- 1Department of General Surgery, Shandong Provincial Qianfoshan Hospital, Cheeloo College of Medicine, Shandong University, Jinan, China
- 2Medical Research Center, Shandong Provincial Qianfoshan Hospital, Cheeloo College of Medicine, Shandong University, Jinan, China
- 3Department of General Surgery, The First Affiliated Hospital of Shandong First Medical University, Jinan, Shandong, China
- 4Department of Breast Disease, Peking University People’s Hospital, Beijing, China
Objective: To investigate the effects of sleeve gastrectomy (SG) on diabetes-related cognitive decline (DCD) in rats with diabetic mellitus (DM).
Methods and methods: Forty Wistar rats were randomly divided into control (CON) group (n=10), diabetes mellitus (DM) group (n=10), sham operation (SHAM) group (n=10) and SG group (n=10). DM model was established by high-fat diet (HFD) combined with intraperitoneal injection of streptozocin (STZ). Behavioral evaluation was given using Morris water maze test and Y-maze. In addition, PET-CT, TUNEL assay, histological analysis, transmission electron microscopy (TEM), immunohistochemistry (IHC) and Western blot analysis were used to evaluate the alleviating effects and potential mechanisms of SG on DCD in DM rats.
Results: Compared with the sham group, SG induced significant improvement in the metabolic indices such as blood glucose and body weight. Besides, it could attenuate the insulin resistance compared with SHAM group. In addition, SG could improve the cognitive function of DM rats, which were featured by significant decrease in the escape latency (P<0.05), and significant increase in the time in target quadrant and platform crossings (P<0.05) compared with the SHAM group. SG induced significant elevation in the spontaneous alternation compared with SHAM group (P<0.05). Moreover, SG could improve the arrangement and biosynthesis of hippocampus neuron. Moreover, SG triggered the inhibition of apoptosis of hippocampus neurons, and Western blot analysis showed SG induced significant increase in the ratios of Bcl-2/Bax and Caspase3/cleaved Caspase 3. TEM demonstrated SG could significantly improve the microstructure of hippocampus neurons compared with the SHAM group. Western blot and IHC confirmed the significant decrease in the phosphorylation of tau at Ser404 and Ser396 sites in the SG group. Furthermore, SG activated the PI3K signaling pathway by elevating the phosphorylation of PI3K and Akt and GSK3β compared with the SHAM group.
Conclusion: SG attenuated the DCD in DM rats, which may be related to the activation of PI3K signaling pathway.
Introduction
Diabetes mellitus (DM), with a sharp increase in the prevalence in the last three decades, is considered the ninth leading cause of death worldwide (1). Patients with DM usually show involvement of the nervous system, resulting in cognitive decline (2, 3). As a major complication of DM, the diabetes-related cognitive decline (DCD) is characterized by abnormalities in learning, memory, attention and speed of information processing (4). To date, impairment of hippocampus has been closely associated with the learning and memory loss, while in animals with DCD, the main pathological features of the hippocampus are neurogenic fibrillary tangles due to tau hyperphosphorylation and neuronal apoptosis (5).
Bariatric surgery, initially utilized for treating morbid obesity, is later reported to show a moderate effect on DM and its complications (6, 7). Bariatric surgery can ameliorate hyperglycemia (8) and reduce the body weight (9). In addition, patients underwent such type of surgery showed significant improvement in the cognitive function (10).
PI3K/Akt signaling pathway plays a key role in the pathogenesis of DCD (11, 12), which mediates biological growth and crucial cellular metabolic processes, such as glucose homeostasis, lipid metabolism, protein synthesis and cell proliferation and survival (13). In diabetic rats or glucose-induced hippocampal neuronal impairments, there was decrease in the phosphorylation of AKT, resulting in a decrease in the phosphorylation level of glycogen synthase kinase 3β (GSK3β) (14, 15), serving as a key enzyme that inhibits glycogen synthesis and one of the key kinases for tau phosphorylation (16). Physiologically, activation of the PI3K insulin signaling pathway could inhibit the hippocampal neuronal apoptosis. In addition, it could ensure that tau phosphorylation is maintained at a normal level by inactivating GSK3β (17).
Sleeve gastrectomy (SG) serving as one of the most popular bariatric surgeries for obesity has been reported to improve the cognitive function among the obesity patients (18, 19). However, little is known about the exact mechanisms for this process. In this study, a DM rat model was established in order to investigate how SG improved the cognitive function, and at the same time, we determined the activity of PI3K/AKT signaling pathway, with an aim to illustrate its roles in this process.
Materials and methods
Animals
Forty male Wistar rats (90–110g; 6-week-old), purchased from Vital River Laboratory Animal Technology (Beijing, China), were housed in the animal laboratory of Shandong Provincial Qianfoshan Hospital of Shandong University, under specific pathogen-free housing conditions at 20–26°C in a humidity of 50–60%. All animals were fed on a standard diet containing 15% of fat for 1 week for acclimatization. This animal study was approved by the Institutional Animal Care and Use Committee of Shandong Qianfoshan Hospital, Shandong University.
Grouping
The animals were randomly divided into the following four groups: (i) control (CON) group (n=10), rats subject to a standard diet; (ii) DM group (n=10), rats subject to DM induction; (iii) sham operation (SHAM) group (n = 10), and (iv) SG group (n=10). One week before the surgical intervention in the SG and SHAM groups, Y-maze test was performed to confirm the differences in cognitive ability among the groups (Figure S1). Animals in the SG group were subject to SG after a 12 hrs fast. Animals in the SHAM group were subject to DM induction, followed by operations that were the same as the above surgery before occlusion of gastric blood vessels. There were no interventions in the SHAM group except exposure of abdominal organs such as the stomach, small intestine, and liver. The flowchart of the study was shown in Figure 1.
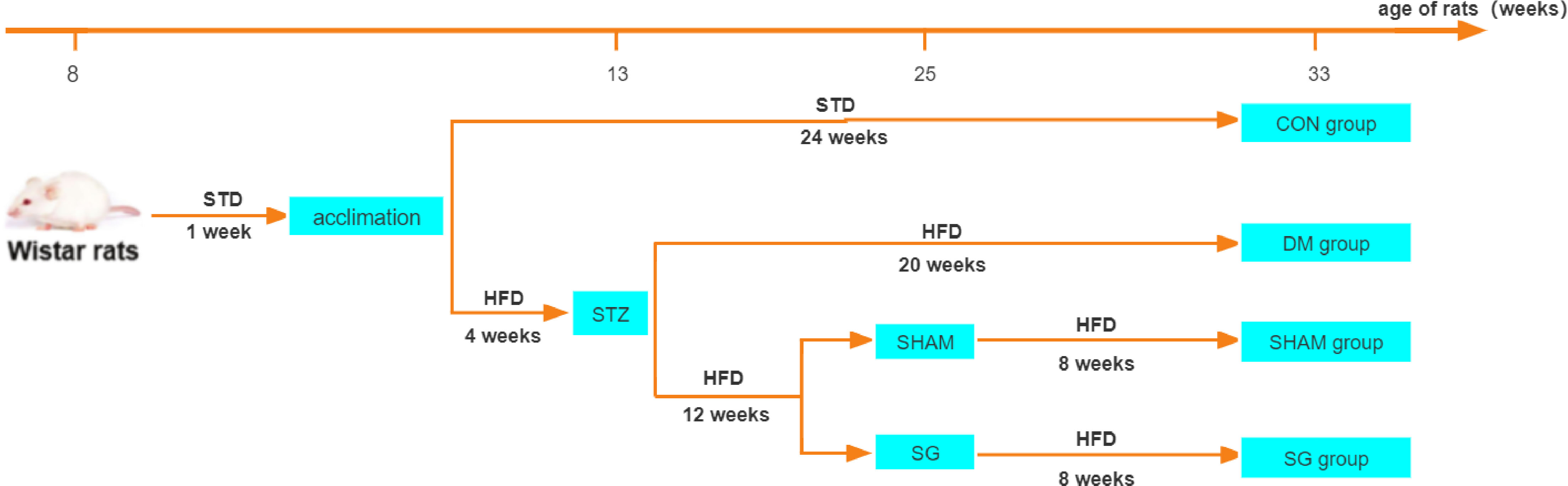
Figure 1 Flowchart of animal experiments. N = 10 for each group. STD, standard diet; HFD, high-fat diet; STZ, streptozotocin; CON, control group; DM, diabetes mellitus; SHAM, sham operation; SG, sleeve gastrectomy.
DM induction
Animals were given a 4-week high fiber diet (HFD, 40% fat; Xietong Pharmaceutical Bio-engineering, Nanjing, China), followed by a 12 hrs fast. Then the rats received a single intraperitoneal injection of streptozocin (STZ, 35 mg/kg, Sigma-Aldrich, St. Louis, MO, US). Three days after STZ injection, random blood glucose was measured 3 times consecutively, and a glucose level of ≥ 16.7 mmol/L was considered a successful model (20).
SG procedures
The procedures of SG were performed as previously reported (21). All rats were fasted for 12 hrs before surgery and then anesthetized by continuous inhalation of 2% isoflurane gas using a mask. First, a 4 cm incision was made in the middle of the upper abdomen. Then, the stomach was separated until a clear distinguish of the gastric blood vessels. The blood vessels of the fundus and the greater curvature of the stomach were ligated with a 7-0 thread (Chenghe Microsurgical Instruments, Ningbo, China), and then cut off after ligation. Subsequently, an incision of about 0.5 cm parallel to the greater curvature of the stomach was made in the fundus to remove the gastric contents. The fundus and most of the gastric body on the greater curvature of the stomach were removed, and the remaining part was sutured with 5-0 sutures (Cheng-He Microsurgical Instruments Factory, Ningbo, China). No active bleeding was confirmed and the anatomical position of the abdominal organs was restored. Finally, close the abdominal wall layer by layer with 3-0 sutures (Chenghe Microsurgical Instruments, Ningbo, China).
Body weight and food intake measurement
For the animals in each group, we measured the baseline body weight and food intake. Then the body weight and food intake were measured at 2, 4, 6, and 8 weeks afterwards.
Homeostasis model assessment of insulin resistance
Upon fasting for 12 hrs, the blood samples were collected from the tail vein after anesthesia, and centrifuged at 3,000 rpm for 8 min. Fasting blood glucose (FBG) was measured using a blood glucose meter (One Touch Ultra, Johnson & Johnson, CA, USA). Then serum insulin was detected with EZRMI-13K kit (One Touch Ultra, Johnson & Johnson, CA, USA). Finally, the Homeostasis Model Assessment of Insulin Resistance (HOMA-IR) was calculated to assess the degree of insulin resistance, using the following formula: HOMA-IR=fasting serum insulin (mIU/L)×FBG (mmol/L)/22.5 (22).
Oral glucose tolerance test and insulin tolerance Test
For the OGTT, glucose (1 g/kg) was given to each rat via intragastrical administration. Then the blood glucose was measured at 0, 10, 30, 60, and 120 min, respectively. About 24 hrs after OGTT, ITT was performed after a 12-hour fast. The rats were intraperitoneally injected with insulin (0.5 IU/kg, Tonghua Dongbao Pharmacy. Gansu, China), and then the blood glucose was measured at 0, 10, 30, 60, and 120 min, respectively. Finally, the area under the curve (AUC) of OGTT and ITT was calculated with the trapezoidal method according to the previous description (23, 24).
Morris water maze test
MWM test was used to measure hippocampus-dependent cognitive function as previously described (25). All behavioral tests were performed during the active period of the photoperiod. A video analysis system (Calvin Biotech, Nanjing, China) was utilized to record the swimming patterns of each rat. The pool was filled with water at a constant temperature of 20°C. An escape platform with a diameter of 4.5 cm was placed in the swimming pool. The top of the platform was approximately 1.5 cm below the water surface.
The 5-day hidden platform test was used to detect the learning ability of rats. Rats were trained for 5 days. Animals failed to find the platform within 120 sec were placed on the platform for 10 sec. Spatial memory for each rat was determined based on the probe trial. Hidden platforms in the target quadrant were removed after training for 5 days. On day 6, a probe trial was performed and the rats were allowed to swim freely in the pool for 2 min.
Y-maze test
Spatial memory status in rats was tested by measuring the percentage of alternation in Y-maze test according to the previous description (26). The test device consisted of three equal-length arms (50×18×35 cm) in a Y-shape and an intermediate region. Rats were placed at the end of either arm, and were allowed to explore freely for 8 min. Subsequently, the total number and sequence of entries into each arm were recorded. The percentage of alternations was determined based on the following equation: (spontaneous alternations)/(total number of arm entries-2).
Positron-emission tomography and image processing
Before euthanasia, rats fasted for 12 h were maintained under anesthesia with 1.5% isoflurane for PET. After intravenous injection of 18F-FDG (800 μCi, 29.6 MBq), the entire body of the rat was continuously scanned for 21 min with a PET scanner (Metis 1800, Madic Technology, Linyi, China) in the coronal, sagittal and transverse dimensions, followed by a focused scan of the brain for 15 min, especially the hippocampus. The brain glucose uptake was analyzed by measuring the mean standard uptake values (SUVMean) using PMOD 4.1 software (PMOD Technology, Zurich, Switzerland).
Histological analysis
Hippocampal tissues were fixed with 4% paraformaldehyde and embedded in paraffin. Paraffin sections (5 μm) were stained with hematoxylin-eosin to evaluate the structure of four vital sub-regions of the hippocampus. After dewaxing, the sections were stained with hematoxylin staining solution (G1004, ServiceBio, Wuhan, China) for 5 min, and then stained with eosin staining solution (G1001, Wuhan, China, ServiceBio, Wuhan, China) for 5 min. In addition, Nissl staining was performed on the sections to evaluate the neuronal damage. The samples were processed in the same way as H&E staining, then stained with Nissl’s staining solution (G1036, ServiceBio, Wuhan, China) for 5 min, and finally sealed with neutral resin. Digital slides were prepared by a Pannorama digital slide scanner (Pannoramic DESK, P-MIDI, P250, and P1000, 3DHISTECH, Budapest, Hungary).
TUNEL assay
Apoptosis in the hippocampus tissues was detected by commercial TUNEL Apoptosis kit (C1086, Beyotime Biotechnology, Shanghai, China), according to the manufacturer’s instructions. The positive cells in each group were counted under a microscope. Apoptotic cells were stained in green color.
Transmission electron microscopy
Tissue squares (1 mm×1 mm×1 mm) were fixed with electron microscopy fixative (G1102, ServiceBio, Wuhan, China) for 2-4 hrs at 4°C, and then were post-treated in 1% osmium tetroxide for 2 hrs at 4°C. Subsequently, the samples were dehydrated through an ethanol series and infiltrated using acetone and 812 embedding medium (905529-77-4, SPI). After complete polymerization, the sections were observed under the TEM (Hitachi, HT-7700, Japan).
Immunohistochemistry
Paraffin sections (5 μm) were deparaffinized, and washed 3 times with PBS (G0002-2L, Servicebio, Wuhan, China). The antigens were retrieved in a microwave oven with citrate buffered saline (C1032, Solarbio, Beijing, China). Sections were incubated overnight with primary antibodies including p-tau (Ser404) (1:200, ab92676, Abcam, Cambridge, USA) and p-tau (Ser396) (1:4000, ab109390, Abcam, Cambridge, USA), followed by washing three times with PBS. Sections were then incubated with a universal two-step detection kit (PV-9000, ZSGB-BIO, Beijing, China) following the manufacturer’s instructions. After washing 3 times with PBS, the sections were stained with diaminobenzidine (DAB, ZLI-9017, ZSGB-BIO, Beijing, China) and hematoxylin. Finally, the sealed sections were made into digital slides by a panoramic digital slide scanner (Panorama Desk, P-MIDI, P250 and P1000, 3DHISTECH).
Western blot analysis
Hippocampal tissues were homogenized in RIPA cold buffer (89901; Thermofisher, USA) containing protease inhibitor (ST506, Beyotime Biotech, Shanghai, China). Protein samples were quantified using the BCA protein assay kit (E-BC-K318-M, Elabscience, Wuhan, China). Protein samples (50 μg) were separated by SDS-PAGE gel (PG212, EpiZyme, Shanghai, China) and then transferred to a polyvinylidene fluoride (PVDF) membrane. PVDF membranes were blocked with 5% nonfat dry milk for 1 h, and incubated with primary antibody overnight at 4°C [p-tau (Ser404), 1:2000, ab92676, Abcam, USA; p-tau(Ser396), 1:50000, ab109390, Abcam, USA; Tau, 1:10000, sc-32274, Santa Cruz Biotechnology, Beijing; p-PI3K, 1:1000, 13857S, Cell Signaling Technology, USA; PI3K, 1:1000, 3358S, Cell Signaling Technology, USA; Akt, 1: 1000, 4685S, Cell Signaling Technology, USA; p-Akt, 1:2000, 4060S, Cell Signaling Technology; GSK3β, 1:1000, 9315S, Cell Signaling Technology, USA; p-GSK3β, 1:1000, 9315S, Cell Signaling Technology, USA; Bcl-2, 1:5000, 60178-1-Ig, Proteintech, China; Bax, 1:10000, 50599-1-Ig, Proteintech, China; Caspase 3, 1:1000, 9662S, Cell Signaling Technology, USA; cleaved Caspase 3, 1:1000, 9664S, Cell Signaling Technology, USA; β actin, 1:20000, 66009-1-Ig, Proteintech, China]. Then, the membrane was washed and incubated with secondary antibodies (goat anti-mouse IgG, 1:10000, ab216776, Abcam; goat anti-rabbit IgG, 1:10000, ab6721, Abcam). Protein bands were visualized by ECL (Millipore) and quantified using ImageJ software (National Institutes of Health).
Statistical analysis
Data were analyzed using Graph Pad Prism 8.0 (San Diego, CA, USA). Data were presented as mean ± standard error of mean. One-way ANOVA was utilized to compare the differences between groups, together with Tukey’s multiple comparison test. Statistical outliers were determined using the Grubbs test. P <0.05 was considered to be statistically significant.
Results
SG improved basic metabolic parameters in diabetic rats
The body weight and food intake were significantly higher in the DM group than these of the CON group. Compared with the DM group, significant decrease was noticed in the body weight and food intake of rats in SG group (Figures 2A, B). In addition, compared with the SHAM group, the FBG showed significant decrease in the SG group within 2 weeks after surgery (Figure 2C). By recording FBG and serum insulin levels for HOMA-IR assessment, we found a significant decrease in insulin resistance in the SG group compared to the sham group (Figures 2C-2E). Consistently, AUCOGTT and AUCITT further validated the improvement in insulin resistance in the SG group (Figures 2F, G). All these indicated that SG could significantly improve the basic metabolic parameters in diabetic obese rats.
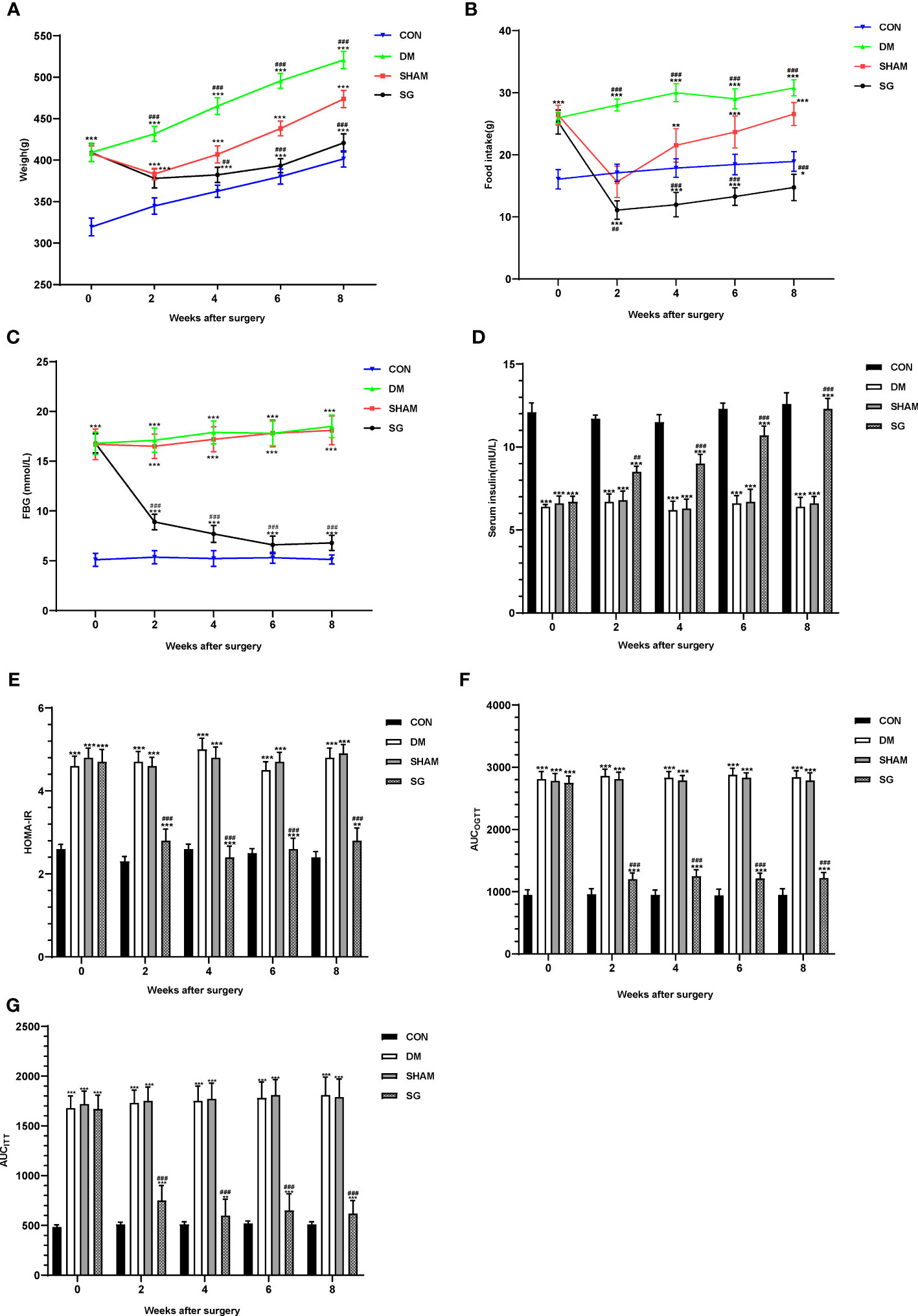
Figure 2 Changes of metabolic parameters including body weight (A), food intake (B), FBG (C), serum insulin (D), HOMA-IR (E), AUCOGTT (F), and AUCITT (G) before and after surgery. Data were expressed as means ± SEM for n = 10 per group. **p < 0.01 vs. CON group, ***p < 0.001 vs. CON group; ##p < 0.01 vs. SHAM group, ###p < 0.001 vs. SHAM group. FBG, fasting blood glucose; HOMA-IR, homeostasis model assessment of insulin resistance; AUCOGTT, the area under the curve of the oral glucose tolerance test; AUCITT, the area under the curve of the insulin tolerance test; CON, control; DM, diabetes mellitus; SHAM, sham operation; SG, sleeve gastrectomy.
SG ameliorated cognitive function in diabetic rats
The escape latency of the rats in the DM group and the SHAM group was significantly longer than that of the control (Figure 3A). The escape latency in the SG group was significantly shorter than that of the DM group (Figure 3A). Meanwhile, there was no statistical difference in the swimming speed of the rats in each group (Figure 3B). The travelled distance of rats in each group showed gradual decrease in a time-dependent manner. The travelled distance in the SG group was significantly shorter than the SHAM group on day 4 and 5 (Figure 3C). Compared with normal rats, the percentage of time in target quadrant (Figure 3D) and the number of platform crossing (Figure 3E) were significantly shortened in the DM and SHAM groups. In contrast, the percentage of time and number of platform crossings in SG group showed significant increase compared with the SHAM group (Figures 3D, E). These indicated that SG significantly improved spatial memory and learning ability in diabetic rats. In addition, the Y-maze test showed that the percentage of spontaneous alternation in the DM group was lower than that in the control group, while the percentage of spontaneous alternation in the SG group was significantly different compared to the SHAM group (Figure 3F), with no significant changes in total arm entries (Figure 3G). This suggested that SG may improve the ability of diabetic rats to recognize novel environment, and could partially improve the DCD.
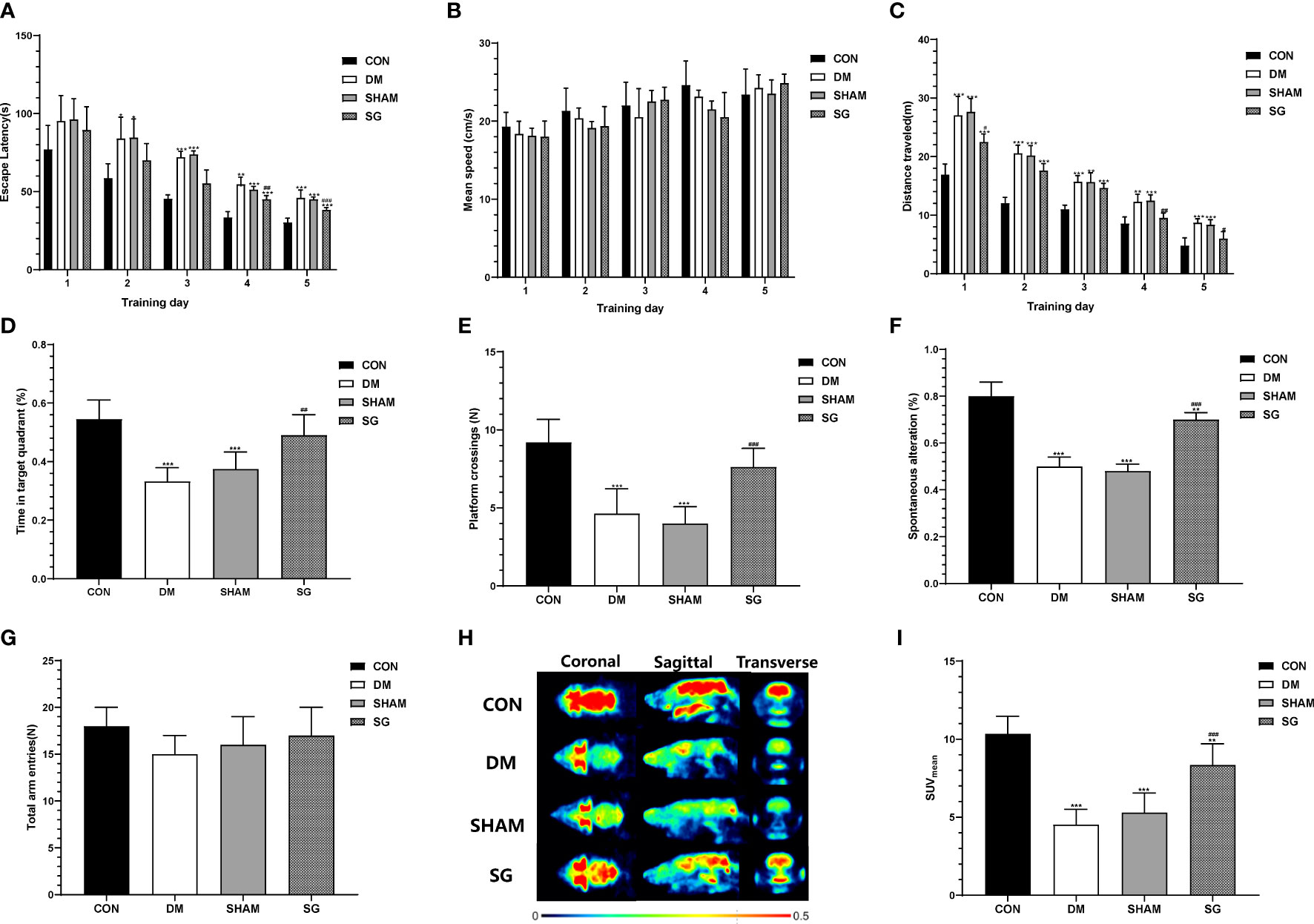
Figure 3 SG induced significant improvement in the animal behaviors in the Morris water maze test (A-E) and Y-maze test (F, G), and PET imaging system validated the improvement of glucose uptake in brain (H, I). Data were expressed as means ± SEM for n = 10 per group. *p < 0.05 vs. CON group, **p < 0.01 vs. CON group, ***p < 0.001 vs. CON group; #p < 0.05 vs. SHAM group, ##p < 0.01 vs. SHAM group, ###p < 0.001 vs. SHAM group. PET, positron-emission tomography; SUVMean, the average standard uptake value; CON, control; DM, diabetes mellitus; SHAM, sham operation; SG, sleeve gastrectomy.
SG significantly improved cerebral glucose uptake in diabetic rats
We evaluated glucose uptake in brain tissues using a PET scanner (Figure 3H). The SUVmean of the control group and SG group was significantly higher than that of the DM group and sham group, respectively (Figure 3I). These results suggested that SG improved the diabetes-induced obstruction of cerebral glucose uptake.
SG significantly reversed hippocampal histopathology in diabetic rats
Changes in the hippocampus tissue underlie cognitive decline as central nervous system diseases and diabetes progress (27, 28). Compared with the CON group, the number of neurons in the hippocampal cornu ammonis (CA)1, CA2, CA3, and dentate gyrus (DG) regions of the DM group showed significant decrease, which were featured by presence of swollen cells, nuclear fragmented or disappearance, and irregular arrangement of cell. Notably, SG significantly improved these changes (Figure 4A). To assess the damage of hippocampal neurons, the hippocampus of each group was analyzed using Nissl staining. DM resulted in the reduction of Nissl bodies in the CA1, CA3 and DG regions of the hippocampus, indicating significant neuronal damage. Whereas, the number of Nissl bodies in the SG group showed significant increase compared with SHAM group (Figure 4B). In conclusion, SG ameliorated the histological changes in the hippocampus induced by diabetes.
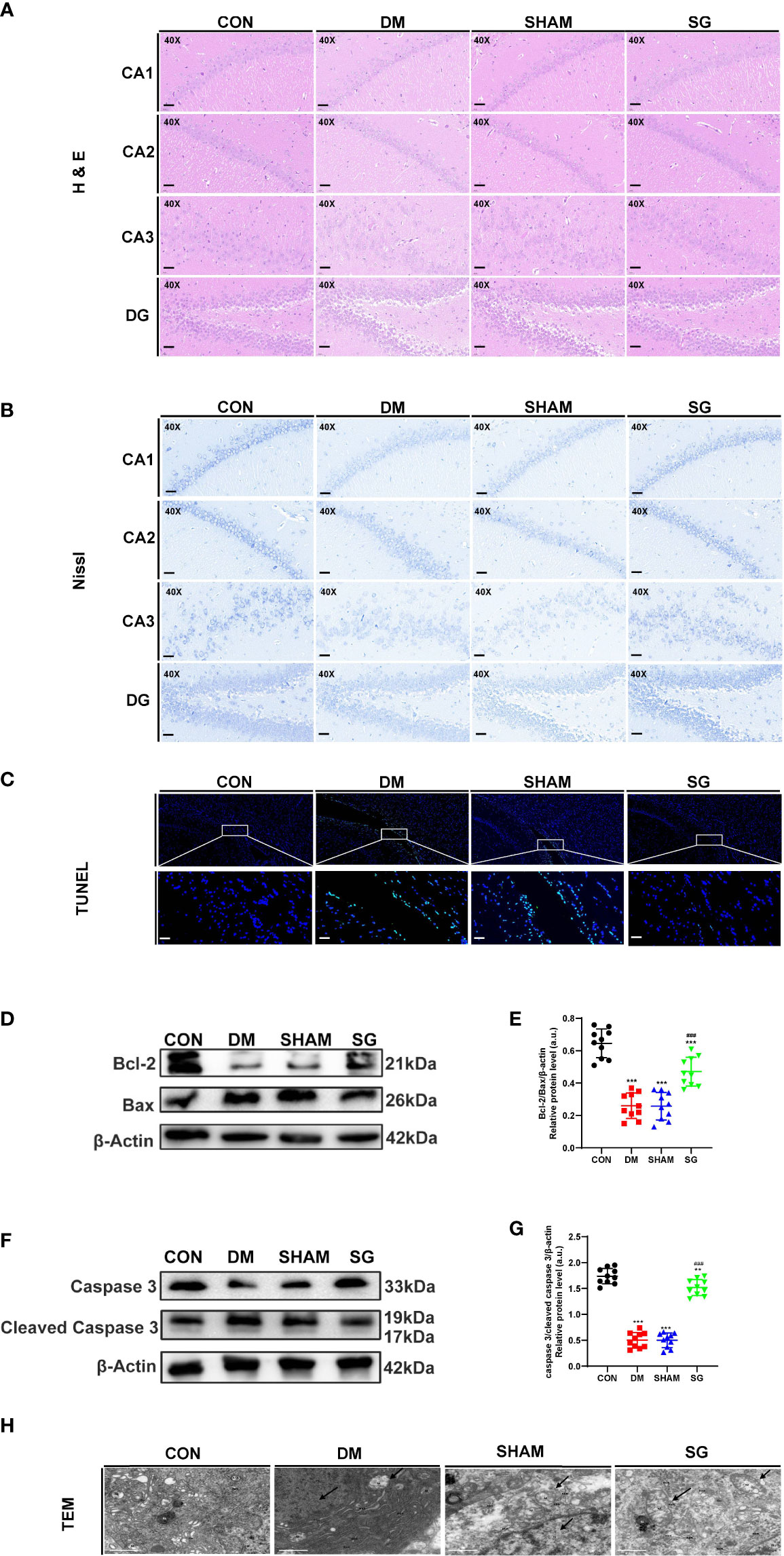
Figure 4 SG reversed the histological features (A), induce the increase of Nissl’s bodies (B), and inhibited the apoptosis of hippocampus neurons (C-G), and improved the microstructure of hippocampus neurons under TEM (H). The scale bar for A-C was 100 µm, while that for H was 1 µm. Data were expressed as means ± SEM for n = 10 per group. **p < 0.01 vs. CON group, ***p < 0.001 vs. CON group; ###p < 0.001 vs. SHAM group. H&E, hematoxylin and eosin; TUNEL, terminal deoxynucleotidyl transferase-mediated dUTP nick end labeling; CA1, cornu ammonis1; CA2, cornu ammonis2; CA3, cornu ammonis3; DG, dentate gyrus; M, mitochondrion; N, nucleus; RER, rough endoplasmic reticulum; GO, Golgi apparatus; CON, control; DM, diabetes mellitus; SHAM, sham operation; SG, sleeve gastrectomy.
SG alleviated hippocampal neuronal apoptosis in diabetic rats
In DM group, there was a significant increase in the number of positive neurons in the hippocampus, indicating significant increase in the apoptosis compared with the CON group. In contrast, SG alleviated the situation of neuronal apoptosis (Figure 4C). Western blot analysis showed that Bcl-2 and Caspase 3 were down-regulated in DM group and SHAM group compared with these of the CON group, while significant up-regulation was seen in the expressions of Bax and cleaved Caspase 3 in DM group (Figures 4D, F). The ratio of Bcl-2 to Bax and Caspase 3 to cleaved Caspase 3 in the SG group showed significant increase compared with that of SHAM group (Figures 4E, G). Taken together, we concluded that SG can ameliorate diabetes-induced apoptosis of hippocampal neurons.
SG significantly improved the fine structure of hippocampal neurons
TEM indicated pyknosis, severe edema, condensed cell matrix, obvious swelling of organelles in the hippocampal neurons in the DM group and SHAM group (Figure 4H), together with obvious vacuolar degeneration. In CON group and the SG group, the nuclear membrane was intact. In addition, the chromatin was uniform, and the cell membrane was intact. Moreover, the intracellular matrix was abundant and evenly distributed. It was worth noting that the mitochondria in the SG group were slightly swollen, and the cristae were fragmented and reduced, but not as severe as the SHAM group.
SG inhibited tau phosphorylation in the hippocampus of diabetic rats
There was increased phosphorylation of tau at Ser404 and Ser396 in the hippocampus of DM and SHAM group compared with CON group (Figure 5A). In contrast, SG decreased the phosphorylation of these two tau sites (Figures 5B, C), which was verified by IHC results (Figure 5D). Thus, SG alleviated hyperphosphorylation of tau in the hippocampus of diabetic rats.
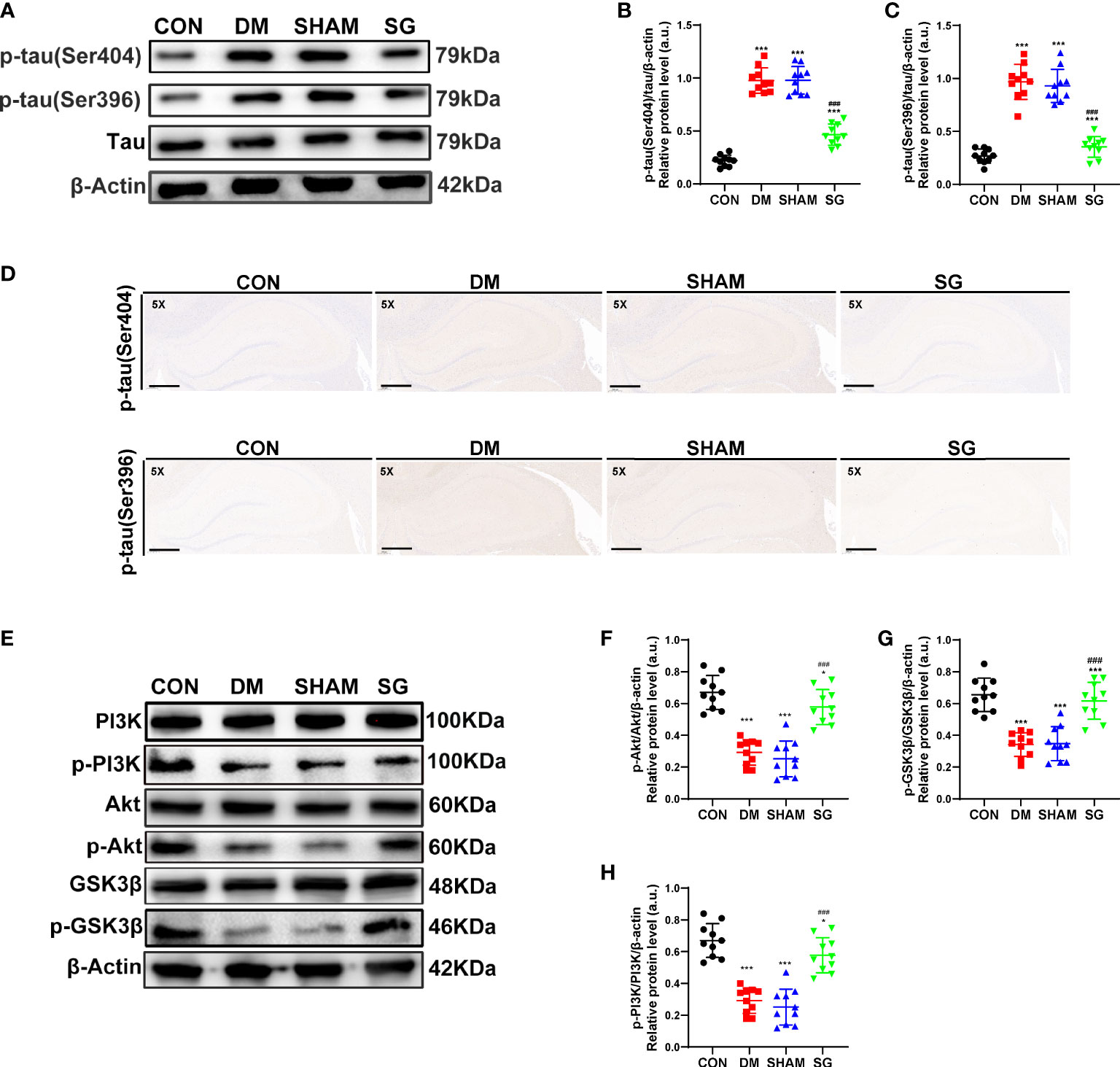
Figure 5 SG inhibited the phosphorylation of tau (A-D) and activated the PI3K signaling pathway (E-H). The scale bar for D was 800 µm. Data were expressed as means ± SEM for each group (n = 10). *p < 0.05 vs. CON group, ***p < 0.001 vs. CON group; ###p < 0.001 vs. SHAM group. CON, control; DM, diabetes mellitus; SHAM, sham operation; SG, sleeve gastrectomy.
Effects of SG on PI3K signaling pathways
Compared with the CON group, the hippocampal expression of p-PI3K, p-Akt, and p-GSK3β showed significant decrease in both DM and SHAM groups, while SG induced significant increase in their expression (Figure 5E). The ratios of p-PI3K to total PI3K, p-Akt to total Akt and p-GSK3β to total GSK3β were consistent with the above results (Figures 5F-5H). In conclusion, we infer that SG partially ameliorated diabetes-induced cognitive impairment, which was associated with activation of the PI3K signaling pathway.
Discussion
DM and its complications were indeed a serious threat to global health. On this basis, there has been widespread interests in the treatment of diabetes (1). DCD has been more and more widely recognized as a serious complication for DM (29). Slowly progressive DCD occurs in all age groups, not limited to the aged population (30). The main pathological features of DCD include hyperphosphorylation of tau and apoptosis of hippocampal neurons, leading to progressive impairment of hippocampal function (31–33). A large number of DCD patients are more likely to present mild cognitive impairment (MCI) or dementia, showing a poor prognosis even after treatment (33, 34). Conventional treatment options for DCD include measurements for lowering blood glucose, lifestyle interventions, and cognitive rehabilitation training. In addition, delivery of insulin to targeted brain tissues has been proposed as a potential strategy for treating cognitive impairment in DM patients (35). Unfortunately, this strategy is not universally effective due to poor adherence and individual variability (36). Therefore, it is urgently to develop more effective treatment options for these patients.
In the 1990s, bariatric surgery began to be recognized as a form of inducing weight loss, which may improve the symptoms of DM and its complications (37). Recently, it has gradually considered as the best treatment strategy for DM and obesity, with the advances in the surgical safety (38). In terms of animal models, due to the similarity of metabolic characteristics, the most commonly used diabetes model is the DM rodent model, which is used to investigate the pathogenesis and treatment of DM (39). In this study, such model was used to investigate the therapeutic effects of SG on DCD and its associated mechanisms. According to the previous studies, cognitive decline was sufficiently induced in this model about 8-9 weeks after induction of DM (40, 41). Our data showed that the cognitive function of rats was significantly impaired at week 12 after DM. Meanwhile, SG showed significant effects on reducing body weight, together with improving hyperglycemia and reversing insulin resistance. However, some rats showed signs of rebound in body weight and blood sugar after surgery, which we consider to be caused by maintaining a high-fat diet during the experiments. The CA1, CA3 and DG regions of the hippocampus were crucial for learning and spatial memory, and there was a unidirectional tri-synaptic pathway between these regions (42). In this study, we found that cognitive function decreased. HE and Nissl staining revealed neuronal damage in the CA1, CA3, and DG regions in DM rats. Interestingly, these negative effects were improved to varying degrees after SG. The above notion was further supported by the changes in neuronal microstructure under TEM. Taken together, SG could improve the symptoms in diabetic rats with DCD.
PET, employing molecules labeled with positron-emitting radioisotopes to provide direct and specific measurements of biochemical processes in regions of interest, has been used to gain a deeper understanding on the neural mechanisms underlying behavioral and cognitive processes (43). In cognition-related regions, there is an association between insulin resistance and reduced brain glucose metabolism (44). Therefore, PET scans of rat brains were performed and SUV was utilized to assess glucose uptake in brain, particularly the hippocampus. PET scan showed significant increase in the SUV in SG group, but it did not reach the level of the CON group. And confirmed that SG significantly reversed cerebral glucose uptake in diabetic rats.
Pathological alternations of hippocampus are closely associated with the pathogenesis of DCD with the main pathological manifestations as hyperphosphorylation of tau and increased neuronal apoptosis (45). These pathological changes would trigger the inhibition of PI3K/Akt signaling pathway (46). Previous study indicated that many Tau sites can be phosphorylated and inactivated, including Ser404, Ser396, Ser202 and Thr205 (47). In our study, Western blot analysis and IHC showed that the expression of p-tau (Ser404) and p-tau (Ser396) showed significant up-regulation in the DM group and SHAM group compared with those of the CON group. According to the previous studies, DM could mediate the increased apoptosis of hippocampal neurons by inhibiting the PI3K pathway (48, 49). Consistently, our TUNEL assay indicated the increased expression of apoptosis-related proteins in hippocampus. Therefore, we concluded that SG could improve the pathological changes. Additionally, our data provided solid evidence for the neuroprotective effects of bariatric surgery by inhibiting neuronal apoptosis and tau phosphorylation.
PI3K signaling pathway plays crucial roles in several biological processes, such as glucose homeostasis, cell growth and proliferation (50). The activation of Akt and inactivation of GSK3β was highly depending on phosphorylation of corresponding serine residues, which functioned as serine/threonine kinases (51, 52). PI3K was activated by direct interaction with insulin receptor substrate 1 (IRS-1), and then the Akt was phosphorylated, which in turn induced the phosphorylation of GSK3β and ultimately promoted the balance of blood glucose (53). It has been assumed that there is a potential link between PI3K signaling pathway and the pathogenesis of DM or Alzheimer’s disease (AD) as there is confirmed impairment of PI3K signaling pathway in the DM. Inhibition of glycogen synthesis and inactivation of tau have been reported to trigger hyperglycemia and cognitive decline, respectively (54). Meanwhile, increased ratio of GSK3β to p-GSK3β resulted in increased apoptosis of hippocampal neurons in DM rats (55). Notably, the expression of p-PI3K, p-Akt, and p-GSK3β was significantly up-regulated after SG, which suggested that the activation of PI3K signaling pathway may play an important role in the attenuation of DCD mediated by SG (Figure 6).
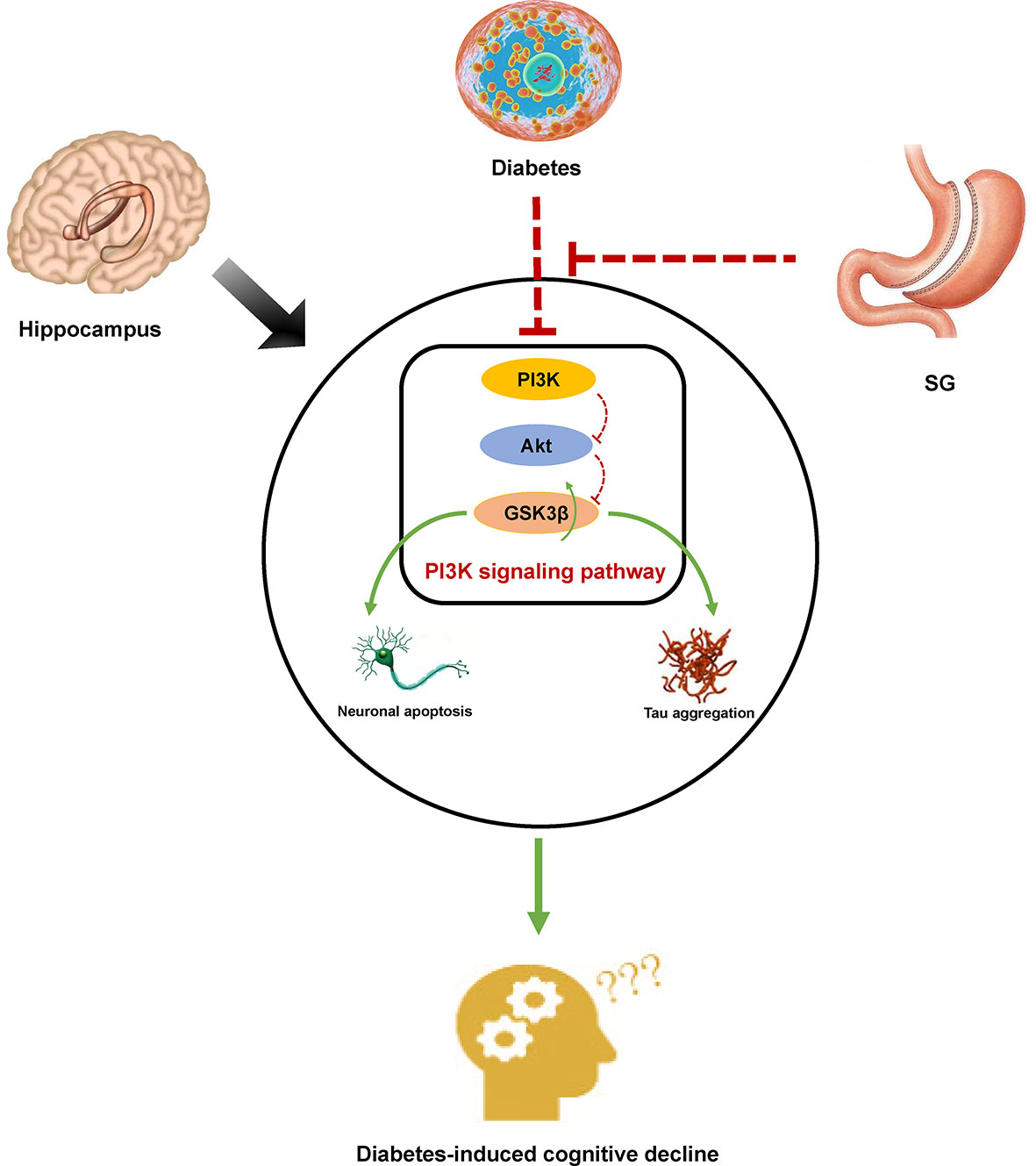
Figure 6 Diagram of a possible mechanism by which SG improved DCD. In DM rats, inhibition of PI3K signaling resulted in histological changes in the hippocampus by promoting tau phosphorylation and inducing apoptosis of hippocampal neurons. Activation of PI3K signaling pathway was associated with SG-induced amelioration of DCD.
Indeed, our study has some limitations. Some patients may present rebounding of blood glucose and weight even after bariatric surgery (56). It is still difficult to predict the state of diabetes-induced cognitive impairment following re-elevation of blood glucose and weight. Therefore, it is necessary to extend the observation time in the following studies to discuss the long-term effects of SG on diabetes-induced cognitive impairment. In addition, both glycemic control and weight loss have positive effects on metabolic status. Thus, it is not clear which is the crucial factor in the improvement of cognitive decline in SG-induced diabetes. On this basis, further studies are required to illustrate the exact mechanism of diabetes-induced cognitive impairment improvement. Finally, to further elucidate the mechanism by which SG attenuated the diabetes-induced cognitive impairment, we need to explore the interaction between GSK3β, Tau and neuronal apoptosis.
In conclusion, SG could reverse the tissue morphology of the hippocampus, decrease of glucose uptake in the hippocampus, and attenuate cognitive dysfunction induced by hippocampal neuronal apoptosis and hyperphosphorylation of Tau in DM rats. Additionally, the SG could reverse the inhibition of PI3K signaling pathway in rats with DCD. The reduction of diabetes-induced cognitive function by SG was associated with reactivation of the PI3K signaling pathway. In the future, inhibition of PI3K signaling may be a potential target for treating patients with DCD.
Data availability statement
The raw data supporting the conclusions of this article will be made available by the authors, without undue reservation.
Ethics statement
The animal study was reviewed and approved by Institutional Animal Care and Use Committee of Shandong Qianfoshan Hospital, Shandong University.
Author contributions
GZ and MZ contributed to conception and design of the study. Material preparation and data collection were performed by HD, CL, SZ, BL, QX and BS. Data analysis was performed by SL, SD, XM and YZ. HD, CL and SZ wrote the first draft of the manuscript. BL, QX, BS, SL, SD, XM and YZ wrote sections of the manuscript. GZ and MZ provided critical review of the article. All authors contributed to manuscript revision, read, and approved the submitted version.
Funding
This project was supported by the National Natural Science Foundation of China (Grant No. 81873647) and Major Basic Research Project of Natural Science Foundation of Shandong Province (Grant No. ZR2020ZD15).
Conflict of interest
The authors declare that the research was conducted in the absence of any commercial or financial relationships that could be construed as a potential conflict of interest.
Publisher’s note
All claims expressed in this article are solely those of the authors and do not necessarily represent those of their affiliated organizations, or those of the publisher, the editors and the reviewers. Any product that may be evaluated in this article, or claim that may be made by its manufacturer, is not guaranteed or endorsed by the publisher.
Supplementary material
The Supplementary Material for this article can be found online at: https://www.frontiersin.org/articles/10.3389/fendo.2022.1015819/full#supplementary-material
Supplementary Figure 1 | DM induced significant impairment in the animal behaviors in the Y-maze test(A, B). Data were expressed as means ± SEM for n = 10 per group. *p < 0.05 vs. CON group, **p < 0.01 vs. CON group, ***p < 0.001 vs. CON group. CON, control; DM, diabetes mellitus; SHAM, sham operation; SG, sleeve gastrectomy.
References
1. Zheng Y, Ley SH, Hu FB. Global aetiology and epidemiology of type 2 diabetes mellitus and its complications. Nat Rev Endocrinol (2018) 14(2):88–98. doi: 10.1038/nrendo.2017.151
2. Zilliox LA, Chadrasekaran K, Kwan JY, Russell JW. Diabetes and cognitive impairment. Curr Diabetes Rep (2016) 16(9):87. doi: 10.1007/s11892-016-0775-x
3. Moheet A, Mangia S, Seaquist ER. Impact of diabetes on cognitive function and brain structure. Ann New York Acad Sci (2015) 1353:60–71. doi: 10.1111/nyas.12807
4. Biessels GJ, Nobili F, Teunissen CE, Simó R, Scheltens P. Understanding multifactorial brain changes in type 2 diabetes: A biomarker perspective. Lancet Neurol (2020) 19(8):699–710. doi: 10.1016/s1474-4422(20)30139-3
5. Dutta BJ, Singh S, Seksaria S, Das Gupta G, Singh A. Inside the diabetic brain: Insulin resistance and molecular mechanism associated with cognitive impairment and its possible therapeutic strategies. Pharmacol Res (2022) 182:106358. doi: 10.1016/j.phrs.2022.106358
6. Goldberg I, Nie L, Yang J, Docimo S, Obici S, Talamini M, et al. Impact of bariatric surgery on the development of diabetic microvascular and macrovascular complications. Surg Endosc (2021) 35(7):3923–31. doi: 10.1007/s00464-020-07848-2
7. Xiong Y, Zhu W, Xu Q, Ruze R, Yan Z, Li J, et al. Sleeve gastrectomy attenuates diabetic nephropathy by upregulating nephrin expressions in diabetic obese rats. Obes Surg (2020) 30(8):2893–904. doi: 10.1007/s11695-020-04611-3
8. Via MA, Mechanick JI. The role of bariatric surgery in the treatment of type 2 diabetes: Current evidence and clinical guidelines. Curr Atheroscl Rep (2013) 15(11):366. doi: 10.1007/s11883-013-0366-0
9. Pareek M, Schauer PR, Kaplan LM, Leiter LA, Rubino F, Bhatt DL. Metabolic surgery: Weight loss, diabetes, and beyond. J Am Coll Cardiol (2018) 71(6):670–87. doi: 10.1016/j.jacc.2017.12.014
10. Morledge MD, Pories WJ. Bariatric surgery and cognitive impairment. Obes (Silver Spring Md) (2021) 29(8):1239–41. doi: 10.1002/oby.23187
11. Yang W, Liu Y, Xu QQ, Xian YF, Lin ZX. Sulforaphene ameliorates neuroinflammation and hyperphosphorylated tau protein Via regulating the Pi3k/Akt/Gsk-3β pathway in experimental models of alzheimer's disease. Oxid Med Cell Longev (2020) 2020:4754195. doi: 10.1155/2020/4754195
12. Wang BN, Wu CB, Chen ZM, Zheng PP, Liu YQ, Xiong J, et al. Dl-3-N-Butylphthalide ameliorates diabetes-associated cognitive decline by enhancing Pi3k/Akt signaling and suppressing oxidative stress. Acta Pharmacol Sin (2021) 42(3):347–60. doi: 10.1038/s41401-020-00583-3
13. Abeyrathna P, Su Y. The critical role of akt in cardiovascular function. Vasc Pharmacol (2015) 74:38–48. doi: 10.1016/j.vph.2015.05.008
14. Manning BD, Toker A. Akt/Pkb signaling: Navigating the network. Cell (2017) 169(3):381–405. doi: 10.1016/j.cell.2017.04.001
15. Wang S, He B, Hang W, Wu N, Xia L, Wang X, et al. Berberine alleviates tau hyperphosphorylation and axonopathy-associated with diabetic encephalopathy Via restoring Pi3k/Akt/Gsk3β pathway. J Alzheimer's Dis JAD (2018) 65(4):1385–400. doi: 10.3233/jad-180497
16. De Simone A, Tumiatti V, Andrisano V, Milelli A. Glycogen synthase kinase 3β: A new gold rush in anti-alzheimer's disease multitarget drug discovery? J Med Chem (2021) 64(1):26–41. doi: 10.1021/acs.jmedchem.0c00931
17. Tang SS, Ren Y, Ren XQ, Cao JR, Hong H, Ji H, et al. Erα and/or erβ activation ameliorates cognitive impairment, neurogenesis and apoptosis in type 2 diabetes mellitus mice. Exp Neurol (2019) 311:33–43. doi: 10.1016/j.expneurol.2018.09.002
18. Hornack SE, Nadler EP, Wang J, Hansen A, Mackey ER. Sleeve gastrectomy for youth with cognitive impairment or developmental disability. Pediatrics (2019) 143(5):2. doi: 10.1542/peds.2018-2908
19. Li P, Shan H, Liang S, Nie B, Liu H, Duan S, et al. Sleeve gastrectomy recovering disordered brain function in subjects with obesity: A longitudinal fmri study. Obes Surg (2018) 28(8):2421–8. doi: 10.1007/s11695-018-3178-z
20. Zhang KQ, Tian T, Hu LL, Wang HR, Fu Q. Effect of probucol on autophagy and apoptosis in the penile tissue of streptozotocin-induced diabetic rats. Asian J Androl (2020) 22(4):409–13. doi: 10.4103/aja.aja_89_19
21. Bruinsma BG, Uygun K, Yarmush ML, Saeidi N. Surgical models of roux-En-Y gastric bypass surgery and sleeve gastrectomy in rats and mice. Nat Protoc (2015) 10(3):495–507. doi: 10.1038/nprot.2015.027
22. Ozmen MM, Guldogan CE, Gundogdu E. Changes in homa-ir index levels after bariatric surgery: Comparison of single anastomosis duodenal switch-proximal approach (Sads-p) and one anastomosis gastric bypass-mini gastric bypass (Oagb-mgb). Int J Surg (London England) (2020) 78:36–41. doi: 10.1016/j.ijsu.2020.04.008
23. Dolo PR, Huang K, Widjaja J, Li C, Zhu X, Yao L, et al. Distal gastric mucosa ablation induces significant weight loss and improved glycemic control in type 2 diabetes sprague-dawley rat model. Surg Endosc (2020) 34(10):4336–46. doi: 10.1007/s00464-019-07200-3
24. Harris MI, Hadden WC, Knowler WC, Bennett PH. International criteria for the diagnosis of diabetes and impaired glucose tolerance. Diabetes Care (1985) 8(6):562–7. doi: 10.2337/diacare.8.6.562
25. Ozkan A, Aslan MA, Sinen O, Munzuroglu M, Derin N, Parlak H, et al. Effects of adropin on learning and memory in rats tested in the morris water maze. Hippocampus (2022) 32(4):253–63. doi: 10.1002/hipo.23403
26. Lamtai M, Azirar S, Zghari O, Ouakki S, El Hessni A, Mesfioui A, et al. Melatonin ameliorates cadmium-induced affective and cognitive impairments and hippocampal oxidative stress in rat. Biol Trace Elem Res (2021) 199(4):1445–55. doi: 10.1007/s12011-020-02247-z
27. Rorabaugh JM, Chalermpalanupap T, Botz-Zapp CA, Fu VM, Lembeck NA, Cohen RM, et al. Chemogenetic locus coeruleus activation restores reversal learning in a rat model of alzheimer's disease. Brain (2017) 140(11):3023–38. doi: 10.1093/brain/awx232
28. Zhang S, Li H, Zhang L, Li J, Wang R, Wang M. Effects of troxerutin on cognitive deficits and glutamate cysteine ligase subunits in the hippocampus of streptozotocin-induced type 1 diabetes mellitus rats. Brain Res (2017) 1657:355–60. doi: 10.1016/j.brainres.2016.12.009
29. Biessels GJ, Despa F. Cognitive decline and dementia in diabetes mellitus: Mechanisms and clinical implications. Nat Rev Endocrinol (2018) 14(10):591–604. doi: 10.1038/s41574-018-0048-7
30. McCrimmon RJ, Ryan CM, Frier BM. Diabetes and cognitive dysfunction. Lancet (London England) (2012) 379(9833):2291–9. doi: 10.1016/s0140-6736(12)60360-2
31. Hanyu H. Diabetes-related dementia. Adv Exp Med Biol (2019) 1128:147–60. doi: 10.1007/978-981-13-3540-2_8
32. Jash K, Gondaliya P, Kirave P, Kulkarni B, Sunkaria A, Kalia K. Cognitive dysfunction: A growing link between diabetes and alzheimer's disease. Drug Dev Res (2020) 81(2):144–64. doi: 10.1002/ddr.21579
33. Ehtewish H, Arredouani A, El-Agnaf O. Diagnostic, prognostic, and mechanistic biomarkers of diabetes mellitus-associated cognitive decline. Int J Mol Sci (2022) 23(11):9. doi: 10.3390/ijms23116144
34. Ryan CM, van Duinkerken E, Rosano C. Neurocognitive consequences of diabetes. Am Psychol (2016) 71(7):563–76. doi: 10.1037/a0040455
35. Novak V, Gomez F, Dias AC, Pimentel DA, Alfaro FJ. Diabetes-related cognitive decline, a global health issue, and new treatments approaches. Int J Priv Health Inf Manage (2017) 5(2):58–70. doi: 10.4018/ijphim.2017070104
36. Kravitz E, Schmeidler J, Schnaider Beeri M. Type 2 diabetes and cognitive compromise: Potential roles of diabetes-related therapies. Endocrinol Metab Clinics North America (2013) 42(3):489–501. doi: 10.1016/j.ecl.2013.05.009
37. Pories WJ, Swanson MS, MacDonald KG, Long SB, Morris PG, Brown BM, et al. Who would have thought it? an operation proves to be the most effective therapy for adult-onset diabetes mellitus. Ann Surg (1995) 222(3):339–50. doi: 10.1097/00000658-199509000-00011
38. Affinati AH, Esfandiari NH, Oral EA, Kraftson AT. Bariatric surgery in the treatment of type 2 diabetes. Curr Diabetes Rep (2019) 19(12):156. doi: 10.1007/s11892-019-1269-4
39. Gheibi S, Kashfi K, Ghasemi A. A practical guide for induction of type-2 diabetes in rat: Incorporating a high-fat diet and streptozotocin. BioMed Pharmacother (2017) 95:605–13. doi: 10.1016/j.biopha.2017.08.098
40. Yang H, Tang L, Qu Z, Lei SH, Li W, Wang YH. Hippocampal insulin resistance and the sirtuin 1 signaling pathway in diabetes-induced cognitive dysfunction. Neural Regen Res (2021) 16(12):2465–74. doi: 10.4103/1673-5374.313051
41. Ye T, Meng X, Zhai Y, Xie W, Wang R, Sun G, et al. Gastrodin ameliorates cognitive dysfunction in diabetes rat model Via the suppression of endoplasmic reticulum stress and Nlrp3 inflammasome activation. Front Pharmacol (2018) 9:1346. doi: 10.3389/fphar.2018.01346
42. Alkadhi KA. Cellular and molecular differences between area Ca1 and the dentate gyrus of the hippocampus. Mol Neurobiol (2019) 56(9):6566–80. doi: 10.1007/s12035-019-1541-2
43. Guzzardi MA, Iozzo P. Brain functional imaging in obese and diabetic patients. Acta Diabetol (2019) 56(2):135–44. doi: 10.1007/s00592-018-1185-0
44. Baker LD, Cross DJ, Minoshima S, Belongia D, Watson GS, Craft S. Insulin resistance and Alzheimer-like reductions in regional cerebral glucose metabolism for cognitively normal adults with prediabetes or early type 2 diabetes. Arch Neurol (2011) 68(1):51–7. doi: 10.1001/archneurol.2010.225
45. Hamed SA. Brain injury with diabetes mellitus: Evidence, mechanisms and treatment implications. Expert Rev Clin Pharmacol (2017) 10(4):409–28. doi: 10.1080/17512433.2017.1293521
46. Gao C, Liu Y, Jiang Y, Ding J, Li L. Geniposide ameliorates learning memory deficits, reduces tau phosphorylation and decreases apoptosis Via Gsk3β pathway in streptozotocin-induced Alzheimer rat model. Brain Pathol (Zurich Switzerland) (2014) 24(3):261–9. doi: 10.1111/bpa.12116
47. Xu W, Liu J, Ma D, Yuan G, Lu Y, Yang Y. Capsaicin reduces Alzheimer-associated tau changes in the hippocampus of type 2 diabetes rats. PloS One (2017) 12(2):e0172477. doi: 10.1371/journal.pone.0172477
48. Li H, Tang Z, Chu P, Song Y, Yang Y, Sun B, et al. Neuroprotective effect of phosphocreatine on oxidative stress and mitochondrial dysfunction induced apoptosis in vitro and in vivo: Involvement of dual Pi3k/Akt and Nrf2/Ho-1 pathways. Free Radical Biol Med (2018) 120:228–38. doi: 10.1016/j.freeradbiomed.2018.03.014
49. Wang G, Fang H, Zhen Y, Xu G, Tian J, Zhang Y, et al. Sulforaphane prevents neuronal apoptosis and memory impairment in diabetic rats. Cell Physiol Biochem (2016) 39(3):901–7. doi: 10.1159/000447799
50. Maffei A, Lembo G, Carnevale D. Pi3kinases in diabetes mellitus and its related complications. Int J Mol Sci (2018) 19(12):10. doi: 10.3390/ijms19124098
51. Jayaraj RL, Azimullah S, Beiram R. Diabetes as a risk factor for alzheimer's disease in the middle East and its shared pathological mediators. Saudi J Biol Sci (2020) 27(2):736–50. doi: 10.1016/j.sjbs.2019.12.028
52. Deng S, Dai G, Chen S, Nie Z, Zhou J, Fang H, et al. Dexamethasone induces osteoblast apoptosis through ros-Pi3k/Akt/Gsk3β signaling pathway. BioMed Pharmacother (2019) 110:602–8. doi: 10.1016/j.biopha.2018.11.103
53. Huang X, Liu G, Guo J, Su Z. The Pi3k/Akt pathway in obesity and type 2 diabetes. Int J Biol Sci (2018) 14(11):1483–96. doi: 10.7150/ijbs.27173
54. Zhang Y, Huang NQ, Yan F, Jin H, Zhou SY, Shi JS, et al. Diabetes mellitus and alzheimer's disease: Gsk-3β as a potential link. Behav Brain Res (2018) 339:57–65. doi: 10.1016/j.bbr.2017.11.015
55. Sun LJ, Hou XH, Xue SH, Yan F, Dai YJ, Zhao CH, et al. Fish oil modulates glycogen synthase kinase-3 signaling pathway in diabetes-induced hippocampal neurons apoptosis. Brain Res (2014) 1574:37–49. doi: 10.1016/j.brainres.2014.05.050
Keywords: diabetes-related cognitive decline, sleeve gastrectomy, PI3K, tau, neuronal apoptosis
Citation: Ding H, Liu C, Zhang S, Li B, Xu Q, Shi B, Li S, Dong S, Ma X, Zhang Y, Zhong M and Zhang G (2022) Sleeve gastrectomy attenuated diabetes-related cognitive decline in diabetic rats. Front. Endocrinol. 13:1015819. doi: 10.3389/fendo.2022.1015819
Received: 10 August 2022; Accepted: 19 October 2022;
Published: 03 November 2022.
Edited by:
Wah Yang, The First Affiliated Hospital of Jinan University, ChinaReviewed by:
Yanmin Wang, California Medical Innovations Institute, United StatesJason Widjaja, Fudan UniversityChina
Cunchuan Wang, First Affiliated Hospital of Jinan University, China
Copyright © 2022 Ding, Liu, Zhang, Li, Xu, Shi, Li, Dong, Ma, Zhang, Zhong and Zhang. This is an open-access article distributed under the terms of the Creative Commons Attribution License (CC BY). The use, distribution or reproduction in other forums is permitted, provided the original author(s) and the copyright owner(s) are credited and that the original publication in this journal is cited, in accordance with accepted academic practice. No use, distribution or reproduction is permitted which does not comply with these terms.
*Correspondence: Guangyong Zhang, guangyongzhang@hotmail.com