- 1The First Clinical Medical College, Lanzhou University, Lanzhou, China
- 2The Second Clinical Medical College, Lanzhou University, Lanzhou, China
- 3Gansu Province Clinical Research Center for Endocrine Disease, Lanzhou, China
- 4Endocrine Disease Clinical Medical Research Center of Gansu Province, Lanzhou, China
Diabetic retinopathy (DR) is a common diabetic complication and the main cause of blindness worldwide, which seriously affects the quality of life of patients. Studies have shown that noncoding RNA (ncRNA) has distinct differentiated expression in DR and plays an important role in the occurrence and development of DR. ncRNAs represented by microRNAs (miRNAs), lncRNAs (lncRNAs), and circRNAs (circRNAs) have been shown to be widely involved in the regulation of gene expression and affect multiple biological processes of retinopathy. This article will review three RNAs related to the occurrence and development of DR on the basis of previous studies (especially their effects on retinal microangiopathy, retinal pigment epithelial cells, and retinal nerve cells) and discuss their underlying mechanisms and connections. Overall, this review will help us better understand the role of ncRNAs in the occurrence and development of DR and provide ideas for exploring potential therapeutic directions and targets.
Introduction
Diabetic retinopathy is one of the most common microvascular complications of type 1 and type 2 diabetes (Figure 1) and is the leading cause of blindness worldwide (1, 2). As the social environment changes, the impact of DR on human vision has become increasingly prominent. There is evidence that the current prevalence of DR in the United States is approximately 35% (3, 4). In the past 30 years, the prevalence of diabetic retinopathy has increased by 7.7%, but the crude prevalence of other blinding diseases has decreased (5). As a complication of a chronic disease, the prevalence and severity of DR are positively correlated with age and the course of the disease (6). The relatively long course of DR can be divided into mild DR or nonproliferative DR (NPDR), characterized by an increase in the number of microaneurysms, and proliferative DR (PDR), characterized by the formation of new blood vessels on the posterior surface of the retina and vitreous, which is more severe than NPDR. Increased vascular permeability, macular edema, retinal distortion and detachment caused by angiogenesis and fibrous tissue contraction and neovascular bleeding are all progressive pathological changes in the development of DR under continuous hyperglycemia stimulation (7). Without treatment, most patients with DR may lose vision within 5-10 years of diagnosis. Currently, there is no optimal clinical treatment method. Vascular endothelial growth factor (VEGF) is considered a promising target, and research has found that VEGF may influence inflammation in DR (8). VEGF can only achieve good curative effects in the late stage of DR, however, and VEGF administration is cumbersome, which does not lead to an ideal treatment method. Therefore, there are broad prospects for the discovery of new therapeutic target molecules and research over therapeutic applications.
A number of studies have confirmed that the pathological process of diabetic retinopathy is related to the differential expression of vascular dysfunction-related proteins, such as VEGF, transforming growth factor (TGF), and sirtuin (SIRT), and their expression levels are closely related to oxidative stress, apoptosis and angiogenesis in the course of DR (9–12). miRNAs, lncRNAs, and circRNAs can be used as upstream regulators or interacting elements to participate in their functioning process. Previous studies have found that 131 circRNAs (13), 8miRNAs (14), and 427 lncRNAs with expression differences during the development of DR compared with normal tissues (15), which suggests that the three RNAs molecules are closely related to the occurrence and development of DR. This review reveals the influence of miRNAs, circRNAs, and lncRNAs on the development of DR, which may increase our understanding of the role of these important molecules and provide new perspectives for clinical DR molecular targeted therapy.
miRNA
miRNAs are single-stranded ncRNAs consisting of 20-24 nucleotides. In the classical pathway, miRNA is a primary miRNA transcript formed by RNA polymerase II transcription of precursor miRNA genes. After the stem-loop structure is formed, it is cleaved into precursor miRNA and proceeds to mature miRNA by the Disher enzyme (16–18). miRNA can interact with the 3’-untranslated region (UTR) of targeted mRNA through its 6 nt seed sequence, mediate posttranscriptional gene silencing (PTGS) in the cytoplasm (19), and participate in almost all cellular activity regulation, including cell proliferation, migration, differentiation and apoptosis (20, 21). An increasing number of studies have demonstrated that miRNAs play an indispensable role in the development of DR lesions.
CircRNA
CircRNA was first discovered by Hsu in 1979 to be expressed in the cytoplasm of mammals (22). CircRNAs are a new type of ncRNA generated from linear precursor mRNAs via nonclassical splicing (23). Because its closed loop structure is difficult to degrade by nucleases, circRNA is more stable than its linear transcript (24). The composition of circRNA can be divided into exon circRNA (ecircRNAs), intron circRNA (ciRNAs), and exon and intron circRNA (elciRNAs) (25). It has been confirmed that circRNAs mainly regulate the physiological and pathological functions of cells in four ways: serving as miRNA sponges, protein regulators, translation templates and gene expression regulators (23).
LncRNA
LncRNAs are noncoding protein transcripts composed of more than 200 nucleotides. Studies have shown that a small number of lncRNAs have the potential to encode proteins (26), but this is not the main role of lncRNA in the regulation of cell life activities. LncRNAs mainly regulate gene expression at the pretranscription, posttranscription and posttranscriptional levels, such as epigenetic regulation (group protein methylation, and chromatin remodeling), regulatory transcription factors, endogenous competitive RNA, and antisense lncRNAs (27–29). In many diseases, lncRNAs are differentially expressed, and DR is no exception. Gene therapy based on lncRNAs is an emerging disease treatment strategy and has great potential in the treatment of DR.
Three Types of RNA Influence the Development of DR Through Microvascular Endothelial Cells
The retinal vessel wall is composed of three layers: intima, media, and adventitia. The innermost intima consists of a single layer of endothelial cells in direct contact with the vascular cavity, and the media is composed of multiple layers of smooth muscle cells and pericytes. The blood basement membrane forms an outer membrane on the outside (30, 31). Retinal vascular endothelial cells (HRVECs) in the HG state will activate, proliferate and migrate abnormally, which may lead to changes in retinal vascular function (32). Increasing evidence shows that three types of RNAs have important regulatory effects on the process of HG-stimulating dysfunction of retinal vascular endothelial cells (Figure 2).
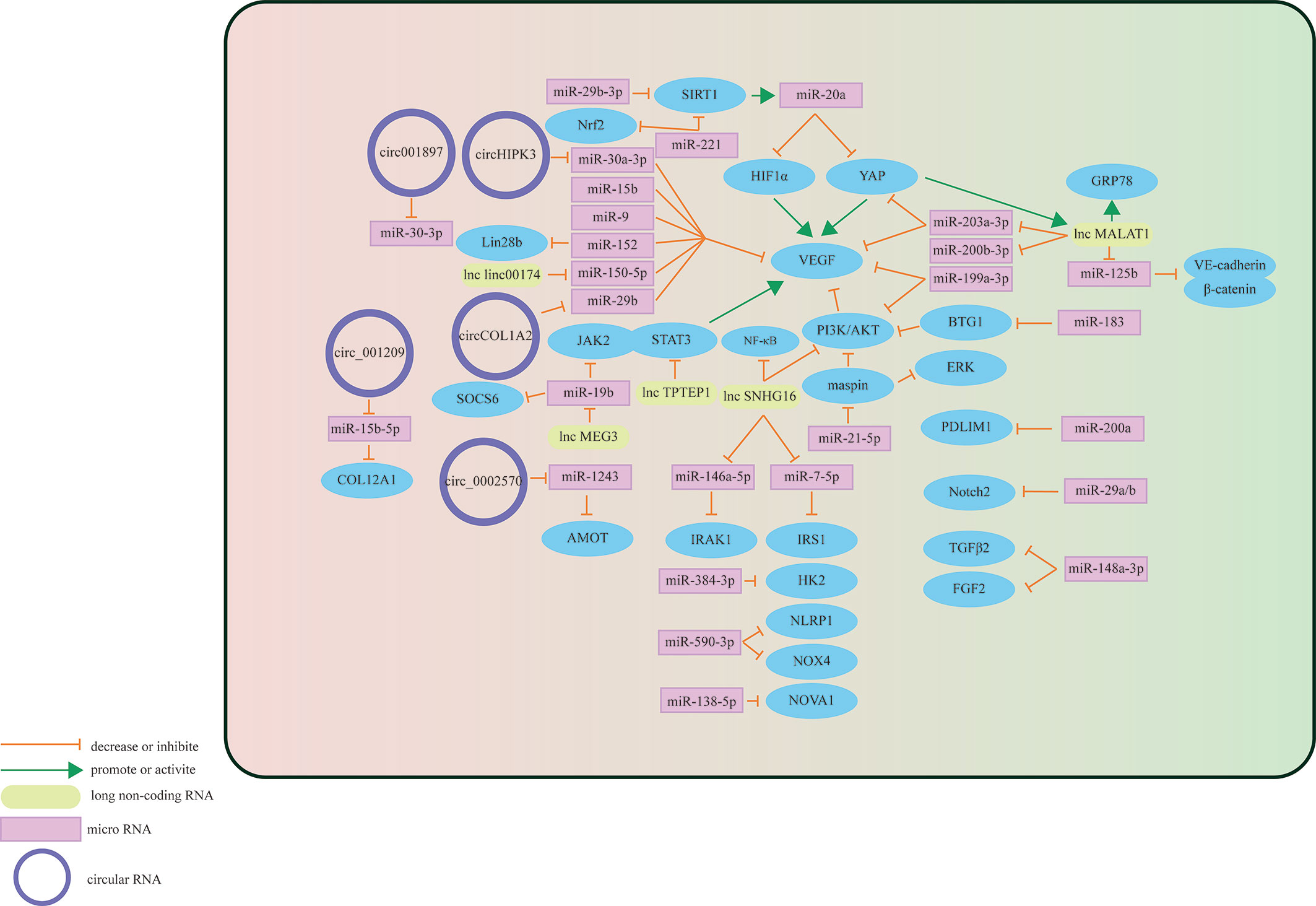
Figure 2 Schematic overview of miRNAs, lncRNAs, and circRNAs affecting vascular endothelial cells and contributing to the pathogenesis of DR.
miRNA-Mediated Mechanism
Some miRNAs may target specific molecules to protect retinal microvascular endothelial cells (RMECs) in DR. The most important target is VEGF. For example, miR-29b-3p can negatively regulate the expression levels of VEGFA and platelet-derived growth Factor B (PDGFB), inhibiting cell cycle progression by decreasing the expression levels of cell cycle-related proteins (cyclins A2, D1, and E1), which prevents the abnormal proliferation of RMECs (33). Previous studies have also found that miR-29b-3p can downregulate SIRT1 to promote RMEC apoptosis (34). However, Pan et al. found that SIRT1 can positively regulate the expression of miR-20a so that miR-20a induces YAP/HIF1α to downregulate VEGF activity and hinder the abnormal proliferation of RMECs in the HG state, suggesting that the restoration of SIRTI may protect RMECs in the HG state (35). MiR-199a-3p might be another target for regulating VEGF. It can downregulate the expression of VEGF and inhibit the PI3K/AKT pathway related to VEGF, protecting RMECs from HG (36). miR-203a-3p is downregulated under the induction of HG, and high expression can decrease the expression levels of VEGFA and HIF-1α to reduce the pathological retinal angiogenesis of PDR (37). Previous studies have shown that miR-15b (38), miR-9 (39), and miR-152 (40) can directly or indirectly downregulate VEGF in RMECs treated with HG, reduce the proliferation of endothelial cells, and hinder the progression of PDR. In addition to VEGF, many miRNAs protect RMECs in the DR state through other signaling pathways. PDLIM1 is a cytoskeletal protein associated with stress fibers, and miR-200a can downregulate the expression of PDLIM1 in RMECs and reduce the apoptotic state of RMECs, decreasing retinal microvascular leakage (41), which implies that miR-200a may be an efficient therapeutic target for the early stage of DR. miR-148a-3p specifically binds to the 3’ untranslated regions of TGFB2 and FGF2, downregulates their expression, and reduces the apoptosis of microvascular epithelial cells in the HG state (42). OPN regulates the inflammatory response at multiple levels (43). In the capillary endothelial cells of DR, it can inhibit miR-29a, indirectly increase the synthesis of type IV collagen, thicken the basement membrane, and aggravate the pathological changes of DR (44). Research in recent years found that miR-29a/b (45), miR-590-3p (46), miR-138-5p (47), and miR-384-3p (48) all have the ability to protect microvascular endothelial cells in DR.
In addition to the protective effect, some miRNAs regulate downstream signals to make RMECs proliferate, promote the formation of new blood vessels, and aggravate the PDR process. HG upregulates miR-183, reduces the expression of BTG1, and activates VEGF-related pathways to upregulate VEGF expression and promote the proliferation of diabetic retinal vascular endothelial cells (49). miR-21-5p is the upstream signal of maspin. After high expression, it enhances the cellular viability of RMECs and promotes the angiogenesis of PDR (50). Some miRNAs promote RMEC apoptosis and aggravate the progression of PDR in the HG state. Overexpressed miR-221 can bind to the 3’UTR of SIRT1 mRNA and downregulate its expression, blocking the Nrf2 pathway and increasing the apoptosis of RMECs (51).
In summary, miRNAs have broad potential in DR treatment, but the therapeutic effect may be difficult to grasp. For example, miR-148a-3p, which is downregulated in RMECs under DR conditions, can hinder the formation of new blood vessels after upregulation, but it will also damage the blood-retinal barrier (42).
CircRNA-Mediated Mechanism
The differential expression of circRNAs in HRVECs has a significant impact on the progression of DR, which may cause vascular dysfunction and/or promote the formation of new blood vessels and participate in nonproliferative and proliferative DR lesions. Overexpressed circ_001209 serves as a miR-15b-5p sponge in HRVECs, indirectly regulating the expression of COL12A1, causing vascular endothelial cell dysfunction, significantly thinner retinal thickness, and increased apoptosis (52). Similarly, the expression of circHIPK3 located in the cytoplasm of HRVECs increased, downregulating the activity of miR-30a-3p and thereby upregulating the expression of VEGFC, FZD4 and WNT2. Knockout of circHIPK3 can reduce the abnormal proliferation, migration and tubular formation of HRVECs cultured in vitro, decreasing retinal acellular capillaries and vascular leakage (53). Therefore, circHIPK3 may play a role in the progression of NPDR. As a sponge of miR-29b, circCOL1A2 promotes the proliferation of retinal microvascular endothelial cells (HRMECs) and inhibits cell apoptosis under the action of VEGF (54). Tissue-specific expression of angiomotin can promote the proliferation of vascular endothelial cells and make them tubular. hsa_circ_0002570 is an inhibitor of miR-1243, which upregulates the expression of angiomotin and promotes the formation of new blood vessels in DR (55). circ001897 is circRNA whose expression level in DR tissues is significantly higher than that in the control group. By targeting miR-30-3p and downregulating its expression level, it can achieve the effect of HRMEC migration and proliferation (56). However, the downstream targeting molecule of miR-30-3p has not yet been determined.
In addition to vascular endothelial cells, circRNAs also play an important regulatory role in pericytes. The histopathological feature in the earliest stage of DR is the loss of pericytes (57). Under the stimulation of diabetes, CZNF532 acts as a sponge of miR-29a-3p to induce increased expression of NG2, LOXL2 and CDK2 to protect retinal pericytes and alleviate pericyte degeneration and vascular dysfunction (58). Therefore, cZNF532 is essential for maintaining pericyte function and vascular homeostasis. Similarly, cPWWP2A in the cytoplasm of pericytes acts as an endogenous miR-579 sponge to inhibit miR-579 activity, which increases the expression of angiopoietin 1, occludin, and SIRT1 (59). Intervening in the expression of cPWWP2A or miR579 may be a treatment strategy for diabetic microvascular complications. A major cause of vascular dysfunction is abnormalities in pericyte-endothelial cell crosstalk (60). There is evidence that circRNAs may be involved in this pathway. CircEhmt1, which is transferred from pericytes to endothelial cells through exosomes, is highly expressed in the nucleus of pericytes and upregulates the level of transcription factor (NFIA), which ultimately inhibits the production of the NLRP3 inflammasome. Activation of the HIF pathway may play an important role in the activation of the NFIA/NLRP3 pathway.
In summary, circRNAs can regulate the functional changes of endothelial cells and pericytes in the DR state through different pathways (Table 1), possibly due to the different roles that endothelial cells and pericytes play in neovascularization. DR damage is the main pathway by which circRNAs regulate endothelial cells, while DR protection is the main counterpart in pericytes. The development of circRNA-targeted drugs is helpful for the derivation of new strategies for the clinical treatment of DR.
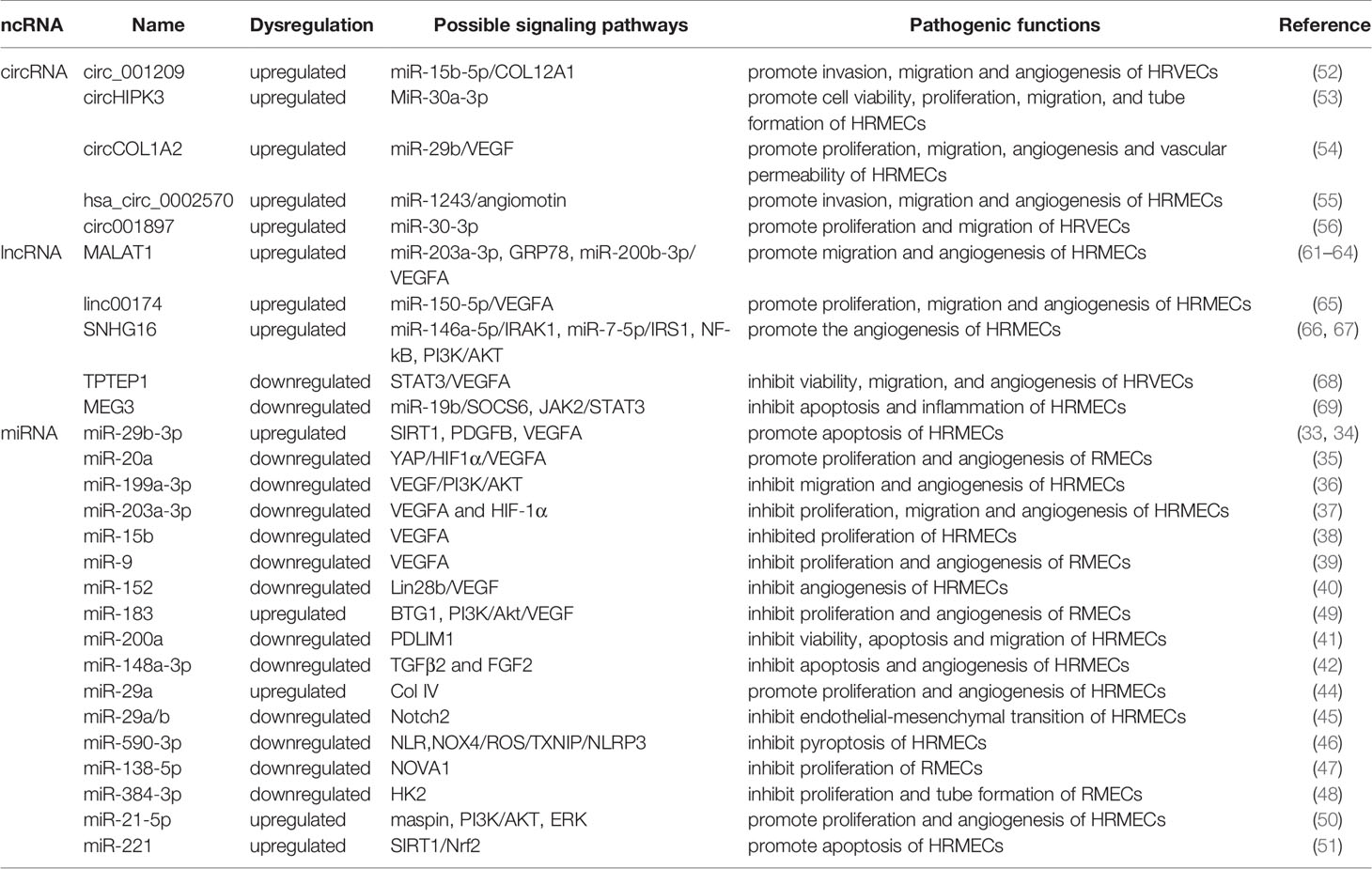
Table 1 The role of miRNA, lncRNA and circRNA in DR and their mechanisms through vascular endothelial cell and targets.
LncRNA-Mediated Mechanism
Existing studies have shown that adjusting the expression of specific target mRNAs or miRNAs enables lncRNAs to regulate endothelial cell diseases. In 2014, studies found that lncRNA MALAT1 may cause damage to diabetic endothelial cells (70). Recently, the damage mechanism of MALAT1 in DR vascular endothelial cells was further explored, and it was discovered that it may promote the development of DR by mediating the activation and inhibition of different pathways. Highly expressed MALAT1 is involved in HG-induced angiogenesis, ERS and inflammation, which may be achieved by MALAT1’s inhibition of the expression of GRP78 (61). Other studies have found that highly expressed YAP1 may upregulate the expression of MALAT1 to promote the proliferation of endothelial cells, which may be the effect of downregulating miR-200b-3p and increasing the expression of VEGF simultaneously (62). miR-125b has also been proven to be a downstream targeting molecule of MALAT1. The decreased expression of miR-125b can upregulate the expression of the VE-cadherin/MALAT1-catenin complex and neovascularization-related proteins to participate in the process of DR (63). miR-203a-3p is also a downstream target molecule of MALAT1 that has been explored in HRMECs under HG treatment (64), but the downstream signal of miRNA needs to be further studied. There are many other lncRNAs involved in the pathological process of HRMEC. Upregulated Linc00174 can target miR-150-5p, upregulate VEGF, and promote the angiogenesis of DR (65). Similarly, SNHG16 is also upregulated in HG-induced vascular endothelial cells and activates the NF-kB pathway through miR-146a-5p/IRAK1 and miR-7-5p/IRS1, with the NF-kB pathway triggered by the PI3K/AKT pathway, promoting hRMEC dysfunction (66). In addition to promoting HRMEC lesions induced by HG, some lncRNAs have a protective effect on HRMECs. As an upstream regulator of miR-195, after upregulating SNHG16, H2O2-induced tube formation of HMRECs was significantly inhibited (67). Likewise, TPTEP1 can inhibit the phosphorylation and nuclear translocation of STAT3, thereby downregulating VEGFA mRNA and protein levels and hindering the formation of new blood vessels under HG conditions (68). MEG3 negatively regulates miR-19b to inhibit HG-induced inflammatory factors generated from HMRECs, caspase-3/7 and cell apoptosis (69). Therefore, reversing the expression level of lncRNAs under certain conditions may play a role in promoting the alleviation of DR.
Three Types of RNA Influence the Development of DR Through Retinal Pigment Epithelial Cells
The retinal pigment epithelium (RPE) is a lifelong layer of highly polarized pigment epithelial cells located between the photoreceptors and the choroid (71). The RPE participates in the formation of the external visual blood barrier, and the melanin particles in RPE can absorb light to protect it from lesions due to photooxidative stress (72). In addition to phagocytosis, degradation of the photoreceptor outer ganglion (POS) terminal, maintenance of the retinoic acid cycle, and protection against light and oxidative stress, RPE can also secrete proteins in a polarized manner; for example, vascular endothelial growth factor (VEGF) is secreted mainly toward the basal direction, which is essential for the growth of choroidal blood vessels (73) and is also an important factor in promoting the progression of DR in the proliferative phase. An increasing number of studies have found that miRNAs, lncRNAs, and circRNAs are differentially expressed in RPE induced by HG and have certain effects on its pathological changes (Figure 3) (Table 2). Therefore, understanding the role of these three RNAs in RPE lesions may help us to understand the development of DR.
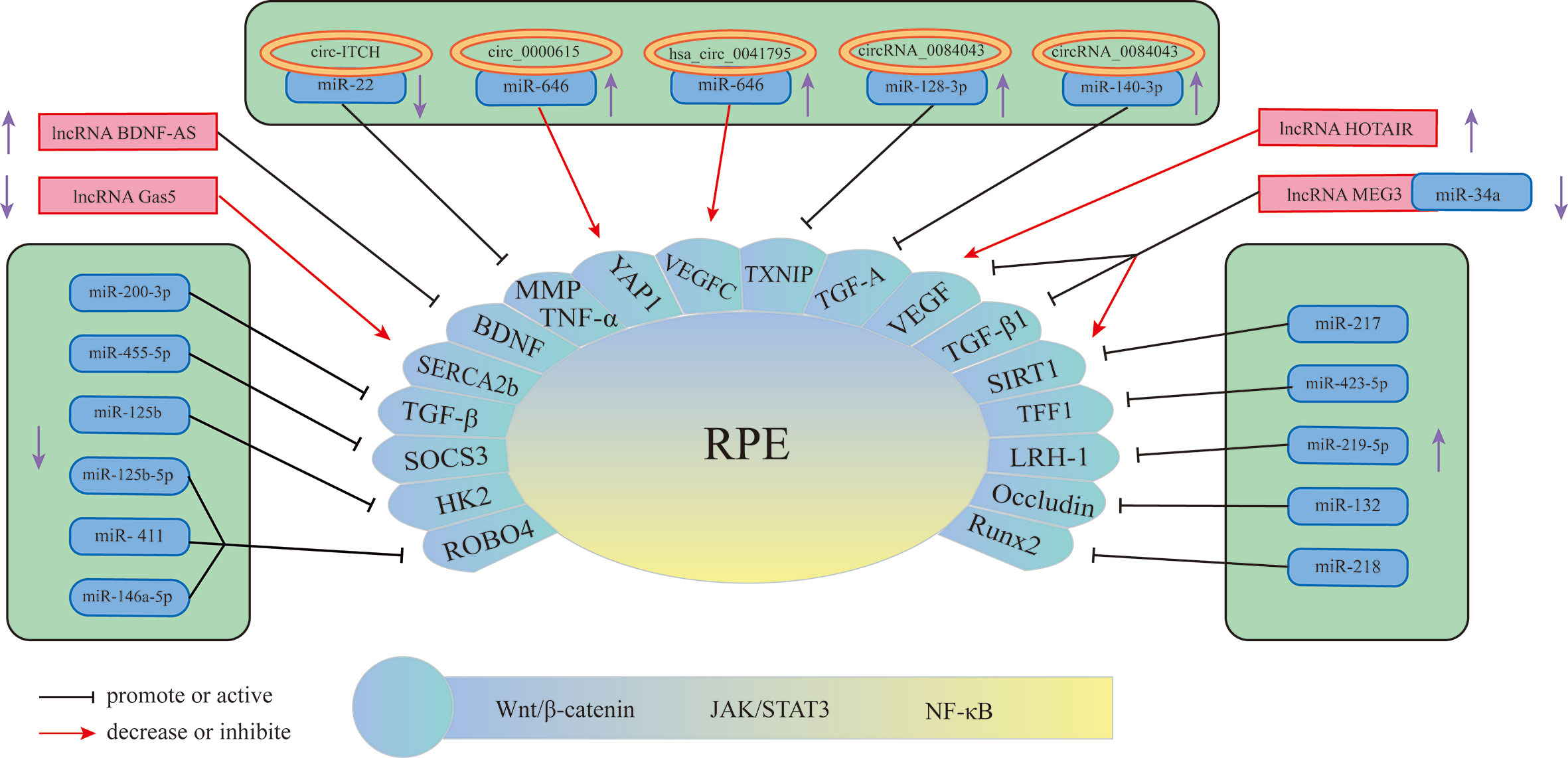
Figure 3 Schematic overview of miRNAs, lncRNAs, and circRNAs affecting RPE and contributing to the pathogenesis of DR.
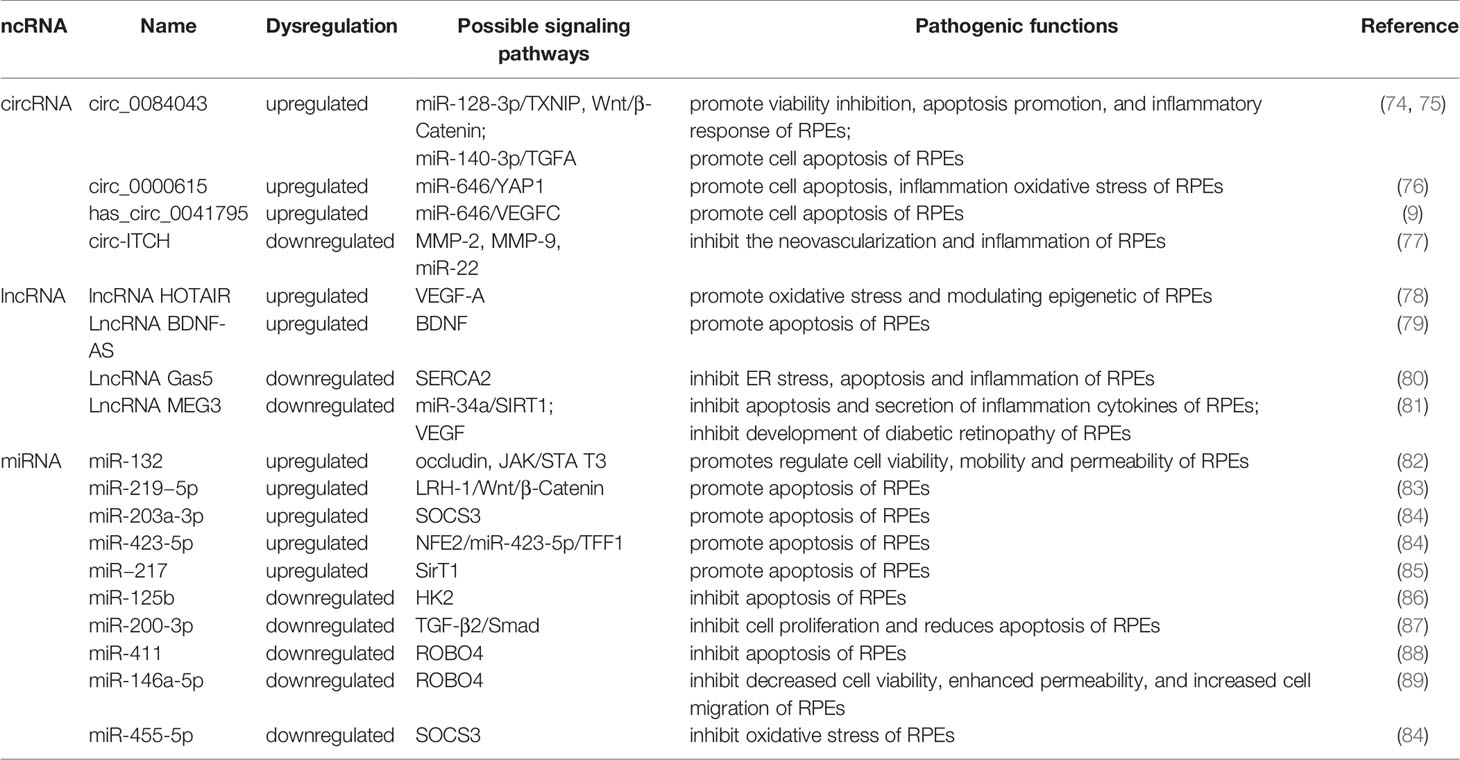
Table 2 The role of miRNA, lncRNA and circRNA in DR and their mechanisms through retinal pigment epithelial cell and targets.
miRNA-Mediated Mechanism
Currently, miRNAs have been reported to play a key role in regulating RPE apoptosis, proliferation, and migration. Abnormally expressed miRNAs promote pathological changes in the retinal pigment epithelium by activating different signaling pathways. Some miRNAs play a role in promoting RPE damage. For example, miR-132 is highly expressed in the HG environment and targets occludin to activate the JAK/STAT3 pathway, which increases the mobility and permeability of RPE and decreases its survival rate (82). Suppressors of cytokine signaling (SOCS3) are involved in the endoplasmic reticulum stress pathway of tumor cells (90). Under hypoxic conditions induced by high glucose, Mi-203a-3p can perform targeted inhibition of the expression of SOCS3 and promote RPE apoptosis (91). As a target of miR-218, the transcription factor Runx2 is inhibited, promoting RPE apoptosis in the HG state (92). The transcription factor NFE2 can directly upregulate the activity of miR-423-5p, regulate the expression of TFF1 in RPE cells in the HG environment, and activate the NF-κB pathway, aggravating RPE damage (85). This result suggests that the NFE2/miR-423-5p/TFF1 axis plays a role in HG-induced apoptosis in RPE. Similarly, miR-217 can downregulate the expression of SIRT1, aggravate the inflammatory response of RPE and activate the NF-κB signaling pathway (93). Zhao et al. found that inhibiting the expression of miR-219-5p can significantly increase the activity of RPE. The damaging effect of miR-219-5p on RPE may be caused by the activation of Wnt/β-catenin by LRH-1 (83), while LRH-1 plays a key role in the regulation of apoptosis (94).
In addition to the abovementioned miRNAs that promote RPE damage, the expression of some miRNAs will also protect RPE in the DR state. HG induction downregulates miR-200-3p in RPE, and further research proves that miR-200-3p can reduce the inflammatory effect by inhibiting the TGF-β2/Smad signaling pathway, which provides a new target for DR treatment in the clinic (87). miR-411 plays a protective role in HG or RPE in a hypoxic state. It can downregulate the expression of ROBO4 and reduce the permeability of RPE, thereby improving the survival rate of RPE (88). Another study showed that ROBO4 in RPE can be inhibited by miR-125b-5p in the HG state, while ROBO4 in RPE can be inhibited by miR-146a-5p in the hypoxic state (89). It is suggested that different stresses in the HG state will lead to the activation of different signaling pathways in RPE, and miR-411, miR-125b-5p, and miR-146a-5p may all target ROBO4 to protect RPE. A previous article showed that miR-203a-3p targeting SOCS3 damages PRE, but another study found that SOCS3 is also the target of miR-455-5p, and overexpression of miR-455-5p can downregulate SOCS3 to protect RPE (84). Under HG conditions, the RPE glycolytic pathway is in a disordered state, and miR-125b can target hexokinase 2 (HK2), reducing the glycolytic activity of RPE to delay the progression of DR (86).
CircRNA-Mediated Mechanism
CircRNAs play an important role in the process of RPE lesions by virtue of their biological properties. CircRNAs can regulate the function and state of cells through a variety of biological functions, such as interacting with proteins or serving as translation templates. However, current evidence has shown that amid the regulation of RPE pathological changes, circRNAs are mainly used as miRNA sponges to regulate the expression level of downstream proteins, thereby utilizing the regulatory network in the process. circ-ITCH was found to be circRNA that was abnormally downregulated in RPE in DR rats (77). circ-ITCH can reduce the expression of miR-22 through sponging miR-22, inhibiting important regulators of angiogenesis, such as matrix metalloproteinases-2 (MMP-2) and MMP-9, and the expression of tumor necrosis factor α (TNF-α) together with other inflammatory factors, alleviating the pathological trend of DR. Qiang et al. (76) discovered a stable circRNA with increased expression under the induction of HG—circ_0000615 that has a miR-646 binding site, which can downregulate the expression level of miR-646 in a targeted manner. After knocking out the circ_0000615 gene, Bcl-2 increased, the activity of Bax and C-caspase3 decreased, the viability of HG-induced RPE cells was restored, the inflammatory response was weakened, and the expression of proinflammatory cytokines decreased (TNF-α, IL-1β, and IL-6). YAP1 is a key effector of the Hippo pathway, and it plays an important role in regulating cell survival (95). Studies have found that YAP1 is a downstream targeted regulatory protein of miR-646. Upregulation of miR-646 can antagonize the inhibition of YAP1 expression after overexpression of circ_0000615, promoting the repair of endothelial cells. Another study on circRNA (9) found that miR-646 has different signal transduction pathways in HG-treated RPE. In this study, miR-646 could be sponged by hsa_circ_0041795 to downregulate the expression, and miR-646 could interact with VEGFC to downregulate the expression of VEGFC. In HG-induced RPE, the overexpression of hsa_circ_0041795 increased VEGFC by downregulating miR-646, which ultimately promoted RPE apoptosis, accelerating the progression of DR. Different circRNAs can regulate the pathological process of RPE through common miRNA, and the same circRNA can also play a role in DR through different miRNAs. Li et al. found (74) that the activity of circRNA_0084043 was significantly upregulated under HG induction and promoted ARPE-19 cell proliferation, HG-induced apoptosis, oxidative stress and inflammatory reactions. Further experiments proved that circRNA_0084043 can target miR-140-3p, which indirectly regulates the expression level of transforming growth factor-A (TGF-A). In the study of Zhang et al. (75), after RPE was treated with HG, the activity of circ_0084043 was upregulated, and the expression of miR-128-3p was inhibited by sponging. TXNIP proved to be a downstream regulator of miR-128-3p. In addition, circRNA_0084043 regulates the Wnt/β-catenin signaling pathway through the miR-128-3p/TXNIP axis. The Wnt/b-catenin signaling pathway is related to the promotion of inflammation and vascular exudation and is involved in the progression of DR (96).
LncRNA-Mediated Mechanism
Many lncRNAs have been shown to aggravate RPE damage in the HG environment, suggesting that they play an important role in the development of DR. VEGF is a key factor in the formation of new blood vessels during the development of DR, plays an important role in diabetic macular edema and is currently one of the targets of DR treatment (97). Some lncRNAs in RPE can act on VEGF in different ways to regulate the progression of DR. Research (78) discovered that under HG conditions, the expression of lncRNA HOTAIR in the cytoplasm and nucleus of RPE increased. After inhibiting its expression, the expression of a variety of transcripts related to angiogenesis (VEGF-A, ET-1, ANGPTL4, PGF, HIF-1α) and epigenetic regulation (EZH2, SUZ12, DNMT1, DNMT3A, DNMT3B, CTCF, P300) was downregulated, which improved cell survival. In addition, HOTAIR can dynamically regulate the levels of RNA polymerase II and acetylation factor (P300) in the distal and proximal promoter regions of its downstream target VEGF-A by interacting with histone-modifying enzymes, which promotes the epigenetic activation of VEGF-A, leading to the overexpression of VEGF-A and the formation of new blood vessels. Moreover, HOTAIR is also related to vascular permeability in DR, suggesting that lncRNAs may function in the nonproliferative stage. Another study showed that there was a decrease in the expression level of lncRNA MEG3 in the serum of DR patients, and the serum VEGF level was significantly higher than the normal level. Cell experiments have found that the overexpression of MEG3 has a negative regulatory effect on the expression level of VEGF, but the specific form of VEGF regulation has not been clearly studied (81). SERCA is a key molecule for the cytoplasm and endoplasmic reticulum to carry out Ca2+ transfer and maintain Ca2+ homeostasis (98), and it can regulate Ca2+-related signaling pathways. The lncRNA Gas5/SERCA axis was proven to regulate endoplasmic reticulum stress, the inflammatory response, and apoptosis of RPE cells treated with HG, and both Gas5 and SERCA were expressed at low levels in RPE cells treated with HG (80), suggesting that these signaling pathways may act as a protective factor for RPE to inhibit the progression of DR. Brain-derived neurotrophic factor (BDNF) is a type of nerve growth factor that is abundantly expressed in the brain and peripheral system and plays a role in promoting DR (79, 99). LncRNA BDNF-AS is highly expressed in HG-induced RPE and acts on BDNF mRNA to inhibit BDNF expression and promote cell apoptosis (79). In summary, these lncRNAs that play a role in RPE lesions induced by HG may have potential therapeutic effects. Reversing its expression level may delay the damage process of RPE.
miRNAs and lncRNAs Affect the Development of DR Through Retinal Nerve Cells
The retinal tissue is composed of blood vessel tissue, neurons, and supporting glial cells. These cells interact to form a structure called the neurovascular unit (NVU) (100). DR has two interrelated components: diabetic retinal neurodegeneration (DRN) and diabetic retinal vascular disease (DRV) (101). Nerve cell apoptosis of ganglion, amacrine and Müller cells and activation of microglia occur in DRN (102). Diabetic patients with early DR, or even before the occurrence of DR, have neurodegeneration such as thinning of the ganglion cell layer (GCL) and retinal nerve fiber layer (RNFL) (103, 104). Previous studies have shown that miRNAs, lncRNAs, and circRNAs can affect the progression of neuropathy in the process of DR (Figure 4). We summarized these related research results to provide new ideas for the study and treatment of DR from the perspective of neuropathy.
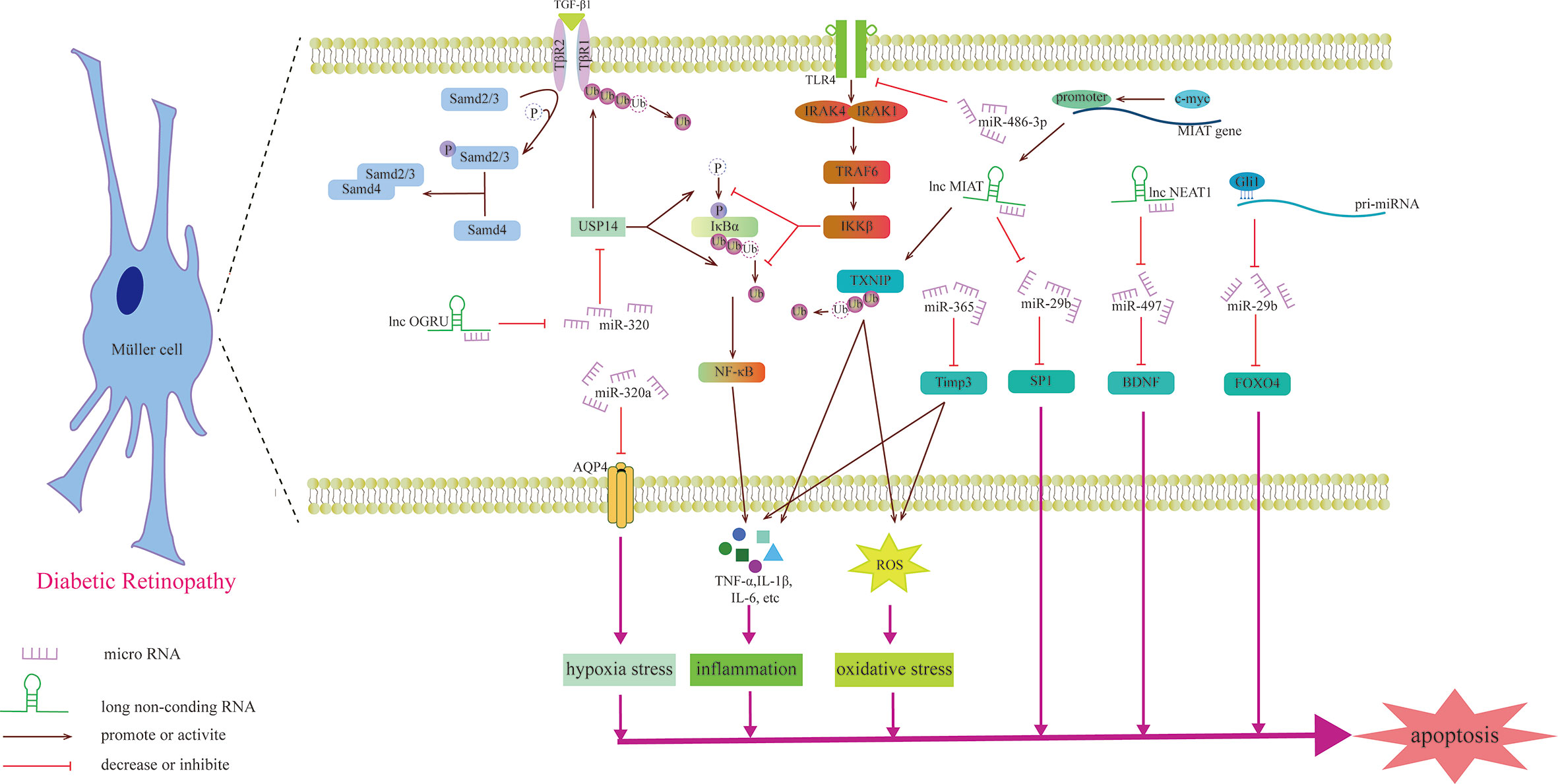
Figure 4 Schematic diagram of the mechanism by which miRNAs and lncRNAs affect Müller cells and participate in the development of DR.
Previous studies have shown that miRNAs may be involved in the degeneration of Müller cells caused by DR. For example, upregulating the expression of miR-486-3P can protect Müller cells from oxidative stress, inflammation and apoptosis in the HG state and inhibit the TLR4/NF-κB axis (105). Aquaporin-4 (AQP4) can promote the diffusion of water in the cell membrane, and miR-320a can promote the internalization of AQP4, alleviating the edema of Müller cells under hypoxic stress, and may be a potential therapeutic target (106). miR-29a/bs, which are affected by Gli1 and downregulated in the HG state, all target FOXO4 and bind to FOXO4 mRNA to negatively regulate its expression, aggravating the damage to retinal Müller cells (107). The gliosis of Müller cells is positively correlated with the expression of miR-365. In the HG state, miR-365 can downregulate the expression of TIMP3 to promote retinal oxidative stress and gliosis and aggravate DR disease (108). Zhang et al. explored the role of miRNA in retinal ganglion cells and found that miR-495 is involved in ganglion cell apoptosis in the HG state. From a mechanistic point of view, it may be that miR-495 affects the transmission of PTEN/Akt signaling by targeting Notch1, aggravating the damage to ganglion cells (109). S100A12 is a member of the calcium binding protein family. The level of plasma S100A12 relates to the presence of DR, which can activate the inflammatory response of immune cells (110). S100A12 can inhibit the expression of miR-30a, and miR-30a activates retinal microglia in an NLRP3-dependent manner and promotes the progression of DR (111).
Recently, the role of lncRNAs in Müller cells was studied (Table 3). OGRU is a type of lncRNA that is highly expressed in Müller cells in the DR state and aggravates the oxidative stress of Müller cells. Exploration of this mechanism has found that OGRU can regulate the expression of USP14 by downregulating miR-320. USP14 directly prevents the ubiquitination and degradation of transforming growth factor-β1 receptors (113). c-myc is the factor that regulates inflammatory mediators (115). In Müller cells, the promoter of lncRNA MIAT can be activated by c-myc. The overexpression of TXNIP caused by upregulated MIAT further activates inflammatory factors (IL-1β, TNF-α and IL-6), aggravating Müller cell damage induced by HG (112). However, another study found that MIAT has a highly homologous target sequence with miR-29b, which can negatively regulate the level of miR-29b and regulate Müller cell apoptosis in DR through the miR-29b/Sp1 axis (116). In addition to the damaging effect, lncRNAs also play a protective role in the nerve cells of DR. For example, upregulating the expression of NEAT1 in Müller cells in the HG state can downregulate miR-497 and increase the expression of BDNF, hindering the damaging effect of HG on Müller cells (114). Some lncRNAs can directly change the morphology of photoreceptors, such as MALAT1, which is upregulated through DR induction, which makes cones appear sparsely arranged and assumes the form of relatively short outer segments. This specific signaling pathway, however, has not been explored (117).
The abnormal expression of circRNA has been shown to be an intermediate phase in a variety of signaling pathways involved in the process of diabetic peripheral neuropathy, especially peripheral nerve pain (118). For example, knocking out circHIPK3 can restore the expression of miR-124, inhibit neuroinflammation and alleviate neuralgia in diabetic rats (119). However, the role of circRNA in nerve cell damage caused by DR has not been verified, and it may be a new research direction.
Conclusion
DR is a complication of diabetes caused by multiple risk factors, and its pathogenesis is complex. A thorough understanding of the molecular mechanism of DR will help determine new and effective diagnostic and therapeutic targets. In recent years, researchers have discovered that miRNAs, circRNAs, and lncRNAs play an important role in many fields, such as tumors, chronic diseases and related complications. This article reviews some RNAs and their molecular mechanisms that play an important role in the DR progression process of retinal microvascular endothelial cells, retinal pigment epithelial cells and retinal nerve cells. The vast majority of circRNAs and lncRNAs are used as upstream regulators of miRNAs to downregulate the expression of miRNAs, which in turn affects the pathological process of different cells. VEGF is the intersection of many RNAs in DR-related signaling pathways and has an important influence on the DR process. Although a variety of miRNAs, circRNAs, and lncRNAs have been found to be involved in DR disease, such as oxidative stress and endothelial dysfunction, the mechanism of action of many ncRNAs is still unclear, and the same ncRNA may play different roles in different models. Therefore, it is necessary to further study their mode of action in the etiology and pathology of the disease to clarify their role in the pathogenesis of DR. However, a standard for estimating ncRNA activity has not been established, and which specific ncRNA plays a dominant role in regulation needs to be further studied. The ultimate goal of basic research is to solve the problems encountered in clinical work. A deep understanding of these three ncRNAs will help develop new strategies to effectively treat this disease and reduce the chance of blindness due to the progression of retinopathy. The goal of molecular therapy targeting retinal pigment epithelial cells and nerve cells is to inhibit their apoptosis, and for microvascular endothelial cells, the proportion of their apoptosis and regeneration must be controlled. Excessive apoptosis and regeneration will aggravate the progression of DR.
There are some shortcomings in the current research on these three types of RNA. First, DR is a chronic disease. In the process of HG-induced cell modeling, it is impossible to simulate the actual situation of the retinal microcirculation of diabetic patients. Optimizing existing experimental methods to simulate HG-induced lesions as much as possible may be more helpful for clinical treatment. Studies on the early stage of DR are not as comprehensive as those on PDR. Second, there are few studies on circRNAs in DR, especially on the mechanism in retinal neuropathy, and the functions of circRNAs, other than miRNA sponges, have not been explored in DR. Finally, most of the exploration targeting RNAs in retinal neuropathy is focused on Müller cells, while there are few studies on rods, cones, bipolar cells, and ganglion cells. Given that neuropathy exists in the early stages of DR, it may emerge as a relatively influential therapeutic target. In summary, the deficiencies of current research may offer directions for future exploration and contribute to the improvement of the understanding of the pathogenesis of DR.
Author Contributions
Conceptualization and wrote the original draft of the manuscript, XC and GZ. Methodology, ZC. Software, YW and RL. Validation, XT and CM. Conceptualization and Funding acquisition, SF. All authors contributed to the article and approved the submitted version.
Funding
The Project of Local Science and Technology Development guided by the Central Government (innovative platform for improving the Prevention and Treatment of Multiple Diseases in Gansu), the Construction Plan of Gansu Province Clinical Research Center for Endocrine Disease (20JR10FA667), the Project of Gansu Natural Science Foundation (20JR10RA681), Lanzhou Science and Technology Development guiding Plan Project (2019-ZD-38), College Students’ Innovation, Entrepreneurship and Excellence Program of Lanzhou University in 2020 (20200060103), and College Students’ Innovation and Entrepreneurship in Lanzhou University in 2021 (20210060155).
Conflict of Interest
The authors declare that the research was conducted in the absence of any commercial or financial relationships that could be construed as a potential conflict of interest.
Publisher’s Note
All claims expressed in this article are solely those of the authors and do not necessarily represent those of their affiliated organizations, or those of the publisher, the editors and the reviewers. Any product that may be evaluated in this article, or claim that may be made by its manufacturer, is not guaranteed or endorsed by the publisher.
References
1. Ogurtsova K, da Rocha Fernandes JD, Huang Y, Linnenkamp U, Guariguata L, Cho NH, et al. IDF Diabetes Atlas: Global Estimates for the Prevalence of Diabetes for 2015 and 2040. Diabetes Res Clin Pract (2017) 128:40–50. doi: 10.1016/j.diabres.2017.03.024
2. Wong TY, Cheung CM, Larsen M, Sharma S, Simó R. Diabetic Retinopathy. Nat Rev Dis Primers (2016) 2:16012. doi: 10.1038/nrdp.2016.12
3. Liu Y, Song Y, Tao L, Qiu W, Lv H, Jiang X, et al. Prevalence of Diabetic Retinopathy Among 13473 Patients With Diabetes Mellitus in China: a Cross-Sectional Epidemiological Survey in Six Provinces. BMJ Open (2017) 7:e013199. doi: 10.1136/bmjopen-2016-013199
4. Park DW, Mansberger SL. Eye Disease in Patients With Diabetes Screened With Telemedicine. Telemed J E Health (2017) 23:113–8. doi: 10.1089/tmj.2016.0034
5. Flaxman SR, Bourne RRA, Resnikoff S, Ackland P, Braithwaite T, Cicinelli MV, et al. Global Causes of Blindness and Distance Vision Impairment 1990-2020: a Systematic Review and Meta-Analysis. Lancet Glob Health (2017) 5:e1221–34. doi: 10.1016/s2214-109x(17)30393-5
6. Leley SP, Ciulla TA, Bhatwadekar AD. Diabetic Retinopathy in the Aging Population: A Perspective of Pathogenesis and Treatment. Clin Interv Aging (2021) 16:1367–78. doi: 10.2147/cia.S297494
7. Solomon SD, Chew E, Duh EJ, Sobrin L, Sun JK, VanderBeek BL, et al. Diabetic Retinopathy: A Position Statement by the American Diabetes Association. Diabetes Care (2017) 40:412–8. doi: 10.2337/dc16-2641
8. Giurdanella G, Anfuso CD, Olivieri M, Lupo G, Caporarello N, Eandi CM, et al. Aflibercept, Bevacizumab and Ranibizumab Prevent Glucose-Induced Damage in Human Retinal Pericytes In Vitro, Through a PLA2/COX-2/VEGF-A Pathway. Biochem Pharmacol (2015) 96:278–87. doi: 10.1016/j.bcp.2015.05.017
9. Sun H, Kang X. Hsa_Circ_0041795 Contributes to Human Retinal Pigment Epithelial Cells (ARPE 19) Injury Induced by High Glucose via Sponging miR-646 and Activating VEGFC. Gene (2020) 747:144654. doi: 10.1016/j.gene.2020.144654
10. Li Y, Cheng T, Wan C, Cang Y. circRNA_0084043 Contributes to the Progression of Diabetic Retinopathy via Sponging miR-140-3p and Inducing TGFA Gene Expression in Retinal Pigment Epithelial Cells. Gene (2020) 747:144653. doi: 10.1016/j.gene.2020.144653
11. Ke N, Pi LH, Liu Q, Chen L. Long Noncoding RNA SNHG7 Inhibits High Glucose-Induced Human Retinal Endothelial Cells Angiogenesis by Regulating miR-543/SIRT1 Axis. Biochem Biophys Res Commun (2019) 514:503–9. doi: 10.1016/j.bbrc.2019.04.141
12. Bonfiglio V, Platania CBM, Lazzara F, Conti F, Pizzo C, Reibaldi M, et al. TGF-β Serum Levels in Diabetic Retinopathy Patients and the Role of Anti-VEGF Therapy. Int J Mol Sci (2020) 21(24):9558. doi: 10.3390/ijms21249558
13. He M, Wang W, Yu H, Wang D, Cao D, Zeng Y, et al. Comparison of Expression Profiling of Circular RNAs in Vitreous Humour Between Diabetic Retinopathy and non-Diabetes Mellitus Patients. Acta Diabetol (2020) 57:479–89. doi: 10.1007/s00592-019-01448-w
14. Platania CBM, Maisto R, Trotta MC, D’Amico M, Rossi S, Gesualdo C, et al. Retinal and Circulating miRNA Expression Patterns in Diabetic Retinopathy: An in Silico and In Vivo Approach. Br J Pharmacol (2019) 176:2179–94. doi: 10.1111/bph.14665
15. Wang J, Gao X, Liu J, Wang J, Zhang Y, Zhang T, et al. Effect of Intravitreal Conbercept Treatment on the Expression of Long Noncoding RNAs and mRNAs in Proliferative Diabetic Retinopathy Patients. Acta Ophthalmol (2019) 97:e902–12. doi: 10.1111/aos.14083
16. Lee Y, Kim M, Han J, Yeom KH, Lee S, Baek SH, et al. MicroRNA Genes are Transcribed by RNA Polymerase II. EMBO J (2004) 23:4051–60. doi: 10.1038/sj.emboj.7600385
17. Lee Y, Ahn C, Han J, Choi H, Kim J, Yim J, et al. The Nuclear RNase III Drosha Initiates microRNA Processing. Nature (2003) 425:415–9. doi: 10.1038/nature01957
18. Suster I, Feng Y. Multifaceted Regulation of MicroRNA Biogenesis: Essential Roles and Functional Integration in Neuronal and Glial Development. Int J Mol Sci (2021) 22(13):6765. doi: 10.3390/ijms22136765
19. Jonas S, Izaurralde E. Towards a Molecular Understanding of microRNA-Mediated Gene Silencing. Nat Rev Genet (2015) 16:421–33. doi: 10.1038/nrg3965
20. Zhou X, Yang PC. MicroRNA: a Small Molecule With a Big Biological Impact. Microrna (2012) 1:1. doi: 10.2174/2211536611201010001
21. Munk R, Panda AC, Grammatikakis I, Gorospe M, Abdelmohsen K. Senescence-Associated MicroRNAs. Int Rev Cell Mol Biol (2017) 334:177–205. doi: 10.1016/bs.ircmb.2017.03.008
22. Hsu MT, Coca-Prados M. Electron Microscopic Evidence for the Circular Form of RNA in the Cytoplasm of Eukaryotic Cells. Nature (1979) 280:339–40. doi: 10.1038/280339a0
23. Yu CY, Kuo HC. The Emerging Roles and Functions of Circular RNAs and Their Generation. J BioMed Sci (2019) 26:29. doi: 10.1186/s12929-019-0523-z
24. Enuka Y, Lauriola M, Feldman ME, Sas-Chen A, Ulitsky I, Yarden Y. Circular RNAs are Long-Lived and Display Only Minimal Early Alterations in Response to a Growth Factor. Nucleic Acids Res (2016) 44:1370–83. doi: 10.1093/nar/gkv1367
25. Guo JU, Agarwal V, Guo H, Bartel DP. Expanded Identification and Characterization of Mammalian Circular RNAs. Genome Biol (2014) 15:409. doi: 10.1186/s13059-014-0409-z
26. Razooky BS, Obermayer B, O’May JB, Tarakhovsky A. Viral Infection Identifies Micropeptides Differentially Regulated in smORF-Containing lncRNAs. Genes (Basel) (2017) 8(8):206. doi: 10.3390/genes8080206
27. Qi D, Li J, Que B, Su J, Li M, Zhang C, et al. Long non-Coding RNA DBCCR1-003 Regulate the Expression of DBCCR1 via DNMT1 in Bladder Cancer. Cancer Cell Int (2016) 16:81. doi: 10.1186/s12935-016-0356-8
28. Khalil AM, Guttman M, Huarte M, Garber M, Raj A, Rivea Morales D, et al. Many Human Large Intergenic Noncoding RNAs Associate With Chromatin-Modifying Complexes and Affect Gene Expression. Proc Natl Acad Sci USA (2009) 106:11667–72. doi: 10.1073/pnas.0904715106
29. Zou T, Jaladanki SK, Liu L, Xiao L, Chung HK, Wang JY, et al. H19 Long Noncoding RNA Regulates Intestinal Epithelial Barrier Function via MicroRNA 675 by Interacting With RNA-Binding Protein HuR. Mol Cell Biol (2016) 36:1332–41. doi: 10.1128/mcb.01030-15
30. Russell RW. Evidence for Autoregulation in Human Retinal Circulation. Lancet (1973) 2:1048–50. doi: 10.1016/s0140-6736(73)92658-5
31. Candiello J, Cole GJ, Halfter W. Age-Dependent Changes in the Structure, Composition and Biophysical Properties of a Human Basement Membrane. Matrix Biol (2010) 29:402–10. doi: 10.1016/j.matbio.2010.03.004
32. Dou X, Duerfeldt AS. Small-Molecule Modulation of PPARs for the Treatment of Prevalent Vascular Retinal Diseases. Int J Mol Sci (2020) 21(23):9251. doi: 10.3390/ijms21239251
33. Zeng Y, Cui Z, Liu J, Chen J, Tang S. MicroRNA-29b-3p Promotes Human Retinal Microvascular Endothelial Cell Apoptosis via Blocking SIRT1 in Diabetic Retinopathy. Front Physiol (2019) 10:1621. doi: 10.3389/fphys.2019.01621
34. Tang W, Guo J, Gu R, Lei B, Ding X, Ma J, et al. MicroRNA-29b-3p Inhibits Cell Proliferation and Angiogenesis by Targeting VEGFA and PDGFB in Retinal Microvascular Endothelial Cells. Mol Vis (2020) 26:64–75.
35. Pan Q, Gao Z, Zhu C, Peng Z, Song M, Li L. Overexpression of Histone Deacetylase SIRT1 Exerts an Antiangiogenic Role in Diabetic Retinopathy via miR-20a Elevation and YAP/Hif1α/VEGFA Depletion. Am J Physiol Endocrinol Metab (2020) 319:E932–e943. doi: 10.1152/ajpendo.00051.2020
36. Wang L, Liu WX, Huang XG. MicroRNA-199a-3p Inhibits Angiogenesis by Targeting the VEGF/PI3K/AKT Signalling Pathway in an In Vitro Model of Diabetic Retinopathy. Exp Mol Pathol (2020) 116:104488. doi: 10.1016/j.yexmp.2020.104488
37. Han N, Xu H, Yu N, Wu Y, Yu L. MiR-203a-3p Inhibits Retinal Angiogenesis and Alleviates Proliferative Diabetic Retinopathy in Oxygen-Induced Retinopathy (OIR) Rat Model via Targeting VEGFA and HIF-1α. Clin Exp Pharmacol Physiol (2020) 47:85–94. doi: 10.1111/1440-1681.13163
38. Xu Y, Xie SC, Ma YC. Low Expression of microRNA-15b Promotes the Proliferation of Retinal Capillary Endothelial Cells and Pericytes by Up-Regulating VEGFA in Diabetic Rats. Eur Rev Med Pharmacol Sci (2019) 23:6018–25. doi: 10.26355/eurrev_201907_18413
39. Liu WL. MicroRNA-9 Inhibits Retinal Neovascularization in Rats With Diabetic Retinopathy by Targeting Vascular Endothelial Growth Factor A. J Cell Biochem (2018) 10:1002. doi: 10.1002/jcb.28081
40. Fu X, Ou B. miR-152/LIN28B Axis Modulates High-Glucose-Induced Angiogenesis in Human Retinal Endothelial Cells via VEGF Signaling. J Cell Biochem (2020) 121:954–62. doi: 10.1002/jcb.28978
41. Wan W, Long Y, Jin X, Li Q, Wan W, Liu H, et al. Protective Role of microRNA-200a in Diabetic Retinopathy Through Downregulation of PDLIM1. J Inflamm Res (2021) 14:2411–24. doi: 10.2147/jir.S303540
42. Wang J, Yao Y, Wang K, Li J, Chu T, Shen H. MicroRNA-148a-3p Alleviates High Glucose-Induced Diabetic Retinopathy by Targeting TGFB2 and FGF2. Acta Diabetol (2020) 57:1435–43. doi: 10.1007/s00592-020-01569-7
43. Kahles F, Findeisen HM, Bruemmer D. Osteopontin: A Novel Regulator at the Cross Roads of Inflammation, Obesity and Diabetes. Mol Metab (2014) 3:384–93. doi: 10.1016/j.molmet.2014.03.004
44. Duan P, Chen S, Zeng Y, Xu H, Liu Y. Osteopontin Upregulates Col IV Expression by Repressing miR-29a in Human Retinal Capillary Endothelial Cells. Mol Ther Nucleic Acids (2020) 20:242–51. doi: 10.1016/j.omtn.2020.02.001
45. Zhang J, Zeng Y, Chen J, Cai D, Chen C, Zhang S, et al. miR-29a/B Cluster Suppresses High Glucose-Induced Endothelial-Mesenchymal Transition in Human Retinal Microvascular Endothelial Cells by Targeting Notch2. Exp Ther Med (2019) 17:3108–16. doi: 10.3892/etm.2019.7323
46. Gu C, Draga D, Zhou C, Su T, Zou C, Gu Q, et al. miR-590-3p Inhibits Pyroptosis in Diabetic Retinopathy by Targeting NLRP1 and Inactivating the NOX4 Signaling Pathway. Invest Ophthalmol Vis Sci (2019) 60:4215–23. doi: 10.1167/iovs.19-27825
47. Bao XY, Cao J. MiRNA-138-5p Protects the Early Diabetic Retinopathy by Regulating NOVA1. Eur Rev Med Pharmacol Sci (2019) 23:7749–56. doi: 10.26355/eurrev_201909_18984
48. Xia F, Sun JJ, Jiang YQ, Li CF. MicroRNA-384-3p Inhibits Retinal Neovascularization Through Targeting Hexokinase 2 in Mice With Diabetic Retinopathy. J Cell Physiol (2018) 234:721–30. doi: 10.1002/jcp.26871
49. Zhang ZZ, Qin XH, Zhang J. MicroRNA-183 Inhibition Exerts Suppressive Effects on Diabetic Retinopathy by Inactivating BTG1-Mediated PI3K/Akt/VEGF Signaling Pathway. Am J Physiol Endocrinol Metab (2019) 316:E1050–e1060. doi: 10.1152/ajpendo.00444.2018
50. Qiu F, Tong H, Wang Y, Tao J, Wang H, Chen L. Inhibition of miR-21-5p Suppresses High Glucose-Induced Proliferation and Angiogenesis of Human Retinal Microvascular Endothelial Cells by the Regulation of AKT and ERK Pathways via maspin. Biosci Biotechnol Biochem (2018) 82:1366–76. doi: 10.1080/09168451.2018.1459179
51. Chen B, Wu L, Cao T, Zheng HM, He T. MiR-221/SIRT1/Nrf2 Signal Axis Regulates High Glucose Induced Apoptosis in Human Retinal Microvascular Endothelial Cells. BMC Ophthalmol (2020) 20:300. doi: 10.1186/s12886-020-01559-x
52. Wang F, Zhang M. Circ_001209 Aggravates Diabetic Retinal Vascular Dysfunction Through Regulating miR-15b-5p/COL12A1. J Transl Med (2021) 19:294. doi: 10.1186/s12967-021-02949-5
53. Shan K, Liu C, Liu BH, Chen X, Dong R, Liu X, et al. Circular Noncoding RNA HIPK3 Mediates Retinal Vascular Dysfunction in Diabetes Mellitus. Circulation (2017) 136:1629–42. doi: 10.1161/circulationaha.117.029004
54. Zou J, Liu KC, Wang WP, Xu Y. Circular RNA COL1A2 Promotes Angiogenesis via Regulating miR-29b/VEGF Axis in Diabetic Retinopathy. Life Sci (2020) 256:117888. doi: 10.1016/j.lfs.2020.117888
55. Liu G, Zhou S, Li X, Ding X, Tian M. Inhibition of Hsa_Circ_0002570 Suppresses High-Glucose-Induced Angiogenesis and Inflammation in Retinal Microvascular Endothelial Cells Through miR-1243/Angiomotin Axis. Cell Stress Chaperones (2020) 25:767–77. doi: 10.1007/s12192-020-01111-2
56. Zeng Q, Liu J. Silencing Circ_0001879 Inhibits the Proliferation and Migration of Human Retinal Microvascular Endothelial Cells Under High-Glucose Conditions via Modulating miR-30-3p. Gene (2020) 760:144992. doi: 10.1016/j.gene.2020.144992
57. Hammes HP, Feng Y, Pfister F, Brownlee M. Diabetic Retinopathy: Targeting Vasoregression. Diabetes (2011) 60:9–16. doi: 10.2337/db10-0454
58. Jiang Q, Liu C, Li CP, Xu SS, Yao MD, Ge HM, et al. Circular RNA-ZNF532 Regulates Diabetes-Induced Retinal Pericyte Degeneration and Vascular Dysfunction. J Clin Invest (2020) 130:3833–47. doi: 10.1172/jci123353
59. Liu C, Ge HM, Liu BH, Dong R, Shan K, Chen X, et al. Targeting Pericyte-Endothelial Cell Crosstalk by Circular RNA-Cpwwp2a Inhibition Aggravates Diabetes-Induced Microvascular Dysfunction. Proc Natl Acad Sci USA (2019) 116:7455–64. doi: 10.1073/pnas.1814874116
60. Hammes HP. Pericytes and the Pathogenesis of Diabetic Retinopathy. Horm Metab Res (2005) 37(Suppl 1):39–43. doi: 10.1055/s-2005-861361
61. Wang Y, Wang L, Guo H, Peng Y, Nie D, Mo J, et al. Knockdown of MALAT1 Attenuates High-Glucose-Induced Angiogenesis and Inflammation via Endoplasmic Reticulum Stress in Human Retinal Vascular Endothelial Cells. BioMed Pharmacother (2020) 124:109699. doi: 10.1016/j.biopha.2019.109699
62. Han N, Tian W, Yu N, Yu L. YAP1 Is Required for the Angiogenesis in Retinal Microvascular Endothelial Cells via the Inhibition of MALAT1-Mediated miR-200b-3p in High Glucose-Induced Diabetic Retinopathy. J Cell Physiol (2020) 235:1309–20. doi: 10.1002/jcp.29047
63. Liu P, Jia SB, Shi JM, Li WJ, Tang LS, Zhu XH, et al. LncRNA-MALAT1 Promotes Neovascularization in Diabetic Retinopathy Through Regulating miR-125b/VE-Cadherin Axis. Biosci Rep (2019) 39(5):BSR20181469. doi: 10.1042/bsr20181469
64. Yu L, Fu J, Yu N, Wu Y, Han N. Long Noncoding RNA MALAT1 Participates in the Pathological Angiogenesis of Diabetic Retinopathy in an Oxygen-Induced Retinopathy Mouse Model by Sponging miR-203a-3p. Can J Physiol Pharmacol (2020) 98:219–27. doi: 10.1139/cjpp-2019-0489
65. Wang J, Wu K, Wang D. A Novel Regulatory Network of Linc00174/miR-150-5p/VEGFA Modulates Pathological Angiogenesis in Diabetic Retinopathy. Can J Physiol Pharmacol (2021) 1–9. doi: 10.1139/cjpp-2021-0036
66. Cai F, Jiang H, Li Y, Li Q, Yang C. Upregulation of Long non-Coding RNA SNHG16 Promotes Diabetes-Related RMEC Dysfunction via Activating NF-κb and PI3K/AKT Pathways. Mol Ther Nucleic Acids (2021) 24:512–27. doi: 10.1016/j.omtn.2021.01.035
67. Zhang R, Ma X, Jiang L, Xia W, Li H, Zhao N, et al. Decreased lncRNA SNHG16 Accelerates Oxidative Stress Induced Pathological Angiogenesis in Human Retinal Microvascular Endothelial Cells by Regulating miR-195/Mfn2 Axis. Curr Pharm Des (2021) 27(27):3047–60. doi: 10.2174/1381612827666210202141541
68. Sun X, Lu Y, Lei T. TPTEP1 Suppresses High Glucose-Induced Dysfunction in Retinal Vascular Endothelial Cells by Interacting With STAT3 and Targeting VEGFA. Acta Diabetol (2021) 58:759–69. doi: 10.1007/s00592-020-01663-w
69. Xiao F, Li L, Fu JS, Hu YX, Luo R. Regulation of the miR-19b-Mediated SOCS6-JAK2/STAT3 Pathway by lncRNA MEG3 is Involved in High Glucose-Induced Apoptosis in hRMECs. Biosci Rep (2020) 40(7):BSR20194370. doi: 10.1042/bsr20194370
70. Liu JY, Yao J, Li XM, Song YC, Wang XQ, Li YJ, et al. Pathogenic Role of lncRNA-MALAT1 in Endothelial Cell Dysfunction in Diabetes Mellitus. Cell Death Dis (2014) 5:e1506. doi: 10.1038/cddis.2014.466
71. Panda-Jonas S, Jonas JB, Jakobczyk-Zmija M. Retinal Pigment Epithelial Cell Count, Distribution, and Correlations in Normal Human Eyes. Am J Ophthalmol (1996) 121:181–9. doi: 10.1016/s0002-9394(14)70583-5
72. Sundelin SP, Nilsson SE, Brunk UT. Lipofuscin-Formation in Cultured Retinal Pigment Epithelial Cells is Related to Their Melanin Content. Free Radic Biol Med (2001) 30:74–81. doi: 10.1016/s0891-5849(00)00444-5
73. Bhutto IA, McLeod DS, Hasegawa T, Kim SY, Merges C, Tong P, et al. Pigment Epithelium-Derived Factor (PEDF) and Vascular Endothelial Growth Factor (VEGF) in Aged Human Choroid and Eyes With Age-Related Macular Degeneration. Exp Eye Res (2006) 82:99–110. doi: 10.1016/j.exer.2005.05.007
74. Li Y, Cheng T, Wan C, Cang Y. Corrigendum to “circRNA_0084043 Contributes to the Progression of Diabetic Retinopathy via T Sponging miR-140-3p and Inducing TGFA Gene Expression in Retinal Pigment Epithelial Cells” [Gene 747 (2020) 144653]. Gene (2021) 800:145789. doi: 10.1016/j.gene.2021.145789
75. Zhang Y, Zheng L, Xu H, Ling L. Circ_0084043 Facilitates High Glucose-Induced Retinal Pigment Epithelial Cell Injury by Activating miR-128-3p/TXNIP-Mediated Wnt/β-Catenin Signaling Pathway. J Cardiovasc Pharmacol (2021) 78:e112–21. doi: 10.1097/fjc.0000000000001039
76. Zeng Q, Luo Y, Fang J, Xu S, Hu YH, Yin M. Circ_0000615 Promotes High Glucose-Induced Human Retinal Pigment Epithelium Cell Apoptosis, Inflammation and Oxidative Stress via miR-646/YAP1 Axis in Diabetic Retinopathy. Eur J Ophthalmol (2021) 11206721211020200. doi: 10.1177/11206721211020200
77. Zhou L, Li FF, Wang SM. Circ-ITCH Restrains the Expression of MMP-2, MMP-9 and TNF-α in Diabetic Retinopathy by Inhibiting miR-22. Exp Mol Pathol (2021) 118:104594. doi: 10.1016/j.yexmp.2020.104594
78. Biswas S, Feng B, Chen S, Liu J, Aref-Eshghi E, Gonder J, et al. The Long Non-Coding RNA HOTAIR Is a Critical Epigenetic Mediator of Angiogenesis in Diabetic Retinopathy. Invest Ophthalmol Vis Sci (2021) 62:20. doi: 10.1167/iovs.62.3.20
79. Li Y, Xu F, Xiao H, Han F. Long Noncoding RNA BDNF-AS Inversely Regulated BDNF and Modulated High-Glucose Induced Apoptosis in Human Retinal Pigment Epithelial Cells. J Cell Biochem (2018) 119:817–23. doi: 10.1002/jcb.26245
80. Jiang L, Wang C, Shen X. LncRNA GAS5 Suppresses ER Stress−Induced Apoptosis and Inflammation by Regulating SERCA2b in HG−treated Retinal Epithelial Cell. Mol Med Rep (2020) 22:1072–80. doi: 10.3892/mmr.2020.11163
81. Zhang D, Qin H, Leng Y, Li X, Zhang L, Bai D, et al. LncRNA MEG3 Overexpression Inhibits the Development of Diabetic Retinopathy by Regulating TGF-β1 and VEGF. Exp Ther Med (2018) 16:2337–42. doi: 10.3892/etm.2018.6451
82. Wang SS, Liao X, Liu F, Zhang Q, Qiu JJ, Fu SH. miR-132 Mediates Cell Permeability and Migration by Targeting Occludin in High-Glucose -Induced ARPE-19 Cells. Endocr J (2021) 68:531–41. doi: 10.1507/endocrj.EJ20-0277
83. Zhao J, Gao S, Zhu Y, Shen X. Significant Role of microRNA−219−5p in Diabetic Retinopathy and its Mechanism of Action. Mol Med Rep (2018) 18:385–90. doi: 10.3892/mmr.2018.8988
84. Chen P, Miao Y, Yan P, Wang XJ, Jiang C, Lei Y. MiR-455-5p Ameliorates HG-Induced Apoptosis, Oxidative Stress and Inflammatory via Targeting SOCS3 in Retinal Pigment Epithelial Cells. J Cell Physiol (2019) 234:21915–24. doi: 10.1002/jcp.28755
85. Xiao Q, Zhao Y, Xu J, Li WJ, Chen Y, Sun HJ. NFE2/miR-423-5p/TFF1 Axis Regulates High Glucose-Induced Apoptosis in Retinal Pigment Epithelial Cells. BMC Mol Cell Biol (2019) 20:39. doi: 10.1186/s12860-019-0223-2
86. Huang JF, Cheng KP, Wang SJ, Huang HM, Wang ZJ. MicroRNA-125b Protects Hyperglycemia-Induced, Human Retinal Pigment Epithelial Cells (RPE) From Death by Targeting Hexokinase 2. Int J Clin Exp Pathol (2018) 11:3111–8.
87. Xue L, Xiong C, Li J, Ren Y, Zhang L, Jiao K, et al. miR-200-3p Suppresses Cell Proliferation and Reduces Apoptosis in Diabetic Retinopathy via Blocking the TGF-β2/Smad Pathway. Biosci Rep (2020) 40(11):BSR20201545. doi: 10.1042/bsr20201545
88. Hu K, Li JL, Yuan XW. MicroRNA-411 Plays a Protective Role in Diabetic Retinopathy Through Targeted Regulating Robo4. Eur Rev Med Pharmacol Sci (2019) 23:9171–9. doi: 10.26355/eurrev_201911_19407
89. Gong Q, Xie J, Li Y, Liu Y, Su G. Enhanced ROBO4 is Mediated by Up-Regulation of HIF-1α/SP1 or Reduction in miR-125b-5p/miR-146a-5p in Diabetic Retinopathy. J Cell Mol Med (2019) 23:4723–37. doi: 10.1111/jcmm.14369
90. Huang Y, Wang Y, Li X, Chen Z, Li X, Wang H, et al. Molecular Mechanism of ER Stress-Induced Gene Expression of Tumor Necrosis Factor-Related Apoptosis-Inducing Ligand (TRAIL) in Macrophages. FEBS J (2015) 282:2361–78. doi: 10.1111/febs.13284
91. Zhang H, Li T, Cai X, Wang X, Li S, Xu B, et al. MicroRNA-203a-3p Regulates CoCl(2)-Induced Apoptosis in Human Retinal Pigment Epithelial Cells by Targeting Suppressor of Cytokine Signaling 3. J Diabetes Complications (2020) 34:107668. doi: 10.1016/j.jdiacomp.2020.107668
92. Yao R, Yao X, Liu R, Peng J, Tian T. Glucose-Induced microRNA-218 Suppresses the Proliferation and Promotes the Apoptosis of Human Retinal Pigment Epithelium Cells by Targeting RUNX2. Biosci Rep (2019) 39(12):BSR20192580. doi: 10.1042/bsr20192580
93. Xiao H, Liu Z. Effects of microRNA−217 on High Glucose−Induced Inflammation and Apoptosis of Human Retinal Pigment Epithelial Cells (ARPE−19) and its Underlying Mechanism. Mol Med Rep (2019) 20:5125–33. doi: 10.3892/mmr.2019.10778
94. Baquié M, St-Onge L, Kerr-Conte J, Cobo-Vuilleumier N, Lorenzo PI, Jimenez Moreno CM, et al. The Liver Receptor Homolog-1 (LRH-1) is Expressed in Human Islets and Protects β-Cells Against Stress-Induced Apoptosis. Hum Mol Genet (2018) 27:406. doi: 10.1093/hmg/ddx402
95. Mo JS, Park HW, Guan KL. The Hippo Signaling Pathway in Stem Cell Biology and Cancer. EMBO Rep (2014) 15:642–56. doi: 10.15252/embr.201438638
96. Lee K, Hu Y, Ding L, Chen Y, Takahashi Y, Mott R, et al. Therapeutic Potential of a Monoclonal Antibody Blocking the Wnt Pathway in Diabetic Retinopathy. Diabetes (2012) 61:2948–57. doi: 10.2337/db11-0300
97. Simó R, Sundstrom JM, Antonetti DA. Ocular Anti-VEGF Therapy for Diabetic Retinopathy: the Role of VEGF in the Pathogenesis of Diabetic Retinopathy. Diabetes Care (2014) 37:893–9. doi: 10.2337/dc13-2002
98. Inoue M, Sakuta N, Watanabe S, Zhang Y, Yoshikaie K, Tanaka Y, et al. Structural Basis of Sarco/Endoplasmic Reticulum Ca(2+)-ATPase 2b Regulation via Transmembrane Helix Interplay. Cell Rep (2019) 27:1221–1230.e1223. doi: 10.1016/j.celrep.2019.03.106
99. Zuccato C, Cattaneo E. Brain-Derived Neurotrophic Factor in Neurodegenerative Diseases. Nat Rev Neurol (2009) 5:311–22. doi: 10.1038/nrneurol.2009.54
100. Antonetti DA, Lieth E, Barber AJ, Gardner TW. Molecular Mechanisms of Vascular Permeability in Diabetic Retinopathy. Semin Ophthalmol (1999) 14:240–8. doi: 10.3109/08820539909069543
101. Duh EJ, Sun JK, Stitt AW. Diabetic Retinopathy: Current Understanding, Mechanisms, and Treatment Strategies. JCI Insight (2017) 2(14):e93751. doi: 10.1172/jci.insight.93751
102. Barber AJ. A New View of Diabetic Retinopathy: a Neurodegenerative Disease of the Eye. Prog Neuropsychopharmacol Biol Psychiatry (2003) 27:283–90. doi: 10.1016/s0278-5846(03)00023-x
103. Simó R, Hernández C. Novel Approaches for Treating Diabetic Retinopathy Based on Recent Pathogenic Evidence. Prog Retin Eye Res (2015) 48:160–80. doi: 10.1016/j.preteyeres.2015.04.003
104. van Dijk HW, Verbraak FD, Kok PH, Stehouwer M, Garvin MK, Sonka M, et al. Early Neurodegeneration in the Retina of Type 2 Diabetic Patients. Invest Ophthalmol Vis Sci (2012) 53:2715–9. doi: 10.1167/iovs.11-8997
105. Li W, Jin L, Cui Y, Nie A, Xie N, Liang G. Bone Marrow Mesenchymal Stem Cells-Induced Exosomal microRNA-486-3p Protects Against Diabetic Retinopathy Through TLR4/NF-κb Axis Repression. J Endocrinol Invest (2021) 44:1193–207. doi: 10.1007/s40618-020-01405-3
106. Chen Z, Yang Z, Li X, Wang H, Wang Y, Ding C, et al. microRNA-320a Prevent Müller Cells From Hypoxia Injury by Targeting Aquaporin-4. J Cell Biochem (2020) 10:1002. doi: 10.1002/jcb.29524
107. Zhang J, Wu L, Chen J, Lin S, Cai D, Chen C, et al. Downregulation of MicroRNA 29a/B Exacerbated Diabetic Retinopathy by Impairing the Function of Müller Cells via Forkhead Box Protein O4. Diabetes Vasc Dis Res (2018) 15:214–22. doi: 10.1177/1479164118756239
108. Wang J, Zhang J, Chen X, Yang Y, Wang F, Li W, et al. miR-365 Promotes Diabetic Retinopathy Through Inhibiting Timp3 and Increasing Oxidative Stress. Exp Eye Res (2018) 168:89–99. doi: 10.1016/j.exer.2017.11.006
109. Zhang X, Yang Y, Feng Z. Suppression of microRNA-495 Alleviates High-Glucose-Induced Retinal Ganglion Cell Apoptosis by Regulating Notch/PTEN/Akt Signaling. BioMed Pharmacother (2018) 106:923–9. doi: 10.1016/j.biopha.2018.07.018
110. Foell D, Frosch M, Sorg C, Roth J. Phagocyte-Specific Calcium-Binding S100 Proteins as Clinical Laboratory Markers of Inflammation. Clin Chim Acta (2004) 344:37–51. doi: 10.1016/j.cccn.2004.02.023
111. Dong N, Wang Y. MiR-30a Regulates S100A12-Induced Retinal Microglial Activation and Inflammation by Targeting Nlrp3. Curr Eye Res (2019) 44:1236–43. doi: 10.1080/02713683.2019.1632350
112. Zhang J, Chen C, Wu L, Wang Q, Chen J, Zhang S. Chen, Z. C-Myc Contributes to the Release of Müller Cells-Derived Proinflammatory Cytokines by Regulating lncRNA MIAT/XNIP Pathway. Int J Biochem Cell Biol (2019) 114:105574. doi: 10.1016/j.biocel.2019.105574
113. Fu S, Zheng Y, Sun Y, Lai M, Qiu J, Gui F, et al. Suppressing Long Noncoding RNA OGRU Ameliorates Diabetic Retinopathy by Inhibition of Oxidative Stress and Inflammation via miR-320/USP14 Axis. Free Radic Biol Med (2021) 169:361–81. doi: 10.1016/j.freeradbiomed.2021.03.016
114. Li XJ. Long non-Coding RNA Nuclear Paraspeckle Assembly Transcript 1 Inhibits the Apoptosis of Retina Müller Cells After Diabetic Retinopathy Through Regulating miR-497/Brain-Derived Neurotrophic Factor Axis. Diabetes Vasc Dis Res (2018) 15:204–13. doi: 10.1177/1479164117749382
115. Liu T, Zhou Y, Ko KS, Yang H. Interactions Between Myc and Mediators of Inflammation in Chronic Liver Diseases. Mediators Inflamm (2015) 2015:276850. doi: 10.1155/2015/276850
116. Zhang J, Chen M, Chen J, Lin S, Cai D, Chen C, et al. Long non-Coding RNA MIAT Acts as a Biomarker in Diabetic Retinopathy by Absorbing miR-29b and Regulating Cell Apoptosis. Biosci Rep (2017) 37(2):BSR20170036. doi: 10.1042/bsr20170036
117. Zhang YL, Hu HY, You ZP, Li BY, Shi K. Targeting Long non-Coding RNA MALAT1 Alleviates Retinal Neurodegeneration in Diabetic Mice. Int J Ophthalmol (2020) 13:213–9. doi: 10.18240/ijo.2020.02.03
118. Zhang JR, Sun HJ. Roles of Circular RNAs in Diabetic Complications: From Molecular Mechanisms to Therapeutic Potential. Gene (2020) 763:145066. doi: 10.1016/j.gene.2020.145066
Keywords: diabetic retinopathy, miRNA, lncRNA, circRNA, diabetes
Citation: Chang X, Zhu G, Cai Z, Wang Y, Lian R, Tang X, Ma C and Fu S (2021) miRNA, lncRNA and circRNA: Targeted Molecules Full of Therapeutic Prospects in the Development of Diabetic Retinopathy. Front. Endocrinol. 12:771552. doi: 10.3389/fendo.2021.771552
Received: 06 September 2021; Accepted: 25 October 2021;
Published: 10 November 2021.
Edited by:
Md Shahidul Islam, University of KwaZulu-Natal, South AfricaReviewed by:
Claudio Bucolo, University of Catania, ItalyHamed Shoorei, Birjand University of Medical Sciences, Iran
Ming Zhang, Jilin University, China
Copyright © 2021 Chang, Zhu, Cai, Wang, Lian, Tang, Ma and Fu. This is an open-access article distributed under the terms of the Creative Commons Attribution License (CC BY). The use, distribution or reproduction in other forums is permitted, provided the original author(s) and the copyright owner(s) are credited and that the original publication in this journal is cited, in accordance with accepted academic practice. No use, distribution or reproduction is permitted which does not comply with these terms.
*Correspondence: Songbo Fu, ZnVzYkBsenUuZWR1LmNu
†These authors have contributed equally to this work and share first authorship