- 1Helmholtz Institute for Metabolic, Obesity and Vascular Research (HI-MAG), Helmholtz Zentrum München, University Hospital Leipzig, University of Leipzig, Leipzig, Germany
- 2Basic Scientific Research of Lymphological Diseases and Patient-oriented Improvement of Diagnosis and Treatment Ly.Search GmbH, Cologne, Germany
- 3Medical Department III – Endocrinology, Nephrology, Rheumatology, University of Leipzig Medical Center, Leipzig, Germany
Lipedema may be considered a model for healthy expandability of subcutaneous adipose tissue (SAT). This condition is characterized by the disproportional and symmetrical SAT accumulation in the lower-body parts and extremities, avoiding the abdominal area. There are no circulating biomarkers facilitating the diagnosis of lipedema. We tested the hypothesis that women living with lipedema present a distinct pattern of circulating parameters compared to age- and BMI-matched women. In 26 women (Age 48.3 ± 13.9 years, BMI 32.6 ± 5.8 kg/m2; lipedema group: n=13; control group: n=13), we assessed circulating parameters of glucose and lipid metabolism, inflammation, oxidative stress, sex hormones and a proteomics panel. We find that women with lipedema have better glucose metabolism regulation represented by lower HbA1c (5.55 ± 0.62%) compared to controls (6.73 ± 0.85%; p<0.001); and higher adiponectin levels (lipedema: 4.69 ± 1.99 mmol/l; control: 3.28 ± 1.00 mmol/l; p=0.038). Despite normal glycemic parameters, women with lipedema have significantly higher levels of total cholesterol (5.84 ± 0.70 mmol/L vs 4.55 ± 0.77 mmol/L in control; p<0.001), LDL-C (3.38 ± 0.68 mmol/L vs 2.38 ± 0.66 mmol/L in control; p=0.002), as well as higher circulating inflammation (top 6 based on p-values: TNFSF14, CASP8, EN-RAGE, EIF4EBP1, ADA, MCP-1) and oxidative stress markers (malondialdehyde, superoxide dismutase and catalase). Our findings suggest that the expected association between activation of inflammatory and oxidative stress pathways and impaired glucose metabolism are counterbalanced by protective factors in lipedema.
Introduction
Lipedema is a loose connective tissue disease characterized by the disproportional and excessive accumulation of fibrotic subcutaneous adipose tissue (SAT) mainly around the buttocks, hips and limbs (1–4). Therefore, lipedema could serve as a model to investigate mechanisms linking impaired SAT expandability to increased cardio-metabolic risk. In this context, it has been shown that higher leg fat accumulation and low trunk fat are associated with lower cardiovascular disease risk in postmenopausal women with normal BMI (5). Consistently, lipedema has been associated with a lower risk for diabetes and other cardio-metabolic dysfunctions (4, 6). Indeed, based on clinical observations, the excessive SAT accumulation in lipedema is predominant in the arms and lower-body parts (legs), sparing the abdominal area contrarily to obesity (7–9).
Lipedema is mostly diagnosed in women and its onset and development are generally recorded in periods of hormonal, weight and shape shifts such as during puberty, pregnancy, childbirth and menopause (1). The main clinical component concomitant to the increase of SAT mass, but distinguishing lipedema from obesity is the reported pain (“painful fat”), which together with the increased tissue fibrosis is speculated to be a consequence of high inflammation in lipedema tissue (1, 10, 11). Moreover, the reduction of AT mass by various weight loss measures is less effective in lipedema patients compared to obesity, which severely affects patients’ quality of life and leads to psychological problems and an increased risk of suicide (3, 4, 8, 10, 12, 13). Although lipedema was described decades ago (14), the relative scarcity of data and understanding of the mechanisms associated with its onset, progression and clinical manifestation is surprising. Furthermore, there is currently no epidemiology-based data on the prevalence of lipedema, which is estimated to vary between 7 – 18% of women across different studies’ cohorts (4, 8, 15–18).
Lipedema is one of the most often under/misdiagnosed fat accumulation disorders (9, 19). This is mainly due to the similarities in phenotypic features with other excessive fat accumulation like obesity (13). However, the dysregulations related to excessive SAT accumulation have been well described in the context of obesity (20). Indeed, adipose tissue (AT) dysfunction may develop in response to progressive and continuous lipids storage in obesity (20). This is characterized among others by impaired adipogenesis (21, 22) and angiogenesis (23, 24), fibrosis (20, 25) and hypoxia (26, 27), dysregulated adipokine secretion (27–29), AT inflammation (26, 28, 30) as well as oxidative stress (27, 31). These metabolic processes can interfere with insulin signalling pathways, contributing to the development of insulin resistance, type 2 diabetes (T2D) and other obesity-associated metabolic diseases (20, 32–34). Some of these dysregulations have also been described in lipedema, including dysregulated adipogenesis (35, 36) and angiogenesis (9, 37), fibrosis and hypoxia (38, 39), immune cell infiltration and AT inflammation (35, 37, 40, 41). However, it remains unknown whether and to which extent the excessive expansion of AT in lipedema influences whole-body metabolic health in these patients.
With regard to the similarities in some components of AT dysfunction described in both conditions, the resulting obesity-associated metabolic dysregulations could be expected in lipedema as well. Indeed, adipocyte hypertrophy in obesity is associated with increased systemic insulin resistance, and inflammatory and oxidative stress (33, 34, 42). However, lipedema patients are reported with a relatively lower incidence of T2D although at advanced stages these patients seem to present a higher risk for developing obesity and cardiovascular diseases (8, 17). This suggests dissimilar mechanisms associating SAT accumulation to metabolic health status between lipedema and obesity. AT exerts its effect on whole-body homeostasis partially through the endocrine action of adipocytokines and lipids released into the circulation, which thereby affects the function of other tissues (43). For instance, increased AT macrophage infiltration in obesity influences local and systemic inflammation through dysregulated cytokine secretion (29, 44). Adipose-derived biomolecules may therefore represent a valuable target for an early diagnosis and treatment of lipedema. We hypothesize that circulating factors may mediate the beneficial effects of adipose tissue expandability to protect against cardio-metabolic diseases. Therefore, we aimed to characterize circulating biomarkers of glucose and lipid metabolism, inflammation and oxidative stress in lipedema patients, and evaluate the association between these markers and metabolic status. The outcomes of this study would allow profiling of systemic biomarkers dysregulated in lipedema and improve the current understanding of the mechanisms underlying the clinical manifestation of this condition.
Methods
Study participants
To investigate the molecular alterations in lipedema, 13 women diagnosed with lipedema aged from 24 to 71 years, with a body mass index (BMI) from 23.7 to 41.5 kg/m2 were enrolled in this study. These women were clinically diagnosed by two independent specialists in lymphology and related conditions based on defined clinical criteria (1, 16, 45). As there is currently no consensus to clearly categorize lipedema patients, the diagnosis relies on the reported symptoms and clinical observations, with no absolute phenotypic features or confirmatory tests. The control group was selected from women treated in our obesity outpatients clinic (n=13) to be age- and BMI-matched to the lipedema patients, but without any clinical symptoms of lipedema. The study was performed in agreement with the Declaration of Helsinki and approved by the Ethics Committee of the University of Leipzig (approval number: 159-12-21052012 and 017/12-ek). All individuals gave written informed consent before participating in the study.
Basic anthropometry and sample collection
Basic anthropometric measures (weight and height) were recorded from all participants. Limb volumes were measured in lipedema patients using a perometer (Pero-System Meters Ltd. Germany) in lipedema patients. To measure circulating biomarkers, blood samples from participants of both groups were collected between 08 - 10 am in EDTA tubes after overnight fasting. EDTA-blood samples were centrifuged for 10 minutes at room temperature, at 3260g (sigma 2-16P, Germany), and plasma samples as well as red blood cells sub-fraction were aliquot and stored at -20°C.
Plasma parameter analyses
Biomarkers of glucose and lipid metabolism, liver function, inflammation and oxidative stress as well as sex hormones were assessed in plasma samples of patients from both groups using commercially available kits according to the manufacturer’s instructions. Briefly, plasma concentrations of glucose (ACN8717), triglycerides (ACN7881), total cholesterol (ACN8798), low-density lipoprotein cholesterol (LDL-C; ACN8552), high-density lipoprotein cholesterol (HDL-C; ACN8454), alanine transaminase (ALAT), aspartate aminotransferase (ASAT) and gamma-glutamyltransferase (gGT; ACN8220), as well as lipase (ACN789) were determined by homogeneous enzymatic colorimetric assays (COBAS 8000-c502 and c701; Roche Diagnostics, Germany). Chronic hyperglycemia was estimated by the measure of haemoglobin A1c (HbA1c) levels in full-blood samples using immunoturbidimetric assay (HITADO Super-ID-System; Germany). Insulin (10-1113-01; Mercodia, Uppsala, Sweden), C-peptide (10-1136-01; Mercodia), leptin (E07; Mediagnost, Reutlingen, Germany), adiponectin (AG-45A-0001YEK-KI01; AdipoGen Life Sciences, San Diego, CA, USA), tumor necrosis factor-alpha (TNF-α; HSTA00E; R&D Systems, Minnesota, Minneapolis, USA), as well as circulating concentrations of 17-β estradiol (ab108667; Abcam, Cambridge, UK) and progesterone (ab108670; Abcam) were measured using commercially available ELISA kits. Testosterone levels were measured by a liquid chromatography-tandem mass spectrometry (LC-MS/MS) method as previously described (46). The plasma concentration of C-reactive protein IV (CRP4) was assessed by particle-enhanced immunoturbidimetric assay (ACN8256; COBAS 8000-c701; Roche Diagnostics, Germany). To evaluate the oxidative stress status, plasma concentrations of malondialdehyde (MDA) (ab118970; Abcam), and activities of catalase (EIACATC; Invitrogen, Waltham, MA, USA) and superoxide dismutase (SOD) (EIASODC; Invitrogen) were assessed by colorimetric assays using commercially available kits.
In an unbiased proteomics approach, 92 additional circulating parameters of inflammation were assessed using the Olink multiplex proximity extension assay (Olink® Proteomics, Uppsala, Sweden). Briefly, 92 oligonucleotides labelled antibody probe pairs were incubated with one microliter of plasma. This results in labelled antibody pairs binding to their respective target proteins when present in the evaluated samples, and the formation of a PCR reporter sequence by a proximity-dependent DNA polymerization. The reporter sequence was subsequently amplified and quantified by real-time PCR (Fluidigm® Biomark™ HD system, San Francisco, CA, USA). Potential intra-assay variability was controlled by analyzing all samples in a single homogeneous 96-well format.
Statistical analyses
Data are reported as mean ± standard deviation (when normally distributed) or median – interquartile range (when skewed) for patients’ basic characteristics and systemic biomarkers concentrations. Skewed data (i.e. p values < 0.05 from Shapiro-Wilks normality test) were log-transformed before analyses. The comparison of the limb volumes of lipedema patients was performed using a paired t-test. The difference in anthropometry and systemic biochemical markers was analyzed using a Mann-Withney Wilcoxon Test and significance levels were set at p < 0.05. The associations between the significantly different biomarkers and anthropometry and clinical measures were evaluated using Pearson correlations.
Multiplex inflammatory protein levels were reported as normalized protein expression (NPX), an arbitrary unit on a log2 scale. The comparison of protein expression levels between the groups was performed using Mann-Whitney Wilcoxon Test, and p values were adjusted for multiple comparison testing using Benjamini-Hochberg (false discovery rate – FDR) method. The heatmap was generated via hierarchical clustering using pheatmap. The reported significance levels correspond to adjusted p values (p_adj) < 0.05. Gene ontology (GO) enrichment analysis was performed and enriched biological processes were considered significant for p_adj <0.01. The analyses were performed using R software (Version 4.1.1, R Foundation for Statistical Computing 2022).
Results
Cohort characteristics
Twenty-six age- and BMI-matched women were included in this study and categorized into a lipedema and control group (Table 1). Women with lipedema have been further classified into different stages. Nine out of 13 lipedema patients were at stage 3, two patients were at stage 2 and two patients were at stage 1 (Table 1). Interestingly, there was a trend for increasing age (Table 1) and BMI (Stage 1: 24.36 ± 0.97 kg/m2, Stage 2: 26.81 ± 1.04 kg/m2; Stage 3: 35.77 ± 3.61 kg/m2) with increasing lipedema stages. The average volume of the arms was 3871 ml (± 517 ml) and that of the legs was 12061 ml (± 2021 ml) in the lipedema group. There were no significant differences between right and left limbs (arms: p=0.828 and legs: p=0.372; Table 1), supporting bilateral and symmetrical SAT accumulation as described in lipedema patients (1–4, 7, 8). There was no significant difference in age (p=0.980) and BMI (p=0.898) between participants from lipedema and control groups (Table 1).
Parameters of glucose metabolism and sex hormones
A panel of biologically plausible biomarkers in both lipedema and control groups are summarized in Table 2. Interestingly, we found lower HbA1c levels in patients with lipedema compared to controls (p<0.001). In addition, fasting insulin and adiponectin concentrations were higher in lipedema compared to the control group (p<0.05). We did not find significant differences in circulating leptin levels between the groups, reflecting the group matching for BMI and suggesting that differences in body fat mass were not significant between the groups (Table 2). Sample haemolysis interfered in the measurement of glucose concentrations which were therefore not reported and insulin sensitivity indices could not be calculated. None of the other measurements was affected by haemolysis, except for ASAT concentrations as reported below. There were no significant differences in circulating levels of C-peptide as well as sex hormones concentrations (17β-Estradiol, progesterone and testosterone) between the groups (p>0.05). Noteworthy, sex hormone concentrations were highly variable between the individuals in the same groups, potentially due to the difference in the menstruation cycle of patients, which was not considered at the time of sample collection, representing a limitation of this study. Despite this inter-individual variability in circulating sex hormone concentrations, there was a trend for higher levels of circulating progesterone (median approx. 3-fold higher) in lipedema compared to the control group (p=0.180; Table 2).
Lipid profile and liver enzymes
Lipid profile analyses revealed higher concentrations of total cholesterol and LDL-C in lipedema compared to control patients (p<0.01), without differences in triglycerides and HDL-C levels between the groups (Table 2). In addition, we assessed the hepatic function by measuring circulating levels of liver enzymes and found higher concentrations of ALAT and ASAT in lipedema compared to the control group (p<0.01) while gGT and lipase levels did not differ between the groups (Table 2). To verify that these changes might be lipedema-related rather than associated with the sex-hormone state of the study participants, we adjusted the significant differences in systemic metabolic circulating cholesterol and liver enzyme concentrations for patients’ age. The reported differences between the groups persisted after adjustment (ALAT: p<0.001; ASAT: p<0.01; total cholesterol: p<0.001; LDL-C: p=0.001). After adjusting these parameters for samples haemolysis index, ASAT concentration was no longer significantly different between the groups (p>0.05).
Distinct levels of systemic oxidative stress markers in lipedema patients
Oxidative stress can be evaluated by the assessment of lipids peroxidation through surrogate measures of biomolecules such as MDA concentrations in biological materials (31). Catalase and SOD represent the first lines of oxidative defence as antioxidant enzymes and can also be measured to evaluate oxidative stress (31). We found significantly higher MDA concentration and catalase and SOD activities in lipedema compared to the control group (p<0.01; Table 2). The cytokine TNFα is one of the most studied markers of inflammation and has been associated with increased oxidative stress (47). Concomitant with higher oxidative stress parameters, we found higher TNFα concentrations in lipedema compared to the control group (p<0.05; Table 2). In contrast, we did not find differences in circulating CRP between the groups.
Correlations between systemic biochemical markers and metabolic parameters
The differences in metabolic status in lipedema compared to individuals with overweight and obesity were further investigated by performing correlation analyses between the distinct biomarkers and selected phenotypic parameters. We tested the hypothesis that associations between age and circulating parameters or among biomarkers are distinct for people with and without lipedema. Interestingly in the lipedema group, insulin (r=0.577; p=0.039), TNFα (r=0.741; p=0.004), total cholesterol (r=0.662; p=0.014) and LDL-C concentrations (r=0.693; p= 0.009) were positively associated with age while none of these correlations was significant for the control group (Figures 1A–D). In both groups, TNFα levels positively correlated with insulin concentrations (lipedema: r=0.605; p=0.029; control: r=0.733; p=0.004). Finally, TNFα concentration was positively associated with HbA1c in the control group (r=0.693; p=0.009) and not in the lipedema group (r=-0.149; p=0.626) (Figures 1E, F).
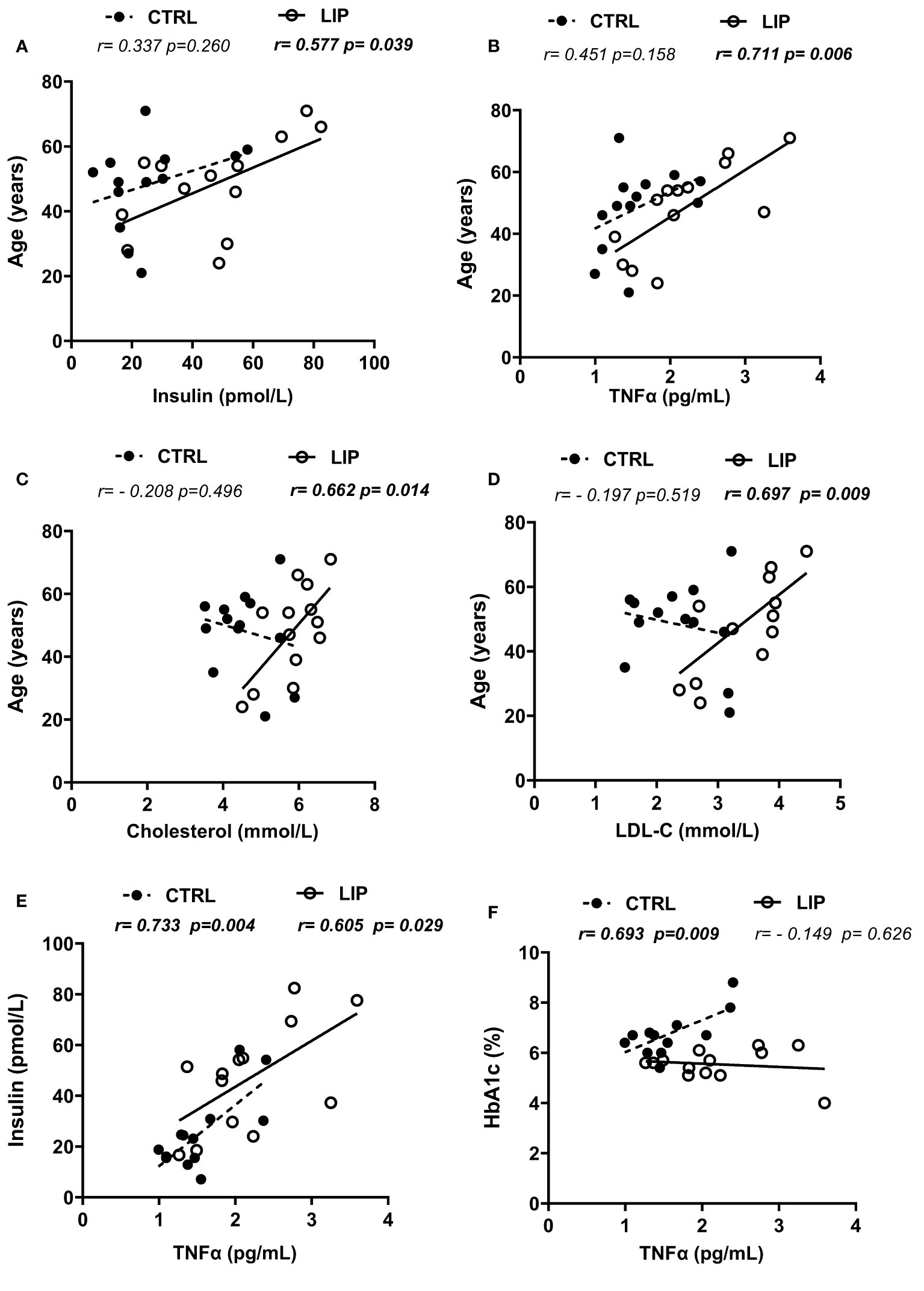
Figure 1 Correlations between biochemical markers and metabolic parameters in lipedema and control groups. (A–D): association between age and circulating concentrations of insulin, TNFα, total cholesterol and LDL-C respectively; (E): correlation between plasma insulin and TNFα levels; (F): correlation between HbA1c percentage and TNFα plasma levels. r coefficients and p values are from Pearson’s pairwise correlations; HbA1c, Hemoglobin A1c; LDL-C, Low-density lipoprotein cholesterol; TNFα, Tumour Necrosis Factor-alpha; CTRL, Control group; LIP, Lipedema group.
Parameters of systemic inflammation in patients with lipedema
Given the higher levels of TNFα and oxidative stress markers in lipedema patients compared to the control group, we further sought to investigate the pattern of pro-inflammatory markers in both groups. Using a high-sensitivity protein expression multiplex proteomics method, we evaluated 92 proteins that play a role in the systemic inflammatory response. Out of the 92 plasma proteins, 14 were excluded from further analysis (IL-2RB, IL-1 alpha, IL-2, TSLP, IL-22 RA1, Beta-NGF, IL-24, IL-13, IL-20, IL-33, IL-4, LIF, NRTN, IL-5) due to the expression levels below the limit of detection in more than 30% of the samples for the given protein. Some of these proteins such as IL-1α, IL-2, TSLP, IL-22 RA1, IL-13, IL-20 and IL-33 have also been reported to be undetectable in both plasma and serum of lean and obese men and women (48). In our study, a total of 78 inflammatory proteins were further included in the analyses (85% detectability percentage). Interestingly, 21 inflammatory proteins were significantly upregulated in the plasma of lipedema patients compared to the control group (adjusted p <0.05), and none were higher in the control compared to the lipedema group (Figure 2A). The upregulated inflammatory proteins in lipedema sorted by lowest adjusted p-value consisted of TNFSF14, CASP8, EN-RAGE (S100-A12), EIF4EBP1, ADA, MCP-1, SIRT2, CXCL11, CCL3, STAMBP, CD6, MCP-3, CCL4, MCP-2, ST1A1, IL-8, LAP TGF-beta-1, AXIN1, MCP-4, TGF-alpha, VEGFA (Figure 2A; Table 3). We further investigated the biological processes and molecular activities represented by these differentially expressed circulating inflammatory proteins. Interestingly, the pathways enriched by these inflammatory proteins were consistently associated with immune cell chemotaxis and migration processes (adjusted p<0.01; Figure 2B). Therefore, a higher systemic inflammatory profile in lipedema could reflect the inflammatory state in the affected SAT, by an increased immune cell attraction and infiltration in this tissue.
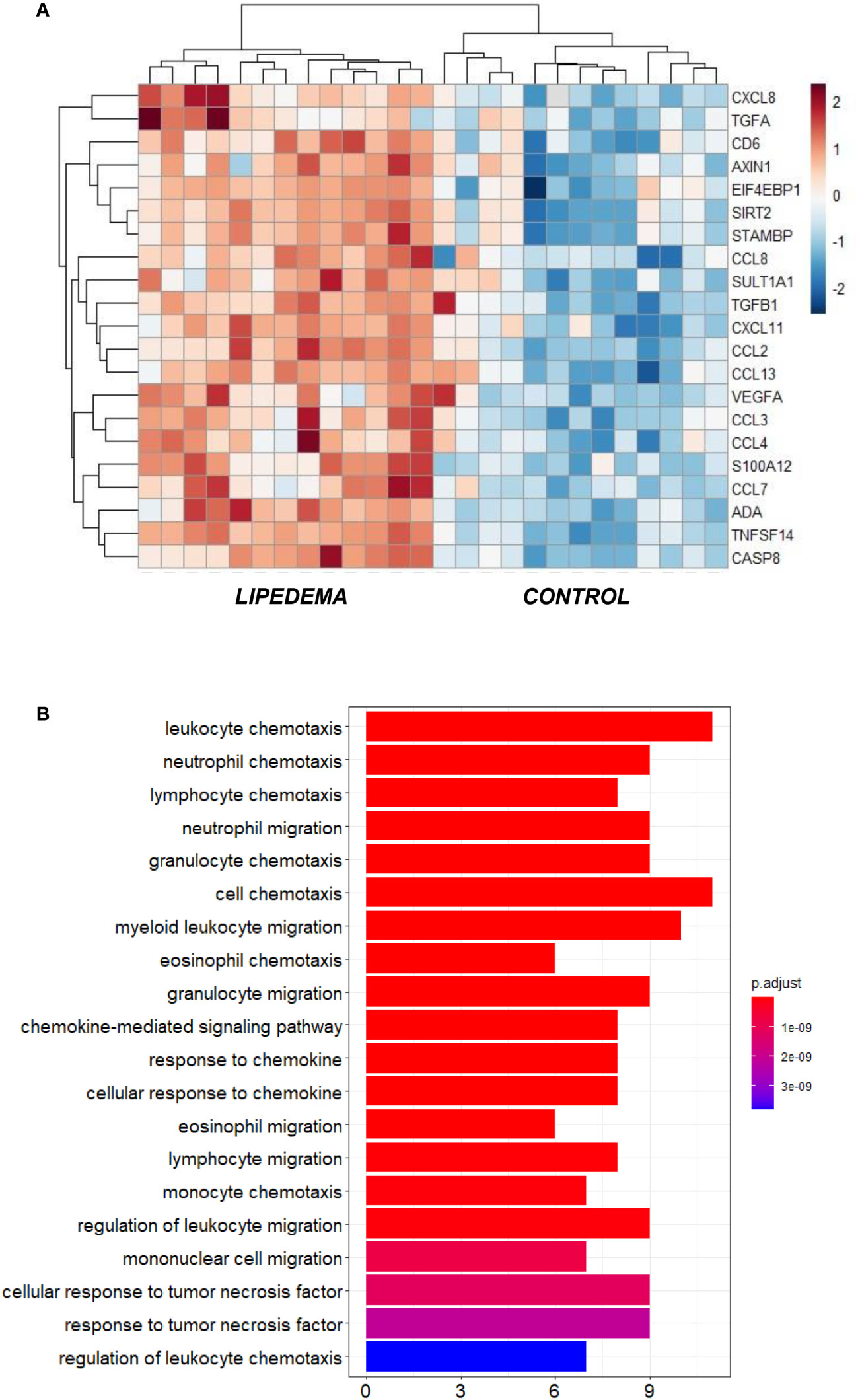
Figure 2 (A) Heatmap visualization of the significantly different inflammatory protein expression in plasma samples of lipedema compared to age- and BMI-matched control group (p_adj < 0.05); (B) Gene ontology enrichment analysis presenting the top 20 biological processes (GO terms) enriched by the differential expressed plasma inflammatory proteins between lipedema and control groups.
Discussion
Using lipedema as a human model to understand mechanisms linking AT (impaired) expandability to metabolic outcomes, and given the current limited data availability on circulating biomarkers of lipedema, we aimed to systematically characterize the plasma profile of carefully selected lipedema patients and to identify the most differentially regulated molecules between lipedema and overweight or obesity patients. We found an unexpected dissociation of lower HbA1c and higher adiponectin in lipedema patients, despite higher fasting insulin concentrations and higher circulating parameters of liver function, inflammation and oxidative stress, compared to age- and BMI-matched women without lipedema. These data generate several novel hypotheses. Higher inflammation and oxidative stress parameters may reflect inflammatory processes in SAT. Activation of inflammatory pathways may subsequently contribute to peripheral insulin resistance that is compensated for by higher insulin secretion. Higher insulin secretion may contribute to lower glucose metabolism parameters in a proposed compensated state. Our data may alternatively suggest that the expected association between activation of inflammatory and oxidative stress pathways and impaired glucose metabolism are counterbalanced by protective factors such as higher adiponectin secretion. Although further studies are warranted to validate these proposed models, we find indications for both explanations, in our comparison between lipedema and control patients.
Lower rates of T2D have been reported in lipedema despite the excessive and disproportionate fat accumulation in this condition (6). We did not assess insulin sensitivity by euglycemic-hyperinsulinemic clamps or HOMA-IR and acknowledge that as one limitation of our study. Higher fasting plasma insulin in our cohort of lipedema patients suggests impaired insulin sensitivity, but in contrast, higher adiponectin plasma concentrations have been associated with improved peripheral and whole-body insulin sensitivity (49–52). Adiponectin is an adipokine almost exclusively secreted by adipocytes, and to a lesser extent during AT expansion in central obesity (53), and higher concentrations of adiponectin correlated with higher AT accumulation in lower extremities (54). Indeed, the influence of expanded AT on whole-body metabolic status is more related to the tissue location (AT distribution) than its total amount (AT mass) (54, 55). Thus, a gynoid distribution of AT has been suggested to be protective against the development of fat-related metabolic complications among which, insulin resistance (54–56). The lower level of HbA1C and reported reduced diabetes risk in lipedema could therefore result from the predominant fat accumulation in the lower body, in contrast to central fat accumulation in obesity.
In lipedema patients, we found higher plasma concentrations of total cholesterol and LDL-C compared to controls. This is similar to the findings of Felmerer et al. where serum levels of total cholesterol and LDL-C ranged from upper normal to pathological values in lipedema compared to BMI-matched controls (40). A less favorable lipid profile could be reflective, among others, of a dysregulated liver function or higher liver fat accumulation. Accordingly, ALAT concentrations were higher in lipedema patients, ranging from the upper half to the normal range. From these findings, we could not suggest a defect in liver function but rather indicative of deterioration in lipids metabolism or liver fat accumulation (57). Noteworthy, our phenotyping did not include analyses of liver fat content, which therefore requires further studies on ectopic fat accumulation despite lipedema. Although higher plasma levels of total cholesterol and LDL-C did not correlate with liver enzymes, these were positively associated with age in lipedema patients. Similarly, the plasma concentration of TNFα (established marker of systemic inflammation) positively correlated with age only in lipedema patients. Interestingly, there was a trend for increased lipedema stages with patients’ age. This suggests that the dysregulation in lipid metabolism and inflammatory state in lipedema may depend on the disease stage. The low number of patients in the different lipedema stages in this study prevents us to subdivide the data to verify this hypothesis.
Lipedema is a suggested inflammatory disease, mostly due to a chronic low-grade pro-inflammatory state in AT. Indeed, an increased immune cell infiltration (mostly macrophages) was shown in AT and skin samples of lipedema patients (36–38, 40, 41). A trend of increase in inflammatory gene expression was also found in adipocytes differentiated from lipedema adipose precursor cells (38). However, fewer studies have investigated systemic inflammation in lipedema. Slightly elevated concentrations of three (IL-11, IL-28A and IL-29) out of 39 evaluated inflammatory proteins were recently shown in the serum of lipedema patients (58). In contrast, no changes in plasma levels of IL-6 were found in lipedema compared to BMI-matched controls (40). We found that 21 of the 78 circulating inflammatory proteins evaluated in our cohort were highly expressed in lipedema compared to obese controls supporting a strong systemic pro-inflammatory signature in lipedema compared to the well-described low-grade inflammation characterizing obesity. Notably, the pathways enriched by these inflammatory proteins consisted of immune cell chemotaxis and migration, suggesting that increased pro-inflammation in lipedema patients could derive from the previously shown increased immune cell infiltration in lipedema SAT (37, 40, 41).
Elevated levels of systemic inflammatory cytokines may not only reflect an increased immune cell infiltration in lipedema SAT but also an impairment in processes related to the vascular system (37). For instance, the cytokines VEGFA, TGFα and TGFβ1 were among the inflammatory markers upregulated in lipedema. VEGF is a lymphatic-related cytokine and marker of angiogenesis, which has been related to increased macrophage infiltrate, inflammation, alteration of vascular permeability and lipedema progression (41, 58, 59). In addition, TGFα is involved in angiogenesis-related processes and inflammation (60, 61), while TGFβ is involved in fibroblast growth, collagen production, fibrosis and angiogenesis (62, 63). In response to excess lipids accumulation, AT mass expansion necessitates extensive remodelling of the extracellular matrix and angiogenesis which in turn require inflammation in the adipocyte microenvironment (64, 65). Therefore the higher inflammatory state described in lipedema AT by other studies could be the consequence of the tissue expansion as observed in lipedema rather than a pathological state in the tissue per se. This could also explain the lack of association between higher inflammation and insulin resistance in lipedema, but the mechanisms that may protect lipedema patients from developing diabetes warrant further investigations.
Increased inflammation can also influence oxidative stress status (31, 47). Accordingly, we show increased oxidative stress in lipedema, determined by elevated concentrations of MDA a surrogate measure of lipids peroxidation in the circulation. One previous study described increased oxidative stress in lipedema with higher serum concentrations of MDA and plasma protein carbonyls in lipedema (59). High levels of MDA may indicate severe preexisting oxidative stress which could be found in long-standing adipocyte inflammatory processes and likely represent an accelerated lipid peroxidation in lipedema AT (66). In addition to high MDA levels, we show an upregulation of antioxidant enzymes catalase and SOD. Oxidative stress results from an imbalance between the production of reactive oxygen species (ROS) or pro-oxidant, and antioxidant enzymes to clear the ROS and maintain the oxidative stress balance (67). Higher levels of antioxidant enzyme activity could be a compensatory response to higher ROS production (67, 68). Oxidative stress can cause damage in AT which has been associated with the activation of stress signalling pathways, impaired adipogenesis, autophagy and apoptosis (31). This could lead to dysregulated adipocytokine signalling, and further increase immune cell infiltration and inflammation (31). In turn, inflammatory cytokines can increase oxidative stress in AT (47). This would generate a vicious cycle between oxidative stress and inflammation, further aggravating the pro-inflammatory state in lipedema. Therefore, oxidative stress might play a pivotal role in the development, or the clinical manifestations of lipedema.
Although this study established differences in circulating markers between lipedema and overweight and obesity, it was limited by the small number of patients and the lack of uniformity in the patient’s menstrual cycle during blood sample collection, particularly for the evaluation of circulating sex hormones and the lack of more insulin sensitivity/resistance measures. Moreover, the pre- vs post-menopausal status of the patients was not considered in this study. These limitations were compensated by the comparable phenotypes of the patients involved (gender, age and BMI) and the extensive measurements of the several circulating biomarkers in both groups, making our findings novel and contributing to advancing the understanding of lipedema pathophysiology.
Conclusion
Patients affected with lipedema have a seemingly preserved glycemic control when compared to obesity, but higher concentrations of total and LDL-cholesterol, ALAT, and inflammatory and oxidative stress parameters. We did not find a single parameter with clinical relevance for lipedema diagnosis. However, we suggest several inflammatory and oxidative stress biomarkers which might reflect the disease, as they are differentially regulated in lipedema compared to overweight and obesity. Further studies are needed to understand whether the most different inflammatory markers between these groups (TNFSF14, CASP8, EN-RAGE, EIF4EBP1, ADA, MCP-1) play a mechanistic role in lipedema development and perpetuation. Although higher circulating inflammation may reflect extensive changes in AT processes, the development and clinical manifestation of lipedema may not be limited to AT function given the other associated symptoms like pain. Therefore, further research studies with a larger sample size should be performed and include AT biopsies and possibly other tissue or systems such as vascular and central nervous systems.
Data availability statement
The raw data supporting the conclusions of this article will be made available by the authors, without undue reservation.
Ethics statement
The studies involving human participants were reviewed and approved by Ethics Committee of the University of Leipzig. The patients/participants provided their written informed consent to participate in this study.
Author contributions
MB and MC conceived the study. PN, MC and NK generated the data. PN analyzed the data. PN and MB wrote the first draft of the manuscript. All authors contributed to the article and approved the submitted version.
Acknowledgments
We thank all women that participated in this study. We also thank the nurses, the clinical and laboratory staff of Ly.search GmbH and the Medical Department III of the University Hospital of Leipzig who assisted with the sample collection and analyses.
Conflict of interest
MB received honoraria as a consultant and speaker from Amgen, AstraZeneca, Bayer, Boehringer-Ingelheim, Lilly, Novo Nordisk, Novartis and Sanofi. MC is the research director of Ly.Search GmbH, Germany.
The remaining authors declare that the research was conducted in the absence of any commercial or financial relationships that could be construed as a potential conflict of interest.
Publisher’s note
All claims expressed in this article are solely those of the authors and do not necessarily represent those of their affiliated organizations, or those of the publisher, the editors and the reviewers. Any product that may be evaluated in this article, or claim that may be made by its manufacturer, is not guaranteed or endorsed by the publisher.
References
1. Herbst KL, Kahn LA, Iker E, Ehrlich C, Wright T, McHutchison L, et al. Standard of care for lipedema in the united states. Phlebology (2021) 36(10):779–96. doi: 10.1177/02683555211015887
2. Herbst KL. Rare adipose disorders (RADs) masquerading as obesity. Acta Pharmacol Sin (2012) 33(2):155–72. doi: 10.1038/aps.2011.153
3. Child AH, Gordon KD, Sharpe P, Brice G, Ostergaard P, Jeffery S, et al. Lipedema: an inherited condition. Am J Med Genet A (2010) 152A(4):970–6. doi: 10.1002/ajmg.a.33313
4. Torre YS, Wadeea R, Rosas V, Herbst KL. Lipedema: friend and foe. Horm Mol Biol Clin Investig (2018) 33(1):1–15. doi: 10.1515/hmbci-2017-0076
5. Chen GC, Arthur R, Iyengar NM, Kamensky V, Xue X, Wassertheil-Smoller S, et al. Association between regional body fat and cardiovascular disease risk among postmenopausal women with normal body mass index. Eur Heart J (2019) 40(34):2849–55. doi: 10.1093/eurheartj/ehz391
6. Beltran K, Herbst KL. Differentiating lipedema and dercum’s disease. Int J Obes (Lond) (2017) 41(2):240–5. doi: 10.1038/ijo.2016.205
7. Cornely M. [Lymphology]. J Dtsch Dermatol Ges (2006) 4(7):564–78. doi: 10.1111/j.1610-0387.2006.05954.x
8. Buso G, Depairon M, Tomson D, Raffoul W, Vettor R, Mazzolai L. Lipedema: A call to action! Obes (Silver Spring) (2019) 27(10):1567–76. doi: 10.1002/oby.22597
9. Paolacci SPV, Acquaviva F, Chiurazzi P, Fulcheri E, Pinelli M, Buffeli F, et al. Genetics of lipedema: new perspectives on genetic research and molecular diagnoses. Eur Rev Med Pharmacol Sci (2019) 23:5581–94. doi: 10.26355/eurrev_201907_18292
10. Al-Ghadban S, Herbst KL, Bunnell BA. Lipedema: A painful adipose tissue disorder. In: Adipose tissue–an update. London, UK: IntechOpen (2019).
11. Cornely ME, Hasenberg T, Cornely OA, Ure C, Hettenhausen C, Schmidt J. Persistent lipedema pain in patients after bariatric surgery: a case series of 13 patients. Surg Obes Relat Dis (2022) 18:628–33. doi: 10.1016/j.soard.2021.12.027
12. Dudek JE, Bialaszek W, Ostaszewski P. Quality of life in women with lipoedema: a contextual behavioral approach. Qual Life Res (2016) 25(2):401–8. doi: 10.1007/s11136-015-1080-x
13. Buck DW 2nd, Herbst KL. Lipedema: A relatively common disease with extremely common misconceptions. Plast Reconstr Surg Glob Open (2016) 4(9):e1043. doi: 10.1097/GOX.0000000000001043
14. Allen EV HE. Lipedema of the legs: a syndrome characterised by fat legs and orthostatic edema. Proc Staff Meet Mayo Clin (1940) 15:184–7. doi: 10.7326/0003-4819-34-5-1243
15. Scherer PE. The many secret lives of adipocytes: implications for diabetes. Diabetologia (2019) 62(2):223–32. doi: 10.1007/s00125-018-4777-x
16. Reich-Schupke S, Schmeller W, Brauer WJ, Cornely ME, Faerber G, Ludwig M, et al. S1 guidelines: Lipedema. J Dtsch Dermatol Ges (2017) 15(7):758–67. doi: 10.1111/ddg.13036
17. Herbst KL, Mirkovskaya L, Bharhagava A, Chava Y, Te CHT. Lipedema fat and signs and symptoms of illness, increase with advancing stage. Arch Med (2015) 7:1–8.
18. M. M, and S.- S. C., prävalenz des lipödems bei berufstätigen frauen in deutschland (Lipödem-3-Studie). Phlebologie (2011) 3:127–34. doi: 10.1055/s-0037-162176
19. Cornely ME. Fatter through lipids or water: Lipohyperplasia dolorosa versus lymphedema. Am J Cosmetic Surg (2014) 31(3):189–95. doi: 10.5992/AJCS-D-12-00026.1
20. Blüher M. Adipose tissue dysfunction contributes to obesity related metabolic diseases. Best Pract Res Clin Endocrinol Metab (2013) 27(2):163–77. doi: 10.1016/j.beem.2013.02.005
21. Almuraikhy S, Kafienah W, Bashah M, Diboun I, Jaganjac M, Al-Khelaifi F, et al. Interleukin-6 induces impairment in human subcutaneous adipogenesis in obesity-associated insulin resistance. Diabetologia (2016) 59(11):2406–16. doi: 10.1007/s00125-016-4031-3
22. Dubois SG, Heilbronn LK, Smith SR, Albu JB, Kelley DE, Ravussin E, et al. Decreased expression of adipogenic genes in obese subjects with type 2 diabetes. Obes (Silver Spring) (2006) 14(9):1543–52. doi: 10.1038/oby.2006.178
23. Gealekman O, Guseva N, Hartigan C, Apotheker S, Gorgoglione M, Gurav K, et al. Depot-specific differences and insufficient subcutaneous adipose tissue angiogenesis in human obesity. Circulation (2011) 123(2):186–94. doi: 10.1161/CIRCULATIONAHA.110.970145
24. Bouloumie A, Lolmede K, Sengenes C, Galitzky J, Lafontan M. Angiogenesis in adipose tissue. Annales d’Endocrinol (Paris) (2002) 63:91–5.
25. Divoux A, Tordjman J, Lacasa D, Veyrie N, Hugol D, Aissat A, et al. Fibrosis in human adipose tissue: Composition, distribution, and link with lipid metabolism and fat mass loss. Diabetes (2010) 59(11):2817–25. doi: 10.2337/db10-0585
26. Pasarica M, Sereda OR, Redman LM, Albarado DC, Hymel DT, Roan LE, et al. Reduced adipose tissue oxygenation in human obesity: evidence for rarefaction, macrophage chemotaxis, and inflammation without an angiogenic response. Diabetes (2009) 58(3):718–25. doi: 10.2337/db08-1098
27. Trayhurn P. Hypoxia and adipose tissue function and dysfunction in obesity. Physiol Rev (2013) 93(1):1–21. doi: 10.1152/physrev.00017.2012
28. Suganami T, Nishida J, Ogawa Y. A paracrine loop between adipocytes and macrophages aggravates inflammatory changes: role of free fatty acids and tumor necrosis factor alpha. Arterioscler Thromb Vasc Biol (2005) 25(10):2062–8. doi: 10.1161/01.ATV.0000183883.72263.13
29. Blüher M. Adipose tissue dysfunction in obesity. Exp Clin Endocrinol Diabetes (2009) 117:241–50. doi: 10.1055/s-0029-1192044
30. Pellegrinelli V, Carobbio S, Vidal-Puig A. Adipose tissue plasticity: how fat depots respond differently to pathophysiological cues. Diabetologia (2016) 59(6):1075–88. doi: 10.1007/s00125-016-3933-4
31. Nono Nankam PA, Nguelefack TB, Goedecke JH, Blüher M. Contribution of adipose tissue oxidative stress to obesity-associated diabetes risk and ethnic differences: Focus on women of African ancestry. Antioxidants (2021) 10(4):1. doi: 10.3390/antiox10040622
32. Lacobini C, Pugliese G, Fantauzz BC, Federici M, Menini S. Metabolically healthy versus metabolically unhealthy obesity. Metab Clin Exp (2019) 92:51–60. doi: 10.1016/j.metabol.2018.11.009
33. Maury E, Brichard SM. Adipokine dysregulation, adipose tissue inflammation and metabolic syndrome. Mol Cell Endocrinol (2010) 314(1):1–16. doi: 10.1016/j.mce.2009.07.031
34. McLaughlin T, Lamendola C, Coghlan N, Liu TC, Lerner K, Sherman A, et al. Subcutaneous adipose cell size and distribution: relationship to insulin resistance and body fat. Obes (Silver Spring) (2014) 22(3):673–80. doi: 10.1002/oby.20209
35. Ishaq M, Bandara N, Morgan S, Nowell C, Mehdi AM, Lyu R, et al. Key signaling networks are dysregulated in patients with the adipose tissue disorder, lipedema. Int J Obes (Lond) (2021) 46:502–14. doi: 10.1038/s41366-021-01002-1
36. Suga H, Araki J, Aoi N, Kato H, Higashino T, Yoshimura K. Adipose tissue remodeling in lipedema: adipocyte death and concurrent regeneration. J Cutaneous Pathol (2009) 36(12):1293–8. doi: 10.1111/j.1600-0560.2009.01256.x
37. Al-Ghadban S, Cromer W, Allen M, Ussery C, Badowski M, Harris D, et al. Dilated blood and lymphatic microvessels, angiogenesis, increased macrophages, and adipocyte hypertrophy in lipedema thigh skin and fat tissue. J Obes 2019 (2019) p:8747461. doi: 10.1155/2019/8747461
38. Al-Ghadban S, Diaz ZT, Singer HJ, Mert KB, Bunnell BA. Increase in leptin and PPAR-gamma gene expression in lipedema adipocytes differentiated in vitro from adipose-derived stem cells. Cells (2020) 9(2):1–13. doi: 10.3390/cells9020430
39. Szel E, Kemeny L, Groma G, Szolnoky G. Pathophysiological dilemmas of lipedema. Med Hypotheses (2014) 83(5):599–606. doi: 10.1016/j.mehy.2014.08.011
40. Felmerer G, Stylianaki A, Hagerling R, Wang A, Strobel P, Hollmen M, et al. Adipose tissue hypertrophy, an aberrant biochemical profile and distinct gene expression in lipedema. J Surg Res (2020) 253:294–303. doi: 10.1016/j.jss.2020.03.055
41. Felmerer G, Stylianaki A, Hollmen M, Strobel P, Stepniewski A, Wang A, et al. Increased levels of VEGF-c and macrophage infiltration in lipedema patients without changes in lymphatic vascular morphology. Sci Rep (2020) 10(1):10947. doi: 10.1038/s41598-020-67987-3
42. Kloting N, Fasshauer M, Dietrich A, Kovacs P, Schon MR, Kern M, et al. Insulin-sensitive obesity. Am J Physiol Endocrinol Metab (2010) 299(3):E506–515. doi: 10.1152/ajpendo.00586.2009
43. Blüher M. Clinical relevance of adipokines. Diabetes Metab J (2012) 36(5):317–27. doi: 10.4093/dmj.2012.36.5.317
44. Sell H, Habich C, Eckel J. Adaptive immunity in obesity and insulin resistance. Nat Rev Endocrinol (2012) 8(12):709–16. doi: 10.1038/nrendo.2012.114
45. KL. H. Subcutaneous adipose tissue diseases: Dercum disease, lipedema, familial multiple lipomatosis, and madelung disease. In: Purnell J, Perreault L, editors. South Dartmouth (MA: Endotext: MDText.com (2019).
46. Gaudl A, Kratzsch J, Bae YJ, Kiess W, Thiery J, Ceglarek U. Liquid chromatography quadrupole linear ion trap mass spectrometry for quantitative steroid hormone analysis in plasma, urine, saliva and hair. J Chromatogr A (2016) 1464:64–71. doi: 10.1016/j.chroma.2016.07.087
47. Huang CJ, McAllister MJ, Slusher AL, Webb HE, Mock JT, Acevedo EO. Obesity-related oxidative stress: the impact of physical activity and diet manipulation. Sports Med Open (2015) 1(1):32. doi: 10.1186/s40798-015-0031-y
48. Rajan MR, Sotak M, Barrenas F, Shen T, Borkowski K, Ashton NJ, et al. Comparative analysis of obesity-related cardiometabolic and renal biomarkers in human plasma and serum. Sci Rep (2019) 9(1):15385. doi: 10.1038/s41598-019-51673-0
49. Blüher M. Do adipokines link obesity to its related metabolic and cardiovascular diseases? Clin Lipidol (2010) 5(1):95–107. doi: 10.2217/clp.09.86
50. Li N, Zhao S, Zhang Z, Zhu Y, Gliniak CM, Vishvanath L, et al. Adiponectin preserves metabolic fitness during aging. Elife (2021) 10:1–21. doi: 10.7554/eLife.65108
51. Morley TS, Xia JY, Scherer PE. Selective enhancement of insulin sensitivity in the mature adipocyte is sufficient for systemic metabolic improvements. Nat Commun (2015) 6:7906. doi: 10.1038/ncomms8906
52. Ye R, Scherer PE. Adiponectin, driver or passenger on the road to insulin sensitivity? Mol Metab (2013) 2(3):133–41. doi: 10.1016/j.molmet.2013.04.001
53. Turer AT, Scherer PE. Adiponectin: mechanistic insights and clinical implications. Diabetologia (2012) 55(9):2319–26. doi: 10.1007/s00125-012-2598-x
54. Turer AT, Khera A, Ayers CR, Turer CB, Grundy SM, Vega GL, et al. Adipose tissue mass and location affect circulating adiponectin levels. Diabetologia (2011) 54(10):2515–24. doi: 10.1007/s00125-011-2252-z
55. Grundy SM, Adams-Huet B, Vega GL. Variable contributions of fat content and distribution to metabolic syndrome risk factors. Metab Syndr Relat Disord (2008) 6(4):281–8. doi: 10.1089/met.2008.0026
56. Pinnick KE, Nicholson G, Manolopoulos KN, McQuaid SE, Valet P, Frayn KN, et al. Distinct developmental profile of lower-body adipose tissue defines resistance against obesity-associated metabolic complications. Diabetes (2014) 63:3785–97. doi: 10.2337/db14-0385
57. Burgert TS, Taksali SE, Dziura J, Goodman TR, Yeckel CW, Papademetris X, et al. Alanine aminotransferase levels and fatty liver in childhood obesity: associations with insulin resistance, adiponectin, and visceral fat. J Clin Endocrinol Metab (2006) 91(11):4287–94. doi: 10.1210/jc.2006-1010
58. Wolf S, Deuel JW, Hollmen M, Felmerer G, Kim BS, Vasella M, et al. A distinct cytokine profile and stromal vascular fraction metabolic status without significant changes in the lipid composition characterizes lipedema. Int J Mol Sci (2021) 22(7):1–14. doi: 10.3390/ijms22073313
59. Siems W, et al. Anti-fibrosclerotic effects of shock wave therapy in lipedema and cellulite. Biofactors Oxf Engl (2005) 24:275–82. doi: 10.1002/biof.5520240132
60. Peluzzo AM, Autieri MV. Challenging the paradigm: Anti-inflammatory interleukins and angiogenesis. Cells (2022) 11(3):1–11. doi: 10.3390/cells11030587
61. Leker RR, Toth ZE, Shahar T, Cassiani-Ingoni R, Szalayova I, Key S, et al. Transforming growth factor alpha induces angiogenesis and neurogenesis following stroke. Neuroscience (2009) 163(1):233–43. doi: 10.1016/j.neuroscience.2009.05.050
62. Kubiczkova L, Sedlarikova L, Hajek R, Sevcikova S. TGF-β – an excellent servant but a bad master. J Trans Med (2012) 10(183):1–24. doi: 10.1186/1479-5876-10-183
63. Lee SG, Kim JS, Kim HJ, Schlaepfer DD, Kim IS, Nam JO. Endothelial angiogenic activity and adipose angiogenesis is controlled by extracellular matrix protein TGFBI. Sci Rep (2021) 11(1):9644. doi: 10.1038/s41598-021-88959-1
64. Asterholm IW, Tao C, Morley TS, Wang QA, Delgado-Lopez F, Wang ZV, et al. Adipocyte inflammation is essential for healthy adipose tissue expansion and remodeling. Cell Metab (2014) 20:103–18. doi: 10.1016/j.cmet.2014.05.005
65. Fischer IP, Irmler M, Meyer CW, Sachs SJ, Neff F, Hrabe de Angelis M, et al. A history of obesity leaves an inflammatory fingerprint in liver and adipose tissue. Int J Obes (Lond) (2018) 42(3):507–17. doi: 10.1038/ijo.2017.224
66. Fife CE, Maus EA, Carter MJ. Lipedema: a frequently misdiagnosed and misunderstood fatty deposition syndrome. Adv Skin Wound Care (2010) 23(2):81–92. doi: 10.1097/01.ASW.0000363503.92360.91
67. Perriotte-Olson C, Adi N, Manickam DS, Westwood RA, Desouza CV, Natarajan G, et al. Nanoformulated copper/zinc superoxide dismutase reduces adipose inflammation in obesity. Obes (Silver Spring) (2016) 24(1):148–56. doi: 10.1002/oby.21348
Keywords: lipedema, adipose tissue, inflammation, oxidative stress, glucose metabolism
Citation: Nono Nankam PA, Cornely M, Klöting N and Blüher M (2022) Is subcutaneous adipose tissue expansion in people living with lipedema healthier and reflected by circulating parameters? Front. Endocrinol. 13:1000094. doi: 10.3389/fendo.2022.1000094
Received: 25 July 2022; Accepted: 14 October 2022;
Published: 31 October 2022.
Edited by:
Francesca Bonomini, University of Brescia, ItalyReviewed by:
Shangang Zhao, University of Texas Southwestern Medical Center, United StatesPetra Kotzbeck, Medical University of Graz, Austria
Copyright © 2022 Nankam, Cornely, Klöting and Blüher. This is an open-access article distributed under the terms of the Creative Commons Attribution License (CC BY). The use, distribution or reproduction in other forums is permitted, provided the original author(s) and the copyright owner(s) are credited and that the original publication in this journal is cited, in accordance with accepted academic practice. No use, distribution or reproduction is permitted which does not comply with these terms.
*Correspondence: Pamela A. Nono Nankam, pamela.nononankam@helmholtz-muenchen.de; Matthias Blüher, bluma@medizin.uni-leipzig.de