- 1Department of Endocrinology, National Health Commission Key Laboratory of Endocrinology, Peking Union Medical College Hospital, Chinese Academy of Medical Sciences and Peking Union Medical College, Beijing, China
- 2Department of Cardiology, Peking Union Medical College Hospital, Chinese Academy of Medical Sciences and Peking Union Medical College, Beijing, China
- 3Department of Endocrinology, Qilu Hospital, Cheeloo College of Medicine, Shandong University, Jinan, China
Background and objectives: Osteogenesis imperfecta (OI) is a rare disorder of abnormal production or modification of type I collagen, which is caused by mutations in COL1A1, COL1A2 or other genes. We investigate the cardiac abnormalities and its correlation with pathogenic mutations in OI children.
Methods: A cross-sectional comparative study was completed in a relatively large sample of OI children, who were matched in body surface area (BSA) with healthy controls. All echocardiography was performed by experienced cardiologists using Vivid 7 equipment (GE Medical Systems, Horton, Norway). The resting standard 12-lead electrocardiogram (ECG) were obtained in OI patients by FX-8600 machine. Skeletal phenotypes of OI patients were evaluated, including information of bone fractures, deformities, motility, and bone mineral density (BMD). Pathogenic mutations of OI were detected by a next-generation sequencing panel and confirmed by Sanger sequencing.
Results: A total of 69 OI children and 42 healthy children matched in BSA were enrolled. Abnormalities of echocardiography were found in 6 OI children, including enlarged left atrium (n=5), increased internal diameter of the left ventricle (n=1), who all carried the COL1A1 mutation. Mild regurgitation of mitral or tricuspid valves was observed in 26 OI patients. Abnormal ECG manifestations were found in 8 OI children, including deep Q wave, T wave change, premature ventricular complexes, short P-R interval, incomplete bundle branch block and high voltage of left ventricular. Compared with healthy controls, OI children had significant larger values in the main pulmonary artery (1.84 vs 1.60 cm, P < 0.01), left atrial diameter (2.58 vs 2.11 cm, P < 0.001), left ventricular internal dimension at end-diastolic (LVEDd) (3.85 vs 3.50 cm, P < 0.05) and lower left ventricular ejection fraction (LVEF) (68.40% vs 71.74%, P < 0.01). Moreover, OI patients with COL1A1 mutation tended to have greater main pulmonary artery, larger diameters of left atrial and LVEDd, and lower LVEF than healthy controls. COL1A1 mutation was correlated to dilated MPA (β = 1.557, P < 0.01), LAD (β = 3.915, P < 0.001), and LVEDd (β = 2.714, P < 0.01), and decreased LVEF (β = -3.249, P < 0.01).
Conclusions: Cardiovascular alterations were identified in OI children, including increased dimensions of the main pulmonary artery and left chamber, and low LVEF. The cardiovascular abnormalities seemed to be correlated to COL1A1 mutation and defects of type I collagen, which expanded our understandings of the cardiac phenotypes of OI children.
Introduction
Osteogenesis imperfecta (OI) is a heterogeneous hereditary connective tissue disorder caused by genetic defects in synthesis or modification of type I collagen, which is characterized by increased bone fragility, recurrent bone fractures and progressive bone deformity (1). OI is estimated to affect about 1/13,500-15,000 neonates (2). According to clinical severity, OI is clinically categorized into five subgroups, with type I being mild, type II lethal perinatally, type III the most severe among the survivors, type IV of intermediate severity, and type V with calcification of the interosseous membranes and/or hypertrophic callus (3). In recent years, the classification of OI has evolved with advances in molecular diagnosis which results in at least 20 forms of OI according to different gene mutations (4). Other collagen-rich tissues can also be affected in OI patients, including sclera, skin, heart and tendon, and so on (5). Extra-skeletal manifestations of OI include blue sclera, dentinogenesis imperfecta, joint hypermobility, hearing impairment, cardiovascular and pulmonary complications (6).
Recently, studies have indicated that morbidity and mortality of OI are closely associated with the abnormalities in cardiovascular and pulmonary systems (7, 8). Abnormalities in the cardiovascular system are found to be common in OI patients, including valvular disease, atrial fibrillation and heart failure, and the more severe the OI phenotype, the higher the risk of cardiovascular abnormalities (9, 10). As valves, tendinous cords, fibrous rings, and the cardiac septum of hearts are mainly composed of type I and III collagen, type I collagen contributes to the stiffer and rigid property of the myocardium (11). Therefore, a decrease of the collagen was associated with a more compliant ventricle, which results in increased LV diastolic internal diameters and volume (12). Moreover, the degree of collagen crosslinking was associated with cardiac function. A more compliant and less stiff collagen fibers would hinder the force development of the myocytes and disrupted the systolic function of hearts (13). Taken together, collagen fibers in the cardiac extra cellular matrix (ECM) contribute to keeping the rigidity and the architecture of hearts (12). However, few studies investigate the cardiovascular abnormalities of OI children, and it is unclear whether the cardiovascular abnormalities have a correlation with the genotypes of OI children.
The present study aimed to investigate the cardiovascular characteristics by echocardiography and electrocardiogram in a relatively large sample of OI children. Furthermore, we tried to analyze the relationship between cardiovascular alterations with skeletal phenotypes and pathogenic gene mutations in OI children.
Subjects and methods
This was a single-center, cross-sectional study conducted from January 2017 to January 2022 in Endocrinology Department of Peking Union Medical College Hospital (PUMCH). The patients were diagnosed as OI if they met the following criteria: (1) Patients had a history of more than one fracture under minor trauma, and with an age- and sex-adjusted BMD Z-score less than or equal to -1.0 at lumbar spine (LS) or femoral neck (FN). (2) Patients with BMD Z-scores less than or equal to -2.0 at LS or FN. (3) The diagnosis was confirmed by pathogenic mutation detection (14). Aside from abnormal hemodynamics, body size was an important affecting factors for evaluating cardiovascular structures (15). Therefore, healthy children with matched body surface areas (BSA) were included as the control group, who came to the clinic for physical examinations and completed the examinations of echocardiography. These controls had no history of cardiac diseases, skeletal diseases, chronic hepatic or kidney disorders, or other hereditary diseases. Informed consents were obtained from the parents or guardians of OI children and healthy controls.
For OI children and healthy controls, echocardiographic examinations were performed with Vivid I equipment (GE Medical Systems, Horton, Norway) using a 3.4-MHz multifrequency transducer. The echocardiography protocol consisted of complete cardiac examinations, which were performed by at least two experienced cardiologists at the same time to reduce the measurement error. The echocardiogram was interpreted by experienced cardiologists to minimize variability. The investigator did not know the clinical group of OI patients or controls. Proximal ascending aorta diameter (PAO), main pulmonary artery (MPA), right ventricular end-diastolic dimension (RVEDd), left atrial anteroposterior dimension (LAD), left ventricular end-diastolic dimension (LVEDd), interventricular septal wall thickness (IVST), LV posterior wall thickness (LVPWT), LV ejection fraction (LVEF) were measured following the Guideline of the American Society of Echocardiography (16). LAD, LVEDd, IVST, and LVPWT were measured in the parasternal long-axis view using M-mode echocardiography. LV mass (LVM) was calculated by the Devereux formula (15). RVEDd and LVEF were assessed using the modified biplane Simpson’s equation in the apical four-chamber and two-chamber views. All detectable regurgitations were included and divided into mild, moderate or severe following the recommendations of the American Society of Echocardiography (15). According to previous reports (9, 17–21), we defined the echocardiographic abnormalities as follows: (1) Increased dimensions of cardiac chambers or great arteries out of the normal range for the patient’s peer (Z-score > 2.0); (2) Moderate-severe valve regurgitation; (3) Other possible echocardiographic abnormalities (15). The resting standard 12-lead electrocardiogram (ECG) was obtained in OI patients by a FX-8600 machine (Fukuda sangyo, Japan). Resting heart rates, PR intervals, QRS duration, QRS axis, and QT intervals were examined and reviewed by experienced ECG analyzers. ECG pathologies included short PR interval, abnormal Q wave, high voltage, T wave abnormalities, the incomplete bundle branch block (RBBB) and premature ventricular complexes (PVC) (22, 23).
Demographic data of gender, age, height, and weight of OI children were collected. BSA were calculated using the Haycock formula (24). The skeletal phenotypes were evaluated, including sites and number of fractures, bone deformities, and movement ability. Serum levels of calcium (Ca), and phosphate (P) were measured by automated analyzers (ADVIA1800, Siemens, Germany). The serum level of 25-hydroxyvitamin D (25OHD) was measured using an automated electrochemiluminescence system (E170; Roche Diagnostics, Switzerland). The extra-skeletal phenotypes were evaluated carefully, including sclera, dentin, joints, and ligaments. The joint hypermobility was defined with Beighton scores (25). BMD at lumbar spine 2-4 (LS) and proximal hip of OI patients were measured by dual energy X-ray absorptiometry (DXA, Lunar Prodigy Advance, GE Healthcare, USA). BMD Z-scores of LS and FN were calculated on the basis of the reference data of BMD in Chinese and Asian children (26, 27). X-ray films of anteroposterior and lateral spines were completed and vertebral compression fracture was determined by Genant semi-quantitative method.
Pathogenic gene mutations of OI patients were identified by a next-generation sequencing (NGS) panel (Illumina HiSeq2000 platform, Illumina, Inc., San Diego, CA, USA), which covered currently known pathogenic genes of OI and 700 candidate genes of inherited bone disorders. The sequencing was performed with a coverage rate of 98.95% and an average depth of 200× on target regions in each of the chromosomes (14). To verify the gene mutation detected by NGS, polymerase chain reaction (PCR) and Sanger sequencing were conducted. According to pathogenic mutation genes, OI patients were divided into groups of COL1A1, COL1A2, or other rare gene mutations. According to the effects of pathogenic mutations on type I collagen synthesis, OI patients with COL1A1 or COL1A2 mutations were classified as (28): haplo-insufficiency which included mutations in COL1A1 introducing stop codons or leading to frameshifts mutations, structural change which included mutations in COL1A1 or COL1A2 leading to glycine substitutions in the triple-helical domain of the type I collagen, and the rest types of mutations were pooled together into the others group.
Statistical analysis
Continuous variables were presented as averages ± standard deviation for normal distribution (such as age, height, BSA, echocardiographic parameters and so on), or as median and ranges for abnormal distribution (such as number of fractures). Categorical variables were described as percentages, including immobility, bone deformities, blue sclera, and so on. Group differences in dichotomous variables were tested for significances using the chi-square test. Comparison of continuous variables between OI patients and controls were completed by two independent-sample t tests. Differences between subgroups of OI (for example, Sillence classification, and genotypes of OI) and the control group were evaluated using one-way analyses of variance. Simple linear regression model was applied to investigate which variables affected echocardiographic parameters in OI patients. The respective correlation between OI, Sillence types, OI genotypes, and echocardiographic measurements, which adjusted for age, gender, and BSA were analyzed by multiple linear regression analysis.
All tests were two-tailed and a P value less than 0.05 was considered statistically significant. Statistical analyses were performed using SPSS software of version 26.0 (SPSS Inc., Chicago, IL, USA).
Results
Baseline characteristics of the subjects
A total of 69 OI patients and 42 BSA-matched healthy children were included. Baseline characteristics were shown in Table 1. According to the Sillence classification, there were 42 patients with OI type I, 6 with type III, 17 with type IV, and 4 with type V. The mean age of OI patients was 9.2 ± 4.3 years at receiving echocardiographic examinations, and 41 (60.8%) patients were males. The control group had 28 boys and 14 girls, with the mean age of 8.7 ± 5.1 years. BSA were similar between OI patients and the control (1.12 versus 1.09 m2, P = 0.751).
Skeletal and extra-skeletal manifestations of OI children were displayed in Table 1. The mean age of the patients was 5.7 ± 3.9 years at diagnosis, with the median fractures number of 3. There were 25 (36.2%) patients with limited mobility, 21 (30.4%) with bone deformities, and 15 (21.7%) patients with scoliosis. Blue sclera (82.6%) and joints hypermobility (52.2%) were common extra-skeletal manifestations of OI children.
Echocardiographic and electrocardiogram abnormalities of OI patients
An atrial septal defect was found in 1 OI patient after birth, which self-healed at 2 years old, and no obvious heart disease was found in OI patients. However, we observed 6 (8.7%) OI patients with asymptomatic cardiac abnormalities during echocardiography examinations. Among them, 1 patient had an increased internal diameter of LV, and 5 patietns with enlarged LA. Detailed information of the 6 patients were displayed in Table 2, 2 of them with Sillence type IV, the other 4 patients with type I. All 6 patients carried the COL1A1 mutation, including 2 missense mutations (c. 2560G>A, p. Gly854Ser; c.1003G>A, p.Gly335Ser), 2 frameshift mutations (c.2739delT, p.Gly914Argfs*; c.1001delC, p.Pro334Leufs*), 1 nonsense mutation (c.3076C>T, p.Arg1026*), and 1 splice site mutation (c.751-2A>C). There were 26 (37.7%) OI patients with mild mitral or tricuspid valve regurgitation, which was higher than controls (4.8%, P < 0.001) and required no intervention. No moderate to severe valve regurgitation was found in OI children.
Interestingly, OI patients had larger internal dimensions of the main pulmonary artery, atrium and ventricle of heart than healthy controls (Table 3), with significant differences in MPA (1.84 versus 1.60 cm, P = 0.001), LAD (2.58 versus 2.11 cm, P < 0.001), and LVEDd (3.85 versus 3.50 cm, P = 0.004). LVEF was lower in OI patients than controls (68.40% versus 71.74%, P = 0.003), whereas no differences were observed in PAO, RVEDd, IVST, LVPWT, or LVM. These differences remained when OI patients were grouped according to the severity of OI, including mild (Sillence type I) and moderate-severe (Sillence type III/IV/V) patients. Compared with the control group, MPA (1.86 versus 1.60 cm, P = 0.004), LAD (2.61 versus 2.11 cm, P < 0.001) and LVEDd (3.90 versus 3.50 cm, P = 0.024) were larger and LVEF was lower, especially in mild OI patients. No obvious differences of echocardiographic measurements were found between mild and moderate-severe OI patients.
8 (11.6%) OI patients were detected with ECG abnormalities, including deep Q wave (n = 2), T wave change (n = 2), PVC (n = 1), short P-R interval (n = 1), incomplete RBBB (n = 1) and high voltage of LV (n = 1). 5 patients were Sillence type I, and the other 3 patients with OI type III, IV, and V, respectively. Pathogenic OI mutations of these patients were composed of COL1A1 (n = 5), COL1A2 (n = 1), P3H1 (n = 1) and IFITM5 (n = 1).
Relationship of echocardiographic alterations and genotypes of OI patients
Pathogenic variants were detected in 57 OI patients, 39 patients with COL1A1 mutation, 10 with COL1A2 mutation, and 8 with other rare genes mutations (IFITM5 n = 4, P3H1 n = 2, WNT1 n = 1, FKBP10 n = 1). Compared to BSA-matched controls, OI patients with COL1A1 mutation had greater internal diameters of MPA (1.83 versus 1.60 cm, P = 0.002), LAD (2.62 versus 2.11 cm, P < 0.001), LVEDd (3.90 versus 3.50 cm, P = 0.011), and lower LVEF (68.00% versus 71.74%, P = 0.008) (Table 4). After exclusion of the 6 OI patients with increased dimensions of cardiac chambers out of the normal range, parameters of heart chambers still increased in the COL1A1 mutation group than control group. However, the echocardiographic parameters of patients with COL1A2 mutation or other rare gene mutations showed no significant differences from control group. No differences were detected between any two of the three OI subgroups with different genotypes.
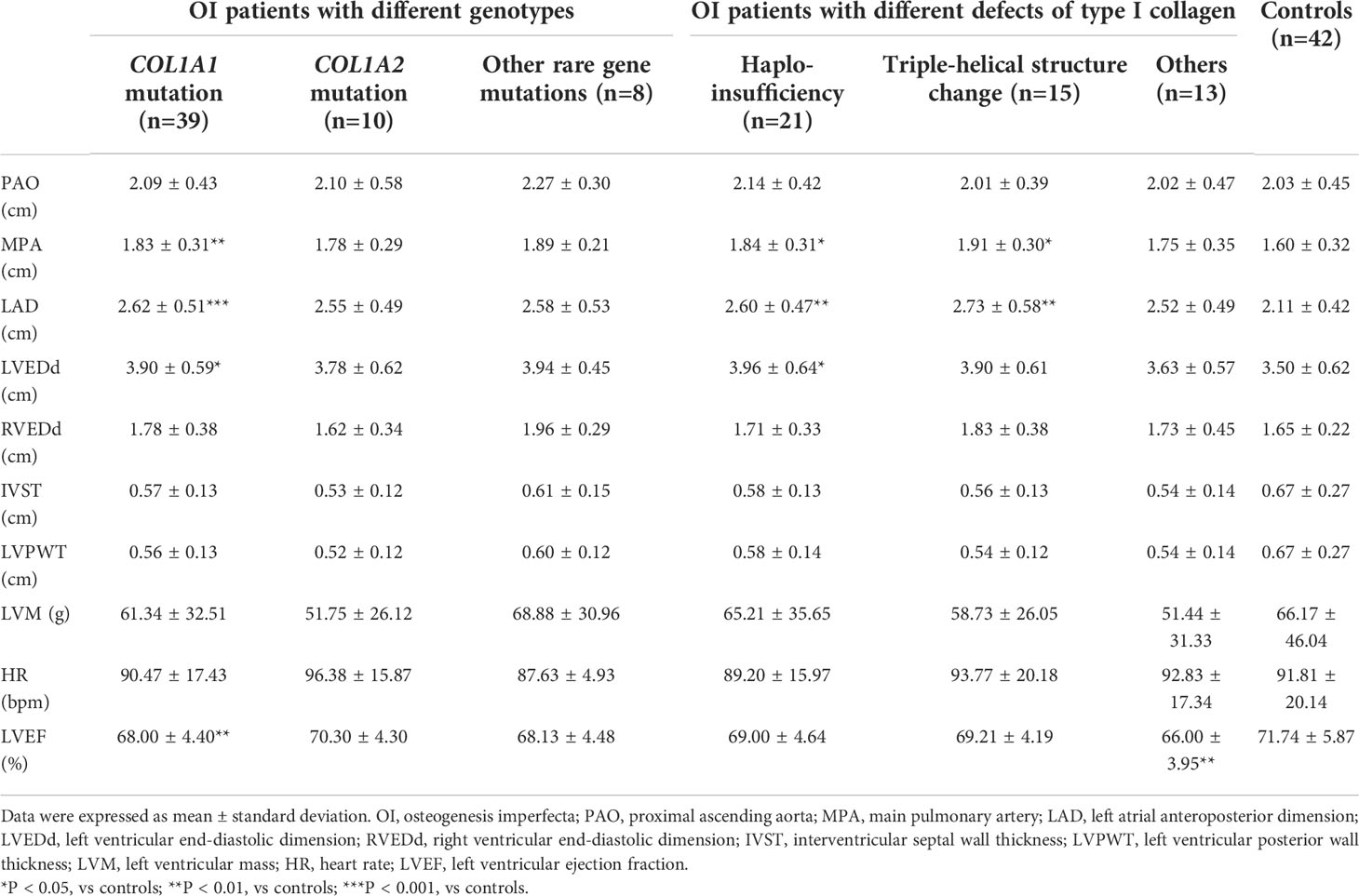
Table 4 Echocardiographic parameters in OI patients with different genotypes and defects of type I collagen compared with controls.
According to the effects of gene mutation on synthesis of type I collagen, OI patients with COL1A1 or COL1A2 mutation were further divided into three subgroups, including haplo-insufficiency of type I collagen (n = 21), triple-helical structure change (n = 15), and others (n = 13). Patients with haplo-insufficiency of type I collagen had larger MPA (1.84 versus 1.60 cm, P = 0.039), LAD (2.60 versus 2.11 cm, P = 0.002), and LVEDd (3.96 versus 3.50 cm, P = 0.037) than BSA-matched controls. Patients with structure change of type I collagen had larger diameters in MPA (1.91 versus 1.60 cm, P = 0.011), and LAD (2.73 versus 2.11 cm, P = 0.006) than control group (Table 4). While LVEF only showed significant difference in the group with other defects of type I collagen from control group (66.00% versus 71.74%, P = 0.008).
As shown in Table 5, age, male, and BSA had significantly positive correlation with echocardiographic parameters, except for LVEF. Diagnosis of OI showed a significant relationship with dilated MPA (β = 2.341, P < 0.001), LAD (β = 4.754, P < 0.001), LVEDd (β = 3.532, P = 0.004) and lower LVEF (β =-3.341, P = 0.001). COL1A1 mutation had a correlation with larger MPA (β = 1.565, P = 0.022), LAD (β = 3.796, P < 0.001), LVEDd (β = 2.807, P = 0.031) and lower LVEF (β = -3.269, P = 0.003). This correlation was not found in patients with COL1A2 or other rare genes mutations. After adjusted for age, gender, and BSA, OI, COL1A1 mutation was still significantly correlated with larger internal dimensions of MPA (β = 1.557, P = 0.001), LAD (β = 3.915, P < 0.001), LVEDd (β = 2.714, P = 0.001), and lower LVEF (β = -3.249, P = 0.004) (Table 6).
Discussion
In this relatively large sample study, we found OI children had cardiovascular alterations, including dilated main pulmonary artery, larger internal dimensions of left hearts, and lower LVEF. These changes in cardiac structure and function seemed to be more common in patients carrying COL1A1 mutation.
As we know, heart and blood vessels are rich in collagen, of which approximately 85% is the type I collagen, which is also the important component of valves, tendinous cords, fibrous rings, and the interventricular septum of hearts (9). Collagen fibers contribute both to maintaining the architecture and rigidity of the heart and artery (29). The reduction and disorganization of type I collagen might not only change the structure, but also affect the function of the heart (10). OI is a complex disease in which the quantity or the structure of collagen could be changed, therefore, hearts and main pulmonary arteries might be involved in OI patients (5). A previous study revealed that the heart and main pulmonary artery had decreased numbers and disorganization of collagens in perinatal lethal OI type II patients (30). In a small sample study, OI children were found to have greater dimensions of the main aorta and LVEDd than control group, but without significant change in heart function (31). Compare with control group, OI children exhibited larger LV internal dimensions and dilated main pulmonary arteries (32, 33). Our study confirmed the alterations of heart and aorta in OI children, including larger internal diameters of MPA and left heart chambers, with lower LVEF than BSA-matched healthy children. Moreover, the systolic function of the LV could be considered as its ability to generate pressure and to evacuate left ventricular blood volume. Although the smaller LVEF had no predominant clinically significance at the current stage of children and adolescents, we speculated that the cardiac function of OI patients may be changed or even be damaged as time goes on, and the patient’s cardiac function was worthy of long-term observation and follow-up.
Previous studies analyzed the correlation of cardiovascular changes with the severity of OI, and results were inconsistent. A study in 58 OI children found no cardiac abnormalities in type I OI patients, but cardiac alterations existed in type III OI children, including increased aortic root diameter and LVPW thickness (34). A recent comparative study showed that mild and moderate-severe OI patients both had larger internal diameters of the main pulmonary artery and left heart than control group, but only the moderate-severe group had lower LVEF than control group (33). In our study, echocardiographic abnormalities existed both in mild and moderate-severe OI patients, and there were no significant differences in echocardiographic measurements between these two subgroups. We speculated the divergence of the relationship between the cardiac abnormalities and OI Sillence classification may result not only from the complexity of etiology and phenotypes, but also from the insufficient sample size of OI cohort.
Few studies have evaluated the relationship of cardiac abnormalities and pathogenic gene mutations in OI patients. In this study, we found for the first time that COL1A1 mutation of OI was correlated with larger diameters of the main pulmonary artery, larger left heart chambers and lower LVEF. We found OI children with haplo-insufficiency and triple-helical structure change of type I collagen both tended to have dilated MPA and LAD, and only patients with haplo-insufficiency of type I collagen had increased internal diameters of LVEDd than normal controls. In Aga2 mouse model with reduced COL1A1 production, greater LVEDd and lower LVEF than wild-type mouse was found (35). Moreover, in Oim mouse model with defects of α2 chains of type I collagen, a disturbance in the ascending aorta was found, but without significant changes in LV structures and functions (36, 37). As type I collagen is a heterotrimer of two α1 and one α2 chains (5), we speculated that the difference of echocardiographic abnormalities might be correlated to the different changes in the structure and metabolism of type I collagen induced by various pathogenic gene mutations.
Moreover, studies reported that the cardiac valve disease was common in adult OI patients (8, 20, 38), which had not been well investigated in OI children. In a cohort of 99 OI adults and 45 healthy controls, moderate mitral regurgitation was found in 7.1% of OI patients, none in control group (39). In this study, we found OI children had significant higher percentage of mild mitral or triple valve regurgitation than control group, which was worthy of a long-term follow-up. We did not find moderate to severe valvular prolapse or regurgitation in this OI cohort, which was consistent with other studies in OI children (33). This difference of detection rate of valvular abnormalities between OI children and adults indicated that cardiovascular diseases might develop with aging in OI patients, so it was necessary to evaluate the cardiovascular structure and function of OI patients during the long-term follow-up.
We found some unspecific ECG changes in OI children, such as T-wave changes, deep Q waves, RBBB and so on. Another study also found a higher risk of unspecific ECG changes in a cohort of OI adults than normal controls (17). In this study, we found 6 OI patients with echocardiographic abnormalities, who had no obvious ECG abnormalities. We did not find the relationship between abnormalities of the echocardiography and ECG in this OI cohort. However, since the sample size of this cohort was relatively small and ECG recordings of healthy controls were not collected, it was difficult to determine whether the abnormal echocardiogram of OI patients was related to the abnormal ECG. The Framingham study indicated that asymptomatic subjects with nonspecific electrocardiographic abnormalities of ST and T-wave would have an increased risk to develop cardiovascular diseases (40). Although it is unclear the relationship of unspecific ECG changes with cardiovascular diseases, the cardiovascular changes of OI patients were still worthy of a long-term follow-up.
In this study, the cardiac structure and function of OI children were evaluated in detail, and their relationship with the clinical severity and pathogenic gene mutations were comprehensively analyzed for the first time in Asian OI patients, which expanded the understanding of the extraosseous manifestations of OI patients. However, there were some limitations in this study. We could not rule out the influence of the abnormal physical proportions of OI patients on the echocardiographic diameters and ECG recordings. Baseline characteristics and ECGs of the control group were not collected, so it was difficult to elucidate the clinical significance of the unspecific ECG changes in OI children. Since the number of different OI genotypes were disproportionate, the strength of this sub-analysis may be limited. Additionally, as the sample size was relatively small, it was difficult to investigate the effects of different gene mutations on cardiac structure and function of OI patients, especially for patients with the rare autosomal recessive gene mutations. Further researches are required to elucidate the influence of various mutation genes of OI on the cardiovascular system.
Conclusion
Cardiovascular abnormalities are not uncommon in children with OI, which include larger dimensions of the left atrial and ventricle, dilated main pulmonary artery, and reduced left ventricular ejection fraction than healthy control. The cardiovascular system involvement seems to be more frequent in OI children with COL1A1 mutation, and cardiovascular changes are correlated with the quantity and structural changes of type I collagen. Therefore, dynamic evaluation of cardiovascular abnormalities in OI patients has important clinical significance.
Data availability statement
The datasets for this article are not publicly available due to concerns regarding participant/patient anonymity. Requests to access the datasets should be directed to the corresponding authors. The datasets presented in this article are not readily available because No access to raw dataset of NGS is allowed other than the Beijing Genomics institution in charge of NGS. Requests to access the datasets should be directed to https://www.genomics.cn/contact.html.
Ethics statement
The studies involving human participants were reviewed and approved by ethic committee of Peking Union Medical College Hospital. Written informed consent to participate in this study was provided by the participants’ legal guardian/next of kin.Written informed consent was obtained from the individual(s), and minor(s)’ legal guardian/next of kin, for the publication of any potentially identifiable images or data included in this article.
Author contributions
DZ carried out the data collection, molecular genetic studies, the sequence alignment, performed statistical analysis and drafted the manuscript. JH contributed to data collection and genetic detection. JL, and YL contributed to review and edit the manuscript. QZ, OW, YJ, XX, and WX contributed to review the manuscript. ML designed the research, interpreted the data, and revised the manuscript. All authors contributed to the article and approved the submitted version.
Funding
This study is supported by National Key R&D Program of China (2018YFA0800801, 2021YFC2501704), CAMS Innovation Fund for Medical Sciences(CIFMS)(2021-I2M-C&T-B-007, 2021-I2M-1-051), National Natural Science Foundation of China (No.81873668, 82070908), and Beijing Natural Science Foundation (7202153).
Acknowledgments
We appreciate the patients and their families for their participation into this study. And sincerely thank all healthy children and their parents for offering the echocardiographic profiles voluntarily.
Conflict of interest
The authors declare that the research was conducted in the absence of any commercial or financial relationships that could be construed as a potential conflict of interest.
Publisher’s note
All claims expressed in this article are solely those of the authors and do not necessarily represent those of their affiliated organizations, or those of the publisher, the editors and the reviewers. Any product that may be evaluated in this article, or claim that may be made by its manufacturer, is not guaranteed or endorsed by the publisher.
References
1. Jovanovic M, Guterman-Ram G, Marini JC. Osteogenesis imperfecta: mechanisms and signaling pathways connecting classical and rare OI types. Endocr Rev (2022) 43(1):61–90. doi: 10.1210/endrev/bnab017
2. Garibaldi N, Besio R, Dalgleish R, Villani S, Barnes AM, Marini JC, et al. Dissecting the phenotypic variability of osteogenesis imperfecta. Dis Model Mech (2022) 15(5):dmm049398. doi: 10.1242/dmm.049398
3. Tournis S, Dede AD. Osteogenesis imperfecta - a clinical update. Metabolism (2018) 80:27–37. doi: 10.1016/j.metabol.2017.06.001
4. Chetty M, Roomaney IA, Beighton P. The evolution of the nosology of osteogenesis imperfecta. Clin Genet (2021) 99(1):42–52. doi: 10.1111/cge.13846
5. Bacakova L, Novotna K, Hadraba D, Musilkova J, Slepicka P, Beran M, et al. Influence of biomimetically mineralized collagen scaffolds on bone cell proliferation and immune activation. Polymers (Basel) (2022) 14(3):602. doi: 10.3390/polym14030602
6. Marom R, Rabenhorst BM, Morello R. Osteogenesis imperfecta: an update on clinical features and therapies. Eur J Endocrinol (2020) 183(4):R95–106. doi: 10.1530/EJE-20-0299
7. Storoni S, Treurniet S, Micha D, Celli M, Bugiani M, van den Aardweg JG, et al. Pathophysiology of respiratory failure in patients with osteogenesis imperfecta: a systematic review. Ann Med (2021) 53(1):1676–87. doi: 10.1080/07853890.2021.1980819
8. Folkestad L. Mortality and morbidity in patients with osteogenesis imperfecta in Denmark. Dan Med J (2018) 65(4):B5454. doi: 10.1080/07853890.2021.1980819
9. Ashournia H, Johansen FT, Folkestad L, Diederichsen AC, Brixen K. Heart disease in patients with osteogenesis imperfecta - a systematic review. Int J Cardiol (2015) 196:149–57. doi: 10.1016/j.ijcard.2015.06.001
10. Folkestad L, Hald JD, Gram J, Langdahl BL, Hermann AP, Diederichsen AC, et al. Cardiovascular disease in patients with osteogenesis imperfecta - a nationwide, register-based cohort study. Int J Cardiol (2016) 225:250–7. doi: 10.1016/j.ijcard.2016.09.107
11. Ristori T, Bouten CVC, Baaijens FPT, Loerakker S. Predicting and understanding collagen remodeling in human native heart valves during early development. Acta Biomater (2018) 80:203–16. doi: 10.1016/j.actbio.2018.08.040
12. Schelbert EB, Butler J, Diez J. Why clinicians should care about the cardiac interstitium. JACC Cardiovasc Imaging (2019) 12(11):2305–18. doi: 10.1016/j.jcmg.2019.04.025
13. van den Berg NWE, Neefs J, Kawasaki M, Nariswari FA, Wesselink R, Fabrizi B, et al. Extracellular matrix remodeling precedes atrial fibrillation: results of the PREDICT-AF trial. Heart Rhythm. (2021) 18(12):2115–25. doi: 10.1016/j.hrthm.2021.07.059
14. Liu Y, Asan, Ma D, Lv F, Xu X, Wang J, et al. Gene mutation spectrum and genotype-phenotype correlation in a cohort of Chinese osteogenesis imperfecta patients revealed by targeted next generation sequencing. Osteoporos Int (2017) 28(10):2985–95. doi: 10.1007/s00198-017-4143-8
15. Lopez L, Colan SD, Frommelt PC, Ensing GJ, Kendall K, Younoszai AK, et al. Recommendations for quantification methods during the performance of a pediatric echocardiogram: a report from the pediatric measurements writing group of the American society of echocardiography pediatric and congenital heart disease council. J Am Soc Echocardiogr (2010) 23(5):465–95. doi: 10.1016/j.hlc.2013.05.640
16. Lang RM, Badano LP, Mor-Avi V, Afilalo J, Armstrong A, Ernande L, et al. Recommendations for cardiac chamber quantification by echocardiography in adults: an update from the American society of echocardiography and the European association of cardiovascular imaging. J Am Soc Echocardiogr (2015) 28(1):1–39. doi: 10.1016/j.echo.2014.10.003
17. Radunovic Z, Steine K. Prevalence of cardiovascular disease and cardiac symptoms: left and right ventricular function in adults with osteogenesis imperfecta. Can J Cardiol (2015) 31(11):1386–92. doi: 10.1016/j.cjca.2015.04.016
18. Zoghbi WA, Adams D, Bonow RO, Enriquez-Sarano M, Foster E, Grayburn PA, et al. Recommendations for noninvasive evaluation of native valvular regurgitation: a report from the american society of echocardiography developed in collaboration with the society for cardiovascular magnetic resonance. J Am Soc Echocardiogr (2017) 30(4):303–71. doi: 10.1016/j.echo.2017.01.007
19. Vandersteen AM, Lund AM, Ferguson DJ, Sawle P, Pollitt RC, Holder SE, et al. Four patients with sillence type I osteogenesis imperfecta and mild bone fragility, complicated by left ventricular cardiac valvular disease and cardiac tissue fragility caused by type I collagen mutations. Am J Med Genet A (2014) 164A(2):386–91. doi: 10.1002/ajmg.a.36285
20. Soma K, Abe H, Takeda N, Shintani Y, Takazawa Y, Kojima T, et al. Myocardial involvement in patients with osteogenesis imperfecta. Int Heart J (2012) 53(1):75–7. doi: 10.1536/ihj.53.75
21. Lamanna A, Fayers T, Clarke S, Parsonage W. Valvular and aortic diseases in osteogenesis imperfecta. Heart Lung Circ (2013) 22(10):801–10. doi: 10.1016/j.hlc.2013.05.640
22. Surawicz B, Childers R, Deal BJ, Gettes LS, Bailey JJ, Gorgels A, et al. AHA/ACCF/HRS recommendations for the standardization and interpretation of the electrocardiogram: part III: intraventricular conduction disturbances: a scientific statement from the American heart association electrocardiography and arrhythmias committee, council on clinical cardiology; the American college of cardiology foundation; and the heart rhythm society. J Am Coll Cardiol (2009) 53(11):976–81. doi: 10.1016/j.jacc.2008.12.013
23. Rautaharju PM, Surawicz B, Gettes LS, Bailey JJ, Childers R, Deal BJ, et al. AHA/ACCF/HRS recommendations for the standardization and interpretation of the electrocardiogram: part IV: the ST segment, T and U waves, and the QT interval: a scientific statement from the American heart association electrocardiography and arrhythmias committee, council on clinical cardiology; the American college of cardiology foundation; and the heart rhythm society. J Am Coll Cardiol (2009) 53(11):982–91. doi: 10.1016/j.jacc.2008.12.014
24. Sigurdsson TS, Lindberg L. Six commonly used empirical body surface area formulas disagreed in young children undergoing corrective heart surgery. Acta Paediatr (2020) 109(9):1838–46. doi: 10.1111/apa.15208
25. Martínez-Sebastián C, Molina-García C, Ramos-Petersen L, Gijón-Noguerón G, Evans AM. Evaluation of the relationship between lower limb hypermobility and ankle muscle strength in a pediatric population: protocol for a cross sectional study. Int J Environ Res Public Health (2022) 19(12):7264. doi: 10.3390/ijerph19127264
26. Xu H, Zhao Z, Wang H, Ding M, Zhou A, Wang X, et al. Bone mineral density of the spine in 11,898 Chinese infants and young children: a cross-sectional study. PloS One (2013) 8(12):e82098. doi: 10.1371/journal.pone.0082098
27. Khadilkar AV, Sanwalka NJ, Chiplonkar SA, Khadilkar VV, Mughal MZ. Normative data and percentile curves for dual energy X-ray absorptiometry in healthy Indian girls and boys aged 5-17 years. Bone (2011) 48(4):810–9. doi: 10.1016/j.bone.2010.12.013
28. Li LJ, Lyu F, Song YW, Wang O, Jiang Y, Xia WB, et al. Genotype-phenotype relationship in a large cohort of osteogenesis imperfecta patients with COL1A1 mutations revealed by a new scoring system. Chin Med J (Engl) (2019) 132(2):145–53. doi: 10.1097/CM9.0000000000000013
29. Ezeani M, Noor A, Alt K, Lal S, Donnelly PS, Hagemeyer CE, et al. Collagen-targeted peptides for molecular imaging of diffuse cardiac fibrosis. J Am Heart Assoc (2021) 10(18):e022139. doi: 10.1161/JAHA.121.022139
30. Wheeler VR, Cooley NR Jr, Blackburn WR. Cardiovascular pathology in osteogenesis imperfecta type IIA with a review of the literature. Pediatr Pathol (1988) 8(1):55–64. doi: 10.3109/15513818809022279
31. Karamifar H, Ilkhanipoor H, Ajami G, Karamizadeh Z, Amirhakimi G, Shakiba AM. Cardiovascular involvement in children with osteogenesis imperfecta. Iran J Pediatr (2013) 23(5):513–8.
32. Rush ET, Li L, Goodwin JL, Kreikemeier RM, Craft M, Danford DA, et al. Echocardiographic phenotype in osteogenesis imperfecta varies with disease severity. Heart (2017) 103(6):443–8. doi: 10.1136/heartjnl-2016-310099
33. Pinheiro BS, Barrios PM, Souza LT, Félix TM. Echocardiographic study in children with osteogenesis imperfecta. Cardiol Young (2020) 30(10):1490–5. doi: 10.1017/S1047951120002474
34. Vetter U, Maierhofer B, Müller M, Lang D, Teller WM, Brenner R, et al. Osteogenesis imperfecta in childhood: cardiac and renal manifestations. Eur J Pediatr (1989) 149(3):184–7. doi: 10.1007/BF01958277
35. Thiele F, Cohrs CM, Flor A, Lisse TS, Przemeck GK, Horsch M, et al. Cardiopulmonary dysfunction in the osteogenesis imperfecta mouse model Aga2 and human patients are caused by bone-independent mechanisms. Hum Mol Genet (2012) 21(16):3535–45. doi: 10.1093/hmg/dds183
36. Pfeiffer BJ, Franklin CL, Hsieh FH, Bank RA, Phillips CL. Alpha 2(I) collagen deficient oim mice have altered biomechanical integrity, collagen content, and collagen crosslinking of their thoracic aorta. Matrix Biol (2005) 24(7):451–8. doi: 10.1016/j.matbio.2005.07.001
37. Weis SM, Emery JL, Becker KD, McBride DJ Jr, Omens JH, McCulloch AD. Myocardial mechanics and collagen structure in the osteogenesis imperfecta murine (oim). Circ Res (2000) 87(8):663–9. doi: 10.1161/01.res.87.8.663
38. Najib MQ, Schaff HV, Ganji J, Lee HR, Click RL, Miller DC, et al. Valvular heart disease in patients with osteogenesis imperfecta. J Card Surg (2013) 28(2):139–43. doi: 10.1111/jocs.12064
39. Radunovic Z, Wekre LL, Steine K. Right ventricular and pulmonary arterial dimensions in adults with osteogenesis imperfecta. Am J Cardiol (2012) 109(12):1807–13. doi: 10.1016/j.amjcard.2012.01.402
Keywords: osteogenesis imperfecta, cardiovascular abnormalities, gene mutation, type I collagen, echocardiography
Citation: Zhao D, Liu Y, Liu J, Hu J, Zhang Q, Wang O, Jiang Y, Xia W, Xing X and Li M (2022) Cardiovascular abnormalities and its correlation with genotypes of children with osteogenesis imperfecta. Front. Endocrinol. 13:1004946. doi: 10.3389/fendo.2022.1004946
Received: 27 July 2022; Accepted: 30 September 2022;
Published: 20 October 2022.
Edited by:
Janet L Crane, Johns Hopkins University, United StatesReviewed by:
Peng Xue, Third Hospital of Hebei Medical University, ChinaHao Zhang, Shanghai Jiao Tong University, China
Angela VERARDO, RWJBarnabas Health, United States
Copyright © 2022 Zhao, Liu, Liu, Hu, Zhang, Wang, Jiang, Xia, Xing and Li. This is an open-access article distributed under the terms of the Creative Commons Attribution License (CC BY). The use, distribution or reproduction in other forums is permitted, provided the original author(s) and the copyright owner(s) are credited and that the original publication in this journal is cited, in accordance with accepted academic practice. No use, distribution or reproduction is permitted which does not comply with these terms.
*Correspondence: Mei Li, bGltZWlsemhAc2luYS5jb20=