- 1New York University (NYU) Grossman School of Medicine, NYU Langone Health, New York, NY, United States
- 2Department of Medicine, NYU Langone Health, New York, NY, United States
Prolactin is a polypeptide hormone that is well known for its role in reproductive physiology. Recent studies highlight its role in neurohormonal appetite regulation and metabolism. Elevated prolactin levels are widely associated with worsening metabolic disease, but it appears that low prolactin levels could also be metabolically unfavorable. This review discusses the pathophysiology of prolactin related metabolic changes, and the less commonly recognized effects of prolactin on adipose tissue, pancreas, liver, and small bowel. Furthermore, the effect of dopamine agonists on the metabolic profiles of patients with hyperprolactinemia are discussed as well.
Prolactin physiology
Prolactin is a polypeptide hormone best known for its role in lactation and breast development. It is primarily produced by lactotrophs in the anterior pituitary, which make up 15-20% of the total number of pituitary cells in both males and females (1, 2). Prolactin is regulated via negative feedback from dopamine and also acts in a self-regulatory manner by promoting dopamine release (3, 4). Dopamine is secreted by neurons in the arcuate nucleus of the hypothalamus and acts on dopamine receptors (D2) in the anterior pituitary to inhibit prolactin synthesis and secretion. It was previously thought that prolactin was only produced by lactotrophs in the anterior pituitary, but recent literature indicates prolactin is also produced by the central nervous system (CNS), adipose tissue, immune system, uterus, mammary glands, and prostate gland (3, 5). The presence of prolactin receptors in nearly all organs suggests complex systemic effects of prolactin far beyond its role in reproduction, reclassifying it as a unique circulating hormone with autocrine, paracrine, and endocrine effects.
During pregnancy and lactation, prolactin plays an important role in systemic signaling. It leads to maternal adaptations that help meet the increased metabolic demands of pregnancy (6, 7). High prolactin levels promote food intake, weight gain, leptin resistance and the insulin resistant state seen in pregnancy which allows glucose to be diverted to the developing fetus (1, 7–11). These metabolic effects of prolactin are crucial to maintain metabolic homeostasis of the mother-fetus unit. This review summarizes the effects of prolactin on metabolism in the non-pregnant state.
Prolactinoma
While there are many causes of elevated prolactin levels, prolactinomas are the leading cause of hyperprolactinemia (Table 1) (17, 20). Prolactinomas arise from lactotroph cells of the anterior pituitary and make up about 40% of all pituitary adenomas. Ninety percent of these tumors are less than 1 cm and are categorized as microadenomas, and the other 10% are greater than 1 cm and are considered to be macroadenomas (3, 12). Although older guidelines suggest higher cut-offs (>250 ng/mL) to diagnosis prolactinomas, newer studies highlight the ratio of prolactin to tumor volume and lower levels, between 55-94 ng/mL, may also suggest prolactin secreting pituitary tumors (13, 35–37). This distinction is important to keep in mind when differentiating prolactin secreting tumors from other causes of hyperprolactinemia.
Prolactinomas are most often seen in female patients between the ages of 20 to 40. Young females may experience symptoms of galactorrhea and amenorrhea. Males may experience impotence and erectile dysfunction, but often present later in their course with headaches and visual deficit secondary to mass effects from larger tumors (3). Regardless of the cause, hyperprolactinemia is strongly correlated with widespread metabolic alterations including obesity, insulin resistance, dyslipidemia, non-alcohol fatty liver disease (NAFLD) and endothelial dysfunction (Figure 1) (6, 38–40).
The pathophysiology of hyperprolactinemia and infertility is complex; there is significant crossover of the neuropeptides involved in the regulation of fertility and metabolism. In women, elevated prolactin levels are thought to cause infertility by suppressing pulsatile gonadotropin releasing hormone (GnRH) release that is necessary to induce luteinizing hormone (LH) and follicle stimulating hormone (FSH) secretion and ovulation (41). The pulsatile release of GnRH is stimulated by a neuropeptide called kisspeptin (17). Kisspeptin is secreted by the kisspeptin, neurokinin B, and dynorphin neurons (KNDy) in the hypothalamus. KNDy neurons express prolactin receptors and hyperprolactinemia causes a decrease in kisspeptin secretion hence decreasing pulsatile GnRH release (Figure 2) (41). The therapeutic role of kisspeptin in patients with hyperprolactinemia-induced infertility has recently been studied. Kisspeptin may be used to stimulate GnRH secretion and restore ovarian function (42, 43).
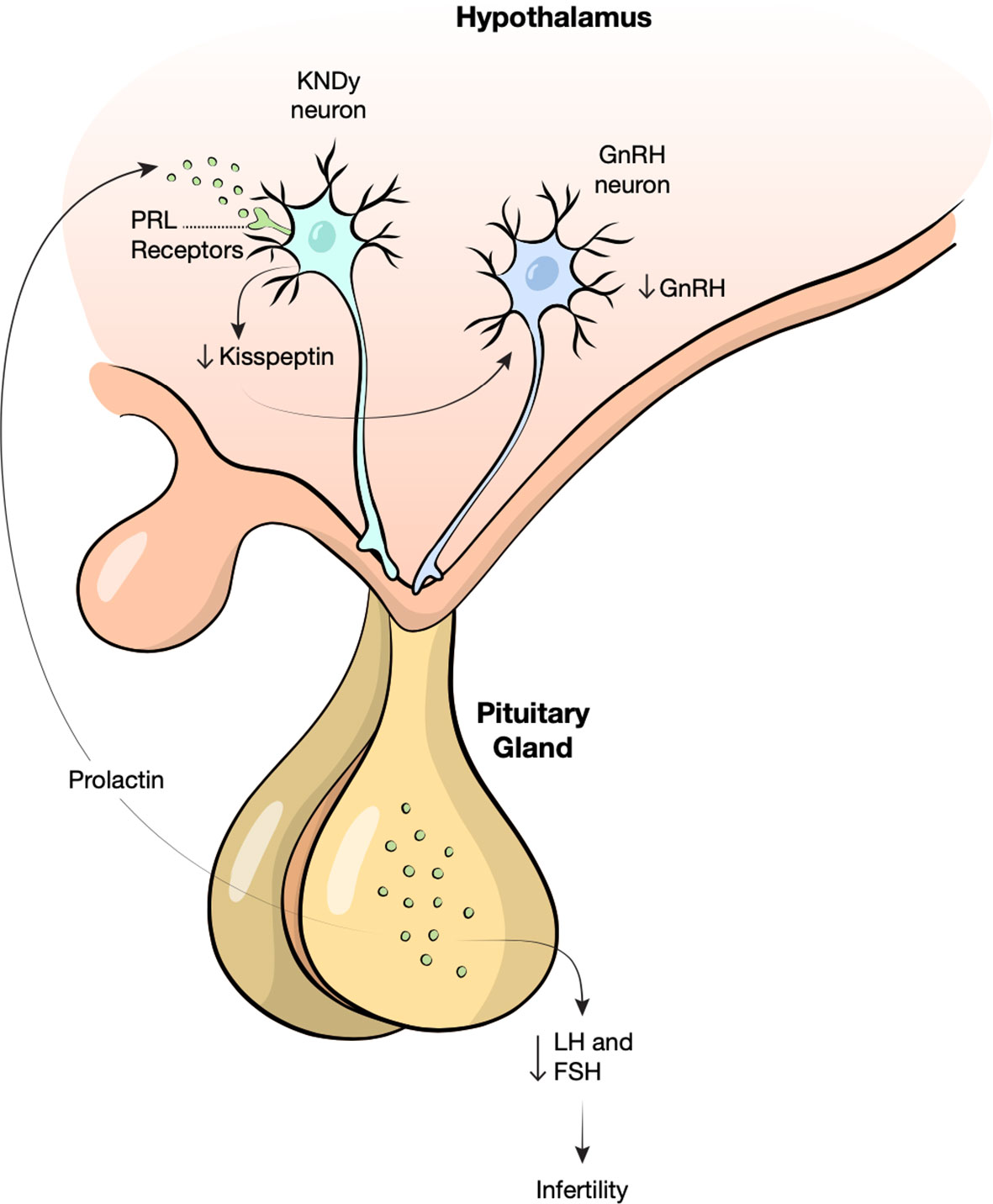
Figure 2 Proposed mechanism of hyperprolactinemia induced infertility. Prolactin (PRL) binds receptors on kisspeptin, neurokinin B, and dynorphin neurons (KNDy) in the hypothalamus, decreasing the release of Kisspeptin. Low kisspeptin may cause loss of gonadotropic releasing hormone (GnRH) pulsatility resulting in decreased follicle stimulating hormone (FSH) and luteinizing hormone (LH) and therefore infertility.
Kisspeptin also exerts metabolic effects both directly via receptors in the brain, pancreas and adipose tissue and indirectly though the GnRH reproductive axis pathway (44). Kisspeptin synthesis and release is regulated by metabolic factors, such that it is only secreted in situations of energy surplus to sustain reproduction (45, 46). Several animal studies support the theory that kisspeptin may be involved in regulating insulin secretion and lipid metabolism, suggesting there may be a role of kisspeptin in the treatment of these metabolic derangements (47–49).
Metabolic effects of prolactin
Neurohormonal appetite regulation
Prolactin plays a significant role in the neurologic regulation of appetite and satiety (Figure 3). The arcuate nucleus of the hypothalamus, and specifically, anorectic pro-opiomelanocortin (POMC), orexigenic neuropeptide Y (NYP), and agouti-related peptide neurons (AgRP) are key players in the regulation of appetite (6, 50). The arcuate nucleus integrates signals from circulating nutrients and hormones such as leptin and insulin to maintain metabolic homeostasis (51). Leptin is an adipokine secreted in direct proportion to the both the size and quantity of adipocytes in the body; it suppresses NYP and AgRP neurons at the arcuate nucleus, thereby suppressing appetite (52, 53). Prolactin may influence the hypothalamic appetite pathway in different ways. Though the molecular mechanism remains elusive, prolactin may exert influence on the arcuate nucleus indirectly by causing leptin insensitivity (54). Chronically elevated prolactin can create a pseudopregnancy state with resultant hyperphagia and weight gain (55, 56).
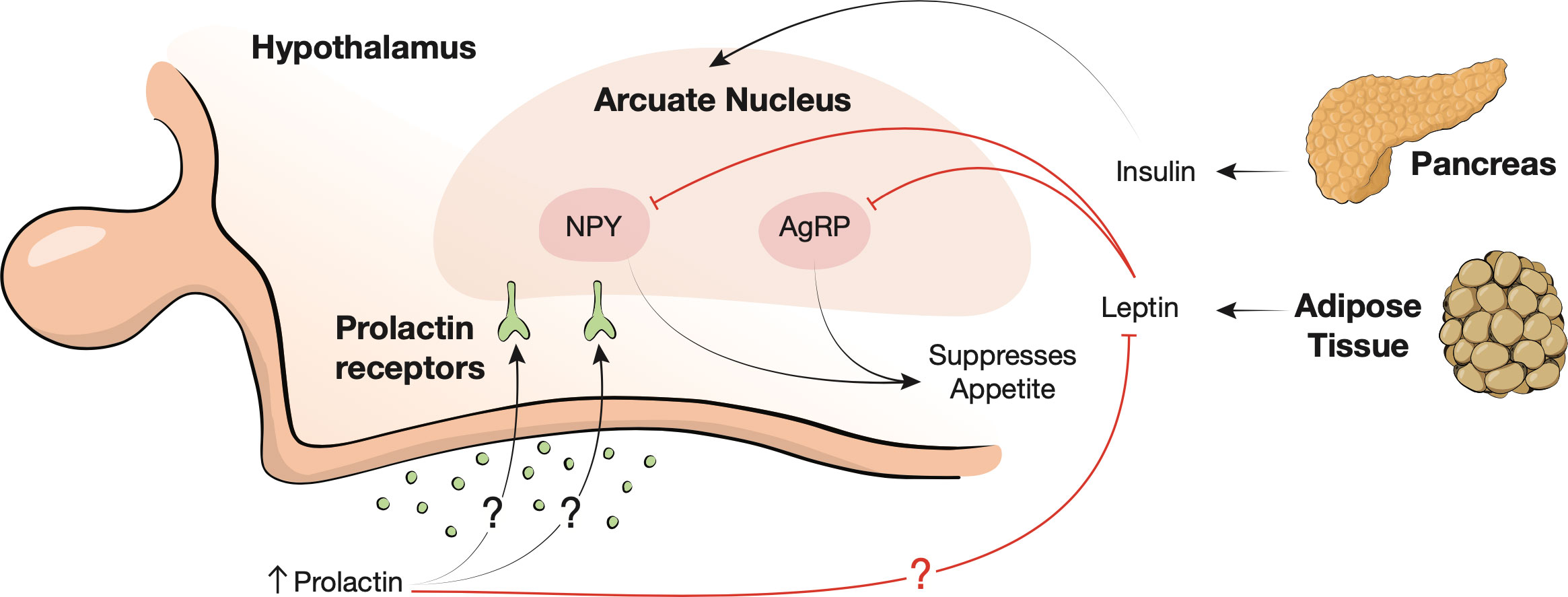
Figure 3 Neurohormonal appetite regulation. The arcuate nucleus of the hypothalamus integrates signals from insulin, leptin, and circulating nutrients. Leptin is secreted by adipose tissue and suppresses agouti-related protein (AgRP) and neuropeptide Y (NYP) neurons (red inhibition lines) to suppress appetite. Prolactin may also bind to the arcuate nucleus receptors leading to leptin insensitivity (red inhibition line).
Additionally, disruption of CNS dopaminergic tone with relative dopamine deficiency may lead to hyperphagia. As described above, prolactin self regulates by increasing dopamine release (4). In high prolactin states, central dopaminergic neurons become refractory to prolactin, contributing to weight gain and insulin resistance (7, 38, 57). Therefore, in addition to prolactin’s potential influence on eating behaviors through leptin insensitivity, prolactin may further stimulate hyperphagia by decreasing dopaminergic tone (7). Patients with polycystic ovarian syndrome (PCOS) have low hypothalamic dopaminergic tone leading to inappropriate prolactin and LH secretion. Ironically, high prolactin levels in this disease state can be associated with improved metabolic profiles (31, 32, 58, 59). However further discussion of PCOS related hyperprolactinemia is outside the scope of this article (32, 59, 60).
Adipose tissue
At physiologic levels (male 2-18 ng/mL, female 2-30 ng/mL), prolactin is important in maintaining adipogenesis and adipocyte differentiation. It is secreted by human adipocytes and has autocrine effects causing decreased activity of lipoprotein lipase and inhibiting lipolysis (38). Kok et al. demonstrated that obese women have higher prolactin secretion compared to healthy weight controls (61). They found that spontaneous prolactin release is increased in obese women, proportional to both body mass index (BMI) and visceral fat likely linked to decreased D2 activity (61). While it is recognized that significantly elevated prolactin levels are associated with weight gain, obesity, and overall metabolic dysfunction, the metabolic effects of prolactin vary even within a physiologic range (6, 38, 62–66). A recent study by Liu et al. categorized patients as metabolically healthy obese (i.e. BMI ≥ 30 with normal triglycerides, high density lipoprotein (HDL), blood pressure, and fasting blood glucose), metabolically unhealthy obese (i.e. BMI ≥ 30 with abnormal triglycerides, HDL, blood pressure, or fasting blood glucose), and controls (67). They found that metabolic healthy obese patients had slightly higher, although still physiological, prolactin levels (19.9 ng/mL) compared to metabolically unhealthy obese patients (14.4 ng/mL) and controls (12.4 ng/mL). These findings suggest that normal prolactin levels may serve as a regulatory mechanism, favoring energy metabolism in the obese state (67, 68).
Prolactin is also involved in metabolism through its effect on adiponectin, a hormone secreted by adipose tissue. Low adiponectin levels are associated with insulin resistance, obesity, and atherosclerosis (69). A study on adiponectin during pregnancy suggests that prolactin likely suppresses maternal adiponectin, thereby contributing to the new homeostatic set point characteristic of pregnancy (69). Although there have not been definitive studies demonstrating the same role for adiponectin in the non-pregnant state, animal studies have shown that adiponectin secretion is significantly suppressed by prolactin in mice in vivo and in human adipose tissue in vitro (70).
Pancreas
Prolactin acts to modulate beta cell growth and insulin resistance through direct action on the pancreas (71, 72). In fetal life, prolactin is important in pancreatic islet cell development. It has been shown that prolactin receptor deficient mice have reduced beta cell mass and lower insulin content, implying that prolactin plays a key role in developing beta cells during embryogenesis and gestation (7, 73, 74). In addition to dopamine’s role in regulating prolactin, dopamine impacts glucose homeostasis through a variety of mechanisms that are entirely independent of prolactin. Dopamine receptors (D2 and D3) found in the pancreas play a role in regulating insulin secretion (75). Blocking dopamine receptors can result in glucose intolerance, hyperinsulinemia, and weight gain. In fact, bromocriptine which is FDA approved for use in patients with type 2 diabetes, is a dopamine agonist that increases insulin sensitivity and decrease glycogen formation (76). Bromocriptine is effective in reducing hemoglobin A1C by 0.4-0.8% and improving hyperlipidemia and obesity, thereby decreasing cardiovascular risk by up to 40% (77, 78).
The concentration of prolactin influences the relationship between prolactin and insulin resistance. A study by Wagner et al. with participants between ages 18-80, found that over the age of 29 higher prolactin levels were associated with insulin sensitivity and lower glucose levels (AUC, P<0.0005) (79). It is hypothesized that this relationship may be due to differences in dopaminergic tone in the CNS and its effect on peripheral metabolism (79). Although causality is hard to predict and larger studies are needed, this suggests an age-dependent relationship of prolactin and insulin sensitivity (79). Park et al. found that in diabetic mice, beta cell expansion is stimulated by both low (25 µg/kg bw/12 h) and high (250 µg/kg bw/12 h) dose prolactin injections (80). At the end of the experimental period, mice receiving low dose prolactin injections had a mean prolactin level of 43.8 +/- 4.2 ng/mL and improved hepatic insulin sensitivity and insulin secretion. In contrast, mice receiving high prolactin doses had a mean prolactin level of 205.3 +/- 6.8 ng/mL with increased insulin resistance and impaired glucose tolerance (80, 81). The effect of treatment with prolactin lowering drugs, i.e. dopamine agonists, on various metabolic parameters is discussed in section 4.
Liver
At physiologic levels, prolactin may have a protective role in the liver. Prolactin receptors are expressed widely throughout the liver. Prolactin acts on these receptors to prevent hepatic steatosis by decreasing triglyceride accumulation (6, 82, 83). The CD36 fatty acid transporter is central in the pathogenesis of hepatic steatosis. Increased hepatic prolactin receptor expression is associated with decreased CD36 gene expression, thereby protecting against the development of hepatic steatosis (84). Zhang et al. found that prolactin levels are lower in patients with NAFLD compared to healthy controls (84). Furthermore, prolactin levels were found to be lower in patients with severe hepatic steatosis when compared to patients with mild to moderate disease (84). This does not hold true in pathological hyperprolactinemia secondary to pituitary adenomas. In these patients with significantly elevated prolactin, the protective effects of the hormone disappear. Patients may develop increased triglyceride and lipid droplet accumulation, favoring development of hepatic steatosis [25]. The underlying mechanism of this is poorly understood.
In addition to its role in hepatic steatosis, prolactin has been shown to impact insulin sensitivity. Prolactin improves hepatic insulin sensitivity by inducing signal transducer and activator of transcription 5 (STAT5) phosphorylation (6). Prolactin receptor expression is increased in insulin sensitive states and decreased in insulin resistant states both in vitro and in vivo. Supporting this proposed mechanism, it has been shown that hepatic prolactin receptor knock-out mice display impaired glucose tolerance and worsening insulin resistance (85).
Small bowel
Though not classically considered to be a metabolic organ, the small bowel is essential for nutrient absorption and therefore can impact weight gain and metabolism. Throughout pregnancy and lactation, prolactin is thought to act on receptors expressed throughout epithelial cells of the small intestine. A study in rodents showed that prolactin causes the small bowel surface area to increase, through both increasing height of the villi and length of the small intestine to allow for maximum nutrient absorption (86, 87). Though this is a compelling theory, studies on this topic are conflicting (88). Interestingly, prolactin’s effect on the intestinal mucosa has been specifically shown to increase intestinal calcium absorption in rats in vivo and human tissue in vitro which further supports the demands of pregnancy and lactation (89). Little research has been conducted on the influence of prolactin on the small bowel outside of the context of reproduction.
Metabolic effects of dopamine agonists in patients with hyperprolactinemia
It is well established that hyperprolactinemia causes metabolically deleterious effects (6, 38). Dopamine agonists bromocriptine (BRC) and cabergoline (CAB) are usually first line, though some patients require pituitary surgery and rarely radiation therapy to control their hyperprolactinemia. Table 2 summarizes seminal studies evaluating the metabolic effects of dopamine agonists in patients with hyperprolactinemia. We reviewed 17 studies from 2002 to 2021 that looked at changes in metabolic parameters including BMI, low density lipoprotein (LDL), and homeostatic model assessment for insulin resistance (HOMA-IR) (90–106). The average sample size was 28 and 11 of the studies reviewed were prospective. Across all studies, the mean age of patients at baseline was 35.1 years. Approximately 51% of the patients studied were female and 43% of tumors were macroprolactinomas. All 17 studies used a dopamine agonist as treatment (10 used CAB only, 6 used BRC or CAB, 2 used BRC only), and in 2 of these studies pituitary surgery was used in addition to medical therapy. No notable differences were seen between the 2 studies that used surgery in addition to medical treatment compared to those that used medical treatment alone, indicating that these results were independent of the modality of treatment.
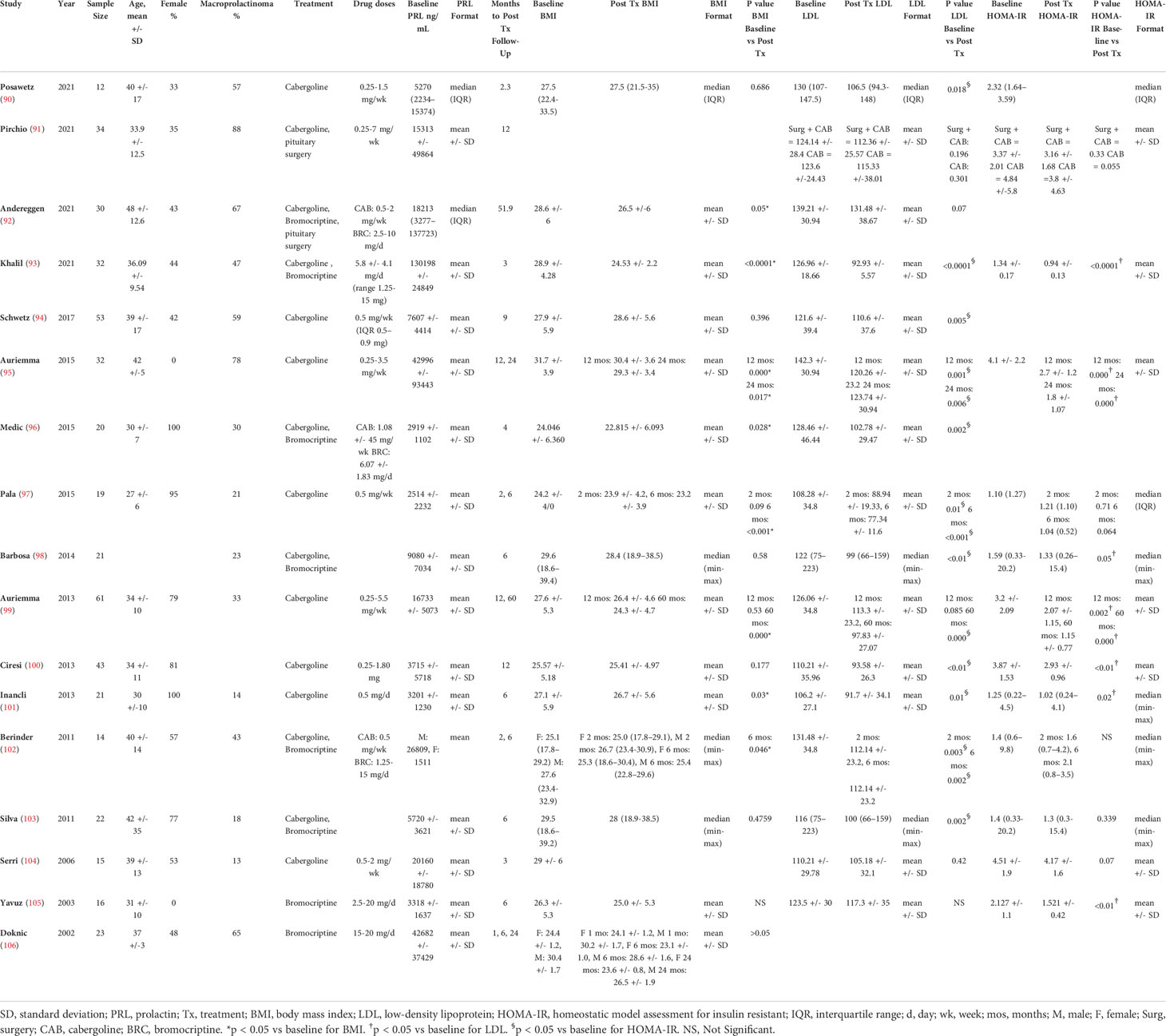
Table 2 Summary of recent studies evaluating the effects of dopamine agonists on metabolic parameters.
The mechanism by which prolactin levels maintain metabolic homeostasis is not clearly understood. Of the 17 studies listed in Table 2, 15 evaluated the effects of treatment of elevated prolactin on BMI. Of these studies, 8 found a statistically significant decrease (p < 0.05) in BMI (mean of 27.2 pre-treatment vs. 25.5 post-treatment, average decrease of 1.92) after a mean of 14.4 months of treatment (92, 93, 95–97, 99, 101, 102), while 7 did not (90, 94, 98, 100, 103, 105, 106). Of the 16 studies that reported LDL values, 12 studies found a statistically significant decrease (p < 0.05) in LDL (mean of 121.2 mg/dL pre-treatment vs. 100.8 mg/dL post-treatment, average decrease of 20.4 mg/dL) after a mean of 12 months of intervention (90, 93–103), while 4 did not reach significance (91, 92, 104, 105). Pala et al. found that improvement in lipid parameters is not related to weight loss or BMI, suggesting that there is likely a direct effect of dopamine agonists on lipids that is independent of weight (97). Likewise, Auriemma et al. found that weight, waist circumference, and BMI are significantly reduced after 60 months of CAB treatment (99). The prevalence of metabolic syndrome appears to decrease in a shorter different time frame, at 12 months, again supporting the idea that metabolic syndrome is at least somewhat independent of weight loss (99). Though prolactin is involved in lipid metabolism, D2 receptors are also present on adipose tissue, implying an independent regulatory role for dopamine (99). Ciresi et al. found no association between prolactin levels and metabolic parameters at baseline or at 12 months follow up (100). They report that the relationship between decrease in prolactin levels and improvement of metabolic parameters appears to reflect the effect of treatment with dopamine agonists, rather than prolactin normalization (100). This supports that idea that dopamine agonists offer a prolactin-independent mechanism of improving a patient’s metabolic profile. This discrepancy between improvement in BMI and LDL in the reported studies could possibly be due to the independent effects of dopamine and prolactin.
Of the 12 studies that evaluated HOMA-IR improvement in hyperprolactinemic patients treated with dopamine agonists, 7 studies found a statistically significant decrease (p < 0.05) in HOMA-IR (mean of 2.93 pre-treatment vs. 1.67 post-treatment, average decrease of 1.26) after a mean of 16.7 months of intervention (93, 95, 98–101, 103, 105), while 5 did not (91, 97, 102–104). Pirchio et al. explain that improvement in glucose profile correlates with dopamine agonist dose rather than with prolactin level (91). As discussed previously, dopamine plays a role in glucose homeostasis by binding D2 and D3 receptors in the pancreas preventing excessive sympathetic activity and hepatic glucose production, thereby improving insulin sensitivity. Additionally, it often takes greater than 12 weeks of follow-up to see improvement in HOMA-IR which would go along the normal course of dopamine agonist treatment. Analyzed together, this data provides compelling evidence that dopamine agonists have a direct effect on metabolism, beyond their role in prolactin normalization.
Prolactin: A “goldilocks zone”
Though it is clear that high prolactin levels are metabolically unfavorable, multiple studies have also shown low prolactin levels to be associated with increased metabolic disease (107–109). For example, Manshaei et al. found that prolactin levels in diabetic patients were significantly lower (5.32 ± 0.36 ng/mL) when compared to healthy controls (18.38 ± 2.3 ng/mL) (109). There may be a “goldilocks zone” for serum prolactin that promotes metabolic homeostasis, though exact ranges are variable amongst studies (1, 110, 111). Li et al. showed that normal to high prolactin levels (median 24, range 16-35 ng/mL) were associated with lower risk of type 2 diabetes at 20 years follow up compared to women with lower prolactin levels (median 6, range 5-8 ng/mL ng/mL) (112). In a smaller case control study of 134 patients, the average concentration of prolactin in controls (18.38 µg/L) was found to be significantly higher than that of diabetic patients (5.39 µg/L) (109). Wang et al. also similarly showed that a high normal prolactin range (11.61-26.29 ng/mL) was most protective against insulin resistance and diabetes in a large cohort of patients with hyperprolactinemia (107, 113). Furthermore, as previously described, Liu et al. found data supporting the idea that normal prolactin levels are metabolically beneficial, favoring energy metabolism in the obese state (67, 68).
The metabolic effects of prolactin at the different ranges are variable. Macotela et al. suggest a scale where intermediate levels of prolactin (25-100 ng/mL) are considered metabolically favorable with least untoward consequences, whereas low-normal prolactin levels (1-5 ng/mL) and significantly elevated levels (>100 ng/mL) are considered metabolically detrimental (1). More recently, in a study of 18 to 45 year-old women on CAB, the cardiometabolic profile of patients with low prolactin levels (less than 5 ng/mL) were compared to those within the reference range of 5-25 ng/mL (110). The hypoprolactinemic patients had higher glucose levels, hemoglobin A1C, triglycerides, insulin resistance and lower levels of HDL on analysis. Although this was a small study of 16 patients with low prolactin levels, the mechanistic link between low prolactin levels and metabolic dysregulation is certainly noteworthy (110).
In several studies (discussed in section 4), prolactin lowering drugs i.e. dopamine agonists are shown to improve metabolic profiles. Although this may appear counterintuitive to the “goldilocks zone” hypothesis, it is suggested that increased dopamine and higher levels of prolactin can improve metabolic fitness through distinct mechanisms, as delineated above. In fact, there may even be a role for prolactin enhancing drugs in the treatment of metabolic disease. Animal studies show that amisulpride, a dopamine receptor antagonist which enhances prolactin levels, improves the glucose profile in obese mice (114). Future prospective studies on this topic are clearly needed.
Conclusion
Prolactin is a unique hormone with action at various extra-pituitary sites, categorizing it as a hormone with classic, autocrine and paracrine activity. It is critical in pregnancy and lactation, during which prolactin levels rise to allow for metabolic adaptations through the cycle of reproduction but its role in metabolism is distinctive as well. Hyperprolactinemia causes a vast array of unfavorable metabolic effects but suppressed prolactin levels can also increase cardiometabolic risk. It is suggested that there may be a “goldilocks zone” where a high-normal prolactin is associated with optimal metabolic health; however the prolactin ranges are variable across studies. While some studies show improvement in BMI, LDL and HOMA-IR after prolactin normalizing treatment, the results are inconsistent. Large prospective studies with definitive metabolic end points are required to determine the most favorable prolactin level for metabolic health as well as the role for prolactin lowering and enhancing medications.
Author contributions
PK – Manuscript drafting and editing. JK - Manuscript drafting and editing. SS – Manuscript drafting and editing. NA – Crafting of manuscript contents, oversight of manuscript drafting and editing. All authors contributed to the article and approved the submitted version.
Acknowledgments
Figures 1, 2, and 3 created by Kristen Dancel-Manning.
Conflict of interest
Author NA is on the advisory board for Corcept Therapeutic and is a principal Investigator for institution-directed research grants (Chiasm Acromegaly Registry).
The remaining authors declare that the research was conducted in the absence of any commercial or financial relationships that could be construed as a potential conflict of interest.
Publisher’s note
All claims expressed in this article are solely those of the authors and do not necessarily represent those of their affiliated organizations, or those of the publisher, the editors and the reviewers. Any product that may be evaluated in this article, or claim that may be made by its manufacturer, is not guaranteed or endorsed by the publisher.
References
1. Macotela Y, Triebel J, Clapp C. Time for a new perspective on prolactin in metabolism. Trends Endocrinol Metab (2020) 31(4):276–86. doi: 10.1016/j.tem.2020.01.004
2. Freeman ME, Kanyicska B, Lerant A, Nagy G. Prolactin: Structure, function, and regulation of secretion. Physiol Rev (2000) 80(4):1523–631. doi: 10.1152/physrev.2000.80.4.1523
3. Al-Chalabi M, Bass AN, Alsalman I. Physiology, prolactin. In: Statpearls. Treasure Island (FL: StatPearls Publishing Copyright © 2022, StatPearls Publishing LLC (2022).
4. Lyons DJ, Broberger C. Tidal waves: Network mechanisms in the neuroendocrine control of prolactin release. Front Neuroendocrinol (2014) 35(4):420–38. doi: 10.1016/j.yfrne.2014.02.001
5. Nagano M, Kelly PA. Tissue distribution and regulation of rat prolactin receptor gene expression. Quant Anal by Polymerase Chain React J Biol Chem (1994) 269(18):13337–45. doi: 10.1016/S0021-9258(17)36838-2
6. Lopez-Vicchi F, De Winne C, Brie B, Sorianello E, Ladyman SR, Becu-Villalobos D. Metabolic functions of prolactin: Physiological and pathological aspects. J Neuroendocrinol (2020) 32(11):e12888. doi: 10.1111/jne.12888
7. Ben-Jonathan N, Hugo ER, Brandebourg TD, LaPensee CR. Focus on prolactin as a metabolic hormone. Trends Endocrinol Metab (2006) 17(3):110–6. doi: 10.1016/j.tem.2006.02.005
8. Ramos-Román MA. Prolactin and lactation as modifiers of diabetes risk in gestational diabetes. Horm Metab Res (2011) 43(9):593–600. doi: 10.1055/s-0031-1284353
9. Huang C, Snider F, Cross JC. Prolactin receptor is required for normal glucose homeostasis and modulation of beta-cell mass during pregnancy. Endocrinology (2009) 150(4):1618–26. doi: 10.1210/en.2008-1003
10. Freemark M, Fleenor D, Driscoll P, Binart N, Kelly P. Body weight and fat deposition in prolactin receptor-deficient mice. Endocrinology (2001) 142(2):532–7. doi: 10.1210/endo.142.2.7979
11. Ponce AJ, Galván-Salas T, Lerma-Alvarado RM, Ruiz-Herrera X, Hernández-Cortés T, Valencia-Jiménez R, et al. Low prolactin levels are associated with visceral adipocyte hypertrophy and insulin resistance in humans. Endocrine (2020) 67(2):331–43. doi: 10.1007/s12020-019-02170-x
12. Melmed S. Pituitary-tumor endocrinopathies. N Engl J Med (2020) 382(10):937–50. doi: 10.1056/NEJMra1810772
13. Melmed S, Casanueva FF, Hoffman AR, Kleinberg DL, Montori VM, Schlechte JA, et al. Diagnosis and treatment of hyperprolactinemia: An endocrine society clinical practice guideline. J Clin Endocrinol Metab (2011) 96(2):273–88. doi: 10.1210/jc.2010-1692
14. Fernandez A, Karavitaki N, Wass JA. Prevalence of pituitary adenomas: A community-based, cross-sectional study in Banbury (Oxfordshire, uk). Clin Endocrinol (Oxf) (2010) 72(3):377–82. doi: 10.1111/j.1365-2265.2009.03667.x
15. Peuskens J, Pani L, Detraux J, De Hert M. The effects of novel and newly approved antipsychotics on serum prolactin levels: A comprehensive review. CNS Drugs (2014) 28(5):421–53. doi: 10.1007/s40263-014-0157-3
16. Montejo ÁL, Arango C, Bernardo M, Carrasco JL, Crespo-Facorro B, Cruz JJ, et al. Spanish Consensus on the risks and detection of antipsychotic drug-related hyperprolactinaemia. Rev Psiquiatr Salud Ment (2016) 9(3):158–73. doi: 10.1016/j.rpsm.2015.11.003
17. Samperi I, Lithgow K, Karavitaki N. Hyperprolactinaemia. J Clin Med (2019) 8(12):1–24. doi: 10.3390/jcm8122203
18. Maiter D, Delgrange E. Therapy of endocrine disease: The challenges in managing giant prolactinomas. Eur J Endocrinol (2014) 170(6):R213–27. doi: 10.1530/eje-14-0013
19. Chiloiro S, Giampietro A, Bianchi A, Tartaglione T, Capobianco A, Anile C, et al. Diagnosis of endocrine disease: Primary empty sella: A comprehensive review. Eur J Endocrinol (2017) 177(6):R275–r85. doi: 10.1530/eje-17-0505
20. Molitch ME. Medication-induced hyperprolactinemia. Mayo Clin Proc (2005) 80(8):1050–7. doi: 10.4065/80.8.1050
21. Ajmal A, Joffe H, Nachtigall LB. Psychotropic-induced hyperprolactinemia: A clinical review. Psychosomatics (2014) 55(1):29–36. doi: 10.1016/j.psym.2013.08.008
22. Sharma LK, Sharma N, Gadpayle AK, Dutta D. Prevalence and predictors of hyperprolactinemia in subclinical hypothyroidism. Eur J Intern Med (2016) 35:106–10. doi: 10.1016/j.ejim.2016.07.012
23. Levine S, Muneyyirci-Delale O. Stress-induced hyperprolactinemia: Pathophysiology and clinical approach. Obstet Gynecol Int (2018) 2018:9253083. doi: 10.1155/2018/9253083
24. Muneyyirci-Delale O, Goldstein D, Reyes FI. Diagnosis of stress-related hyperprolactinemia. evaluation of the hyperprolactinemia rest test. N Y State J Med (1989) 89(4):205–8.
25. Lo JC, Beck GJ, Kaysen GA, Chan CT, Kliger AS, Rocco MV, et al. Hyperprolactinemia in end-stage renal disease and effects of frequent hemodialysis. Hemodial Int (2017) 21(2):190–6. doi: 10.1111/hdi.12489
26. Fountas A, Chai ST, Kourkouti C, Karavitaki N. Mechanisms of endocrinology: Endocrinology of opioids. Eur J Endocrinol (2018) 179(4):R183–r96. doi: 10.1530/eje-18-0270
27. Crowley WR. Neuroendocrine regulation of lactation and milk production. Compr Physiol (2015) 5(1):255–91. doi: 10.1002/cphy.c140029
28. Balakrishnan CH, Rajeev H. Correlation of serum prolactin level to child pugh scoring system in cirrhosis of liver. J Clin Diagn Res (2017) 11(7):Oc30–oc3. doi: 10.7860/jcdr/2017/24730.10273
29. Romeo JH, Dombrowski R, Kwak YS, Fuehrer S, Aron DC. Hyperprolactinaemia and verapamil: Prevalence and potential association with hypogonadism in men. Clin Endocrinol (Oxf) (1996) 45(5):571–5. doi: 10.1046/j.1365-2265.1996.00859.x
30. Vilar L, Fleseriu M, Bronstein MD. Challenges and pitfalls in the diagnosis of hyperprolactinemia. Arq Bras Endocrinol Metabol (2014) 58(1):9–22. doi: 10.1590/0004-2730000003002
31. Gómez R, Ferrero H, Delgado-Rosas F, Gaytan M, Morales C, Zimmermann RC, et al. Evidences for the existence of a low dopaminergic tone in polycystic ovarian syndrome: Implications for ohss development and treatment. J Clin Endocrinol Metab (2011) 96(8):2484–92. doi: 10.1210/jc.2011-0075
32. Albu A, Florea S, Fica S. Is prolactin the missing link in adipose tissue dysfunction of polycystic ovary syndrome patients? Endocrine (2016) 51(1):163–73. doi: 10.1007/s12020-015-0655-1
33. Nass RD, Sassen R, Elger CE, Surges R. The role of postictal laboratory blood analyses in the diagnosis and prognosis of seizures. Seizure (2017) 47:51–65. doi: 10.1016/j.seizure.2017.02.013
34. Lusić I, Pintarić I, Hozo I, Boić L, Capkun V. Serum prolactin levels after seizure and syncopal attacks. Seizure (1999) 8(4):218–22. doi: 10.1053/seiz.1999.0284
35. Wright K, Lee M, Escobar N, Pacione D, Young M, Fatterpekar G, et al. Tumor volume improves preoperative differentiation of prolactinomas and nonfunctioning pituitary adenomas. Endocrine (2021) 74(1):138–45. doi: 10.1007/s12020-021-02744-8
36. Karavitaki N, Thanabalasingham G, Shore HC, Trifanescu R, Ansorge O, Meston N, et al. Do the limits of serum prolactin in disconnection hyperprolactinaemia need re-definition? a study of 226 patients with histologically verified non-functioning pituitary macroadenoma. Clin Endocrinol (Oxf) (2006) 65(4):524–9. doi: 10.1111/j.1365-2265.2006.02627.x
37. Huang Y, Ding C, Zhang F, Xiao D, Zhao L, Wang S. Role of Prolactin/Adenoma maximum diameter and Prolactin/Adenoma volume in the differential diagnosis of prolactinomas and other types of pituitary adenomas. Oncol Lett (2018) 15(2):2010–6. doi: 10.3892/ol.2017.7462
38. Shibli-Rahhal A, Schlechte J. The effects of hyperprolactinemia on bone and fat. Pituitary (2009) 12(2):96–104. doi: 10.1007/s11102-008-0097-3
39. Gerardo-Gettens T, Moore BJ, Stern JS, Horwitz BA. Prolactin stimulates food intake in a dose-dependent manner. Am J Physiol (1989) 256(1 Pt 2):R276–80. doi: 10.1152/ajpregu.1989.256.1.R276
40. Al Sabie F, Tariq Z, Erickson D, Donegan D. Association between prolactinoma and body mass index. Endocr Pract (2021) 27(4):312–7. doi: 10.1016/j.eprac.2020.09.001
41. Bernard V, Young J, Binart N. Prolactin - a pleiotropic factor in health and disease. Nat Rev Endocrinol (2019) 15(6):356–65. doi: 10.1038/s41574-019-0194-6
42. Millar RP, Sonigo C, Anderson RA, George J, Maione L, Brailly-Tabard S, et al. Hypothalamic-Pituitary-Ovarian axis reactivation by kisspeptin-10 in hyperprolactinemic women with chronic amenorrhea. J Endocr Soc (2017) 1(11):1362–71. doi: 10.1210/js.2017-00328
43. Hoskova K, Kayton Bryant N, Chen ME, Nachtigall LB, Lippincott MF, Balasubramanian R, et al. Kisspeptin overcomes gnrh neuronal suppression secondary to hyperprolactinemia in humans. J Clin Endocrinol Metab (2022) 107(8):e3515–e25. doi: 10.1210/clinem/dgac166
44. Kokay IC, Petersen SL, Grattan DR. Identification of prolactin-sensitive gaba and kisspeptin neurons in regions of the rat hypothalamus involved in the control of fertility. Endocrinology (2011) 152(2):526–35. doi: 10.1210/en.2010-0668
45. Navarro VM. Metabolic regulation of kisspeptin - the link between energy balance and reproduction. Nat Rev Endocrinol (2020) 16(8):407–20. doi: 10.1038/s41574-020-0363-7
46. Wolfe A, Hussain MA. The emerging Role(S) for kisspeptin in metabolism in mammals. Front Endocrinol (Lausanne) (2018) 9:184. doi: 10.3389/fendo.2018.00184
47. Song WJ, Mondal P, Wolfe A, Alonso LC, Stamateris R, Ong BW, et al. Glucagon regulates hepatic kisspeptin to impair insulin secretion. Cell Metab (2014) 19(4):667–81. doi: 10.1016/j.cmet.2014.03.005
48. Pruszyńska-Oszmałek E, Kołodziejski PA, Sassek M, Sliwowska JH. Kisspeptin-10 inhibits proliferation and regulates lipolysis and lipogenesis processes in 3t3-L1 cells and isolated rat adipocytes. Endocrine (2017) 56(1):54–64. doi: 10.1007/s12020-017-1248-y
49. Schwetz TA, Reissaus CA, Piston DW. Differential stimulation of insulin secretion by glp-1 and kisspeptin-10. PloS One (2014) 9(11):e113020. doi: 10.1371/journal.pone.0113020
50. Flak JN, Myers MG Jr. Minireview: Cns mechanisms of leptin action. Mol Endocrinol (2016) 30(1):3–12. doi: 10.1210/me.2015-1232
51. Jais A, Brüning JC. Arcuate nucleus-dependent regulation of metabolism-pathways to obesity and diabetes mellitus. Endocr Rev (2022) 43(2):314–28. doi: 10.1210/endrev/bnab025
52. Gruzdeva O, Borodkina D, Uchasova E, Dyleva Y, Barbarash O. Leptin resistance: Underlying mechanisms and diagnosis. Diabetes Metab Syndr Obes (2019) 12:191–8. doi: 10.2147/dmso.S182406
53. Ruegsegger GN, Booth FW. Running from disease: Molecular mechanisms associating dopamine and leptin signaling in the brain with physical inactivity, obesity, and type 2 diabetes. Front Endocrinol (Lausanne) (2017) 8:109. doi: 10.3389/fendo.2017.00109
54. Kopelman PG. Physiopathology of prolactin secretion in obesity. Int J Obes Relat Metab Disord (2000) 24 Suppl 2:S104–8. doi: 10.1038/sj.ijo.0801291
55. Ladyman SR, Grattan DR. Region-specific reduction in leptin-induced phosphorylation of signal transducer and activator of transcription-3 (Stat3) in the rat hypothalamus is associated with leptin resistance during pregnancy. Endocrinology (2004) 145(8):3704–11. doi: 10.1210/en.2004-0338
56. Naef L, Woodside B. Prolactin/Leptin interactions in the control of food intake in rats. Endocrinology (2007) 148(12):5977–83. doi: 10.1210/en.2007-0442
57. Wang GJ, Volkow ND, Logan J, Pappas NR, Wong CT, Zhu W, et al. Brain dopamine and obesity. Lancet (2001) 357(9253):354–7. doi: 10.1016/s0140-6736(00)03643-6
58. Hernández I, Parra A, Méndez I, Cabrera V, Cravioto MC, Mercado M, et al. Hypothalamic dopaminergic tone and prolactin bioactivity in women with polycystic ovary syndrome. Arch Med Res (2000) 31(2):216–22. doi: 10.1016/s0188-4409(00)00059-x
59. Glintborg D, Altinok M, Mumm H, Buch K, Ravn P, Andersen M. Prolactin is associated with metabolic risk and cortisol in 1007 women with polycystic ovary syndrome. Hum Reprod (2014) 29(8):1773–9. doi: 10.1093/humrep/deu133
60. Yang H, Di J, Pan J, Yu R, Teng Y, Cai Z, et al. The association between prolactin and metabolic parameters in pcos women: A retrospective analysis. Front Endocrinol (Lausanne) (2020) 11:263. doi: 10.3389/fendo.2020.00263
61. Kok P, Roelfsema F, Frölich M, Meinders AE, Pijl H. Prolactin release is enhanced in proportion to excess visceral fat in obese women. J Clin Endocrinol Metab (2004) 89(9):4445–9. doi: 10.1210/jc.2003-032184
62. Schmid C, Goede DL, Hauser RS, Brändle M. Increased prevalence of high body mass index in patients presenting with pituitary tumours: Severe obesity in patients with macroprolactinoma. Swiss Med Wkly (2006) 136(15-16):254–8. doi: 10.4414/smw.2006.10955
63. Landgraf R, Landraf-Leurs MM, Weissmann A, Hörl R, von Werder K, Scriba PC. Prolactin: A diabetogenic hormone. Diabetologia (1977) 13(2):99–104. doi: 10.1007/bf00745135
64. Weaver JU, Noonan K, Kopelman PG. An association between hypothalamic-pituitary dysfunction and peripheral endocrine function in extreme obesity. Clin Endocrinol (Oxf) (1991) 35(1):97–102. doi: 10.1111/j.1365-2265.1991.tb03503.x
65. Sobrinho LG, Horseman ND. Prolactin and human weight disturbances: A puzzling and neglected association. Rev Endocr Metab Disord (2019) 20(2):197–206. doi: 10.1007/s11154-019-09503-1
66. Greenman Y, Tordjman K, Stern N. Increased body weight associated with prolactin secreting pituitary adenomas: Weight loss with normalization of prolactin levels. Clin Endocrinol (Oxf) (1998) 48(5):547–53. doi: 10.1046/j.1365-2265.1998.00403.x
67. Liu J, Zhang L, Fu J, Wang Q, Wang G. Circulating prolactin level is increased in metabolically healthy obesity. Endocr Connect (2021) 10(4):484–91. doi: 10.1530/ec-21-0040
68. Ruiz-Herrera X, de Los Ríos EA, Díaz JM, Lerma-Alvarado RM, Martínez de la Escalera L, López-Barrera F, et al. Prolactin promotes adipose tissue fitness and insulin sensitivity in obese males. Endocrinology (2017) 158(1):56–68. doi: 10.1210/en.2016-1444
69. Asai-Sato M, Okamoto M, Endo M, Yoshida H, Murase M, Ikeda M, et al. Hypoadiponectinemia in lean lactating women: Prolactin inhibits adiponectin secretion from human adipocytes. Endocr J (2006) 53(4):555–62. doi: 10.1507/endocrj.k06-026
70. Nilsson L, Binart N, Bohlooly YM, Bramnert M, Egecioglu E, Kindblom J, et al. Prolactin and growth hormone regulate adiponectin secretion and receptor expression in adipose tissue. Biochem Biophys Res Commun (2005) 331(4):1120–6. doi: 10.1016/j.bbrc.2005.04.026
71. Khant Aung Z, Kokay IC, Grattan DR, Ladyman SR. Prolactin-induced adaptation in glucose homeostasis in mouse pregnancy is mediated by the pancreas and not in the forebrain. Front Endocrinol (Lausanne) (2021) 12:765976. doi: 10.3389/fendo.2021.765976
72. Houssay BA, Anderson E. Diabetogenic action of purified anterior pituitary hormones. Endocrinology (1949) 45(6):627–9. doi: 10.1210/endo-45-6-627
73. Holstad M, Sandler S. Prolactin protects against diabetes induced by multiple low doses of streptozotocin in mice. J Endocrinol (1999) 163(2):229–34. doi: 10.1677/joe.0.1630229
74. Brelje TC, Parsons JA, Sorenson RL. Regulation of islet beta-cell proliferation by prolactin in rat islets. Diabetes (1994) 43(2):263–73. doi: 10.2337/diab.43.2.263
75. Kabir MT, Ferdous Mitu J, Akter R, Akhtar MF, Saleem A, Al-Harrasi A, et al. Therapeutic potential of dopamine agonists in the treatment of type 2 diabetes mellitus. Environ Sci pollut Res Int (2022) 29(31):46385–404. doi: 10.1007/s11356-022-20445-1
76. Framnes-DeBoer SN, Bakke E, Yalamanchili S, Peterson H, Sandoval DA, Seeley RJ, et al. Bromocriptine improves glucose tolerance independent of circadian timing, prolactin, or the melanocortin-4 receptor. Am J Physiol Endocrinol Metab (2020) 318(1):E62–e71. doi: 10.1152/ajpendo.00325.2019
77. Valiquette G. Bromocriptine for diabetes mellitus type ii. Cardiol Rev (2011) 19(6):272–5. doi: 10.1097/CRD.0b013e318229d2d2
78. Defronzo RA. Bromocriptine: A sympatholytic, D2-dopamine agonist for the treatment of type 2 diabetes. Diabetes Care (2011) 34(4):789–94. doi: 10.2337/dc11-0064
79. Wagner R, Heni M, Linder K, Ketterer C, Peter A, Böhm A, et al. Age-dependent association of serum prolactin with glycaemia and insulin sensitivity in humans. Acta Diabetol (2014) 51(1):71–8. doi: 10.1007/s00592-013-0493-7
80. Park S, Kim DS, Daily JW, Kim SH. Serum prolactin concentrations determine whether they improve or impair β-cell function and insulin sensitivity in diabetic rats. Diabetes Metab Res Rev (2011) 27(6):564–74. doi: 10.1002/dmrr.1215
81. Park S, Kang S, Lee HW, Ko BS. Central prolactin modulates insulin sensitivity and insulin secretion in diabetic rats. Neuroendocrinology (2012) 95(4):332–43. doi: 10.1159/000336501
82. Hartwell HJ, Petrosky KY, Fox JG, Horseman ND, Rogers AB. Prolactin prevents hepatocellular carcinoma by restricting innate immune activation of c-myc in mice. Proc Natl Acad Sci U.S.A. (2014) 111(31):11455–60. doi: 10.1073/pnas.1404267111
83. Shao S, Yao Z, Lu J, Song Y, He Z, Yu C, et al. Ablation of prolactin receptor increases hepatic triglyceride accumulation. Biochem Biophys Res Commun (2018) 498(3):693–9. doi: 10.1016/j.bbrc.2018.03.048
84. Zhang P, Ge Z, Wang H, Feng W, Sun X, Chu X, et al. Prolactin improves hepatic steatosis Via Cd36 pathway. J Hepatol (2018) 68(6):1247–55. doi: 10.1016/j.jhep.2018.01.035
85. Yu J, Xiao F, Zhang Q, Liu B, Guo Y, Lv Z, et al. Prlr regulates hepatic insulin sensitivity in mice Via Stat5. Diabetes (2013) 62(9):3103–13. doi: 10.2337/db13-0182
86. Cripps AW, Williams VJ. The effect of pregnancy and lactation on food intake, gastrointestinal anatomy and the absorptive capacity of the small intestine in the albino rat. Br J Nutr (1975) 33(1):17–32. doi: 10.1079/bjn19750005
87. Hammond KA. Adaptation of the maternal intestine during lactation. J Mammary Gland Biol Neoplasia (1997) 2(3):243–52. doi: 10.1023/a:1026332304435
88. Muller E, Dowling RH. Prolactin and the small intestine. effect of hyperprolactinaemia on mucosal structure in the rat. Gut (1981) 22(7):558–65. doi: 10.1136/gut.22.7.558
89. Ryszka F, Klimas R, Dolinska B, Lopata K. Influence of prolactin and calcium gluconate concentration on permeation and intestinal absorption of Ca(Ii) ions. Protein Pept Lett (2012) 19(8):804–7. doi: 10.2174/092986612801619633
90. Posawetz AS, Trummer C, Pandis M, Aberer F, Pieber TR, Obermayer-Pietsch B, et al. Adverse body composition and lipid parameters in patients with prolactinoma: A case-control study. BMC Endocr Disord (2021) 21(1):81. doi: 10.1186/s12902-021-00733-6
91. Pirchio R, Auriemma RS, Solari D, Arnesi M, Pivonello C, Negri M, et al. Effects of pituitary surgery and high-dose cabergoline therapy on metabolic profile in patients with prolactinoma resistant to conventional cabergoline treatment. Front Endocrinol (Lausanne) (2021) 12:769744. doi: 10.3389/fendo.2021.769744
92. Andereggen L, Frey J, Andres RH, Luedi MM, Gralla J, Schubert GA, et al. Impact of primary medical or surgical therapy on prolactinoma patients' bmi and metabolic profile over the long-term. J Clin Transl Endocrinol (2021) 24:100258. doi: 10.1016/j.jcte.2021.100258
93. Khalil G, Khan FA, Jamal QM, Saleem A, Masroor H, Abbas K. Change in insulin sensitivity and lipid profile after dopamine agonist therapy in patients with prolactinoma. Cureus (2021) 13(9):e17824. doi: 10.7759/cureus.17824
94. Schwetz V, Librizzi R, Trummer C, Theiler G, Stiegler C, Pieber TR, et al. Treatment of hyperprolactinaemia reduces total cholesterol and ldl in patients with prolactinomas. Metab Brain Dis (2017) 32(1):155–61. doi: 10.1007/s11011-016-9882-2
95. Auriemma RS, Galdiero M, Vitale P, Granieri L, Lo Calzo F, Salzano C, et al. Effect of chronic cabergoline treatment and testosterone replacement on metabolism in Male patients with prolactinomas. Neuroendocrinology (2015) 101(1):66–81. doi: 10.1159/000371851
96. Medic-Stojanoska M, Icin T, Pletikosic I, Bajkin I, Novakovic-Paro J, Stokic E, et al. Risk factors for accelerated atherosclerosis in young women with hyperprolactinemia. Med Hypotheses (2015) 84(4):321–6. doi: 10.1016/j.mehy.2015.01.024
97. Pala NA, Laway BA, Misgar RA, Dar RA. Metabolic abnormalities in patients with prolactinoma: Response to treatment with cabergoline. Diabetol Metab Syndr (2015) 7:99. doi: 10.1186/s13098-015-0094-4
98. Barbosa FR, Silva CM, Lima GA, Warszawski L, Domingues RC, Dominic M, et al. Prevalence of obstructive sleep apnea in patients with prolactinoma before and after treatment with dopamine agonists. Pituitary (2014) 17(5):441–9. doi: 10.1007/s11102-013-0524-y
99. Auriemma RS, Granieri L, Galdiero M, Simeoli C, Perone Y, Vitale P, et al. Effect of cabergoline on metabolism in prolactinomas. Neuroendocrinology (2013) 98(4):299–310. doi: 10.1159/000357810
100. Ciresi A, Amato MC, Guarnotta V, Lo Castro F, Giordano C. Higher doses of cabergoline further improve metabolic parameters in patients with prolactinoma regardless of the degree of reduction in prolactin levels. Clin Endocrinol (Oxf) (2013) 79(6):845–52. doi: 10.1111/cen.12204
101. Inancli SS, Usluogullari A, Ustu Y, Caner S, Tam AA, Ersoy R, et al. Effect of cabergoline on insulin sensitivity, inflammation, and carotid intima media thickness in patients with prolactinoma. Endocrine (2013) 44(1):193–9. doi: 10.1007/s12020-012-9857-y
102. Berinder K, Nyström T, Höybye C, Hall K, Hulting AL. Insulin sensitivity and lipid profile in prolactinoma patients before and after normalization of prolactin by dopamine agonist therapy. Pituitary (2011) 14(3):199–207. doi: 10.1007/s11102-010-0277-9
103. dos Santos Silva CM, Barbosa FR, Lima GA, Warszawski L, Fontes R, Domingues RC, et al. Bmi and metabolic profile in patients with prolactinoma before and after treatment with dopamine agonists. Obes (Silver Spring) (2011) 19(4):800–5. doi: 10.1038/oby.2010.150
104. Serri O, Li L, Mamputu JC, Beauchamp MC, Maingrette F, Renier G. The influences of hyperprolactinemia and obesity on cardiovascular risk markers: Effects of cabergoline therapy. Clin Endocrinol (Oxf) (2006) 64(4):366–70. doi: 10.1111/j.1365-2265.2006.02469.x
105. Yavuz D, Deyneli O, Akpinar I, Yildiz E, Gözü H, Sezgin O, et al. Endothelial function, insulin sensitivity and inflammatory markers in hyperprolactinemic pre-menopausal women. Eur J Endocrinol (2003) 149(3):187–93. doi: 10.1530/eje.0.1490187
106. Doknic M, Pekic S, Zarkovic M, Medic-Stojanoska M, Dieguez C, Casanueva F, et al. Dopaminergic tone and obesity: An insight from prolactinomas treated with bromocriptine. Eur J Endocrinol (2002) 147(1):77–84. doi: 10.1530/eje.0.1470077
107. Wang T, Lu J, Xu Y, Li M, Sun J, Zhang J, et al. Circulating prolactin associates with diabetes and impaired glucose regulation: A population-based study. Diabetes Care (2013) 36(7):1974–80. doi: 10.2337/dc12-1893
108. Balbach L, Wallaschofski H, Völzke H, Nauck M, Dörr M, Haring R. Serum prolactin concentrations as risk factor of metabolic syndrome or type 2 diabetes? BMC Endocr Disord (2013) 13:12. doi: 10.1186/1472-6823-13-12
109. Manshaei N, Shakibaei F, Fazilati M, Salavati H, Negahdary M, Palizban A. An investigation of the association between the level of prolactin in serum and type ii diabetes. Diabetes Metab Syndr (2019) 13(5):3035–41. doi: 10.1016/j.dsx.2018.07.007
110. Krysiak R, Kowalcze K, Okopień B. Cardiometabolic profile of young women with hypoprolactinemia. Endocrine (2022) 78:135–41. doi: 10.1007/s12020-022-03145-1
111. Krysiak R, Kowalcze K, Okopień B. Sexual function and depressive symptoms in men with hypoprolactinaemia secondary to overtreatment of prolactin excess: A pilot study. Endocrinol Diabetes Nutr (Engl Ed) (2022) 69(4):279–88. doi: 10.1016/j.endien.2021.03.004
112. Li J, Rice MS, Huang T, Hankinson SE, Clevenger CV, Hu FB, et al. Circulating prolactin concentrations and risk of type 2 diabetes in us women. Diabetologia (2018) 61(12):2549–60. doi: 10.1007/s00125-018-4733-9
113. Wang T, Xu Y, Xu M, Ning G, Lu J, Dai M, et al. Circulating prolactin and risk of type 2 diabetes: A prospective study. Am J Epidemiol (2016) 184(4):295–301. doi: 10.1093/aje/kwv326
114. Zhang X, van den Pol AN. Dopamine/Tyrosine hydroxylase neurons of the hypothalamic arcuate nucleus release gaba, communicate with dopaminergic and other arcuate neurons, and respond to dynorphin, met-enkephalin, and oxytocin. J Neurosci (2015) 35(45):14966–82. doi: 10.1523/jneurosci.0293-15.2015
Keywords: prolactin, hyperprolactinemia, prolactinoma, metabolic syndrome, pituitary, metabolic dysfunction, dopamine agonist
Citation: Kirsch P, Kunadia J, Shah S and Agrawal N (2022) Metabolic effects of prolactin and the role of dopamine agonists: A review. Front. Endocrinol. 13:1002320. doi: 10.3389/fendo.2022.1002320
Received: 25 July 2022; Accepted: 15 September 2022;
Published: 30 September 2022.
Edited by:
Lucio Vilar, Federal University of Pernambuco, BrazilReviewed by:
David Norris, University of Colorado Boulder, United StatesYazmín Macotela, Institute of Neurobiology, National Autonomous University of Mexico, Mexico
Yue Liu, Beijing University of Chinese Medicine, China
Copyright © 2022 Kirsch, Kunadia, Shah and Agrawal. This is an open-access article distributed under the terms of the Creative Commons Attribution License (CC BY). The use, distribution or reproduction in other forums is permitted, provided the original author(s) and the copyright owner(s) are credited and that the original publication in this journal is cited, in accordance with accepted academic practice. No use, distribution or reproduction is permitted which does not comply with these terms.
*Correspondence: Nidhi Agrawal, TmlkaGkuYWdyYXdhbEBueXVsYW5nb25lLm9yZw==