- 1Urology Unit, Department of Woman, Child and General and Specialized Surgery, University of Campania “Luigi Vanvitelli”, Caserta, Italy
- 2Department of Neurosciences, Reproductive Sciences and Odontostomatology, Urology Unit, University of Naples “Federico II”, Naples, Italy
- 3Department of Woman, Child and General and Specialized Surgery, Urology Unit, University of Campania Luigi Vanvitelli, Naples, Italy
The purpose of this mini review is to provide data about pre-clinical and clinical evidence exploring the impact of circadian desynchrony on spermatogenesis. Several lines of evidence exist demonstrating that disruption of circadian rhythms may interfere with male fertility. Experimental knock-out or knock-down of clock genes, physiologically involved in the regulation of circadian rhythms, are associated with impairments of fertility pathways in both animal and human models. Moreover, disruption of circadian rhythms, due to reduction of sleep duration and/or alteration of its architecture can negatively interfere in humans with circulating levels of male sexual hormones as well as with semen parameters. Unfortunately, current evidence remains low due to study heterogeneity.
Introduction
Globally, it has been estimated that infertility affects approximately 8-12% of couples, with a male factor being a primary or contributing cause in about 50% of couples (1, 2). Unfortunately, the cause of male infertility is unknown in about 30% of these cases (3, 4). Environmental endocrine disruptors, often consequences of human activity, have been widely investigated as agents potentially involved into the pathogenesis of infertility in animals and humans (5, 6). Over the past 30 years primary focus has been directed to the effects of chemicals environmental endocrine disruptors found in plasticizers, pharmaceuticals, and pesticides such as bisphenol A or glyphosate (7, 8). In recent years, however, the effects of non-chemical environmental endocrine disruptors such as that interfering with circadian rhythms (CR) leading to circadian desynchrony (CD) also gained growing interest in the pathophysiology of male infertility (5). The aim of this mini review is to provide the latest information on pre-clinical and clinical evidence about the relationship between CD and spermatogenesis.
Methods
The authors conducted a literature search of available sources evaluating the pathophysiology and clinical evidence about the relationship between CD and impaired male reproduction, with a special focus on spermatogenesis. Web of Science, PubMed, and Scopus databases were searched to find relevant articles. The information found in the selected studies was carefully evaluated and it is described and discussed in the following sections.
CR and CD: Definition and Pathophysiology
CR consist in daily oscillations in physiology processes (gene expression, metabolism, activity patterns and serum hormone levels) and behavior recurring with a 24h period. CR represent an ubiquitous feature in living organisms: they modulate function since unicellular life and gained an higher complexity multicellular organisms (9, 10).
In vertebrates, CR are hierarchically organized. They are both autonomous, based on cellular cycle which builds rhythmic activity of tissues, and controlled by synchronization through environmental signals (11). The main regulator of these rhythms is represented by the hypothalamic suprachiasmatic nucleus (SCN) (12). The cells in the SCN orchestrate rhythms in endocrine, physiological, and behavioral parameters through the activation of other central circadian oscillators (e.g., the hypothalamus and pituitary gland), with a period close to, but not exactly 24 hours (13, 14).
CR are strongly entrained by the daily photoperiod as they are influenced by the environmental light-dark cycle which has been reported to modulate the expression of several genes (15). Therefore, light is the most effective environmental synchronizing agent for the clock of human beings: it represents a direct drive to the nervous system through activation of intrinsic photosensitive retinal ganglion cells and transduced directly to the SCN through the retinohypothalamic tract (16), and an indirectly through the intergeniculate leaflet (17, 18).
Clock genes play a central role in orchestrating CR. In mammals, the main clock genes include: Period genes (Per 1/2/3, Period Circadian Regulator1/2/3), Circadian Locomotor Output Cycles Kaput (Clock) gene, Cryptochrome genes (Cry 1/2, Cryptochrome Circadian Regulator 1/2) and Aryl hydrocarbon receptor nuclear translocator-like protein 1 (ARNTL, also known as MOP3 or Bmal1) (19). Clock genes are involved in several physiological processes and diseases including ageing, metabolism, fertility, cardiovascular health, and cell proliferation (20, 21).
Throughout the last two centuries, modern lifestyles increasingly deprive us of natural zeitgebers (dt. time-givers) and technological advances have dramatically changed individual work and rest patterns (22–24). Electricity and constant accessibility to light, energy, food, the possibility to travel across time zones created an environment that is different from the one of the last two centuries. These environmental perturbances strongly contribute to the pathophysiology of CD, a condition defined for the first time by Sack & Lewy in 1997 as a specific type of circadian disruption occurring when endogenous rhythms become misaligned with daily photoperiodic cycles (25–27).
Growing evidence support the hypothesis that disruption of CR is involved in the pathophysiology of several diseases such as metabolic impairment, cardiovascular and sleep disorders, psychiatric illness, cancers such as urothelial carcinoma, and infertility (28–32).
CD and Male Infertility
Physiological processes regulating fertility need to be appropriately synchronized with the external environment to guarantee reproductive success. In the testis several models of temporal organization had been found. The complexity of its rhythmic function is linked to its structure divided into compartments (33). In the seminiferous tubule there is a spermatogenic wave that travels along in length to determine the timing of the commitment of spermatogonia to differentiate, the phases of meiotic division, and the rate of differentiation of the post-meiotic germ cells (33). Leydig cells, localized in the interstitial space, produce steroid hormones to ensure spermatozoa maturation and the sexual characteristics (33).
Several lines of evidence exist suggesting that disruption of mechanisms involved in CR may interfere with male fertility.
Mutations of Clock Genes and Male Infertility
Clock proteins have been reported to be expressed in male germ epithelium. In details, BMAL1 localizes mainly in Leydig cells but it can also be found in the nucleus and cytoplasm of germ cells and CLOCK was observed having a higher expression level in the cytoplasm of round spermatids (34).
Both pre-clinical and clinical evidence suggest the involvement of clock genes in the pathophysiology of male infertility.
Table 1 summarizes evidence from pre-clinical animal studies.
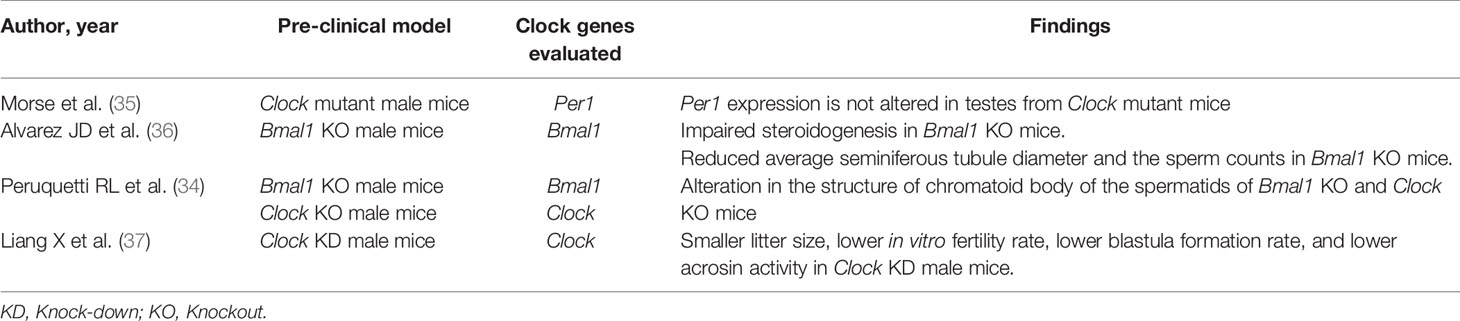
Table 1 Findings from pre-clinical studies investigating the role of clock genes on spermatogenesis.
Alvarez et al. used Bmal1 knockout (KO) mice in order to clarify the role of this circadian clock protein in the fertility process and its role in testosterone production (36). Authors found that male Bmal1 KO mice were infertile and had lower testosterone and higher luteinizing hormone (LH) serum concentrations, compared to wild-type mice thus suggesting a defect in testicular Leydig cells. Testes and other steroidogenic tissues of Bmal1 KO mice exhibited reduced expression of steroidogenic genes. Expression of the steroidogenic acute regulatory protein (StAR) gene and protein, which regulates the rate-limiting step of steroidogenesis, was decreased in testes from Bmal1 KO mice. Microscopically, testes from Bmal1 KO mice exhibited reduced average seminiferous tubule diameter (by approximately 20%) and the sperm counts were reduced by approximately 70%. Homozygous Bmal1 KO mice were unable to breed with each other (36).
Peruquetti et al. analyzed the role of two genes (Clock and Bmal1) in the chromatoid body. This cytoplasmic organelle plays a crucial role in RNA post-transcriptional and translation regulation during the germ cell differentiation. They detect an alteration in the structure of chromatoid body of the spermatids of Bmal1 KO and Clock KO mice (34).
Liang et al. knocked down the Clock gene expression in the testes of male mice and determined its effect on fertility. Authors recorded lower in vitro fertilization rate, lower blastula formation rate, and a lower acrosin activity in the Clock Knockdown sperms, as well as a delay in dispersing cumulus cells. These results demonstrate that acrosin activity could be regulated by Clock and that Clock contributes to the regulation of male fertility and blastula formation (37).
Cheng et al. further corroborated these observation: they verified that acrosin activity and in vitro fertilization rate were reduced in SERPINA3K-treated sperm, producing a pattern of phenotypes very similar to that previously observed in the Clock knockdown sperm (38).
Unfortunately, the circadian expression of clock genes in testis tissue remains controversial.
Morse et al. investigated the role and the expression of Per1 in the mice testis, reporting a constant expression of the gene during the 12-h light and 12-h dark cycles. Moreover, the levels of Per1 proteins were constant during the day (35).
Human studies also provided evidence about the involvement of clock genes in male infertility.
Zhang et al. demonstrated, for the first time, an association between CLOCK genetic variants and altered semen quality in a human population with idiopathic infertility (39). In details, authors investigated the association between genetic variants of CLOCK and semen quality in humans. Authors examined three Single-Nucleotide-Polimorfism (SNP) of the CLOCK gene, (i.e. rs3749474, rs1801260 and rs3817444) to assess the association between these variants and semen quality in men with idiopathic infertility. The results indicated a strong association between the C allele carriers (CC or CT) of rs374947 and significantly reduced semen volume and sperm number per ejaculate. Moreover, associations between the A allele carriers (CA or AA) of rs3817444 and significantly reduced semen volume as well as between both the rs3749474 CC genotype and rs1801260 TC genotype and significantly decreased sperm motility were found (40).
CD and Reproductive Hormones
Circulating levels of male sexual hormones are modulated by circadian clock, light exposure, and sleep duration. The circadian pattern of LH and testosterone have been widely investigated in healthy males. In healthy young men, serum testosterone concentrations rise with sleep onset, reaches the peak during the first REM episode, remains stable until awakening, and then rapidly declines (41). Sleep-related elevations in LH have been also reported. Total sleep time and durations of stage 2 and REM have been reported to be positively related to morning testosterone levels (41). Zhang et al. found a significant correlation between sleep duration (measured by actigraphy) and follicle stimulating hormone (FSH) levels as well as by rapid eye movement sleep and FSH in healthy young men (42).
Total sleep deprivation or sleep restriction have been shown to impair the secretory activity of the pituitary-testis axis.
Leproult et al. investigated the effect of 1 week of sleep restriction to 5 hours per night (a condition experienced by at least 15% of the US working population) on testosterone levels in young healthy men and found a daytime decrease of testosterone levels by 10% to 15% (43).
Schmid et al. aimed to discriminate the effects of sleep duration and sleep timing on serum concentrations of LH and testosterone (44). They failed to find differences in terms of serum LH and testosterone concentrations between patents with sleep time restriction to 4 h for two consecutive nights (bedtime, 02:45 -07:00 h) and a control condition of 8 h regular sleep (bedtime, 22:45-07:00 h). However, total sleep deprivation and 4·5 h of sleep restricted to the first night-half (bedtime, 22:30-03:30 h) markedly decreased morning testosterone concentrations (44).
Interestingly, testosterone level has been reported to recover basal concentrations after one night of recovery sleep. However, extending sleep duration by approximately 1.2 h/night over six nights has minimal effects on hormonal responses to total sleep deprivation (41).
Yoon et al. investigated the levels of urinary LH in normal young men aged between 19 and 30 years following early morning light exposure (05:00 – 06.00). They found that LH excretion was increased 69.5% after bright light exposure but was not changed by placebo light exposure (45).
Despite previous results, Chen et al. failed to find significant association between sleep duration and reproductive hormone levels (follicle-stimulating hormone, LH, estradiol, progesterone, testosterone, and prolactin (46).
CD and Spermatogenesis in Humans
Evidence about the influence of CD on spermatogenesis in humans derive mainly from studies about the relationship between sleep duration/architecture and sperm parameters (Table 2).
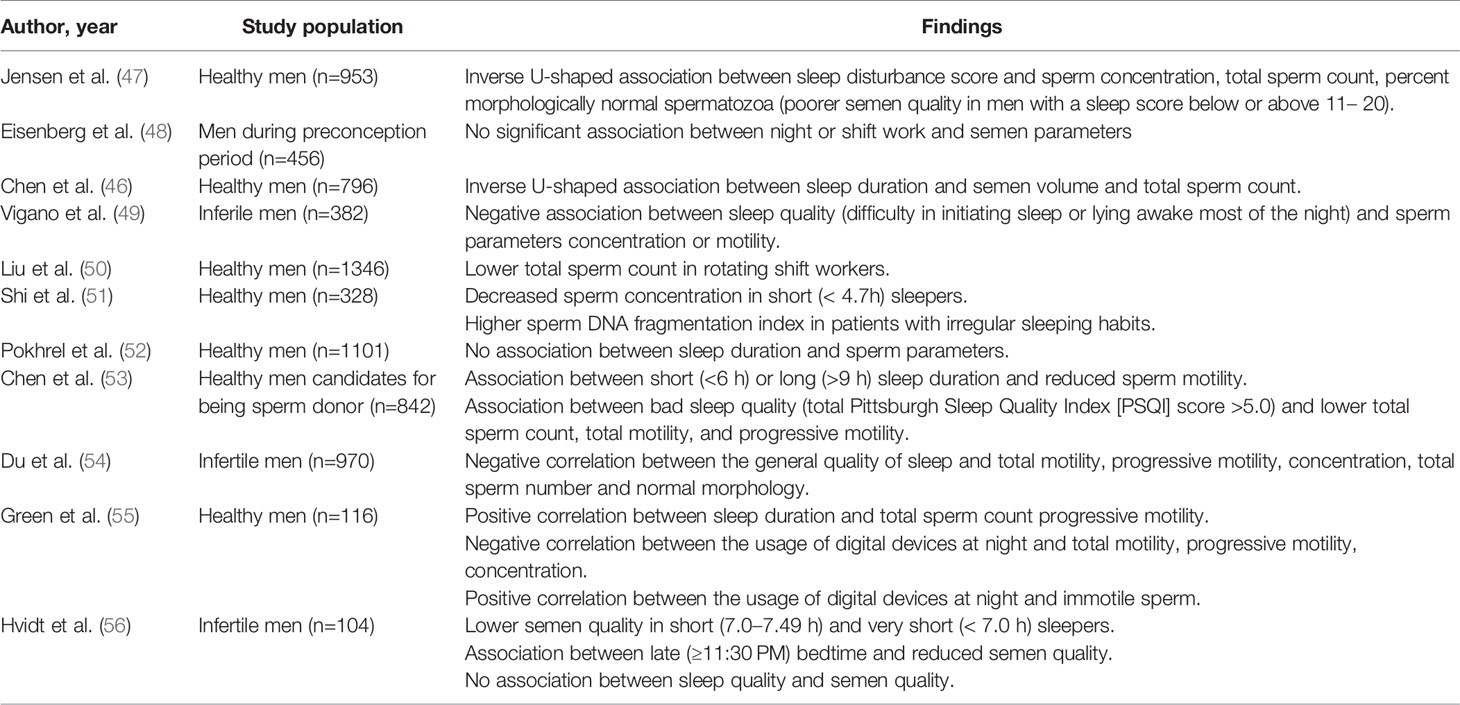
Table 2 Findings from clinical studies investigating the association between sleep duration/quality and semen parameters.
Zhang et al. found a significant correlation between sleep duration (measured by actigraphy) and testis volume (42).
Chen Q. et al. recorded an inversed U-shaped association between the entire sleep duration and two of the semen parameter (semen volume and total sperm count) with 7.0-7.5 h/d as the “turning point”: for those whose sleep duration was below 7.0 h/d the semen parameters increased with longer sleep duration; but the semen parameters decreased, with longer sleep duration, for those whose sleep duration was over 7.5 h/d (46).
Similarly, Chen H-G. et al. investigated association between sleep duration and quality of the semen samples (53). Authors found a better quality of the semen (sperm volume, concentration and total count) in men who slept between 8.0 and 8.5 hours per day (53). Men who slept less than 6.0 h/day and higher than 9.0 h/day had lower sperm volume of 12% and 3.9%, respectively. Men who slept less than 6.0 h/day had lower total and progressive sperm motility of 4.4% and 5.0%, respectively. Moreover, men reporting poor sleep quality (total Pittsburgh Sleep Quality Index [PSQI] score >5.0) had lower total sperm count, total motility, and progressive motility of 8.0%, 3.9%, and 4.0%, respectively (53).
Jensen et al. demonstrated an inverse U-shaped association between sleep disturbance score and sperm concentration, total sperm count, percent morphologically normal spermatozoa (47).
Du et al. recorded a negative correlation between the general quality of sleep and several semen parameters (total motility, progressive motility, concentration, total sperm number and normal morphology) although semen volume and reproductive hormones were not markedly altered (54).
Green et al. recorded a positive statistically significant correlation between sleep duration and some semen parameters (total sperm count and progressive motility) (55). Additionally, authors recorded for the first time a negative significant correlation between the usage of digital devices, especially smartphones, at night, and sperm quality (total motility, progressive motility, concentration) and a positive statistically significant correlation between the usage of these devices and immotile sperm. It was hypothesized that the pathological mechanism underlying this phenomenon was the alteration in melatonin secretion induced by the short wavelength light emitted from the screens of these electronic devices (55). These results were corroborated by several studies, that report an association between elevated melatonin levels and oligospermia or azoospermia in men (57, 58).
Liu et al. compared sperm parameters between rotating shift workers (RSW) and permanent shift workers (PSW). They recorded a significantly lower total sperm count in RSW, compared to PSW. RSW was associated with higher risk of low total sperm count, after adjusting for age, education level, average monthly household income, abstinence period, sampling time point, tobacco smoking, alcohol drinking and body mass index (50).
Liu et al. conducted the same analyses on a cohort of male undergraduates in order to identify semen quality differences associated with non-work-related CD between school days and days off. Total sperm count in men with less than 0.5 h of CD was significantly higher compared to men with more than 2 h of CD (50).
Hvidt et al. found that early bedtime (< 10:30 PM) was more often associated with normal semen quality compared with both regular (10:30 PM-11:29 PM) and late (≥11:30 PM) bedtime. Similarly, regular sleep duration (7.5–7.99 h) was more often associated with normal semen quality than both short (7.0–7.49 h) and very short (< 7.0 h) sleep duration (56).
Of note, Shi et al. found significant associations between sperm DNA fragmentation index and irregular sleep habits (51).
Despite previous evidence, other studies failed to find significant association between sleep duration and or quality and sperm parameters (48, 52).
Discussion
The relationship between disruption of CR and male infertility is supported by several pre-clinical and clinical lines of evidence. First, experimental manipulation or spontaneous mutations of clock genes, such as Bmal1 and Clock negatively interfere with fertility pathways in both pre-clinical models and humans. Second, alterations of CR due to altered sleep duration and/or impairment of sleep architecture may negatively interfere with circulating levels of reproductive hormones and semen parameters in humans. Unfortunately, the current level of evidence is still low, and findings are often controversial due to heterogeneity in terms of study design, study populations, and standardization of measurements. Therefore, further studies are needed to further elucidate the interactions between CR, CD, and male infertility. In details, the impact of the duration of CD, genetic predisposing factors, as well the reversibility of these alterations deserve further investigations. Based on available evidence, clinicians facing infertile males should discuss, in everyday clinical practice, the potential detrimental ole of reduced sleep duration, altered sleep architecture or exposure to artificial light at night on reproduction.
Author Contributions
Conception and design: FF, NL, and MCr. Acquisition of data: LS, LL, GF, and LC. Analysis and interpretation of data: MS, DA, and LN. Drafting of the manuscript: SM, GCa, CT, and GS. Critical revision of the manuscript for important intellectual content: MCr, GCe, MCa, RR, FF, FM, VM, and NL. Supervision: FF, NL, and MCr. All authors contributed to the article and approved the submitted version.
Conflict of Interest
The authors declare that the research was conducted in the absence of any commercial or financial relationships that could be construed as a potential conflict of interest.
Publisher’s Note
All claims expressed in this article are solely those of the authors and do not necessarily represent those of their affiliated organizations, or those of the publisher, the editors and the reviewers. Any product that may be evaluated in this article, or claim that may be made by its manufacturer, is not guaranteed or endorsed by the publisher.
References
1. Agarwal A, Saradha B, Neel P, Chak-Lam C, Ralf H, Sarah V, et al. Male Infertility. Lancet (London England) (2021) 397(10271):319–35. doi: 10.1016/S0140-6736(20)32667-2
2. Verze P, Arcaniolo D, Imbimbo C, Cai T, Venturino L, Spirito L, et al. General and Sex Profile of Women With Partner Affected by Premature Ejaculation: Results of a Large Observational, Non-Interventional, Cross-Sectional, Epidemiological Study (IPER-F). Andrology (2018) 6(5):714–19. doi: 10.1111/andr.12545
3. Arcaniolo D, Favilla V, Tiscione D, Pisano F, Bozzini G, Creta M, et al. Is There a Place for Nutritional Supplements in the Treatment of Idiopathic Male Infertility? Archivio Italiano Di Urologia Andrologia: Organo Ufficiale [Di] Societa Italiana Di Ecografia Urologica E Nefrologica (2014) 86(3):164–70. doi: 10.4081/aiua.2014.3.164
4. Verze P, La Rocca R, Spirito L, Califano G, Venturino L, Napolitano L, et al. Premature Ejaculation Patients and Their Partners: Arriving at a Clinical Profile for a Real Optimization of the Treatment. Archivio Italiano Di Urologia Andrologia: Organo Ufficiale [Di] Societa Italiana Di Ecografia Urologica E Nefrologica (2021) 93(1):42–7. doi: 10.4081/aiua.2021.1.42
5. Russart KLG, Nelson RJ. Light at Night as an Environmental Endocrine Disruptor. Physiol Behav (2018) 190(June):82–9. doi: 10.1016/j.physbeh.2017.08.029
6. Napolitano L, Barone B, Morra S, Celentano G, La Rocca R, Capece M, et al. Hypogonadism in Patients With Prader Willi Syndrome: A Narrative Review. Int J Mol Sci (2021) 22(4):1993. doi: 10.3390/ijms22041993
7. Li C, Zhang L, Ma T, Gao L, Yang L, Wu M, et al. Bisphenol A Attenuates Testosterone Production in Leydig Cells via the Inhibition of NR1D1 Signaling. Chemosphere (2021) 263(January):128020. doi: 10.1016/j.chemosphere.2020.128020
8. Zhao L, Zhang J, Yang L, Zhang H, Zhang Y, Gao D, et al. Glyphosate Exposure Attenuates Testosterone Synthesis via NR1D1 Inhibition of StAR Expression in Mouse Leydig Cells. Sci Total Environ (2021) 785(September):147323. doi: 10.1016/j.scitotenv.2021.147323
9. Balsalobre A, Damiola F, Schibler U. A Serum Shock Induces Circadian Gene Expression in Mammalian Tissue Culture Cells. Cell (1998) 93(6):929–37. doi: 10.1016/s0092-8674(00)81199-x
10. Rosbash M. Why the Rat-1 Fibroblast Should Replace the SCN as the in Vitro Model of Choice. Cell (1998) 93(6):917–19. doi: 10.1016/s0092-8674(00)81197-6
11. Peterlin A, Kunej T, Peterlin B. The Role of Circadian Rhythm in Male Reproduction:”. Curr Opin Endocrinol Diabetes Obes (2019) 26(6):313–16. doi: 10.1097/MED.0000000000000512
12. Weaver DR. The Suprachiasmatic Nucleus: A 25-Year Retrospective. J Biol Rhythms (1998) 13(2):100–12. doi: 10.1177/074873098128999952
13. Reppert SM, Weaver DR. Coordination of Circadian Timing in Mammals. Nature (2002) 418(6901):935–41. doi: 10.1038/nature00965
14. Gamble KL, Resuehr D, Hirschie Johnson C. Shift Work and Circadian Dysregulation of Reproduction. Front Endocrinol (2013) 4:92. doi: 10.3389/fendo.2013.00092
15. Huang W, Ramsey KM, Marcheva B, Bass J. Circadian Rhythms, Sleep, and Metabolism. J Clin Invest (2011) 121(6):2133–41. doi: 10.1172/JCI46043
16. Reid KJ. Assessment of Circadian Rhythms. Neurologic Clinics (2019) 37(3):505–26. doi: 10.1016/j.ncl.2019.05.001
17. Harrington ME. The Ventral Lateral Geniculate Nucleus and the Intergeniculate Leaflet: Interrelated Structures in the Visual and Circadian Systems. Neurosci Biobehav Rev (1997) 21(5):705–27. doi: 10.1016/s0149-7634(96)00019-x
18. Rüger M, Scheer FAJL. Effects of Circadian Disruption on the Cardiometabolic System. Rev Endocrine Metab Disord (2009) 10(4):245–60. doi: 10.1007/s11154-009-9122-8
19. Takahashi JS, Hong H-K, Ko CH, McDearmon EL. The Genetics of Mammalian Circadian Order and Disorder: Implications for Physiology and Disease. Nat Rev Genet (2008) 9(10):764–75. doi: 10.1038/nrg2430
20. Litlekalsoy J, Rostad K, Kalland K-H, J G. Hostmark, Didrik Laerum O. Expression of Circadian Clock Genes and Proteins in Urothelial Cancer Is Related to Cancer-Associated Genes. BMC Cancer (2016) 16(July):549. doi: 10.1186/s12885-016-2580-y
21. Morales-Santana S, Morell S, Leon J, Carazo-Gallego A, Jimenez-Lopez JC, Morell M. An Overview of the Polymorphisms of Circadian Genes Associated With Endocrine Cancer. Front Endocrinol (2019) 10:104. doi: 10.3389/fendo.2019.00104
22. Vetter C. Circadian Disruption: What Do We Actually Mean? Eur J Neurosci (2020) 51(1):531–50. doi: 10.1111/ejn.14255
23. Alterman T, Luckhaupt SE, Dahlhamer JM, Ward BW, Calvert GM. Prevalence Rates of Work Organization Characteristics Among Workers in the U.S.: Data From the 2010 National Health Interview Survey. Am J Ind Med (2013) 56(6):647–95. doi: 10.1002/ajim.22108
24. Centers for Disease Control and Prevention (CDC). Short Sleep Duration Among Workers–United States 2010. In: MMWR. Morbidity and Mortality Weekly Report vol. 61. Atlanta, GA, USA: Centers for Disease Control and Prevention (2012). p. 281–5.
25. Sack RL, Lewy AJ. Melatonin as a Chronobiotic: Treatment of Circadian Desynchrony in Night Workers and the Blind. J Biol Rhythms (1997) 12(6):595–603. doi: 10.1177/074873049701200615
26. Castro JM, Stoerzinger A, Barkmeier D, Ellen P. Medial Septal Lesions: Disruptions of Microregulatory Patterns and Circadian Rhythmicity in Rats. J Comp Physiol Psychol (1978) 92(1):71–84. doi: 10.1037/h0077443
27. Stevens RG, Rea MS. Light in the Built Environment: Potential Role of Circadian Disruption in Endocrine Disruption and Breast Cancer. Cancer Causes Control: CCC (2001) 12(3):279–87. doi: 10.1023/a:1011237000609
28. Foster RG, Peirson SN, Wulff K, Winnebeck E, Vetter C, Roenneberg T. Sleep and Circadian Rhythm Disruption in Social Jetlag and Mental Illness. Prog Mol Biol Trans Sci (2013) 119:325–46. doi: 10.1016/B978-0-12-396971-2.00011-7
29. Fritschi L, Groß JV, Wild U, Heyworth JS, Glass DC, Erren TC. Shift Work That Involves Circadian Disruption and Breast Cancer: A First Application of Chronobiological Theory and the Consequent Challenges. Occup Environ Med (2018) 75(3):231–4. doi: 10.1136/oemed-2017-104441
30. Scheer FAJL, Hilton MF, Mantzoros CS, Shea SA. Adverse Metabolic and Cardiovascular Consequences of Circadian Misalignment. Proc Natl Acad Sci USA (2009) 106(11):4453–8. doi: 10.1073/pnas.0808180106
31. Califano G, Xylinas E. Re: Phase II Trial of Neoadjuvant Systemic Chemotherapy Followed by Extirpative Surgery in Patients With High Grade Upper Tract Urothelial Carcinoma. Eur Urol (2020) 78(1):113–45. doi: 10.1016/j.eururo.2020.04.008
32. Califano G, Ouzaid I, Verze P, Stivalet N, Hermieu J-F, Xylinas E. New Immunotherapy Treatments in Non-Muscle Invasive Bladder Cancer. Archivos Espanoles Urologia (2020) 73(10):945–535.
33. Bittman EL. Timing in the Testis. J Biol Rhythms (2016) 31(1):12–36. doi: 10.1177/0748730415618297
34. Peruquetti RL, de Mateo S, Sassone-Corsi P. Circadian Proteins CLOCK and BMAL1 in the Chromatoid Body, a RNA Processing Granule of Male Germ Cells.” Edited by Laszlo Tora. PloS One (2012) 7(8):e426955. doi: 10.1371/journal.pone.0042695
35. Morse D, Cermakian N, Brancorsini S, Parvinen M, Sassone-Corsi P. No Circadian Rhythms in Testis: Period1 Expression Is Clock Independent and Developmentally Regulated in the Mouse. Mol Endocrinol (Baltimore Md) (2003) 17(1):141–51. doi: 10.1210/me.2002-0184
36. Alvarez JD, Hansen A, Ord T, Bebas P, Chappell PE, Giebultowicz JM. Carmen Williams, Stuart Moss, and Amita Sehgal. 2008. “The Circadian Clock Protein BMAL1 Is Necessary for Fertility and Proper Testosterone Production in Mice. J Biol Rhythms (2008) 23(1):26–36. doi: 10.1177/0748730407311254
37. Liang X, Cheng S, Jiang X, He X, Wang Y, Jiang Z, et al. The Noncircadian Function of the Circadian Clock Gene in the Regulation of Male Fertility. J Biol Rhythms (2013) 28(3):208–17. doi: 10.1177/0748730413486873
38. Cheng S, Liang X, Wang Y, Jiang Z, Liu Y, Hou W, et al. The Circadian Clock Gene Regulates Acrosin Activity of Sperm Through Serine Protease Inhibitor A3K. Exp Biol Med (2016) 241(2):205–15. doi: 10.1177/1535370215597199
39. Zhang J, Ding X, Li Y, Xia Y, Nie J, Yi C, et al. Association of CLOCK Gene Variants With Semen Quality in Idiopathic Infertile Han-Chinese Males. Reprod BioMed Online (2012) 25(5):536–42. doi: 10.1016/j.rbmo.2012.07.018
40. Shen O, Ding X, Nie J, Xia Y, Wang X, Tong J, et al. Variants of the CLOCK Gene Affect the Risk of Idiopathic Male Infertility in the Han-Chinese Population. Chronobiology Int (2015) 32(7):959–65. doi: 10.3109/07420528.2015.1056305
41. Arnal PJ, Drogou C, Sauvet F, Regnauld J, Dispersyn G, Faraut B, et al. Effect of Sleep Extension on the Subsequent Testosterone, Cortisol and Prolactin Responses to Total Sleep Deprivation and Recovery. J Neuroendocrinol (2016) 28(2):12346. doi: 10.1111/jne.12346
42. Zhang W, Piotrowska K, Chavoshan B, Wallace J, Liu PY. Sleep Duration Is Associated With Testis Size in Healthy Young Men. J Clin Sleep Medicine: JCSM: Off Publ Am Acad Sleep Med (2018) 14(10):1757–64. doi: 10.5664/jcsm.7390
43. Leproult R, Van Cauter E. Effect of 1 Week of Sleep Restriction on Testosterone Levels in Young Healthy Men. JAMA (2011) 305(21):2173–4. doi: 10.1001/jama.2011.710
44. Schmid SM, Hallschmid M, Jauch-Chara K, Lehnert H, Schultes B. Sleep Timing May Modulate the Effect of Sleep Loss on Testosterone. Clin Endocrinol (2012) 77(5):749–54. doi: 10.1111/j.1365-2265.2012.04419.x
45. Yoon I-Y, Kripke DF, Elliott JA, Youngstedt SD. Luteinizing Hormone Following Light Exposure in Healthy Young Men. Neurosci Lett (2003) 341(1):25–8. doi: 10.1016/s0304-3940(03)00122-8
46. Chen Q, Yang H, Zhou N, Sun L, Bao H, Tan L, et al. Inverse U-Shaped Association Between Sleep Duration and Semen Quality: Longitudinal Observational Study (MARHCS) in Chongqing, China. Sleep (2016) 39(1):79–86. doi: 10.5665/sleep.5322
47. Jensen TK, Andersson A-M, Skakkebaek NE, Joensen UN, Jensen MB, Lassen TH, et al. Association of Sleep Disturbances With Reduced Semen Quality: A Cross-Sectional Study Among 953 Healthy Young Danish Men. Am J Epidemiol (2013) 177(10):1027–37. doi: 10.1093/aje/kws420
48. Eisenberg ML, Chen Z, Ye A, Buck Louis GM. Relationship Between Physical Occupational Exposures and Health on Semen Quality: Data From the Longitudinal Investigation of Fertility and the Environment (LIFE) Study. Fertility Sterility (2015) 103(5):1271–77. doi: 10.1016/j.fertnstert.2015.02.010
49. Viganò P, Chiaffarino F, Bonzi V, Salonia A, Ricci E, Papaleo E, et al. Sleep Disturbances and Semen Quality in an Italian Cross Sectional Study. Basic Clin Androl (2017) 27:16. doi: 10.1186/s12610-017-0060-0
50. Liu K, Hou G, Wang X, Chen H, Shi F, Liu C, et al. Adverse Effects of Circadian Desynchrony on the Male Reproductive System: An Epidemiological and Experimental Study. Hum Reprod (2020) 35(7):1515–28. doi: 10.1093/humrep/deaa101
51. Shi X, Chan CPS, Waters T, Chi L, Yiu Leung Chan D, Li T-C. Lifestyle and Demographic Factors Associated With Human Semen Quality and Sperm Function. Syst Biol Reprod Med (2018) 64(5):358–67. doi: 10.1080/19396368.2018.1491074
52. Pokhrel G, Yihao S, Wangcheng W, Upadhyaya Khatiwada S, Zhongyang S, Jianqiao Y, et al. The Impact of Sociodemographic Characteristics, Lifestyle, Work Exposure and Medical History on Semen Parameters in Young Chinese Men: A Cross-Sectional Study. Andrologia (2019) 51(8):e133245. doi: 10.1111/and.13324
53. Chen H-G, Sun B, Chen Y-J, Chavarro JE, Hu S-H, Xiong C-L, et al. Sleep Duration and Quality in Relation to Semen Quality in Healthy Men Screened as Potential Sperm Donors. Environ Int (2020) 135(February):105368. doi: 10.1016/j.envint.2019.105368
54. Du C-Q, Yang Y-Y, Chen J, Feng L, Lin W-Q. Association Between Sleep Quality and Semen Parameters and Reproductive Hormones: A Cross-Sectional Study in Zhejiang, China. Nat Sci Sleep (2020) 12:11–8. doi: 10.2147/NSS.S235136
55. Green A, Barak S, Shine L, Kahane A, Dagan Y. Exposure by Males to Light Emitted From Media Devices at Night Is Linked With Decline of Sperm Quality and Correlated With Sleep Quality Measures. Chronobiology Int (2020) 37(3):414–24. doi: 10.1080/07420528.2020.1727918
56. Hvidt JEM, Knudsen UB, Zachariae R, Jakob Ingerslev H, Tholstrup Philipsen M, Frederiksen Y. Associations of Bedtime, Sleep Duration, and Sleep Quality With Semen Quality in Males Seeking Fertility Treatment: A Preliminary Study. Basic Clin Andrology (2020) 30:5. doi: 10.1186/s12610-020-00103-7
57. Sciarra F, Franceschini E, Campolo F, Gianfrilli D, Pallotti F, Paoli D, et al. Disruption of Circadian Rhythms: A Crucial Factor in the Etiology of Infertility. Int J Mol Sci (2020) 21(11):3943. doi: 10.3390/ijms21113943
Keywords: circadian desynchrony, circadian rhythms, clock genes, spermatogenesis, fertility
Citation: Fusco F, Longo N, De Sio M, Arcaniolo D, Celentano G, Capece M, La Rocca R, Mangiapia F, Califano G, Morra S, Turco C, Spena G, Spirito L, Fusco GM, Cirillo L, De Luca L, Napolitano L, Mirone V and Creta M (2021) Impact of Circadian Desynchrony on Spermatogenesis: A Mini Review. Front. Endocrinol. 12:800693. doi: 10.3389/fendo.2021.800693
Received: 23 October 2021; Accepted: 24 November 2021;
Published: 16 December 2021.
Edited by:
Qing Chen, Army Medical University, ChinaReviewed by:
Yongxin Ma, Sichuan University, ChinaMary Anna Venneri, Sapienza University of Rome, Italy
Copyright © 2021 Fusco, Longo, De Sio, Arcaniolo, Celentano, Capece, La Rocca, Mangiapia, Califano, Morra, Turco, Spena, Spirito, Fusco, Cirillo, De Luca, Napolitano, Mirone and Creta. This is an open-access article distributed under the terms of the Creative Commons Attribution License (CC BY). The use, distribution or reproduction in other forums is permitted, provided the original author(s) and the copyright owner(s) are credited and that the original publication in this journal is cited, in accordance with accepted academic practice. No use, distribution or reproduction is permitted which does not comply with these terms.
*Correspondence: Ferdinando Fusco, ZmVyZGluYW5kby1mdXNjb0BsaWJlcm8uaXQ=