- 1Department of Biostatistics and Epidemiology, Rutgers University, Rutgers School of Public Health, Piscataway, NJ, United States
- 2Environmental and Occupational Health Sciences Institute, Rutgers University, Piscataway, NJ, United States
- 3Department of Environmental and Occupational Health Sciences, University of Washington, Seattle, WA, United States
- 4Robert Wood Johnson Medical School, Rutgers University, Piscataway, NJ, United States
- 5Department of Pediatrics and Department of Environmental Medicine, New York University School of Medicine, New York, NY, United States
- 6Department of Gynecology and Obstetrics, Department of Psychiatry and Behavioral Sciences, Emory University School of Medicine, Atlanta, GA, United States
- 7Hunter Medical Research Institute, University of Newcastle, Newcastle, NSW, Australia
- 8Department of Preventive Medicine, University of Tennessee Health Science Center, Memphis, TN, United States
- 9Department of Psychiatry and Behavioral Sciences, University of California San Francisco, San Francisco, CA, United States
- 10Seattle Children’s Research Institute, University of Washington, Seattle, WA, United States
- 11Department of Epidemiology, University of Washington, Seattle, WA, United States
- 12Department of Pediatrics, University of Washington, Seattle, WA, United States
- 13Department of Pediatrics, Division of Developmental Medicine, University of California San Francisco, San Francisco, CA, United States
Polycyclic aromatic hydrocarbons (PAHs) are ubiquitous endocrine-disrupting combustion by-products that have been linked to preterm birth. One possible mechanism is through disruption of placental corticotropin releasing hormone (pCRH), a key hormone implicated in parturition. As an extension of recent research identifying pCRH as a potential target of endocrine disruption, we examined maternal PAH exposure in relation to pCRH in a large, diverse sample. Participants, drawn from the CANDLE cohort, part of the ECHO-PATHWAYS Consortium, completed study visits at 16-29 weeks (V1) and 22-39 weeks (V2) gestation (n=812). Seven urinary mono-hydroxylated PAH metabolites (OH-PAHs) were measured at V1 and serum pCRH at V1 and V2. Associations between individual log-transformed OH-PAHs (as well as two summed PAH measures) and log(pCRH) concentrations across visits were estimated using mixed effects models. Minimally-adjusted models included gestational age and urinary specific gravity, while fully-adjusted models also included sociodemographic characteristics. We additionally evaluated effect modification by pregnancy complications, fetal sex, and maternal childhood trauma history. We observed associations between 2-OH-Phenanthrene (2-OH-PHEN) and rate of pCRH change that persisted in fully adjusted models (β=0.0009, 0.00006, 0.0017), however, positive associations with other metabolites (most notably 3-OH-Phenanthrene and 1-Hydroxypyrene) were attenuated after adjustment for sociodemographic characteristics. Associations tended to be stronger at V1 compared to V2 and we observed no evidence of effect modification by pregnancy complications, fetal sex, or maternal childhood trauma history. In conclusion, we observed modest evidence of association between OH-PAHs, most notably 2-OH-PHEN, and pCRH in this sample. Additional research using serial measures of PAH exposure is warranted, as is investigation of alternative mechanisms that may link PAHs and timing of birth, such as inflammatory, epigenetic, or oxidative stress pathways.
Introduction
Polycyclic aromatic hydrocarbons (PAHs) are common environmental pollutants that occur due to incomplete combustion of organic matter (1). PAH exposure occurs through ambient air pollution and tobacco smoke as well as through food sources and occupational hazards, resulting in nearly ubiquitous exposure around the world (2–4). Even among non-smokers, PAH exposure is widespread, with recent research suggesting 96% of non-smoking Americans have detectable levels of one or more urinary PAH metabolites (5). Evidence of PAHs’ carcinogenic, teratogenic, and mutagenic properties (6, 7) has led to their designation as priority pollutants by the United State EPA and the European Commission (8, 9). In addition, research increasingly demonstrates their endocrine disrupting properties, with in vitro and animal model evidence indicating impacts on estrogen (10–12), thyroid (13–15), and progesterone pathways (16, 17).
The widespread PAH exposure documented in pregnant people is of particular concern given fetal vulnerability to environmental contaminants, including endocrine disruptors (18). Recent work from our group examined urinary hydroxylated PAH metabolites (OH-PAHs), a common biomarker of PAH exposure, reporting that higher second trimester urinary 2-hydroxynaphthalene (2-OH-NAP) was associated with earlier gestational age at birth and higher 1-hydroxypyrene (1-OH-PYR) with increased odds of spontaneous preterm birth among participants carrying female infants (19). Several studies examining alternative measures of PAH exposure, such PAH-DNA adducts and air monitoring, have similarly reported associations with shorter gestation (20) and increased risk of preterm birth (21, 22). Beyond potential impacts on timing of birth, the detection of PAH biomarkers in cord blood at delivery (23) and spontaneously aborted fetal tissue (24), demonstrates that they can cross the placental barrier to reach the developing fetus, and some evidence suggests associations with adverse downstream child health outcomes, including cognition and behavior (25–27), growth and pubertal development (28, 29), and asthma and allergic outcomes (30–32).
While prenatal exposures to PAHs (and environmental chemicals more generally) are often presumed to impact child development through direct effects on the fetus’ developing tissues, it is also possible that indirect effects may occur through alterations in placental development and physiology, with downstream effects on fetal/child health and development (33). Supporting this premise is prior research showing that PAHs are present in measurable levels in the placenta (23, 34) and in experimental models, PAH exposure reduces trophoblast cell function and viability (35–37).
Given the placenta’s role as a primary endocrine organ during pregnancy, the impact of PAH exposure on placental hormone production merits consideration. Of particular relevance is placental corticotropin releasing hormone (pCRH), which rises exponentially across pregnancy [reviewed in 38]. Mid-late pregnancy pCRH and the rise in pCRH across pregnancy have been linked to preterm birth (39–42), pregnancy complications (43, 44), postpartum depression (45, 46), and offspring development (47, 48). Although the same molecule is also produced by the hypothalamus as part of the hypothalamic-pituitary-adrenal (HPA) axis (49), given that CRH levels are 10,000 times higher in pregnant versus non-pregnant individuals, virtually all detectable CRH in maternal circulation is of placental origin (pCRH). Surprisingly, this important hormone has received little attention in the context of chemical exposures in pregnancy, although several recent studies have shown alterations in pCRH in relation to prenatal maternal exposures to endocrine-disrupting phthalates (50, 51) and per- and poly-fluoroalkyl substances (52).
To our knowledge, to date, only one epidemiological study has examined associations between prenatal OH-PAH exposures and placental hormones, including pCRH (53). In that study of 707 pregnant participants from the PROTECT cohort in Puerto Rico, interquartile (IQR) increases in OH-PAHs in mid-late pregnancy were associated with 14-24% increases in pCRH, with some evidence indicating stronger associations among pregnant people carrying male fetuses.
In light of epidemiological evidence that PAH exposures are associated with shorter gestation as well as results from the PROTECT study indicating direct associations between OH-PAHs and pCRH concentrations, here we extend the current, limited literature on this topic by examining maternal urinary OH-PAHs in relation to pCRH concentrations in mid- and late-pregnancy. We do so within the context of the Conditions Affecting Neurocognitive Development and Learning in Early Childhood (CANDLE) study, a socioeconomically and racially diverse, well-characterized pregnancy cohort located in the Southeastern United States.
Materials and methods
Study population and overview
From 2006-2011, pregnant participants were recruited into the CANDLE study through participating prenatal clinics in Shelby County, Tennessee, USA (54). Eligible participants had low medical-risk pregnancies at enrollment, were carrying a singleton fetus, 16-29 weeks pregnant, and 16-40 years old, with plans to deliver at a participating hospital. Low-medical risk was assessed as lacking major medical conditions at the time of consent, including (but not limited to) insulin-dependent diabetes and other endocrine disorders as well as chronic hypertension. Two study visits were conducted, roughly corresponding to mid- (Visit 1 [V1]; 16-29 weeks) and late (Visit 2 [V2]; 22-39 weeks) pregnancy, allowing for flexibility of timing to coordinate with clinical care and minimize participant burden. Prior to any study activities, Institutional Review Board approvals were obtained from the University of Tennessee Health Sciences Center (primary data collection site) and participants signed written, informed consent. The current analysis was facilitated by the NIH’s Environmental Influences of Child Health Outcomes (ECHO) program and in particular, the ECHO-PATHWAYS Consortium.
Inclusion in the current analysis was determined based on having data on urinary OH-PAH concentrations (measured at V1) as well as plasma pCRH concentrations (measured at V1 and V2). Given the strong impact of active smoking on PAH exposures as well as pregnancy physiology, in our main analyses, we excluded women who self-reported smoking during pregnancy and/or had urinary cotinine levels exceeding 200 ng/mL (55).
Exposure assessment: Maternal urinary PAH metabolites
At V1 in mid-pregnancy, participants provided a spot urine sample. Specific gravity (SG) was measured with a handheld refractometer after which samples were aliquoted and frozen at -80°C until shipment on dry ice to the Wadsworth Laboratory, New York State Department of Health, Albany, New York. Using methods previously described elsewhere (56), twelve OH-PAH metabolites were measured including: two metabolites of naphthalene (1-OH-NAP, 2-OH-NAP), four metabolites of phenanthrene (2-hydroxyphenanthrene [2-OH-PHEN], 3-hydroxyphenanthrene [3-OH-PHEN], 4-hydroxyphenanthrene [4-phen], combined 1/9-hydroxyphenanthrene [1/9-OH-PHEN]), combined 2/3/9-hydroxyfluorene (2/3/9-OH-FLUO), 1-OH-PYR, 3-hydroxybenzo[c]phenanthrene (3-BCP), two metabolites of hydroxychrysene (1-hydroxychrysene [1-OH-CHRY], 6-hydroxychrysene [6-OH-CHRY]), and 1-hydroxybenz[a]anthracene (1-OH-BAA). Briefly, 10 ng of isotopically-labeled internal standard mixture was added to 500 µL urine samples, and then combined with 1 mL of 0.5 M ammonium acetate buffer containing 200 units/mL of β-glucuronidase/sulfatase enzyme (MP Biomedicals, LLC, Solon, OH, USA). Following an overnight incubation, the sample mixtures were diluted with 2 mL of HPLC-grade water and extracted with a 7 mL pentane:toluene solution for one hour. Samples were centrifuged for 20 minutes at 3600 x g and the resulting supernatant was transferred for instrumental analysis. A Waters Acquity I-Class UPLC system (Waters; Milford, MA, USA) connected with an Eclipse Plus C18 RRHD column (100 mm × 2.1 mm, 1.8 μm, Agilent; Santa Clara, CA, USA) was used for chromatographic separation of the OH-PAH metabolites followed by quantification using an ABSCIEX 5500 triple quadrupole mass spectrometer (Applied Biosystems; Foster City, CA, USA). Two Standard Reference Materials (SRM 3672, SRM 3673) were used as quality assurance protocols and analyte recovery in the SRMs was 79-109%. To ensure instrument stability, during the sample run, calibration standards were periodically injected. The limits of detection varied by metabolite ranging from 0.02-0.12 ng/mL We limited the current analyses to seven PAH metabolites above the LOD in >60% of samples, specifically: 1-OH-NAP, 2-OH-NAP, 2-OH-PHEN, 3-OH-PHEN, 1/9-OH-PHEN, 2/3/9-OH-FLUO, and 1-OH-PYR. For these metabolites, values below the LOD were replaced with LOD/√2. We additionally calculated ∑NAP as the sum of 1-OH-NAP and 2-OH-NAP, and ∑PHEN as the sum of 2-OH-PHEN, 3-OH-PHEN, 4-OH-PHEN, and 1/9-OH-PHEN.
Outcome assessment: pCRH
At V1 and V2, participants provided blood samples in EDTA plasma separator tubes. Blood was processed and frozen at -80°CC until it was shipped on dry ice to University of Newcastle, Australia for pCRH analysis. pCRH was measured in pg/mL using radioimmunoassay according to previously published protocols (57). The inter- and intra-assay coefficients of variation were 8.7% and 7.3% respectively. pCRH concentrations were non-normally distributed and were therefore log-transformed for subsequent analysis.
Covariates
Data on key covariates, selected a priori based on the literature, were collected from questionnaires administered during pregnancy as well as from clinical chart reviews. Participants reported on age, highest level of educational attainment (categorized for this analysis as less than high school, high school/GED/technical school, or college or higher), parity (parous/nulliparous), and pre-pregnancy weight and height (used to calculate body mass index in kg/m2). Participants also reported race and ethnicity, which were included here (categorized as non-Hispanic White, non-Hispanic Black, Hispanic, or Other) as proxies for chronic exposure to discrimination and systemic racism, which may contribute to variation in exposures as well as alter endocrine activity in pregnancy. Relevant to our focus on pCRH, participants additionally reported on history of childhood exposures to traumatic stressors via three items from the Traumatic Life Events Questionnaire (TLEQ) specifically querying history of physical abuse and family violence before age 18 and sexual abuse prior to age 13. These items were used to construct a counts (discrete) variable for total number of types of childhood traumatic exposures, ranging from 0-3 (58, 59). Gestational age at sample collection was determined based on the medical record. In general, clinical determination was based on date of last menstrual period and confirmed with ultrasound dating, with the latter being prioritized in cases of discrepancies. Maternal gestational diabetes and hypertension were self-reported shortly after delivery, then confirmed by medical record abstraction and are included in the current analysis based on prior work indicating associations with pCRH in this cohort (51, 60). Finally, although participants who reported actively smoking or had cotinine levels >200 ng/mL were excluded from our primary analyses, exposure to environmental tobacco smoke was assessed by measurement of cotinine in spot urine samples collected at V1 (considered continuously in models).
Statistical analysis
Participants who: (1) had OH-PAH metabolite data; (2) had pCRH data at one or more timepoint; and (3) did not report smoking during pregnancy (and had cotinine ≤200 ng/mL), were eligible for inclusion in our primary analyses. We calculated descriptive statistics to characterize the sample including geometric mean and SD, median, min, max, quartiles, %<LOD, percentages, and frequencies) followed by bivariate analyses to examine relationships between the variables of interest, including the exposures and outcomes. We used Spearman correlations to examine associations among the OH-PAHs.
We fitted a set of individual linear regression models for each of the seven OH-PAHs included in the current analysis as well as two summed PAH variables (ΣNAP and ΣPHEN). For each OH-PAH, we examined the rate of change in pCRH between V1 and V2 using a mixed effects model with gestational day at pCRH sampling as a continuous variable and random intercepts for subjects. For each metabolite, we fit three staged models (minimally adjusted, fully adjusted, and extended), with covariates determined a priori based on the literature, particularly our recent publications on pCRH in this cohort (51, 60). Minimally adjusted models considered only those covariates most relevant to the biomarker measures themselves, namely specific gravity (as a measure of urine dilution) and gestational age at blood collection (relevant given the strong temporal changes in pCRH across pregnancy). Our primary, fully adjusted models additionally included maternal and pregnancy-related covariates associated with pCRH concentrations (and in some cases, with OH-PAH concentrations) including maternal age, race/ethnicity, education, pre-pregnancy BMI, cotinine, parity, childhood traumatic events, and fetal sex. In extended models, we additionally considered gestational diabetes and gestational hypertension as they have been confounders of pCRH in prior work, but could arguably be on the pathway between exposure and outcome (61, 62). Results are reported for the interaction between each of the OH-PAHs and time in days. Additionally, based on our prior work on pCRH in this cohort, we evaluated effect modification by the binary variables fetal sex, maternal childhood traumatic events (any/none), gestational hypertension, and gestational diabetes (51). To do so we refitted extended models adding a multiplicative interaction term (e.g. OH-PAH*fetal sex) with the p-value for the interaction term used to determine statistical significant differences by group (at alpha level=0.05).
Secondarily, we fit linear regression models examining pCRH at the two outcome timepoints (V1 and V2) separately. We additionally conducted a series of sensitivity analyses to evaluate the robusticity of our results. First, we refitted minimally adjusted, fully adjusted, and extended models using PAH levels pre-adjusted for specific gravity before model fitting (rather than including specific gravity as a covariate in the primary analysis) using the formula:
In this formula, P represents measured urinary OH-PAH concentration, SG represents individual participants’ specific gravity, and SGmedian is the median SG for the batch (63). We additionally refitted models: (1) including smokers and women with urinary cotinine >200ng/mL; (2) omitting preterm (<37 weeks) and post-term (>42 weeks) births; (3) omitting women with a history of prior preterm birth; and (4) without adjustment for childhood traumatic events. Finally, to avoid concerns around overlap in timing of V1 and V2, we refit models that more strictly adhered to trimester definitions (e.g. only including V1 occurring prior to 27 weeks and V2 occurring after 27 weeks gestation). All analyses were conducted using R 4.1.3 (R Foundation for Statistical Computing, Vienna, Austria).
Results
In total, 812 CANDLE participants were included in our main analyses (Table 1). Participants were on average 26.8 ± 5.6 years old at enrollment with a pre-pregnancy BMI of 28.1 ± 7.8 kg/m2. Most participants were non-Hispanic Black (62.0%) or non-Hispanic White (32.0%), with few identifying as Hispanic (1.0%) or another race (5.0%). The majority of participants had a high school/GED/or technical school-level education (55.0%), with an additional 37.0% completing college or higher. In total, 41.0% of participants were nulliparous and just over half (52.0%) were carrying female fetuses. A small percentage of participants developed gestational diabetes (6.0%) or gestational hypertension (10.0%) during the index pregnancy.
Of the OH-PAHs measured, 1-OH-NAP, 2-OH-NAP, 3-OH-PHEN, and 2/3/9-OH-FLUO were found in >96% of participants, with average 2-OH-NAP concentrations being highest (geometric mean 5.37 ± 2.41 ng/mL; Table 2). Correlations between the OH-PAH metabolites tended to be moderate to high, ranging from a low of r=0.22 for 1/9-OH-PHEN and 2-OH-NAP to a high of 0.85 for 2-OH-PHEN and 3-OH-PHEN (Supplementary Table S1). Overall, PAH metabolites tended to be higher among younger participants, less educated participants, participants with higher BMI, and non-Hispanic Black participants (compared to non-Hispanic White; Supplementary Table S2).
In mixed effect models integrating both outcome timepoints, all interaction terms for OH-PAHs and gestational days (time) indicated positive associations with the rate of the pCRH rise (Table 3). In minimally adjusted models, the strongest associations with the rate of the pCRH rise were observed for 2-OH-PHEN (β=0.0010; 95% CI:0.0002, 0.0018), 3-OH-PHEN (β=0.0008; 95% CI: -0.000002, 0.0016); and 1-OH-PYR (0.0007; 95% CI: -0.00003, 0.0013). In fully adjusted models, after adjustment for sociodemographic covariates, associations with the rate of the pCRH rise were moderately attenuated for all three metabolites: 2-OH-PHEN (β=0.0009; 95% CI:0.00006, 0.0017), 3-OH-PHEN (β=0.0007; 95% CI: -0.0002, 0.0015); and 1-OH-PYR (β=0.0005; 95% CI: -0.0002, 0.0010). Results of the extended models were similar to those of the fully adjusted models, with all confidence intervals including the null, with the exception of 2-OH-PHEN (β=0.0008; 95% CI:0.00002, 0.0016). In analyses evaluating effect modification by fetal sex, maternal exposure to childhood trauma, gestational diabetes, and gestational hypertension, little evidence of effect modification was observed (Supplementary Table S3).
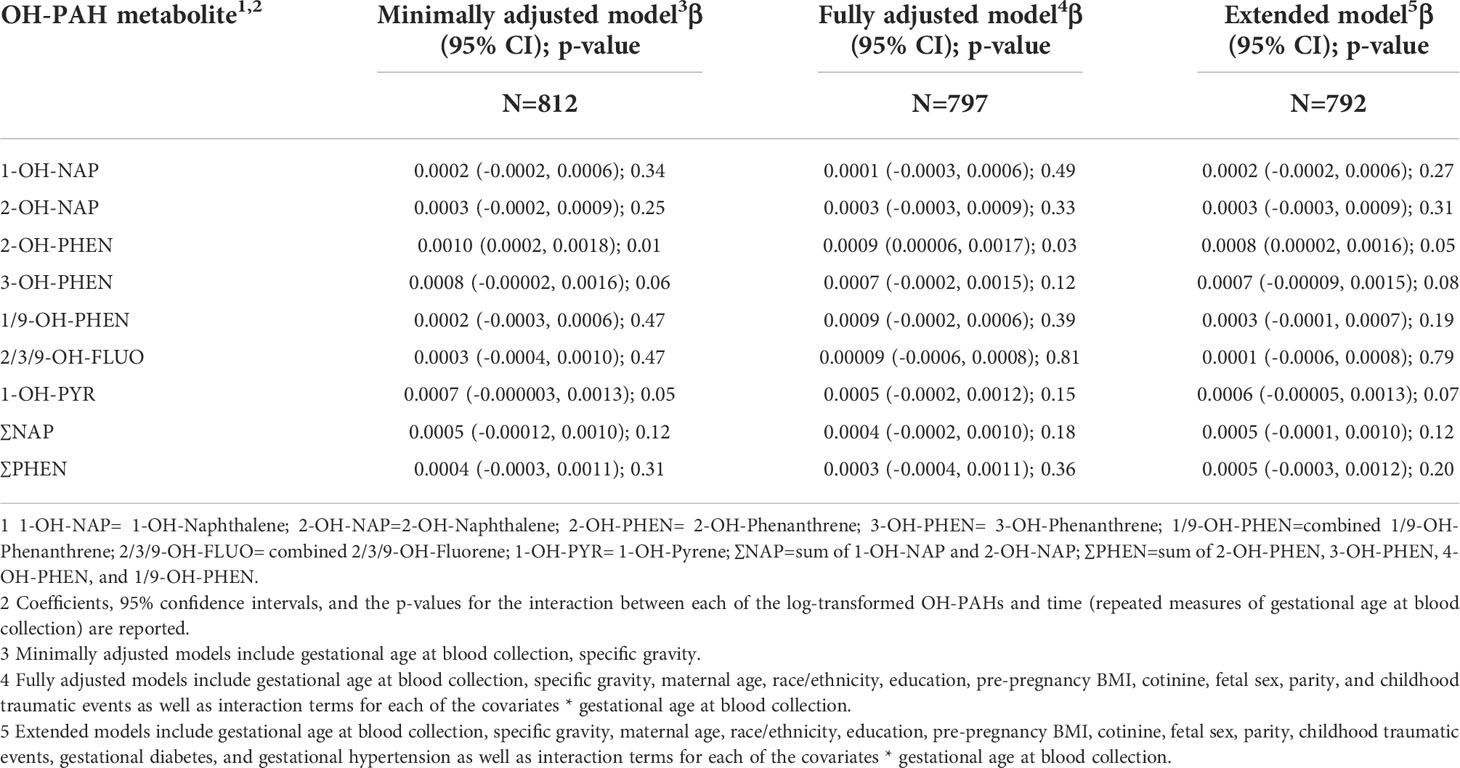
Table 3 Mixed effect models examining log-transformed PAH metabolite concentrations in relation to change in pCRH concentrations across mid-late pregnancy.
In secondary models examining the association between OH-PAH concentrations and pCRH at individual timepoints (Supplementary Table S4), we similarly observed associations in minimally adjusted models that were attenuated after adjustment for covariates. In minimally adjusted models, examining pCRH at V1, we observed significant associations with 3-OH-PHEN (β=-0.09, 95%CI: -0.16, -0.01), 2/3/9-OH-FLUO (β=-0.07, 95% CI: -0.13, -0.001), 1-OH-PYR (β=-0.10, 95%CI: -0.16, -0.03), and ∑NAP (β=-0.06, 95% CI: -0.12, -0.01), with a trend towards association observed for 2-OH-PHEN (β=-0.07, 95%CI: -0.14, 0.01). Most associations between OH-PAHs and V2 pCRH were inverse, but non-significant. Only 1-OH-PYR was significantly associated with lower V2 pCRH (β=-0.08, 95% CI: -0.15, -0.004). However, in fully adjusted models including sociodemographic and lifestyle-related covariates, associations were generally in the positive direction, though estimates were small in magnitude and 95% confidence intervals included the null across all OH-PAHs and all outcome measures (Supplementary Table S4). In extended models also including pregnancy complications, results were nearly identical to the fully adjusted models and again, null for all exposure-outcome associations.
In sensitivity analyses using specific gravity-adjusted OH-PAH values rather than including specific gravity as a covariate, results were unchanged (not shown). We additionally refit models to also including smokers and participants with urinary cotinine >200 ng/mL (an additional 119 participants), observing that overall, associations were similar, but modestly strengthened (Supplementary Table S5), with significant associations observed for 2-OH-PHEN and 3-OH-PHEN in the fully adjusted models. Results were similarly robust to the exclusion of preterm (<37 weeks) and post-term (>42 weeks) births (68 participants excluded), with positive associations and/or trends observed for 2-OH-PHEN, 3-OH-PHEN, and 1-OH-PYR in fully adjusted models (Supplementary Table S6). Results were virtually unchanged when we restricted analyses to participants with no history of prior preterm birth (60 participants excluded), although associations with 1-OH-PYR were stronger and reached statistical significance (β=0.008; 95% CI: 0.001, 0.0015; Supplementary Table S7). Results were nearly identical to our main models in sensitivity analyses excluding maternal childhood traumatic events as a covariate (Supplementary Table S8). Finally, patterns of association were robust to stricter adherence to visit timing by trimester (i.e. analyses restricted to participants with V1 occurring at or prior to 27 weeks and V2 occurring after 27 weeks gestation; Supplementary Table S9), although estimates were modestly attenuated, likely due to the smaller sample size (n=713).
Discussion
In this analysis, we examined associations between OH-PAHs and pCRH in a large, socioeconomically and racially diverse pregnancy cohort, observing that higher maternal 2-OH-PHEN concentrations (and to a lesser extent, 3-OH-PHEN and 1-OH-PYR) were associated with a more rapid rate of increase in pCRH in mid-late pregnancy, after adjustment for covariates. In models examining pCRH at individual timepoints, associations between OH-PAHs and pCRH in mid-pregnancy were attenuated after adjustment for sociodemographic and lifestyle factors, and no associations with late pregnancy pCRH were observed. We additionally observed no evidence of effect modification by fetal sex, childhood traumatic history, or pregnancy complications. Overall, our results suggest that maternal PAH exposure, particularly to 2-OH-PHEN, 3-OH-PHEN, and 1-OH-PYR, may amplify pCRH production in mid-late pregnancy, extending the limited prior literature on PAHs and pCRH.
In the prior study on this topic in the Puerto Rico-based PROTECT cohort (n=659), across nearly all metabolites, participants with higher PAH exposures had considerably higher pCRH concentrations, echoing the positive associations observed in our mixed effect models (50). In PROTECT, comparing women in the highest quartile of PAH exposure relative to the lowest, pCRH concentrations were higher in relation to 1-OH-NAP (14.0%, 95% CI: 4.06%, 24.9%), 1-OH-PYR (9.21%; 95% CI: -1.93%, 21.6%), 2-OH-FLU (15.30%; 95% CI: 4.54%, 27.10%), 1-OH-PHE (24.3%; 95% CI: 13.0%, 36.7%), and sum2,3-OH-PHE (18.1%, 95% CI: 7.00%, 30.4%). We similarly observed positive associations in relation to 1-OH-PYR as well as with 2-OH-PHEN and 3-OH-PHEN (considered individually in our study, but summed in PROTECT). However, in contrast to that study, we did not observe associations between pCRH and 1-OH-NAP nor the 2/3/9-hydroxyfluorene metabolites. In PROTECT, associations tended to be stronger in early in pregnancy (16-20 weeks) compared to the later visits (20-24 and 24-28 weeks); while we also observed stronger associations with pCRH in mid- (versus late) pregnancy, in models examining individual outcome timepoints, results were attenuated and confidence intervals included the null after adjustment for sociodemographic factors.
Despite the different geographic study locations, with likely differences in patterns of exposure to air pollutants as well as dietary sources of PAHs, concentrations of the four OH-PAHs measured in common across both studies (1-OH-NAP, 2-OH-NAP,1-OH-PYR, 4-OH-PHEN) were similar and, in some cases, slightly higher in CANDLE as compared to PROTECT. Across the two cohorts, pCRH concentrations were similar at the earlier study visit, with CANDLE median levels of 57.1 pg/mL at 16-29 weeks gestation and PROTECT median levels of 56.3 pg/mL at 16-20 weeks gestation. However, at the later study visit, CANDLE median pCRH levels rose to 360.3 pg/mL, whereas PROTECT levels stayed flat at 54.9 pg/mL. While some of this difference may be attributable to differences in the timing of the later visit (22-39 weeks in CANDLE vs 24-28 weeks in PROTECT), a more likely explanation is the use of enzyme-linked immunosorbent assay (ELISA) to perform the assays in PROTECT as compared to RIA in CANDLE. A recent study of 169 women with a prior preterm birth compared pCRH measured both by RIA and ELISA, showed that pCRH concentrations measured by ELISA were stable across gestation, whereas the same samples assayed using RIA showed a steep rise in late pregnancy (64). The flat pCRH levels across pregnancy as measured by ELISA are likely due to binding protein (CRH-BP) interference, which could bias results. While it is possible to perform ELISA with an extraction step that reduces this interference, more commonly that step is not included. Of note, in the comparison study, pCRH levels measured by RIA (but not ELISA) were associated with preterm birth, providing further evidence of pCRH as a “placental clock”.
The positive associations observed between OH-PAH concentrations and pCRH in the PROTECT study were hypothesized to be evidence of a potential mechanism by which PAHs may contribute to preterm birth (53). At present, evidence linking PAHs to preterm birth has been somewhat inconsistent, possibly due to methodological differences regarding study design, inclusion of smokers, preterm birth subtypes, adjustment for confounders, and varied approaches to exposure ascertainment (19–22, 65, 66). Within studies, results can be similarly inconsistent; for instance, in a Fresno, California study, participants in the highest quartile of PAH exposure during the last six weeks of pregnancy had 2.74 times the odds of delivering extremely preterm (20-27 weeks gestation) compared to the lowest quartile (21). However, the association did not hold for later preterm deliveries and, in some models examining other exposure windows, associations appeared to be protective. Most recently, we analyzed harmonized data from CANDLE and two additional cohorts, observing that 10-fold higher second trimester 2-OH-NAP concentrations were associated with 1.6 day shorter gestation, with some evidence of greater vulnerability to spontaneous preterm birth among female fetuses (19). In the current analysis associations between 2-OH-NAP and pCRH were weak and included the null. Conversely, OH-PAH metabolites associated in this analysis with higher rate of pCRH change across pregnancy (such as 2-OH-PHEN) showed little association with preterm birth in that prior analysis. Overall, these results suggest that if there are associations between OH-PAHs and preterm birth and/or shorter gestation, alternative mechanisms may warrant consideration, including induction of oxidative stress (65), changes in DNA methylation (20, 67), and disruption of other placental hormones, such as estriol (53).
A notable strength of this study is the socioeconomic and racial diversity of the sample, which included participants across a broad range of SES and was comprised of over 60% non-Hispanic Black women, a group often underrepresented in pregnancy cohort studies. Our use of a urinary biomarker of PAH exposure is also a strength of the current analysis. The urinary biomarker reflects exposure through multiple sources, including diet, which may account for over 90% of PAH exposure in non-smokers (68, 69). We used gold standard RIA for pCRH analysis of maternal plasma, which is considered superior to pCRH assays conducted using other analytic approaches or sample types (64, 70). Given the well-characterized nature of the CANDLE cohort, moreover, we were able to assess a number of confounders and potential effect modifiers that could influence our analyses; indeed, the significant associations observed in some minimally adjusted models were attenuated after adjustment for factors related to the social determinants of health, such as education, race, and childhood trauma. We observed inequalities in exposure such that OH-PAH concentrations were notably higher among Black and Hispanic participants (compared to non-Hispanic White), participants with lower levels of educational attainment, and overweight or obese participants. The higher OH-PAH concentrations observed in some sub-populations are likely the product of systemic racism and discrimination that leads to greater environmental pollution in marginalized communities. Our results were robust to a number of sensitivity analyses, including restricting the sample to term births, suggesting that associations were not driven by women with prior preterm birth history, who may have differing pCRH profiles.
We note several limitations of the current work. First, OH-PAH metabolites have a half-life of approximately 2-6 hours in the body (71) and in other studies, intra-class correlations characterizing OH-PAH concentrations across pregnancy have been in the low to moderate range (72). In this study, OH-PAHs were measured at a single timepoint in mid-pregnancy raising the possibility of exposure misclassification. Future work examining repeated OH-PAH exposures across pregnancy in relation to placental hormone production would be beneficial. Finally, although the diversity of the sample and its representativeness of Shelby County, Tennessee is a strength and findings are likely generalizable to other, understudied communities in the U.S. South, we cannot assume generalizability to the U.S. as a whole and certain sub-groups, like Latina and Asian women, are under-represented in our analysis. Additionally, by design, CANDLE participants were of relatively low medical risk at the time of recruitment and although some women ultimately developed pregnancy complications, by excluding higher risk pregnancies and women with major medical conditions (e.g. chronic hypertension requiring therapy, endocrine disease, and insulin-dependent diabetes) at enrollment, we may have reduced our ability to detect associations.
In conclusion, in this large pregnancy cohort, we observed some, limited evidence that mid-pregnancy OH-PAH metabolites, particularly 2-OH-PHEN, 3-OH-PHEN, and 1-OH-PYR, are associated with a more rapid increase in production of the placental hormone pCRH in mid to late pregnancy. Future research on disruption of placental hormone activity should examine OH-PAHs repeatedly across pregnancy and additional work is needed to identify alternative biological mechanisms that may link PAHs to preterm birth.
Data availability statement
The data analyzed in this study is subject to the following licenses/restrictions: The datasets analyzed for this study are not publicly available but de-identified data may be available on request, subject to approval by the internal review board and under a formal data use agreement. Contact the corresponding author for more information. Requests to access these datasets should be directed to ZW1pbHkuYmFycmV0dEBlb2hzaS5ydXRnZXJzLmVkdQ==.
Ethics statement
The studies involving human participants were reviewed and approved by University of Tennessee Health Sciences Center Institutional Review Board. The patients/participants provided their written informed consent to participate in this study.
Author contributions
EB led the analysis concept and manuscript writing and revision. TW, MH, SK, and CL led data analysis and interpretation and edited the manuscript. KK and MR conducted the chemical analyses, drafted manuscript sections, and edited the manuscript. RS conducted pCRH analyses and edited the manuscript. AS and QZ contributed to study design and implementation and edited the manuscript. KL, SS, and NB obtained funding, led study design, and edited the manuscript.
Funding
The ECHO PATHWAYS Consortium is funded by NIH UG3/UH3OD023271. The CANDLE study is funded by the Urban Child Institute as well as CIHR award number MWG-146331. Additional support for this analysis was provided by NIH P30ES005022 and UW NIEHS sponsored Biostatistics, Epidemiologic and Bioinformatic Training in Environmental Health (BEBTEH) Training Grant: NIEHS T32ES015459. This manuscript has been reviewed by PATHWAYS for scientific content and consistency of data interpretation with previous PATHWAYS publications. The content is solely the responsibility of the authors and does not necessarily represent the official views of the National Institutes of Health.
Acknowledgments
We thank the CANDLE participants as well as the staff of CANDLE and ECHO PATHWAYS.
Conflict of interest
The authors declare that the research was conducted in the absence of any commercial or financial relationships that could be construed as a potential conflict of interest.
Publisher’s note
All claims expressed in this article are solely those of the authors and do not necessarily represent those of their affiliated organizations, or those of the publisher, the editors and the reviewers. Any product that may be evaluated in this article, or claim that may be made by its manufacturer, is not guaranteed or endorsed by the publisher.
Supplementary material
The Supplementary Material for this article can be found online at: https://www.frontiersin.org/articles/10.3389/fendo.2022.1011689/full#supplementary-material
References
1. Keyte IJ, Harrison RM, Lammel G. Chemical reactivity and long-range transport potential of polycyclic aromatic hydrocarbons–a review. Chem Soc Rev (2013) 42:9333–91. doi: 10.1039/c3cs60147a
2. Murawski A, Roth A, Schwedler G, Schmied-Tobies MIH, Rucic E, Pluym N, et al. Polycyclic aromatic hydrocarbons (PAH) in urine of children and adolescents in Germany - human biomonitoring results of the German environmental survey 2014-2017 (GerES V). Int J Hyg Environ Health (2020) 226:113491. doi: 10.1016/j.ijheh.2020.113491
3. Keir JLA, Cakmak S, Blais JM, White PA. The influence of demographic and lifestyle factors on urinary levels of PAH metabolites-empirical analyses of cycle 2 (2009-2011) CHMS data. J Expo Sci Environ Epidemiol (2021) 31:386–97. doi: 10.1038/s41370-020-0208-4
4. Souza MCO, Rocha BA, Ximenez JPB, Devóz PP, Santana A, Campíglia AD, et al. Urinary levels of monohydroxylated polycyclic aromatic hydrocarbons in Brazilian children and health risk assessment: a human biomonitoring-based study. Environ Sci pollut Res Int (2022) 29:47298–309. doi: 10.1007/s11356-022-19212-z
5. Hudson-Hanley B, Smit E, Branscum A, Hystad P, Kile ML. Trends in urinary metabolites of polycyclic aromatic hydrocarbons (PAHs) in the non-smoking U.S. population, NHANES 2001-2014. Chemosphere (2021) 276:130211. doi: 10.1016/j.chemosphere.2021.130211
6. Boström CE, Gerde P, Hanberg A, Jernström B, Johansson C, Kyrklund T, et al. Cancer risk assessment, indicators, and guidelines for polycyclic aromatic hydrocarbons in the ambient air. Environ Health Perspect (2002) 110 Suppl 3:451–88. doi: 10.1289/ehp.110-1241197
7. IARC. Polynuclear aromatic compounds, part 1: chemical, environmental, and experimental data., IARC monographs on the evaluation of the carcinogenic risk of chemicals to humans. (Lyon, France: International Agency for Research on Cancer) (1982).
8. EPA. Priority pollutant list. Environmental Protection Agency (2014). Available at: https://www.epa.gov/sites/default/files/2015-09/documents/priority-pollutant-list-epa.pdf.
9. EC. Priority substances and certain other pollutants according to annex II of directive 2008/105/EC, environment. European Commission (2008). Available at: https://ec.europa.eu/environment/water/water-framework/priority_substances.htm.
10. Kummer V, Masková J, Zralý Z, Neca J, Simecková P, Vondrácek J, et al. Estrogenic activity of environmental polycyclic aromatic hydrocarbons in uterus of immature wistar rats. Toxicol Lett (2008) 180:212–21. doi: 10.1016/j.toxlet.2008.06.862
11. Hýžd'alová M, Pivnicka J, Zapletal O, Vázquez-Gómez G, Matthews J, Neca J, et al. Aryl hydrocarbon receptor-dependent metabolism plays a significant role in estrogen-like effects of polycyclic aromatic hydrocarbons on cell proliferation. Toxicol Sci (2018) 165:447–61. doi: 10.1093/toxsci/kfy153
12. Sahay D, Lloyd SE, Rivera JA, Jezioro J, McDonald JD, Pitiranggon M, et al. Prenatal polycyclic aromatic hydrocarbons, altered ERα pathway-related methylation and expression, and mammary epithelial cell proliferation in offspring and grandoffspring adult mice. Environ Res (2021) 196:110961. doi: 10.1016/j.envres.2021.110961
13. Pěnčíková K, Ciganek M, Neča J, Illés P, Dvořák Z, Vondráček J, et al. Modulation of endocrine nuclear receptor activities by polyaromatic compounds present in fractionated extracts of diesel exhaust particles. Sci Total Environ (2019) 677:626–36. doi: 10.1016/j.scitotenv.2019.04.390
14. Shirdel I, Kalbassi MR, Shokri M, Olyaei R, Sharifpour I. The response of thyroid hormones, biochemical and enzymological biomarkers to pyrene exposure in common carp (Cyprinus carpio). Ecotoxicol Environ Saf (2016) 130:207–13. doi: 10.1016/j.ecoenv.2016.03.023
15. He C, Zuo Z, Shi X, Sun L, Wang C. Pyrene exposure influences the thyroid development of sebastiscus marmoratus embryos. Aquat Toxicol (2012) 124-125:28–33. doi: 10.1016/j.aquatox.2012.07.007
16. Zhang Y, Dong S, Wang H, Tao S, Kiyama R. Biological impact of environmental polycyclic aromatic hydrocarbons (ePAHs) as endocrine disruptors. Environ pollut (2016) 213:809–24. doi: 10.1016/j.envpol.2016.03.050
17. Drwal E, Rak A, Grochowalski A, Milewicz T, Gregoraszczuk EL. Cell-specific and dose-dependent effects of PAHs on proliferation, cell cycle, and apoptosis protein expression and hormone secretion by placental cell lines. Toxicol Lett (2017) 280:10–9. doi: 10.1016/j.toxlet.2017.08.002
18. Gore AC, Chappell VA, Fenton SE, Flaws JA, Nadal A, Prins GS, et al. EDC-2: The endocrine society's second scientific statement on endocrine-disrupting chemicals. Endocr Rev (2015) 36:E1–e150. doi: 10.1210/er.2015-1010
19. Freije SL, Enquobahrie DA, Day DB, Loftus C, Szpiro AA, Karr CJ, et al. Prenatal exposure to polycyclic aromatic hydrocarbons and gestational age at birth. Environ Int (2022) 164:107246. doi: 10.1016/j.envint.2022.107246
20. Suter MA, Aagaard KM, Coarfa C, Robertson M, Zhou G, Jackson BP, et al. Association between elevated placental polycyclic aromatic hydrocarbons (PAHs) and PAH-DNA adducts from superfund sites in Harris county, and increased risk of preterm birth (PTB). Biochem Biophys Res Commun (2019) 516:344–9. doi: 10.1016/j.bbrc.2019.06.049
21. Padula AM, Noth EM, Hammond SK, Lurmann FW, Yang W, Tager IB, et al. Exposure to airborne polycyclic aromatic hydrocarbons during pregnancy and risk of preterm birth. Environ Res (2014) 135:221–6. doi: 10.1016/j.envres.2014.09.014
22. Wilhelm M, Ghosh JK, Su J, Cockburn M, Jerrett M, Ritz B. Traffic-related air toxics and preterm birth: a population-based case-control study in Los Angeles county, California. Environ Health (2011) 10:89. doi: 10.1186/1476-069X-10-89
23. Zhang X, Li X, Jing Y, Fang X, Zhang X, Lei B, et al. Transplacental transfer of polycyclic aromatic hydrocarbons in paired samples of maternal serum, umbilical cord serum, and placenta in shanghai, China. Environ pollut (2017) 222:267–75. doi: 10.1016/j.envpol.2016.12.046
24. Hatch MC, Warburton D, Santella RM. Polycyclic aromatic hydrocarbon-DNA adducts in spontaneously aborted fetal tissue. Carcinogenesis (1990) 11:1673–5. doi: 10.1093/carcin/11.9.1673
25. Liu R, DeSerisy M, Fox NA, Herbstman JB, Rauh VA, Beebe B, et al. Prenatal exposure to air pollution and maternal stress predict infant individual differences in reactivity and regulation and socioemotional development. J Child Psychol Psychiatry (2022) 63(11):1359–1367. doi: 10.1111/jcpp.13581
26. Wallace ER, Ni Y, Loftus CT, Sullivan A, Masterson E, Szpiro AA, et al. Prenatal urinary metabolites of polycyclic aromatic hydrocarbons and toddler cognition, language, and behavior. Environ Int (2022) 159:107039. doi: 10.1016/j.envint.2021.107039
27. Peterson BS, Rauh VA, Bansal R, Hao X, Toth Z, Nati G, et al. Effects of prenatal exposure to air pollutants (polycyclic aromatic hydrocarbons) on the development of brain white matter, cognition, and behavior in later childhood. JAMA Psychiatry (2015) 72:531–40. doi: 10.1001/jamapsychiatry.2015.57
28. Kehm RD, Oskar S, Tehranifar P, Zeinomar N, Rundle AG, Herbstman JB, et al. Associations of prenatal exposure to polycyclic aromatic hydrocarbons with pubertal timing and body composition in adolescent girls: Implications for breast cancer risk. Environ Res (2021) 196:110369. doi: 10.1016/j.envres.2020.110369
29. Rundle A, Hoepner L, Hassoun A, Oberfield S, Freyer G, Holmes D, et al. Association of childhood obesity with maternal exposure to ambient air polycyclic aromatic hydrocarbons during pregnancy. Am J Epidemiol (2012) 175:1163–72. doi: 10.1093/aje/kwr455
30. Rosa MJ, Jung KH, Perzanowski MS, Kelvin EA, Darling KW, Camann DE, et al. Prenatal exposure to polycyclic aromatic hydrocarbons, environmental tobacco smoke and asthma. Respir Med (2011) 105:869–76. doi: 10.1016/j.rmed.2010.11.022
31. Jung KH, Perzanowski M, Rundle A, Moors K, Yan B, Chillrud SN, et al. Polycyclic aromatic hydrocarbon exposure, obesity and childhood asthma in an urban cohort. Environ Res (2014) 128:35–41. doi: 10.1016/j.envres.2013.12.002
32. Perzanowski MS, Chew GL, Divjan A, Jung KH, Ridder R, Tang D, et al. Early-life cockroach allergen and polycyclic aromatic hydrocarbon exposures predict cockroach sensitization among inner-city children. J Allergy Clin Immunol (2013) 131:886–93. doi: 10.1016/j.jaci.2012.12.666
33. Adibi JJ, Layden AJ, Birru RL, Miragaia A, Xun X, Smith MC, et al. First trimester mechanisms of gestational sac placental and foetal teratogenicity: a framework for birth cohort studies. Hum Reprod Update (2021) 27:747–70. doi: 10.1093/humupd/dmaa063
34. Gladen BC, Zadorozhnaja TD, Chislovska N, Hryhorczuk DO, Kennicutt MC 2nd, Little RE. Polycyclic aromatic hydrocarbons in placenta. Hum Exp Toxicol (2000) 19:597–603. doi: 10.1191/096032700671433928
35. Jo YS, Ko HS, Kim AY, Jo HG, Kim WJ, Choi SK. Effects of polycyclic aromatic hydrocarbons on the proliferation and differentiation of placental cells. Reprod Biol Endocrinol (2022) 20:47. doi: 10.1186/s12958-022-00920-7
36. Raez-Villanueva S, Perono GA, Jamshed L, Thomas PJ, Holloway AC. Effects of dibenzothiophene, a sulfur-containing heterocyclic aromatic hydrocarbon, and its alkylated congener, 2,4,7-trimethyldibenzothiophene, on placental trophoblast cell function. J Appl Toxicol (2021) 41:1367–79. doi: 10.1002/jat.4128
37. Drwal E, Rak A, Gregoraszczuk EL. Differential effects of ambient PAH mixtures on cellular and steroidogenic properties of placental JEG-3 and BeWo cells. Reprod Toxicol (2019) 86:14–22. doi: 10.1016/j.reprotox.2019.03.003
38. Thomson M. The physiological roles of placental corticotropin releasing hormone in pregnancy and childbirth. J Physiol Biochem (2013) 69:559–73. doi: 10.1007/s13105-012-0227-2
39. Hobel CJ, Dunkel-Schetter C, Roesch SC, Castro LC, Arora CP. Maternal plasma corticotropin-releasing hormone associated with stress at 20 weeks' gestation in pregnancies ending in preterm delivery. Am J Obstet Gynecol (1999) 180:S257–63. doi: 10.1016/S0002-9378(99)70712-X
40. Ruiz RJ, Gennaro S, O'Connor C, Dwivedi A, Gibeau A, Keshinover T, et al. CRH as a predictor of preterm birth in minority women. Biol Res Nurs (2016) 18:316–21. doi: 10.1177/1099800415611248
41. Sandman CA, Glynn L, Schetter CD, Wadhwa P, Garite T, Chicz-DeMet A, et al. Elevated maternal cortisol early in pregnancy predicts third trimester levels of placental corticotropin releasing hormone (CRH): priming the placental clock. Peptides (2006) 27:1457–63. doi: 10.1016/j.peptides.2005.10.002
42. Wadhwa PD, Garite TJ, Porto M, Glynn L, Chicz-DeMet A, Dunkel-Schetter C, et al. Placental corticotropin-releasing hormone (CRH), spontaneous preterm birth, and fetal growth restriction: a prospective investigation. Am J Obstet Gynecol (2004) 191:1063–9. doi: 10.1016/j.ajog.2004.06.070
43. Harville EW, Savitz DA, Dole N, Herring AH, Thorp JM. Stress questionnaires and stress biomarkers during pregnancy. J Womens Health (Larchmt) (2009) 18:1425–33. doi: 10.1089/jwh.2008.1102
44. Laatikainen T, Virtanen T, Kaaja R, Salminen-Lappalainen K. Corticotropin-releasing hormone in maternal and cord plasma in pre-eclampsia. Eur J Obstet Gynecol Reprod Biol (1991) 39:19–24. doi: 10.1016/0028-2243(91)90136-9
45. Glynn LM, Sandman CA. Evaluation of the association between placental corticotrophin-releasing hormone and postpartum depressive symptoms. Psychosom Med (2014) 76:355–62. doi: 10.1097/PSY.0000000000000066
46. Yim IS, Glynn LM, Dunkel-Schetter C, Hobel CJ, Chicz-DeMet A, Sandman CA. Risk of postpartum depressive symptoms with elevated corticotropin-releasing hormone in human pregnancy. Arch Gen Psychiatry (2009) 66:162–9. doi: 10.1001/archgenpsychiatry.2008.533
47. Howland MA, Sandman CA, Glynn LM, Crippen C, Davis EP. Fetal exposure to placental corticotropin-releasing hormone is associated with child self-reported internalizing symptoms. Psychoneuroendocrinology (2016) 67:10–7. doi: 10.1016/j.psyneuen.2016.01.023
48. Sandman CA, Curran MM, Davis EP, Glynn LM, Head K, Baram TZ. Cortical thinning and neuropsychiatric outcomes in children exposed to prenatal adversity: A role for placental CRH? Am J Psychiatry (2018) 175:471–9. doi: 10.1176/appi.ajp.2017.16121433
49. Taylor AL, Fishman LM. Corticotropin-releasing hormone. N Engl J Med (1988) 319:213–22. doi: 10.1056/NEJM198807283190405
50. Cathey AL, Watkins D, Rosario ZY, Vélez C, Alshawabkeh AN, Cordero JF, et al. Associations of phthalates and phthalate replacements with CRH and other hormones among pregnant women in Puerto Rico. J Endocr Soc (2019) 3:1127–49. doi: 10.1210/js.2019-00010
51. Barrett ES, Corsetti M, Day D, Thurston SW, Loftus CT, Karr CJ, et al. Prenatal phthalate exposure in relation to placental corticotropin releasing hormone (pCRH) in the CANDLE cohort. Environ Int (2022) 160:107078. doi: 10.1016/j.envint.2022.107078
52. Eick SM, Goin DE, Cushing L, DeMicco E, Smith S, Park JS, et al. Joint effects of prenatal exposure to per- and poly-fluoroalkyl substances and psychosocial stressors on corticotropin-releasing hormone during pregnancy. J Expo Sci Environ Epidemiol (2021) 32(1):27–36. doi: 10.1038/s41370-021-00386-6
53. Cathey AL, Watkins DJ, Rosario ZY, Vélez Vega CM, Loch-Caruso R, Alshawabkeh AN, et al. Polycyclic aromatic hydrocarbon exposure results in altered CRH, reproductive, and thyroid hormone concentrations during human pregnancy. Sci Total Environ (2020) 749:141581. doi: 10.1016/j.scitotenv.2020.141581
54. Sontag-Padilla L, Burnms R, Shih R, Griffin B, Martin L, Chandra A, et al. The urban child institute CANDLE study: Methodological overview and baseline sample description. (United States: RAND Corporation) (2015).
55. Schick SF, Blount BC, Jacob PR, Saliba NA, Bernert JT, El Hellani A, et al. Biomarkers of exposure to new and emerging tobacco delivery products. Am J Physiol Lung Cell Mol Physiol (2017) 313:L425–l452. doi: 10.1152/ajplung.00343.2016
56. Guo Y, Senthilkumar K, Alomirah H, Moon HB, Minh TB, Mohd MA, et al. Concentrations and profiles of urinary polycyclic aromatic hydrocarbon metabolites (OH-PAHs) in several Asian countries. Environ Sci Technol (2013) 47:2932–8. doi: 10.1021/es3052262
57. Smith R, Smith JI, Shen X, Engel PJ, Bowman ME, McGrath SA, et al. Patterns of plasma corticotropin-releasing hormone, progesterone, estradiol, and estriol change and the onset of human labor. J Clin Endocrinol Metab (2009) 94:2066–74. doi: 10.1210/jc.2008-2257
58. Kubany ES, Leisen MB, Kaplan AS, Kelly MP. Validation of a brief measure of posttraumatic stress disorder: the distressing event questionnaire (DEQ). Psychol Assess (2000) 12:197–209. doi: 10.1037/1040-3590.12.2.197
59. Slopen N, Roberts AL, LeWinn KZ, Bush NR, Rovnaghi CR, Tylavsky F, et al. Maternal experiences of trauma and hair cortisol in early childhood in a prospective cohort. Psychoneuroendocrinology (2018) 98:168–76. doi: 10.1016/j.psyneuen.2018.08.027
60. Steine IM, LeWinn KZ, Lisha N, Tylavsky F, Smith R, Bowman M, et al. Maternal exposure to childhood traumatic events, but not multi-domain psychosocial stressors, predict placental corticotrophin releasing hormone across pregnancy. Soc Sci Med (2020) 266:113461. doi: 10.1016/j.socscimed.2020.113461
61. Lee I, Park H, Kim MJ, Kim S, Choi S, Park J, et al. Exposure to polycyclic aromatic hydrocarbons and volatile organic compounds is associated with a risk of obesity and diabetes mellitus among Korean adults: Korean national environmental health survey (KoNEHS) 2015-2017. Int J Hyg Environ Health (2022) 240:113886. doi: 10.1016/j.ijheh.2021.113886
62. Mallah MA, Mallah MA, Liu Y, Xi H, Wang W, Feng F, et al. Relationship between polycyclic aromatic hydrocarbons and cardiovascular diseases: A systematic review. Front Public Health (2021) 9:763706. doi: 10.3389/fpubh.2021.763706
63. Levine L, Fahy J. Evaluation of urinary lead concentrations. i. the significance of the specific gravity. J Ind Hyg Toxicol (1945) 27:217–23.
64. Herrera CL, Bowman ME, McIntire DD, Nelson DB, Smith R. Revisiting the placental clock: Early corticotrophin-releasing hormone rise in recurrent preterm birth. PLoS One (2021) 16:e0257422. doi: 10.1371/journal.pone.0257422
65. Agarwal P, Singh L, Anand M, Taneja A. Association between placental polycyclic aromatic hydrocarbons (PAHS), oxidative stress, and preterm delivery: A case-control study. Arch Environ Contam Toxicol (2018) 74:218–27. doi: 10.1007/s00244-017-0455-0
66. Patel CJ, Yang T, Hu Z, Wen Q, Sung J, El-Sayed YY, et al. Investigation of maternal environmental exposures in association with self-reported preterm birth. Reprod Toxicol (2014) 45:1–7. doi: 10.1016/j.reprotox.2013.12.005
67. Le Vee M, Kolasa E, Jouan E, Collet N, Fardel O. Differentiation of human placental BeWo cells by the environmental contaminant benzo(a)pyrene. Chem Biol Interact (2014) 210:1–11. doi: 10.1016/j.cbi.2013.12.004
68. Polachova A, Gramblicka T, Parizek O, Sram RJ, Stupak M, Hajslova J, et al. Estimation of human exposure to polycyclic aromatic hydrocarbons (PAHs) based on the dietary and outdoor atmospheric monitoring in the Czech republic. Environ Res (2020) 182:108977. doi: 10.1016/j.envres.2019.108977
69. Zhang Y, Ding J, Shen G, Zhong J, Wang C, Wei S, et al. Dietary and inhalation exposure to polycyclic aromatic hydrocarbons and urinary excretion of monohydroxy metabolites–a controlled case study in Beijing, China. Environ pollut (2014) 184:515–22. doi: 10.1016/j.envpol.2013.10.005
70. Latendresse G, Ruiz RJ. Bioassay research methodology: measuring CRH in pregnancy. Biol Res Nurs (2008) 10:54–62. doi: 10.1177/1099800408320970
71. Li Z, Romanoff L, Bartell S, Pittman EN, Trinidad DA, McClean M, et al. Excretion profiles and half-lives of ten urinary polycyclic aromatic hydrocarbon metabolites after dietary exposure. Chem Res Toxicol (2012) 25:1452–61. doi: 10.1021/tx300108e
Keywords: polycyclic aromatic hydrocarbons, pregnancy, endocrine disruption, hormones, placenta, corticotropin releasing hormone
Citation: Barrett ES, Workman T, Hazlehurst MF, Kauderer S, Loftus C, Kannan K, Robinson M, Smith AK, Smith R, Zhao Q, LeWinn KZ, Sathyanarayana S and Bush NR (2022) Prenatal polycyclic aromatic hydrocarbon (PAH) exposure in relation to placental corticotropin releasing hormone (pCRH) in the CANDLE pregnancy cohort. Front. Endocrinol. 13:1011689. doi: 10.3389/fendo.2022.1011689
Received: 04 August 2022; Accepted: 26 October 2022;
Published: 11 November 2022.
Edited by:
Joëlle Rüegg, Uppsala University, SwedenCopyright © 2022 Barrett, Workman, Hazlehurst, Kauderer, Loftus, Kannan, Robinson, Smith, Smith, Zhao, LeWinn, Sathyanarayana and Bush. This is an open-access article distributed under the terms of the Creative Commons Attribution License (CC BY). The use, distribution or reproduction in other forums is permitted, provided the original author(s) and the copyright owner(s) are credited and that the original publication in this journal is cited, in accordance with accepted academic practice. No use, distribution or reproduction is permitted which does not comply with these terms.
*Correspondence: Emily S. Barrett, RW1pbHkuYmFycmV0dEBlb2hzaS5ydXRnZXJzLmVkdQ==