- 1Millennium Institute on Immunology and Immunotherapy, Facultad de Ciencias de la Vida, Departamento de Ciencias Biológicas, Universidad Andres Bello, Santiago, Chile
- 2Instituto de Ciencias Naturales, Facultad de Medicina Veterinaria y Agronomía, Universidad de las Américas, Santiago, Chile
- 3Millennium Institute on Immunology and Immunotherapy, Departamento de Genética Molecular y Microbiología, Facultad de Ciencias Biológicas, Pontificia Universidad Católica de Chile, Santiago, Chile
- 4Departamento de Endocrinología, Facultad de Medicina, Escuela de Medicina, Pontificia Universidad Católica de Chile, Santiago, Chile
Fetus and infants require appropriate thyroid hormone levels and iodine during pregnancy and lactation. Nature endorses the mother to supply thyroid hormones to the fetus and iodine to the lactating infant. Genetic variations on thyroid proteins that cause dyshormonogenic congenital hypothyroidism could in pregnant and breastfeeding women impair the delivery of thyroid hormones and iodine to the offspring. The review discusses maternal genetic variations in thyroid proteins that, in the context of pregnancy and/or breastfeeding, could trigger thyroid hormone deficiency or iodide transport defect that will affect the proper development of the offspring.
Introduction
Pregnancy and lactation are challenging physiological periods due to the mother supplies thyroid hormones and iodine to the fetus and infant, respectively. Given that, there is an increase on iodine and thyroid hormone requirements, a proper iodine status and thyroid hormone levels in pregnant and breastfeeding women must be guaranteed (1). Unfortunately, iodine insufficiency and thyroid hormone (TH) deficiency are frequent in pregnancy and breastfeeding (2). The statistics showed that 2.5% of pregnant and breastfeeding women consume insufficient iodine in their diet (3) and the prevalence of hypothyroidism is about 2%–3% in pregnant women (4). The progeny gestated and/or lactated under iodine deficiency or thyroid hormone deficiency, like hypothyroidism or hypothyroxinemia, is at higher risk of suffering irreversible cognitive impairment like attentional deficit, low intelligent quotient, and intellectual disability (5). Women carrying thyroid gene variations could be more sensitive to suffer thyroid hormone deficiency during pregnancy and/or iodide transport defect (ITD) during lactation. Special attention are those women that were diagnosed at birth with transient congenital hypothyroidism (TCH). TCH is a temporary deficiency of thyroid hormones identified after birth (6). TCH has several different causes like endemic iodine deficiency, iodine excess, maternal antithyroid medication, maternal antibodies, and genetics. This review will focus on thyroid gene variations that will risk the pregnant or lactating women to suffer thyroid hormone deficiency or ITD during pregnancy and/or lactation (7, 8). We will review these thyroid gene variations reported in the literature that caused dyshormonogenic TCH. The point of this review is to provide awareness to scientists and physicians to prevent thyroid hormone deficiency and ITD during pregnancy and lactation in those women diagnosed with dyshormonogenic TCH. These maternal genetic mutations on thyroid proteins could irreversibly damage the appropriate development of the offspring.
Functional Maternal Thyroid Proteins Are Needed for Fetus Development
In the first 5 months of pregnancy, the maternal thyroid gland will be challenged to supply THs to the fetus, especially T4 (9, 10). Thus, the mother will synthesize THs for her own needs and the fetus (Figure 1) (11, 12). This new scenery causes physiological stress to the maternal thyroid gland. To overcome this challenge, the pregnant woman should increase her daily iodine intake to 250 μg/day. The thyroid-stimulating hormone (TSH) and the human chorionic gonadotropin (hCG) will stimulate the maternal thyroid gland to produce T4 and T3 during the first trimester of pregnancy (13, 14). The hCG shares structural features with TSH (15). hCG and TSH will increase iodine uptake and thyroid hormone synthesis by binding to the TSH receptor (16). TSH and hCG will stimulate maternal thyroid gland growth and the expression of thyroid proteins like Na+/I− symporter (NIS), pendrin (PDS), thyroglobulin (TG), thyroid peroxidase (TPO), dual oxidase 2 (DUOX2), and dual oxidase maturation factor 2 (DUOXA2) (17). The expression of NIS at the basolateral membrane of the thyrocyte will increase the uptake of iodide from the blood into the thyrocyte. Iodide will diffuse through the cytoplasm to the apical side of the thyrocyte, where transporters like PDS, anoctamin-1 (ANO1), and SLA26A7 will transport it to the colloid (18). The thyrocytes also express the cystic fibrosis transmembrane conductance regulator (CFTR) (18). CFTR transports chloride, and more studies are required to evaluate whether CFTR transports iodide. At the colloid, iodide will be oxidized and incorporated in the tyrosyl residues of TG by TPO (18). The organification of TG requires hydrogen peroxide (H2O2) which is supplied by dual oxidase system (DUOX). It has been described that two DUOX enzymes (DUOX1 and DUOX2) and their respective maturation factors (DUOXA1 and DUOXA2). These maturations factors reside at the endoplasmic reticulum, and they are essential for DUOX function (Figure 1). hCG and TSH will stimulate the endocytosis of iodinated TG. In the endo-lysosomes, TG will be digested by proteolytic enzymes like lysosomal dipeptidase, glutamate carboxypeptidase, and cathepsins B, K, L, and S releasing T3 and T4. THs will efflux the thyroid cell to the blood stream mainly by transporters like monocarboxylate transporter (MCT8) (19). It has been reported that certain genetic variations in thyroid proteins affects the function of the thyroid gland (20–22). There are mutations in thyroid genes that cause thyroid dyshormonogenesis, which is responsible for 10%–15% of congenital hypothyroidism (CH) (23). Newborns diagnosed with CH must be treated immediately with T4 to avoid neurological damage (23). Moreover, the etiology of CH should be determined by measurement of serum T3, T4, TSH, and TG and thyroid ultrasonography, thyroid scintigraphy, and perchlorate discharge test should be performed (23, 24). These biochemical and clinical analyses will reveal if the infant suffers transient congenital hypothyroidism (TCH) or permanent congenital hypothyroidism (PCH) (24). Those patients that have PCH will be kept for life with T4 treatment. Instead, thyroid function will be reassessed to those patients diagnosed with TCH at 3 years of age. If their thyroid function has been normalized, the T4 treatment will stop. This situation occurs for patients with TCH given that by progressing in age they require less thyroid hormone production related to their weight and the thyroid gland will be able to overcome the demands (23–26). Moreno et al. emphasize that it is crucial for those women that have been diagnosed with TCH due to monoallelic or biallelic mutations on thyroid proteins to be followed up during pregnancy for the risk of suffering hypothyroidism in this period and therefore will affect the appropriate development of the fetus (23, 27). Thyroid hormone synthesis can be impaired in pregnant women diagnosed at birth with TCH. Even though they were euthyroid before pregnancy, they could fall back in thyroid hormone deficiency due to expressing variants of thyroid protein that are in some degree functionally impaired (28). The thyroid gland of these women during pregnancy could be at risk of dyshormonogenesis endangering the proper development of the fetus. The recommendation of the Endo-European reference network (ERN) that patients with TCH should receive genetic testing to identify mutations causing thyroid dyshormonogenesis is of importance (23, 24). In the next section, the mutations in thyroid proteins that have been described to cause TCH will be discussed.
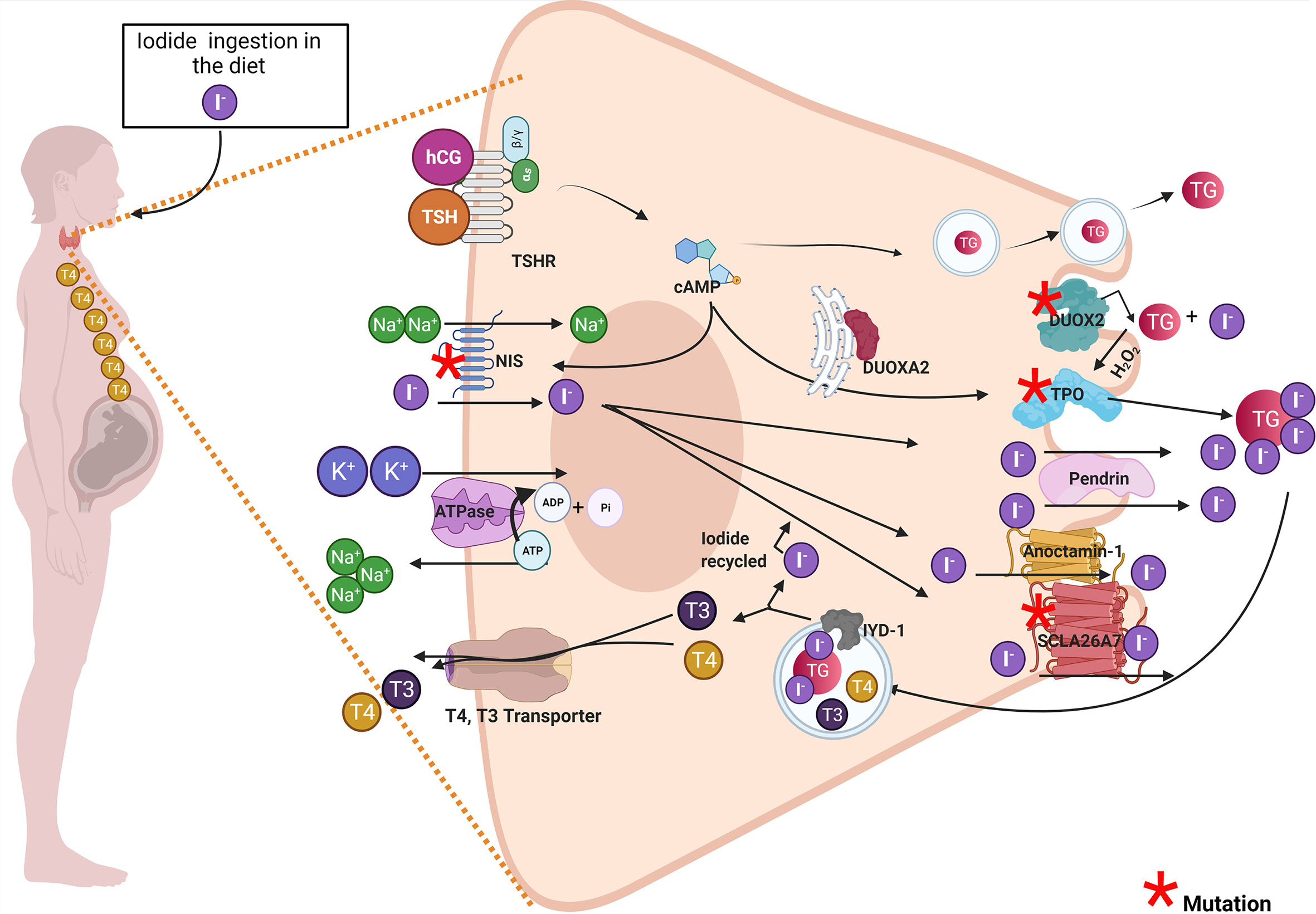
Figure 1 Pregnant woman diagnosed with transient congenital hypothyroidism due to mutations in thyroid proteins could develop thyroid hormone deficiency and impaired the offspring’s development. The drawing shows a thyroid cell of a pregnant woman and the principal thyroid proteins involved in thyroid hormonogenesis. Proteins with red asterisk have been associated in the literature with thyroid dyshormonogenesis in transient congenital hypothyroid patients. Among these proteins are DUOX2, DUOXA2, DUOX1, DUOXA1, TPO, NIS, and SLCA27 (see red asterisk). The maternal thyroid gland must synthesize thyroid hormones for mother and the fetus. The high demand for thyroid hormone synthesis in pregnancy could stress the thyroid gland especially in women carrying genetic variations on thyroid proteins and that were diagnosed with transient congenital hypothyroidism (TCH). These women diagnosed with TCH (carrying monogenic mutations in DUOX2 or DUOXA2 or digenic mutations in DUOX2 and DUOXA1 or mutations in TPO or NIS) that were euthyroid before pregnancy could develop thyroid hormone deficiency like hypothyroidism or hypothyroxinemia during pregnancy. Both hypothyroxinemia and hypothyroidism are thyroid hormone deficiency conditions that risk the proper fetus development and have deleterious consequences in the offspring.
Variations in Maternal Thyroid Genes That Could Impair the Development of the Fetus
It has been described in the literature that variations on thyroid proteins like NIS, DUOX2, DUOXA2, TPO and Tg are responsible for TCH. In this section we briefly discuss the relevance of of these proteins variations in TCH women when they reached pregnancy. The NIS mutants could induce ITD causing TCH or PCH (29). However, the severity and the onset of the disease phenotype will depend on the type of NIS mutation, if it is monoallelic or biallelic, the period of life, and iodide ingestion (30, 31). Therefore, physicians should prevent thyroid hormone deficiency in pregnant women diagnosed with TCH carrying NIS mutations (Figure 1). There are other transporters expressed in the thyroid cells like PDS, ANO-1, SLC26A7, and CFTR (32–34). PDS, ANO-1, and SLC26A7 are localized at the apical side of the thyrocyte, and their physiological role have been associated with iodide efflux into the colloid (35). Mutations on SLC26A4 that encodes PDS has been associated with Pendred syndrome, characterized by congenital bilateral sensorineural hearing loss and in childhood can appear diffuse or multinodular goiter (36). Most of the patients with Pendred syndrome are euthyroid; however, their thyroid function can be impaired if they are exposed to low iodine intake (37). ANO-1 and CFTR variants have not been associated with TCH. A study performed in Saudi Arabia, by using whole-exome sequencing (WES) described mutations in SLC26A7 that could be responsible in part for CH (38). Another thyroid protein variants that have been reported to be responsible for TCH are monoallelic and biallelic mutations of DUOX2 (27, 39, 40). DUOX2 is a transmembrane protein localized at the apical side of the cell, and it belongs to (NADPH)-oxidase family whose function is involved in H2O2 generation (Figure 1). H2O2 is required for thyroid hormone synthesis specifically for iodide organification in tyrosyl residues of TG by TPO (Figure 1) (35). The prevalence of DUOX2 mutations is highly variable in the population. From 2019, approximately 105 DUOX2 mutations are described. Some of them were in-frame deletions, missense, nonsense, splice site, and frameshift mutations (41, 42). Moreover, mutations in the gene DUOXA2 were also reported in TCH patients (43). DUOXA2 is an endoplasmic reticulum transmembrane protein that helps DUOX2 mature through the ER and translocate to the plasma membrane (44). Zamproni et al. found a biallelic mutation in the DUOXA2 gene that leads to a partial iodide organification defect (45) and Liu et al. found a monoallelic missense mutation in DUOXA2 that causes mild TCH (46). Besides, DUOX2 and DUOXA2 thyrocytes also express DUOX1 and DUOXA1. DUOX1 has 83% protein sequence homology with DUOX2; however, its expression is lesser than DUOX2 and its function in the thyroid gland is not clear yet as DUOX1 mutations do not cause CH (47). Aycan et al. described two patients with severe CH that have biallelic mutations in DUOX2 and DUOX1, suggesting that DUOX1 can replace the function of DUOX2 when this enzyme is not available (48). DUOXA1 in similar fashion as DUOXA2 helps DUOX1 to mature through the ER and mutations in DUOXA1 has not been associated yet with CH (47). The possible implication of DUOX1 and DUOXA1 in CH is under intense debate, as the related mutations were reported to be always associated with DUOX2 or DUOXA2 mutants which are the principal proteins involved in the thyroid H2O2-generating system. Interestingly, Wang et al. sequence 16 genes related with CH by next-generation sequence (NGS) in 377 CH patients and found patients with biallelic mutations in DUOX2 and DUOXA1, suggesting that DUOX1 and DUOXA1 could also play a role in thyroid hormone synthesis (49). TPO is responsible for iodide organification and the coupling of tyrosyl residues, essential steps in thyroid hormone synthesis (50). Thus, the expression of TPO variants will severely affect thyroid hormone synthesis (37). In fact, it has been reported that TPO biallelic mutations caused PCH (51). Another study, performed in 243 Russian patients also showed an increased number of variants identified in thyroid dyshormonogenesis-associated genes mostly of the variants found in the TPO gene (52). It has been shown that certain patients with monoallelic mutations in TPO could develop PCH (53, 54), mild hypothyroidism (55), and TCH (56). It has been reported that there are 229 mutations in TG, the precursor of thyroid hormones (57). Some of them occur as biallelic or monoallelic and some are found inherited as monogenic or polygenic (57). A targeted NGS study performed in 19 patients mostly from France with CH due to dyshormonogenesis showed that TG was a site with the higher number of identified mutations followed by DUOXA2 (58). Interestingly, mutations in TG cause euthyroidism to mild or severe CH (57). Variants in iodotyrosine deiodinase (IYD) cause dyshormonogenic PCH, and there are no monoallelic mutations associated to TCH (57). Molecular biology techniques, like NGS and WES will help to understand the genetic component of CH. These molecular techniques like NGS and WES will provide data to fill the complex analysis of gene panels aiming to understand the mechanisms underlying disease development (59). Genetic counseling is recommended for women previously diagnosed with TCH to prevent thyroid hormone deficiency during gestation, and it is highly recommendable to follow thyroid function during pregnancy.
Proper Iodide Nutrition Is an Essential Requirement for Infant Thyroid Hormone Synthesis
Neonates and lactating infants require thyroid hormones for proper physical and neurological development. Even though there are traces of maternal thyroid hormones in the milk, the main source of these hormones for the infant derives from their own thyroid gland (60, 61). Thus, neonates and infants are required to ingest iodine for thyroid hormone synthesis (25). The iodine demand for the newborn is higher than an adult if we ponder their weight (62). Newborns, until 5 months of age need 15 µg/kg/day of iodine, and infants are required 90 µg/day of iodine (63). The newborn thyroid gland has a small iodine reserve close to 100 µg. Therefore, by considering their high demand for this micronutrient, the infants should have a daily intake of iodine (64). Breast milk is an excellent source of iodine; however, its content can vary from 5.4 to 2.170 μg/L (26, 65). Physiologically, iodine content in breast milk will depend mainly on the mother’s iodine consumption being influenced by iodization programs (25, 40). According to the WHO/UNICEF and the International Council for Control of Iodine Deficiency Disorders (ICCIDD), the recommended iodine intake for lactating women is 250 μg/day to ensure 100–150 µg of iodine/dl in the breast milk (26). Iodine is accumulated into the mother’s milk in the form of iodide, and this transport is mediated by NIS that is expressed in the mammary gland physiologically only during lactation (Figure 2) (67). NIS transports iodide against its electrochemical gradient from the blood into lactocytes, and its expression is regulated by oxytocin and prolactin (67). Other transporters like CFTR and ANO-1, that efflux iodide at the thyrocyte, are also expressed in the mammary gland (68, 69). Prolactin also regulates the expression of PDS, an apical thyroid iodide transporter, in lactocytes during lactation (70). The role of these transporters in iodide transport in the mammary gland must yet be elucidated. Iodide inside the lactocytes is oxidized and incorporated into casein by a lactoperoxidase (LPO), forming iodocasein molecules (Figure 2). These iodocasein molecules are aggregated inside micelles and will be secreted into breast milk (Figure 2) (71). The concentration of iodide in breast milk depends on environmental and genetic factors. Women living in regions with insufficient iodine intake are at risk of iodine deficiency during lactation (72). Among the environmental factors are the mother’s diet and the ingestion of thiocyanate and perchlorate (73–75). Perchlorate and thiocyanate are NIS inhibitors of iodide transport (29, 76, 77). Perchlorate is a competitive inhibitor of iodide; Llorente-Esteban et al. showed that the tiny perchlorate addition increases NIS Km for iodide impairing thyroid hormone synthesis (78). Thiocyanate is a potent NIS inhibitor, and it has been described as a noncompetitive inhibitor (76, 79, 80). Therefore, the presence of these molecules in the mother’s diet will reduce the iodide uptake in lactocytes, diminish the concentration of iodide in breast milk, and reduce thyroid hormone synthesis in the infants affecting their development (81). Concerning genetic factors, particular attention should be taken with congenital hypothyroid women because they can carry NIS or LPO variants that impair the accumulation of iodide in breast milk during lactation. In the next section, NIS variants will be discussed.
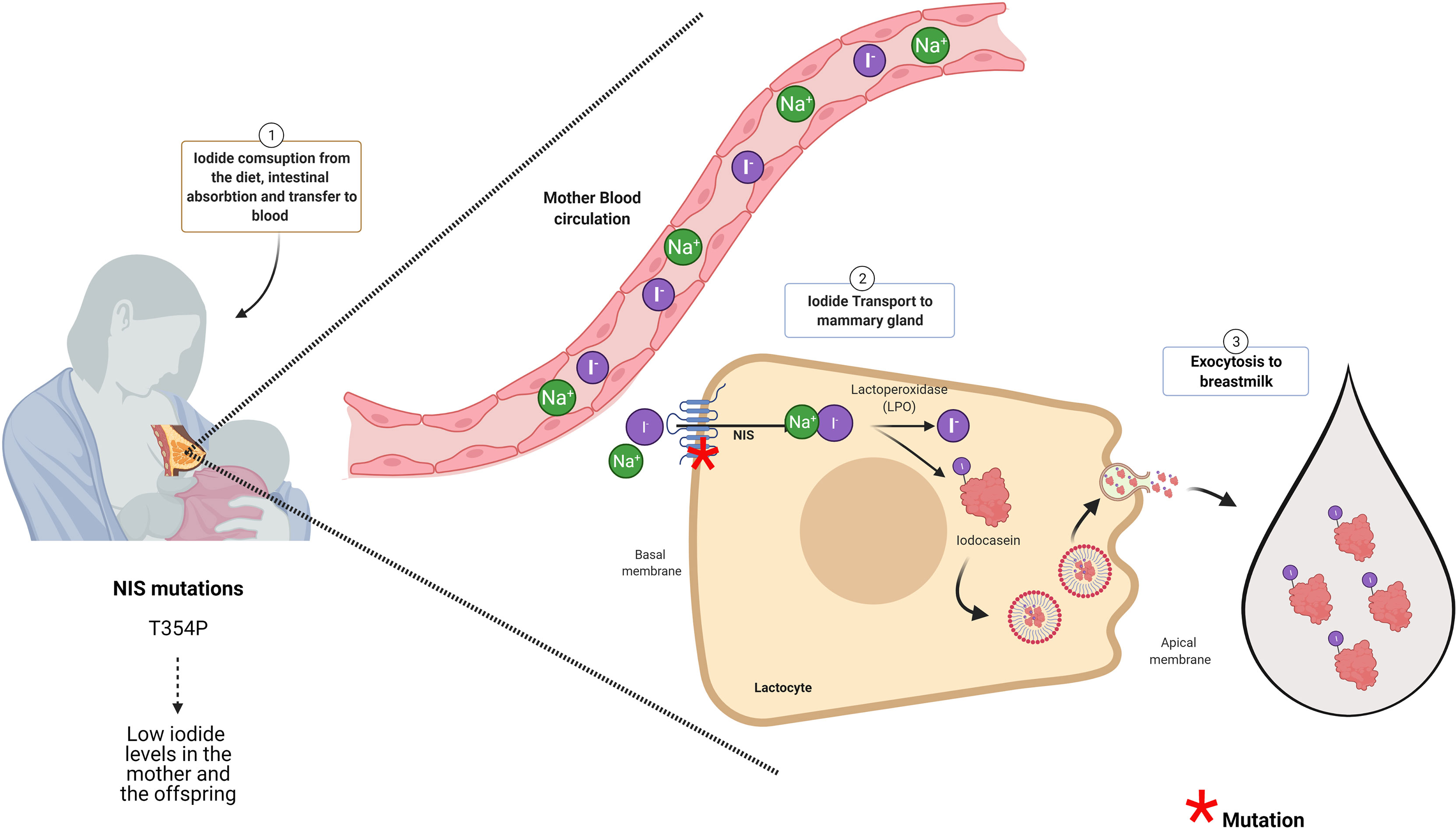
Figure 2 NIS and LPO are essential proteins for iodide accumulation in breast milk. The infant synthesizes its own thyroid hormones. For that, the mother should consume enough iodine to supply her baby with this micronutrient through the breast milk. The Na+/I− symporter (NIS) at the intestine will transport iodide into the blood NIS expressed at the lactocytes will transport iodide from the blood to inside these cells. NIS in the lactocytes is located at the basal membrane. The iodide inside the lactocyte is incorporated into casein by the action of lactoperoxidase (LPO). Iodocasein molecules will be aggregate inside micelles. The micelles will fuse with the apical membrane to release their content by exocytosis to breast milk. Woman diagnosed with congenital hypothyroidism (CH) carrying NIS mutation (like T354P) accumulated low iodide in the milk even though she was under levothyroxine (LT4) treatment. Thus, women carrying NIS mutants that caused congenital hypothyroidism besides T4 treatment should receive iodide supplementation (66). Mutations in proteins with red asterisk have been associated in the literature with low iodide in breast milk as it is for NIS (see red asterisk). Even though, LPO is an essential enzyme for iodide presence in the milk, there are no reports in the literature indicating that mutations in LPO affects iodide content in breast milk.
NIS Variants That Affect Iodide Concentration in Breast Milk
NIS is the principal molecule responsible for iodide uptake in the mammary gland playing a pivotal role in iodide concentration in breast milk (67, 82) (Figure 2). Eighteen NIS mutations have been reported in the Slc5a5, the gene that encodes for NIS (30, 83, 84). The type of mutations in Slc5a5 includes nonsense, alternative splicing, frameshift, deletion, and missense, and they are responsible for causing ITD (20). ITD occurs as autosomal recessive disorder that results in CH (29). It has been reported that the severity and the onset of the CH varies and depends on the type of NIS mutation and iodide ingestion (30, 31). An important issue to consider is the situation of breastfeeding women diagnosed with CH bearing NIS mutation and treated with levothyroxine (LT4) (84). Even though LT4 will protect the mother from suffering hypothyroidism, their lactating offspring will not receive enough iodide, given that NIS is the only iodide transporter described in the mammary gland so far. PDS is also expressed at the mammary gland, its expression is stimulated by prolactin; however, PDS mutations affecting iodine content in breast milk has not been described (85). Regarding the NIS variants, there is only one report by Mizokami et al., showing ITD at the mammary gland during lactation (66). Mizokami et al. reported the case of a woman diagnosed with CH that received LT4 treatment (150 µg/day of LT4) and had low levels of iodide in her breast milk (54 µg/L) (66). Iodine supplementation was given to the mother to reach the minimum level of 90 µg/L of iodide in milk. This amount was accepted as sufficient to fulfill the newborn iodine necessities (66). The breastfeeding woman carried a missense variation in the slc5a5 gene previously described by Fujiwara et al. This variant has a cytosine instead of adenosine in codon 354, leading to an exchange of threonine for proline (T354P) in NIS protein (86). NIS T354 mutant has lost function and is highly prevalent in the Japanese population (87). Regarding LPO, an important enzyme for iodide accumulation in breast milk, there are no specific mutations described yet in this protein that affects iodide content in breast milk.
Discussion and Concluding Remarks
It is well established that patients born with PCH should be immediately treated with LT4 for life. However, those patients with TCH will stop LT4 treatment at a certain age when their thyroid function normalizes and present normal TSH and fT4, in fact most TCH patients are euthyroid at adulthood. Besides that, genetic counseling is highly recommended especially for women. Genetic counseling will allow to identify the mutations that are responsible for thyroid dyshormonogenesis. As we discussed in this review, proper maternal thyroid function in pregnancy is essential for appropriate fetus development. The requirement for thyroid function during pregnancy is higher in pregnant women than for nonpregnant women. Pregnancy requires increasing iodine consumption and the demand for functional thyroid proteins. The identification of mutations in thyroid proteins will help physicians to protect the women during pregnancy to suffer hypothyroidism or hypothyroxinemia. Women carrying genetic mutations on DUOX2, DUOXA2, TPO, or NIS gene, they must receive prophylactic LT4 treatment during pregnancy due to the higher probability to develop thyroid hormone deficiency during this period. The relevance of NIS mutants surpasses pregnancy, given they are also significant for infant development during breastfeeding. If the CH woman carries mutations of NIS protein, besides T4 treatment, she must receive prophylactic iodine supplementation during breastfeeding. Given that T4 treatment will only rescue the mother from hypothyroidism and not his/her lactating infant for thyroid hormone deficiency, medical doctors should care to supply the mother with enough iodide. One approach was described by Mizokami et al. The authors administered iodide to the lactating woman that carries NIS T354P mutation and follows the measurement of breast milk iodine concentration (BMIC) (66). This action restored the normal levels of iodide and protected the neonate from suffering the health consequences of hypothyroidism (66, 85). There are certain NIS variants that cause severe CH, and in these cases, mother supplementation with iodide will not rescue the lactating infant. In these cases, it will be highly recommendable to directly supplement the baby by giving him/her iodine through baby formula (63). Baby formula should have 10–60 µg of iodine/100 kcal or 5–75 µg of iodine/100 kcal according to the regulations of the Commission of European communities (88) and the US Food and Drug Administration (FDA), respectively (89).
Genetic counseling is essential for CH women to determine if they will need iodide supplementation during breastfeeding to ensure the proper development of their infant. LPO mutants had not yet been described in the literature, and they have not even been related to low iodide levels in breast milk. Based on the physiological relevance of iodide for the infant’s proper development, we highly recommend measuring its content in breast milk.
Conclusions
The concluding remark of this mini review is to highly recommend women that have been diagnosed with dyshormonogenic CH to counsel for genetic study before pregnancy, aiming to determine which thyroid gene/s is or are mutated. By using this strategy, medical doctors could design the prophylactic treatment by giving the patient LT4 and/or iodide. This information will protect the offspring during gestation and breastfeeding from cognitive impairment. The mother is the only source of thyroid hormones at the early pregnancy and the source of iodide during breastfeeding. Women diagnosed with TCH who bear mutations in DUOX2, DUOXA2, TPO, TG, SLC26A7, or NIS genes and are euthyroid before pregnancy should be prophylactic treated with LT4 during pregnancy, aiming to have appropriate thyroid hormone level for the development of the fetus. Although women who carry mutations for NIS are treated with LT4 during lactation, they must increase the consumption of iodide, and BMCI should be monitored. The fact that there is only a single report in the literature associating that a lactating woman carrying a NIS mutant should receive iodine supplementation during lactation to protect the development of the lactating infant, increases the alarm for gynecologist and endocrinologist to evaluate for NIS variations in women diagnosed with TID. Moreover, it is necessary to encourage NGS and WES analyses in patients diagnosed with TCH due to dyshormonogenesis. These techniques will unveil the complexity of thyroid genes that are required for the proper function of thyroid gland for the human health protection.
Author Contributions
MO and CR worked: All authors have made a substantial, direct, and intellectual contribution to the work and approved it for publication. All authors have read and agreed to the published version of the manuscript. All authors contributed to the article and approved the submitted version.
Funding
This work was supported by FONDECYT 11180739 (MO), 1191300 (CR), Millennium Institute on Immunology and Immunotherapy (P09/016-F and ICN09_016) (MO, CR), and Nucleus project DI-03-19/N 303 (CR and MO).
Conflict of Interest
The authors declare that the research was conducted in the absence of any commercial or financial relationships that could be construed as a potential conflict of interest.
Publisher’s Note
All claims expressed in this article are solely those of the authors and do not necessarily represent those of their affiliated organizations, or those of the publisher, the editors and the reviewers. Any product that may be evaluated in this article, or claim that may be made by its manufacturer, is not guaranteed or endorsed by the publisher.
References
1. Leung AM, Pearce EN, Braverman LE. Iodine Nutrition in Pregnancy and Lactation. Endocrinol Metab Clinics North Am (2011) 40(4):765–77. doi: 10.1016/j.ecl.2011.08.001
2. Smith A, Eccles-Smith J, D’Emden M, Lust K. Thyroid Disorders in Pregnancy and Postpartum. Aust Prescriber (2017) 40(6):214–9. doi: 10.18773/austprescr.2017.075
3. Moini J, Pereira K, Samsam M. Chapter 5 - Hypothyroidism. In: Epidemiology of Thyroid Disorders. Elsevier (2020). p. 89–120. Available at: https://www.sciencedirect.com/science/article/pii/B9780128185001000050.
4. Mahadik K, Choudhary P, Roy PK. Study of Thyroid Function in Pregnancy, Its Feto-Maternal Outcome; a Prospective Observational Study. BMC Pregnancy Childbirth (2020) 20(1):769. doi: 10.1186/s12884-020-03448-z
6. Bhavani. Transient Congenital Hypothyroidism. Indian J Endocrinol Metab (2011) 15(6):117. doi: 10.4103/2230-8210.83345
7. Moreno J, Visser T. New Phenotypes in Thyroid Dyshormonogenesis: Hypothyroidism Due to DUOX2 Mutations. Endocrine Dev (2007) 10:99–117. doi: 10.1159/000106822
8. Alexander EK, Pearce EN, Brent GA, Brown RS, Chen H, Dosiou C, et al. Guidelines of the American Thyroid Association for the Diagnosis and Management of Thyroid Disease During Pregnancy and the Postpartum. Thyroid (2017) 27(3):315–89. doi: 10.1089/thy.2016.0457
9. Lazarus JH. Thyroid Disease in Pregnancy and Childhood. Minerva Endocrinologica (2005) 30(2):71–87.
10. de Escobar GM, Obregón MJ, del Rey FE. Iodine Deficiency and Brain Development in the First Half of Pregnancy. Public Health Nutr (2007) 10(12A):1554–70. doi: 10.1017/S1368980007360928
11. Delitala AP, Capobianco G, Cherchi PL, Dessole S, Delitala G. Thyroid Function and Thyroid Disorders During Pregnancy: A Review and Care Pathway. Arch Gynecology Obstetrics (2019) 299(2):327–38. doi: 10.1007/s00404-018-5018-8
12. Burrow GN, Fisher DA, Larsen PR. Maternal and Fetal Thyroid Function. N Engl J Med (1994) 331(16):1072–8. doi: 10.1056/NEJM199410203311608
13. Di Renzo GC, Fonseca E, Gratacos E, Hassan S, Kurtser M, Malone F, et al. Good Clinical Practice Advice: Thyroid and Pregnancy. Int J Gynecology Obstetrics (2019) 144(3):347–51. doi: 10.1002/ijgo.12745
14. de Escobar GM, Ares S, Berbel P, Obregón MJ, del Rey FE. The Changing Role of Maternal Thyroid Hormone in Fetal Brain Development. Semin Perinatology (2008) 32(6):380–6. doi: 10.1053/j.semperi.2008.09.002
15. Salmeen KE, Block-Kurbisch IJ. Thyroid Physiology During Pregnancy, Postpartum, and Lactation. In: Kovacs CS, Deal C, editors. Maternal-Fetal and Neonatal Endocrinology: Physiology, Pathophysiology, and Clinical Management. Academic Press (2019). p. 53–60.
16. Kraiem Z, Sadeh O, Blithe DL, Nisula BC. Human Chorionic Gonadotropin Stimulates Thyroid Hormone Secretion, Iodide Uptake, Organification, and Adenosine 3′, 5′-Monophosphate Formation in Cultured Human Thyrocytes. J Clin Endocrinol Metab (1994) 79(2):595–9. doi: 10.1210/jcem.79.2.8045981
17. Alemu A, Terefe B, Abebe M, Biadgo B. Thyroid Hormone Dysfunction During Pregnancy: A Review. Int J Reprod BioMed (2016) 14(11):677–86. doi: 10.29252/ijrm.14.11.677
18. Fong P. Thyroid Iodide Efflux: A Team Effort? J Physiol (2011) 589(24):5929–39. doi: 10.1113/jphysiol.2011.218594
19. Citterio CE, Targovnik H, Arvan P. The Role of Thyroglobulin in Thyroid Hormonogenesis. Nat Rev Endocrinol (2019) 15(6):323–38. doi: 10.1038/s41574-019-0184-8
20. Targovnik HM, Citterio CE, Rivolta CM. Iodide Handling Disorders (NIS, TPO, TG, IYD). Best Pract Res Clin Endocrinol Metab (2017) 31(2):195–212. doi: 10.1016/j.beem.2017.03.006
21. Park S, Chatterjee VK. Genetics of Congenital Hypothyroidism. J Med Genet (2005) 42(5):379–89. doi: 10.1136/jmg.2004.024158
22. Stoupa A, Kariyawasam D, Muzza M, de Filippis T, Fugazzola F, Polak M, et al. New Genetics in Congenital Hypothyroidism. Endocrine (2021) 71(3):696–705. doi: 10.1007/s12020-021-02646-9
23. Kwak MJ. Clinical Genetics of Defects in Thyroid Hormone Synthesis. Ann Pediatr Endocrinol Metab (2018) 23(4):169–75. doi: 10.6065/apem.2018.23.4.169
24. van Trotsenburg AS, Stoupa A, Léger J, Rohrer TR, Peters C, Fugazzola L, et al. Congenital Hypothyroidism: A 2020 Consensus Guidelines Update An ENDO-EUROPEAN REFERENCE NETWORK (ERN) Initiative Endorsed by the European Society for Pediatric Endocrinology and the European Society for Endocrinology. Thyroid (2020) 31(3):387–419. doi: 10.1089/thy.2020.0333
25. Dror DK, Allen LH. Iodine in Humanmilk: A Systematic Review. Adv Nutr (2018) 9(suppl_1):347S–57S. doi: 10.1093/advances/nmy020
26. Azizi F, Smyth P. Breastfeeding and Maternal and Infant Iodine Nutrition. Clin Endocrinol (2009) 70(5):803–9. doi: 10.1111/j.1365-2265.2008.03442.x
27. Moreno JC, Bikker H, Kempers MJE, van Trotsenburg ASP, Baas F, de Vijlder JJM, et al. Inactivating Mutations in the Gene for Thyroid Oxidase 2 ( THOX2 ) and Congenital Hypothyroidism. N Engl J Med (2002) 347(2):95–102. doi: 10.1056/NEJMoa012752
28. Schoenmakers N. Genetic Causes of Congenital Hypothyroidism. In: Huhtaniemi I, Martini L editors. Encyclopedia of Endocrine Diseases (Second Edition). Academic Press (2018). p. 296–312. Available at: https://www.sciencedirect.com/science/article/pii/B9780128012383653369.
29. Ravera S, Reyna-Neyra A, Ferrandino G, Amzel LM, Carrasco N. The Sodium/Iodide Symporter (NIS): Molecular Physiology and Preclinical and Clinical Applications. Annu Rev Physiol (2017) 79:261–89. doi: 10.1146/annurev-physiol-022516-034125
30. Nicola JP, Martin M. Congenital Iodide Transport Defect: Recent Advances and Future Perspectives. J Clin Mol Endocrinol (2016) 1(2):1–6. doi: 10.21767/2572-5432.10009
31. Szinnai G, Kosugi S, Derrien C, Lucidarme N, David V, Czernichow P, et al. Extending the Clinical Heterogeneity of Iodide Transport Defect (ITD): A Novel Mutation R124H of the Sodium/Iodide Symporter Gene and Review of Genotype-Phenotype Correlations in ITD. J Clin Endocrinol Metab (2006) 91(4):1199–204. doi: 10.1210/jc.2005-1832
32. Devuyst O, Golstein PE, Sanches MV, Piontek K, Wilson PD, Guggino WB, et al. Expression of CFTR in Human and Bovine Thyroid Epithelium. Am J Physiol (1997) 272(4 Pt 1):C1299–308. doi: 10.1152/ajpcell.1997.272.4.C1299
33. Iosco C, Cosentino C, Sirna L, Romano R, Cursano S, Mongia A, et al. Anoctamin 1 Is Apically Expressed on Thyroid Follicular Cells and Contributes to ATP- and Calcium-Activated Iodide Efflux. Cell Physiol biochemistry: Int J Exp Cell Physiology Biochemistry Pharmacol (2014) 34(3):966–80. doi: 10.1159/000366313
34. Wémeau J-L, Kopp P. Pendred Syndrome. Best Pract Res Clin Endocrinol Metab (2017) 31(2):213–24. doi: 10.1016/j.beem.2017.04.011
35. Carvalho DP, Dupuy C. Thyroid Hormone Biosynthesis and Release. Mol Cell Endocrinol (2017) 458:6–15. doi: 10.1016/j.mce.2017.01.038
36. Everett L, Glaser B, Beck J, Idol J, Buchs A, Heyman M, et al. Pendred Syndrome Is Caused by Mutations in a Putative Sulphate Transporter Gene (PDS). Nat Genet (1997) 17(4):411–22. doi: 10.1038/ng1297-411
37. Grasberger H, Refetoff S. Genetic Causes of Congenital Hypothyroidism Due to Dyshormonogenesis. Curr Opin Pediatr (2011) 23(4):421–8. doi: 10.1097/MOP.0b013e32834726a4
38. Zou M, Alzahrani AS, Al-Odaib A, Alqahtani MA, Babiker O, Al-Rijjal RA, et al. Molecular Analysis of Congenital Hypothyroidism in Saudi Arabia: SLC26A7 Mutation Is a Novel Defect in Thyroid Dyshormonogenesis. J Clin Endocrinol Metab (2018) 103(5):1889–98. doi: 10.1210/jc.2017-02202
39. Maruo Y, Takahashi H, Soeda I, Nishikura N, Matsui K, Ota Y, et al. Transient Congenital Hypothyroidism Caused by Biallelic Mutations of the Dual Oxidase 2 Gene in Japanese Patients Detected by a Neonatal Screening Program. J Clin Endocrinol Metab (2008) 93(11):4261–7. doi: 10.1210/jc.2008-0856
40. Zheng Z, Yang L, Sun C, Wu J, Luo F, Zhou W, et al. Genotype and Phenotype Correlation in a Cohort of Chinese Congenital Hypothyroidism Patients With DUOX2 Mutations. Ann Trans Med (2020) 8(24):1649–9. doi: 10.21037/atm-20-7165
41. De Deken X, Miot F. DUOX Defects and Their Roles in Congenital Hypothyroidism. In: Methods in Molecular Biology, vol. 1982. Clifton, N.J.:Humana Press Inc (2019). p. 667–93. doi: 10.1007/978-1-4939-9424-3_37
42. Liu S, Han W, Zang Y, Zang H, Wang F, Jiang P, et al. Identification of Two Missense Mutations in DUOX1 (P.R1307Q) and DUOXA1 (P.R56W) That Can Cause Congenital Hypothyroidism Through Impairing H2O2 Generation. Front Endocrinol (2019) 10:526. doi: 10.3389/fendo.2019.00526
43. Sugisawa C, Higuchi S, Takagi M, Hasegawa Y, Taniyama M, Abe K, et al. Homozygous DUOXA2 Mutation (P.Tyr138*) in a Girl With Congenital Hypothyroidism and Her Apparently Unaffected Brother: Case Report and Review of the Literature. Endocrine J (2017) 64(8):807–12. doi: 10.1507/endocrj.EJ16-0564
44. Grasberger H, Refetoff S. Identification of the Maturation Factor for Dual Oxidase: Evolution of an Eukaryotic Operon Equivalent. J Biol Chem (2006) 281(27):18269–72. doi: 10.1074/jbc.C600095200
45. Zamproni I, Grasberger H, Cortinovis F, Vigone MC, Chiumello G, Mora S, et al. Biallelic Inactivation of the Dual Oxidase Maturation Factor 2 (DUOXA2) Gene as a Novel Cause of Congenital Hypothyroidism. J Clin Endocrinol Metab (2008) 93(2):605–10. doi: 10.1210/jc.2007-2020
46. Liu S, Liu L, Niu X, Lu D, Xia H, Yan S. A Novel Missense Mutation (I26M) in DUOXA2 Causing Congenital Goiter Hypothyroidism Impairs Nadph Oxidase Activity But Not Protein Expression. J Clin Endocrinol Metab (2015) 100(4):1225–9. doi: 10.1210/jc.2014-3964
47. Grasberger H. Defects of Thyroidal Hydrogen Peroxide Generation in Congenital Hypothyroidism. Mol Cell Endocrinol (2010) 322(1–2):99–106. doi: 10.1016/j.mce.2010.01.029
48. Aycan Z, Cangul H, Muzza M, Bas VN, Fugazzola L, Chatterjee VK, et al. Digenic DUOX1 and DUOX2 Mutations in Cases With Congenital Hypothyroidism. J Clin Endocrinol Metab (2017) 102(9):3085–90. doi: 10.1210/jc.2017-00529
49. Wang F, Zang Y, Li M, Liu W, Wang Y, Yu X, et al. DUOX2 and DUOXA2 Variants Confer Susceptibility to Thyroid Dysgenesis and Gland-In-Situ With Congenital Hypothyroidism. Front Endocrinol (2020) 0:237. doi: 10.3389/fendo.2020.00237
50. Dunn JT, Dunn AD. Update on Intrathyroidal Iodine Metabolism. Thyroid: Off J Am Thyroid Assoc (2001) 11(5):407–14. doi: 10.1089/105072501300176363
51. Abramowicz MJ, Targovnik HM, Varela V, Cochaux P, Krawiec L, Pisarev MA, et al. Identification of a Mutation in the Coding Sequence of the Human Thyroid Peroxidase Gene Causing Congenital Goiter. J Clin Invest (1992) 90(4):1200–4. doi: 10.1172/JCI115981
52. Makretskaya N, Bezlepkina O, Kolodkina A, Kiyaev A, Vasilyev EV, Petrov V, et al. High Frequency of Mutations in “Dyshormonogenesis Genes” in Severe Congenital Hypothyroidism. PloS One (2018) 13(9):e0204323. doi: 10.1371/journal.pone.0204323
53. Neves S, Mezalira P, Dias V, Chagas A, Viana M, Targovnik H, et al. Monoallelic Thyroid Peroxidase Gene Mutation in a Patient With Congenital Hypothyroidism With Total Iodide Organification Defect. Arq Bras Endocrinol Metabol (2010) 54(8):732–7. doi: 10.1590/S0004-27302010000800012
54. Fugazzola L, Cerutti N, Mannavola D, Vannucchi G, Fallini C, Persani L, et al. Monoallelic Expression of Mutant Thyroid Peroxidase Allele Causing Total Iodide Organification Defect. J Clin Endocrinol Metab (2003) 88(7):3264–71. doi: 10.1210/jc.2002-021377
55. Narumi S, Fox LA, Fukudome K, Sakaguchi Z, Sugisawa C, Abe K, et al. Mild Thyroid Peroxidase Deficiency Caused by TPO Mutations With Residual Activity: Correlation Between Clinical Phenotypes and Enzymatic Activity. Endocrine J (2017) 64(11):1087–97. doi: 10.1507/endocrj.EJ17-0194
56. Huang M, Lu X, Dong G, Li J, Chen C, Yu Q, et al. Analysis of Mutation Spectra of 28 Pathogenic Genes Associated With Congenital Hypothyroidism in the Chinese Han Population. Front Endocrinol (2021) 0:815. doi: 10.3389/fendo.2021.695426
57. Citterio CE, Rivolta CM, Targovnik HM. Structure and Genetic Variants of Thyroglobulin: Pathophysiological Implications. Mol Cell Endocrinol (2021) 528:111227. doi: 10.1016/j.mce.2021.111227
58. Stoupa A, Chehade GAH, Chaabane R, Kariyawasam D, Szinnai G, Hanein S, et al. High Diagnostic Yield of Targeted Next-Generation Sequencing in a Cohort of Patients With Congenital Hypothyroidism Due to Dyshormonogenesis. Front Endocrinol (2020) 11:545339. doi: 10.3389/FENDO.2020.545339
59. Oliver-Petit I, Edouard T, Jacques V, Bournez M, Cartault A, Grunenwald S, et al. Next-Generation Sequencing Analysis Reveals Frequent Familial Origin and Oligogenism in Congenital Hypothyroidism With Dyshormonogenesis. Front Endocrinol (2021) 0:722. doi: 10.3389/fendo.2021.657913
60. Ramezani Tehrani F, Nazarpour S, Behboudi-Gandevani S. Isolated Maternal Hypothyroxinemia and Adverse Pregnancy Outcomes: A Systematic Review. J Gynecology Obstetrics Hum Reprod (2021) 50(7):102057. doi: 10.1016/j.jogoh.2020.102057
61. Raymond J, LaFranchi SH. Fetal and Neonatal Thyroid Function: Review and Summary of Significant New Findings. Curr Opin Endocrinology Diabetes Obes (2010) 17(1):1–7. doi: 10.1097/MED.0b013e328333b0b2
62. Ares S, Quero J, Morreale de Escobar G. Neonatal Iodine Deficiency: Clinical Aspects. J Pediatr Endocrinol Metab (2005) 18(SUPPL. 1):1257–64. doi: 10.1515/JPEM.2005.18.S1.1257
63. Ghirri P, Lunardi S, Boldrini A. Iodine Supplementation in the Newborn. Nutrients (2014) 6(1):382–90. doi: 10.3390/nu6010382
64. Ahad F, Ganie SA. Iodine, Iodine Metabolism and Iodine Deficiency Disorders Revisited. Indian J Endocrinol Metab (2010) 14(1):13–7.
65. Dorea JG. Iodine Nutrition and Breast Feeding. J Trace Elements Med Biol (2002) 16(4):207–20. doi: 10.1016/S0946-672X(02)80047-5
66. Mizokami T, Fukata S, Hishinuma A, Kogai T, Hamada K, Maruta T, et al. Iodide Transport Defect and Breast Milk Iodine. Eur Thyroid J (2016) 5(2):145–8. doi: 10.1159/000446496
67. Tazebay UH, Wapnir IL, Levy O, Dohan O, Zuckier LS, Zhao QH, et al. The Mammary Gland Iodide Transporter is Expressed During Lactation and in Breast Cancer. Nat Med (2000) 6(8):871–8. doi: 10.1038/78630
68. Ferrera L, Caputo A. Galietta LJ V. TMEM16A Protein: A New Identity for Ca(2+)-Dependent Cl– Channels. Physiol (Bethesda Md.) (2010) 25(6):357–63. doi: 10.1152/physiol.00030.2010
69. Poole VL, McCabe CJ. Iodide Transport and Breast Cancer. J Endocrinol (2015) 227(1):R1–R12. doi: 10.1530/JOE-15-0234
70. Rillema JA, Hill MA. Prolactin Regulation of the Pendrin-Iodide Transporter in the Mammary Gland. Am J Physiology-Endocrinology Metab (2003) 284(1):E25–8. doi: 10.1152/ajpendo.00383.2002
71. Aceves C, Anguiano B, Delgado G. Is Iodine a Gatekeeper of the Integrity of the Mammary Gland? J Mammary Gland Biol Neoplasia (2005) 10(2):189–96. doi: 10.1007/s10911-005-5401-5
72. Zibadi S, Watson RR, Preedy VR. Handbook of Dietary and Nutritional Aspects of Human Breast Milk. In: Human Health Handbooks no. 5. Wageningen Academic Publishers (2013). p. 856.
73. Semba RD, Delange F. Iodine in Human Milk: Perspectives for Infant Health. Nutr Rev (2001) 59(8 Pt 1):269–78. doi: 10.1111/j.1753-4887.2001.tb05512.x
74. Etling N, Padovani E, Fouque F, Tato L. First-Month Variations in Total Iodine Content of Human Breast Milks. Early Hum Dev (1986) 13(1):81–5. doi: 10.1016/0378-3782(86)90101-5
75. Delshad H, Azizi F. Iodine Nutrition in Pregnant and Breastfeeding Women: Sufficiency, Deficiency, and Supplementation. Hormones (2019) 19(2):179–86. doi: 10.1007/s42000-019-00160-2
76. Wollman SH. Nature of the Inhibition by Thiocyanate of the Iodide Concentrating Mechanism of the Thyroid Gland. Am J Physiol (1956) 186(3):453–9. doi: 10.1152/ajplegacy.1956.186.3.453
77. Gaitan E. Goitrogens, Environmental. In: Martini L, editor. Encyclopedia of Endocrine Diseases. Elsevier (2004). p. 286–94. Available at: https://www.sciencedirect.com/science/article/pii/B0124755704005461.
78. Llorente-Esteban A, Manville RW, Reyna-Neyra A, Abbott GW, Amzel LM, Carrasco N. Allosteric Regulation of Mammalian Na+/I– Symporter Activity by Perchlorate. Nat Struct Mol Biol (2020) 27(6):533–9. doi: 10.1038/s41594-020-0417-5
79. Saito K, Yamamoto K, Takai T, Yoshida S. Inhibition of Iodide Accumulation by Perchlorate and Thiocyanate in a Model of the Thyroid Iodide Transport System. Acta Endocrinologica (1983) 104(4):456–61. doi: 10.1530/acta.0.1040456
80. Vanderpas J. GOITROGENS AND ANTITHYROID COMPOUNDS. In: Caballero B, editor. Encyclopedia of Food Sciences and Nutrition (Second Edition). Academic Press (2003). p. 2949–57. Available at: https://www.sciencedirect.com/science/article/pii/B012227055X005666.
81. Kirk AB, Martinelango PK, Tian K, Dutta A, Smith EE, Dasgupta PK, et al. Perchlorate and Iodide in Dairy and Breast Milk. Environ Sci Technol (2005) 7):2011–7. doi: 10.1021/es048118t
82. De La Vieja A, Dohan O, Levy O, Carrasco N. Molecular Analysis of the Sodium/ Iodide Symporter: Impact on Thyroid and Extrathyroid Pathophysiology. Physiol Rev (2000) 80(3):1083–105. doi: 10.1152/physrev.2000.80.3.1083
83. Martín M, Geysels RC, Peyret V, Barquero CEB, Masini-Repiso AM, Nicola JP. Implications of Na+/I- Symporter Transport to the Plasma Membrane for Thyroid Hormonogenesis and Radioiodide Therapy. J Endocrine Soc (2019) 3(1):222–34. doi: 10.1210/js.2018-00100
84. Martín M, Bernal Barquero CE, Geysels RC, Papendieck P, Peyret V, Masini-Repiso AM, et al. Novel Sodium/Iodide Symporter Compound Heterozygous Pathogenic Variants Causing Dyshormonogenic Congenital Hypothyroidism. Thyroid: Off J Am Thyroid Assoc (2019) 29(7):1023–6. doi: 10.1089/thy.2019.0046
85. Golan Y, Assaraf YG. Genetic and Physiological Factors Affecting Human Milk Production and Composition. Nutrients (2020) 12(5):1500. doi: 10.3390/nu12051500
86. Fujiwara H, Tatsumi KI, Miki K, Harada T, Miyai K, Takai SI, et al. Congenital Hypothyroidism Caused by a Mutation in the Na+/l - Symporter. Nat Genet (1997) 16:124–5. doi: 10.1038/ng0697-124
87. Kosugi S, Sato Y, Matsuda A, Ohyama Y, Fujieda K, Inomata H, et al. High Prevalence of T354P Sodium/Iodide Symporter Gene Mutation in Japanese Patients With Iodide Transport Defect Who Have Heterogeneous Clinical Pictures 1. J Clin Endocrinol Metab (1998) 83(11):4123–9. doi: 10.1210/jcem.83.11.5229
88. Ares S, Quero J, Duran S, Presas MJ, Herruzo R, Morreale de Escobar G. Iodine Content of Infant Formulas and Iodine Intake of Premature Babies: High Risk of Iodine Deficiency. Arch Dis Childhood (1994) 71(3 Fetal & Neonatal):F184–91. doi: 10.1136/fn.71.3.f184
Keywords: offspring, pregnancy, breastfeeding, iodine, thyroid hormones, congenital hypothyroid women, genetic counseling
Citation: Opazo MC, Rivera JC, Gonzalez PA, Bueno SM, Kalergis AM and Riedel CA (2021) Thyroid Gene Mutations in Pregnant and Breastfeeding Women Diagnosed With Transient Congenital Hypothyroidism: Implications for the Offspring’s Health. Front. Endocrinol. 12:679002. doi: 10.3389/fendo.2021.679002
Received: 10 March 2021; Accepted: 13 September 2021;
Published: 14 October 2021.
Edited by:
Cintia E. Citterio, Institute of Immunology, Genetics and Metabolism (INIGEM) (CONICET), ArgentinaReviewed by:
Xavier De Deken, Université libre de Bruxelles, BelgiumFrederique Savagner, U1048 Institut des Maladies Métaboliques et Cardiovasculaires (INSERM), France
Yi Wang, China Medical University, China
Copyright © 2021 Opazo, Rivera, Gonzalez, Bueno, Kalergis and Riedel. This is an open-access article distributed under the terms of the Creative Commons Attribution License (CC BY). The use, distribution or reproduction in other forums is permitted, provided the original author(s) and the copyright owner(s) are credited and that the original publication in this journal is cited, in accordance with accepted academic practice. No use, distribution or reproduction is permitted which does not comply with these terms.
*Correspondence: Claudia A. Riedel, claudia.riedel@unab.cl