- 1Department of Endocrinology, Hvidovre Hospital, Hvidovre, Denmark
- 2Novo Nordisk Foundation Center for Basic Metabolic Research and Department of Biomedical Sciences, University of Copenhagen, Copenhagen, Denmark
- 3Department of Surgical Gastroenterology, Hvidovre Hospital, Hvidovre, Denmark
- 4Department of Clinical Biochemistry Rigshospitalet, Copenhagen, Denmark
- 5Novo Nordisk Foundation Center for Protein Research, University of Copenhagen, Copenhagen, Denmark
- 6Research and Development, Novo Nordisk A/S, Måløv, Denmark
Background: Altered bile acid (BA) turnover has been suggested to be involved in the improved glucose regulation after Roux-en-Y gastric bypass (RYGB), possibly via stimulation of GLP-1 secretion. We investigated the role of exogenous as well as endogenous BAs for GLP-1 secretion after RYGB by administering chenodeoxycholic acid (CDCA) and the BA sequestrant colesevelam (COL) both in the presence and the absence of a meal stimulus.
Methods: Two single-blinded randomized cross-over studies were performed. In study 1, eight RYGB operated participants ingested 200 ml water with 1) CDCA 1.25 g or 2) CDCA 1.25 g + colesevelam 3.75 g on separate days. In study 2, twelve RYGB participants ingested on separate days a mixed meal with addition of 1) CDCA 1.25 g, 2) COL 3.75 g or 3) COL 3.75 g × 2, or 4) no additions.
Results: In study 1, oral intake of CDCA increased circulating BAs, GLP-1, C-peptide, glucagon, and neurotensin. Addition of colesevelam reduced all responses. In study 2, addition of CDCA enhanced meal-induced increases in plasma GLP-1, glucagon and FGF-19 and lowered plasma glucose and C-peptide concentrations, while adding colesevelam lowered circulating BAs but did not affect meal-induced changes in plasma glucose or measured gastrointestinal hormones.
Conclusion: In RYGB-operated persons, exogenous CDCA enhanced meal-stimulated GLP-1 and glucagon secretion but not insulin secretion, while the BA sequestrant colesevelam decreased CDCA-stimulated GLP-1 secretion but did not affect meal-stimulated GLP-1, C-peptide or glucagon secretion, or glucose tolerance. These findings suggest a limited role for endogenous bile acids in the acute regulation of postprandial gut hormone secretion or glucose metabolism after RYGB.
Introduction
Roux-en-Y gastric bypass (RYGB) is the most effective treatment of both obesity and type 2 diabetes (1, 2). Interestingly, glucose metabolism is improved in patients with type 2 diabetes within days after surgery indicating causal mechanisms other than weight loss (3, 4). Several factors seem to be involved including an accelerated transit of nutrients to the small intestine and augmented absorption, which leads to altered gut hormone secretion and among these a greatly enhanced (up to 10-fold) secretion of glucagon-like peptide-1 (GLP-1) (5, 6). GLP-1 is an insulinotropic and appetite inhibiting hormone, and in studies involving the GLP-1 receptor antagonist, Exendin 9-39, the GLP-1 response has been shown to be important for the reduction in food intake and improved beta-cell function observed after RYGB (7–9).
The mechanisms driving the increased secretion of GLP-1 are not fully clarified, but bile acids have been suggested to play a role. Apart from their role in lipid digestion and absorption, bile acids are signal molecules that stimulate GLP-1 secretion through activation of the G-protein coupled receptor TGR-5 (10), as demonstrated both in vivo (11) and in vitro (12–17). Moreover, activation of the bile-acid sensitive nuclear receptor FXR has also been suggested to modulate GLP-1 secretion (18, 19). After RYGB, increased concentrations of plasma bile acids are positively correlated to the increase in GLP-1 concentrations (20, 21) and improved glucose tolerance (22, 23) months to years after RYGB. These observations led to the hypothesis that bile acids are involved in the metabolic improvements after RYGB. However, there is a dissociation in the timing of the postoperative changes since total plasma bile acid concentrations are unchanged in the early postoperative period (21, 24), whereas postprandial GLP-1 secretion is enhanced immediately after surgery, concomitantly with increased insulin secretion and improved glucose tolerance (25, 26). Thus, the role of bile acids for the exaggerated postprandial GLP-1 secretion and glucose metabolism after RYGB remains unclear.
We have previously demonstrated that administration of the unconjugated primary bile acid chenodeoxycholic acid (CDCA) [a potent TGR5 and FXR agonist (27, 28)] to RYGB operated subjects stimulates both GLP-1, PYY, neurotensin, glucagon, and insulin secretion even in the absence of nutrient ingestion.
The primary aim of the current study was to expand on this observation of a link between bile acids and GLP-1 secretion by examining the effect of CDCA in a different cohort and during meal-ingestion and, more importantly, to investigate the contribution of endogenous bile acids for meal-stimulated GLP-1 secretion. The latter was done by inhibiting bile acid reabsorption through administration of the bile acid sequestrant colesevelam (COL), which efficiently blocks reabsorption by cross-linking of bile acids in the intestinal lumen leading to increased fecal bile acid excretion (29, 30). As acute bile acid-stimulated GLP-1 secretion has been demonstrated to be mediated by activation of basolaterally located TGR5 receptors on the L-cell (27, 31), blocking reabsorption would be expected to reduce GLP-1 secretion. The ability of COL to attenuate bile acid absorption and decrease GLP-1 secretion was tested by administration of exogenous CDCA with and without COL in the absence of nutrients in a sub-study (study 1) before testing the postprandial responses (study 2). In addition, we investigated the modulatory effects of exogenous (CDCA) and endogenous bile acids (by COL administration) on meal-induced plasma concentrations of glucose, including the risk of hypoglycemia, and other glucoregulatory hormones including insulin, glucagon, CCK, neurotensin and FGF-19. Apart from in the N-cell, neurotensin is co-expressed with GLP-1 in some L-cells (32) and its secretion, like GLP-1, depends on intestinal absorption and receptor activation, which might be modulated by CDCA and COL (27, 32).
Methods
Participants
For study 1, eight participants (four women and four men), and for study 2 twelve participants (six women and six men) were recruited from the bariatric surgery program at Hvidovre Hospital (Tables 1 and 2).
Inclusion criteria for both studies were uncomplicated RYGB >3 months prior to inclusion and only participants without diabetes were included (defined as fasting plasma glucose <7.0 mmol/L and HbA1c <48 mmol/mol before and after RYGB). Exclusion criteria were hemoglobin <6.5 mmol/L, cholecystectomy, dysregulated thyroid diseases (TSH outside reference range), use of anti-thyroid medication, renal insufficiency, prior pancreatitis, certain RYGB related complications (severe reactive hypoglycemia or dysphagia), pregnancy or lactation. All participants were weight stable at inclusion (defined as +/− 2 kg within the last month), and none had symptoms of postprandial hypoglycemia. Written informed consent was obtained from all participants before entering the study. The protocols were approved by the Regional Ethical Committee of the Capital Region in accordance with the Helsinki II declaration, by the Danish Data Protection Agency, and both studies were registered at www.clinicaltrial.org (NCT02952963 and NCT02876484).
Study Design
In both studies, participants were instructed to refrain from strenuous physical activity and alcohol for three days and to fast overnight before all study days, which were separated by at least 48 h. On each study day participants were weighed, and a catheter was inserted into a forearm vein for blood sampling.
In study 1, participants were examined on two separate study days performed in randomized order with the following interventions: CDCA: Intake of 1.25 g chenodeoxycholic acid (Xenobilox capsules, Juers Pharma, Germany) suspended in 200 ml water, followed by 50 ml water; CDCA + COL: Intake of 1.25 g chenodeoxycholic acid and 3.75 g colesevelam suspended in 200 ml water followed by 50 ml water. After three basal blood samples (t = −10, −5, 0 min) CDCA or CDCA + COL was ingested evenly during a 5-min supervised period. Blood was sampled frequently for a total of 4 h (at t = 5, 10, 15, 20, 25, 30, 45, 60, 90, 120, 180, 240 min).
In study 2, participants were examined on four separate study days performed in a randomized order: 1. Meal: Mixed meal (1,523 kJ, 53E% carb, 33E% fat, 14E% protein) consisting of both solid and semisolid components: yoghurt, oatmeal, raisins, rye bread, margarine, cheese, and almonds) and with 1 g of paracetamol (PCM) (Pamol, Nycomed, Roskilde, Denmark) added to estimate pouch emptying, 2. Meal + CDCA: Mixed meal with 1.25 g chenodeoxycholic acid (Xenobilox capsules, Juers Pharma, Germany) mixed into the yoghurt, 3. Meal + COL Mixed meal with 3.75 g colesevelam suspended in 25 ml water and mixed into the yoghurt, 4. Meal + COL × 2: Mixed meal with 2 × 3.75 g colesevelam with the first dose of colesevelam given as capsules the night before the study day and the second dose given with the mixed meal as described for meal + COL.
At t = 0 the mixed meal was ingested during 20 supervised minutes, where participants were instructed to ingest the different components of the meal in the same order on every study day. PCM was added to the first bite of the meal. Blood was sampled as in study 1.
The doses of CDCA and COL reflected the clinically recommended daily doses of both drugs. We administered COL in two dosing regimens on separate experimental days, one involving a single dose immediately prior to meal intake and the other with an extra dose of COL given the night before the study day in addition to the dose with the mixed meal. This strategy was chosen because recent scintigraphic data suggests that bile acids in RYGB-operated subjects may be released independently of meal-ingestion and are present in the small intestine already in the fasting state (pre-meal) due to an altered bilio-enteric flow after RYGB, which may be mediated by enhanced passive re-uptake and decreased fecal bile acid loss (33, 34).
Sample Collection and Laboratory Analyses
Blood was collected into a) prechilled EDTA-coated tubes containing in addition the dipeptidyl peptidase-4 substrate valine-pyrrolidide (final concentration 0.01 mmol/L; courtesy of Novo Nordisk) for analyses of plasma GLP-1, CCK, neurotensin, total bile acids (TBA) and fibroblast growth factor 19 (FGF-19) and b) clot activator tubes for analysis of serum C-peptide, insulin, and PCM (PCM, only in study 2).
EDTA tubes were centrifuged at 4°C (2,000 × g for 10 min) immediately after blood collection, and clot activator tubes after 30 min at room temperature for coagulation.
Plasma for analysis of plasma glucose was stored at 5°C before analysis at the end of each experimental day, using YSI model 2300 STAT plus (YSI, Yellow Spring, OH). Plasma and serum for all other analyses were stored at −20 and −80°C, respectively until batch analysis. Serum C-peptide concentrations were measured using an Immulite 2000 analyzer (Siemens Healthcare Diagnostics Inc., Tarrytown, NY, USA). Plasma samples were assayed for total GLP-1 and total neurotensin immunoreactivity using inhouse RIA using antiserum 89390 and 3D97, respectively, as reported previously (35, 36). CCK was measured by RIA using antiserum no. 92128, which binds all the bioactive forms of CCK in circulation (CCK-58, -33, -22, -8) with equal potency as reported previously (37). TBA concentrations were determined using an enzyme cycling based TBA Assay Kit from Diazyme (Cat No DZ042A-K, San Diego, CA, USA). FGF19 concentrations were determined using a commercial human FGF19 sandwich ELISA kit from BioVendor (Cat No RD191107200R, BioVendor, Brno, Czech Republic). Paracetamol was measured using a colorimetric assay (Roche Diagnostics GmbH, Mannheim, Germany).
Statistical Analyses and Calculations
Basal concentrations were estimated as the mean of the three fasting samples. Total area-under-the-curve (AUC) was calculated using the trapezoidal rule and incremental AUC (iAUC) by subtracting baseline values. Positive incremental area-under-the-curve (piAUC) was calculated from postprandial concentrations above baseline concentrations. Beta-cell function was evaluated by two indices based on the results obtained from the mixed meal tests in study 2. Firstly, to estimate the initial beta-cell response during a meal, the insulinogenic index (IGI) was calculated from plasma C-peptide and plasma glucose: (C-peptidet = 30 – C-peptidet = 0)/(plasma glucose t = 30 – plasma glucose t = 0). Secondly, an index of beta-cell function covering the whole postprandial period was calculated as AUCC-peptide/AUCglucose throughout the 240 min of the mixed meal test. Insulin resistance was calculated using the homeostasis model assessment of insulin resistance (HOMA-IR: insulinfasting × glucosefasting/(22.5 × 6.945)). Rate of intestinal nutrient entry was evaluated by using time to peak of plasma paracetamol concentrations.
In study 1, effects of CDCA alone were evaluated as iAUC vs. 0 using paired t-tests, and differences between the two study days were analyzed using paired t-tests.
In study 2, the importance of exogenous and endogenous bile acids for outcome parameters was analyzed separately. Accordingly, the test days with meal + CDCA were compared with meal alone using paired t-tests, and the two days with COL administration were compared with meal in a one-way ANOVA with post hoc comparisons using Wald tests. Logarithmic transformations were used where appropriate. Data are expressed as mean ± SEM. P-values <0.05 were considered significant. Statistical analyses were performed in R version 3.5.1 (http://www.r-project.org/).
Results
Study 1
Total bile acids
TBAs increased markedly after CDCA administration, and increases were almost eliminated (~90%) when COL was co-administered (CDCA + COL) compared with CDCA alone (p < 0.01 for piAUC and peak values) (Figure 1, Table 3).
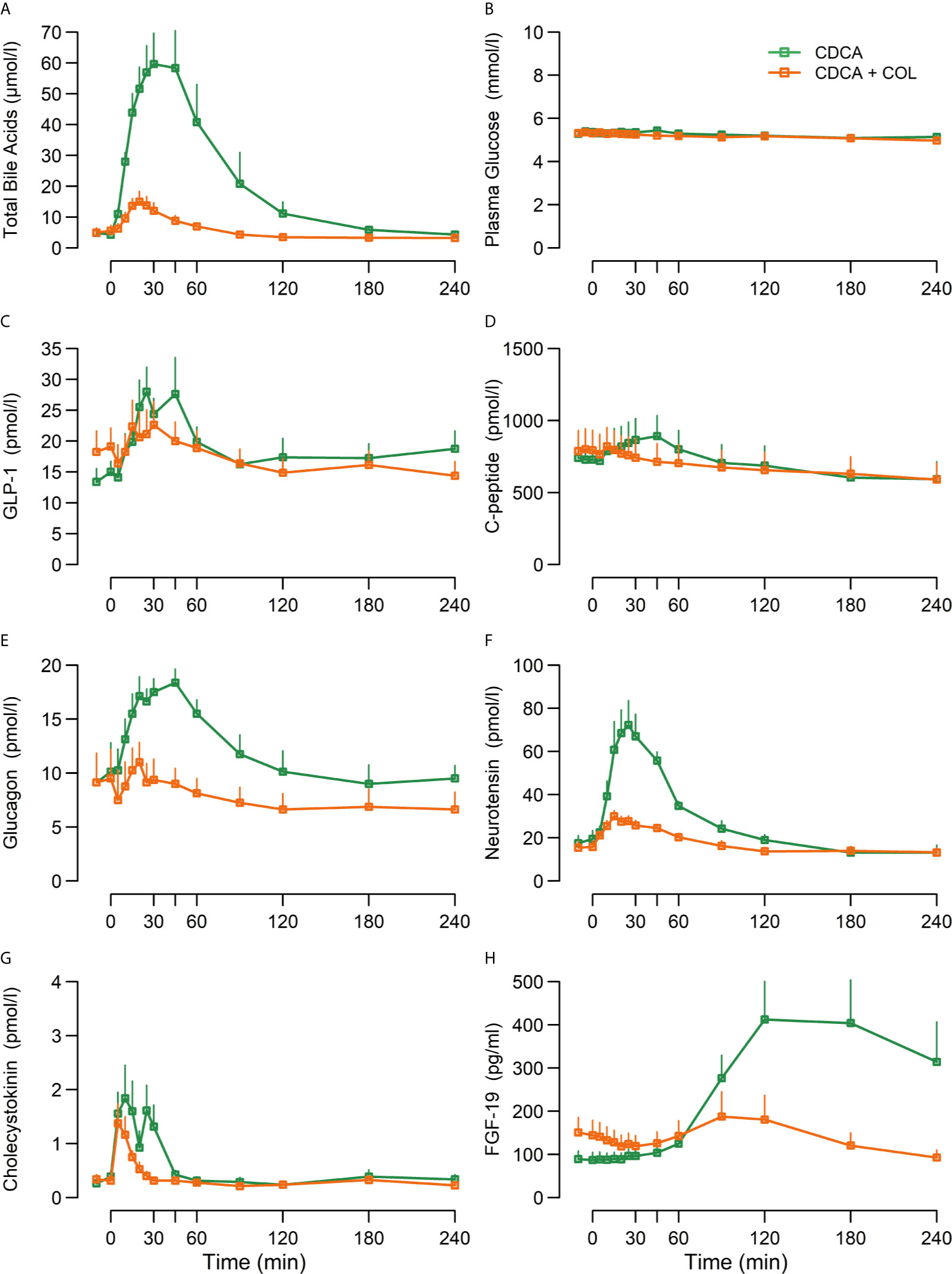
Figure 1 Concentrations of (A) total bile acids, (B) plasma glucose, (C) GLP-1, (D) C-peptide, (E) glucagon, (F) neurotensin, (G) cholecystokinin, and (H) FGF-19 following ingestion of chenodeoxycholic acid (CDCA) or CDCA in combination with the bile acid sequestrant colesevelam (COL) suspended in water (CDCA + COL), n = 8. Data are mean ± SEM.
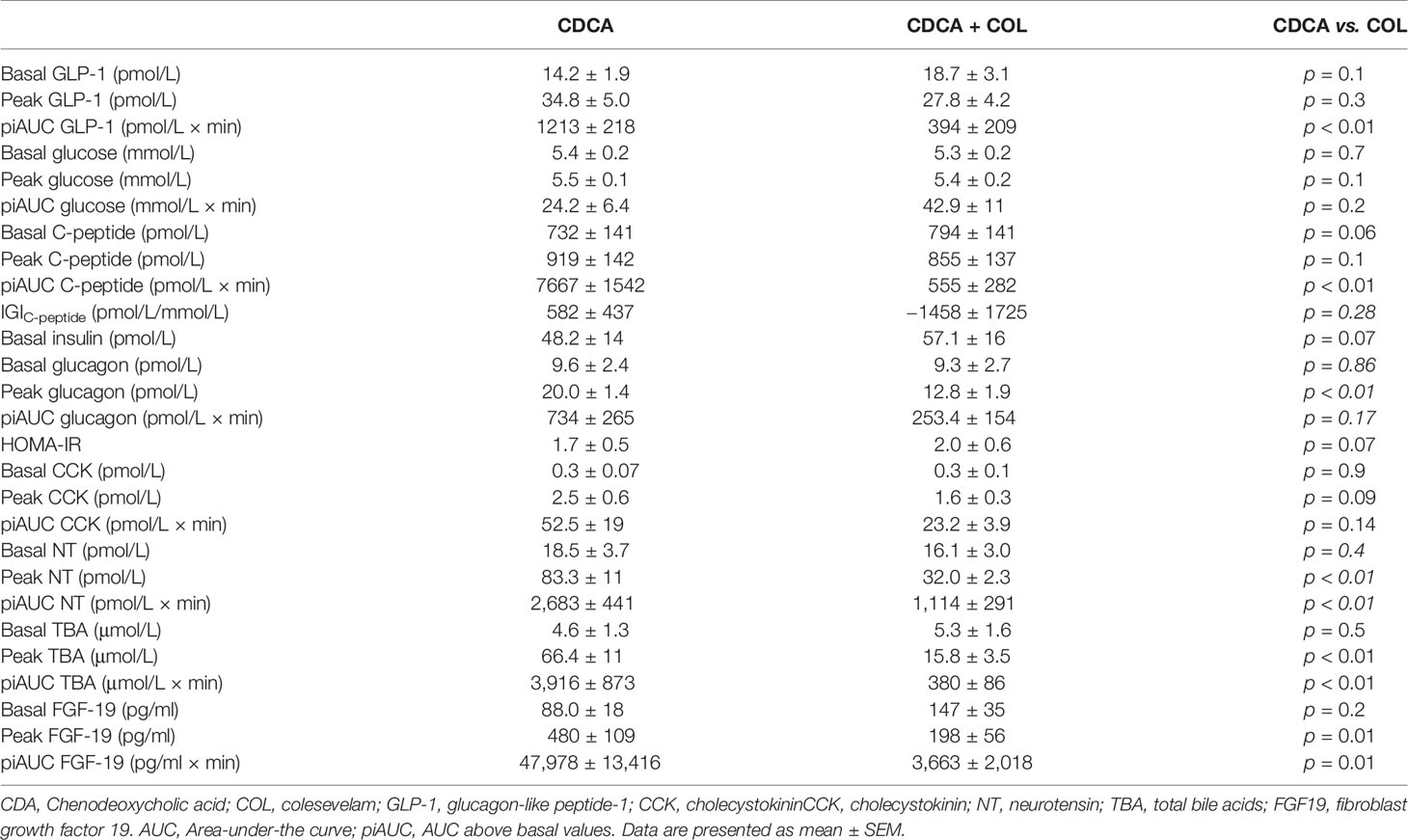
Table 3 Concentrations of glucometabolic- and gut hormones, VAS and blood pressure in response to CDCA administration with or without COL in study 1 (n = 8).
GLP-1, Neurotensin, and CCK
CDCA clearly increased concentrations of GLP-1, NT, and CCK (iAUC vs. 0, p < 0.01) and administration of CDCA + COL decreased the responses of both GLP-1 (piAUC by 67%, p < 0.01) and neurotensin (piAUC by 58%, p < 0.01) compared with CDCA alone. Neither piAUC nor peak of CCK differed between study days, but addition of COL shortened the duration of the CCK response (Figure 1, Table 3).
Plasma Glucose, Serum C-Peptide, Glucagon and FGF-19
Plasma glucose was unaltered by CDCA, whereas C-peptide, glucagon, and FGF-19 increased significantly (iAUC vs. 0, p < 0.01). Neither peak nor piAUC of plasma glucose concentrations differed between study days, but concentrations of C-peptide were lower after CDCA + COL compared with CDCA alone (piAUC p < 0.01), (Table 3, Figure 1). For glucagon, peak concentrations decreased after CDCA + COL compared with CDCA alone (p < 0.01), and a numerical decrease was seen for piAUC (p = 0.17). Concentrations of FGF-19 decreased by >90% after CDCA + COL compared with CDCA (p = 0.01 for both piAUC and peak) (Figure 1, Table 3).
Study 2
Total Bile Acids
Plasma TBA concentrations increased markedly during meal + CDCA compared with meal alone (both peak and piAUC p < 0.01). On the contrary, a significant decrease of piAUC of TBA by ~68% was seen after both doses of COL (both p < 0.01) (Figure 2, Table 4).
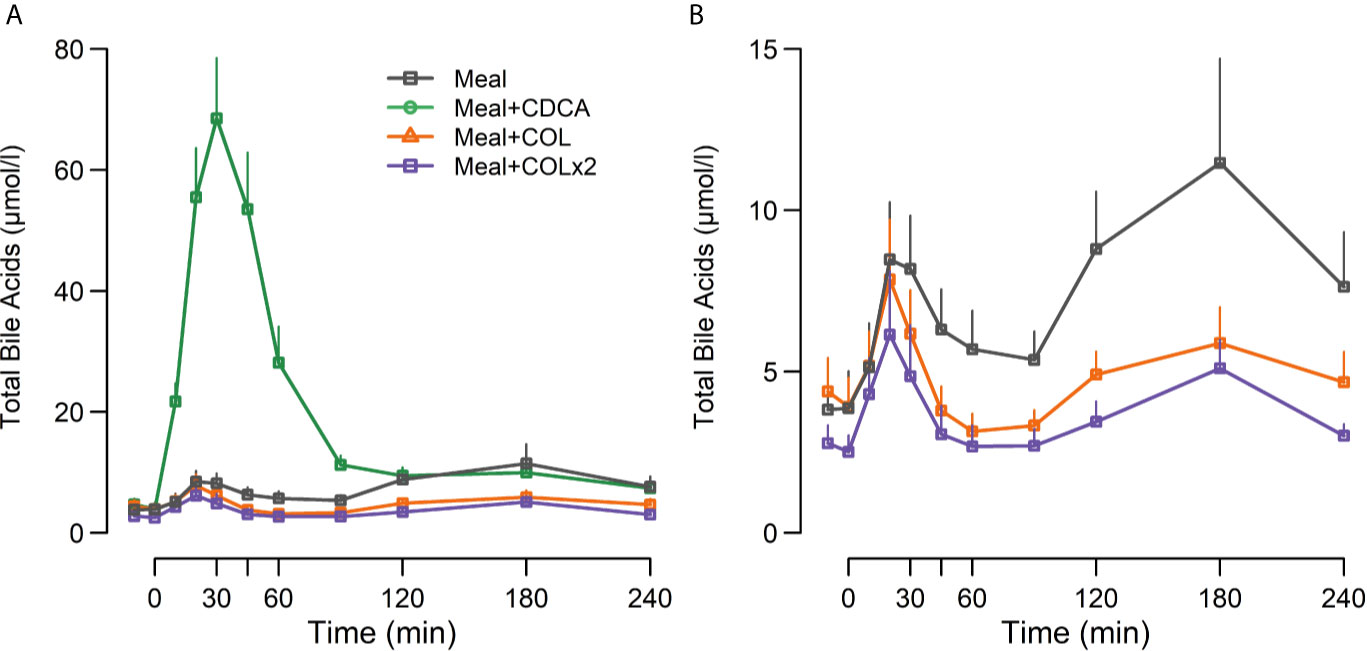
Figure 2 Plasma concentrations of (A) total bile acids and (B) total bile acids (without the CDCA concentration curve drawn) following ingestion of a mixed meal alone (Meal), mixed meal in combination with CDCA added to the meal (Meal + CDCA), mixed meal in combination with COL added to the meal (Meal + COL), or mixed meal in combination with COL administered both the night before the study day as tablets and added to the meal (Meal + COL × 2), n = 12. Data are mean ± SEM.
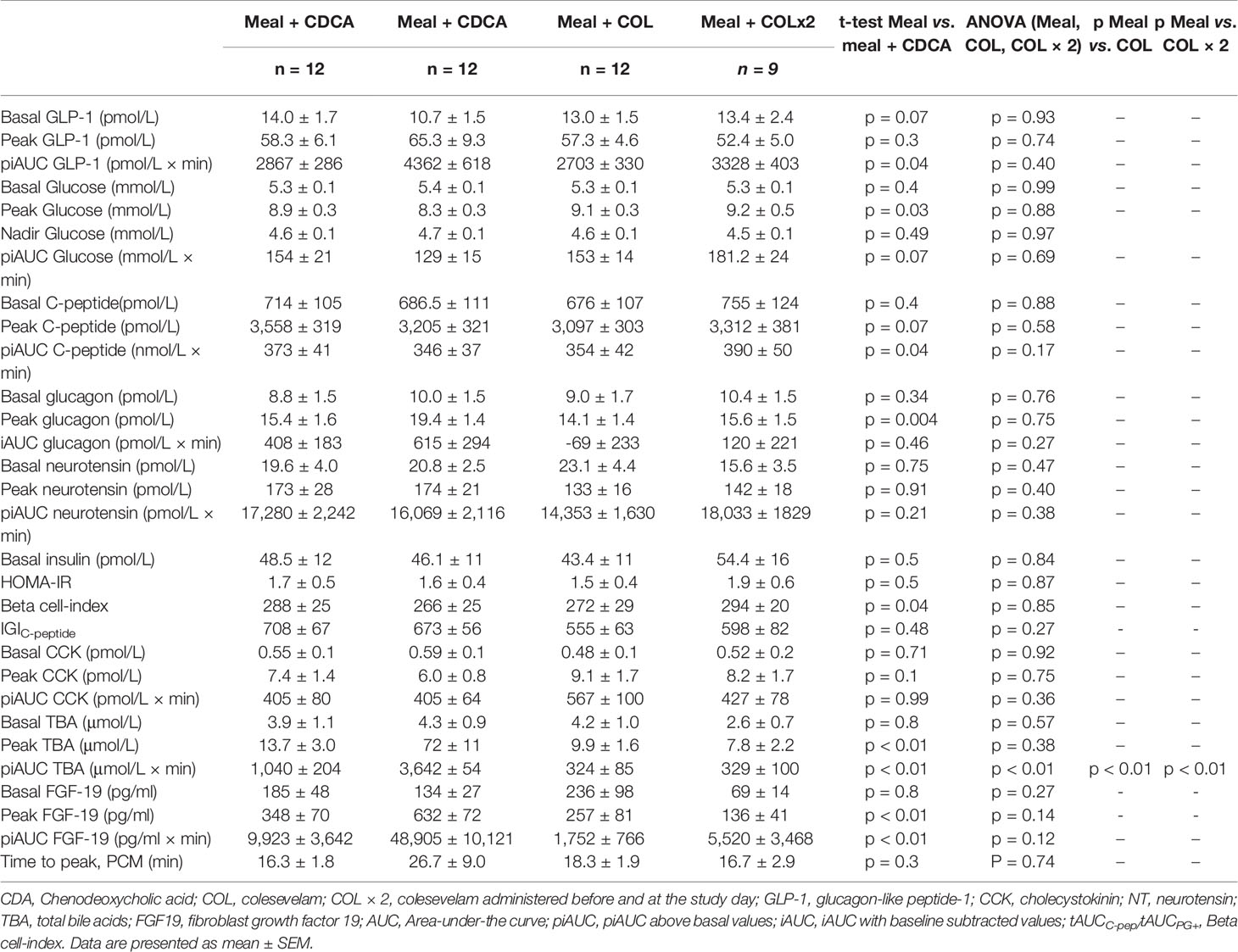
Table 4 Concentrations of glucometabolic- and gut hormones, VAS and blood pressure in response to a mixed meal alone (Meal), meal with CDCA administration (Meal + CDCA), meal with single dosage of COL (Meal + COL) and meal with double dosage of COL (Meal + COL × 2) in study 2.
GLP-1, Neurotensin, and CCK
Postprandial GLP-1 responses were prolonged and higher during meal + CDCA compared with the meal alone (p = 0.04 for piAUC), whereas peak GLP-1 concentrations were comparable (p = 0.3). In contrast, GLP-1 responses after COL administration (both piAUC and peak) were similar to meal alone regardless of COL doses. Concentrations of neurotensin did not differ between test days. Similarly, postprandial CCK secretion appeared unaffected by the addition of CDCA or COL to the meal (Figure 3, Table 4).
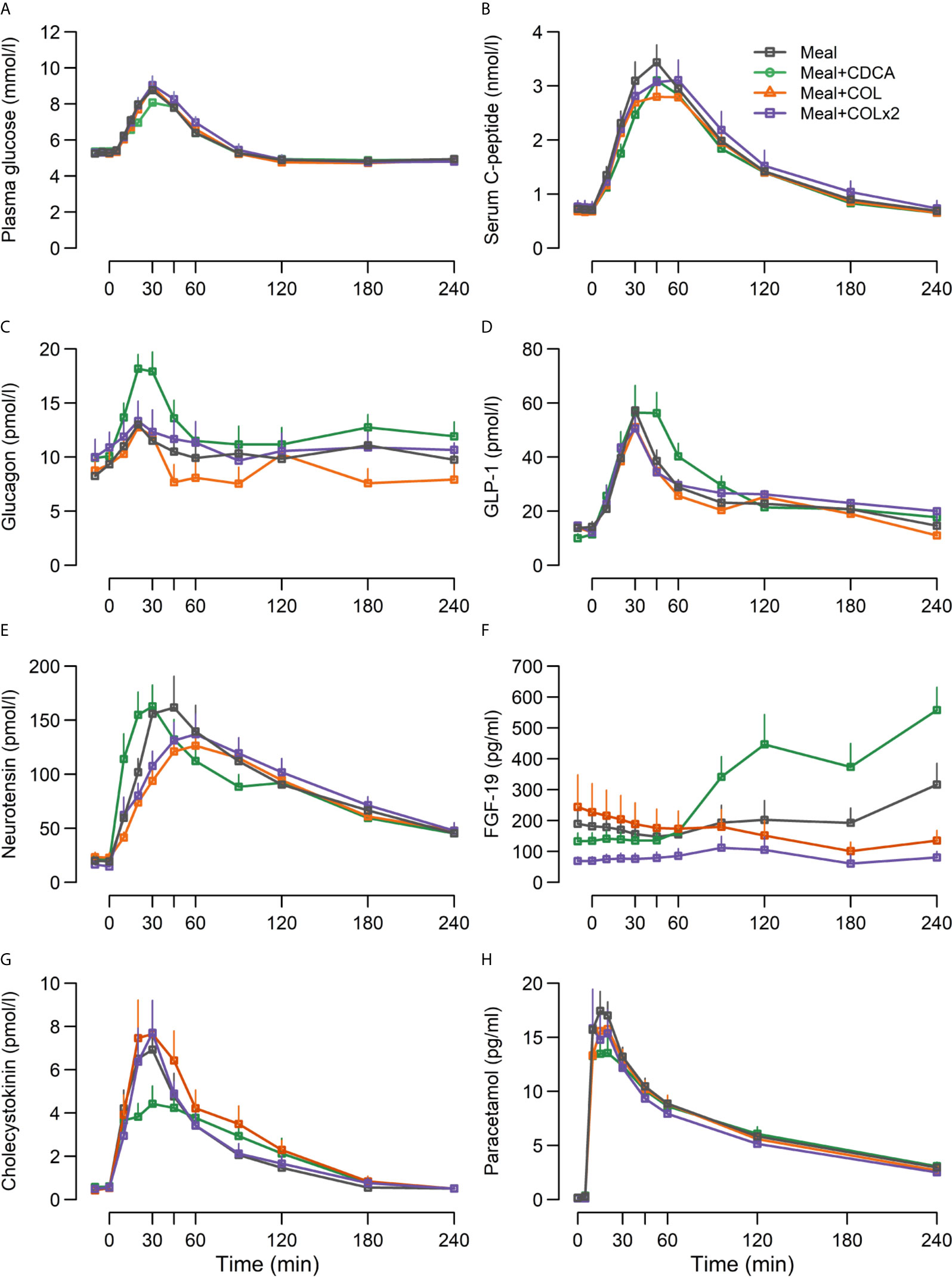
Figure 3 Concentrations of (A) plasma glucose, (B) serum C-peptide, (C) glucagon, (D) GLP-1, (E) neurotensin, (F) FGF-19, (G) CCK and (H) paracetamol following ingestion of a mixed meal alone (Meal), mixed meal in combination with CDCA with the meal (Meal + CDCA), mixed meal in combination with COL added to the meal (Meal + COL), or mixed meal in combination with COL administered both the night before the study day as tablets and added to the meal (Meal + COL × 2), n = 12. Data are mean ± SEM.
Plasma Glucose, Serum C-Peptide, Glucagon, FGF-19 and Paracetamol
Fasting plasma glucose, insulin, and HOMA-IR did not differ between any of the study days (Figure 3, Table 4).
Adding CDCA to the meal tended to decrease piAUC of plasma glucose concentrations compared with meal alone (p = 0.07) and lowered peak of plasma glucose concentrations by ~0.5 mM (p = 0.03). In contrast, adding COL to the meal did not change plasma glucose, neither with single nor double COL dosing. Time to nadir and nadir of plasma glucose did not differ between study days.
The piAUC of C-peptide concentrations decreased slightly (7%) during meal + CDCA compared with meal alone (p = 0.04), whereas neither doses of COL changed C-peptide concentrations. Peak concentrations of glucagon increased when CDCA was added to the meal and were unaffected by COL.
FGF-19 concentrations increased during meal + CDCA compared with meal alone (p < 0.01 for both piAUC and peak) and tended to decrease during COL administration (ANOVA p = 0.14 and 0.12 for peak and piAUC, respectively) (Figure 3, Table 4).
Time to peak of PCM increased numerically when CDCA was added to the meal and time to peak was unaffected by COL (Figure 3, Table 4).
Measures of Beta-Cell Function
IGIC-peptide was unaffected both by adding CDCA and by both dosages of COL. The beta-cell index covering the whole postprandial period decreased slightly during CDCA and was unaffected by COL (Table 4).
Discussion
We investigated the effect of exogenous CDCA and the importance of endogenous bile acids for postprandial plasma GLP-1 concentrations and for plasma glucose, insulin, glucagon, CCK, neurotensin, and FGF-19 concentrations in individuals that had undergone RYGB by acute administration of CDCA and the bile acid sequestrant COL.
First, we administered CDCA alone or together with COL to RYGB operated participants in the absence of nutrients. This confirmed that CDCA elicits a rise in plasma GLP-1, glucagon, neurotensin, cholecystokinin, and FGF-19 concentrations. This was reported previously both in RYGB-operated (38) and in non-operated subjects after either gastric (15–17) or rectal administration (12, 13). The combined administration of CDCA and COL attenuated both plasma TBA, GLP-1, glucagon, neurotensin, C-peptide, and FGF-19 responses as compared with CDCA alone. Since bile acids stimulate GLP-1 secretion via activation of basolaterally located TGR-5 receptors on the L-cell (27, 28), absorption of bile acids across the gut epithelium is required for stimulation of GLP-1 secretion (11). Accordingly, the attenuated bile acid absorption and GLP-1 secretion after combined administration of CDCA and COL in study 1 is consistent with this notion and confirms findings from un-operated subjects (15, 39). Importantly, these results also demonstrate that bile acid sequestrants provide a suitable experimental tool for investigations of the physiological effects of meal-induced bile acid absorption also in RYGB-operated patients. Interestingly, the rise in glucagon and neurotensin concentrations seen during CDCA was also attenuated after administration of COL. The responses of neurotensin to CDCA likely involve similar mechanisms as for GLP-1 (27), whereas stimulation of glucagon secretion by CDCA probably involves other mechanisms. The presence of TGR-5 receptors on pancreatic beta and alpha cells is debated, and the stimulatory effects of glucagon by CDCA may be either indirectly or of intestinal origin (27, 40, 41). The CDCA induced glucagon response could be specific to RYGB given the well documented postprandial hyperglucagonemia due to de novo synthesis in the small intestine (26, 40).
Of note, the magnitude of the GLP-1 response obtained after CDCA in study 1 and our previous study (38) was accompanied by a small albeit significant increase in C-peptide without changes in glucose concentrations. When plasma glucose concentrations are within the fasting range, the potentiating effect of GLP-1 on insulin secretion is limited per se (42, 43), and therefore the insulinotropic effect of CDCA mediated GLP-1 secretion was expected to be small.
After RYGB, the postprandial GLP-1 secretion is many-fold increased and the contribution of bile acids to this response has been debated but not directly examined previously. Therefore, in study 2 we investigated postprandial GLP-1 concentrations after addition of an exogenous bile acid (CDCA) to a meal or after inhibiting endogenous bile acid reabsorption with COL in RYGB operated subjects. We found that CDCA increased both postprandial TBA concentrations and stimulated overall GLP-1 secretion, indicating that the GLP-1 secreting L-cells were not maximally stimulated by the meal ingestion alone. Also, peak glucagon concentrations were higher during CDCA administration. Meanwhile, both plasma glucose and C-peptide responses were slightly decreased, and no beneficial effect on beta-cell function was found despite the increased GLP-1 secretion. This finding is surprising, but may be explained by lower peak concentrations of plasma glucose after CDCA, which might be mediated by effects of CDCA on intestinal nutrient entry or glucose absorption although time to peak of paracetamol concentrations, reflecting rate of nutrient absorption, was only numerically increased after CDCA. Notable, bile acids have been suggested to be involved in the pathogenesis of postprandial hypoglycemia via the bile acids-GLP-1-insulin-axis or indirectly via stimulation of FGF-19. Subjects developing postprandial hypoglycemia have been shown to have higher postprandial bile acids levels coinciding with augmented GLP-1 and insulin responses during a mixed meal (44). However, the present study does not support a role for acutely altered intestinal bile acids concentrations for the development of postprandial hypoglycemia as no differences in nadir or in time to nadir of plasma glucose concentrations were found between the four days with mixed meal and differences in plasma bile acid concentrations.
Administration of COL together with the meal decreased postprandial plasma concentrations of TBA with ~68%, indicative of reduced postprandial bile acid absorption, as intended. We added COL in two dosage regimens based on the recent findings with altered bile acid circulation (33), and the extra dose of COL was added to secure binding of the bile acids within the gut lumen already before food ingestion. However, the results obtained from the two different dosage regimens of COL were comparable including fasting bile acid concentrations, which suggests that insufficient inhibition of absorption is not a concern with the single dose of COL. The large decrease in TBA concentrations after COL administration did not affect GLP-1 secretion, which suggests that endogenous bile acids do not appear to be quantitatively important for the total postprandial GLP-1 response after RYGB despite the fact that exogenous CDCA can increase plasma GLP-1 concentrations after RYGB. This difference might be driven by differences in plasma TBA concentrations, which were substantially (nine-fold) higher after adding CDCA suggesting that the levels of endogenous BAs stimulated by meal intake did not have a similar stimulatory capacity. Moreover, CDCA is known to be a relatively good agonist for TGR-5, which is not the case for all endogenous bile acids (27). In previous studies the composition of the different bile acids was not changed after surgery (24, 25) contradicting any special impact of CDCA or other subfractions for the metabolic improvements after RYGB.
The immediate effects of the acute interventions in our studies were anticipated to affect glucose metabolism and GLP-1 secretion mainly through TGR-5 activation. However, bile acids may also affect these axes via the FXR pathway although the precise contribution remains controversial and is unlikely to contribute to postprandial responses within the first hour after meal intake, since FXR is a nuclear receptor and responses depend on transcriptional changes (45). FXR activation is supposed to stimulate release of FGF-19, suppress de-novo bile acid synthesis, stimulate glycogen synthesis, reduce hepatic gluconeogenesis, inhibit gall bladder emptying, and may also regulate insulin sensitivity (46, 47). In both our studies we observed an increase in FGF-19 concentrations with peak values occurring after the 120 min postprandial timepoint in line with previous studies (25, 48). The observed increase in FGF19 was greatly enhanced by adding CDCA to the meal confirming a link between CDCA and activation of the FXR pathway (49). GLP-1 concentrations peak within the first postprandial hour after RYGB, why any acute effects of bile acids on postprandial GLP-1 and insulin secretion seem independent of FXR activation. This is consistent with findings in some rodent models where activation of the FXR-pathway does not stimulate GLP-1 secretion and may even decrease secretion (18, 27). However, results from other pre-clinical models have opposing findings with increased GLP-1 secretion (50, 51). In addition, targeted knockout of the FXR receptors in mice eliminated the beneficial effects of sleeve gastrectomy on glucose metabolism (52), but this finding can be unrelated to GLP-1 secretion and may also be procedure or species specific. We observed a tendency of decreased FGF-19 concentrations during both doses of COL and GLP-1 secretion was unchanged on these days as mentioned above. In general, the role of FXR activation after bariatric surgery in humans remains unknown and further studies are needed to determine the contribution of FXR activity for the changed glucose metabolism, gut hormone secretion and insulin sensitivity after surgery. With a more chronic treatment with bile acid sequestrants, results might differ with accumulated effects on glucose metabolism and gut hormone secretion by manipulating both TGR5 and FXR activation with more efficient binding of bile acids. Especially, effects from changed FXR activation would be expected to be more pronounced in line with findings in un-operated subjects with type 2 diabetes (53).
The relation between bile acids and CCK in RYGB operated subjects appears to be complex. In unoperated individuals the effect of CDCA (15, 16) and bile acids (54) on CCK secretion was inconsistent, whereas liquid mixed meal tests after adding bile acid sequestrants were associated with increased CCK secretion (53, 55). In the meal study, CCK responses were unaltered by both CDCA and colesevelam. The discrepancy between findings in RYGB-operated and unoperated individuals might involve the apparent dissociation of the normal physiological CCK-mediated regulation of gallbladder emptying and postprandial bile acid concentrations after RYGB, where a larger pool of the bile acids is located within the small intestine in the fasting state, attenuating the importance of postprandial gallbladder emptying (33).
Overall, our findings do not support a major role for endogenous bile acids for the altered postprandial secretions of gastrointestinal hormones or glucose metabolism in RYGB operated subjects. In the present studies, we were unable to identify changes in GLP-1 or measures of beta-cell function after acute attenuation of postprandial bile acid absorption by the bile acid sequestrant COL, although the conclusions based on these finding may be limited by the acute dosage regimen and the inclusion of subjects without type 2 diabetes. Our study also seems to contrast data suggesting that the FXR pathway is crucial for glucose tolerance after bariatric surgery (52), although studies with longer and more chronic administration of sequestrants are probably needed to establish this.
In conclusion, administration of the bile acid sequestrant COL to RYGB operated subjects effectively reduced CDCA-induced circulating bile acids and GLP-1. Further, CDCA administered during meal intake enhanced GLP-1 secretion and decreased both peak glucose and C-peptide concentrations with unaltered beta-cell function. COL added to a mixed meal effectively blocked the absorption of bile acids but neither affected GLP-1, glucose concentrations nor beta cell function, suggesting a limited role for endogenous bile acids in the acute regulation of postprandial glucose metabolism after RYGB.
Data Availability Statement
The original contributions presented in the study are included in the article. Further inquiries can be directed to the corresponding authors.
Ethics Statement
The studies involving human participants were reviewed and approved by the Ethical Committee of the Capital Region of Denmark. The patients/participants provided their written informed consent to participate in this study.
Author Contributions
IJ: Investigation, formal analysis, and writing—original draft. KB-M: Conceptualization, methodology, and writing—review and editing. VK: Resources and writing—review and editing. SV: Resources and writing—review and editing. NW: Resources and writing—review and editing. TC: Resources and writing—review and editing. RK: Resources and writing—review and editing. JR: Resources and writing—review and editing. JH: Conceptualization, methodology, writing—review and editing, and funding acquisition. SM: Conceptualization, methodology, writing—review and editing, and supervision. MS: Conceptualization, methodology, formal analysis, writing—original draft, and visualization. All authors contributed to the article and approved the submitted version.
Funding
This project has received funding from the European Research Council (ERC) under the European Union’s Horizon 2020 research and innovation program (grant agreement No 695069-BYPASSWITHOUTSURGERY) and from Amager and Hvidovre Hospital Research Foundation.
Conflict of Interest
RK works for Novo Nordisk A/S, but was at the time of his contribution exclusively employed by University of Copenhagen. TC works for Novo Nordisk A/S and own shares in Novo Nordisk A/S and Zealand Pharma A/S. All other authors declare no conflict of interest in relation to the current manuscript.
Acknowledgments
We thank Alis Sloth Andersen and Sussi Polman (Department of Endocrinology, Hvidovre University Hospital) for the professional help and guidance in the laboratory. Gitte Kølander Hansen from the Department of Obesity Biology, Novo Nordisk A/S in Måløv, Denmark is acknowledged for her expert technical assistance. We also thank Lene Albæk from the University of Copenhagen.
Abbreviations
BA, Bile acids; CCK, Cholecystokinin; CDCA, Chenodeoxycholic acid; COL, Colesevelam; FGF-19, Fibroblast growth factor 19; GLP-1, Glucagon-like peptide-1; piAUC, positive incremental area-under-the-curve; RYGB, Roux-en-Y gastric bypass; TBA, Total bile acids; VAS, Visual analogue scale.
References
1. Mingrone G, Panunzi S, De Gaetano A, Guidone C, Iaconelli A, Capristo E, et al. Metabolic Surgery Versus Conventional Medical Therapy in Patients With Type 2 Diabetes: 10-Year Follow-Up of an Open-Label, Single-Centre, Randomised Controlled Trial. Lancet (2021) 397:293–304. doi: 10.1016/S0140-6736(20)32649-0
2. Schauer PR, Mingrone G, Ikramuddin S, Wolfe B. Clinical Outcomes of Metabolic Surgery: Efficacy of Glycemic Control, Weight Loss, and Remission of Diabetes. Diabetes Care (2016) 39:902–11. doi: 10.2337/dc16-0382
3. Pories WJ, Swanson MS, MacDonald KG, Long SB, Morris PG, Brown BM, et al. Who Would Have Thought it? An Operation Proves to be the Most Effective Therapy for Adult-Onset Diabetes Mellitus. Ann Surg (1995) 222:332–9. doi: 10.1097/00000658-199509000-00011
4. Madsbad S, Dirksen C, Holst JJ. Mechanisms of Changes in Glucose Metabolism and Bodyweight After Bariatric Surgery. Lancet Diabetes Endocrinol (2014) 2:152–64. doi: 10.1016/S2213-8587(13)70218-3
5. Jacobsen SH, Olesen SC, Dirksen C, Jørgensen NB, Bojsen-Møller KN, Kielgast U, et al. Changes in Gastrointestinal Hormone Responses, Insulin Sensitivity, and Beta-Cell Function Within 2 Weeks After Gastric Bypass in non-Diabetic Subjects. Obes Surg (2012) 22:1084–96. doi: 10.1007/s11695-012-0621-4
6. Svane MS, Bojsen-Møller KN, Martinussen C, Dirksen C, Madsen JL, Reitelseder S, et al. Postprandial Nutrient Handling and Gastrointestinal Hormone Secretion After Roux-en-Y Gastric Bypass vs Sleeve Gastrectomy. Gastroenterology (2019) 156:1627–41. doi: 10.1053/j.gastro.2019.01.262
7. Salehi M, Prigeon RL. D’alessio D a. Gastric Bypass Surgery Enhances Glucagon-Like Peptide 1-Stimulated Postprandial Insulin Secretion in Humans. Diabetes (2011) 60:2308–14. doi: 10.2337/db11-0203
8. Svane MS, Jørgensen NB, Bojsen-Møller KN, Dirksen C, Nielsen S, Kristiansen VB, et al. Peptide YY and Glucagon-Like Peptide-1 Contribute to Decreased Food Intake After Roux-en-Y Gastric Bypass Surgery. Int J Obes (Lond) (2016) 40:1699–706. doi: 10.1038/ijo.2016.121
9. Jørgensen NB, Dirksen C, Bojsen-Møller KN, Jacobsen SH, Worm D, Hansen DL, et al. Exaggerated Glucagon-Like Peptide 1 Response is Important for Improved β-Cell Function and Glucose Tolerance After Roux-en-Y Gastric Bypass in Patients With Type 2 Diabetes. Diabetes (2013) 62:3044–52. doi: 10.2337/db13-0022
10. Katsuma S, Hirasawa A, Tsujimoto G. Bile Acids Promote Glucagon-Like Peptide-1 Secretion Through TGR5 in a Murine Enteroendocrine Cell Line STC-1. Biochem Biophys Res Commun (2005) 329:386–90. doi: 10.1016/j.bbrc.2005.01.139
11. Brighton CA, Rievaj J, Kuhre RE, Glass LL, Schoonjans K, Holst JJ, et al. Bile Acids Trigger GLP-1 Release Predominantly by Accessing Basolaterally Located G Protein-Coupled Bile Acid Receptors. Endocrinology (2015) 156:3961–70. doi: 10.1210/en.2015-1321
12. Adrian TE, Gariballa S, Parekh KA, Thomas SA, Saadi H, Al Kaabi J, et al. Rectal Taurocholate Increases L Cell and Insulin Secretion, and Decreases Blood Glucose and Food Intake in Obese Type 2 Diabetic Volunteers. Diabetologia (2012) 55:2343–7. doi: 10.1007/s00125-012-2593-2
13. Wu T, Bound MJ, Standfield SD, Gedulin B, Jones KL, Horowitz M, et al. Effects of Rectal Administration of Taurocholic Acid on Glucagon-Like Peptide-1 and Peptide YY Secretion in Healthy Humans. Diabetes Obes Metab (2013) 15:474–7. doi: 10.1111/dom.12043
14. Adrian TE, Ballantyne GH, Longo WE, Bilchik AJ, Graham S, Basson MD, et al. Deoxycholate is an Important Releaser of Peptide YY and Enteroglucagon From the Human Colon. Gut (1993) 34:1219–24. doi: 10.1136/gut.34.9.1219
15. Hansen M, Scheltema MJ, Sonne DP, Hansen JS, Sperling M, Rehfeld JF, et al. Effect of Chenodeoxycholic Acid and the Bile Acid Sequestrant Colesevelam on Glucagon-Like Peptide-1 Secretion. Diabetes Obes Metab (2016) 18:571–80. doi: 10.1111/dom.12648
16. Meyer-Gerspach AC, Steinert RE, Keller S, Malarski A, Schulte FH, Beglinger C. Effects of Chenodeoxycholic Acid on the Secretion of Gut Peptides and Fibroblast Growth Factors in Healthy Humans. J Clin Endocrinol Metab (2013) 98:3351–8. doi: 10.1210/jc.2012-4109
17. Wu T, Bound MJ, Standfield SD, Jones KL, Horowitz M, Rayner CK. Effects of Taurocholic Acid on Glycemic, Glucagon-Like peptide-1, and Insulin Responses to Small Intestinal Glucose Infusion in Healthy Humans. J Clin Endocrinol Metab (2013) 98:718–22. doi: 10.1210/jc.2012-3961
18. Trabelsi M-S, Daoudi M, Prawitt J, Ducastel S, Touche V, Sayin SI, et al. Farnesoid X Receptor Inhibits Glucagon-Like Peptide-1 Production by Enteroendocrine L-Cells. Nat Commun (2015) 6:7629. doi: 10.1038/ncomms8629.Farnesoid
19. Pathak P, Xie C, Nichols RG, Ferrell JM, Boehme S, Krausz KW, et al. Intestine Farnesoid X Receptor Agonist and the Gut Microbiota Activate G-protein Bile Acid Receptor-1 Signaling to Improve Metabolism. Hepatology (2018) 68:1574–88. doi: 10.1002/hep.29857
20. Pournaras DJ, Glicksman C, Vincent RP, Kuganolipava S, Alaghband-Zadeh J, Mahon D, et al. The Role of Bile After Roux-en-Y Gastric Bypass in Promoting Weight Loss and Improving Glycaemic Control. Endocrinology (2012) 153:3613–9. doi: 10.1210/en.2011-2145
21. Nakatani H, Kasama K, Oshiro T, Watanabe M, Hirose H, Itoh H. Serum Bile Acid Along With Plasma Incretins and Serum High-Molecular Weight Adiponectin Levels are Increased After Bariatric Surgery. Metabolism (2009) 58:1400–7. doi: 10.1016/j.metabol.2009.05.006
22. Dutia R, Embrey M, O’Brien CS, O’Brien S, Haeusler RA, Agénor KK, et al. Temporal Changes in Bile Acid Levels and 12α-Hydroxylation After Roux-en-Y Gastric Bypass Surgery in Type 2 Diabetes. Int J Obes (2016) 40:806–13. doi: 10.1038/ijo.2015.1
23. Gerhard GS, Styer AM, Wood GC, Roesch SL, Petrick AT, Gabrielsen J, et al. A Role for Fibroblast Growth Factor 19 and Bile Acids in Diabetes Remission After Roux-en-y Gastric Bypass. Diabetes Care (2013) 36:1859–64. doi: 10.2337/dc12-2255
24. Steinert RE, Peterli R, Keller S, Meyer-Gerspach AC, Drewe J, Peters T, et al. Bile Acids and Gut Peptide Secretion After Bariatric Surgery: A 1-Year Prospective Randomized Pilot Trial. Obes (Silver Spring) (2013) 21:E660–8. doi: 10.1002/oby.20522
25. Jørgensen NB, Dirksen C, Bojsen-Møller KN, Kristiansen VB, Wulff BS, Rainteau D, et al. Improvements in Glucose Metabolism Early After Gastric Bypass Surgery are Not Explained by Increases in Total Bile Acids and Fibroblast Growth Factor 19 Concentrations. J Clin Endocrinol Metab (2015) 100:E396–406. doi: 10.1210/jc.2014-1658
26. Jorgensen NB, Jacobsen SH, Dirksen C, Bojsen-Moller KN, Naver L, Hvolris L, et al. Acute and Long-Term Effects of Roux-en-Y Gastric Bypass on Glucose Metabolism in Subjects With Type 2 Diabetes and Normal Glucose Tolerance. AJP Endocrinol Metab (2012) 303:E122–31. doi: 10.1152/ajpendo.00073.2012
27. Kuhre RE, Wewer Albrechtsen NJ, Larsen O, Jepsen SL, Balk-Møller E, Andersen DB, et al. Bile Acids are Important Direct and Indirect Regulators of the Secretion of Appetite- and Metabolism-Regulating Hormones From the Gut and Pancreas. Mol Metab (2018) 11:84–95. doi: 10.1016/j.molmet.2018.03.007
28. Kawamata Y, Fujii R, Hosoya M, Harada M, Yoshida H, Miwa M, et al. A G Protein-Coupled Receptor Responsive to Bile Acids. J Biol Chem (2003) 278:9435–40. doi: 10.1074/jbc.M209706200
29. Donovan JM, Von Bergmann K, Setchell KDR, Isaacsohn J, Pappu AS, Illingworth DR, et al. Effects of Colesevelam HCl on Sterol and Bile Acid Excretion in Patients With Type IIa Hypercholesterolemia. Dig Dis Sci (2005) 50:1232–8. doi: 10.1007/s10620-005-2765-8
30. Staels B, Kuipers F. Bile Acid Sequestrants and the Treatment of Type 2 Diabetes Mellitus. Drugs (2007) 67:1383–92. doi: 10.2165/00003495-200767100-00001
31. Ullmer C, Alvarez Sanchez R, Sprecher U, Raab S, Mattei P, Dehmlow H, et al. Systemic Bile Acid Sensing by G Protein-Coupled Bile Acid Receptor 1 (GPBAR1) Promotes PYY and GLP-1 Release. Br J Pharmacol (2013) 169:671–84. doi: 10.1111/bph.12158
32. Grunddal KV, Ratner CF, Svendsen B, Sommer F, Engelstoft MS, Madsen AN, et al. Neurotensin is Coexpressed, Coreleased, and Acts Together With GLP-1 and PYY in Enteroendocrine Control of Metabolism. Endocrinology (2016) 157:176–94. doi: 10.1210/en.2015-1600
33. Eiken A, Fuglsang S, Eiken M, Svane MS, Kuhre RE, Wewer Albrechtsen NJ, et al. Bilio-Enteric Flow and Plasma Concentrations of Bile Acids After Gastric Bypass and Sleeve Gastrectomy. Int J Obes (Lond) (2020) 44:1872–83. doi: 10.1038/s41366-020-0578-7
34. de Siqueira Cardinelli C, Torrinhas RS, Sala P, Pudenzi MA, Fernando F Angolini C, Marques da Silva M, et al. Fecal Bile Acid Profile After Roux-en-Y Gastric Bypass and its Association With the Remission of Type 2 Diabetes in Obese Women: A Preliminary Study. Clin Nutr (2019) 38:2906–12. doi: 10.1016/j.clnu.2018.12.028
35. Orskov C, Rabenhøj L, Wettergren A, Kofod H, Holst JJ. Tissue and Plasma Concentrations of Amidated and Glycine-Extended Glucagon-Like Peptide I in Humans. Diabetes (1994) 43:535–9. doi: 10.2337/diab.43.4.535
36. Kuhre RE, Bechmann LE, Wewer Albrechtsen NJ, Hartmann B, Holst JJ. Glucose Stimulates Neurotensin Secretion From the Rat Small Intestine by Mechanisms Involving SGLT1 and GLUT2, Leading to Cell Depolarization and Calcium Influx. Am J Physiol Metab (2015) 308:E1123–30. doi: 10.1152/ajpendo.00012.2015
37. Rehfeld JF. Accurate Measurement of Cholecystokinin in Plasma. Clin Chem (1998) 44:991–1001. doi: 10.1136/jcp.s1-8.1.26
38. Nielsen S, Svane MS, Kuhre RE, Clausen TR, Kristiansen VB, Rehfeld JF, et al. Chenodeoxycholic Acid Stimulates Glucagon-Like Peptide-1 Secretion in Patients After Roux-en-Y Gastric Bypass. Physiol Rep (2017) 5:e13140. doi: 10.14814/phy2.13140
39. Brønden A, Albér A, Rohde U, Gasbjerg LS, Rehfeld JF, Holst JJ, et al. The Bile Acid-Sequestering Resin Sevelamer Eliminates the Acute GLP-1 Stimulatory Effect of Endogenously Released Bile Acids in Patients With Type 2 Diabetes. Diabetes Obes Metab (2018) 20:362–9. doi: 10.1111/dom.13080
40. Jorsal T, Wewer Albrechtsen NJ, Christensen MM, Mortensen B, Wandall E, Langholz E, et al. Investigating Intestinal Glucagon After Roux-en-Y Gastric Bypass Surgery. J Clin Endocrinol Metab (2019) 104:6403–16. doi: 10.1210/jc.2019-00062
41. Kumar DP, Rajagopal S, Mahavadi S, Mirshahi F, Grider JR, Murthy KS, et al. Activation of Transmembrane Bile Acid Receptor TGR5 Stimulates Insulin Secretion in Pancreatic β Cells. Biochem Biophys Res Commun (2012) 427:600–5. doi: 10.1016/j.bbrc.2012.09.104
42. Toft-Nielsen MB, Madsbad S, Holst JJ. The Effect of Glucagon-Like Peptide I (Glp-I) on Glucose Elimination in Healthy Subjects Depends on the Pancreatic Glucoregulatory Hormones. Diabetes (1996) 45:552–6. doi: 10.2337/diab.45.5.552
43. Vilsbøll T, Krarup T, Madsbad S, Holst JJ. Both GLP-1 and GIP are Insulinotropic At Basal and Postprandial Glucose Levels and Contribute Nearly Equally to the Incretin Effect of a Meal in Healthy Subjects. Regul Pept (2003) 114:115–21. doi: 10.1016/s0167-0115(03)00111-3
44. van den Broek M, de Heide LJM, Sips FLP, Koehorst M, van Zutphen T, Emous M, et al. Altered Bile Acid Kinetics Contribute to Postprandial Hypoglycaemia After Roux-en-Y Gastric Bypass Surgery. Int J Obes (2021) 45:619–30. doi: 10.1038/s41366-020-00726-w
45. Brønden A, Knop FK. Gluco-Metabolic Effects of Pharmacotherapy-Induced Modulation of Bile Acid Physiology. J Clin Endocrinol Metab (2020) 105:362–73. doi: 10.1210/clinem/dgz025
46. Bozadjieva N, Heppner KM, Seeley RJ. Targeting FXR and FGF19 to Treat Metabolic Diseases-Lessons Learned From Bariatric Surgery. Diabetes (2018) 67:1720–8. doi: 10.2337/dbi17-0007
47. Ma K, Saha PK, Chan L, Moore DD. Farnesoid X Receptor is Essential for Normal Glucose Homeostasis. J Clin Invest (2006) 116:1102–9. doi: 10.1172/JCI25604
48. Albaugh VL, Flynn CR, Cai S, Xiao Y, Tamboli RA, Abumrad NN. Early Increases in Bile Acids Post Roux-En-Y Gastric Bypass Are Driven by Insulin-Sensitizing, Secondary Bile Acids. J Clin Endocrinol Metab (2015) 100:E1225–33. doi: 10.1210/jc.2015-2467
49. Ma H, Patti ME. Bile Acids, Obesity, and the Metabolic Syndrome. Best Pract Res Clin Gastroenterol (2014) 28:573–83. doi: 10.1016/j.bpg.2014.07.004
50. Chen L, McNulty J, Anderson D, Liu Y, Nystrom C, Bullard S, et al. Cholestyramine Reverses Hyperglycemia and Enhances Glucose-Stimulated Glucagon-Like Peptide 1 Release in Zucker Diabetic Fatty Rats. J Pharmacol Exp Ther (2010) 334:164–70. doi: 10.1124/jpet.110.166892
51. Shang Q, Saumoy M, Holst JJ, Salen G, Xu G. Colesevelam Improves Insulin Resistance in a Diet-Induced Obesity (F-DIO) Rat Model by Increasing the Release of GLP-1. Am J Physiol Gastrointest Liver Physiol (2010) 298:419–24. doi: 10.1152/ajpgi.00362.2009
52. Ryan KK, Tremaroli V, Clemmensen C, Kovatcheva-Datchary P, Myronovych A, Karns R, et al. FXR is a Molecular Target for the Effects of Vertical Sleeve Gastrectomy. Nature (2014) 509:183–8. doi: 10.1038/nature13135
53. Brønden A, Mikkelsen K, Sonne DP, Hansen M, Våben C, Gabe MN, et al. Glucose-Lowering Effects and Mechanisms of the Bile Acid-Sequestering Resin Sevelamer. Diabetes Obes Metab (2018) 20:1623–31. doi: 10.1111/dom.13272
54. Koide M, Okabayashi Y, Otsuki M. Role of Endogenous Bile on Basal and Postprandial CCK Release in Humans. Dig Dis Sci (1993) 38:1284–90. doi: 10.1007/bf01296080
Keywords: bile acids, colesevelam, RYGB, Roux-en-Y gastric bypass, glucagon-like peptide 1
Citation: Jonsson I, Bojsen-Møller KN, Kristiansen VB, Veedfald S, Wewer Albrechtsen NJ, Clausen TR, Kuhre RE, Rehfeld JF, Holst JJ, Madsbad S and Svane MS (2021) Effects of Manipulating Circulating Bile Acid Concentrations on Postprandial GLP-1 Secretion and Glucose Metabolism After Roux-en-Y Gastric Bypass. Front. Endocrinol. 12:681116. doi: 10.3389/fendo.2021.681116
Received: 15 March 2021; Accepted: 16 April 2021;
Published: 14 May 2021.
Edited by:
Peter Flatt, Ulster University, United KingdomReviewed by:
Mark Walker, Newcastle University, United KingdomNeil G. Docherty, University College Dublin, Ireland
Copyright © 2021 Jonsson, Bojsen-Møller, Kristiansen, Veedfald, Wewer Albrechtsen, Clausen, Kuhre, Rehfeld, Holst, Madsbad and Svane. This is an open-access article distributed under the terms of the Creative Commons Attribution License (CC BY). The use, distribution or reproduction in other forums is permitted, provided the original author(s) and the copyright owner(s) are credited and that the original publication in this journal is cited, in accordance with accepted academic practice. No use, distribution or reproduction is permitted which does not comply with these terms.
*Correspondence: Sten Madsbad, U3Rlbi5NYWRzYmFkQHJlZ2lvbmguZGs=
Maria S. Svane, TWFyaWEuU2F1ci5TdmFuZUByZWdpb25oLmRr
†These authors share last authorship