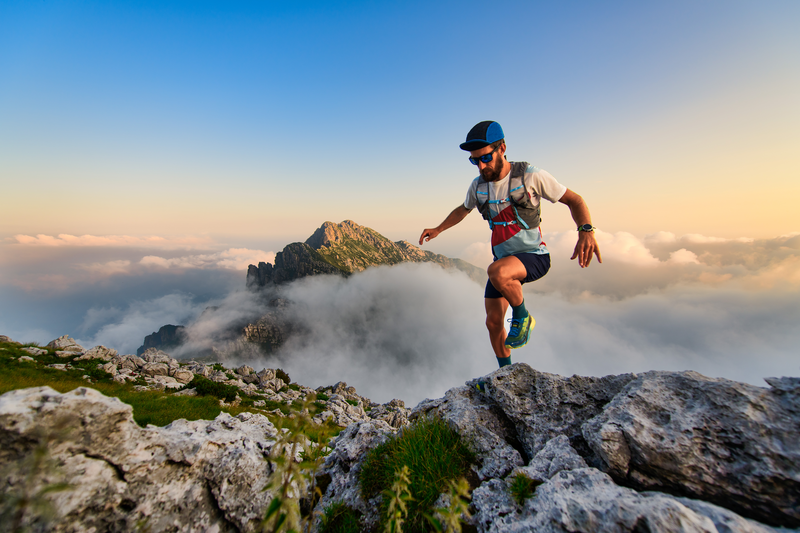
95% of researchers rate our articles as excellent or good
Learn more about the work of our research integrity team to safeguard the quality of each article we publish.
Find out more
REVIEW article
Front. Endocrinol. , 07 December 2021
Sec. Clinical Diabetes
Volume 12 - 2021 | https://doi.org/10.3389/fendo.2021.768850
This article is part of the Research Topic Effects of Pharmacologic Therapy for Diabetes Mellitus on The Endocrine System. View all 7 articles
Non-alcoholic fatty liver disease (NAFLD) is the most prevalent liver disease worldwide, and more than half of individuals diagnosed with type 2 diabetes concurrently present with NAFLD. There is a bidirectional pathological relationship between the two conditions, whereby NAFLD increases the risk of type 2 diabetes, and type 2 diabetes contributes to and accelerates the progression of NAFLD. Furthermore, over 30% of patients with NAFLD progress to non-alcoholic liver steatohepatitis (NASH), which then increases the risk of cirrhosis and hepatocellular carcinoma. Despite its high prevalence and the potential clinical implications, the underlying pathogenesis of NAFLD has yet to be fully elucidated, and there is no consensus regarding standard diagnosis and treatment for either NALFD or NASH. As patients with both NASH and type 2 diabetes have impaired hepatic function owing to chronic inflammation and the resulting structural changes caused by hepatic fat accumulation, they face reduced options for antidiabetic treatment. SGLT-2 inhibitors inhibit glucose reabsorption in the proximal tubule, with increased excretion of glucose in urine and decreased glucose levels in plasma, and their glycemia-lowering effect is insulin-independent. Several other beneficial effects have been reported for SGLT-2 inhibitors, including reduced risks of cardiovascular and renal diseases, improved blood pressure control, body weight reduction, and reductions in liver fat content. Experimental studies in mouse models have suggested that SGLT-2 inhibitors may have beneficial modulatory effects on NAFLD/NASH. Several trials in patients with type 2 diabetes have also suggested that these drugs may be useful in treating both type 2 diabetes and NAFLD or NASH. However, further research is needed to identify the mechanisms by which SGLT-2 inhibitors affect fatty liver and steatohepatitis. In this state-of-the-art review, we explore the literature on the efficacy of SGLT-2 inhibitors in patients with type 2 diabetes and NASH, and present arguments for and against the use of SGLT-2 inhibitors in this patient population.
Worldwide, the increasing prevalence of type 2 diabetes is occurring alongside a corresponding increase in the prevalence of non-alcoholic fatty liver disease (NAFLD). The co-existence of these two conditions is well known; the association is driven by a bidirectional pathological relationship whereby NAFLD increases the risk of type 2 diabetes, and type 2 diabetes contributes to, and accelerates, the progression of NAFLD (1). NAFLD is the most prevalent liver disease worldwide, affecting 25% to 30% of the global population (2). Globally, approximately 55% of patients with type 2 diabetes present with NAFLD, while in Japan, up to 68% of patients with type 2 diabetes concurrently present with NAFLD (3). NAFLD has several phases of progression, with approximately 20% to 30% of those who develop NAFLD progressing to non-alcoholic liver steatohepatitis (NASH) (4). Patients with NASH and concomitant type 2 diabetes are at an increased risk of progression to cirrhosis and hepatocellular carcinoma (5, 6).
NAFLD is commonly characterized by the presence of hepatic steatosis (with ≥5% of hepatocytes affected) without any sign of hepatocyte ballooning and in the absence of any secondary causes. Meanwhile, NASH is a more aggressive disorder that equally exhibits hepatic steatosis, but additionally—and crucially—includes hepatocyte ballooning (i.e., hepatocellular injury) and lobular necroinflammation with or without pericellular and perisinusoidal fibrosis (7, 8).
While patients with NAFLD may have no specific symptoms, some individuals may present with fatigue and right upper quadrant abdominal pain. Patients with NAFLD are also more likely to be obese and hypertensive and to show mild or moderate hepatomegaly. Common laboratory findings in NAFLD patients are hyperlipidemia, hyperglycemia, decreased insulin sensitivity, and mild or moderate elevations of liver enzymes, specifically, alanine and aspartate aminotransferases (ALT and AST) (9). The clinical presentation of NASH is similar to that of NAFLD (8); however, inflammation and hepatic injury must also be present for a diagnosis of NASH.
Currently, the gold-standard histological technique for detecting NAFLD and NASH is liver biopsy. This technique is highly invasive, prone to sampling errors, and may cause complications. Ultrasonography is the primary diagnostic imaging technique for NAFLD (1).
Although the pathogenesis of NAFLD has yet to be fully elucidated, several theories have been proposed. Most widely accepted is the two-hit theory (10), featuring hepatic lipid accumulation as the first hit and development of liver inflammation and fibrogenesis as the second. However, many investigators deem this model too simplistic, as several factors have been implicated in the development of NAFLD and NASH (11, 12). In particular, insulin resistance, imbalance of the lipid metabolism with adipose tissue dysfunction, hyperinsulinemia, lipo- and glucotoxicity, oxidative stress, and inflammation have been recognized as critical events in NAFLD development (6). In patients with insulin resistance, the effect of insulin is suppressed, leading to constant glucose output from the liver. Peripheral insulin resistance mainly occurs in adipose tissue and muscles, whereby glucose uptake and utilization mediated by insulin are disrupted, and there is an increased decomposition of adipose tissue. In skeletal muscle, there is a lack of glucose uptake and glycogen synthesis that leads to postprandial hyperglycemia. Consequently, the liver glucose uptake increases, triggering carbohydrate response element-binding protein (ChREBP) activation, a central metabolic coordinator, which stimulates intracellular glycolysis, contributing to ectopic de novo lipogenesis. In adipose tissue, insulin resistance interferes with the suppression of lipolysis. As a result of unrestrained lipolysis, triglycerides are hydrolyzed, releasing vast amounts of free fatty acids (FFAs) and glycerol. These circulating FFAs accumulate in the liver and are the primary substrates for hepatic triglyceride synthesis, eventually leading to intrahepatic triglyceride accumulation or steatosis. In the liver, selective hepatic insulin resistance increases gluconeogenesis and lipogenesis (13). Excess non-esterified FFAs released into the bloodstream lead to excessive uptake by tissues including the liver, heart, pancreas, muscle, and endothelial cells, causing significant organ dysfunction, i.e., lipotoxicity, which further promotes peripheral insulin resistance, hepatic gluconeogenesis, hyperglycemia, and pancreatic beta-cell dysfunction (14). Diacylglycerols, ceramides, and long-chain fatty acyl-coenzyme A (CoA) are toxic lipids that activate several inflammatory pathways. They can also cause direct damage resulting in hepatocellular injury and liver fibrosis (9) and indirectly increase oxidative stress, thereby triggering further inflammation and apoptosis (14).
Lipotoxicity is closely intertwined with glucotoxicity or chronically elevated plasmatic glucose concentrations causing cellular dysfunction and insulin resistance, and progressive metabolic deterioration is also associated with increased de novo lipogenesis. Both are major contributors to insulin resistance, beta-cell dysfunction, impaired insulin secretion, and ectopic fat deposits. Two pathways for de novo lipogenesis that result from chronically elevated glucose have been described. One is a direct pathway of increased tricarboxylic acid cycle activity and CoA synthesis, a substrate for de novo lipogenesis and gluconeogenesis (15). The other is an indirect pathway that involves increasing ChREBP expression in the liver and extrahepatic tissues, including the pancreas, kidney, and skeletal muscle. Additionally, liver X receptor α (LXRα), a ligand-activated transcription factor, is increasingly expressed, leading to elevated transcription of ATP-citrate lyase, fatty acid synthase and SCD-1 (16). The active complex of LXRα and enhancer-binding protein β binds to the sterol regulator element-binding protein (SREBP)-1c at sites required for insulin induction (17).
The SREBP family plays a key role in lipid homeostasis, mainly that of cholesterol and triglycerides, as well as other important roles in the regulation of physiological functions of other organs (18). SREBP-1c, specifically, is expressed mainly in the brain, liver, white adipose tissue, skeletal muscle and adrenal glands of humans, and it is known to mediate, at least in part, insulin’s effects on hepatic lipogenesis (18, 19). Insulin increases the expression of SREBP-1c, it promotes the mobilization of the SREBP-cleavage activating protein (SCAP)/SREBP-1c complex to the Golgi apparatus, SREBP-1c cleavage, and the successive processes leading to hepatic lipogenesis and hepatic steatosis (20).
Oxidative stress, along with mitochondrial dysfunction, increased expression of pro-inflammatory cytokines and adipokines, and subsequent lipid peroxidation are relevant factors not only in the development of NAFLD (21) but also the progression to NASH (22). Adiponectin is said to have a protective effect in NAFLD by potentiating insulin’s ability to decrease glucose production and output, as well as down-regulating SREBP-1c and suppressing lipogenesis. Lipcalin-2 (LCN-2) and omentin-1, both newly discovered adipokines, seem to have a protective effect in NAFLD (23); however, LCN-2 may also contribute to insulin resistance. Leptin, conversely, is potently mitogenic on hepatic cells, inhibits cell apoptosis, and promotes processes involved in liver fibrosis (24). Other adipokines, such as resistin, seem to be involved in insulin resistance, while tumor necrosis factor alfa (TNF-α) and IL-6, which may be present at early and late stages of NAFLD, increase expression of SREBP-1c in the liver and further promote insulin resistance (21).
Recent studies have also shown that NAFLD may potentially occur in the absence of insulin resistance. Some individuals with single nucleotide polymorphisms within the patatin-like phospholipase 3 (PNPLA3) gene develop hepatic steatosis without first developing insulin resistance (22, 25). Clinical analyses have suggested that transcription factor 7–like 2 (TCF7L2) polymorphism modulates beta-cell function, lipid metabolism, glucose homeostasis, postprandial lipoprotein and adipokine responses, and hepatocyte apoptosis with the ingestion of fats. This polymorphism is associated with a predisposition to developing diabetes and liver injury leading to NAFLD and NASH among patients with familial dyslipidemia (26).
Patients with NASH have impaired hepatic function owing to chronic inflammation and resulting structural changes caused by hepatic fat accumulation. Consequently, because of resultant drug hepatotoxicity, patients with type 2 diabetes and NASH face reduced options for antidiabetic treatment (27). For this reason, it has been suggested that concurrent type 2 diabetes and NAFLD require customized pharmacological management (28) to prevent the exacerbation or worsening of hepatic dysfunction.
The published literature reveals that although the two disorders feature overlapping characteristics, patients with type 2 diabetes and NAFLD or NASH exhibit different pathophysiology than patients with type 2 diabetes only (illustrated in Figure 1). There are also important differences between patient populations with and without diabetes who develop NAFLD and progress to NASH. For instance, NASH in non-diabetic patients is associated with metabolic syndrome, with an increased risk of NASH in patients exhibiting more features of metabolic syndrome (29). Furthermore, patients with NAFLD and metabolic syndrome are significantly more likely to have marked insulin resistance and severe steatosis and portal inflammation as indicated by liver biopsies (30).
Figure 1 A Venn diagram of the symptoms, pathological features, and genetic features of type 2 diabetes and non-alcoholic steatohepatitis.
Sodium-glucose cotransporters (SGLTs) are responsible for glucose absorption from the small intestine as well as glucose reabsorption from the proximal tubule of the kidney (31, 32). As SGLTs actively transport glucose and sodium across membranes, glucose transport across the apical membrane and against the concentration gradient is driven by energy produced by the cotransport of sodium. While there are several SGLTs, SGLT-2 is responsible for more than 90% of the reabsorption of filtered glucose (33).
SGLT-2 inhibitors inhibit the reabsorption of glucose in the proximal tubule, resulting in increased glucose excretion in urine and decreased glucose levels in plasma. The resultant glycemia-lowering effect is insulin-independent (8). Aside from reducing hyperglycemia and increasing insulin sensitivity, several other beneficial clinical effects have been reported for SGLT-2 inhibitors, including reduced risks of cardiovascular and renal diseases, improved blood pressure control, body weight reduction, and reductions in liver fat content (34–37). In addition, experimental studies in mouse models suggest that SGLT-2 inhibition may have beneficial modulatory effects on NAFLD/NASH (38, 39). Of note, in an obese mouse model that exhibited characteristics of NAFLD, remogliflozin effectively improved ALT and AST levels and reduced hepatic triglyceride content (39). More recently, we investigated the effects of the SGLT-2 inhibitor dapagliflozin on glucose metabolism in a mouse model of NASH with concurrent type 2 diabetes. We demonstrated that dapagliflozin improved glycemic control and resolved ascites in the NASH mice compared with the untreated mice (40). Although further research is needed to identify the mechanisms by which SGLT-2 inhibitors affect fatty liver and steatohepatitis, several trials in patients with type 2 diabetes have suggested that these drugs may be useful in treating both type 2 diabetes and NAFLD or NASH (41–46).
Several review articles on the role of antidiabetic agents in the management of NASH have proposed hypothetical models of how the underlying mechanism of action of these drugs might improve NASH parameters (14, 47, 48). A very recent meta-analysis including a total of 1950 patients with type 2 diabetes with and without NAFLD concluded that SGLT-2 inhibitor treatment improved liver structure and function (49). In general, the proposed mechanism explaining the action of SGLT-2 inhibitors in NAFLD/NASH is based on the reduction of plasma glucose due to glucosuria, and reversal of glucotoxicity, as well as reductions in circulating insulin and body weight. These collectively result in decreased peripheral and hepatic insulin resistance, and lead, in turn, to a reduction of de novo lipogenesis in the liver. Plasma glucagon levels are directly increased by SGLT-2 inhibitors, which stimulates beta-oxidation and ketone production, thereby shifting to fatty acid metabolism and ketosis. Although gluconeogenesis may increase, this is counterbalanced by glucosuria. Together, these events hypothetically lead to a decrease in the lipid content in the liver (48).
While SGLT-2 inhibitors reduce the risk of cardiovascular diseases, other antidiabetic medications such as glucagon-like-1 (GLP-1) receptor agonists, and the thiazolidinediones such as pioglitazone, have also been shown to exert cardioprotective effects, albeit via very different mechanisms from SGLT-2 inhibitors. The cardioprotective effect of SGLT-2 inhibitors is attributed to its hemodynamic effects, which have an early onset: reduced blood pressure, decreased intravascular volume, and reduced aortic stiffness. The cardioprotective effect of GLP-1 receptor agonists results from antiatherogenic/anti-inflammatory mechanisms, which have a slow progressive onset, such as reduced plasmatic levels of inflammatory and oxidative stress markers, including IL-6, ICAM-1, nitrotyrosine, 8-iso prostaglandin F2α, MCP-1, and TNFα (50, 51). Although GLP-1 receptor agonists and SGLT-2 inhibitors act via different mechanisms of action, they share common effects such as weight loss (via appetite inhibition and increased excretion of calories in the urine, respectively), amplification of beta-cell function (mediated by GLP-1 receptor and reversal of glucotoxicity, respectively), increased insulin sensitivity owing to weight loss and reversal of glucotoxicity, blood pressure reduction (natriuresis and reduction of intravascular volume), anti-inflammatory effects (mainly GLP-1 receptor agonists), natriuresis, protection against diabetic renal complications, and modest effects on lipid profile. Thus, it is expected that this combination could result in additive benefits, such as enhanced peripheral insulin sensitivity, greater reduction in systolic/diastolic blood pressure, and possibly prevention of diabetic glomerulosclerosis compared with monotherapy. Further, as both GLP-1 receptor agonists and SGLT-2 inhibitors reduce visceral fat, and GLP-1 receptor agonists reduce fat deposits in the liver in patients with NAFLD/NASH (52, 53), this drug combination may be instrumental in the treatment of NAFLD/NASH.
Another class of antidiabetic drugs comprises the thiazolidinediones, which are peroxisome proliferator-activated receptor gamma (PPARγ) agonists. While three isoforms of PPAR exist (alpha [α], beta/delta [β/δ] and γ), PPARγ is most highly expressed in adipose tissue, with even greater expression observed in the liver of patients with NAFLD. The activation of PPARγ results in the synthesis of several adipokines, including adiponectin, which promote FA oxidation, storage of fat in adipose tissue with consequent reduction of lipolysis, and less accumulation of fat in the liver. Although the preclinical evidence obtained from mouse models was promising, clinical evaluation of thiazolidinediones in patients with NASH or NAFLD produced variable outcomes or did not reach statistical significance. However, several meta-analyses of randomized controlled trials have concluded that pioglitazone, although less potent than rosiglitazone, is superior in suppressing hepatic fibrosis and inflammation along with the reversal of steatosis (54). More recent re-evaluations of previously published evidence have suggested that in addition to its glucose-lowering effects, pioglitazone also has cardioprotective effects (55, 56); however, the mechanism underlying these effects remains unclear (56). Pioglitazone exerts a robust protective effect against atherosclerosis-driven cardiac and cerebrovascular events; in preclinical and clinical studies, pioglitazone reduced left ventricular systolic and diastolic dimensions and improved left systolic and diastolic functions. Pioglitazone also directly benefits cardiomyocyte electrophysiology, energetic metabolism, ischemia-reperfusion injury, cardiac remodeling, neurohormonal activation, and pulmonary circulation. However, adverse events related to increases in body weight, peripheral circulation, congestive heart failure, and bone mineral density, among others, must be considered when prescribing this drug (57). A clinical study comparing SGLT-2 inhibitor tofogliflozin vs pioglitazone showed that hepatic steatosis significantly decreased in both groups after 24 weeks of treatment, but to a greater extent with pioglitazone than with tofogliflozin. While tofogliflozin reduced body weight, pioglitazone increased body weight from baseline. However, it is possible that combining both drugs could potentially result in an additive effect on hepatic steatosis and could balance out the effects of pioglitazone on body weight gain (58). A recent preclinical study using a mouse model of type 2 diabetes showed that a combination of the SGLT-2 inhibitor ipragliflozin plus pioglitazone significantly improved multiple NASH parameters, including hyperglycemia, insulin resistance, hyperlipidemia and liver injury (hepatic steatosis and fibrosis). Moreover, these parameters were additively improved with combination treatment versus ipragliflozin monotherapy (59).
This state-of-the-art review explores the current literature reporting the efficacy of SGLT-2 inhibitors in patients with type 2 diabetes and NASH and presents arguments for and against the use of SGLT-2 inhibitors in this patient population, where it plays a regulatory role in adipocyte differentiation, adipogenesis, and lipid metabolism.
Ultimately, the goal of NASH treatment is to arrest and revert steatohepatitis in order to stop the progression of hepatocellular injury and fibrosis. Additionally, and ideally, the risk of cardiovascular events should also be reduced with the selected treatment, regardless of the presence or absence of diabetes. Although there is an increasing need for effective treatments for NAFLD and NASH, until very recently, no specific on-label treatment had been approved or recommended (60). Furthermore, treating patients with both type 2 diabetes and NAFLD or NASH is complex and challenging because of the various comorbidities that frequently exist in these patients (61), and data from clinical trials of antidiabetic treatment typically lack high-quality and clinically relevant endpoints related to liver histology. Of note, saroglitazar, a dual PPARα/γ agonist, was approved in India in March 2020 for the treatment of NASH. However, at the time of writing this review, there are currently no drugs approved by the FDA or European Medicine Agency for this disease. Nevertheless, several drugs being developed for the treatment of NASH are currently in advanced phase 3 clinical trials (e.g., obeticholic acid, cenicriviroc, aramchol, resmetirom, dapagliflozin, and semaglutide). Moreover, others in the pipeline (e.g., elapectin, MSDC-0602K, lanifibranor, efruxifermin, and tesamorelin) are expected to commence late-phase trials in the near future (62).
The primary recommendations for managing patients with type 2 diabetes and concurrent NAFLD or NASH who are overweight or obese include lifestyle modifications (e.g., increased exercise (63) and dietary adjustments) to achieve sustained weight loss. Weight loss of 5% or more has been shown to reduce steatosis and improve liver enzyme levels, glycemic control, and insulin sensitivity. Moreover, weight loss of 7% or more has been associated with NASH improvements, including reduced fibrosis (1, 64). Other recommendations tend to focus on managing the presence of comorbidities. Surgical treatments such as bariatric surgery and liver transplants are high-cost treatment alternatives, with limited evidence regarding efficacy and safety. Furthermore, these treatments are only accessible to a limited number of patients (65).
As noted in Section 1, several off-label treatments have been explored as potentially suitable therapies for patients with NAFLD and NASH. For example, the efficacy of insulin-sensitizing medications, such as thiazolidinediones and metformin, has been assessed in small randomized controlled trials (66). Of these, pioglitazone is the most studied drug in patients with NAFLD and remains the only recommended medication for NAFLD or NASH (although it should be noted that this is off-label usage) (67). We previously compared the effects of six-month treatments with pioglitazone, metformin, sitagliptin, or a non-oral antidiabetic drug (control) combined with guidance on diet and exercise in a cohort of men with type 2 diabetes and NAFLD. Pioglitazone was found to yield the most marked improvements in liver fat levels (68). Importantly, pioglitazone must be prescribed with caution as thiazolidinediones tend to exhibit a range of adverse effects such as weight gain, peripheral edema, cardiac failure, bladder cancer, and bone fragility (69). Other antidiabetic agents which have shown promising preliminary results include the GLP-1 receptor agonists (liraglutide, exenatide, dulaglutide, and semaglutide). A recently published meta-analysis of pooled data from 11 randomized controlled trials of GLP-1 receptor agonists in patients with NAFLD or NASH indicated significant improvements in liver enzyme levels and liver fat content following treatment (70). In addition, several other therapies have been trialed, including antioxidants (e.g., vitamin E), lipid-lowering drugs (e.g., statins, ezetimibe, and peroxisome proliferator-activated receptor-α agonists), pentoxifylline, ursodeoxycholic acid, angiotensin receptor blockers, and n-3 polyunsaturated fatty acids. However, further evidence on the efficacy and safety of these treatments in randomized controlled trials is required before they can be granted regulatory approval for the treatment of NAFLD or NASH (71).
SGLT-2 inhibitors comprise a new family of antidiabetic drugs, of which ipragliflozin achieved the first global approval for clinical use in Japan in 2014 to treat type 2 diabetes (72). There are now seven SGLT-2 inhibitors that have been approved and are currently used in Japan (i.e., ipragliflozin, dapagliflozin, tofogliflozin, luseogliflozin, canagliflozin, ertugliflozin, and empagliflozin). In contrast, only four SGLT-2 inhibitors (dapagliflozin, canagliflozin, empagliflozin, and ertugliflozin) have been approved by the Food and Drug Administration (FDA) in the United States. Aside from their hypoglycemic effects, these antidiabetic drugs are known to have cardiovascular, renal, and hepatic extraglycemic benefits (73). Several pilot studies have been conducted in Japan to determine the efficacy and safety of SGLT-2 inhibitors for patients with type 2 diabetes and NAFLD or NASH (74–77). These studies showed that patients with NAFLD achieved significant reductions in ALT levels, body weight, and fatty liver index. A phase 3 trial of dapagliflozin efficacy and action in NASH (DEAN; ClinicalTrials.gov Identifier NCT03723252) is currently ongoing and aims to assess changes in liver histology as well as various metabolic parameters in patients with NASH. Recently, a meta-analysis of randomized trials evaluated 1950 patients with type 2 diabetes with and without NAFLD and concluded that SGLT-2 inhibitor treatment improved liver structure and function (49). Table 1 lists the typical outcomes measured in the studies conducted to date; these include changes in serum aminotransferase levels, NASH histology scores, and liver fat levels. Additional details including the intervention evaluated, the characteristics of the patient population, and the study duration can be found in Table 2. These outcome measures are generally indicative of the condition of the liver, although some are more sensitive than others. For instance, levels of transaminases should be interpreted with care as some patients with NAFLD or NASH can still present with normal ALT levels. Furthermore, improvements in liver histology after SGLT-2 inhibitor treatment may not be reflected in decreased liver enzymes (34). It is also very important to consider how SGLT2 inhibitors affect the expression of lipid metabolism genes, such as ACC, SREBP-1c, CPT1a, and PPARα, in the liver. Recently, hepatic mitochondrial dysfunction has attracted attention as a common factor underlying both diabetes mellitus and NAFLD/NASH (81); therefore, it is critical to elucidate how SGLT2 inhibitors might impact mitochondrial function. The typical monitoring of patients on SGLT-2 inhibitors to treat this dual condition includes metabolic blood panels (liver enzymes, HbA1c, and fasting plasma glucose) and radiological imaging, including ultrasound, computed tomography, magnetic resonance imaging (MRI), MR spectroscopy, and vibration-controlled transient elastography.
Table 1 Outcome measures commonly recorded in studies of the efficacy of SGLT-2 inhibitors in patients with concurrent type 2 diabetes mellitus and non-alcoholic steatohepatitis.
Table 2 Intervention, interventional group characteristics, and duration of studies exploring the efficacy of SGLT-2 inhibitors in patients with concurrent type 2 diabetes mellitus and non-alcoholic fatty liver disease.
Although formal head-to-head studies are lacking, we have used data from the published literature to conduct a comparative analysis of the efficacies of dapagliflozin, canagliflozin, empagliflozin, ertugliflozin, ipragliflozin, luseogliflozin, and remogliflozin (Table 3). When using MRI to observe change in liver fat content, dapagliflozin combined with omega-3 carboxylic acids improved NAFLD in a randomized, controlled, double-blind trial of patients with type 2 diabetes (41). Furthermore, in patients with concurrent type 2 diabetes and NAFLD who were already receiving standard of care for type 2 diabetes, add-on empagliflozin therapy significantly reduced liver fat compared with standard treatment alone (34).
Table 3 Summary of Japanese clinical and preclinical studies of SGLT-2 inhibitors for type 2 diabetes with or without NAFLD/NASH.
In an observational study in India, it was shown that canagliflozin 100 mg resulted in liver function improvements and reductions in body weight in patients with type 2 diabetes and NAFLD (80). Similarly, ipragliflozin has also been shown to reduce body weight in addition to abdominal fat in a study of ipragliflozin versus pioglitazone, although both drugs showed equal benefits for NAFLD and glycemic control (42). However, a more recent single-arm prospective trial has reported that luseogliflozin 2.5 mg/day significantly reduced HbA1c, transaminases, and hepatic fat content after 24 weeks of treatment (27). This supports previous results observed during a randomized pilot study of luseogliflozin 2.5 mg/day, which resulted in significantly larger reductions in fat liver deposition compared with metformin 1500 mg/day (79). Finally, a study of NAFLD patients with type 2 diabetes who did not respond to incretin-based therapy showed that SGLT-2 inhibitor treatment resulted in good glycemic control, reduced body weight, normalized ALT levels, and a reduced Fibrosis-4 index (78). This was followed by a single-arm, exploratory study of canagliflozin, where it was reported that serum ALT levels decreased, and several markers of hepatic function and fibrosis also improved (74).
One of the main advantages of SGLT-2 inhibitor monotherapy is the ability of a single drug to provide better glycemic control and reduce insulin resistance whilst also improving NAFLD features and reducing body weight. SGLT-2 inhibition occurs independently from β-cell function and secretion of insulin; thus, these agents can be useful for glycemic control for patients with longstanding diabetes with or without preserved renal function (82). Moreover, treatment with SGLT-2 inhibitors has been reported to be well-tolerated, with a low risk of hypoglycemia (on par with the risks posed by metformin and DPP-4 inhibitors) (83). Indeed, a fairly recent meta-analysis evaluated the benefits and harms of SGLT-2 inhibitors and concluded that the risk of adverse effects while receiving treatment with these agents was comparable to that associated with placebo treatment (84).
However, SGLT-2 inhibition monotherapy has some limitations. One important limitation is that these drugs appear to be more effective in younger individuals with a shorter duration of diabetes and higher baseline BMI, fasting glucose level, and HbA1c level (83, 85). Additionally, many of the relevant studies of SGLT-2 inhibitors have been conducted among Japanese patients, and as such, there is a clear need for studies in other populations. Previously, SGLT−2 inhibition was contraindicated for patients with impaired renal function (estimated glomerular filtration rate [eGFR] <45 mL/min/1.73 m2), and thus, this treatment was limited to patients with diabetes and preserved kidney function (83). However, given recent favorable effects in major clinical trials (e.g., CANVAS, CREDENCE, DAPA-CKD) (86–88), both canagliflozin and dapagliflozin have been approved by the FDA for use in patients with chronic kidney disease. Empagliflozin was also approved by the FDA for use in patients with heart failure (regardless of diabetes status) and chronic kidney disease (eGFR ≥20 to < 45 mL/min/1.73 m2), on the basis of findings from the EMPA-KIDNEY trial (82). Moreover, accumulating evidence supports a strong relationship between NAFLD and diabetic nephropathy.
The most frequently reported adverse effects of SGLT-2 inhibitors are mycotic genital infections, which are attributable to the presence of glucosuria and the resulting proliferation of pathogens. Urinary tract infections and volume depletion effects are reportedly rare, and these effects are also related to glucosuria, which can lead to osmotic diuresis (83). Concerns have been raised regarding the risk of euglycemic ketoacidosis, bone fracture, and foot and leg amputation (89–91). Specific caution is warranted when prescribing SGLT-2 inhibitors to frail elderly patients aged >75 years, particularly those with chronic kidney disease and those using loop diuretics (89), as the concomitant use of loop diuretics and SGLT-2 inhibitors can increase the risk of volume depletion (92).
Overall, however, the existing literature implies that the benefits of SGLT-2 inhibitors in patients with type 2 diabetes and NASH far outweigh the potential adverse effects. Combination therapies such as canagliflozin/metformin, dapagliflozin/metformin, empagliflozin/metformin, and empagliflozin/linagliptin, which may also be useful in this patient population, have been approved in several countries. The complementary therapeutic advantages include beneficial metabolic effects that result from the SGLT-2 inhibitor and improvements in insulin sensitivity provided by metformin. However, it is important to recognize that some patients may be more susceptible to adverse events associated with these combination therapies. It is also plausible that combining an SGLT-2 inhibitor with pioglitazone could be a useful approach with favorable effects on glucose homeostasis, insulin sensitivity, and bodyweight. Furthermore, this combination therapy could reduce adverse effects, as the diuretic effect of SGLT-2 inhibitors would counteract the fluid retention caused by the thiazolidinediones. Finally, it has also been proposed that SGLT-2 inhibitors could be combined with a GLP-1 receptor agonist (93) or a DPP-4 inhibitor (38), resulting in additive effects due to these drugs’ different mechanisms of action (48). Treatment for diabetes and NASH with a combination of pioglitazone and either a GLP-1 receptor agonist or SGLT-2 inhibitor may be a cost-effective strategy while lowering the increased cardiovascular risks in this patient population (94).
A recently conducted meta-analysis of eight studies (214 patients in total) of patients receiving SGLT-2 inhibitors for type 2 diabetes mellitus and NAFLD (43) concluded that despite the low-to-moderate quality of evidence from the available pilot and observational studies, SGLT-2 inhibitors appear to improve transaminase levels, fatty liver, and fibrosis, along with providing additional metabolic effects, with the most common adverse effects being genitourinary tract infections. Another recent meta-analysis of 29 randomized controlled trials (2,617 patients in total) focused on treating NAFLD with antihyperglycemic drugs in patients with or without diabetes (70). Of the 29 reviewed studies, seven involved the administration of SGLT-2 inhibitors, whereas the other studies featured treatment with metformin, glitazones, GLP-1 receptor agonists, and DPP-4 inhibitors. Although most randomized controlled trials had adequate liver histological endpoints, most of the efficacy data produced in patients with NASH were for pioglitazone. Both systematic reviews (43, 70) suggest that further research is needed, particularly in the form of large, prospective, phase 3, randomized controlled trials, with multi-racial/multi-ethnic populations and widely accepted histologic endpoints. Nevertheless, many of the preliminary studies conducted to date have reported encouraging results, and it is hoped that future studies of SGLT-2 inhibitors in patients with type 2 diabetes and NAFLD can provide robust evidence to confirm their therapeutic potential within this population.
At present, the exact pathogenic mechanisms of NAFLD and its progression to NASH in the presence or absence of diabetes remain unclear. To design optimal treatment strategies, it is necessary to fully understand the underlying pathogenesis of this dual condition. Moreover, the mechanisms of action of SGLT-2 inhibitors also need to be elucidated. This could be achieved by studies targeting molecular pathways that potentially mediate the therapeutic effects of SGLT-2 inhibitors and by leveraging state-of-the-art approaches, such as transcriptomics, lipidomics, and metabolomics. Pharmacogenomics testing may be beneficial in distinguishing extensive from poor metabolizers of SGLT-2 inhibitors, enabling the individualization of dosing for optimal therapeutic responses. However, this will require the identification of the metabolic enzymes that biotransform this drug class.
The main issues emerging from the two systematic reviews undertaken to date (43, 70) were that existing clinical studies varied considerably in design, interventions, and follow-up periods. Furthermore, most studies were conducted in single racial groups or limited geographic areas. Further research is needed to validate the reported benefits of SGLT-2 inhibitors in treating NAFLD and NASH. Therefore, future study designs should be prospective and allow comparisons of the long-term efficacy and safety profiles of the seven available SGLT-2 inhibitors. Samples should be adequately powered to provide clinically and statistically meaningful results, racially diverse, and include elderly (≥65–74 years) and very elderly (≥75 years) patients. In addition, future studies should evaluate appropriate and validated histologic outcomes as well as surrogate markers and imaging indices.
This review has highlighted the challenges faced in the treatment of patients with concurrent type 2 diabetes mellitus and NAFLD or NASH, including the impact of comorbidities, lack of efficacy, and long-term safety issues. An assessment of the published literature suggests that SGLT-2 inhibitor monotherapy offers a promising option for the treatment of concurrent type 2 diabetes mellitus and NAFLD or NASH. Preliminary data show that this treatment is effective and well-tolerated in the populations studied, which mainly comprised Japanese patients. However, the available studies had several limitations that need to be addressed to solidify these findings. Combination therapies with SGLT-2 inhibitors warrant further study but may provide a feasible alternative for patients who are unresponsive to monotherapy or treatment with other drug classes. Moreover, the pharmacotherapy of patients with concurrent type 2 diabetes mellitus and NAFLD or NASH should be tailored to individual needs and requires a multidisciplinary approach. Further non-invasive diagnostic and follow-up measures are needed to improve the clinical management of this dual condition and optimize drug therapy. Given the incompletely understood pathogeneses of NAFLD and NASH, it is difficult to identify specific therapeutic targets. This issue needs to be addressed to enable optimization of treatment and outcomes in this rapidly growing patient population.
The author confirms being the sole contributor of this work and has approved it for publication.
The author declares that the research was conducted in the absence of any commercial or financial relationships that could be construed as a potential conflict of interest.
All claims expressed in this article are solely those of the authors and do not necessarily represent those of their affiliated organizations, or those of the publisher, the editors and the reviewers. Any product that may be evaluated in this article, or claim that may be made by its manufacturer, is not guaranteed or endorsed by the publisher.
The authors wish to thank Keyra Martinez Dunn, MD of Edanz Inc., for providing medical writing support.
1. Tomah S, Alkhouri N, Hamdy O. Nonalcoholic Fatty Liver Disease and Type 2 Diabetes: Where do Diabetologists Stand? Clin Diabetes Endocrinol (2020) 6:9. doi: 10.1186/s40842-020-00097-1
2. Younossi ZM, Golabi P, de Avila L, Paik JM, Srishord M, Fukui N, et al. The Global Epidemiology of NAFLD and NASH in Patients With Type 2 Diabetes: A Systematic Review and Meta-Analysis. J Hepatol (2019) 71:793–801. doi: 10.1016/j.jhep.2019.06.021
3. Eguchi Y, Hyogo H, Ono M, Mizuta T, Ono N, Fujimoto K, et al. Prevalence and Associated Metabolic Factors of Non-Alcoholic Fatty Liver Disease in the General Population From 2009 to 2010 in Japan: A Multicenter Large Retrospective Study. J Gastroenterol (2012) 47:586–95. doi: 10.1007/s00535-012-0533-z
4. Bellentani S. The Epidemiology of Non-Alcoholic Fatty Liver Disease. Liver Int (2017) 37 Suppl 1:81–4. doi: 10.1111/liv.13299
5. Younossi ZM, Koenig AB, Abdelatif D, Fazel Y, Henry L, Wymer M. Global Epidemiology of Non-Alcoholic Fatty Liver Disease - Meta-Analytic Assessment of Prevalence, Incidence, and Outcomes. Hepatology (2016) 64:73–84. doi: 10.1002/hep.28431
6. Byrne CD, Targher G. Nafld: A Multisystem Disease. J Hepatol (2015) 62(1 Suppl):S47–64. doi: 10.1016/j.jhep.2014.12.012
7. Chalasani N, Younossi Z, Lavine JE, Charlton M, Cusi K, Rinella M, et al. The Diagnosis and Management of Non-Alcoholic Fatty Liver Disease: Practice Guidance From the American Association for the Study of Liver Diseases. Hepatology (2018) 67:328–57. doi: 10.1002/hep.29367
8. Stengel JZ, Harrison SA. Nonalcoholic Steatohepatitis: Clinical Presentation, Diagnosis, and Treatment. Gastroenterol Hepatol (N Y) (2006) 2:440–9.
9. Basaranoglu M, Neuschwander-Tetri BA. Nonalcoholic Fatty Liver Disease: Clinical Features and Pathogenesis. Gastroenterol Hepatol (N Y) (2006) 2:282–91.
10. Day CP, James OF. Steatohepatitis: A Tale of Two “Hits”? Gastroenterology (1998) 114:842–5. doi: 10.1016/s0016-5085(98)70599-2
11. Alkhouri N, McCullough AJ. Noninvasive Diagnosis of NASH and Liver Fibrosis Within the Spectrum of NAFLD. Gastroenterol Hepatol (N Y) (2012) 8:661–8.
12. Petta S, Valenti L, Bugianesi E, Targher G, Bellentani S. Bonino F. A “Systems Medicine” Approach to the Study of Non-Alcoholic Fatty Liver Disease. Dig Liver Dis (2016) 48:333–42. doi: 10.1016/j.dld.2015.10.027
13. Mu W, Cheng XF, Liu Y, Lv QZ, Liu GL, Zhang JG, et al. Potential Nexus of Non-Alcoholic Fatty Liver Disease and Type 2 Diabetes Mellitus: Insulin Resistance Between Hepatic and Peripheral Tissues. Front Pharmacol (2019) 9:1566. doi: 10.3389/fphar.2018.01566
14. Gastaldelli A, Cusi K. From NASH to Diabetes and From Diabetes to NASH: Mechanisms and Treatment Options. JHEP Rep (2019) 1:312–8. doi: 10.1016/j.jhepr.2019.07.002
15. Saponaro C, Gaggini M, Carli F, Gastaldelli A. The Subtle Balance Between Lipolysis and Lipogenesis: A Critical Point in Metabolic Homeostasis. Nutrients (2015) 7:9453–74. doi: 10.3390/nu7115475
16. Abdul-Wahed A, Guilmeau S, Postic C. Sweet Sixteenth for Chrebp: Established Roles and Future Goals. Cell Metab (2017) 26:324–41. doi: 10.1016/j.cmet.2017.07.004
17. Cagen LM, Deng X, Wilcox HG, Park EA, Raghow R, Elam MB. Insulin Activates the Rat Sterol-Regulatory-Element-Binding Protein 1c (SREBP-1c) Promoter Through the Combinatorial Actions of SREBP, LXR, Sp-1 and NF-Y Cis-Acting Elements. Biochem J (2005) 385(Pt 1):207–16. doi: 10.1042/BJ20040162
18. Moslehi A, Hamidi-Zad Z. Role of Srebps in Liver Diseases: A Mini-Review. J Clin Transl Hepatol (2018) 6:332–8. doi: 10.14218/JCTH.2017.00061
19. Deng X, Cagen LM, Wilcox HG, Park EA, Raghow R, Elam MB. Regulation of the Rat SREBP-1c Promoter in Primary Rat Hepatocytes. Biochem Biophys Res Commun (2002) 290:256–62. doi: 10.1006/bbrc.2001.6148
20. Yellaturu CR, Deng X, Park EA, Raghow R, Elam MB. Insulin Enhances the Biogenesis of Nuclear Sterol Regulatory Element-Binding Protein (SREBP)-1c by Post-Transcriptional Down-Regulation of Insig-2A and its Dissociation From SREBP Cleavage-Activating Protein (SCAP).SREBP-1c Complex. J Biol Chem (2009) 284:31726–34. doi: 10.1074/jbc.M109.050914
21. Čolak E, Pap D. The Role of Oxidative Stress in the Development of Obesity and Obesity-Related Metabolic Disorders. J Med Biochem (2021) 40:1–9. doi: 10.5937/jomb0-24652
22. Hazlehurst JM, Woods C, Marjot T, Cobbold JF, Tomlinson JW. Non-Alcoholic Fatty Liver Disease and Diabetes. Metabolism (2016) 65:1096–108. doi: 10.1016/j.metabol.2016.01.001
23. Kim JA, Choi KM. Newly Discovered Adipokines: Pathophysiological Link Between Obesity and Cardiometabolic Disorders. Front Physiol (2020) 11:568800. doi: 10.3389/fphys.2020.568800
24. Saxena NK, Titus MA, Ding X, Floyd J, Srinivasan S, Sitaraman SV, et al. Leptin as a Novel Profibrogenic Cytokine in Hepatic Stellate Cells: Mitogenesis and Inhibition of Apoptosis Mediated by Extracellular Regulated Kinase (Erk) and Akt Phosphorylation. FASEB J (2004) 18:1612–4. doi: 10.1096/fj.04-1847fje
25. Kantartzis K, Peter A, Machicao F, Machann J, Wagner S, Königsrainer I, et al. Dissociation Between Fatty Liver and Insulin Resistance in Rumans Carrying a Variant of the Patatin-Like Phospholipase 3 Gene. Diabetes (2009) 58:2616–23. doi: 10.2337/db09-0279
26. Musso G, Gambino R, Pacini G, Pagano G, Durazzo M, Cassader M. Transcription Factor 7-Like 2 Polymorphism Modulates Glucose and Lipid Homeostasis, Adipokine Profile, and Hepatocyte Apoptosis in NASH. Hepatology (2009) 49:426–35. doi: 10.1002/hep.22659
27. Sumida Y, Murotani K, Saito M, Tamasawa A, Osonoi Y, Yoneda M, et al. Effect of Luseogliflozin on Hepatic Fat Content in Type 2 Diabetes Patients With Non-Alcoholic Fatty Liver Disease: A Prospective, Single-Arm Trial (LEAD Trial). Hepatol Res (2019) 49:64–71. doi: 10.1111/hepr.13236
28. Williams KH, Shackel NA, Gorrell MD, McLennan SV, Twigg SM. Diabetes and Non-Alcoholic Fatty Liver Disease: A Pathogenic Duo. Endocrine Rev (2013) 34:84–129. doi: 10.1210/er.2012-1009
29. Marchesini G, Bugianesi E, Forlani G, Cerrelli F, Lenzi M, Manini R, et al. Non-Alcoholic Fatty Liver, Steatohepatitis, and the Metabolic Syndrome. Hepatology (2003) 37:917–23. doi: 10.1053/jhep.2003.50161
30. Kanwar P, Nelson JE, Yates K, Kleiner DE, Unalp-Arida A, Kowdley KV. Association Between Metabolic Syndrome and Liver Histology Among NAFLD Patients Without Diabetes. BMJ Open Gastroenterol (2016) 3:e000114. doi: 10.1136/bmjgast-2016-000114
31. Vrhovac I, Balen Eror D, Klessen D, Burger C, Breljak D, Kraus O, et al. Localizations of Na(+)-D-Glucose Cotransporters SGLT1 and SGLT2 in Human Kidney and of SGLT1 in Human Small Intestine, Liver, Lung, and Heart. Pflugers Arch (2015) 467:1881–98. doi: 10.1007/s00424-014-1619-7
32. Wright EM, Loo DD, Hirayama BA. Biology of Human Sodium Glucose Transporters. Physiol Rev (2011) 91:733–94. doi: 10.1152/physrev.00055.2009
33. Simes BC, MacGregor GG. Sodium-Glucose Cotransporter-2 (Sglt2) Inhibitors: A Clinician’s Guide. Diabetes Metab Syndr Obes (2019) 12:2125–36. doi: 10.2147/dmso.S212003
34. Kuchay MS, Krishan S, Mishra SK, Farooqui KJ, Singh MK, Wasir JS, et al. Effect of Empagliflozin on Liver Fat in Patients With Type 2 Diabetes and Non-Alcoholic Fatty Liver Disease: A Randomized Controlled Trial (E-LIFT Trial). Diabetes Care (2018) 41:1801–8. doi: 10.2337/dc18-0165
35. Mudaliar S, Polidori D, Zambrowicz B, Henry RR. Sodium-Glucose Cotransporter Inhibitors: Effects on Renal and Intestinal Glucose Transport: From Bench to Bedside. Diabetes Care (2015) 38:2344–53. doi: 10.2337/dc15-0642
36. Neal B, Perkovic V, Mahaffey KW, de Zeeuw D, Fulcher G, Erondu N, et al. Canagliflozin and Cardiovascular and Renal Events in Type 2 Diabetes. N Engl J Med (2017) 377:644–57. doi: 10.1056/NEJMoa1611925
37. Zinman B, Lachin JM, Inzucchi SE. Empagliflozin, Cardiovascular Outcomes, and Mortality in Type 2 Diabetes. N Engl J Med (2016) 374:1094. doi: 10.1056/NEJMc1600827
38. Jojima T, Tomotsune T, Iijima T, Akimoto K, Suzuki K, Aso Y. Empagliflozin (an SGLT2 Inhibitor), Alone or in Combination With Linagliptin (a DPP-4 Inhibitor), Prevents Steatohepatitis in a Novel Mouse Model of Non-Alcoholic Steatohepatitis and Diabetes. Diabetol Metab Syndr (2016) 8:45. doi: 10.1186/s13098-016-0169-x
39. Nakano S, Katsuno K, Isaji M, Nagasawa T, Buehrer B, Walker S, et al. Remogliflozin Etabonate Improves Fatty Liver Disease in Diet-Induced Obese Male Mice. J Clin Exp Hepatol (2015) 5:190–8. doi: 10.1016/j.jceh.2015.02.005
40. Yabiku K, Nakamoto K, Tsubakimoto M. Effects of Sodium-Glucose Cotransporter 2 Inhibition on Glucose Metabolism, Liver Function, Ascites, and Hemodynamics in a Mouse Model of Nonalcoholic Steatohepatitis and Type 2 Diabetes. J Diabetes Res (2020) 2020:1682904. doi: 10.1155/2020/1682904
41. Eriksson JW, Lundkvist P, Jansson PA, Johansson L, Kvarnström M, Moris L, et al. Effects of Dapagliflozin and N-3 Carboxylic Acids on Non-Alcoholic Fatty Liver Disease in People With Type 2 Diabetes: A Double-Blind Randomised Placebo-Controlled Study. Diabetologia (2018) 61:1923–34. doi: 10.1007/s00125-018-4675-2
42. Ito D, Shimizu S, Inoue K, Saito D, Yanagisawa M, Inukai K, et al. Comparison of Ipragliflozin and Pioglitazone Effects on Nonalcoholic Fatty Liver Disease in Patients With Type 2 Diabetes: A Randomized, 24-Week, Open-Label, Active-Controlled Trial. Diabetes Care (2017) 40:1364–72. doi: 10.2337/dc17-0518
43. Raj H, Durgia H, Palui R, Kamalanathan S, Selvarajan S, Kar SS, et al. SGLT-2 Inhibitors in Non-Alcoholic Fatty Liver Disease Patients With Type 2 Diabetes Mellitus: A Systematic Review. World J Diabetes (2019) 10:114–32. doi: 10.4239/wjd.v10.i2.114
44. Seino Y, Sasaki T, Fukatsu A, Ubukata M, Sakai S, Samukawa Y. Efficacy and Safety of Luseogliflozin as Monotherapy in Japanese Patients With Type 2 Diabetes Mellitus: A Randomized, Double-Blind, Placebo-Controlled, Phase 3 Study. Curr Med Res Opin (2014) 30:1245–55. doi: 10.1185/03007995.2014.912983
45. Takeda A, Irahara A, Nakano A, Takata E, Koketsu Y, Kimata K, et al. The Improvement of the Hepatic Histological Findings in a Patient With Non-Alcoholic Steatohepatitis With Type 2 Diabetes After the Administration of the Sodium-Glucose Cotransporter 2 Inhibitor Ipragliflozin. Intern Med (2017) 56(20):2739–44. doi: 10.2169/internalmedicine.8754-16
46. Tanase DM, Gosav EM, Costea CF, Ciocoiu M, Lacatusu CM, Maranduca MA, et al. The Intricate Relationship Between Type 2 Diabetes Mellitus (T2DM), Insulin Resistance (IR), and Nonalcoholic Fatty Liver Disease (NAFLD). J Diabetes Res (2020) 2020:3920196. doi: 10.1155/2020/3920196
47. Budd J, Cusi K. Role of Agents for the Treatment of Diabetes in the Management of Nonalcoholic Fatty Liver Disease. Curr Diabetes Rep (2020) 20:59. doi: 10.1007/s11892-020-01349-1
48. Gharaibeh NE, Rahhal MN, Rahimi L, Ismail-Beigi F. SGLT-2 Inhibitors as Promising Therapeutics for Non-Alcoholic Fatty Liver Disease: Pathophysiology, Clinical Outcomes, and Future Directions. Diabetes Metab Syndr Obes (2019) 12:1001–12. doi: 10.2147/dmso.S212715
49. Dos Santos Coelho F, Borges-Canha M, von Hafe M, Neves JS, Vale C, Leite AR, et al. Effects of Sodium-Glucose Co-Transporter 2 Inhibitors on Liver Parameters and Steatosis: A Meta-Analysis of Randomized Clinical Trials. Diabetes Metab Res Rev (2021) 37:e3413. doi: 10.1002/dmrr.3413
50. DeFronzo RA. Combination Therapy With GLP-1 Receptor Agonist and SGLT2 Inhibitor. Diabetes Obes Metab (2017) 19:1353–62. doi: 10.1111/dom.12982
51. Drucker DJ. The Cardiovascular Biology of Glucagon-Like Peptide-1. Cell Metab (2016) 24:15–30. doi: 10.1016/j.cmet.2016.06.009
52. Svegliati-Baroni G, Saccomanno S, Rychlicki C, Agostinelli L, De Minicis S, Candelaresi C, et al. Glucagon-Like Peptide-1 Receptor Activation Stimulates Hepatic Lipid Oxidation and Restores Hepatic Signalling Alteration Induced by a High-Fat Diet in Nonalcoholic Steatohepatitis. Liver Int (2011) 31:1285–97. doi: 10.1111/j.1478-3231.2011.02462.x
53. Carbone LJ, Angus PW, Yeomans ND. Incretin-Based Therapies for the Treatment of Non-Alcoholic Fatty Liver Disease: A Systematic Review and Meta-Analysis. J Gastroenterol Hepatol (2016) 31:23–31. doi: 10.1111/jgh.13026
54. Liss KH, Finck BN. Ppars and Non-Alcoholic Fatty Liver Disease. Biochimie (2017) 136:65–74. doi: 10.1016/j.biochi.2016.11.009
55. Zhu J, Yu X, Zheng Y, Li J, Wang Y, Lin Y, et al. Association of Glucose-Lowering Medications With Cardiovascular Outcomes: An Umbrella Review and Evidence Map. Lancet Diabetes Endocrinol (2020) 8:192–205. doi: 10.1016/S2213-8587(19)30422-X
56. Nesti L, Tricò D, Mengozzi A, Natali A. Rethinking Pioglitazone as a Cardioprotective Agent: A New Perspective on an Overlooked Drug. Cardiovasc Diabetol (2021) 20:109. doi: 10.1186/s12933-021-01294-7
57. Alam F, Islam MA, Mohamed M, Ahmad I, Kamal MA, Donnelly R, et al. Efficacy and Safety of Pioglitazone Monotherapy in Type 2 Diabetes Mellitus: A Systematic Review and Meta-Analysis of Randomized Controlled Trials. Sci Rep (2019) 9:5389. doi: 10.1038/s41598-019-41854-2
58. Yoneda M, Honda Y, Ogawa Y, Kessoku T, Kobayashi T, Imajo K, et al. Comparing the Effects of Tofogliflozin and Pioglitazone in Non-Alcoholic Fatty Liver Disease Patients With Type 2 Diabetes Mellitus (Topind Study): A Randomized Prospective Open-Label Controlled Trial. BMJ Open Diabetes Res Care (2021) 9:e001990. doi: 10.1136/bmjdrc-2020-001990
59. Tahara A, Takasu T. SGLT2 Inhibitor Ipragliflozin Alone and Combined With Pioglitazone Prevents Progression of Non-Alcoholic Steatohepatitis in a Type 2 Diabetes Rodent Model. Physiol Rep (2019) 7:e14286. doi: 10.14814/phy2.14286
60. Oseini AM, Sanyal AJ. Therapies in Non-Alcoholic Steatohepatitis (NASH). Liver Int (2017) 37 Suppl 1:97–103. doi: 10.1111/liv.13302
61. Finotti M, Romano M, Auricchio P, Scopelliti M, Brizzolari M, Grossi U, et al. Target Therapies for NASH/NAFLD: From the Molecular Aspect to the Pharmacological and Surgical Alternatives. J Pers Med (2021) 11:499. doi: 10.3390/jpm11060499
62. Fraile JM, Palliyil S, Barelle C, Porter AJ, Kovaleva M. Non-Alcoholic Steatohepatitis (NASH) - A Review of a Crowded Clinical Landscape, Driven by a Complex Disease. Drug Des Devel Ther (2021) 15:3997–4009. doi: 10.2147/DDDT.S315724
63. Gerber L, Otgonsuren M, Mishra A, Escheik C, Birerdinc A, Stepanova M, et al. Non-Alcoholic Fatty Liver Disease (NAFLD) Is Associated With Low Level of Physical Activity: A Population-Based Study. Aliment Pharmacol Ther (2012) 36:772–81. doi: 10.1111/apt.12038
64. Musso G, Cassader M, Rosina F, Gambino R. Impact of Current Treatments on Liver Disease, Glucose Metabolism and Cardiovascular Risk in Non-Alcoholic Fatty Liver Disease (NAFLD): A Systematic Review and Meta-Analysis of Randomised Trials. Diabetologia (2012) 55:885–904. doi: 10.1007/s00125-011-2446-4
65. Povsic M, Oliver L, Jiandani NR, Perry R. Bottomley J. A Structured Literature Review of Interventions Used in the Management of Non-Alcoholic Steatohepatitis (NASH). Pharmacol Res Perspect (2019) 7:e00485. doi: 10.1002/prp2.485
66. Khneizer G, Rizvi S, Gawrieh S. Non-Alcoholic Fatty Liver Disease and Diabetes Mellitus. Adv Exp Med Biol (2021) 1307:417–40. doi: 10.1007/5584_2020_532
67. Blazina I, Selph S. Diabetes Drugs for Non-Alcoholic Fatty Liver Disease: A Systematic Review. Syst Rev (2019) 8:295. doi: 10.1186/s13643-019-1200-8
68. Yabiku K, Mutoh A, Miyagi K, Takasu N. Effects of Oral Antidiabetic Drugs on Changes in the Liver-to-Spleen Ratio on Computed Tomography and Inflammatory Biomarkers in Patients With Type 2 Diabetes and Nonalcoholic Fatty Liver Disease. Clin Ther (2017) 39:558–66. doi: 10.1016/j.clinthera.2017.01.015
69. Shah P, Mudaliar S. Pioglitazone: Side Effect and Safety Profile. Expert Opin Drug Saf (2010) 9:347–54. doi: 10.1517/14740331003623218
70. Mantovani A, Petracca G, Beatrice G, Csermely A, Lonardo A, Targher G. Glucagon-Like Peptide-1 Receptor Agonists for Treatment of Nonalcoholic Fatty Liver Disease and Nonalcoholic Steatohepatitis: An Updated Meta-Analysis of Randomized Controlled Trials. Metabolites (2021) 11:73. doi: 10.3390/metabo11020073
71. Takahashi Y, Sugimoto K, Inui H, Fukusato T. Current Pharmacological Therapies for Non-Alcoholic Fatty Liver Disease/Non-Alcoholic Steatohepatitis. World J Gastroenterol (2015) 21:3777–85. doi: 10.3748/wjg.v21.i13.3777
72. Ito H, Shinozaki M, Nishio S, Abe M. SGLT2 Inhibitors in the Pipeline for the Treatment of Diabetes Mellitus in Japan. Expert Opin Pharmacother (2016) 17:2073–84. doi: 10.1080/14656566.2016.1232395
73. Bonora BM, Avogaro A, Fadini GP. Extraglycemic Effects of SGLT2 Inhibitors: A Review of the Evidence. Diabetes Metab Syndr Obes (2020) 13:161–74. doi: 10.2147/dmso.S233538
74. Seko Y, Sumida Y, Tanaka S, Mori K, Taketani H, Ishiba H, et al. Effect of Sodium Glucose Cotransporter 2 Inhibitor on Liver Function Tests in Japanese Patients With Non-Alcoholic Fatty Liver Disease and Type 2 Diabetes Mellitus. Hepatol Res (2017) 47:1072–8. doi: 10.1111/hepr.12834
75. Komiya C, Tsuchiya K, Shiba K, Miyachi Y, Furuke S, Shimazu N, et al. Ipragliflozin Improves Hepatic Steatosis in Obese Mice and Liver Dysfunction in Type 2 Diabetic Patients Irrespective of Body Weight Reduction. PloS One (2016) 11:e0151511. doi: 10.1371/journal.pone.0151511
76. Takase T, Nakamura A, Miyoshi H, Yamamoto C, Atsumi T. Amelioration of Fatty Liver Index in Patients With Type 2 Diabetes on Ipragliflozin: An Association With Glucose-Lowering Effects. Endocr J (2017) 64:363–7. doi: 10.1507/endocrj.EJ16-0295
77. Sumida Y, Yoneda M, Tokushige K, Kawanaka M, Fujii H, Yoneda M, et al. Antidiabetic Therapy in the Treatment of Nonalcoholic Steatohepatitis. Int J Mol Sci (2020) 21:1907. doi: 10.3390/ijms21061907
78. Ohki T, Isogawa A, Toda N, Tagawa K. Effectiveness of Ipragliflozin, a Sodium-Glucose Co-Transporter 2 Inhibitor, as a Second-Line Treatment for Non-Alcoholic Fatty Liver Disease Patients With Type 2 Diabetes Mellitus Who do Not Respond to Incretin-Based Therapies Including Glucagon-Like Peptide-1 Analogs and Dipeptidyl Peptidase-4 Inhibitors. Clin Drug Investig (2016) 36:313–9. doi: 10.1007/s40261-016-0383-1
79. Shibuya T, Fushimi N, Kawai M, Yoshida Y, Hachiya H, Ito S, et al. Luseogliflozin Improves Liver Fat Deposition Compared to Metformin in Type 2 Diabetes Patients With Non-Alcoholic Fatty Liver Disease: A Prospective Randomized Controlled Pilot Study. Diabetes Obes Metab (2018) 20:438–42. doi: 10.1111/dom.13061
80. Gautam A, Agrawal PK, Doneria J, Nigam A. Effects of Canagliflozin on Abnormal Liver Function Tests in Patients of Type 2 Diabetes With Non-Alcoholic Fatty Liver Disease. J Assoc Physicians India (2018) 66:62–6.
81. Yamada T, Murata D, Adachi Y, Itoh K, Kameoka S, Igarashi A, et al. Mitochondrial Stasis Reveals P62-Mediated Ubiquitination in Parkin-Independent Mitophagy and Mitigates Nonalcoholic Fatty Liver Disease. Cell Metab (2018) 28:588–604. doi: 10.1016/j.cmet.2018.06.014
82. Rhee JJ, Jardine MJ, Chertow GM, Mahaffey KW. Dedicated Kidney Disease-Focused Outcome Trials With Sodium-Glucose Cotransporter-2 Inhibitors: Lessons From CREDENCE and Expectations From DAPA-HF, DAPA-CKD, and EMPA-KIDNEY. Diabetes Obes Metab (2020) 22(Suppl 1):46–54. doi: 10.1111/dom.13987
83. Hsia DS, Grove O, Cefalu WT. An Update on Sodium-Glucose Co-Transporter-2 Inhibitors for the Treatment of Diabetes Mellitus. Curr Opin Endocrinol Diabetes Obes (2017) 24:73–9. doi: 10.1097/med.0000000000000311
84. Storgaard H, Gluud LL, Bennett C, Grøndahl MF, Christensen MB, Knop FK, et al. Benefits and Harms of Sodium-Glucose Co-Transporter 2 Inhibitors in Patients With Type 2 Diabetes: A Systematic Review and Meta-Analysis. PloS One (2016) 11:e0166125. doi: 10.1371/journal.pone.0166125
85. Monami M, Nardini C, Mannucci E. Efficacy and Safety of Sodium Glucose Co-Transport-2 Inhibitors in Type 2 Diabetes: A Meta-Analysis of Randomized Clinical Trials. Diabetes Obes Metab (2014) 16:457–66. doi: 10.1111/dom.12244
86. Perkovic V, de Zeeuw D, Mahaffey KW, Fulcher G, Erondu N, Shaw W, et al. Canagliflozin and Renal Outcomes in Type 2 Diabetes: Results From the CANVAS Program Randomized Clinical Trials. Lancet Diabetes Endocrinol (2018) 6:691–704. doi: 10.1016/S2213-8587(18)30141-4
87. Heerspink HJL, Oshima M, Zhang H, Li J, Agarwal R, Capuano G, et al. Canagliflozin and Kidney-Related Adverse Events in Type 2 Diabetes and CKD: Findings From the Randomized CREDENCE Trial. Am J Kidney Dis (2021) S0272–6386(21). doi: 10.1053/j.ajkd.2021.05.005
88. Wheeler DC, Stefánsson BV, Jongs N, Chertow GM, Greene T, Hou FF, et al. DAPA-CKD Trial Committees and Investigators. Effects of Dapagliflozin on Major Adverse Kidney and Cardiovascular Events in Patients With Diabetic and Non-Diabetic Chronic Kidney Disease: A Prespecified Analysis From the DAPA-CKD Trial. Lancet Diabetes Endocrinol (2021) 9:22–31. doi: 10.1016/S2213-8587(20)30369-7
89. Scheen AJ. SGLT2 Inhibition: Efficacy and Safety in Type 2 Diabetes Treatment. Expert Opin Drug Saf (2015) 14:1879–904. doi: 10.1517/14740338.2015.1100167
90. Scheen AJ. Beneficial Effects of SGLT2 Inhibitors on Fatty Liver in Type 2 Diabetes: A Common Comorbidity Associated With Severe Complications. Diabetes Metab (2019) 45:213–23. doi: 10.1016/j.diabet.2019.01.008
91. Singh M, Kumar A. Risks Associated With SGLT2 Inhibitors: An Overview. Curr Drug Saf (2018) 13:84–91. doi: 10.2174/1574886313666180226103408
92. Desouza CV, Gupta N, Patel A. Cardiometabolic Effects of a New Class of Antidiabetic Agents. Clin Ther (2015) 37:1178–94. doi: 10.1016/j.clinthera.2015.02.016
93. Armstrong MJ, Gaunt P, Aithal GP, Barton D, Hull D, Parker R, et al. Liraglutide Safety and Efficacy in Patients With Non-Alcoholic Steatohepatitis (LEAN): A Multicentre, Double-Blind, Randomized, Placebo-Controlled Phase 2 Study. Lancet (2016) 387:679–90. doi: 10.1016/S0140-6736(15)00803-X
Keywords: non-alcoholic fatty liver disease, non-alcoholic steatohepatitis, sodium-glucose cotransporter 2 inhibitors, type 2 diabetes, review
Citation: Yabiku K (2021) Efficacy of Sodium-Glucose Cotransporter 2 Inhibitors in Patients With Concurrent Type 2 Diabetes Mellitus and Non-Alcoholic Steatohepatitis: A Review of the Evidence. Front. Endocrinol. 12:768850. doi: 10.3389/fendo.2021.768850
Received: 01 September 2021; Accepted: 19 November 2021;
Published: 07 December 2021.
Edited by:
Giuseppe Reimondo, University of Turin, ItalyReviewed by:
Roberta Poli, San Luigi Gonzaga University Hospital, ItalyCopyright © 2021 Yabiku. This is an open-access article distributed under the terms of the Creative Commons Attribution License (CC BY). The use, distribution or reproduction in other forums is permitted, provided the original author(s) and the copyright owner(s) are credited and that the original publication in this journal is cited, in accordance with accepted academic practice. No use, distribution or reproduction is permitted which does not comply with these terms.
*Correspondence: Koichi Yabiku, a3lhYmlrdUBtZWQudS1yeXVreXUuYWMuanA=
Disclaimer: All claims expressed in this article are solely those of the authors and do not necessarily represent those of their affiliated organizations, or those of the publisher, the editors and the reviewers. Any product that may be evaluated in this article or claim that may be made by its manufacturer is not guaranteed or endorsed by the publisher.
Research integrity at Frontiers
Learn more about the work of our research integrity team to safeguard the quality of each article we publish.