- 1CARIM School for Cardiovascular Diseases, Maastricht University (UM), Maastricht, Netherlands
- 2Department of Internal Medicine, Maastricht University Medical Center+ (MUMC+), Maastricht, Netherlands
- 3Department of Psychiatry and Neuropsychology, MUMC+, Maastricht, Netherlands
- 4School of Mental Health and Neuroscience, MUMC+, Maastricht, Netherlands
Dysfunction of the neurovascular coupling unit may be an important contributor to dementia. The neurovascular coupling unit comprises neuronal structures (e.g. astrocytes) and vascular structures (e.g. endothelial cells) that functionally interact both at the level of the arterioles as well as at the capillary level (blood-brain barrier) to regulate optimal metabolic conditions in the brain. However, it remains unclear how and to what extent dysfunction of the neurovascular coupling unit contributes to the early-stage pathobiology of dementia. Currently, limited data are available on the association between neurovascular coupling unit dysfunction, as quantified by cerebral imaging techniques, and cognitive performance. In particular, there is a lack of population-based human data (defined as studies with a sample size ~n>500). This is an important limitation because population-based studies, in comparison with smaller clinical studies, provide data which is better representative of the general population; are less susceptible to selection bias; and have a larger statistical power to detect small associations. To acquire population-based data, however, alternative imaging techniques than cerebral imaging techniques may be required. Disadvantages of cerebral imaging techniques, which limit use in population-based studies, are that these techniques are relatively expensive, time-consuming, and/or invasive. In this review, we propose that retinal imaging techniques can be used for population-based studies: on the one hand the retina and brain have many anatomical and physiological similarities; and on the other hand retinal imaging techniques are non-invasive, highly accurate, relatively inexpensive, and require relatively short measurement time. To provide support for this concept, we provide an overview on the human (population-based) evidence on the associations of retinal indices of neurodegeneration, microvascular dysfunction, and dysfunction of the neurovascular coupling unit with magnetic resonance imaging (MRI) features of structural brain abnormalities and cognitive performance.
Introduction
There is an imperative for the development of novel therapeutic strategies that aim to prevent dementia at an early stage (1–3). Dementia can have debilitating consequences on the quality of life, is associated with a high risk of mortality, and has a large burden on healthcare systems (1–3). Globally, approximately ~55 million individuals currently have dementia and a dramatic increase (up to ~90%) is anticipated in the next decades (1, 4). A better understanding of the early pathophysiology of dementia is crucial for development of effective strategies to prevent and/or delay the onset of dementia (3, 5).
Dysfunction of the neurovascular coupling unit is considered an important contributor to the onset of different types of dementia, including Alzheimer’s disease and vascular dementia. The neurovascular coupling unit comprises neuronal structures (e.g. glial cells) and vascular structures (e.g. endothelial cells) in the brain that collaborate in a highly coordinated manner to regulate metabolic circumstances for optimal brain function (6, 7). (Box 1) Degeneration of neuronal and vascular structures can lead to dysfunction of the neurovascular coupling unit, which can predispose to ischemic circumstances resulting in structural brain abnormalities. This then can lead to dysfunction of neuronal networks, that, in turn, contributes to cognitive dysfunction and, ultimately, dementia (6, 7).
Box 1 | The neurovascular coupling unit
The neurovascular coupling unit is responsible for the regulation of the supply of sufficient levels of nutrients and oxygen to neuronal cells in the brain, in order to meet the local energy demand.
Intact function of the neurovascular coupling unit is crucial for optimal neuronal function because the brain lacks energy reserves and is dependent on a continuous and well-regulated delivery of oxygen and nutrients (such as glucose) through the cerebral blood supply (6, 7, 11–13).
The neurovascular coupling unit comprises neuronal and microvascular structures that functionally interact both at the level of the arterioles as well as at the capillary level (blood-brain barrier) to regulate optimal metabolic conditions (Figure 1) (6, 7, 11–13). The neurovascular coupling unit, on the one hand, regulates the extent of blood flow to the capillaries (via regulation of the diameter of arterioles); and, on the other hand, regulates the integrity of the blood-brain barrier, which enables the maintenance of an optimal metabolic milieu for neurons (6, 7, 11–13). These functions are executed via bidirectional interactions between neuronal cells, i.e. neurons and glial cells (astrocytes, microglia, oligodendrocytes) and microvascular structures, i.e. endothelial cells, pericytes, and vascular smooth muscle cells (6, 7, 11–13). The exact communication among different cell types is reviewed elsewhere (6, 12). Briefly, neuronal cells can alter arteriolar diameter via transmitting signals to the endothelial cells in the capillaries, which via endothelial cell-to-cell connections can be transmitted to endothelial cells in the arterioles (i.e. neurovascular coupling). The arteriolar diameter is then modulated via nitric oxide (NO) release from endothelial cells to vascular smooth muscle cells (6, 7, 11–13). Microvascular structures, such as pericytes, may also contribute to the regulation of vascular smooth muscle cells via inducing NO release from neuronal cells (vasculo-neuronal coupling; e.g. via pericyte-astrocyte interactions) (6, 11, 12). Furthermore, the bidirectional interactions between neuronal and microvascular structures might also occur at the level of the venules (6, 7, 11–13).
At present, it remains incompletely understood how and to what extent dysfunction of the neurovascular coupling unit contributes to the early stage pathobiology of dementia (6, 7). Hence it is important to investigate: 1) how dysfunction of the neurovascular coupling unit is associated with cognitive performance; and 2) how degeneration of the components of the neurovascular coupling unit (i.e. neuronal and vascular structures) and loss of the interaction between neuronal and vascular structures are associated with cognitive performance.
To obtain such insight, it is important that large scale population-based evidence from humans is acquired (6, 7). Methodological strengths of population-based studies (in the present review defined as ~n>500) are that such studies provide data that is representative of (thus valid in) the general population; are less susceptible to selection bias than smaller studies which use clinical study populations; and are well-powered (i.e. due to the large size of the study population small associations can be detected) (8).
It is likely not feasible to acquire population-based data using currently existing cerebral imaging techniques. Disadvantages of cerebral imaging techniques are that these techniques are expensive, time-consuming, and do not allow the direct visualization of neurons and blood vessels (6, 7). In addition, a disadvantage of some, but not all, cerebral imaging techniques is that they are invasive (e.g. dynamic contrast-enhanced magnetic resonance imaging [DCE-MRI] requires the administration of exogenous contrast) (9).
As the retina has been postulated to be a window to the brain, imaging the retinal may provide opportunity to move towards quantitative population-based evidence on how and to what extent dysfunction of the neurovascular coupling unit contributes to the early pathobiology of cognitive dysfunction. The retina and brain share many anatomical and physiological similarities (10). Important advantages of retinal imaging are that retinal imaging techniques are non-invasive, highly accurate, relatively inexpensive, require relatively short measurement time, and can be used to directly visualize neuronal and microvascular components of the neurovascular coupling unit (10).
In this review, we demonstrate 1) that there is presently limited data on the association of dysfunction of neurovascular coupling unit, as estimated with cerebral imaging techniques, with cognitive performance; and 2) that there may be an opportunity to, using retinal imaging techniques, acquire population-based data on how and to what extent degeneration of components of the neurovascular coupling unit (i.e. neuronal and microvascular structures) and dysfunction of the neurovascular coupling unit are associated with early stage cognitive dysfunction. In section 1, we review the available human (population-based) evidence on the association of dysfunction of neurovascular coupling unit, as estimated with cerebral imaging techniques, with cognitive performance. In sections 2 and 3, we review the available human (population-based) evidence on the associations of neuronal and microvascular structures and function of the neurovascular coupling unit, as quantified by retinal imaging techniques, with cerebral magnetic resonance imaging (MRI) features of structural brain abnormalities (i.e. brain volume, white matter hyperintensities, lacunar infarcts, and cerebral microbleeds; section 2) and cognitive performance (section 3). A background on the neurovascular coupling unit is given in Panel 1 and Figure 1.
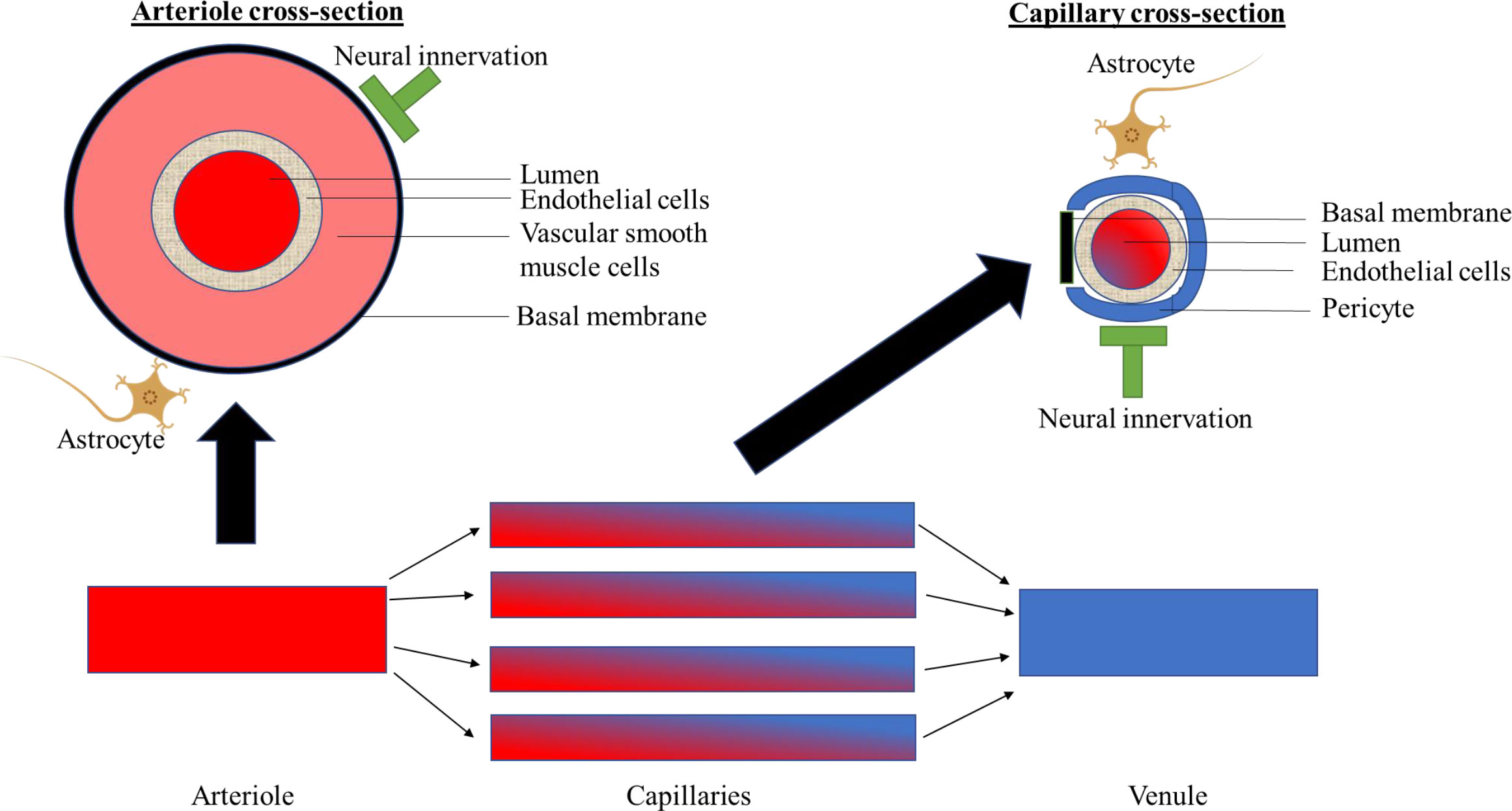
Figure 1 Schematically shows components of the neurovascular coupling unit at the level of the arterioles, capillaries and venules.
Assessment of the function of the neurovascular coupling unit
Recently, a number of brain imaging techniques have been proposed to quantify neurovascular coupling unit function, as reviewed elsewhere (14). These include quantification of cerebral perfusion at rest and during neuronal activity, and quantification of blood-brain barrier function. Intact cerebral perfusion and blood-brain barrier function all presumably require intact function of the neurovascular coupling (14). Cerebral perfusion at rest can be assessed with single-photon emission computed tomography (SPECT) and arterial spin labelling (ASL)-MRI; cerebrovascular vasoreactivity can be assessed with blood oxygen level-dependent (BOLD) fMRI; and the integrity of the blood-brain barrier can be assessed with dynamic contrast enhanced (DCE)-MRI and the cerebrospinal fluid (CSF)/blood-albumin ratio (14).
Pathophysiological mechanisms: Dysfunction of the neurovascular coupling unit and cognitive dysfunction
Impaired function of the neurovascular coupling unit may via several mechanisms lead to structural brain changes, which can lead to cognitive dysfunction (6, 7, 15). As postulated in the “Calcium Hypothesis”, cerebral blood flow deficits may lead to transient large increases of Calcium2+ (Ca2+) that can cause glutamate excitotoxicity and can lead to neurodegeneration (6, 7, 15). Additionally, cerebral blood flow deficits may impair the clearance of waste products from the neuronal tissue, which can result in the accumulation of toxic waste products in neuronal tissue, predisposing to degeneration of neuronal and microvascular structures (6, 7, 15). Further, deterioration of the integrity of the blood-brain barrier may result in the leakage of toxins from the circulation into neuronal tissue, such as amyloid beta, which may damage neuronal and microvascular structures (6, 7, 15).
Causes of degeneration of components of the neurovascular coupling unit
Several potentially modifiable risk factors have been identified that may contribute to degeneration of neuronal and microvascular structures (16–19). Key factors are diabetes, midlife hypertension, midlife obesity, physical inactivity, and smoking, as reviewed elsewhere (16–19).
Of the neuronal components of the neurovascular coupling unit only neurons and astrocytes are shown. For a further explanation, please see Panel 1. Section 1: Summary of human evidence on the association of dysfunction of the neurovascular coupling unit, as quantified by cerebral imaging, with cognitive performance
In this section, we review the present body of evidence on the association of dysfunction of the neurovascular coupling unit, as quantified by lower cerebral perfusion at rest, lower cerebral vasoreactivity, and higher blood-brain barrier permeability, with cognitive performance. We searched for studies that estimated cerebral perfusion at rest from SPECT or ASL-MRI; cerebral vasoreactivity from BOLD fMRI; and blood-brain barrier dysfunction from DCE-MRI or CSF/blood-albumin ratio (for an explanation of these techniques, see Panel 1) (14). Details on the search strategy are given in the Supplemental Methods.
Literature overview
Table 1 shows an overview of our findings. We did not identify any population-based studies (n>500) and, therefore, we show findings for smaller studies in Table 1. We identified three studies on cerebral perfusion at rest estimated with SPECT (one prospective study [n=103] and two cross-sectional studies [n=12-n=104]); four studies on cerebral vasoreactivity estimated with BOLD fMRI (one prospective study [n=116] and three cross-sectional studies [n=59-n=232]); 33 studies on cerebral perfusion at rest estimated from ASL-MRI (of these 33 studies we show the five largest studies [those with a study population sample size of n≥150] in Table 1; other studies are given in Supplemental Table S2]; of these five studies, one study was prospective [n=309] and four studies were cross-sectional [n=162-n=179]); nine studies on blood-brain barrier leakage estimated with DCE-MRI (two prospective studies [n=51 and n=57] and seven cross-sectional studies [n=45-n=102]); and three studies on blood-brain barrier leakage estimated with the CSF/blood albumin ratio (one prospective study [n=118] and two cross-sectional studies [n=45 and n=118]).

Table 1 Association of dysfunction of the neurovascular coupling unit, as quantified by cerebral imaging, with cognitive performance.
Summary of findings
Most studies found that lower cerebral perfusion at rest and lower cerebrovascular vasoreactivity (as quantified with SPECT, BOLD fMRI, and/or ASL-MRI) and higher blood-brain barrier leakage (as quantified with DCE-MRI or CSF/blood albumin ratio) were associated with lower cognitive performance. These findings support the concept that dysfunction of the neurovascular coupling unit may contribute to the early pathobiology of cognitive dysfunction.
Limitations of the evidence
The current evidence has certain methodological limitations. First, most studies were relatively small and included clinical samples only. Data from such smaller studies is relatively less representative of the general population than large population-based studies, thus the generalizability of findings to the general population is limited (8). Second, most studies were cross-sectional, hence these studies do not allow to account for temporality, which is an important criterion for the inference of causality (8). Third, most studies did not adjust in the analysis for potentially important confounders, including cardiovascular risk factors such as blood pressure or hyperglycemia; and lifestyle factors such as physical activity or diet. This may have led to an overestimation of the strength of the association between dysfunction of the neurovascular coupling unit and cognitive performance (8).
Section 2: Summary of human evidence on the associations of neuronal and microvascular structures and function of the neurovascular coupling unit, as quantified by retinal imaging, with cerebral MRI features of structural brain abnormalities
Box 2 gives a background on biological similarities between the retina and brain; and Panel 2 and Figure 2 provide an overview of retinal imaging techniques for the assessment of neuronal and microvascular structures and the function of the neurovascular coupling unit.
Box 2 | Biological similarities between the retina and brain
The retina and the brain have a shared embryology, and have a similar physiology, anatomy, and pathophysiology (10). Embryologically, both the retina and the brain are derived from the neural ectoderm; the retina invaginates from the diencephalon around 23 days of gestation (10). Next, the neurovascular coupling unit in the retina and the brain have similar functions. Like in the brain, neurovascular coupling at the level of the retina regulates cerebral perfusion at rest and during neuronal activity, and it maintains the integrity of the blood-retina barrier, a barrier which is analogous to the blood-brain barrier (10). Further, the same cell types control function of the neurovascular unit in the retina and the brain; and these cells use the same biological mechanisms to control these processes, as reviewed elsewhere (10). For example, both retinal and cerebral neurons use the neurotransmitters glutamate, gaba-aminobutyric acid (GABA), acetylcholine, dopamine, and glycine for intrasynaptic signaling; retinal and cerebral capillaries have similar tight junctions; capillary branching angles; and pericyte-to-endothelial cell ratio (10). Last, the pathophysiological mechanisms that underlie degeneration of components of the neurovascular coupling unit in the brain and retina have been found to be similar (10). For example, amyloid beta accumulation, a hallmark feature of Alzheimer’s disease, has been found in the retina as well as in the brain of individuals with Alzheimer’s disease (10).
In this section, we review the present body of evidence on the associations of neuronal and microvascular structures and function of the neurovascular coupling unit, as quantified by retinal imaging, with cerebral MRI features of structural brain abnormalities. We searched for studies that assessed features of cerebral small vessel disease (i.e. brain volume; white matter hyperintensities, cerebral microbleeds, and lacunar infarcts) (14) and retinal neurodegeneration (assessed as lower retinal nerve fiber layer [RNFL] thickness); retinal microvascular dysfunction (assessed as narrower or wider retinal arterioles and wider retinal venules); or retinal dysfunction of the neurovascular coupling unit (assessed as lower flicker light-induced increase in retinal microvascular diameters; lower perfusion of arterioles and venules at rest [assessed as lower velocity]; or lower capillary perfusion at rest [assessed as greater foveal avascular zone area or lower vascular density]) (10, 17). Details on the search strategy are given in the Supplemental Methods.
Retinal imaging techniques for the assessment of neuronal and microvascular structures and the function of the neurovascular coupling unit
We discuss the following retinal imaging tools as a means for the quantification of neuronal and microvascular structures, respectively: optical coherence tomography (OCT) and fundoscopy; and the following retinal imaging tools as a means for the quantification of the function of the neurovascular coupling unit in the retina: dynamic vessel analyzer, retinal laser Doppler flowmetry, and OCT angiography (OCT-A) (10).
Optical coherence tomography
The thickness of individual retinal layers, including the RNFL, can be assessed with OCT (10). RNFL thickness is thought to reflect the number of retinal ganglion cell axons (thus a lower RNFL thickness indicates more neurodegeneration) and can be assessed in the central macular area (“perifoveal”; Figure 2.1) as well as around the optic nerve head (“peripapillary”; Figure 2.2) (10).
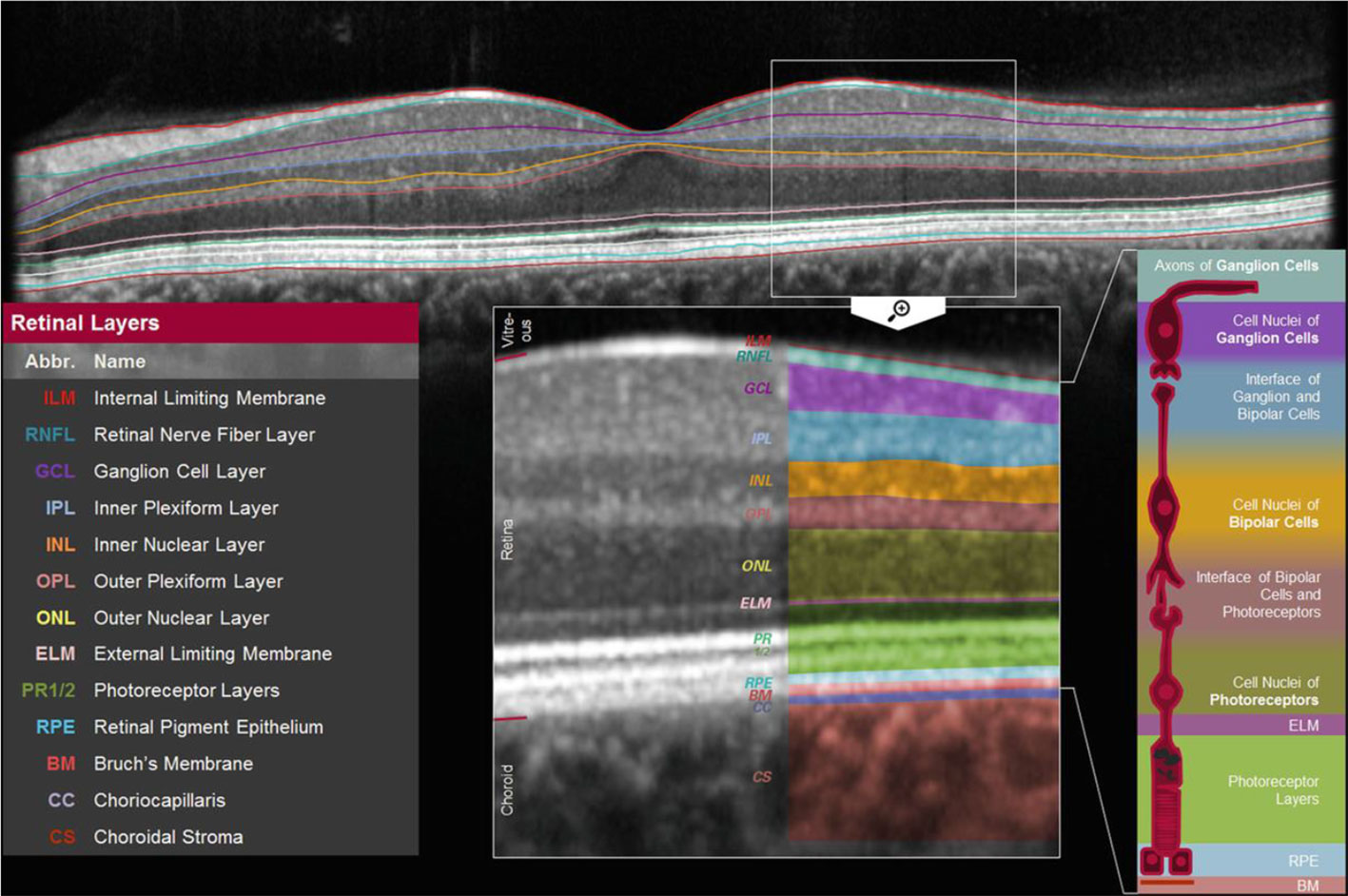
Figure 2.1 Macular (perifoveal) RNFL thickness. Figure 2.1 shows a cross-section of the macula which shows the thickness of individual retinal layers, including the thickness of the retinal nerve fiber layer [adapted from (41)].
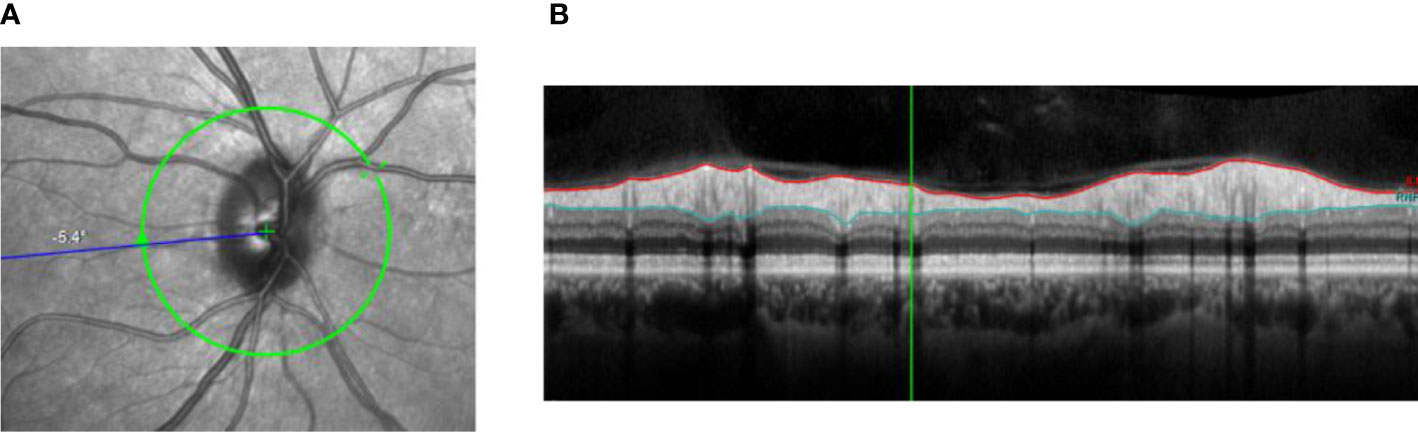
Figure 2.2 Peripapillary RNFL thickness. (A) Shows the location where the thickness of the RNFL can be determined around the optic nerve. Figure 2.2B shows the contours of the RNFL (red and light blue lines). (B) Shows the contours of the RNFL (red and light blue lines).
Fundoscopy
The diameter of retinal arteriolar and venular blood vessels around the optic nerve head can be assessed from fundus images (Figure 2.3) (10). Narrower or wider retinal arterioles and wider retinal venules are thought to reflect more microvascular dysfunction (10, 17).
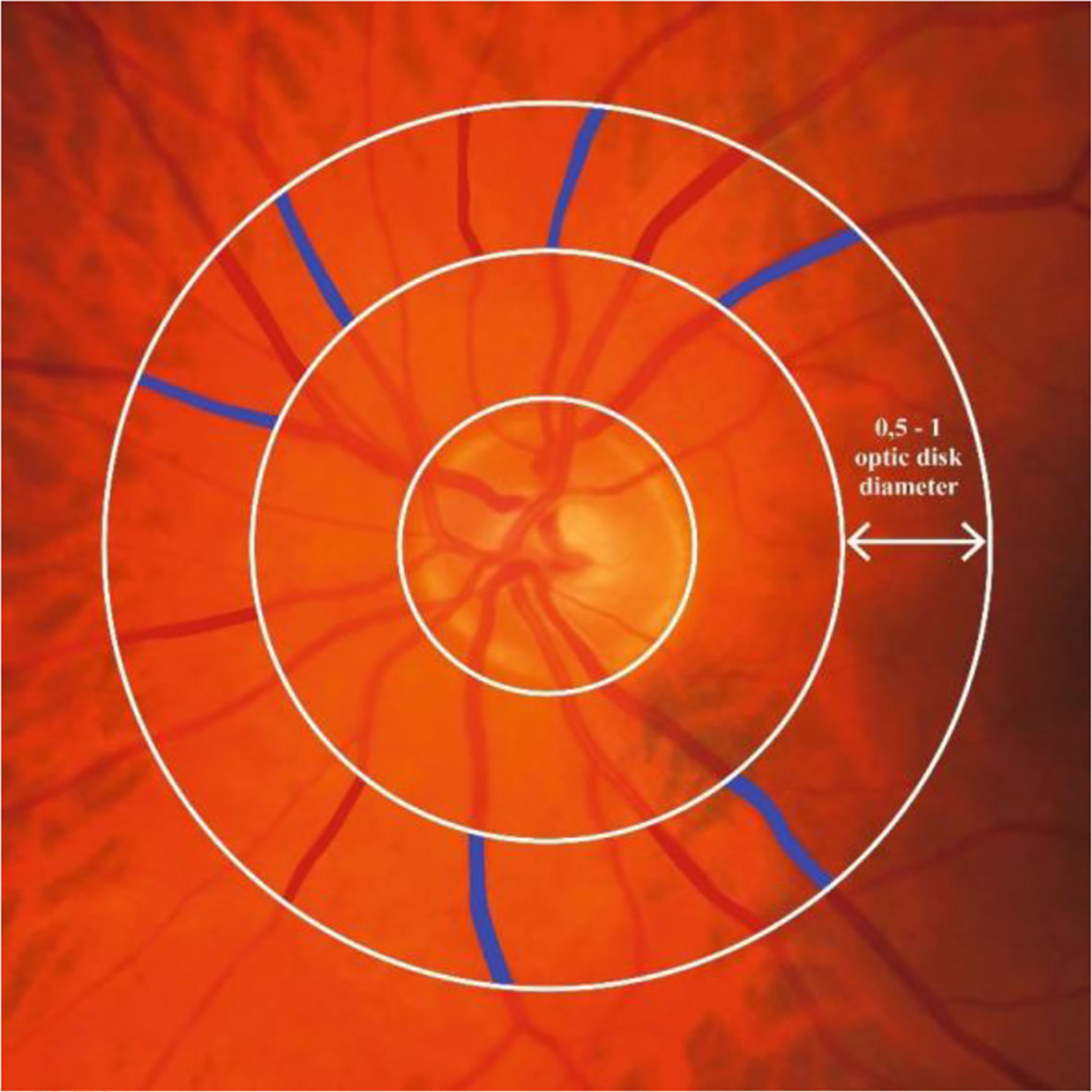
Figure 2.3 Retinal microvascular diameters. Figure 2.3 shows where retinal arteriolar and venular diameters can be assessed around the optic nerve. Red and blue color indicate the area on the fundus photo covered by an arteriole and venule, respectively, at 1 disk diameter from the optic nerve head. Retinal arteriolar and venular diameters are calculated as the average diameter of six arterioles or six venules, respectively.
Dynamic vessel analyzer
The dynamic vessel analyzer is a device that can quantify the change in retinal peripapillary arteriolar and venular diameters in response to exposure to flicker light (Figure 2.4) (42, 43). Flicker light is presumed to increase the metabolic demand of the retina, inducing an increase in retinal perfusion, which leads to widening of retinal arteriolar and venular diameters (42, 43). As the increase in retinal microvascular diameter is presumed to rely on the function of the neurovascular coupling unit, a greater increase in retinal arteriolar and venular diameter in response to flicker-light exposure is presumed to reflect better function of the neurovascular coupling unit (42, 43).
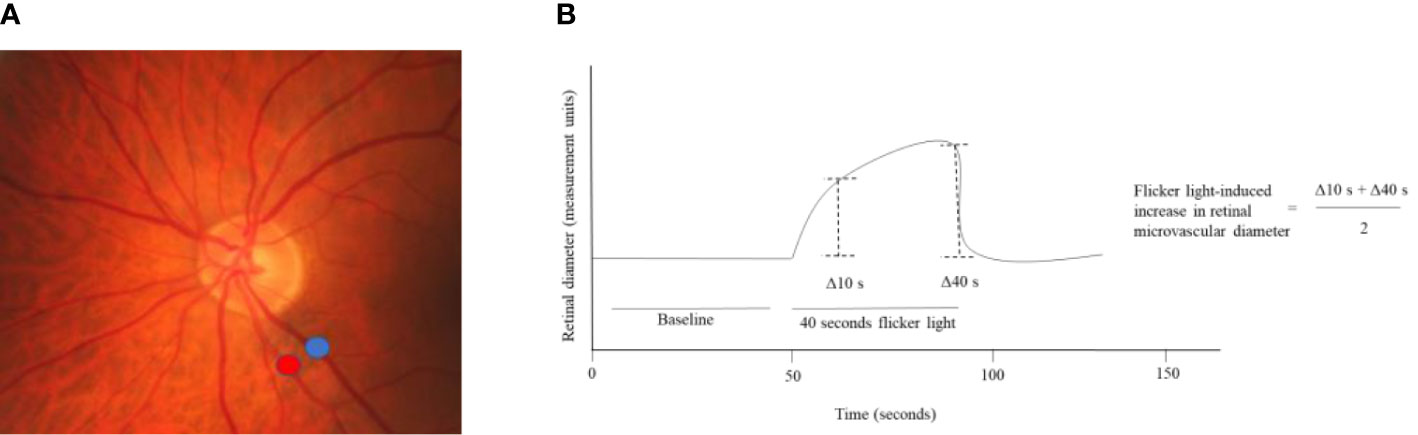
Figure 2.4 Flicker light-induced increase in retinal microvascular diameters. (A) Shows where the increase in retinal arteriolar (red) and venular (blue) diameters in response to flicker light exposure can be assessed around the optic nerve head. (B) Shows that the baseline diameter is assessed during the first 50 seconds of the measurement and that during 40 seconds of flicker light the retinal diameter increases. The flicker light-induced increase in retinal diameters is calculated from the retinal diameter at 10 and 40 seconds.
Retinal laser Doppler flowmetry
Retinal laser Doppler flowmetry can be used to quantify the velocity of retinal blood flow in the arterioles and venules (Figure 2.5) (43). Lower retinal arteriolar and venular perfusion at rest is presumed to reflect worse function of the neurovascular coupling unit (43).
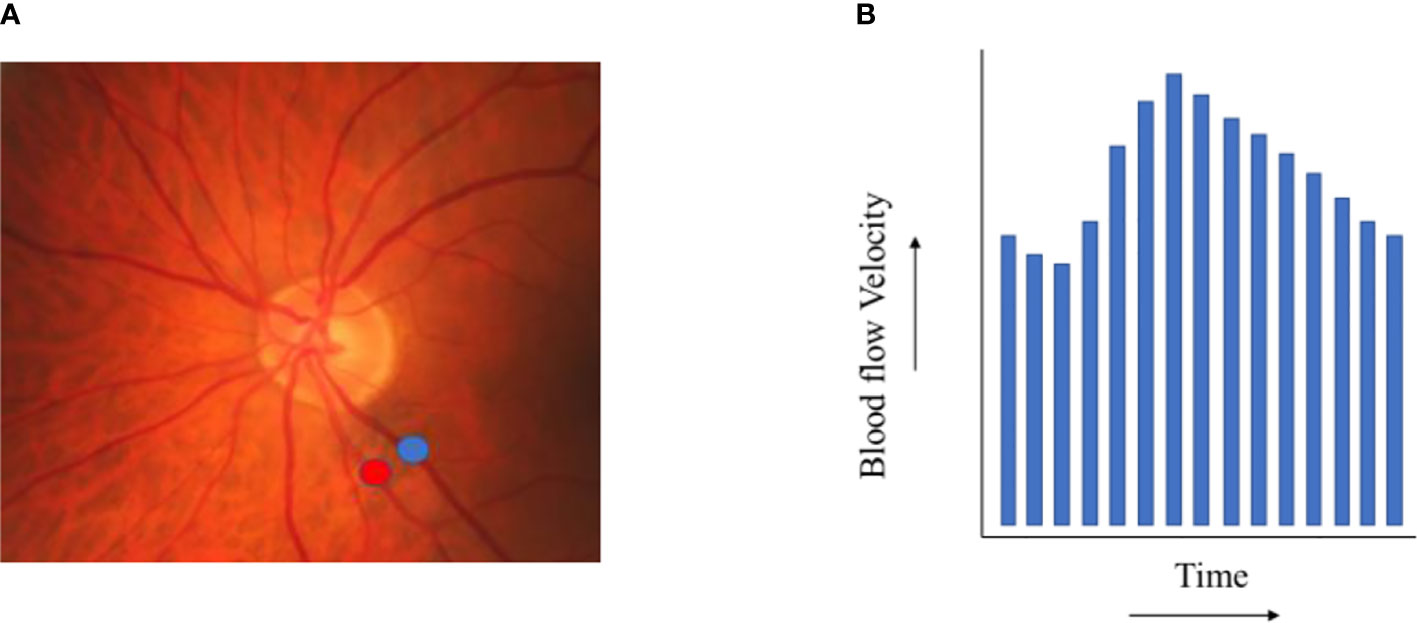
Figure 2.5 Retinal blood flow. (A) shows exemplary locations on a fundus photo where retinal arteriolar (red) and venular (blue) blood flow velocity can be assessed. (B) depicts the results of a retinal blood flow velocity measurement (during one heart beat).
OCT-A
OCT-A can be used to visualize the retinal capillaries (10) and can quantify capillary perfusion at rest based on the size of the foveal avascular zone area and vascular density (Figure 2.6). A greater foveal avascular zone area and a lower vascular density are thought to both reflect lower capillary perfusion at rest (10).
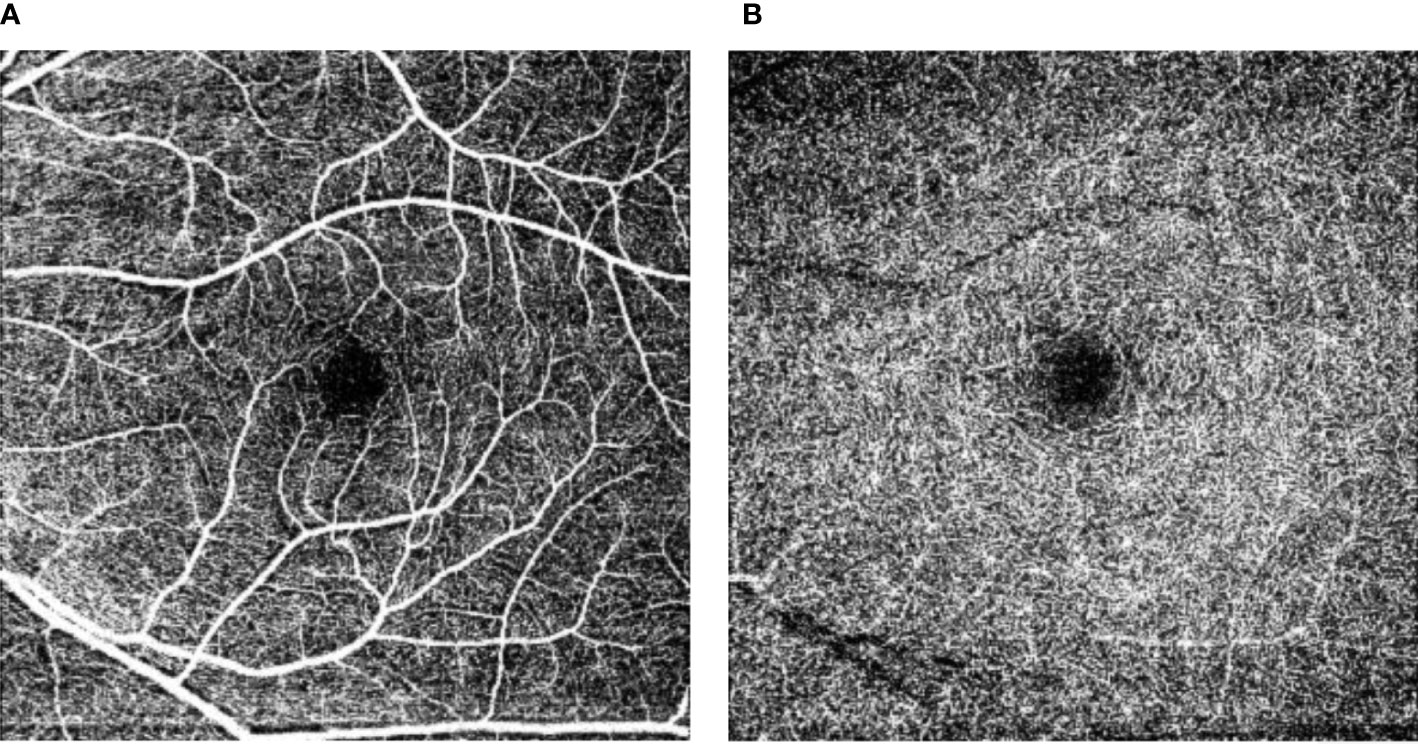
Figure 2.6 Retinal vascular density and foveal avascular zone. Figure 2.6 shows the superficial capillary networks (A) and the deep capillary networks (B) in the perifoveal area. The central black area is the perifoveal avascular zone, where a larger perifoveal avascular area reflects less capillary perfusion at rest. In addition, from these images the capillary perfusion at rest can be estimated as the “vascular density”.
Literature overview
Table 2 shows the results of our search. We identified two population-based studies that investigated the associations of RNFL thickness with cerebral MRI features of structural brain abnormalities (both cross-sectional studies [n=2,124 and n=2,131]); and five population-based studies that investigated the associations of retinal arteriolar and venular diameters with cerebral MRI features of structural brain abnormalities (all cross-sectional studies[n=1,013-n=1,211]; an overview of smaller studies is given in Supplemental Table S3). We did not identify any studies (neither population studies nor smaller clinical studies) that investigated the associations of flicker light-induced increase in retinal microvascular diameters or retinal arteriolar or venular perfusion at rest with cerebral MRI features of structural brain abnormalities. We identified five smaller clinical studies that investigated the associations of capillary perfusion at rest, estimated as vascular density or foveal avascular zone area, with cerebral MRI features of structural brain abnormalities (all cross-sectional studies; n=16-n=85; as there were no population-based data available we showed findings of these smaller studies in Table 2).
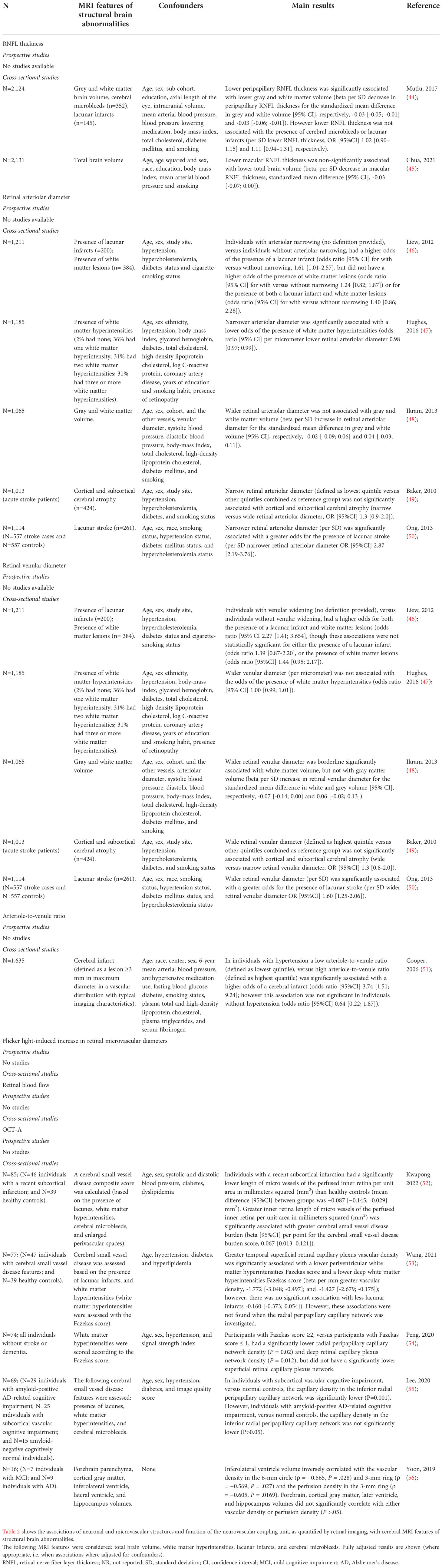
Table 2 Associations of neuronal and microvascular structures and function of the neurovascular coupling unit, as quantified by retinal imaging, with cerebral MRI features of structural brain abnormalities.
Summary of findings
Most population-based studies found that lower RNFL thickness, narrower retinal arteriolar diameters, and wider retinal venular diameters were associated with a higher prevalence of cerebral MRI features of structural brain abnormalities. In addition, most studies (all small and non-population-based) found that lower capillary perfusion at rest, as estimated from lower vascular density, was associated with a higher prevalence of cerebral MRI features of structural brain abnormalities. Next, no studies have been reported that investigated the associations of flicker light-induced increase in retinal microvascular diameters, retinal arteriolar or venular perfusion at rest, or capillary perfusion at rest, as estimated based on the size of the foveal avascular area, with cerebral MRI features of structural brain abnormalities. Overall, these findings are consistent with the concept that the retinal imaging techniques may provide an opportunity to study the early pathobiology of cognitive dysfunction.
Limitations
The present body of evidence has certain limitations. The main limitation of the available population-based data on RNFL thickness and retinal microvascular diameters is that most studies were cross-sectional in design (8). In addition, data were lacking for flicker light-induced increase in retinal microvascular diameters, retinal blood flow at rest, and capillary perfusion estimated from the size of the foveal avascular zone. Further, limitations for data on vascular density were that the sample sizes of study populations were relatively small; and that these studies did not account for a range of potential confounders (e.g. lifestyle factors).
Section 3: Summary of human evidence on the associations of neuronal and microvascular structures and function of the neurovascular coupling unit, as quantified by retinal imaging, with cognitive performance
In this section, we review the present body of evidence on the associations of neuronal and microvascular structures and function of the neurovascular coupling unit, as quantified by retinal imaging, with cognitive performance. We searched for studies that assessed cognitive performance (not limited to a specific type of cognitive function) or cognitive status (defined as with dementia, mild cognitive impairment [MCI], or control) and retinal neurodegeneration (assessed as lower retinal nerve fiber layer [RNFL] thickness); retinal microvascular dysfunction (assessed as narrower or wider retinal arterioles and wider retinal venules); or retinal dysfunction of the neurovascular coupling unit (assessed as lower increase in retinal microvascular diameters in response to flicker light exposure [i.e. during an increase in neuronal activity]; lower perfusion of arterioles and venules at rest [assessed as lower velocity]; or lower capillary perfusion at rest[assessed as greater foveal avascular zone area or lower vascular density) (10, 17). Details on the search strategy are given in the Supplemental Methods.
Literature overview
Table 3 shows the results of the literature search. We identified nine population-based studies that investigated the associations of RNFL thickness with cognitive performance (three studies had prospective data available [n=865-n=2,456] and all nine studies had cross-sectional data available [n=865-n=32,038]); and seven population-based studies that investigated the associations of retinal arteriolar and/or venular diameters with cognitive performance (one study was prospective [n=1,134] and six studies were cross-sectional [n=809-n=8,600]; an overview of smaller studies is given in the Supplemental Table S4 as these studies have previously been reviewed) (81–87). In addition, we identified three studies that investigated the association of flicker light-induced increase in retinal microvascular diameters with cognitive performance (all cross-sectional studies; one population-based study [n=2,049] and two smaller clinical studies [n=54 and n=56]; all shown in Table 3). Also, we identified two studies that investigated the association of retinal arteriolar and/or venular perfusion at rest with cognitive performance (both cross-sectional; n=52 and n=17; both studies are shown in Table 3). We did not find any population-based studies with data on the associations of foveal avascular zone area or vascular density with cognitive performance. We did, however, find a recent meta-analysis (n=541) which showed the results of eight (non-population based) studies; and, in addition, we also found fourteen (non-population based) studies that were not considered for inclusion in the meta-analysis (as these studies were published after the last search date in the meta-analysis). Table 3 shows the results of the meta-analysis as well as the results of the largest studies (studies with a study sample size N≥150) that were not included in this meta-analysis. An overview of the nine smaller studies is presented in Supplemental Table S5.

Table 3 Associations of neuronal and microvascular structures and function of the neurovascular coupling unit, as quantified by retinal imaging, with cognitive performance.
Summary of findings
Most population-based studies found that lower RNFL thickness, narrower retinal arteriolar diameters, and wider retinal venular diameters were associated with lower cognitive performance. In addition, most small studies found that lower flicker light-induced increase in retinal microvascular diameters, lower retinal arteriolar and venular perfusion at rest, lower vascular density, and greater foveal avascular zone area were associated with lower cognitive performance. These findings are consistent with the concept that retinal imaging techniques may provide an opportunity to study the early pathobiology of cognitive dysfunction.
Limitations
The present body of evidence has certain limitations. The main limitation of the available population-based data on the associations of RNFL thickness and retinal microvascular diameters with cognitive performance is that most studies were cross-sectional in design (8). In addition, population-based data were lacking for associations of flicker light-induced increase in retinal microvascular diameters, retinal arteriolar and venular perfusion at rest, and capillary perfusion at rest, estimated from vascular density and the size of the foveal avascular zone, with cognitive performance; and most studies did not account for a range of potential confounders (e.g. lifestyle factors) (8).
Discussion
This review has two main results. First, we found that there is limited data on the association between dysfunction of the neurovascular coupling unit, as quantified with cerebral imaging techniques, and cognitive dysfunction. Second, we found that neurodegeneration, microvascular dysfunction, and dysfunction of the neurovascular coupling unit, as quantified with retinal imaging techniques, are associated with a higher prevalence of MRI features of structural brain abnormalities and lower cognitive performance. Retinal imaging techniques are more easily applied at large scale as compared to cerebral imaging techniques and, thus, may provide an opportunity to study the early pathobiology of dysfunction of the neurovascular coupling unit and cognitive dysfunction. Below we discuss advantages and disadvantages of this proposal and highlight directions for future research.
Advantages of retinal imaging techniques are that these techniques are non-invasive, relatively inexpensive, highly accurate, require relatively short measurement time, and that the biological interpretation of findings is relatively clear. Indeed, neuronal and microvascular structures can be separately assessed and different levels of the microcirculation can be separately assessed (i.e. arterioles, venules, and capillaries) (10).
A potential disadvantage of studying neurovascular coupling at the level of the retina is that is it not possible to study regional differences in the brain (10). Although neurovascular dysfunction may be a generalized phenomenon in the central nervous system, possibly the pathophysiological mechanisms that underlie degeneration of the neurovascular coupling unit may differ between different brain regions (10, 42, 88). Another potential disadvantage is that no direct estimate of blood-retina barrier integrity is available, although structural indices of capillary density as estimated with OCT-A may be related to blood-retina barrier integrity (10, 42, 88). However, this requires further study (10, 42, 88).
Future research is necessary to move towards a better under understanding of dysfunction of the neurovascular coupling unit and, ultimately, the development of early therapeutic strategies (based on the early modification of adverse risk factors such as hyperglycemia) to prevent dysfunction of the neurovascular coupling unit. Future research is needed to evaluate how and to what extent neurodegeneration and microvascular dysfunction, and loss of their interaction, contribute to dysfunction of the neurovascular coupling unit (6, 7, 11–13). As presumedly a bi-directional interaction exists between neuronal and microvascular structures, individuals with both degeneration of neuronal and microvascular structures may be at an increased risk for cognitive decline and dementia. In addition, it is important that future studies quantify how potentially modifiable risk factors are related to loss or dysfunction of the individual components of the neurovascular coupling unit; and investigate whether targeted modification of these risk factors may reduce degeneration of neuronal and microvascular structures, dysfunction of the neurovascular coupling unit, and, can thereby, delay and/or prevent the onset of dementia (2). Further, studies are warranted to investigate whether retinal imaging techniques that assess deterioration of components of the neurovascular coupling unit (i.e. neuronal and microvascular structures) and function of the neurovascular coupling unit may be means for the early stage identification of individuals at risk for cognitive decline and dementia.
An important strength of the present review is that we provided a complete and extensive overview of the presently available population-based data (8). We reported data from many large studies, data which we presume to be relatively of a lower risk of selection bias than smaller clinical studies; and these studies accounted for a large number of potential confounders, which reduces the chance that the results of these studies are spuriously estimated due to residual confounding (8).
This review has certain limitations. To start, as the review reports extensively on findings in the general population, some matters have not been addressed in great detail. We did not detail: 1) findings in subgroups stratified by key characteristics (e.g. age, sex, ethnicity, or apolipoprotein E status); 2) how differences in the classification of mild cognitive impairment or dementia between studies may have affected the results; and 3) exclusion criteria used in different studies (e.g. in some, but not all, studies individuals with eye diseases such as glaucoma and diabetic retinopathy were excluded).
Next, we made certain assumptions, which should be reported as potential limitations: 1) we defined population-based studies as those with sample sizes of n=500 or more assuming this would constitute a representative sample (8); and 2) we presumed that SPECT-, ASL-MRI-, and BOLD fMRI-assessed cerebral blood flow and/or cerebrovascular reactivity reflect function of the neurovascular coupling unit. However, with regard to the latter, this assumption should be made with caution as these measures are indirect, and not direct, measures of the function of the neurovascular coupling unit. We presume that these measures reflect function of the neurovascular coupling unit because cerebral blood flow and cerebrovascular reactivity are to an important extent regulated by the neurovascular coupling unit (14).
Conclusion
This review highlights that 1) the association between dysfunction of the neurovascular coupling unit, assessed in the brain, and early stage cognitive dysfunction remains incompletely understood as population-based data are lacking; and 2) that studying the retina may provide opportunity to acquire population-based data on the association between dysfunction of the neurovascular coupling unit and early-stage cognitive dysfunction, including cerebral small vessel disease. A better understanding of the early pathobiology of cognitive dysfunction may contribute to the development of novel therapeutic strategies that aim to prevent dementia.
Author contributions
FH contributed to conception and design, participated in acquisition of data, analyzed and interpreted data, and drafted the manuscript. NW participated in acquisition of data. TS contributed to conception and design of the study and interpreted the data. All authors revised the manuscript critically for important intellectual content, and provided final approval of the version to be published. FH is the guarantor of this work and, as such, takes responsibility for the integrity of the data and the accuracy of the data analysis. All authors contributed to the article and approved the submitted version.
Funding
TS is supported by a VENI research grant (916.19.074) from The Netherlands Organization for Scientific Research (NWO) and The Netherlands Organization for Health Research and Development (ZonMw), a Dutch Heart Foundation research grant (2018T025), and a Junior Fellowship from the Dutch Diabetes Research Foundation.
Conflict of interest
The authors declare that the research was conducted in the absence of any commercial or financial relationships that could be construed as a potential conflict of interest.
Publisher’s note
All claims expressed in this article are solely those of the authors and do not necessarily represent those of their affiliated organizations, or those of the publisher, the editors and the reviewers. Any product that may be evaluated in this article, or claim that may be made by its manufacturer, is not guaranteed or endorsed by the publisher.
Supplementary material
The Supplementary Material for this article can be found online at: https://www.frontiersin.org/articles/10.3389/fendo.2022.1014287/full#supplementary-material
References
1. Collaborators GBDN. Global, regional, and national burden of neurological disorders, 1990-2016: A systematic analysis for the global burden of disease study 2016. Lancet Neurol (2019) 18:459–80. doi: 10.1016/S1474-4422(18)30499-X
2. Frisoni GB, Molinuevo JL, Altomare D, Carrera E, Barkhof F, Berkhof J, et al. Precision prevention of alzheimer's and other dementias: Anticipating future needs in the control of risk factors and implementation of disease-modifying therapies. Alzheimers Dement (2020) 16:1457–68. doi: 10.1002/alz.12132
3. Hachinski V, Einhaupl K, Ganten D, Alladi S, Brayne C, Stephan BCM, et al. Special topic section: Linkages among cerebrovascular, cardiovascular, and cognitive disorders: Preventing dementia by preventing stroke: The Berlin manifesto. Int J Stroke (2019), 1747493019871915. doi: 10.1177/1747493019871915
4. Hu C, Yu D, Sun X, Zhang M, Wang L and Qin H. The prevalence and progression of mild cognitive impairment among clinic and community populations: A systematic review and meta-analysis. Int Psychogeriatr (2017) 29:1595–608. doi: 10.1017/S1041610217000473
5. Livingston G, Sommerlad A, Orgeta V, Costafreda SG, Huntley J, Ames D, et al. Dementia prevention, intervention, and care. Lancet (2017) 390:2673–734. doi: 10.1016/S0140-6736(17)31363-6
6. Nelson AR, Sweeney MD, Sagare AP and Zlokovic BV. Neurovascular dysfunction and neurodegeneration in dementia and alzheimer's disease. Biochim Biophys Acta (2016) 1862:887–900. doi: 10.1016/j.bbadis.2015.12.016
7. Iadecola C. The neurovascular unit coming of age: A journey through neurovascular coupling in health and disease. Neuron (2017) 96:17–42. doi: 10.1016/j.neuron.2017.07.030
8. Ahlbom A. Modern epidemiology, 4th edition. TL lash, TJ VanderWeele, s haneuse, KJ rothman. wolters kluwer, 2021. Eur J Epidemiol (2021) 36:767–8. doi: 10.1007/s10654-021-00778-w
9. Chagnot A, Barnes SR and Montagne A. Magnetic resonance imaging of blood-brain barrier permeability in dementia. Neuroscience (2021) 474:14–29. doi: 10.1016/j.neuroscience.2021.08.003
10. Kashani AH, Asanad S, Chan JW, Singer MB, Zhang J, Sharifi M, et al. Past, present and future role of retinal imaging in neurodegenerative disease. Prog Retin Eye Res (2021) 83:100938. doi: 10.1016/j.preteyeres.2020.100938
11. Sweeney MD, Ayyadurai S and Zlokovic BV. Pericytes of the neurovascular unit: key functions and signaling pathways. Nat Neurosci (2016) 19:771–83. doi: 10.1038/nn.4288
12. Zhao M, Jiang XF, Zhang HQ, Sun JH, Pei H, Ma LN, et al. Interactions between glial cells and the blood-brain barrier and their role in alzheimer's disease. Ageing Res Rev (2021) 72:101483. doi: 10.1016/j.arr.2021.101483
13. Presa JL, Saravia F, Bagi Z and Filosa JA. Vasculo-neuronal coupling and neurovascular coupling at the neurovascular unit: Impact of hypertension. Front Physiol (2020) 11:584135. doi: 10.3389/fphys.2020.584135
14. Biessels GJ, Nobili F, Teunissen CE, Simo R and Scheltens P. Understanding multifactorial brain changes in type 2 diabetes: A biomarker perspective. Lancet Neurol (2020) 19:699–710. doi: 10.1016/S1474-4422(20)30139-3
15. Iturria-Medina Y, Carbonell FM, Sotero RC, Chouinard-Decorte F, Evans AC and Alzheimer's Disease Neuroimaging I. Multifactorial causal model of brain (dis)organization and therapeutic intervention: Application to alzheimer's disease. Neuroimage (2017) 152:60–77. doi: 10.1016/j.neuroimage.2017.02.058
16. Hamed SA. Brain injury with diabetes mellitus: evidence, mechanisms and treatment implications. Expert Rev Clin Pharmacol (2017) 10:409–28. doi: 10.1080/17512433.2017.1293521
17. Stehouwer CDA. Microvascular dysfunction and hyperglycemia: A vicious cycle with widespread consequences. Diabetes (2018) 67:1729–41. doi: 10.2337/dbi17-0044
18. Iadecola C, Gottesman RF. Neurovascular and cognitive dysfunction in hypertension. Circ Res (2019) 124:1025–44. doi: 10.1161/CIRCRESAHA.118.313260
19. Castillo X, Castro-Obregon S, Gutierrez-Becker B, Gutierrez-Ospina G, Karalis N, Khalil AA, et al. Re-thinking the etiological framework of neurodegeneration. Front Neurosci (2019) 13:728. doi: 10.3389/fnins.2019.00728
20. Alegret M, Cuberas-Borros G, Vinyes-Junque G, Espinosa A, Valero S, Hernandez I, et al. A two-year follow-up of cognitive deficits and brain perfusion in mild cognitive impairment and mild alzheimer's disease. J Alzheimers Dis (2012) 30:109–20. doi: 10.3233/JAD-2012-111850
21. Peng SL, Chen X, Li Y, Rodrigue KM, Park DC and Lu H. Age-related changes in cerebrovascular reactivity and their relationship to cognition: A four-year longitudinal study. Neuroimage (2018) 174:257–62. doi: 10.1016/j.neuroimage.2018.03.033
22. De Vis JB, Peng SL, Chen X, Li Y, Liu P, Sur S, et al. Arterial-spin-labeling (ASL) perfusion MRI predicts cognitive function in elderly individuals: A 4-year longitudinal study. J Magn Reson Imaging (2018) 48:449–58. doi: 10.1002/jmri.25938
23. Andre-Petersson L, Thorsson O, Siennicki-Lantz A. Cognitive abnormalities and cerebral perfusion defects in a community-dwelling cohort of elderly men with MMSE within the normal range. Neuropsychol Dev Cognit B Aging Neuropsychol Cognit (2018) 25:200–12. doi: 10.1080/13825585.2016.1277970
24. Baker JG, Williams AJ, Wack DS and Miletich RS. Correlation of cognition and SPECT perfusion: easy z score and SPM analysis of a pilot sample with cerebral small vessel disease. Dement Geriatr Cognit Disord (2013) 36:290–9. doi: 10.1159/000339587
25. Kim D, Hughes TM, Lipford ME, Craft S, Baker LD, Lockhart SN, et al. Relationship between cerebrovascular reactivity and cognition among people with risk of cognitive decline. Front Physiol (2021) 12:645342. doi: 10.3389/fphys.2021.645342
26. Ni L, Zhang B, Yang D, Qin R, Xu H, Ma J, et al. Lower cerebrovascular reactivity contributed to white matter hyperintensity-related cognitive impairment: A resting-state functional MRI study. J Magn Reson Imaging (2021) 53:703–11. doi: 10.1002/jmri.27376
27. Catchlove SJ, Parrish TB, Chen Y, Macpherson H, Hughes ME and Pipingas A. Regional cerebrovascular reactivity and cognitive performance in healthy aging. J Exp Neurosci (2018) 12:1179069518785151. doi: 10.1177/1179069518785151
28. Osuna JR, Weigand AJ, Edmonds EC, Clark AL, Holmqvist S, Cota IH, et al. Regional hyperperfusion in older adults with objectively-defined subtle cognitive decline. J Cereb Blood Flow Metab (2021) 41:1001–12. doi: 10.1177/0271678X20935171
29. Yew B, Nation DA and Alzheimer's Disease Neuroimaging I. Cerebrovascular resistance: Effects on cognitive decline, cortical atrophy, and progression to dementia. Brain (2017) 140:1987–2001. doi: 10.1093/brain/awx112
30. Mattsson N, Tosun D, Insel PS, Simonson A, Jack CR Jr., Beckett LA, et al. Association of brain amyloid-beta with cerebral perfusion and structure in alzheimer's disease and mild cognitive impairment. Brain (2014) 137:1550–61. doi: 10.1093/brain/awu043
31. Binnewijzend MA, Kuijer JP, Benedictus MR, van der Flier WM, Wink AM, Wattjes MP, et al. Cerebral blood flow measured with 3D pseudocontinuous arterial spin-labeling MR imaging in Alzheimer disease and mild cognitive impairment: a marker for disease severity. Radiology (2013) 267:221–30. doi: 10.1148/radiol.12120928
32. Kerkhofs D, Wong SM, Zhang E, Uiterwijk R, Hoff EI, Jansen JFA, et al. Blood-brain barrier leakage at baseline and cognitive decline in cerebral small vessel disease: a 2-year follow-up study. Geroscience (2021) 43:1643–52. doi: 10.1007/s11357-021-00399-x
33. Verheggen ICM, de Jong JJA, van Boxtel MPJ, Postma AA, Jansen JFA, Verhey FRJ and Backes WH. Imaging the role of blood-brain barrier disruption in normal cognitive ageing. Geroscience (2020) 42:1751–64. doi: 10.1007/s11357-020-00282-1
34. Bowman GL, Dayon L, Kirkland R, Wojcik J, Peyratout G, Severin IC, et al. Blood-brain barrier breakdown, neuroinflammation, and cognitive decline in older adults. Alzheimers Dement (2018) 14:1640–50. doi: 10.1016/j.jalz.2018.06.2857
35. Freeze WM, Jacobs HIL, de Jong JJ, Verheggen ICM, Gronenschild E, Palm WM, et al. White matter hyperintensities mediate the association between blood-brain barrier leakage and information processing speed. Neurobiol Aging (2020) 85:113–22. doi: 10.1016/j.neurobiolaging.2019.09.017
36. Li M, Li Y, Zuo L, Hu W and Jiang T. Increase of blood-brain barrier leakage is related to cognitive decline in vascular mild cognitive impairment. BMC Neurol (2021) 21:159. doi: 10.1186/s12883-021-02189-6
37. Moon Y, Lim C, Kim Y and Moon WJ. Sex-related differences in regional blood-brain barrier integrity in non-demented elderly subjects. Int J Mol Sci (2021) 22:2860. doi: 10.3390/ijms22062860
38. Li Y, Li M, Zhang X, Shi Q, Yang S, Fan H, et al. Higher blood-brain barrier permeability is associated with higher white matter hyperintensities burden. J Neurol (2017) 264:1474–81. doi: 10.1007/s00415-017-8550-8
39. Montagne A, Barnes SR, Sweeney MD, Halliday MR, Sagare AP, Zhao Z, et al. Blood-brain barrier breakdown in the aging human hippocampus. Neuron (2015) 85:296–302. doi: 10.1016/j.neuron.2014.12.032
40. Taheri S, Gasparovic C, Huisa BN, Adair JC, Edmonds E, Prestopnik J, et al. Blood-brain barrier permeability abnormalities in vascular cognitive impairment. Stroke (2011) 42:2158–63. doi: 10.1161/STROKEAHA.110.611731
41. Aumann S, Donner S, Fischer J and Muller F. Optical coherence tomography (OCT): Principle and technical realization. In: Bille JF, editor. High resolution imaging in microscopy and ophthalmology. Cham (CH: New Frontiers in Biomedical Optics (2019). p. 59–85.
42. Czako C, Kovacs T, Ungvari Z, Csiszar A, Yabluchanskiy A, Conley S, et al. Retinal biomarkers for alzheimer's disease and vascular cognitive impairment and dementia (VCID): Implication for early diagnosis and prognosis. Geroscience (2020) 42:1499–525. doi: 10.1007/s11357-020-00252-7
43. Pournaras CJ, Riva CE. Retinal blood flow evaluation. Ophthalmologica (2013) 229:61–74. doi: 10.1159/000338186
44. Mutlu U, Bonnemaijer PWM, Ikram MA, Colijn JM, Cremers LGM, Buitendijk GHS, et al. Retinal neurodegeneration and brain MRI markers: the Rotterdam study. Neurobiol Aging (2017) 60:183–91. doi: 10.1016/j.neurobiolaging.2017.09.003
45. Chua SYL, Lascaratos G, Atan D, Zhang B, Reisman C, Khaw PT, et al. Relationships between retinal layer thickness and brain volumes in the UK biobank cohort. Eur J Neurol (2021) 28:1490–8. doi: 10.1111/ene.14706
46. Liew G, Baker ML, Wong TY, Hand PJ, Wang JJ, Mitchell P, et al. Differing associations of white matter lesions and lacunar infarction with retinal microvascular signs. Int J Stroke (2014) 9:921–5. doi: 10.1111/j.1747-4949.2012.00865.x
47. Hughes AD, Falaschetti E, Witt N, Wijetunge S, Thom SA, Tillin T, et al. Association of retinopathy and retinal microvascular abnormalities with stroke and cerebrovascular disease. Stroke (2016) 47:2862–4. doi: 10.1161/STROKEAHA.116.014998
48. Ikram MK, de Jong FJ, Vernooij MW, Hofman A, Niessen WJ, van der Lugt A, et al. Retinal vascular calibers associate differentially with cerebral gray matter and white matter atrophy. Alzheimer Dis Assoc Disord (2013) 27:351–5. doi: 10.1097/WAD.0b013e31829344ed
49. Baker ML, Wang JJ, Liew G, Hand PJ, De Silva DA, Lindley RI, et al. Differential associations of cortical and subcortical cerebral atrophy with retinal vascular signs in patients with acute stroke. Stroke (2010) 41:2143–50. doi: 10.1161/STROKEAHA.110.594317
50. Ong YT, De Silva DA, Cheung CY, Chang HM, Chen CP, Wong MC, et al. Microvascular structure and network in the retina of patients with ischemic stroke. Stroke (2013) 44:2121–7. doi: 10.1161/STROKEAHA.113.001741
51. Cooper LS, Wong TY, Klein R, Sharrett AR, Bryan RN, Hubbard LD, et al. Retinal microvascular abnormalities and MRI-defined subclinical cerebral infarction: the atherosclerosis risk in communities study. Stroke (2006) 37:82–6. doi: 10.1161/01.STR.0000195134.04355.e5
52. Kwapong WR, Jiang S, Yan Y, Wan J and Wu B. Macular microvasculature is associated with total cerebral small vessel disease burden in recent single subcortical infarction. Front Aging Neurosci (2021) 13:787775. doi: 10.3389/fnagi.2021.787775
53. Wang X, Wei Q, Wu X, Cao S, Chen C, Zhang J, et al. The vessel density of the superficial retinal capillary plexus as a new biomarker in cerebral small vessel disease: an optical coherence tomography angiography study. Neurol Sci (2021) 42:3615–24. doi: 10.1007/s10072-021-05038-z
54. Peng C, Kwapong WR, Xu S, Muse FM, Yan J, Qu M, et al. Structural and microvascular changes in the macular are associated with severity of white matter lesions. Front Neurol (2020) 11:521. doi: 10.3389/fneur.2020.00521
55. Lee JY, Kim JP, Jang H, Kim J, Kang SH, Kim JS, et al. Optical coherence tomography angiography as a potential screening tool for cerebral small vessel diseases. Alzheimers Res Ther (2020) 12:73. doi: 10.1186/s13195-020-00638-x
56. Yoon SP, Thompson AC, Polascik BW, Calixte C, Burke JR, Petrella JR, et al. Correlation of OCTA and volumetric MRI in mild cognitive impairment and alzheimer's disease. Ophthalmic Surg Lasers Imaging Retina (2019) 50:709–18. doi: 10.3928/23258160-20191031-06
57. Ko F, Muthy ZA, Gallacher J, Sudlow C, Rees G, Yang Q, et al. Association of retinal nerve fiber layer thinning with current and future cognitive decline: A study using optical coherence tomography. JAMA Neurol (2018) 75:1198–205. doi: 10.1001/jamaneurol.2018.1578
58. Mutlu U, Colijn JM, Ikram MA, Bonnemaijer PWM, Licher S, Wolters FJ, et al. Association of retinal neurodegeneration on optical coherence tomography with dementia: A population based study. JAMA Neurol (2018) 75:1256–63. doi: 10.1001/jamaneurol.2018.1563
59. Barrett-Young A, Ambler A, Cheyne K, Guiney H, Kokaua J, Steptoe B, et al. Associations between retinal nerve fiber layer and ganglion cell layer in middle age and cognition from childhood to adulthood. JAMA Ophthalmol (2022) 140:262–268. doi: 10.1001/jamaophthalmol.2021.6082
60. Girbardt JLT, Kynast J, Rodriguez FS, Wicklein B, Wirkner K, Engel C, et al. Reading cognition from the eyes: Association of retinal nerve fibre layer thickness with cognitive performance in a population-based study. Brain Commun (2021) 3. doi: 10.1093/braincomms/fcab258
61. Khawaja AP, Chan MP, Yip JL, Broadway DC, Garway-Heath DF, Luben R, et al. Retinal nerve fiber layer measures and cognitive function in the EPIC-Norfolk cohort study. Invest Ophthalmol Vis Sci (2016) 57:1921–6. doi: 10.1167/iovs.16-19067
62. Ward DD, Mauschitz MM, Bonniger MM, Merten N, Finger RP and Breteler MMB. Association of retinal layer measurements and adult cognitive function: A population-based study. Neurology (2020) 95:e1144–52. doi: 10.1212/WNL.0000000000010146
63. Jones-Odeh E, Yonova-Doing E, Bloch E, Williams KM, Steves CJ and Hammond CJ. The correlation between cognitive performance and retinal nerve fibre layer thickness is largely explained by genetic factors. Sci Rep (2016) 6:34116. doi: 10.1038/srep34116
64. van Koolwijk LM, Despriet DD, Van Duijn CM, Oostra BA, van Swieten JC, de Koning I, et al. Association of cognitive functioning with retinal nerve fiber layer thickness. Invest Ophthalmol Vis Sci (2009) 50:4576–80. doi: 10.1167/iovs.08-3181
65. Sanchez D, Castilla-Marti M, Rodriguez-Gomez O, Valero S, Piferrer A, Martinez G, et al. Usefulness of peripapillary nerve fiber layer thickness assessed by optical coherence tomography as a biomarker for alzheimer's disease. Sci Rep (2018) 8:16345. doi: 10.1038/s41598-018-34577-3
66. Lesage SR, Mosley TH, Wong TY, Szklo M, Knopman D, Catellier DJ, et al. Retinal microvascular abnormalities and cognitive decline: the ARIC 14-year follow-up study. Neurology (2009) 73:862–8. doi: 10.1212/WNL.0b013e3181b78436
67. Liew G, Mitchell P, Wong TY, Lindley RI, Cheung N, Kaushik S and Wang JJ. Retinal microvascular signs and cognitive impairment. J Am Geriatr Soc (2009) 57:1892–6. doi: 10.1111/j.1532-5415.2009.02459.x
68. Kim DH, Newman AB, Hajjar I, Strotmeyer ES, Klein R, Newton E, et al. Retinal microvascular signs and functional loss in older persons: the cardiovascular health study. Stroke (2011) 42:1589–95. doi: 10.1161/STROKEAHA.110.605261
69. Wong TY, Klein R, Sharrett AR, Nieto FJ, Boland LL, Couper DJ, et al. Retinal microvascular abnormalities and cognitive impairment in middle-aged persons: the atherosclerosis risk in communities study. Stroke (2002) 33:1487–92. doi: 10.1161/01.STR.0000016789.56668.43
70. Baker ML, Marino Larsen EK, Kuller LH, Klein R, Klein BE, Siscovick DS, et al. Retinal microvascular signs, cognitive function, and dementia in older persons: the cardiovascular health study. Stroke (2007) 38:2041–7. doi: 10.1161/STROKEAHA.107.483586
71. Ding J, Strachan MW, Fowkes FG, Wong TY, Macgillivray TJ, Patton N, et al. Association of retinal arteriolar dilatation with lower verbal memory: the Edinburgh type 2 diabetes study. Diabetologia (2011) 54:1653–62. doi: 10.1007/s00125-011-2129-1
72. Gatto NM, Varma R, Torres M, Wong TY, Johnson PL, Segal-Gidan F and Mack WJ. Retinal microvascular abnormalities and cognitive function in Latino adults in Los Angeles. Ophthalmic Epidemiol (2012) 19:127–36. doi: 10.3109/09286586.2011.615452
73. Rensma SP, van Sloten TT, Houben A, Kohler S, van Boxtel MPJ, Berendschot T, et al. Microvascular dysfunction is associated with worse cognitive performance: The maastricht study. Hypertension (2020) 75:237–45. doi: 10.1161/HYPERTENSIONAHA.119.13023
74. Querques G, Borrelli E, Sacconi R, De Vitis L, Leocani L, Santangelo R, et al. Functional and morphological changes of the retinal vessels in alzheimer's disease and mild cognitive impairment. Sci Rep (2019) 9:63. doi: 10.1038/s41598-018-37271-6
75. Kotliar K, Hauser C, Ortner M, Muggenthaler C, Diehl-Schmid J, Angermann S, et al. Altered neurovascular coupling as measured by optical imaging: a biomarker for alzheimer's disease. Sci Rep (2017) 7:12906. doi: 10.1038/s41598-017-13349-5
76. Feke GT, Hyman BT, Stern RA and Pasquale LR. Retinal blood flow in mild cognitive impairment and alzheimer's disease. Alzheimers Dement (Amst) (2015) 1:144–51. doi: 10.1016/j.dadm.2015.01.004
77. Berisha F, Feke GT, Trempe CL, McMeel JW and Schepens CL. Retinal abnormalities in early alzheimer's disease. Invest Ophthalmol Vis Sci (2007) 48:2285–9. doi: 10.1167/iovs.06-1029
78. Abraham AG, Guo X, Arsiwala LT, Dong Y, Sharrett AR, Huang D, et al. Cognitive decline in older adults: What can we learn from optical coherence tomography (OCT)-based retinal vascular imaging? J Am Geriatr Soc (2021) 69:2524–35. doi: 10.1111/jgs.17272
79. Hui J, Zhao Y, Yu S, Liu J, Chiu K and Wang Y, e0255362. Detection of retinal changes with optical coherence tomography angiography in mild cognitive impairment and Alzheimer’s disease patients: A meta-analysis. PloS One (2021) 16(8):e0255362. doi: 10.1371/journal.pone.0255362
80. Peng SY, Wu IW, Sun CC, Lee CC, Liu CF, Lin YZ and Yeung L. Investigation of possible correlation between retinal neurovascular biomarkers and early cognitive impairment in patients with chronic kidney disease. Transl Vis Sci Technol (2021) 10:9. doi: 10.1167/tvst.10.14.9
81. Wu H, Wang C, Chen C, Xu X, Zhu Y, Sang A, et al. Association between retinal vascular geometric changes and cognitive impairment: A systematic review and meta-analysis. J Clin Neurol (2020) 16:19–28. doi: 10.3988/jcn.2020.16.1.19
82. Heringa SM, Bouvy WH, van den Berg E, Moll AC, Kappelle LJ and Biessels GJ. Associations between retinal microvascular changes and dementia, cognitive functioning, and brain imaging abnormalities: a systematic review. J Cereb Blood Flow Metab (2013) 33:983–95. doi: 10.1038/jcbfm.2013.58
83. Noah AM, Almghairbi D and Moppett IK. Optical coherence tomography in mild cognitive impairment - systematic review and meta-analysis. Clin Neurol Neurosurg (2020) 196:106036. doi: 10.1016/j.clineuro.2020.106036
84. Mejia-Vergara AJ, Restrepo-Jimenez P, Pelak VS. Optical coherence tomography in mild cognitive impairment: A systematic review and meta-analysis. Front Neurol (2020) 11:578698. doi: 10.3389/fneur.2020.578698
85. Cheung CY, Chan VTT, Mok VC, Chen C and Wong TY. Potential retinal biomarkers for dementia: what is new? Curr Opin Neurol (2019) 32:82–91. doi: 10.1097/WCO.0000000000000645
86. Knoll B, Simonett J, Volpe NJ, Farsiu S, Ward M, Rademaker A, et al. Retinal nerve fiber layer thickness in amnestic mild cognitive impairment: Case-control study and meta-analysis. Alzheimers Dement (Amst) (2016) 4:85–93. doi: 10.1016/j.dadm.2016.07.004
87. den Haan J, Verbraak FD, Visser PJ and Bouwman FH. Retinal thickness in alzheimer's disease: A systematic review and meta-analysis. Alzheimers Dement (Amst) (2017) 6:162–70. doi: 10.1016/j.dadm.2016.12.014
Keywords: Dementia, mild cognitive impairment, epidemiology, retinal imaging, optical coherence tomography, blood-brain barrier, neurovascular coupling unit, magnetic resonance imaging
Citation: van der Heide FCT, van Sloten TT, Willekens N and Stehouwer CDA (2022) Neurovascular coupling unit dysfunction and dementia: Retinal measurements as tools to move towards population-based evidence. Front. Endocrinol. 13:1014287. doi: 10.3389/fendo.2022.1014287
Received: 08 August 2022; Accepted: 31 October 2022;
Published: 23 November 2022.
Edited by:
Tao Zhou, Sun Yat-sen University, ChinaReviewed by:
Deborah Gustafson, Downstate Health Sciences University, United StatesPeter Mikael Nilsson, Lund University, Sweden
Copyright © 2022 van der Heide, van Sloten, Willekens and Stehouwer. This is an open-access article distributed under the terms of the Creative Commons Attribution License (CC BY). The use, distribution or reproduction in other forums is permitted, provided the original author(s) and the copyright owner(s) are credited and that the original publication in this journal is cited, in accordance with accepted academic practice. No use, distribution or reproduction is permitted which does not comply with these terms.
*Correspondence: Frank C. T. van der Heide, ZnJhbmsudmFuZGVyLmhlaWRlQG11bWMubmw=