- 1School of Medicine and Surgery, University of Milano - Bicocca, Milan, Italy
- 2Cardiology Unit, Istituto Auxologico Italiano, Istituto di Ricovero e Cura a Carattere Scientifico (IRCCS), Milan, Italy
It has been argued that metabolically healthy obesity (MHO) does not increase the risk of cardiovascular disease. The aim of this study is to evaluate whether, in a population of obese children/adolescents, the metabolically unhealthy obesity (MUO) phenotype is associated with higher left ventricular mass index and/or higher prevalence of left ventricular hypertrophy than the MHO phenotype. We also tested whether the addition of an insulin resistance index (HOMA-index >90th percentile by sex and age) and the presence of hyperuricemia (serum uric acid >90th percentile by sex and age) to the definition of MUO better identified obese children with early cardiac damage. Left ventricular hypertrophy was defined as the presence of left ventricular mass index greater than or equal to the age- and sex-specific 95th percentile.
The study population included 459 obese children (males 53.2%, mean age 10.6 [standard deviation, 2.6] years), of whom 268 (58.4%) were MUO. The left ventricular mass index was higher in MUO children than in MHO children (37.8 vs 36.3 g/m2.7, p=0.015), whereas the percentage of MUO children presenting left ventricular hypertrophy was only slightly higher in MUO children (31.1 vs 40%, p=0.06). Multiple linear regression analyses showed that the variables significantly associated with higher left ventricular mass index were male gender (p<0.01), Body Mass Index z-score (p<0.001) and Waist-to-Height-ratio (p<0.001). Multiple logistic regression analyses showed that the presence of left ventricular hypertrophy was only significantly associated with higher Body Mass Index z-score (p<0.05) and Waist-to-Height-ratio (p<0.05). In spite of the higher left ventricular mass index of MUO as compared to MHO children, the MUO phenotype was not a significant predictor of either higher left ventricular mass index or higher left ventricular hypertrophy prevalence. The MUO phenotype had a low predictive ability on the presence of left ventricular hypertrophy. The area under the receiver operating characteristic curve was 0.57 (sensitivity 0.64, 1-specificity 0.55). The addition of insulin resistance and hyperuricemia to the definition of MUO did not change the results observed with the standard definition of MUO at multivariable analysis.
The MUO phenotype appears to be of little usefulness in identifying the early presence of cardiac damage in a large population of obese children and adolescents. Excess weight and abdominal obesity are confirmed as an important determinant of early organ damage in obese children.
Introduction
Obesity in adults is often associated with several cardiovascular risk factors, such as those characterizing the metabolic syndrome: high blood pressure (BP), low HDL cholesterol, hypertriglyceridemia and hyperglycemia (1). Adults in severe excess weight who have none of these risk factors are called metabolically healthy obese (MHO). In contrast, the metabolically unhealthy obese (MUO) are those who have one or more of these risk factors (2, 3). Although there is some uncertainty in the definition of the different risk factors, these classifications are also used in pediatrics (4, 5). Damanhoury et al. (6) proposed that children with none of the following alterations are considered to have the MHO phenotype: systolic blood pressure (SBP) or diastolic blood pressure (DBP) ≥90th percentile, glycaemia ≥100mg/dl, HDL cholesterol ≤40mg/dl, triglycerides ≥100 mg/dl (children <10 years) or ≥130 mg/dl (children ≥10 years). A recent study showed that, in a population of obese children and adolescents, elevated serum uric acid (SUA) values and HOMA-index were able to predict the possibility of being MUO according to Damanhoury’s classification (6). In adults, the presence of cardiovascular risk factors is frequently associated with organ damage. In pediatric age, early organ damage has been described in at-risk individuals, and left ventricular hypertrophy (LVH) is the organ damage most frequently found in children and adolescents with cardiovascular risk factors (7, 8). Excess weight is an important determinant for the development of LVH in children and adolescents (9), but even hemodynamic and metabolic factors may contribute to the increase in cardiac mass (10–12). The aim of this study is to evaluate whether, in a population of obese children/adolescents, i) individuals defined as MUO according to the Damanhoury classification (6) have higher left ventricular mass index (LVMI) and/or higher prevalence of LVH than MHO individuals ii) the addition of higher-than-normal HOMA-index and SUA values to the four risk factors of the Damanhoury’s classification allows better identification of children with initial cardiac organ damage (i.e. higher LVMI and higher prevalence of LVH).
Methods (for extended form see supplementary material)
Subjects
We studied a cohort of 459 obese children and adolescents, consecutively referred from december 2012 to april 2022 by their primary care pediatricians to our clinic. Exclusion criteria were: impaired glucose tolerance, diabetes, any form of secondary hypertension, treatment with antihypertensive drugs.
Anthropometric parameters and blood pressure measurements
In all children, height, weight and waist circumference (WC) were measured. Body mass index (BMI) and waist-to-height-ratio (WtHr) were calculated. BMI z-scores were calculated using the Centre for Disease and Control prevention charts. Obesity was defined according to the International Obesity Task Force (13). Children were classified as pre-pubertal and pubertal according to Tanner (14).
Blood pressure measurements were performed by an oscillometric device validated in children (Omron 705 IT; Omron Co, Kyoto, Japan). The BP measurement was performed 3 times and the average of the last two measurements was considered. Systolic BP (SBP) and diastolic BP (DBP) percentiles and z-scores were calculated according to the nomograms of the National High Blood Pressure Education Program (NHBPEP) Working Group on High Blood Pressure in Children and Adolescents (15).
Biochemical parameters
Blood samples were taken from all subjects after a 12-hour fasting period in order to measure serum concentrations of high-density lipoprotein (HDL), triglycerides, glucose, insulin and uric acid. HOMA index was calculated by dividing the product of serum insulin (µU/ml) and serum glucose (mmol/L) by 22.5 (16).
Echocardiography
Two-dimensional M-mode echocardiography images were obtained using digital echocardiography equipment (Aloka ProSound SSD Alpha 10, Tokyo, Japan) with 1-5MHz transducers, and following the recommendations for standard M-mode measurements. Left ventricular mass was calculated according to the American Society of Echocardiography convention, and indexed (LVMI) to height (m2.7) (17).
Left ventricular hypertrophy was defined as the presence of a LVMI greater than or equal to the 95th percentile specific for age and gender, according to the reference values by Khoury (18) as recommended by the latest guidelines of the European Society of Cardiology (19).
MHO/MUO definition
Standard MUO phenotype was defined as the presence of at least one of the following risk factors: SBP and/or DBP> 90th percentile, glycaemia >100 mg/dl, HDL cholesterol <40 mg/dl, triglycerides >100 mg/dl (children <10 years) or >130 mg/dl (children>10 years) (6).
New MUO phenotype was defined as the presence of at least one of the following risk factors: SBP and/or DBP> 90th percentile, glycaemia >100 mg/dl, HDL cholesterol <40 mg/dl, triglycerides >100 mg/dl (children <10 years) or >130 mg/dl (children>10 years). HOMA index value >90th percentile by gender and age (20), and SUA value >90th percentile by gender and age (21).
Statistical analysis
Multiple linear regression models were used to assess the impact of standard (or new) classification, gender, age, pubertal status, BMI z-score (or WtHr) on LVMI.
Multiple logistic regression models were used to assess the impact of standard (or new) classification, gender, age, pubertal status, BMI z-score (or WtHr) on the presence of LVH.
To investigate the ability of the two classifications to discriminate among the presence/absence of LVH, sensitivity and 1-specificity were calculated. The probability of having LVH was related to the two classifications in a logistic regression model adjusted by gender, age, pubertal status and BMI to obtain a continuous score by the weighted contribution of the classification and the adjustment factors through the model coefficients. The receiver operating characteristic (ROC) curve of each score was calculated to investigate the ability of the classification to discriminate among the presence/absence of LVH. The area under the ROC curve (AUC) of each classification was calculated as summary discrimination measure.
Statistical analyses were performed with R 4.1.2 (http://www.R-project.org). All p-values were 2-sided, with p-values <0.05 considered statistically significant.
Results
The study population was described in Table 1. We included 459 obese children (males 53.2%), of whom 40.7% had started pubertal development (Table 1). Forty-three percent had SBP and/or DBP values >90th percentile. Regarding metabolic disorders, only 1.3% had serum glucose >100 mg/dl, however 77.8% had HOMA index values >90th percentile. In 19.6% of the children, plasma triglyceride values were above the normal cut-off, and in 16.1% HDL cholesterol was <40 mg/dl. Serum uric acid levels were greater than the 90th percentile in 21.8% of the subjects. The mean LVMI value was 37.1 g/m2.7 and 36.3% of the population had LVH.
Considering the standard definition of the MUO phenotype, 268 (58.4%) children were found to be MUO, whereas the percentage increased significantly using the new classification (n = 414, 90.2%). Specifically, 72.8% of the children classified as MHO with the standard classification had HOMA-index values >90th percentile and 16.2% had SUA values >90th percentile.
With both classifications, MUO children were older (p<0.02) and had higher BMI z-scores and WtHr values than MHO children (p<0.001). The left ventricular mass index was higher in MUO children than in MHO children (37.8 vs 36.3 g/m2.7, p=0.015) when defined by the standard classification, but there were no differences between the LVMI values of MHO and MUO children when defined by the new classification (35.8 vs 37.3 g/m2.7, p=0.61) (Figure 1). Similar results were observed regarding the percentage of children with LVH (31.1 vs 40%, p=0.06 MHO vs MUO, standard classification and 35.6 vs 36.3% p=0.99 MHO vs MUO, new classification) (Table 1).
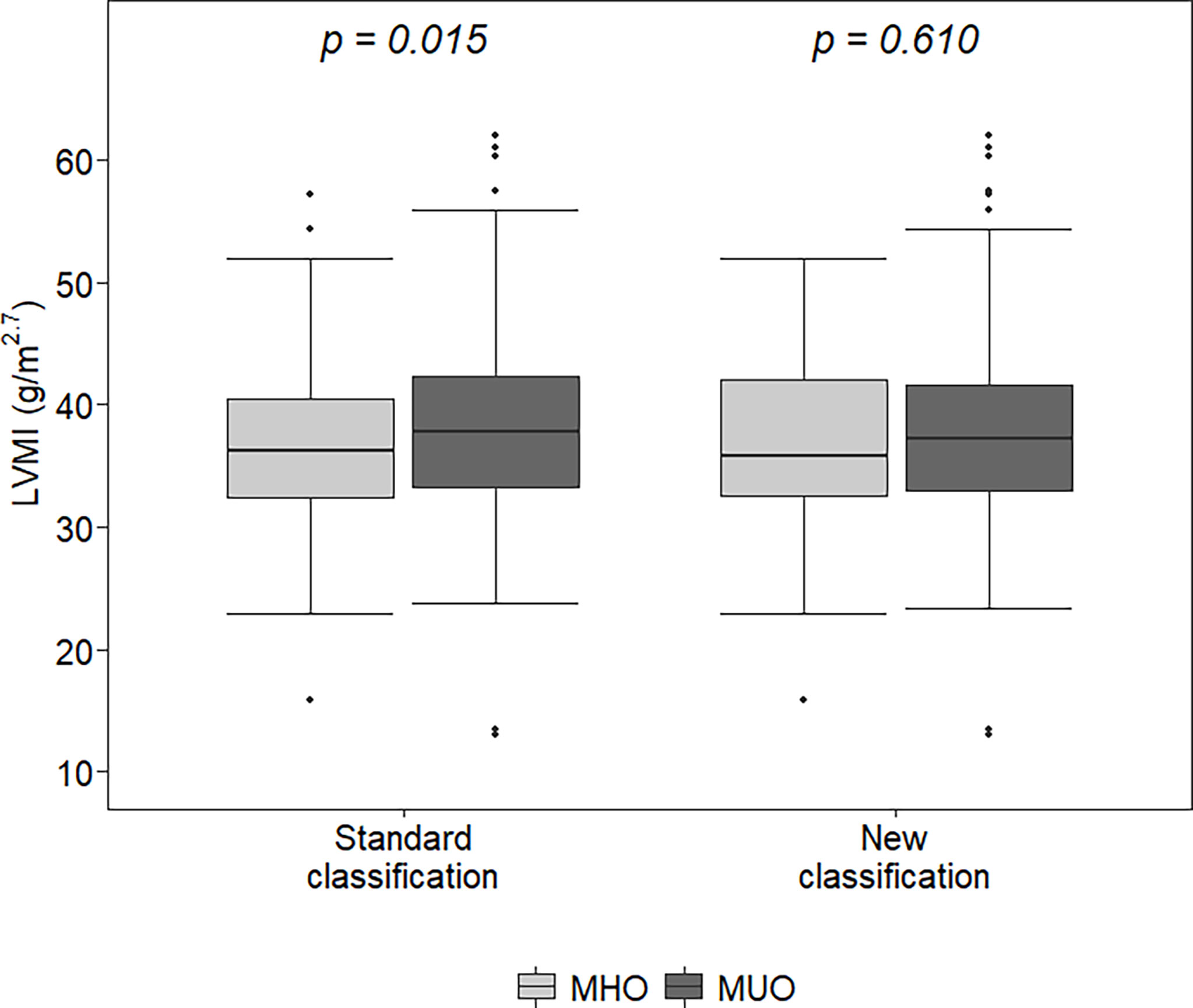
Figure 1 Left ventricular mass index (LVMI) in metabolically healthy obese (MHO) and metabolically unhealthy obese (MUO) according to the two classifications. The lower (and upper) whiskers extend up to the lowest (highest) datum still within 1.5 times IQR of the first (third) quartile.
Multiple linear regression analyses showed that the variables significantly associated with higher LVMI were male gender (p<0.01), BMI z-score (p<0.001, Table 2, Model A) and WtHr (p<0.001, Table 2, Model B), both when the models were adjusted for standard and new MUO classification. Adjusting for WtHr (Model B), an inverse association with age was present with both classifications (p<0.01). Results of the same models after the log-transformation of the outcome were similar(data not shown).
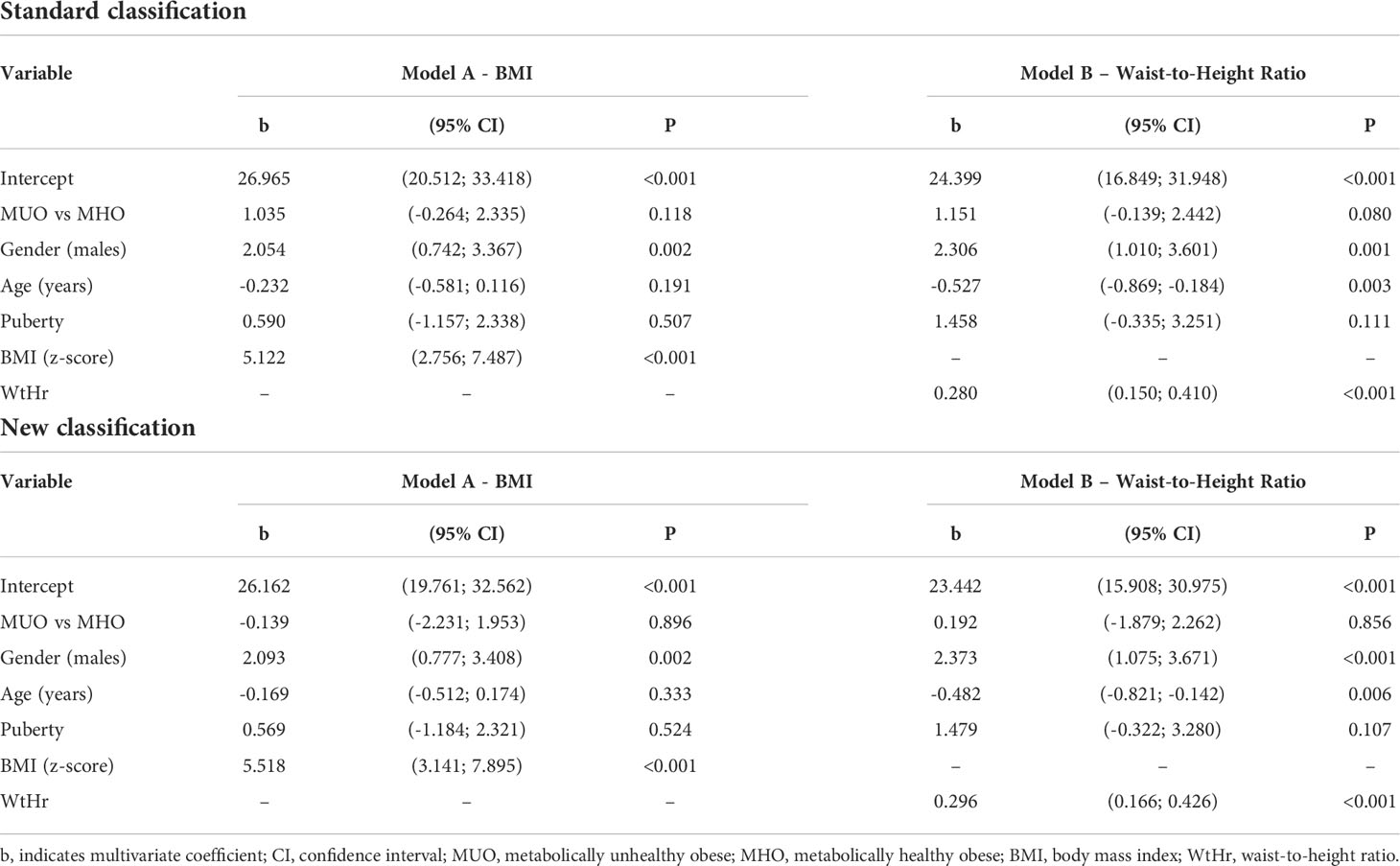
Table 2 Effect of metabolically unhealthy obese phenotype, gender, age, puberty, BMI (Model A) or waist-to-height ratio (Model B) on left ventricular mass index by a multiple linear regression model.
Multiple logistic regression analyses showed that the presence of LVH was only significantly associated with BMI z-score (p<0.05, Table 3, Model A) and WtHr (p<0.05, Table 3, Model B), both when the models were adjusted for the standard and the new classification.
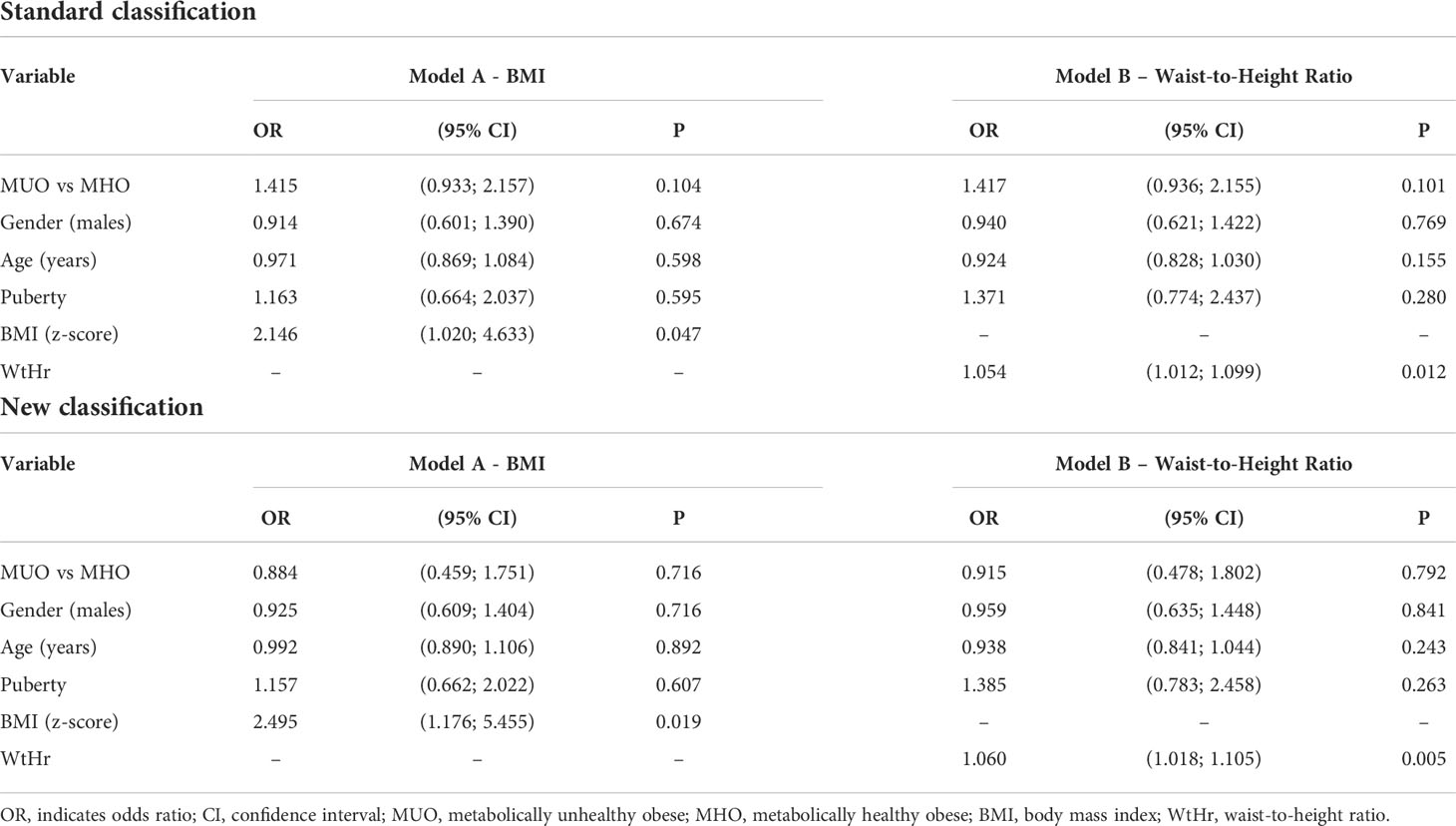
Table 3 Effect of metabolically unhealthy obese phenotype, gender, age, puberty, BMI (Model A) or waist-to-height ratio (Model B) on left ventricular hypertrophy by a multiple logistic regression model.
Additional analyses performed separately in pre-pubertal and pubertal individuals and in males and females confirmed these findings (Tables S1–S4).
The ability of the two classifications to predict the presence of LVH is shown in Figure 2. The AUC of the standard classification was 0.574, while that of the new classification was 0.557. Considering sensitivity, among the 165 children with LVH, 106 (64.2%) were classified as MUO with the standard classification, and 149 (90.3%) with the new classification. In contrast, among the 290 children without LVH, 159 (54.8%) were classified as MUO with the standard classification and 261 (90.0%) with the new classification. Table 4 shows sensitivity, specificity, positive and negative predictive values of the two classifications.
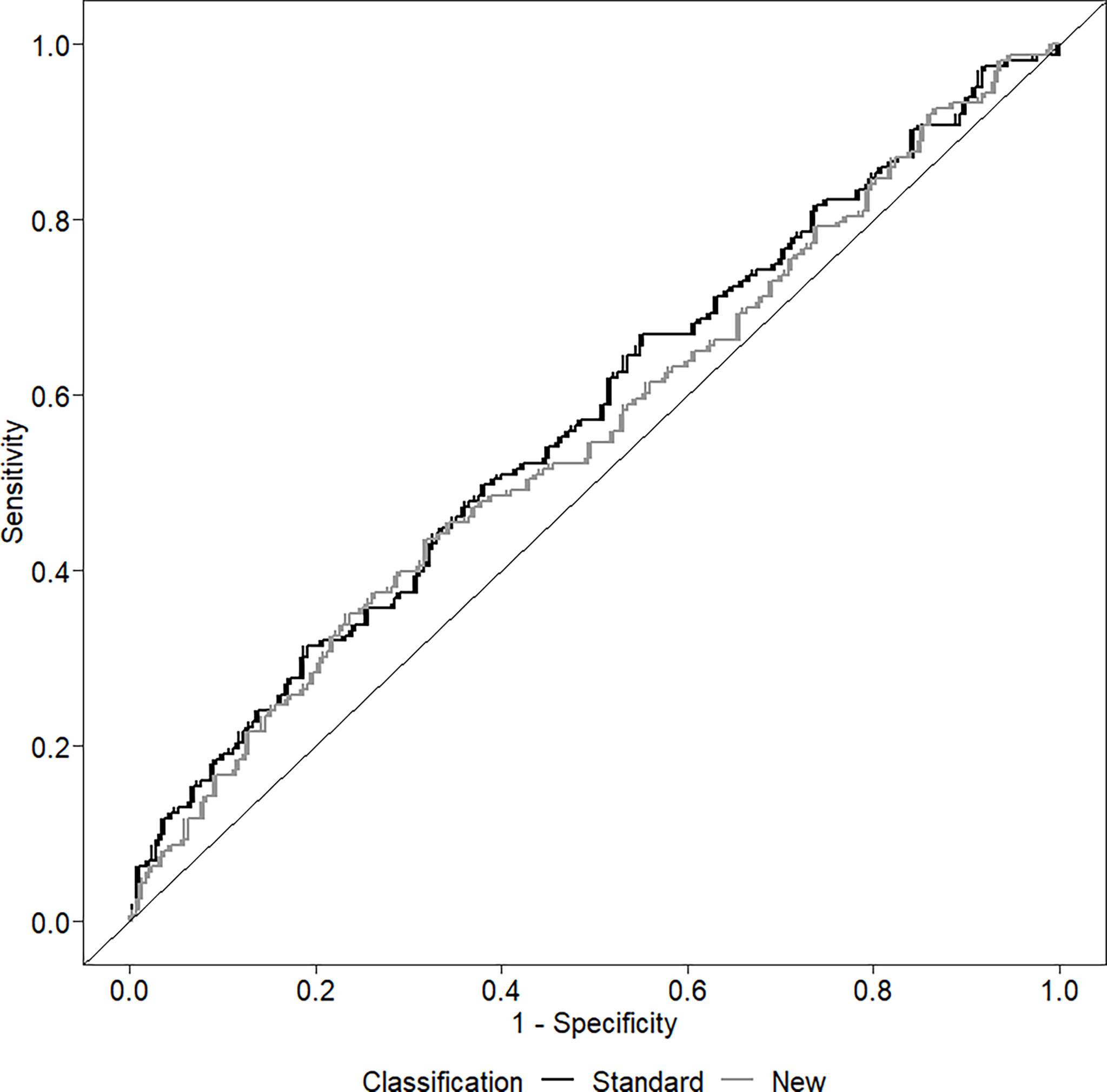
Figure 2 Receiver operating curve (ROC) investigating the ability of the two classifications to discriminate among the presence/absence of left ventricular hypertrophy.
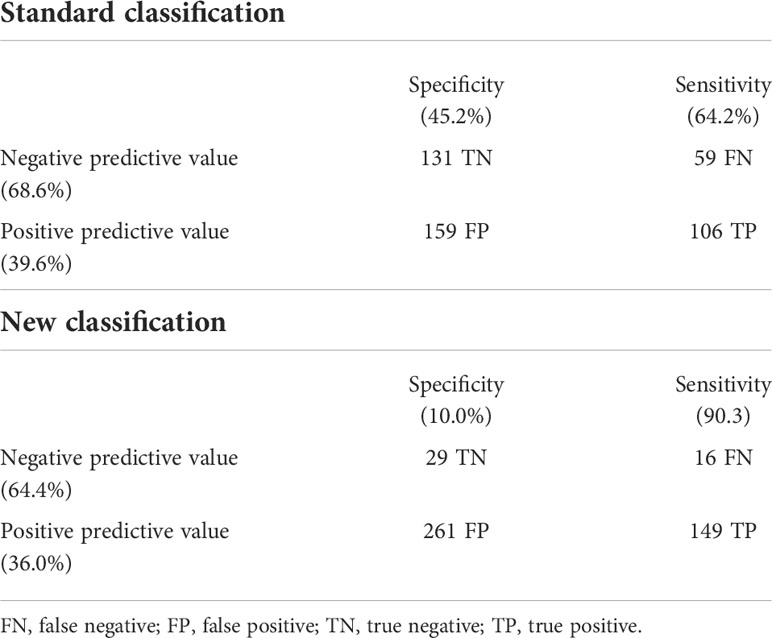
Table 4 Predictive capability of the two classifications to discriminate among the presence/absence of left ventricular hypertrophy.
Discussion
The prevalence of the MUO phenotype in our population of obese children is high. About 60% of the sample is found to have at least one of the criteria included in the standard classification and about 90% have the MUO phenotype considering the new classification. However, both classifications show poor predictive value in identifying children and adolescents with higher LVMI or LVH.
MUO phenotype and cardiac damage: standard classification
In adults, the prevalence of unhealthy obesity ranges from approximately 66 to 91% (22). Regarding pediatric age, the prevalence of the MUO phenotype reported in the literature varies widely (32 to 80%) (23). In our population, when using the standard classification, we find a prevalence of MUO children that is in agreement with that described by Reinehr et al. in a large sample of Caucasian children (24), while it is lower than that reported by other authors in a smaller sample (4).
The MUO children in our sample are older than the MHO children, more often have started pubertal development and more often are male. Both BMI z-score and WtHr are higher in the MUO group. The prevalence of elevated BP in the MUO phenotype is 53%, approximately 30% have dyslipidaemia and only 2% have elevated plasma glucose values. Left ventricular mass index is significantly higher in MUO subjects than in MHO subjects and there is a slightly higher prevalence of LVH.
In multivariable analysis models, the MUO phenotype is associated neither with higher LVMI nor with higher prevalence of LVH, whereas BMI z-score and WtHr are associated with both. The risk of presenting LVH doubles for each BMI z-score point and increases by 5% for each WtHr point.
MUO phenotype and cardiac damage: New classification
If we add an insulin resistance index and the presence of hyperuricemia to the standard definition of MUO, the prevalence of the phenotype almost doubles. This fact leads us to question whether we can really define ‘healthy’ children and adolescents according to Damanhoury’s classification (6).This classification significantly underestimates children with early IR. In the group defined as MHO, compared with 1.6% high plasma glucose levels, 73% have HOMA-index values above the 90th percentile. In addition, 16% had elevated SUA values. These data confirm what has already been observed in another population (25) and suggest the presence of a high percentage of metabolic alterations in obese children and adolescents that is not recognised by the standard definition of MUO.
The MUO phenotype is more frequent in older individuals and those with greater excess weight and waist circumference. With the new classification, no significant differences in LVMI and LVH rates are observed between the two phenotypes, but this could also be due to the fact that the MHO group is poorly represented (less than 10%).
In the multivariable model, the main determinants of cardiac damage (i.e. elevated LVMI and presence of LVH) are BMI and WtHr values: the risk of LVH is two and a half times higher for a one-point increase in the BMI z-score and increases by 6% for each point of WtHr.
Predictive value of the two classifications on LVH
Both classifications have a modest ability to predict the presence of LVH. The area under the ROC curve has a low value for both classifications. The new classification has a high sensitivity, but at the price of very poor specificity, while the standard classification has both poor sensitivity and poor specificity. It therefore seems that, regarding the identification of early cardiac damage in obese children, there is no advantage in considering a cluster of risk factors, rather than assessing the impact of excess weight (or visceral adiposity) as a single risk factor. This result could depend on several causes. Firstly, the impact of different risk factors on early organ damage could differ depending on the organ considered. An increased carotid intima media thickness (cIMT), associated with the MUO phenotype, has been described in children (26). It is possible that metabolic alterations exert a negative effect more on the vessels than on the heart and that this is mediated by the presence of endothelial dysfunction (27, 28). It would be interesting to assess whether adding IR and hyperuricemia to the definition of the MUO phenotype could improve the identification of children with early vascular organ damage. In addition, it is known that BMI is the main determinant of the onset of LVH in children and has an effect three times greater than that of hypertension (29), while the effect of high BP values on vascular stiffness is much more important than that of excess weight (30).
Secondly, it is possible that if the study population included children with a lower degree of excess weight, the MUO phenotype (however defined) might be more useful in identifying early organ damage. Indeed, in this case, we might expect fewer subjects identified as MUO and this might help to better discriminate those at greater risk of developing organ damage.
Third, as in adults the MUO phenotype has been associated with a higher prevalence of LVH than MHO (31, 32),it is possible that the negative impact of BP and metabolic alterations on the heart needs time to manifest itself and does not do so as early as childhood.
Conclusions
The standard classification of the MUO phenotype appears to be of little use in identifying the early presence of cardiac damage in a large population of obese children and adolescents. In addition, most obese individuals classified as MHO have significant metabolic alterations that are worthy of the paediatric physician’s attention. Excess weight and abdominal obesity are confirmed as an important determinant of early cardiac damage in obese children
Data availability statement
The raw data supporting the conclusions of this article will be made available by the authors, without undue reservation.
Ethics statement
The studies involving human participants were reviewed and approved by Ethic Committee Name: Istituto Auxologico Italiano, Approval Code: 2015102002, Approval Date: 20/11/2015. Written informed consent to participate in this study was provided by the participants’ legal guardian/next of kin.
Author contributions
SG conceptualized and designed the study, drafted the initial manuscript, and reviewed and revised the manuscript. IP, GL, AO, MG and MM collected data. ET and LA performed data analysis. GP reviewed and revised the manuscript. All authors approved the final manuscript as submitted and agree to be accountable for all aspects of the work.
Funding
This research was funded by the Italian Ministry of Health.
Conflict of interest
The authors declare that the research was conducted in the absence of any commercial or financial relationships that could be construed as a potential conflict of interest.
Publisher’s note
All claims expressed in this article are solely those of the authors and do not necessarily represent those of their affiliated organizations, or those of the publisher, the editors and the reviewers. Any product that may be evaluated in this article, or claim that may be made by its manufacturer, is not guaranteed or endorsed by the publisher.
Supplementary material
The Supplementary Material for this article can be found online at: https://www.frontiersin.org/articles/10.3389/fendo.2022.1006588/full#supplementary-material
References
1. Reaven GM. Role of insulin resistance in human disease (syndrome x): An expanded definition. Annu Rev Med (1993) 44:121–31. doi: 10.1146/annurev.me.44.020193.001005
2. Primeau V, Coderre L, Karelis A, Brochu M, Lavoie M-E, Messier V, et al. Characterizing the profile of obese patients who are metabolically healthy. Int J Obes (2011) 35:971–81. doi: 10.1038/ijo.2010.216
3. Rey-López JP, de Rezende LF, Pastor-Valero M, Tess BH. The prevalence of metabolically healthy obesity: A systematic review and critical evaluation of the definitions used. Obes Rev (2014) 15:781–90. doi: 10.1111/obr.12198
4. Prince RL, Kuk JL, Ambler KA, Dhaliwal J, Ball GDC. Predictors of metabolically healthy obesity in children. Diabetes Care (2014) 37:1462–8. doi: 10.2337/dc13-1697
5. Vukovic R, Dos Santos TJ, Ybarra M, Atar M. Children with metabolically healthy obesity: A review. Front Endocrinol (Lausanne) (2019) 10:865. doi: 10.3389/fendo.2019.00865
6. Damanhoury S, Newton AS, Rashid M, Hartling L, Byrne JLS, Ball GDC. Defining metabolically healthy obesity in children: a scoping review. Obes Rev (2018) 19:1476–91. doi: 10.1111/obr.12721
7. Urbina EM, Gidding SS, Bao W, Pickoff AS, Berdusis K, Berenson GS. Effect of body size, ponderosity, and blood pressure on left ventricular growth in children and young adults in the bogalusa heart study. Circulation (1995) 91:2400–6. doi: 10.1161/01.CIR.91.9.2400
8. Di Bonito P, Moio N, Scilla C, Cavuto L, Sibilio G, Forziato C, et al. Preclinical manifestations of organ damage associated with the metabolic syndrome and its factors in outpatient children. Atherosclerosis (2010) 213:611–5. doi: 10.1016/j.atherosclerosis.2010.09.017
9. Pieruzzi F, Antolini L, Salerno FR, Giussani M, Brambilla P, Galbiati S, et al. The role of blood pressure, body weight and fat distribution on left ventricular mass, diastolic function and cardiac geometry in children. J Hypertens (2015) 33:1182–92. doi: 10.1097/HJH.0000000000000552
10. Malcolm DD, Burns TL, Mahoney LT, Lauer RM. Factors affecting left ventricular mass in childhood: the muscatine study. Pediatrics (1993) 92:703–9.
11. Trieber FA, McCaffrey F, Pflieger K, Austin Raunikar R, Strong WB, Davis H. Determinants of left ventricular mass in normotensive children. Am J Hypertens (1993) 6:505–13. doi: 10.1093/ajh/6.6.505
12. Daniels SR, Kimball TR, Morrison JA, Khoury P, Witt S, Meyer RA. Effect of lean body mass, fat mass, blood pressure, and sexual maturation on left ventricular mass in children and adolescents: Statistical, biological, and clinical significance. Circulation (1995) 92:3249–54. doi: 10.1161/01.CIR.92.11.3249
13. Cole TJ, Bellizzi MC, Flegal KM, Dietz WH. Establishing a standard definition for child overweight and obesity worldwide: international survey. Bmj (2000) 320:1240–3. doi: 10.1136/bmj.320.7244.1240
14. Tanner JM. Growth and maturation during adolescence. Nutr Rev (1981) 39:43–55. doi: 10.1111/j.1753-4887.1981.tb06734.x
15. National high blood pressure education program working group on high blood pressure in children and adolescents. The fourth report on the diagnosis, evaluation, and treatment of high blood pressure in children and adolescents. Pediatrics (2004) 114:555–76. doi: 10.1542/peds.114.2.S2.555
16. Matthews DR, Hosker JP, Rudenski AS, Naylor BA, Treacher DF, Turner RC. Homeostasis model assessment: Insulin resistance and β-cell function from fasting plasma glucose and insulin concentrations in man. Diabetologia (1985) 28:412–9. doi: 10.1007/BF00280883
17. Lang RM, Bierig M, Devereux RB, Flachskampf FA, Foster E, Pellikka PA, et al. Recommendations for chamber quantification: A report from the American society of echocardiography’s guidelines and standards committee and the chamber quantification writing group, developed in conjunction with the European association of echocardiograph. J Am Soc Echocardiogr (2005) 18:1440–63. doi: 10.1016/j.echo.2005.10.005
18. Khoury PR, Mitsnefes M, Daniels SR, Kimball TR. Age-specific reference intervals for indexed left ventricular mass in children. J Am Soc Echocardiogr (2009) 22:709–14. doi: 10.1016/j.echo.2009.03.003
19. de Simone G, Mancusi C, Hanssen H, Genovesi S, Lurbe E, Parati G, et al. Hypertension in children and adolescents. Eur Heart J (2022), 43(35):3290–301. doi: 10.1093/eurheartj/ehac328
20. Shashaj B, Luciano R, Contoli B, Morino GS, Spreghini MR, Rustico C, et al. Reference ranges of HOMA-IR in normal-weight and obese young caucasians. Acta Diabetol (2016) 53:251–60. doi: 10.1007/s00592-015-0782-4
21. Moulin-Mares SRA, Zaniqueli D, Oliosa PR, Alvim RO, Bottoni JP, Mill JG. Uric acid reference values: Report on 1750 healthy Brazilian children and adolescents. Pediatr Res (2021) 89:1855–60. doi: 10.1038/s41390-020-01185-9
22. Blüher M. Are there still healthy obese patients? Curr Opin Endocrinol Diabetes Obes (2012) 19:341–6. doi: 10.1097/MED.0b013e328357f0a3
23. Blüher S, Schwarz P. Metabolically healthy obesity from childhood to adulthood - does weight status alone matter? Metabolism (2014) 63:1084–92. doi: 10.1016/j.metabol.2014.06.009
24. Reinehr T, Wolters B, Knop C, Lass N, Holl RW. Strong effect of pubertal status on metabolic health in obese children: A longitudinal study. J Clin Endocrinol Metab (2015) 100:301–8. doi: 10.1210/jc.2014-2674
25. Genovesi S, Antolini L, Orlando A, Gilardini L, Bertoli S, Giussani M, et al. Cardiovascular risk factors associated with the metabolically healthy obese (MHO) phenotype compared to the metabolically unhealthy obese (MUO) phenotype in children. Front Endocrinol (Lausanne) (2020) 11:27. doi: 10.3389/fendo.2020.00027
26. Zhao M, López-Bermejo A, Caserta CA, Medeiros CCM, Kollias A, Bassols J, et al. Metabolically healthy obesity and high carotid intima-media thickness in children and adolescents: International childhood vascular structure evaluation consortium. Diabetes Care (2019) 42:119–25. doi: 10.2337/dc18-1536
27. Genovesi S, Giussani M, Orlando A, Lieti G, Viazzi F, Parati G. Relationship between endothelin and nitric oxide pathways in the onset and maintenance of hypertension in children and adolescents. Pediatr Nephrol (2022) 37:537–45. doi: 10.1007/s00467-021-05144-2
28. Gallo G, Volpe M, Savoia C. Endothelial dysfunction in hypertension: Current concepts and clinical implications. Front Med (2022) 8:798958. doi: 10.3389/fmed.2021.798958
29. Antolini L, Giussani M, Orlando A, Nava E, Valsecchi MG, Parati G, et al. Nomograms to identify elevated blood pressure values and left ventricular hypertrophy in a paediatric population: American academy of pediatrics clinical practice vs. fourth Report/European society ofHypertension guidelines. J Hypertens (2019) 37:1213–22. doi: 10.1097/HJH.0000000000002069
30. Genovesi S, Salvi P, Nava E, Tassistro E, Giussani M, Desimone I, et al. Blood pressure and body weight have different effects on pulse wave velocity and cardiac mass in children. J Clin Med (2020) 9:1–12. doi: 10.3390/jcm9092954
31. Zhang N, Chen Y, Guo X, Sun G, Dai D, Sun Y. Metabolic abnormalities, but not metabolically healthy obesity, are associated with left ventricular hypertrophy. Hear Lung Circ (2017) 26:251–7. doi: 10.1016/j.hlc.2016.06.1212
Keywords: adolescents, children, left ventricular mass index, left ventricular hypertrophy, metabolically healthy obesity, HOMA-index, uric acid
Citation: Genovesi S, Tassistro E, Giussani M, Lieti G, Patti I, Orlando A, Montemerlo M, Antolini L and Parati G (2022) Association of obesity phenotypes with left ventricular mass index and left ventricular hypertrophy in children and adolescents. Front. Endocrinol. 13:1006588. doi: 10.3389/fendo.2022.1006588
Received: 29 July 2022; Accepted: 12 September 2022;
Published: 29 September 2022.
Edited by:
Bo Xi, Shandong University, ChinaReviewed by:
Costantino Mancusi, Federico II University Hospital, ItalyMario Bianchetti, University of Italian Switzerland, Switzerland
Cristiano Fava, University of Verona, Italy
Copyright © 2022 Genovesi, Tassistro, Giussani, Lieti, Patti, Orlando, Montemerlo, Antolini and Parati. This is an open-access article distributed under the terms of the Creative Commons Attribution License (CC BY). The use, distribution or reproduction in other forums is permitted, provided the original author(s) and the copyright owner(s) are credited and that the original publication in this journal is cited, in accordance with accepted academic practice. No use, distribution or reproduction is permitted which does not comply with these terms.
*Correspondence: Simonetta Genovesi, c2ltb25ldHRhLmdlbm92ZXNpQHVuaW1pYi5pdA==
†ORCID: SimonettaGenovesi, orcid.org/0000-0002-4699-4149