M, Ganjibakhsh M, Mousazadeh H, Nejati K. Increased pro-apoptotic and anti-proliferative activities of simvastatin encapsulated PCL-PEG nanoparticles on human breast cancer adenocarcinoma cells. JoCS (2022), 1–12. doi: 10.1007/s10876-021-02217-y
66. Radogna F, Cristofanon S, Paternoster L, D’Alessio M, De Nicola M, Cerella C, et al. Melatonin antagonizes the intrinsic pathway of apoptosis via mitochondrial targeting of bcl-2. J Pineal Res (2008) 44(3):316–25. doi: 10.1111/j.1600-079X.2007.00532.x
67. Fernández A, Ordóñez R, Reiter RJ, González-Gallego J, Mauriz JL. Melatonin and endoplasmic reticulum stress: relation to autophagy and apoptosis. J Pineal Res (2015) 59(3):292–307. doi: 10.1111/jpi.12264
68. Herr I, Debatin K-M. Cellular stress response and apoptosis in cancer therapy. Blood J Am Soc Hematol (2001) 98(9):2603–14. doi: 10.1182/blood.V98.9.2603
69. Cunard R, Sharma K. The endoplasmic reticulum stress response and diabetic kidney disease. Am J physiology-renal Physiol (2011) 300(5):F1054–F61. doi: 10.1152/ajprenal.00021.2011
70. Szegezdi E, Logue SE, Gorman AM, Samali A. Mediators of endoplasmic reticulum stress-induced apoptosis. EMBO Rep (2006) 7(9):880–5. doi: 10.1038/sj.embor.7400779
71. Tabas I, Ron D. Integrating the mechanisms of apoptosis induced by endoplasmic reticulum stress. Nat Cell Biol (2011) 13(3):184–90. doi: 10.1038/ncb0311-184
72. Li Y, Guo Y, Tang J, Jiang J, Chen Z. New insights into the roles of CHOP-induced apoptosis in ER stress. Acta Biochim Biophys Sin (2014) 46(8):629–40. doi: 10.1093/abbs/gmu048
73. Morishima N, Nakanishi K, Takenouchi H, Shibata T, Yasuhiko Y. An endoplasmic reticulum stress-specific caspase cascade in apoptosis: cytochrome c-independent activation of caspase-9 by caspase-12. J Biol Chem (2002) 277(37):34287–94. doi: 10.1074/jbc.M204973200
74. Salek-Maghsoudi A, Hassani S, Momtaz S, Shadboorestan A, Ganjali MR, Ghahremani MH, et al. Biochemical and molecular evidence on the role of vaspin in early detection of the insulin resistance in a rat model of high-fat diet and use of diazinon. Toxicology (2019) 411:1–14. doi: 10.1016/j.tox.2018.10.014
75. Mozaffar M, Shahrbaf MA, Azimi B, Arabzadeh A. Management of celiac trunk and superior mesenteric artery synchronous aneurysms as an extremely rare manifestation of wegener granulomatosis. J Vasc Surg cases innovative techniques (2019) 5(4):525–8. doi: 10.1016/j.jvscit.2019.05.005
76. Nna VU, Abu Bakar AB, Ahmad A, Eleazu CO, Mohamed M. Oxidative stress, NF-κb-mediated inflammation and apoptosis in the testes of streptozotocin–induced diabetic rats: Combined protective effects of malaysian propolis and metformin. Antioxidants (2019) 8(10):465. doi: 10.3390/antiox8100465
77. Shojaeii S, Dehghani Firoozabadi A, Rasouli MB, Mahdavi Shahri N, Alireza H. Morphological evaluation of testis tissue of rats in various time points after diabetes type 1 induction. Iranian J Diabetes Obes (2013) 5(3):98–106.
78. Reiter RJ, Calvo JR, Karbownik M, Qi W, Tan DX. Melatonin and its relation to the immune system and inflammation. Ann New York Acad Sci (2000) 917(1):376–86. doi: 10.1111/j.1749-6632.2000.tb05402.x
79. Ramaswamy S, Weinbauer GF. Endocrine control of spermatogenesis: Role of FSH and LH/testosterone. Spermatogenesis (2014) 4(2):e996025. doi: 10.1080/21565562.2014.996025
80. Huhtaniemi I. A short evolutionary history of FSH-stimulated spermatogenesis. Hormones (2015) 14(4):468–78. doi: 10.14310/horm.2002.1632
81. Chen S-R, Liu Y-X. Regulation of spermatogonial stem cell self-renewal and spermatocyte meiosis by sertoli cell signaling. Reproduction (2015) 149(4):R159–67. doi: 10.1530/REP-14-0481
82. Emanuele MA, Emanuele N. Alcohol and the male reproductive system. Alcohol Res Health (2001) 25(4):282.
83. Yu K, Deng S-L, Sun T-C, Li Y-Y, Liu Y-X. Melatonin regulates the synthesis of steroid hormones on male reproduction: a review. Molecules (2018) 23(2):447. doi: 10.3390/molecules23020447
84. Srivastava RK, Krishna A. Melatonin affects steroidogenesis and delayed ovulation during winter in vespertilionid bat, scotophilus heathi. J Steroid Biochem Mol Biol (2010) 118(1-2):107–16. doi: 10.1016/j.jsbmb.2009.11.001
85. Revel FG, Masson-Pévet M, Pévet P, Mikkelsen JD, Simonneaux V. Melatonin controls seasonal breeding by a network of hypothalamic targets. Neuroendocrinology (2009) 90(1):1–14. doi: 10.1159/000219588
86. Rocha CS, Martins AD, Rato L, Silva BM, Oliveira PF, Alves MG. Melatonin alters the glycolytic profile of sertoli cells: implications for male fertility. Mol Hum Reprod (2014) 20(11):1067–76. doi: 10.1093/molehr/gau080
87. O’Shaughnessy PJ, Johnston H, Willerton L, Baker PJ. Failure of normal adult leydig cell development in androgen-receptor-deficient mice. J Cell Sci (2002) 115(17):3491–6. doi: 10.1242/jcs.115.17.3491
88. Basha B, Samuel SM, Triggle CR, Ding H. Endothelial dysfunction in diabetes mellitus: possible involvement of endoplasmic reticulum stress? Exp Diabetes Res (2012) 2012:481840. doi: 10.1155/2012/481840
89. Maresch CC, Stute DC, Alves MG, Oliveira PF, de Kretser DM, Linn T. Diabetes-induced hyperglycemia impairs male reproductive function: a systematic review. Hum Reprod Update (2018) 24(1):86–105. doi: 10.1093/humupd/dmx033
Keywords: diabetes mellitus, hyperglycemia, melatonin, oxidative stress, genitalia
Citation: Armandeh M, Bameri B, Haghi-Aminjan H, Foroumadi R, Ataei M, Hassani S, Samadi M, Shayesteh MRH and Abdollahi M (2022) A systematic review on the role of melatonin and its mechanisms on diabetes-related reproductive impairment in non-clinical studies. Front. Endocrinol. 13:1022989. doi: 10.3389/fendo.2022.1022989
Received: 19 August 2022; Accepted: 23 September 2022;
Published: 11 October 2022.
Edited by:
Faisal Raza, Shanghai Jiao Tong University, ChinaReviewed by:
Amjad Hayat Khan, University of Science Malaysia, MalaysiaCopyright © 2022 Armandeh, Bameri, Haghi-Aminjan, Foroumadi, Ataei, Hassani, Samadi, Shayesteh and Abdollahi. This is an open-access article distributed under the terms of the Creative Commons Attribution License (CC BY). The use, distribution or reproduction in other forums is permitted, provided the original author(s) and the copyright owner(s) are credited and that the original publication in this journal is cited, in accordance with accepted academic practice. No use, distribution or reproduction is permitted which does not comply with these terms.
*Correspondence: Hamed Haghi-Aminjan, SGFtZWRoYWdoaS5hQGdtYWlsLmNvbQ==; Mohammad Abdollahi, TW9oYW1tYWRAVFVNUy5BYy5Jcg==; TW9oYW1tYWQuQWJkb2xsYWhpQFVUb3JvbnRvLkNh
†These authors have contributed equally to this work
‡ORCID: Mohammad Abdollahi, orcid.org/0000-0003-0123-1209
Disclaimer: All claims expressed in this article are solely those of the authors and do not necessarily represent those of their affiliated organizations, or those of the publisher, the editors and the reviewers. Any product that may be evaluated in this article or claim that may be made by its manufacturer is not guaranteed or endorsed by the publisher.
Research integrity at Frontiers
Learn more about the work of our research integrity team to safeguard the quality of each article we publish.