- 1Department of Neurology, Beijing Tiantan Hospital, Capital Medical University, Beijing, China
- 2Department of Neurology, Tianjin Huanhu Hospital, Tianjin, China
Background: Abnormal orexin-A levels in cerebrospinal fluid (CSF) have been identified in Alzheimer’s disease (AD) and other neurodegenerative diseases. However, few studies have focused on Lewy body disease (LBD) and often with debatable outcomes. Thus, we performed this systematic review and meta-analysis to investigate orexin-A levels in LBD by incorporating data from different studies.
Methods: We gathered studies comparing orexin-A levels in patients with LBD and controls (including healthy controls and other dementia subtypes). In the initial search, 117 relevant articles were identified. After a selection process, seven studies, conducted in Japan, USA, Spain, Switzerland, France, Italy, and Netherlands, were chosen.
Results: In total, 179 patients with LBD and 253 controls were included. Patients with LBD had significantly lower mean orexin-A CSF levels when compared with patients with AD [standard mean difference (SMD): −0.35, 95% confidence interval (CI): −0.70 to −0.00, Z = 1.96, P = 0.05], whereas mean orexin-A levels were significantly higher when compared with patients with frontotemporal lobar degeneration (FTLD) (SMD: 0.61, 95% CI: 0.23–0.99, Z = 3.12, P = 0.002). Orexin-A CSF levels in LBD patients were approximately equal to levels in healthy elderly individuals, whereas they were significantly decreased in LBD patients with excessive daytime sleepiness (EDS) (SMD: -0.15, 95% CI: -0.59 to 0.29, Z = 0.67, P = 0.50).
Conclusions: We showed that orexin-A levels in patients with LBD were not very different from those in normal elderly individuals, whereas they were lower than those in AD patients and higher than those in FTLD patients. The influence of hypersomnia on orexin-A levels should be carefully interpreted.
Systematic Review Registration: https://www.crd.york.ac.uk/prospero/, identifier CRD42021265900.
Introduction
Lewy body disease (LBD) is characterized by the presence of Lewy bodies (LBs) and Lewy neurites, with senile plaque (SP) and neurofibrillary tangle (NFT) deposition (1). LBD comprises a diagnostic spectrum that includes Parkinson’s disease (PD), PD with dementia (PDD), and dementia with Lewy bodies (DLB). Currently, DLB and PDD are differentiated based on the “one-year rule,” which accounts for the relationship between parkinsonism symptoms and dementia.
Sleep disorders, neuropsychiatric symptoms, and parkinsonism symptoms are common clinical characteristics of LBD, given their high prevalence and association with poorer health-related outcomes (2). Although the exact pathophysiology is poorly understood, the orexinergic system has been implicated in some regulatory mechanisms. The hypothalamic neurotransmitter, orexin-A (hypocretin-1), is believed to play a crucial role in the orexinergic system; it contributes to the regulation of the sleep–wake cycle by increasing arousal levels and maintaining wakefulness and maintaining memory and spatial cognition functions (3, 4). Also, orexin-A interacts with multiple areas in the brain stem and other neurotransmitter systems, including dopamine (5).
Neuropathological studies have identified a 25% reduction in hypothalamic hypocretin neurons and LBs in PD patients at early stage I disease and a 62% reduction in advanced PD patients (6). Several clinical studies have investigated orexin-A levels in cerebrospinal fluid (CSF) in patients with LBD; however, study findings and associations with sleep disturbance remain unclear (7). Previous studies indicated that narcolepsy was linked with reduced orexin-A levels (8), and a correlation was identified between neocortical orexin-A levels and hypersomnolence in patients with DLB (9). Orexinergic overexpression may have important roles in the manifestation of rapid-eye-movement sleep behavior disorders (RBDs) in DLB; previous evidence described a significant positive correlation between RBD severity and CSF orexin-A levels (10).
PD is the second most common neurodegenerative disease and performs complex clinical manifestations. PDD can also occur in many patients with PD up to 90% in their long-term, with illness progression (11). The incidence of DLB, which is the second most common neurodegenerative dementia, is approximately 3.5 per 100,000 person-years (12) and accounts for 10%–20% of all dementias (13, 14). The prevalence of sleep disturbance in LBD is 44%–55% (15) and is more prominent than in Alzheimer’s disease (AD) patients. While little research has been conducted on orexin-A levels in LBD, there is ongoing debate in the literature regarding orexin-A associations with LBD clinical manifestations (6, 9, 16, 17).
To address this knowledge gap, we designed this systematic review and meta-analysis to summarize orexin-A levels in CSF in LBD and explore associations between these levels and sleep disturbance. We hypothesized that lower CSF orexin-A levels in LBD could be a reliable biomarker to discriminate between LBD and AD/frontotemporal lobar degeneration (FTLD).
Methods
Data Sources and Literature Searches
This systematic review and meta-analysis were prepared using Preferred Reporting Items for Systematic reviews and Meta-Analyses (PRISMA) guidelines (18). We conducted an electronic literature search using PubMed, Embase, Web of Science, Cochrane library, Wanfang Data, China National Knowledge Infrastructure (CNKI), and China Biology Medicine Disc (CBM) databases from the earliest available date to June 1, 2021. The literature search was conducted using the following search terms/keywords, without limits: (“Lewy body disease” OR “Diffuse Lewy Body Disease” OR “Lewy Body Dementia” OR “Cortical Lewy Body Disease” OR “Lewy Body Disease, Cortical” OR “Lewy Body Type Senile Dementia” OR “Lewy Body Disease, Diffuse” OR “Dementia, Lewy Body”) AND (“Orexin” OR “Hypocretins” OR “Hypocretin” OR “Orexin” OR “Orexin-B” OR “Orexin B” OR “Hypocretin-2” OR “Hypocretin 2” OR “Orexin-A” OR “Orexin A” OR “Hypocretin-1” OR “Hypocretin 1”). The search was not restricted by region, race, or age. In addition, bibliographies in retrieved papers and reviews were manually searched to capture eligible studies. Two authors (JG and ZC) independently performed literature searches and screened all titles, abstracts, and full texts of eligible studies. Differences in opinion were discussed with the corresponding author (YJ) and resolved by mutual consensus.
A flowchart, based on PRISMA guidelines, of search and screening strategies and eligibility criteria is shown (Figure 1).
Inclusion and Exclusion Criteria
Our analyses included studies that met the following criteria: 1) The primary eligibility criterion was a comparison of CSF orexin-A levels in patients with LBD and healthy controls/any other dementia subtypes; 2) Studies that provided a complete definition of participants. The diagnosis of different types of dementia/healthy controls was defined using clinical evaluations and neuropsychological tests: AD was defined by the Diagnostic and Statistical Manual of Mental Disorders, Fourth Edition; the International Statistical Classification of Diseases and Related Health Problems, 10th Revision, criteria of the National Institute of Neurological Communicative Diseases and Stroke, and the Alzheimer’s Disease and Related Disorders Association. PDD was defined by the United Kingdom Parkinson’s Disease Society Brain Bank. DLB was defined by DLB consensus criteria. FTLD was defined by international FTLD consensus criteria; and 3) Orexin-A levels were measured in CSF samples, with sufficient data (pg/dl).
Similarly, studies were excluded if they met any of the following criteria: 1) no available CSF orexin-A data; 2) the publication was a letter, review, meta-analysis, or animal study; and/or 3) the orexin-A detection method differed from the guidelines.
Statistical Analysis
Review Manager 5.3 (Nordic Cochrane Centre, Cochrane Collaboration, Copenhagen, Denmark) was used for statistical analyses and the graphical presentation of data. Since CSF orexin-A levels were continuous, the standard mean difference (SMD) was calculated and used for statistical analyses. Medians and interquartile ranges were converted to means and standard deviation (SD) in accordance with the methodology of Hozo et al. (19).
A fixed-effects model was used for the meta-analysis due to its superior statistical power over the random-effects model. The heterogeneity statistic was I2 < 10%. Statistical heterogeneity among studies was investigated using a χ2 test, with calculated Q statistics, corresponding P values, and I2 statistics. A P > 0.1 or I2 < 50% value indicated no significant heterogeneity among studies. A P < 0.05 or I2 > 75% indicated significant heterogeneity, and a random-effects model was performed.
Publication bias was assessed using funnel plots. Where a distribution was not roughly symmetrical, an increased risk of bias was indicated.
Results
Study Selection and Characteristics
In total, we identified 117 studies. After removing duplicates (n = 40), 77 studies remained. From these, 54 studies were excluded because they were reviews (n = 29), letters (n = 3), conference abstracts (n = 15), experimental animal studies or other disease investigations (n = 7). From the remaining 23 studies, eight were excluded after abstract review, six were excluded after full-text review, and two excluded due to a lack of CSF orexin-A data. The remaining seven articles, with 432 participants, 179 with LBD and 253 controls, were finally included in the meta-analysis (6, 7, 10, 16, 17, 20, 21) (Table 1). Two studies (7, 17) came from the same research team and had the same control group; therefore, we analyzed them together. The results are shown (Figure 2).
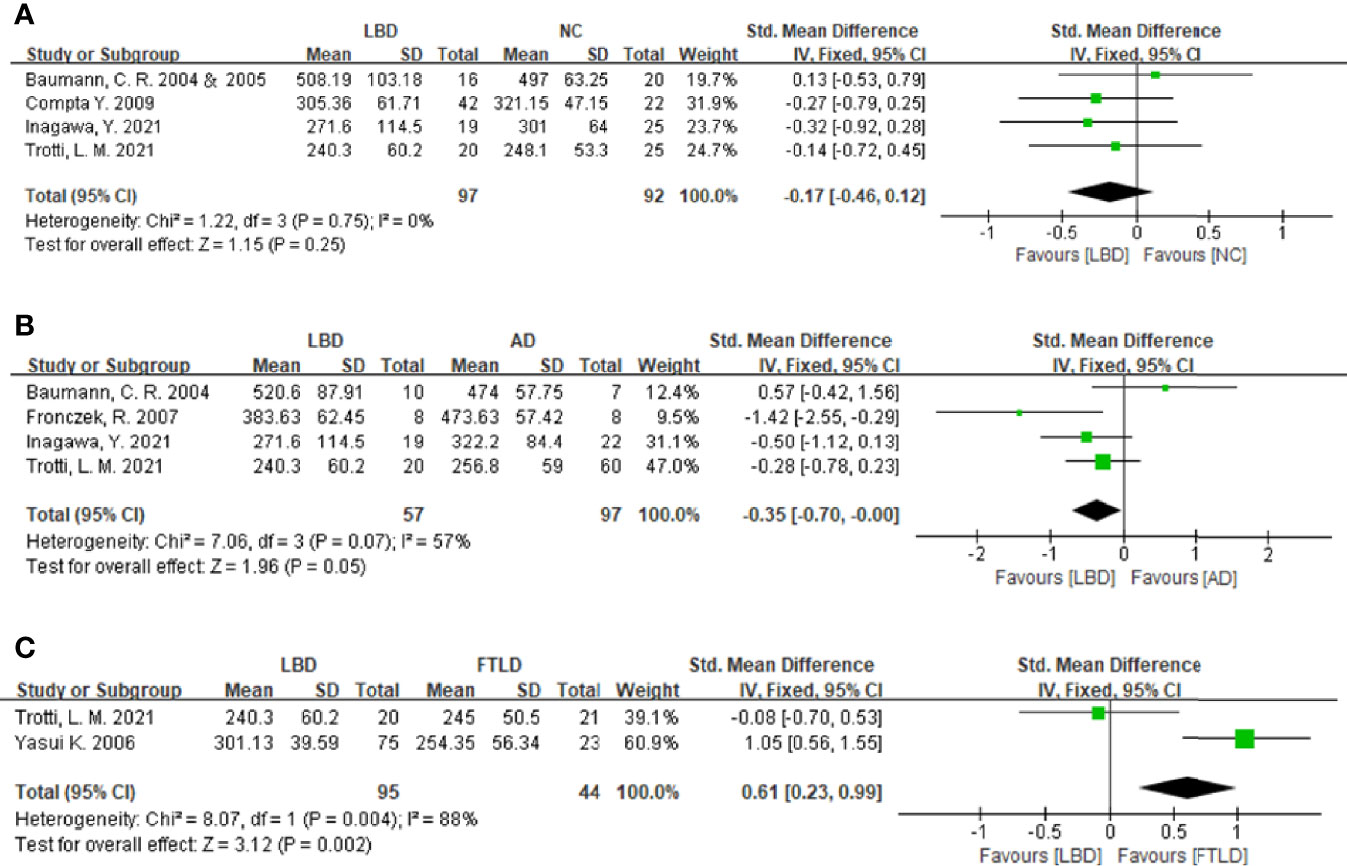
Figure 2 Forest plots of the CSF orexin-A levels in LBD compared with NCs/other diseases. (A) Forest plot of CSF orexin-A levels in LBD compared with NCs. (B) Forest plot of CSF orexin-A levels in LBD compared with AD. (C) Forest plot of CSF orexin-A levels in LBD compared with FTLD. CI, confidence interval; CSF, cerebrospinal fluid; LBD, Lewy body disease; NC, normal controls; AD, Alzheimer’s disease; FTLD, frontotemporal lobar degeneration.
Cerebrospinal Fluid Orexin-A Levels in Lewy Body Disease vs. Normal Controls
To compare CSF orexin-A levels in LBD patients against NCs, five studies ( 7, 10, 16, 17, 20) were included (97 LBD patients vs. 92 NCs). Studies showed low heterogeneity (I2 = 0%, P = 0.75), so a fixed-effects model was used to calculate the pooled SMD. A forest plot demonstrated slightly lower mean CSF orexin-A levels in LBD when compared with NCs; however, this was not statistically significant (SMD: -0.17, 95% CI: −0.46 to 0.12, Z = 1.15, P = 0.25) (Figure 2A).
Cerebrospinal Fluid Orexin-A Levels in Lewy Body Disease vs. Alzheimer’s Disease
Four studies were included in this section of the meta-analysis. LBD and AD patient numbers were 57 and 97, respectively. Study heterogeneity was low (P = 0.07, I2 = 57%), so a fixed-effects model was used to calculate the pooled SMD. A forest plot demonstrated significantly lower mean CSF orexin-A levels in LBD patients when compared with AD patients (SMD: −0.35, 95% CI: −0.70 to −0.00, Z = 1.96, P = 0.05) (Figure 2B).
Cerebrospinal Fluid Orexin-A Levels in Lewy Body Disease vs. Frontotemporal Lobar Degeneration
Sixteen progressive supranuclear palsy (PSP) and 7 corticobasal degeneration (CBD) patients were included as controls in the study by Yasui et al. (21). Since PSP and CBD are untypical FTLD subtypes (22), two studies (20, 21) were included in the meta-analysis. LBD and FTLD patients were 95 and 44, respectively. Study heterogeneity was high (P = 0.004, I2 = 88%). A forest plot demonstrated significantly higher mean CSF orexin-A levels in LBD patients when compared with FTLD patients (SMD: 0.61, 95% CI: 0.23–0.99, Z = 3.12, P = 0.002) (Figure 2C).
Cerebrospinal Fluid Orexin-A Levels in Lewy Body Disease With Excessive Daytime Sleepiness vs. Normal Controls
A subgroup analysis was conducted, and pooled SMD estimates were stratified by EDS. CSF orexin-A levels in LBD patients with EDS were lower than those in NCs but were not statistically significant (SMD: -0.15, 95% CI: -0.59 to 0.29, Z = 0.67, P = 0.50) (Figure 3).

Figure 3 Forest plots of the CSF orexin-A levels in LBD with EDS compared with NCs. CSF, cerebrospinal fluid; LBD, Lewy body disease; NCs, normal controls; EDS, excessive daytime sleepiness; CI, confidence interval.
Publication Bias
Publication bias was assessed using a visual inspection of funnel plots for each analysis. All showed relatively symmetrical distributions indicating a low risk of publication bias (Figures 4A–D).
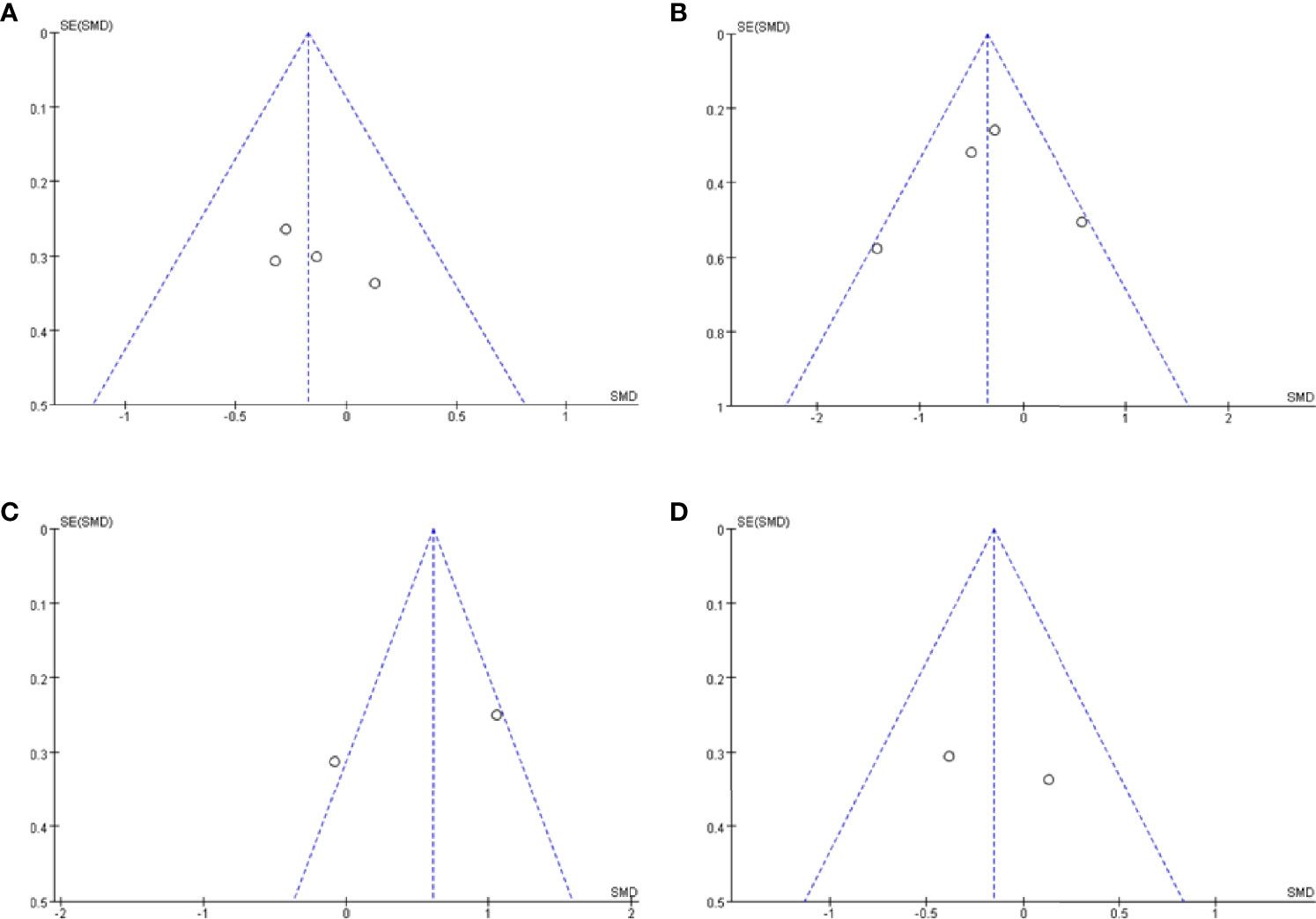
Figure 4 Funnel plot of CSF orexin-A levels in LBD compared with NCs/other diseases. (A) Funnel plot of CSF orexin-A levels in LBD compared with NC. (B) Funnel plot of CSF orexin-A levels in LBD compared with AD. (C) Funnel plot of CSF orexin-A levels in LBD compared with FTLD. (D) Funnel plot of CSF orexin-A levels in LBD with EDS compared with NCs. CSF, cerebrospinal fluid; LBD, Lewy body disease; NCs, normal controls; AD, Alzheimer’s disease; FTLD, frontotemporal lobar degeneration; EDS, excessive daytime sleepiness; SMD, standardized mean difference.
Discussion
We demonstrated the potential role of CSF orexin-A levels in the differential diagnosis of LBD and other diseases. In this meta-analysis, mean CSF orexin-A levels were significantly lower in LBD patients when compared with those in AD patients and higher when compared with those in FTLD patients. Mean CSF orexin-A levels in LBD patients were lower than NCs but lacked statistical significance. Thus, CSF orexin-A levels could be used as a reliable biomarker to discriminate between LBD and AD/FTLD and be used to support clinical and neuroimaging findings to increase the accuracy of clinical diagnoses for these conditions.
Orexin consists of orexin-A and orexin-B and are neuropeptides involved in the regulation of the sleep–wake cycle, learning and memory, motor control, and autonomic nervous function by its wide range of intracranial projections and neurotransmitters (23, 24). CSF orexin-A levels have been extensively studied in sleep disturbance studies (e.g., narcolepsy, insomnia, and RBD) and AD. Equally, several small-sample studies on LBD manifestations have also been published.
Cerebrospinal Fluid Orexin-A Levels in Lewy Body Disease Patients and Normal Controls
We demonstrated that mean CSF orexin-A levels in LBD were similar to levels in NCs. DLB and PD are the two main forms of LBD and share common clinical and neuropathological traits (25). Despite previous studies showing reductions in neocortical orexin-A levels in patients with DLB (9) and significantly decreased orexinergic neurons in patients with parkinsonian traits and PD animal models, the mechanisms underlying these reductions remain poorly understood. Perhaps the loss of orexin neurons is insufficient to cause significant changes in CSF orexin-A levels. A previous animal study reported that 15% damage to orexinergic neurons did not affect CSF orexin levels, whereas more than 70% damage led to a 50% decline in these levels (26). A recent study on orexin-A plasma levels during PD progression showed that levels were increased in early and medium disease stages and were decreased at advanced PD stages. Orexin-A levels in PD patients were significantly negatively correlated with disease duration and the Movement Disorder Society Unified Parkinson's Disease Rating Scale (MDS-UPDRS) Part III scores (27). These findings may explain how plasma orexin-A levels occur during different PD stages. However, apart from the studies included in this analysis (6, 7), Wennstrom et al. (28) and Lessig et al. (9) reported that orexin-A levels in DLB were significantly lower than those in NCs. While our results are controversial, discrepancies may have arisen due to differences in patient numbers, average patient ages, and cognitive functions.
Cerebrospinal Fluid Orexin-A Levels in Lewy Body Disease and Alzheimer’s Disease Patients
Consistent with the animal and human literature (29, 30), we generally concluded that patients with LBD have lower orexin-A levels when compared with AD patients, potentially suggesting different orexin-A roles in AD and LBD. Sleep disturbance is a common feature of many neurodegenerative diseases, and critically, orexin-A is closely associated with sleep disturbance.
Previous investigations suggested that orexinergic signaling overexpression primarily altered the sleep–wake cycle to promote wakefulness and secondarily induced Beta-amyloid (Aβ) accumulation and Tau-mediated neurodegeneration (31, 32). Both human and animal models suggested that orexin-A may play a role in AD pathogenesis. Aβ levels were significantly increased during acute or long-term sleep distribution and orexin infusion, whereas levels were decreased upon infusion with a dual orexin receptor antagonist. An observational study of 17 AD patients showed that orexin-A was significantly related to Tau (r = 0.463, P < 0.001) and phosphorylated Tau (r = 0.630, P < 0.0001) (33, 34), and that orexin receptor downregulation in the hippocampus, especially the cornu ammonis, was caused by Aβ formation and Tau hyperphosphorylation (32). Therefore, we speculate that disease-specific alterations (orexinergic system damage Aβ modulation in narcolepsy and amyloid pathology in AD) induce the loss of reciprocal Aβ modulation between orexin and amyloid-42 CSF levels (32). Perturbations in both orexin signaling and the sleep–wake cycle may induce adverse effects on maintaining sleep and Aβ dynamics.
A previous study indicated that hypersomnia was more prevalent in LBD than AD (35), in agreement with sleepiness traits using LBD diagnostic criteria (2). Recent evidence has suggested that hypersomnia is associated with LBD (36); increased immunocompetence of orexin neurons and decreased CSF orexin-A levels may account for this finding (37). When orexin neurons are deficient, the balance between sleep and wakefulness is disturbed. Interactions between sleep-active neurons and monoamine-induced wakefulness were weakened in the ventrolateral preoptic nucleus, which reduced balance stability between sleep and wakefulness, leading to narcolepsy. Thus, hypersomnia was identified in animal models bearing orexin receptor gene mutations or receptor knockout phenotypes. In other research involving LBD (including DLB, PD, or PDD) and AD, CSF orexin-A levels in LBD patients were significantly lower than those in the AD group (9, 28). In addition, significant correlations were identified between CSF orexin-A levels and sleep disturbance severity (10) or the scores of Epworth sleepiness scale (ESS), a scale used to evaluate EDS/hypersomnia (7), after calculation. These findings on the connection between AD and LBD remain controversial. In our study, we observed no significant decrease in orexin-A levels in LBD patients when compared with AD groups in selected studies (7, 10, 20). Similarly, we observed no statistically significant differences in orexin-A plasma levels between patients with AD and patients with PD (38).
CSF orexin-A reductions (7, 10, 21) and immunoreactivity in DLB (9) are both correlated with hypersomnia and α-synuclein accumulation, as proposed for PD (6, 21) and an experimental animal model (39). Also, previous postmortem studies revealed the existence of LBs in the hypothalamus of PD patients (40). A recent study also identified α-synuclein accumulation in orexinergic neurons in an A53T mouse model of PD (41). Lessig et al. (9) demonstrated a significant negative correlation (r = −0.447, P < 0.05) between orexin-A levels and α-synuclein aggregation in DLB patients, consistent with previous PD studies showing α-synuclein accumulation in hypocretin-containing neurons in the hypothalamus (6, 42). Unfortunately, few studies have investigated α-synuclein and orexin-A levels in humans; therefore, further studies are required.
Cerebrospinal Fluid Orexin-A Levels in Lewy Body Disease and Frontotemporal Lobar Degeneration Patients
FTLD is a clinically and pathologically diverse group of younger-onset diseases, with patients reportedly developing hypersomnia, insomnia, and other sleep-related symptoms (38, 43, 44). Sleep disturbance in FTLD forms part of a much broader spectrum of homeostatic and related behavioral alterations; the plasma or CSF orexin-A reductions were correlated with hypersomnia (38, 44).
Several small-scale studies compared orexin-A levels between LBD and FTLD patients and reported that reduced levels were related to poor-quality sleep or hypersomnia in FTLD; however, these data were inconclusive (21, 38), as other studies identified no alterations in orexin-A levels (20, 44). Liguori et al. (44) observed no significant differences in CSF orexin-A levels between FTLD patients and controls, whereas their Spearman Rank Order data identified a significant negative correlation between CSF orexin-A levels and ESS scores (r = -0.91, P < 0.0001) in the global FTLD population. A group of 10 consecutive patients with FTLD showed statistically lower orexin-A plasma levels (106 ± 29 pg/ml) than patients with AD (154 ± 74 pg/ml) and patients with PD (135 ± 92 pg/ml) (38). In this cohort, two FTLD patients underwent polysomnographic monitoring, followed by the multiple sleep latency test, and showed that alterations in sleep quality and continuity were associated with hypersomnia. Age and dementia severity may also influence data from comparative studies, as can differences between testing methods. It was reported that CSF orexin-A levels were more sensitive and specific for the diagnosis of sleep disorders (in particular narcolepsy), and that orexin-A functions may be better investigated during CSF analyses (45). Also, damage to blood–brain barrier permeability could be a reason for increased orexin-A plasma levels in PD patients; however, whether plasma orexin-A levels reflect central orexin-A activity remains controversial.
Orexin-A was shown to interact with multiple regions of the brain stem and other neurotransmitter systems, including dopamine (46). The loss of dopamine neurons from the central amygdala decreased dopamine release in DLB and was related to hypersomnia in DLB. Patients with DLB exhibited an uptake reduction in 123I-FP-CIT SPECT, an imaging technology that measures extrastriatal serotonergic transporters in the amygdala. Also, a previous study reported that hypersomnia was associated with depression in DLB (47). Depression and hypersomnia in DLB may be driven by deficits in the noradrenergic system and impact arousal, cognition, and mood (48). Notably, dopamine agonists (DAs) increased hypersomnia or narcolepsy in DLB, though these therapeutics improved motor symptoms in patients with DLB. Therefore, the adverse effects of DAs on sleep in patients with LBD should be fully considered, particularly for patients with hypersomnia.
Currently, studies on CSF orexin-A levels in LBD are lacking. Additionally, different studies in this investigation were controversial. In terms of comparisons between LBD and AD, four studies were identified; hence, the trustworthiness of these results is questionable in this meta-analysis. In terms of the discrimination potential of orexin-A between LBD and FTLD patients, we identified only two studies; therefore, further studies are required before definitive conclusions can be made on the reliability of this biomarker.
Conclusions
Our meta-analysis indicated that CSF orexin-A levels could function as a reliable biomarker to discriminate between LBD and AD/FTLD patients and potentially increase the accuracy of clinical diagnoses for these conditions. In the future, larger sample-size studies must be conducted to confirm and investigate the mechanisms underpinning our findings.
Data Availability Statement
The original contributions presented in the study are included in the article. Further inquiries can be directed to the corresponding author.
Author Contributions
JG conceived the research design. JG, ZC, and JH conducted literature search and data extraction. JG, X-DW, and LM performed the statistical analysis and contributed to writing of the report. SL and YJ reviewed and edited the article in detail. All authors contributed to the article and approved the submitted version.
Funding
This work was supported by the National Natural Science Foundation (grant number 82171182), the National Key Research and Development Program of China (grant number 2016YFC1306305), Science and Technology Project of Tianjin Municipal Health and Health Committee (grant number ZC20121), and Science and Technology Project of Tianjin Municipal Health and Health Committee (grant number KJ20048).
Conflict of Interest
The authors declare that the research was conducted in the absence of any commercial or financial relationships that could be construed as a potential conflict of interest.
Publisher’s Note
All claims expressed in this article are solely those of the authors and do not necessarily represent those of their affiliated organizations, or those of the publisher, the editors and the reviewers. Any product that may be evaluated in this article, or claim that may be made by its manufacturer, is not guaranteed or endorsed by the publisher.
References
1. Foguem C, Manckoundia P. Lewy Body Disease: Clinical and Pathological "Overlap Syndrome" Between Synucleinopathies (Parkinson Disease) and Tauopathies (Alzheimer Disease). Curr Neurol Neurosci Rep (2018) 18(5):24. doi: 10.1007/s11910-018-0835-5
2. Mckeith IG, Boeve BF, Dickson DW, Halliday G, Taylor JP, Weintraub D, et al. Diagnosis and Management of Dementia With Lewy Bodies: Fourth Consensus Report of the DLB Consortium. Neurology (2017) 89(1):88. doi: 10.1212/WNL.0000000000004058
3. Liguori C, Nuccetelli M, Izzi F, Sancesario G, Romigi A, Martorana A, et al. Rapid Eye Movement Sleep Disruption and Sleep Fragmentation are Associated With Increased Orexin-A Cerebrospinal-Fluid Levels in Mild Cognitive Impairment Due to Alzheimer’s Disease. Neurobiol Aging (2016) 40:120–6. doi: 10.1016/j.neurobiolaging.2016.01.007
4. Roh JH, Jiang H, Finn MB, Stewart FR, Mahan TE, Cirrito JR, et al. Potential Role of Orexin and Sleep Modulation in the Pathogenesis of Alzheimer’s Disease. J Exp Med (2014) 211(13):2487–96. doi: 10.1084/jem.20141788
5. Liu MF, Xue Y, Liu C, Liu YH, Diao HL, Wang Y, et al. Orexin-A Exerts Neuroprotective Effects via OX1R in Parkinson’s Disease. Front Neurosci (2018) 12:835. doi: 10.3389/fnins.2018.00835
6. Fronczek R, Overeem S, Lee SY, Hegeman IM, van Pelt J, van Duinen SG, et al. Hypocretin (Orexin) Loss in Parkinson’s Disease. Brain J Neurol (2007) 130(Pt 6):1577–85. doi: 10.1093/brain/awm090
7. Baumann CR, Dauvilliers Y, Mignot E, Bassetti CL. Normal CSF Hypocretin-1 (Orexin A) Levels in Dementia With Lewy Bodies Associated With Excessive Daytime Sleepiness. Eur Neurol (2004) 52(2):73–6. doi: 10.1159/000079749
8. Mahoney CE, Cogswell A, Koralnik IJ, Scammell TE. The Neurobiological Basis of Narcolepsy. Nat Rev Neurosci (2019) 20(2):83–93. doi: 10.1038/s41583-018-0097-x
9. Lessig S, Ubhi K, Galasko D, Adame A, Pham E, Remidios K, et al. Reduced Hypocretin (Orexin) Levels in Dementia With Lewy Bodies. Neuroreport (2010) 21(11):756–60. doi: 10.1097/WNR.0b013e32833bfb7c
10. Inagawa Y, Shimizu S, Takenoshita N, Tsugawa A, Hirose D, Kanbayashi T, et al. Association Between Cerebrospinal Fluid Orexin A Level and Severity of Rapid Eye Movement Sleep Behaviour Disorder in Dementia With Lewy Bodies. Psychogeriatrics Off J Japanese Psychogeriatric Soc (2021) 21(1):128–30. doi: 10.1111/psyg.12618
11. Gratwicke J, Jahanshahi M, Foltynie T. Parkinson’s Disease Dementia: A Neural Networks Perspective. Brain J Neurol (2015) 138(Pt 6):1454–76. doi: 10.1093/brain/awv104
12. Savica R, Grossardt BR, Bower JH, Boeve BF, Ahlskog JE, Rocca WA. Incidence of Dementia With Lewy Bodies and Parkinson Disease Dementia. JAMA Neurol (2013) 70(11):1396–402. doi: 10.1001/jamaneurol.2013.3579
13. Kane JPM, Surendranathan A, Bentley A, Barker SAH, Taylor JP, Thomas AJ, et al. Clinical Prevalence of Lewy Body Dementia. Alzheimer’s Res Ther (2018) 10(1):19. doi: 10.1186/s13195-018-0350-6
14. Gan J, Liu S, Wang X, Shi Z, Shen L, Li X, et al. Clinical Characteristics of Lewy Body Dementia in Chinese Memory Clinics. BMC Neurol (2021) 21(1):144. doi: 10.1186/s12883-021-02169-w
15. Borroni B, Agosti C, Padovani A. Behavioral and Psychological Symptoms in Dementia With Lewy-Bodies (DLB): Frequency and Relationship With Disease Severity and Motor Impairment. Arch Gerontol Geriatrics (2008) 46(1):101–6. doi: 10.1016/j.archger.2007.03.003
16. Compta Y, Santamaria J, Ratti L, Tolosa E, Iranzo A, Muñoz E, et al. Cerebrospinal Hypocretin, Daytime Sleepiness and Sleep Architecture in Parkinson’s Disease Dementia. Brain J Neurol (2009) 132(Pt 12):3308–17. doi: 10.1093/brain/awp263
17. Baumann C, Ferini-Strambi L, Waldvogel D, Werth E, Bassetti CL. Parkinsonism With Excessive Daytime Sleepiness: A Narcolepsy-Like Disorder? J Neurol (2005) 252(2):139–45. doi: 10.1007/s00415-005-0614-5
18. Liberati A, Altman DG, Tetzlaff J, Mulrow C, Gøtzsche PC, Ioannidis JP, et al. The PRISMA Statement for Reporting Systematic Reviews and Meta-Analyses of Studies That Evaluate Healthcare Interventions: Explanation and Elaboration. Bmj (2009) 339:b2700. doi: 10.1136/bmj.b2700
19. Hozo SP, Djulbegovic B, Hozo I. Estimating the Mean and Variance From the Median, Range, and the Size of a Sample. BMC Med Res Method (2005) 5:13. doi: 10.1186/1471-2288-5-13
20. Trotti LM, Bliwise DL, Keating GL, Rye DB, Hu WT. Cerebrospinal Fluid Hypocretin and Nightmares in Dementia Syndromes. Dementia Geriatric Cogn Disord Extra (2021) 11(1):19–25. doi: 10.1159/000509585
21. Yasui K, Inoue Y, Kanbayashi T, Nomura T, Kusumi M, Nakashima K. CSF Orexin Levels of Parkinson's Disease, Dementia With Lewy Bodies, Progressive Supranuclear Palsy And Corticobasal Degeneration. J Neurol Sci (2006) 250(1-2):120–3. doi: 10.1016/j.jns.2006.08.004
22. Erkkinen MG, Kim MO, Geschwind MD. Clinical Neurology and Epidemiology of the Major Neurodegenerative Diseases. Cold Spring Harbor Perspect Biol (2018) 10(4). doi: 10.1101/cshperspect.a033118
23. de Lecea L, Kilduff TS, Peyron C, Gao X, Foye PE, Danielson PE, et al. The Hypocretins: Hypothalamus-Specific Peptides With Neuroexcitatory Activity. Proc Natl Acad Sci USA (1998) 95(1):322–7. doi: 10.1073/pnas.95.1.322
24. Kayaba Y, Nakamura A, Kasuya Y, Ohuchi T, Yanagisawa M, Komuro I, et al. Attenuated Defense Response and Low Basal Blood Pressure in Orexin Knockout Mice. Am J Physiol Regul Integr Comp Physiol (2003) 285(3):R581–93. doi: 10.1152/ajpregu.00671.2002
25. Wakabayashi K, Tanji K, Odagiri S, Miki Y, Mori F, Takahashi H. The Lewy Body in Parkinson’s Disease and Related Neurodegenerative Disorders. Mol Neurobiol (2013) 47(2):495–508. doi: 10.1007/s12035-012-8280-y
26. Drouot X, Moutereau S, Nguyen JP, Lefaucheur JP, Créange A, Remy P, et al. Low Levels of Ventricular CSF Orexin/Hypocretin in Advanced PD. Neurology (2003) 61(4):540–3. doi: 10.1212/01.wnl.0000078194.53210.48
27. Huang S, Zhao Z, Ma J, Hu S, Li L, Wang Z, et al. Increased Plasma Orexin-A Concentrations are Associated With the non-Motor Symptoms in Parkinson’s Disease Patients. Neurosci Lett (2021) 741:135480. doi: 10.1016/j.neulet.2020.135480
28. Wennström M, Londos E, Minthon L, Nielsen HM. Altered CSF Orexin and α-Synuclein Levels in Dementia Patients. J Alzheimers Dis: JAD (2012) 29(1):125–32. doi: 10.3233/jad-2012-111655
29. Shimizu S, Takenoshita N, Inagawa Y, Tsugawa A, Hirose D, Kaneko Y, et al. Positive Association Between Cognitive Function and Cerebrospinal Fluid Orexin A Levels in Alzheimer’s Disease. J Alzheimer’s Dis JAD (2020) 73(1):117–23. doi: 10.3233/jad-190958
30. Dauvilliers YA, Lehmann S, Jaussent I, Gabelle A. Hypocretin and Brain β-Amyloid Peptide Interactions in Cognitive Disorders and Narcolepsy. Front Aging Neurosci (2014) 6:119. doi: 10.3389/fnagi.2014.00119
31. Liguori C. Orexin and Alzheimer’s Disease. In: Lawrence AJ, de Lecea L, editors. Behavioral Neuroscience of Orexin/Hypocretin. Cham: Springer International Publishing (2017). p. 305–22.
32. Kang JE, Lim MM, Bateman RJ, Lee JJ, Smyth LP, Cirrito JR, et al. Amyloid-Beta Dynamics are Regulated by Orexin and the Sleep-Wake Cycle. Sci (New York NY) (2009) 326(5955):1005–7. doi: 10.1126/science.1180962
33. Ju YE, McLeland JS, Toedebusch CD, Xiong C, Fagan AM, Duntley SP, et al. Sleep Quality and Preclinical Alzheimer Disease. JAMA Neurol (2013) 70(5):587–93. doi: 10.1001/jamaneurol.2013.2334
34. Deuschle M, Schilling C, Leweke FM, Enning F, Pollmächer T, Esselmann H, et al. Hypocretin in Cerebrospinal Fluid is Positively Correlated With Tau and Ptau. Neurosci Lett (2014) 561:41–5. doi: 10.1016/j.neulet.2013.12.036
35. Ferman TJ, Smith GE, Dickson DW, Graff-Radford NR, Lin SC, Wszolek Z, et al. Abnormal Daytime Sleepiness in Dementia With Lewy Bodies Compared to Alzheimer’s Disease Using the Multiple Sleep Latency Test. Alzheimer’s Res Ther (2014) 6(9):76. doi: 10.1186/s13195-014-0076-z
36. Abbott RD, Ross GW, Duda JE, Shin C, Uyehara-Lock JH, Masaki KH, et al. Excessive Daytime Sleepiness and Topographic Expansion of Lewy Pathology. Neurology (2019) 93(15):e1425–e32. doi: 10.1212/wnl.0000000000008241
37. Fronczek R, van Geest S, Frölich M, Overeem S, Roelandse FW, Lammers GJ, et al. Hypocretin (Orexin) Loss in Alzheimer’s Disease. Neurobiol Aging (2012) 33(8):1642–50. doi: 10.1016/j.neurobiolaging.2011.03.014
38. Çoban A, Bilgiç B, Lohmann E, Küçükali C, Benbir G, Karadeniz D, et al. Reduced Orexin-A Levels in Frontotemporal Dementia: Possible Association With Sleep Disturbance. Am J Alzheimers Dis Other Demen (2013) 28(6):606–11. doi: 10.1177/1533317513494453
39. Stanojlovic M, Pallais JP, Lee MK, Kotz CM. Pharmacological and Chemogenetic Orexin/Hypocretin Intervention Ameliorates Hipp-Dependent Memory Impairment in the A53T Mice Model of Parkinson’s Disease. Mol Brain (2019) 12(1):87. doi: 10.1186/s13041-019-0514-8
40. Kalia LV, Kalia SK. α-Synuclein and Lewy Pathology in Parkinson’s Disease. Curr Opin Neurol (2015) 28(4):375–81. doi: 10.1097/wco.0000000000000215
41. Stanojlovic M, Pallais JP, Kotz CM. Chemogenetic Modulation of Orexin Neurons Reverses Changes in Anxiety and Locomotor Activity in the A53T Mouse Model of Parkinson’s Disease. Front Neurosci (2019) 13:702. doi: 10.3389/fnins.2019.00702
42. Thannickal TC, Lai YY, Siegel JM. Hypocretin (Orexin) Cell Loss in Parkinson’s Disease. Brain J Neurol (2007) 130(Pt 6):1586–95. doi: 10.1093/brain/awm097
43. Bonakis A, Economou NT, Paparrigopoulos T, Bonanni E, Maestri M, Carnicelli L, et al. Sleep in Frontotemporal Dementia is Equally or Possibly More Disrupted, and at an Earlier Stage, When Compared to Sleep in Alzheimer’s Disease. J Alzheimer’s Dis JAD (2014) 38(1):85–91. doi: 10.3233/jad-122014
44. Liguori C, Romigi A, Mercuri NB, Nuccetelli M, Izzi F, Albanese M, et al. Cerebrospinal-Fluid Orexin Levels and Daytime Somnolence in Frontotemporal Dementia. J Neurol (2014) 261(9):1832–6. doi: 10.1007/s00415-014-7455-z
45. Dalal MA, Schuld A, Haack M, Uhr M, Geisler P, Eisensehr I, et al. Normal Plasma Levels of Orexin A (Hypocretin-1) in Narcoleptic Patients. Neurology (2001) 56(12):1749–51. doi: 10.1212/wnl.56.12.1749
46. Hajak G, Geisler P. Orchestrating Sleep-Wake Functions in the Brain. Nat Med (2003) 9(2):170–1. doi: 10.1038/nm0203-170
47. Elder GJ, Colloby SJ, Lett DJ, O’Brien JT, Anderson KN, Burn DJ, et al. Depressive Symptoms are Associated With Daytime Sleepiness and Subjective Sleep Quality in Dementia With Lewy Bodies. Int J Geriatric Psychiatry (2016) 31(7):765–70. doi: 10.1002/gps.4389
48. Szot P, White SS, Greenup JL, Leverenz JB, Peskind ER, Raskind MA. Compensatory Changes in the Noradrenergic Nervous System in the Locus Ceruleus and Hippocampus of Postmortem Subjects With Alzheimer’s Disease and Dementia With Lewy Bodies. J Neurosci Off J Soc Neurosci (2006) 26(2):467–78. doi: 10.1523/jneurosci.4265-05.2006
Keywords: orexin-A (hypocretin-1), Lewy body disease, Parkinson’s disease, excessive daytime sleepiness, systematic review, meta-analysis
Citation: Gan J, Chen Z, Han J, Ma L, Liu S, Wang X-D and Ji Y (2021) Orexin-A in Patients With Lewy Body Disease: A Systematic Review and Meta-Analysis. Front. Endocrinol. 12:765701. doi: 10.3389/fendo.2021.765701
Received: 27 August 2021; Accepted: 27 October 2021;
Published: 17 November 2021.
Edited by:
Hiroyuki Kaiya, National Cerebral and Cardiovascular Center, JapanReviewed by:
Brynn Dredla, Mayo Clinic Florida, United StatesMariusz Sieminski, Medical University of Gdansk, Poland
Copyright © 2021 Gan, Chen, Han, Ma, Liu, Wang and Ji. This is an open-access article distributed under the terms of the Creative Commons Attribution License (CC BY). The use, distribution or reproduction in other forums is permitted, provided the original author(s) and the copyright owner(s) are credited and that the original publication in this journal is cited, in accordance with accepted academic practice. No use, distribution or reproduction is permitted which does not comply with these terms.
*Correspondence: Yong Ji, aml5b25ndXNhQDEyNi5jb20=