- 1College of Fisheries and Life Science, Dalian Ocean University, Dalian, China
- 2Key Laboratory of Environment Controlled Aquaculture, Ministry of Education, Dalian, China
- 3College of Marine Science and Environment Engineering, Dalian Ocean University, Dalian, China
- 4College of Life Science, Liaoning Normal University, Dalian, China
To examine the effect and mechanism of thyroid hormone on gonadal sex differentiation, Takifugu rubripes larvae were treated with goitrogen (methimazole, MET, 1000 μg/g), and thyroxine (T4, 2nM) from 25 to 80 days after hatching (dah). Gonadal histology and sex ratios of fish were then determined at 80 dah. MET treatment induced masculinization, but T4 treatment did not induce feminization in T. rubripes larvae. Transcriptomic analysis of gonads at 80 dah was then conducted. Among the large number of differentially expressed genes between the groups, the expression of foxl2, cyp19a1a, and dmrt1 was altered. The expression of foxl2, cyp19a1a, dmrt1 and gsdf at 25, 40, 55 days after treatment (dat) was further analyzed by qPCR. MET treatment suppressed the expression of foxl2 and cyp19a1a, and induced the expression of dmrt1 in genetic females (p < 0.05). Additionally, T4 treatment induced an increase in the expression of cyp19a1a in genetic XY gonads only at 25 dat. However, the increase in cyp19a1a expression did not continue to 40 and 55 dat. This may explain why feminization of larvae was not found in the T4-treated group. Thus, the present study provides the first evidence that MET treatment causes masculinization in teleost fish. The effects of MET-induced masculinization in T. rubripes may act primarily via suppression of the expression of foxl2 and cyp19a1a, and stimulation of the expression of dmrt1. Moreover, the effects of higher concentrations of T4 or different concentrations of T3, on sex differentiation require further testing.
Introduction
Sex determination and differentiation, which develops in either the ovary or testis, is one of the most fundamental and surprisingly diverse biological processes. Across vertebrates, sex differentiation exhibits great variability and diversity. In mammals, sex determination and differentiation are strictly genetically controlled where the sex of the offspring is decided at the moment of fertilization the differential inheritance of sex chromosomes. In contrast, sexual differentiation of teleost vertebrates often happens in response to external signals (eg. social cues, temperature, light, or pH) at an early stage of development or even in adulthood (1–4). Endocrine processes play a critical role in sex determination, and gonadal fate. For example, during the critical period of sex differentiation, sex steroids regulate of steroidogenic enzyme genes by certain transcription factors that are essential for gonadal fate and sex (5). In Odontesthes bonariensis and the Paralichthys olivaceus, glucocorticoids were shown to downregulate cyp19a1a expression resulting in testicular differentiation (6, 7). Additionally, in P. olivaceus, cortisol was involved in suppressing cyp19a1a expression by binding to glucocorticoid responsive elements upstream of the cyp19a1a promoter (7).
Thyroid hormones (THs) have been well demonstrated in regulating various biological processes, such as morphogenesis, growth, reproduction, osmoregulation, and skin pigmentation (8). However, the role of TH in fish gonadal sex differentiation has been explored in only two species of teleost, the three-spined stickleback (Gasterosteus aculeatus) (8) and zebrafish (Danio rerio) (9–11). In G. aculeatus, TH-synthesis inhibitor (perchlorate) treatment induced hermaphroditism in genetic females (12). Exposure of D. rerio larvae to thyroxine during the early stages of sex differentiation causes male-biased populations in a concentration-dependent manner. However, goitrogen treatment did not cause a permanent feminization, but results in some delays in the ovary-to-testis transformation of genetic males (9, 10). These results leave open the question of whether TH plays a consistent role in the regulation of sex differentiation in teleosts. Among amphibians, goitrogens treatment was shown to induce gonadal masculinization (13) or feminization (13, 14), depending on the species. Sharma et al. further examined the effects of TH on the expression of several sex-related genes involved in gonadal sex differentiation in D. rerio (11). The results showed that T4 induced masculinizing in larvae by inhibiting female genes such as cyp19a1a, esr1, esr2a, and esr2b, and stimulating male sex-related genes amh and ar. Meanwhile, goitrogen (methimazole, MET) treatment increased the expression of esr1, esr2a, and esr2b, and reduced the expression of amh and ar. However, the inability of inducing cyp19a1a expression may explain the reversible “feminizing” activity of goitrogen on gonadal sex determination. Knowledge of the roles of non-classical hormones such as TH during gonadal sex differentiation would be of value not only to the understanding of the process of sex determination and differentiation, but also to the advancement of comparative endocrinology and development. However, the molecular changes of the effects of thyroid endocrine manipulation have still not been widely investigated either in amphibian or teleosts.
The tiger pufferfish, Takifugu rubripes, is one of the most valuable commercial fish cultured in Asia. On the market, male fish are more highly valued than females since the mature testes of tiger pufferfish are regarded as a delicacy. Therefore, all-male stocks are preferred in aquaculture. T. rubripes is a gonochoristic fish having an XX/XY sex determination system. This species has the most compact genome sequence, and an allelic variation in the amhr2 gene, which codes for the amh receptor, is responsible for maleness (15, 16). With this knowledge, T. rubripes is an excellent organism to investigate genetic function in relation to sex determination and differentiation. Similar to other teleosts, sex steroids hormones and temperature seem to modulate the process of gonadal sex differentiation in this species (17–20). However, the involvement of non-classical hormones such as TH on sex differentiation has not been examined in T. rubripes. Thus, this study aims to investigate the role of TH using MET as an inhibitor of thyroid function and exogenous treatment with T4. This study may provide a basis for gaining a comprehensive understanding of the molecular mechanisms underlying thytoxine (T4) or MET treatment. In this way, the regulatory network might be elucidated at the critical stage of molecular sex differentiation in T. rubripes.
Materials and Methods
Treatment of T. rubripes Larvae and Sampling
T. rubripes larvae at 20 days after hatching (dah) were obtained from a fishery in Dalian (Dalian Fugu Aquatic Product Co., Ltd) in March 2019. All animal experiments were performed according to the Guide for the Care and Use of Laboratory Animals in Dalian Ocean University, Dalian, China. All animal experiments were approved by the animal study ethical committee of Dalian Ocean University and they are in compliance with Chinese laws, regulations, and ethics. After accommodation for 5 days, T. rubripes were randomly (950 larvae/tank) divided into three groups, including the control group, the MET-treated group, and the TH-treated group. All conditions were done with three replicates. For the MET treatment, larvae were fed with a commercial diet mixed with MET (1000 μg/g diet, Sigma-Aldrich, USA) from 25 to 80 dah. In the T4 treatment group, larvae were immersed in aquaria containing T4 (Sigma-Aldrich) with a final concentration of 2 nmol/mL from 25 to 80 dah. Briefly, 0.45 ml stock solutions (400 nmol/mL) were added to each tank (with 90 L water) to give final immersion concentrations of 2 nmol/L. After immersion, the water was renewed twice (80 L/change) to remove residual hormone. The treatment concentration was based on previous studies in zebrafish (11). T. rubripes larvae were fed a commercial diet (SanTong Company, Weifang, China) with or without treatment six times a day, and were reared at 21-22°C under a natural photoperiod. The bottom of the tank was cleaned to remove debris, excess feed, and dead larvae, and the water was renewed twice daily to maintain the quality of the rearing water. Salinity, temperature, dissolved oxygen, and pH were monitored daily. The larvae were maintained at a salinity of 33 ppt. The oxygen level was maintained > 8 mg L−1 and the pH was maintained at 7.9–8.1. Ammonia and nitrites were measured in water samples taken from the tanks weekly, and the mean values were always < 0.2 and < 0.05 mg L−1, respectively. The bottom of the tank was cleaned to remove debris, excess feed, and dead larvae, and the water was renewed twice daily to maintain the quality of the rearing water.
At 40 and 55 days after treatment (dat), ten larvae from each tank were chosen randomly to be anesthetized on ice. The body length and wet weight were measured. Based on the daily mortality and the number of sampling, final observed survival rate (%) estimates were calculated according to the previous study (21). For histological observations, tissue samples (trunks that contained the gonads) were collected at 55 dat (n = 30/treatment) and fixed in Bouin’s solution. Additionally, gonads of 100 individuals at 25, 40, and 55 dat were dissected and each gonad was placed into a tube with 100 μl of RNAlater reagent (Thermo Fisher Scientific, Baltics, USA). Samples were stored at −80°C prior to RNA extraction. In order to identify the sex of each individual, a piece of tissue sample was stored in a 1.5 tube containing 100% alcohol in the freezer at −20°C.
Histology of Gonads, Genetical Sex Identification, and RNA Extraction
After fixation in Bouin’s fluid for 24 h, gonads were transferred to 70% ethanol, and then samples were dehydrated in ascending concentrations of ethanol. Dehydrated samples were embedded in paraffin. Tissue sections of 6-μm thickness were cut and stained with hematoxylin–eosin. Photographs were taken using a Nikon ECLIPSE Ci-L microscope (Tokyo, Japan). To distinguish the sex-reversal fish, the remained paraffin blocked tissue from 55 dat after histological observation were subjected to DNA extraction using the TIANamp FFPE DNA kit (Tiangen, China). The genetic sex of each fish was identified using SNP markers (a region containing exon 9 of the amhr2 gene) as described previously (15, 22). For transcriptomic analysis and qPCR experiments, genetic sex identification was performed before RNA extraction. DNA of each sample was extracted using the TIANamp Marine Animals DNA kit (Tiangen).
For RNA extraction, ten ovaries or ten testes were pooled for RNA extraction in each sampling point using the Qiagen RNeasy Micro Kit (Qiagen, USA). After genomic DNA elimination (DNAfree kit, Qiagen), RNase inhibitor (Takara, Japan) was added into the purified RNA samples before they were stored at −80°C. RNA purity and integrity were examined with a NanoDrop ND-1000 spectrophotometer (Thermo Scientifc, Wilmington, DE, USA) and an Agilent 2100 Bioanalyzer (Agilent Technologies, Santa Clara, CA, USA).
RNA-Sequencing
For each RNA sample preparation, 1 µg RNA of T. rubripes gonads at 55 dat was used as input material. In total, six sequencing libraries were generated using the NEBNext Ultra RNA Library Prep Kit for Illumina (NEB, USA) following the manufacturer’s recommendations as described elsewhere (22). The libraries included control genetic female (C_XX), control genetic male (C_XY), MET-treated female (MET_XX), MET-treated male (MET_XY), T4-treated female (T4_XX) and T4-treated male (T4_XY). The library fragments were purified with the AMPure XP system (Beckman Coulter, Beverly, USA) to select cDNA fragments of preferentially 250-300 bp in length. Subsequently, size-selected, adaptor-ligated cDNA were mixed with 3 µl USER Enzyme (NEB) for 15 min at 37°C followed by 5 min at 95°C before PCR. Then, Phusion High-Fidelity DNA polymerase, Universal PCR primers and Index (X) Primer were used for PCR. Finally, after purifying PCR products using the AMPure XP system, the library quality was evaluated using an Agilent Bioanalyzer 2100 system. Index codes were added to attribute sequences to each sample. The clustering of the index-coded samples was carried out on a cBot Cluster Generation System according to the manufacturer’s instructions (TruSeq PE Cluster Kit v3-cBot-HS, Illumia). After cluster generation, all six libraries were sequenced on an Illumina Novaseq platform and 150 bp paired-end reads were generated. The reference genomes were downloaded from the website ftp://ftp.ncbi.nlm.nih.gov/genomes/all/GCF_000180615.1_FUGU5/. Clean data from each library were aligned to the genome of T. rubripes using Hisat2 v2.0.5. Fragments per kilobase of exon models per million mapped reads, based on the length of the gene and read count mapped to this gene, were used for calculating gene expression levels. DESeq2 R package was used for differential expression analysis of two libraries. The resulting P values were adjusted using the Benjamini, Hochberg method (23). Corrected P-value of 0.05 and absolute fold change of 2 was set as the threshold for significantly differential expression. Differentially expressed genes (DEGs) were categorized based on Gene Ontology (GO) and Kyoto Encyclopedia of Genes and Genomes (KEGG) enrichment analysis. GO terms with corrected P value less than 0.05 were considered significantly enriched by differential expressed genes.
qPCR Verified
Primers for qPCR were designed using Primer Premier 5.0 program (Table 1). The relative abundance of the selected gene was evaluated based on the 2−ΔΔCT method. The levels of foxl2, cyp19a1a, dmrt1, and gsdf (a gene identified in our previous study) in the gonads of larvae at 25, 40, and 55 dat were determined in each group using the Applied Biosystems 7900 HT Real-Time PCR System. The stability of two commonly used reference genes β-actin and elongation factor 1-alpha (ef1α) of T. rubripes was evaluated using Normfinder (v 0.953) (17–20). The evaluation revealed that ef1α is more stable reference gene in this study. Hence, ef1α was used as a reference gene in the qPCR analysis as described previously (22).
Statistical Analyses
One-way ANOVA followed by the Duncan’s test (IBM SPSS statistics version 22.0, IBM, Chicago, IL, USA) was performed to examine the statistical significance of growth, survival rate and gene expression levels between control groups and treatment groups, respectively. A p value of < 0.05 was considered significant.
Results
Growth, Survival Rate of the Larvae, and Histological Analysis of the Gonads
At 40 dat, the body length of larvae in the T4 p (32.63 ± 0.61 mm) and MET treatment groups (31.84 ± 2.60 mm) was similar to the control group (29.98 ± 1.33 mm) (p > 0.05) (Figure 1A). No significant difference in wet weight was observed between the treatment and control groups (p > 0.05) (Figure 1B). At 55 dat, the body length of larvae in the T4 (54.57 ± 2.66 mm) and the MET (52.62 ± 1.26 mm) treatment groups was significantly higher than the control group (49.06 ± 1.33 mm) (p < 0.05) (Figure 1A). The wet weight of larvae in the T4 (5.92 ± 0.12 g) and the MET (5.42± 0.16 g) treatment group were significantly higher than the control group (4.15 ± 0.05 g) (p < 0.05) at 55 dat (Figure 1B).
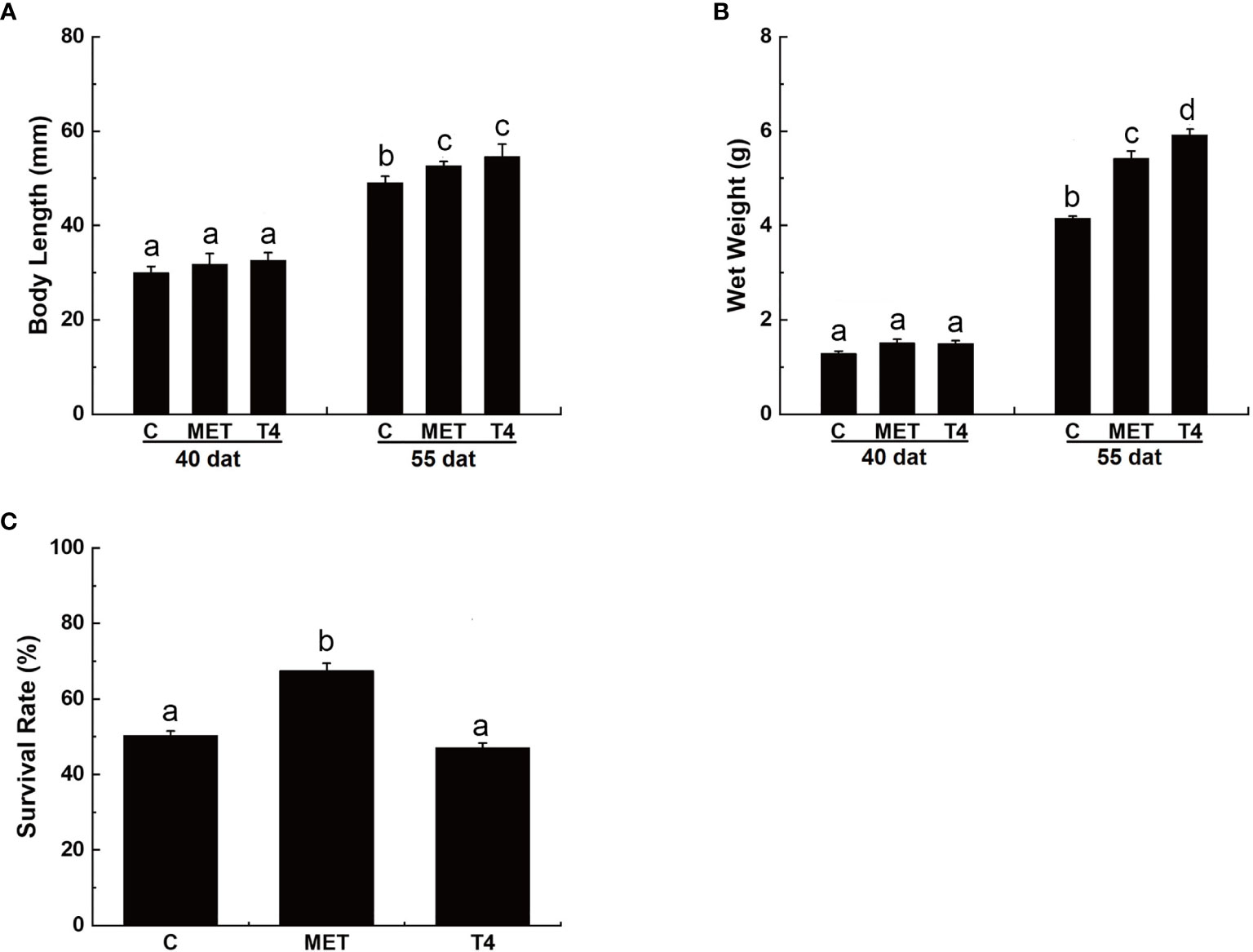
Figure 1 Growth and survival rate (%) of Takifugu rubripes larvae in different experimental groups. (A) Body length of T. rubripes larvae at 40 and 55 days after treatment (dat). (B) Wet weight of T. rubripes larvae at 40 and 55 dat. (C) Survival rate of T. rubripes larvae at 55 dat. C, control; MET, MET-treated group; T4, T4-treated group. Each value represents the mean ± SD of three measurements, different lowercase letters indicate significant differences between each treatment (one-way ANOVA, P < 0.05, n = 3).
By 55 dat, no significant difference in the survival rate of larvae was observed in the T4-treated group compared with the control group (p > 0.05). However, the survival rate of larvae in the MET treatment group was significantly higher (67.54% ± 1.93%) than the control group (50.33% ± 1.14%) (p < 0.05) (Figure 1C).
At 80 dah (55 dat) no sex reversal phenomenon was observed in the control group, while the oviposition plate and the ovary cavity were clearly observed in the ovary (Figure 2A and Table 2). There was a large amount of oogonia and a small number of oocytes on the oviposition plate. In the testis, along the circumference of the testes, a large number of spermatocytes was observed, but no sex reversal larvae was found (Figure 2B and Table 2). MET successfully induced 100% masculinization, and all the samples had intermediate gonads having empty lumina (Figures 2C–E and Table 2). In the T4-treated group, no sex reversal larvae were found (Figures 2F–H and Table 2).
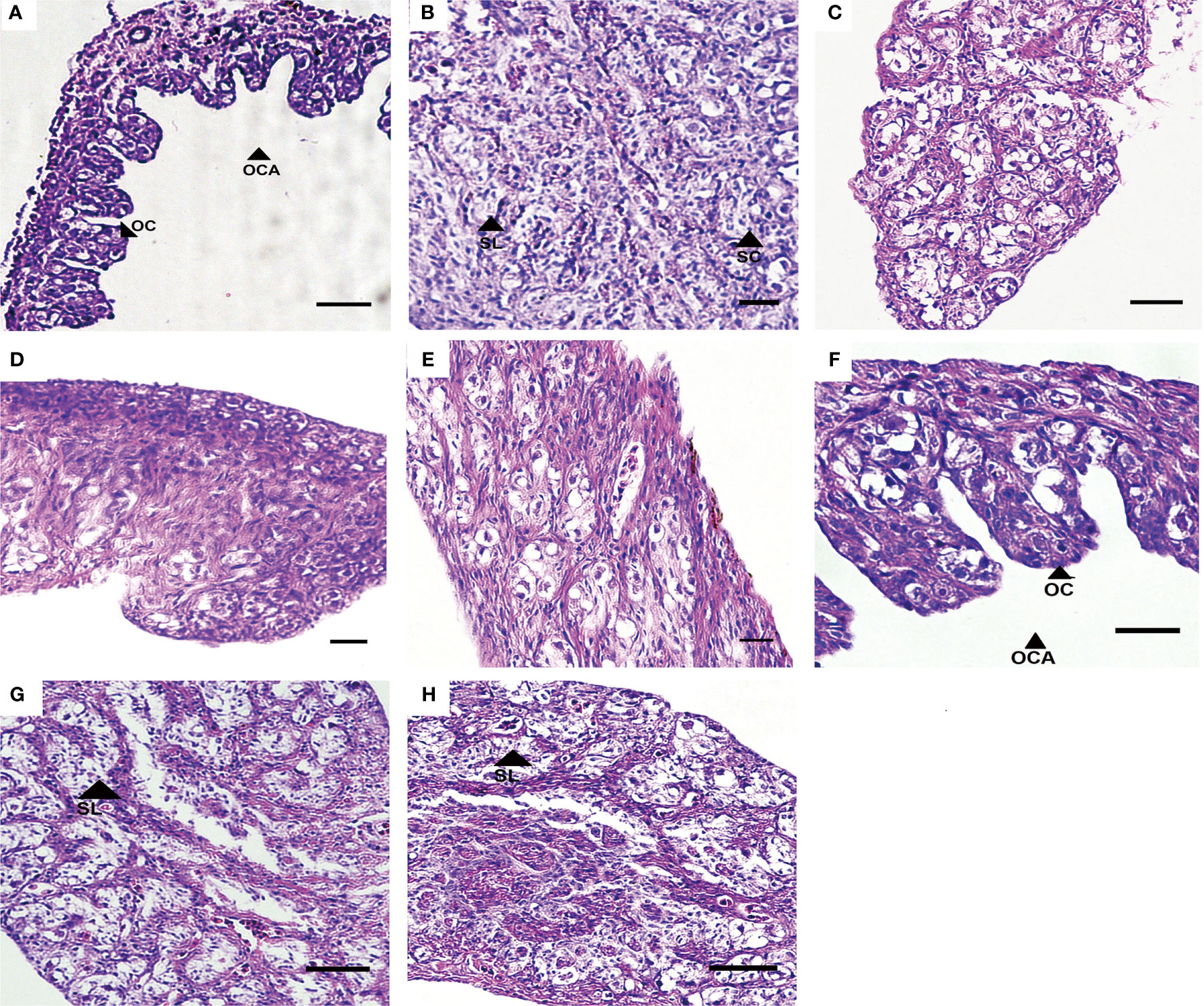
Figure 2 Gonad development in T. rubripes at 55 dat. (A) and (B), XX and XY of control groups; (C) and (D), MET-treated XX, (E), MET-treated XY; (F), T4-treated XX; (G) and (H), T4-treated XX. OC, oocyte, OCA, ovarian cavity, SL, spermatogenic cysts, SC, spermatocyte. Scale bar, 20 μm.
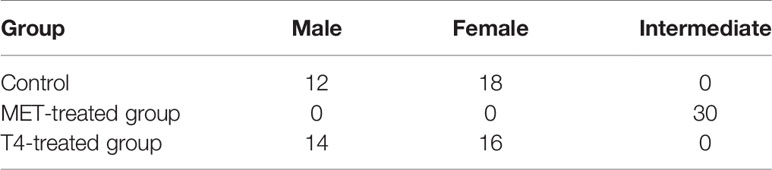
Table 2 Sex ratio of T. rubripes at 55 dat in different treatment groups according to the phenotype of gonads.
Sequencing and Mapping
Raw reads of 45,874,794 (C_XX), 45,793,670 (C_XY), 47,301,088 (MET_XX), 47,429,562 (MET_XY), 46,075,656 (T4_XX), and 47,710,900 (T4_XY) were generated by sequencing the six cDNA libraries derived from gonads of T. rubripes from three experiment groups, respectively (Table 3). The raw sequence reads have been submitted to the Short Read Archive with the following accession numbers: SRR12364833, SRR12358231, SRR12364848, SRR12364847, SRR12364850, and SRR12364849. After filtering out low-quality data, clean reads (44, 634, 680 in C_XX, 44, 951, 110 in C_XY, 46, 011, 968 in MET_XX, 46, 634, 316 in MET_XY, 44, 872, 284 in T4_XX, and 46, 537, 592 in T4_XY) were obtained. The data showed that 90.87%, 90.42%, 90.79%, 88.30%, 90.65%, and 90.75% of the clean reads were uniquely mapped to the FUGU genome (Table 3).
Global Gene Expression Profiles in the Different Groups
Approximately 1,270 DEGs were identified between the MET_XX and C_XX, including 907 down-regulated genes, including cyp19a1, zp3-like, zp4-like, foxl2, hsd17b1, P450scc, piwi2, pgr, cyp17a2, and esr1. Likewise, 363 up-regulated genes, such as dmrt1, dmrt3, and lhcgr were identified (Figures 3A, 4, and Table 4). Between T4_XY and C_XY, 356 DEGs were identified, of which 220 genes were down-regulated, such as cyp17a1, and cyp11c1, and 136 genes were up-regulated, such as hsp70, cyp19a1a, foxl2, and hsp90a (Figures 3B, 4, and Table 4). Moreover, no sexual dimorphic expression of three deiodinases (dio1, dio2, dio3a), and two thyroid receptors (trα and trβ) have been found between testes and ovaries, and the changes in their expression were not found after T4 or MET treatment by RNA-Seq. Yet, dio3 was identified as DEGs between C_XX and C_XY fugu, and both T4 and MET treatment downregulated its expression in XY T. rubripes.
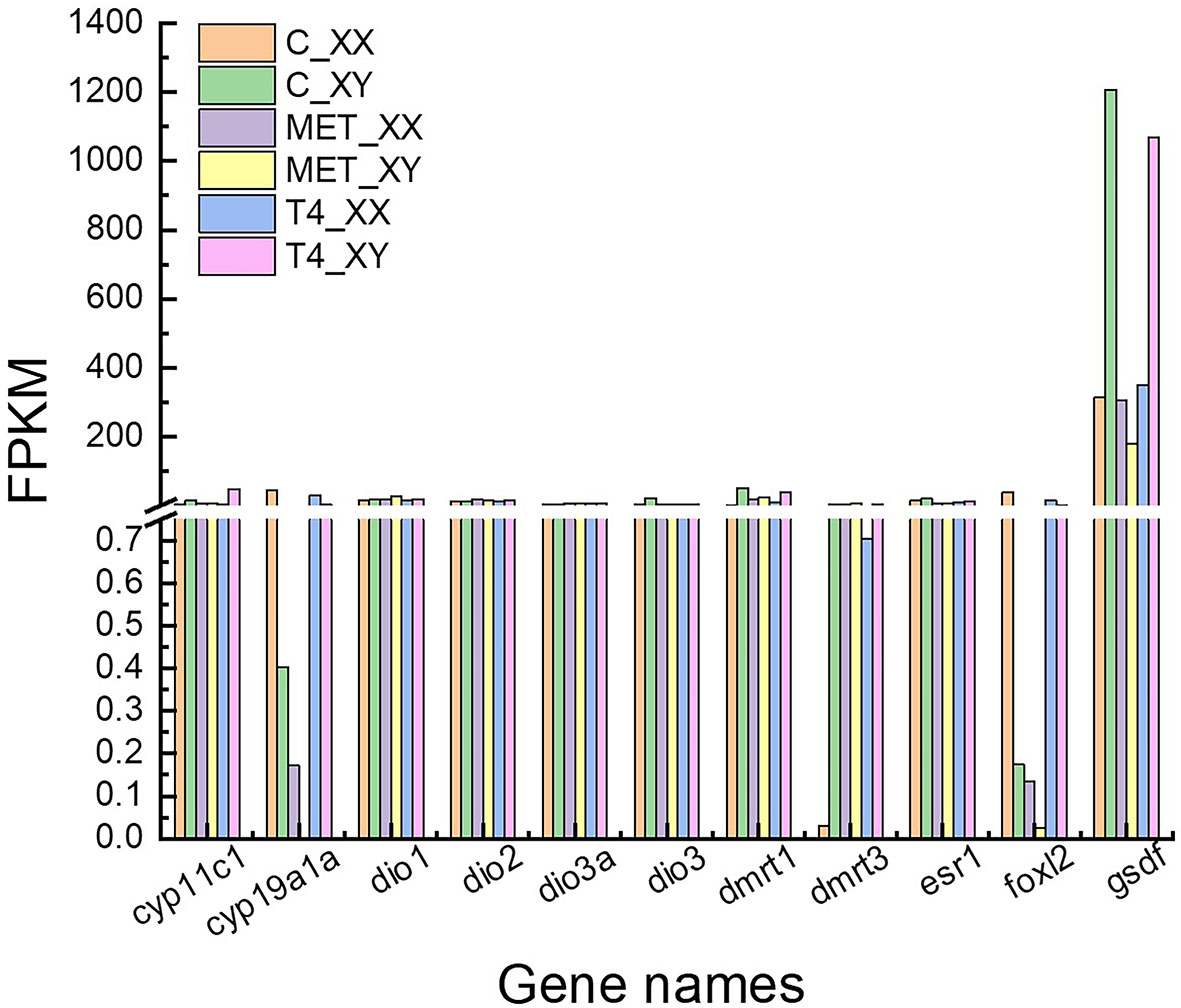
Figure 4 FPKM (reads per kb per million reads) of cyp19a1a, dio1, dio2, dio3a, dmrt1, dmrt3, esr1, foxl2, gsdf, thrα, thrβ and cyp19a1a obtained by RNA-seq.
The most significantly enriched top 30 terms are shown in Figure 5. In MET_XX versus C_XX (Figure 5A), and T4_XY versus C_XY (Figure 5B), the number of GO terms in the molecular function was most prevalent, followed by biological process and cellular component. In MET_XX versus C_XX, genes involved in peptidase activity, acting on L-amino acid peptides, peptidase activity, and endopeptidase activity were highly represented for the molecular function; extracellular region and cytoskeleton were highly represented for the cellular component; proteolysis was highly represented for the biological process. In T4_XY versus C_XY, peptidase activity, acting on L-amino acid peptides, peptidase activity, and endopeptidase activity were highly represented for the molecular function; myosin complex, actin cytoskeleton, extracellular region, non-membrane-bounded organelle, etc. were highly represented for the cellular component; proteolysis was highly represented for the biological process.
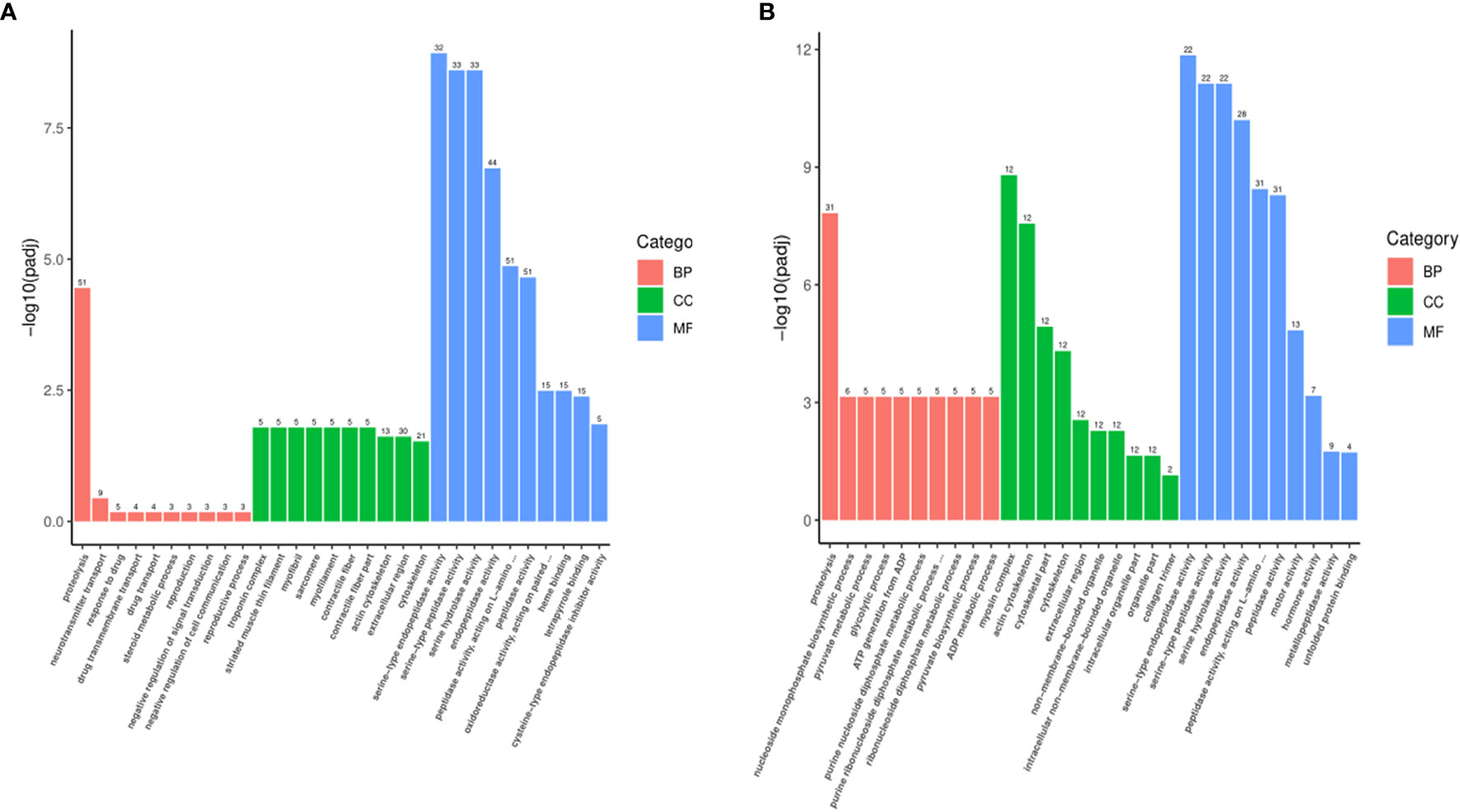
Figure 5 Gene ontology (GO) classifications of DEGs for MET_XX versus C_XX (A), and T4_XY versus C_XY (B).
According to KEGG analysis, the top 20 most enriched KEGG signaling pathways between groups are shown in Figure 6. In MET_XX versus C_XX, the most enriched KEGG pathways were neuroactive ligand-receptor interaction, calcium signaling pathway, cardiac muscle contraction, adrenergic signaling in cardiomyocytes, wnt signaling pathway, tight junction, steroid hormone biosynthesis, and phototransduction. In T4_XY versus C_XY, the most enriched KEGG pathways were neuroactive ligand-receptor interaction, glycolysis/gluconeogenesis, carbon metabolism, biosynthesis of amino acids, steroid hormone biosynthesis, protein processing in endoplasmic reticulum.
The Expression Profiles of foxl2, cyp19a1a, dmrt1, and gsdf in Response to MET and T4 Treatment by qPCR
In the control group, the expression levels of foxl2 and cyp19a1a were significantly higher in XX gonads than that in XY gonads, while the expression level of dmrt1 and gsdf mRNA were significantly higher in XY gonads than in XX gonads at 25, 40 and 55 dat (p < 0.05) (Figures 7 and 8). In the MET treatment group, the level of foxl2 and cyp19a1a expression in the genetic XX gonads was immediately decreased from 25 dat. At 55 dat, the level of foxl2 and cyp19a1a expression in MET treated-XX gonads was not significantly different from the control XY (p > 0.05). The expression of dmrt1 increased in the gonads of MET-treated XX T. rubripes from 25 dat, but was significantly lower than that in genetic XY individuals in the control group and in the MET-treated group at 40, and 55 dat (p < 0.05). Compared with the control groups, the expression of gsdf in genetic XX individuals in the MET-treated group did not increase at 25, 40, and 55 dat (p > 0.05) (Figure 7). After T4 treatment, the expression levels of cyp19a1a genes in genetic XY were significantly increased compared with the genetic XY in the control group at 25 dat (p < 0.05). However, the increasing tendency was not continued at 40 and 55 dat. At 25, 40, and 55 dat, the level of foxl2 expression in T4 treated-XY gonads was not significantly different from the control XY (p > 0.05). At 40 dat and 55 dat, the level of cyp19a1a expression in T4 treated-XY gonads was not significantly different from the control XY (p > 0.05). Moreover, compared to the control-XY, the significant decrease in the level of dmrt1 expression in the T4-treated-XY was only observed at 40 dat (p < 0.05). The significant increase in the level of gsdf expression in T4-treated-XY was observed at 55 dat when compared with the control-XY (p < 0.05) (Figure 8). Interestingly, a decreased expression of foxl2 and cyp19a1a in the T4-treated-XX was observed at 25 and 40 dat (p < 0.05).
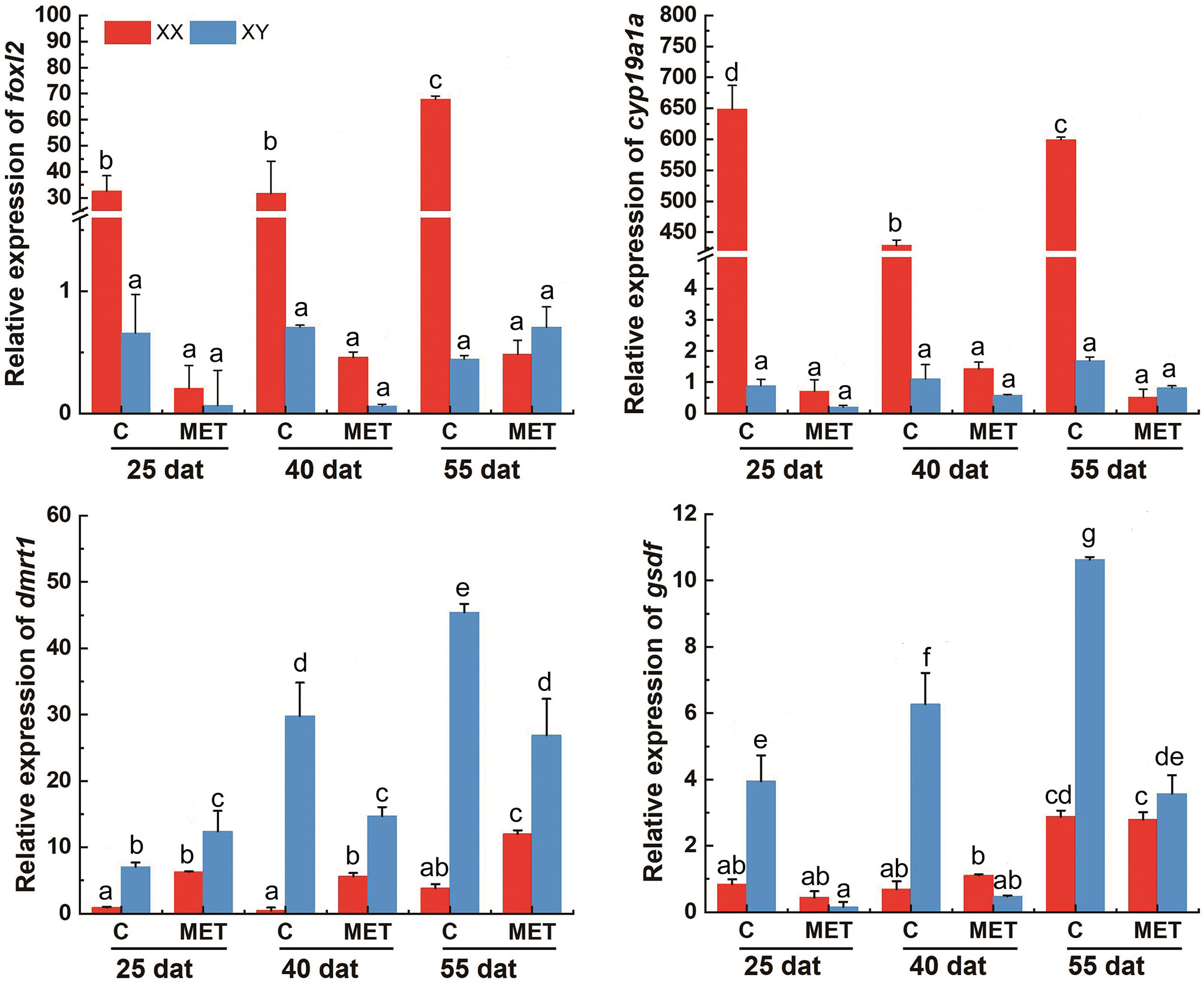
Figure 7 The levels of foxl2, cyp19a1a, dmrt1, and gsdf expression in T. rubripes gonads after MET treatment. C, Control group; MET, MET-treated group. Each value represents the mean ± SD of three measurements, different lowercase letters indicate significant differences between each treatment (one-way ANOVA, P < 0.05, n = 3); dat, days after treatment.
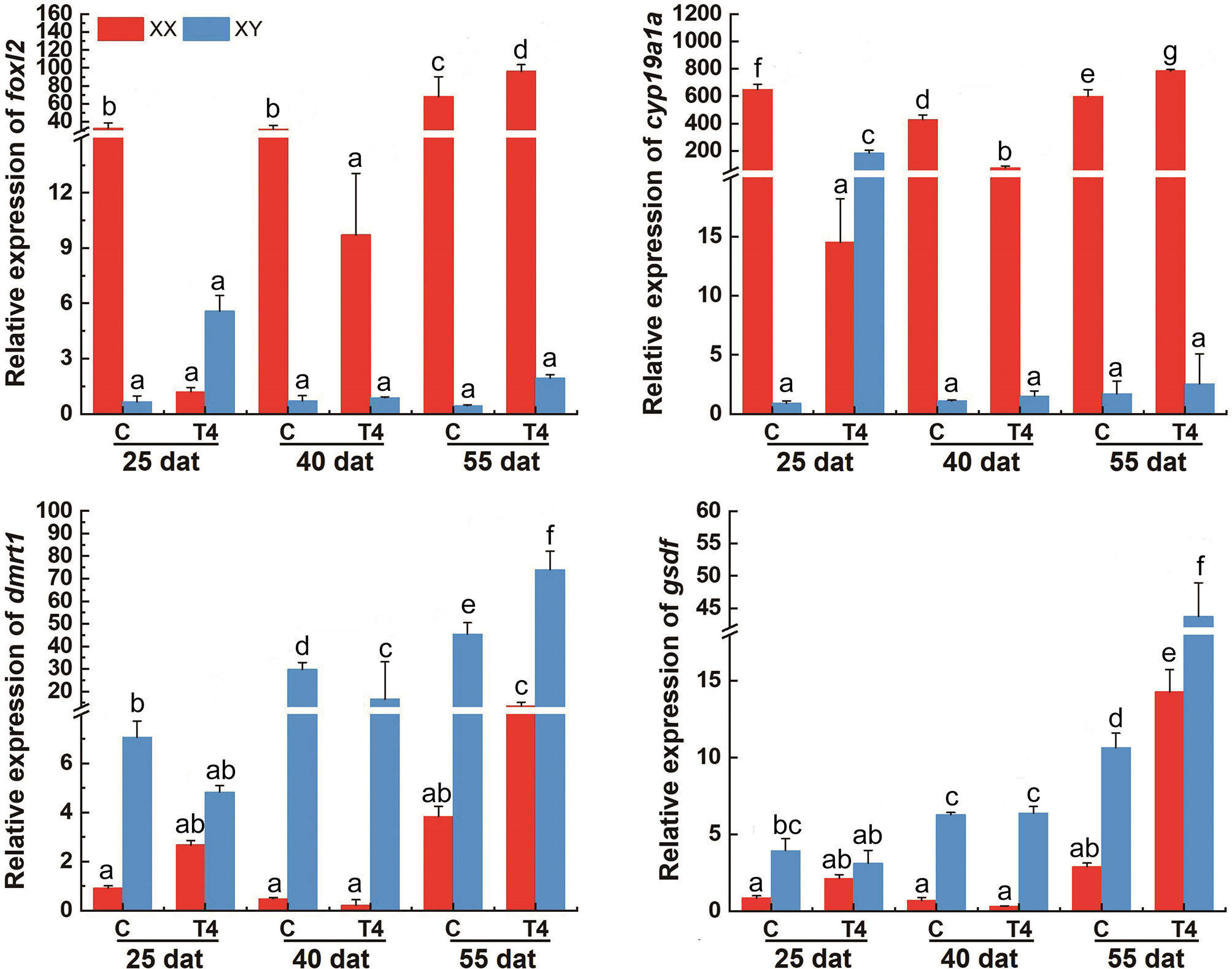
Figure 8 The levels of foxl2, cyp19a1a, dmrt1, and gsdf in T. rubripes gonads after T4 treatment. C, Control group; T4, T4-treated group. Each value represents the mean ± SD of three measurements, different lowercase letters indicate significant differences between each treatment (one-way ANOVA, P < 0.05, n = 3); dat, days after treatment.
Discussion
By the end of the study, exposure to MET did not affect T. rubripes body length, but did increase body weight and survival rate compared with controls. However, in zebrafish, MET treatment was shown to reduce the body length of the larvae (24). In addition, exposure of fathead minnow eggs to 100 ppm ammonium perchlorate from 0 to 28 days post-fertilization caused a reduction in body length (25). Ammonium perchlorate inhibits TH synthesis by inhibiting iodide uptake into the thyroid follicles. However, the survival rate of fathead minnow and zebrafish was not affected by perchlorate treatment (9, 25). Moreover, in the present study T4 did not affect T. rubripes survival, but increased growth compared with controls. This finding is different from reports in zebrafish, where lethally toxic effects of T4 was observed. In this case, T4 treatment causes several developmental effects, such as an abnormal acceleration in lower jaw growth (9). Taken together, these results demonstrated the effects of TH and TH inhibitors on growth and survival is species-specific. Yet, it is unknown why T4 exposure induced higher larval mortality (9). Aggressive behavior or cannibalism that is commonly found in fish can lead to mass mortality of larvae (26, 27). Loss of fish can also be attributed to increased bacterial or fungal infections, or even by fish cannibalism (28–30). Larvae of T. rubripes exhibit aggressive behavior and cannibalism from 6 dah (31). In the rearing period, most of the dead fish showed signs of aggression including dermal bite marks. It is possible that MET may decrease potential cannibalism, which resulted in a lower overall mortality rate in our present study. However, how the level of TH effects on cannibalism needs further study in the near future.
Previous works on the effect of the thyroid on sex differentiation based on zebrafish and three-spined sticklebacks reported contradictory results. For example, treatment of zebrafish T4-induced hyperthyroid conditions produced more female offspring, while treatment with goitrogen, perchlorate or MET induced hypothyroid conditions result in more male offspring during early development (9–11). However, perchlorate-induced hypothyroid conditions caused functional hermaphrodites by masculinizing genetically female threespine sticklebacks (12). Similar controversial results of hypothyroid induction of female or male biased populations at the time of gonadal sex differentiation were also observed in amphibians (13, 14). The present study found that MET could induce masculinization. This finding provides the first evidence that MET treatment can cause masculinization in teleosts. This finding is contrary to previous studies in zebrafish (10). Moreover, although this study used a TH dosage that has been used in zebrafish, TH-induced sex reversal was not observed under the overall experimental conditions. Further study should be conducted using a higher dosage to clarify this issue. It is critical to understand the effects of TH on sex differentiation and its underlying molecular mechanism in more teleost species. Collecting the tiny gonads from larvae and juvenile fish is challenging and the total RNA that can be extracted from a single gonad in T. rubripes is often insufficient. Additionally, RNA sample pooling strategies have also been used in other teleosts such as Nile tilapia (32, 33). Pooling RNA samples is a good option for insufficient RNA and is effective in maintaining statistical power when testing DEGs with low-to-medium abundance levels (34). Therefore, pooled RNA samples were used for RNA-seq without biological repeats. Moreover, sex-related gene expression was examined by qPCR with pooled samples in triplicate. These genes exhibited similar expression profiles using both qPCR analysis and FPKM values by transcriptome analysis in treated and control fugu gonads, suggesting that we obtained high quality and convincing gonadal transcriptome data.
Larvae treated with MET showed a suppression of genes involved in steroid hormone biosynthesis, such as foxl2 and cyp19a1a in genetic XX gonads. The expression of foxl2 and cyp19a1a at 25, 40, and 55 dat was also compared between the MET-treated group and the control group using qPCR. The results were in accordance with the results of RNA-Seq. Cytochrome P450 aromatase, which is encoded by cyp19a1a, is responsible for the transformation of androgens into estrogens (predominantly E2) in the ovary (35–37). Blockage of endogenous estrogen synthesis via an aromatase inhibitor (e.g., fadrozole, letrozole) during the critical period of sex differentiation has been demonstrated to induce masculinization in a wide range of teleosts (38–42). Recent studies also indicated that long-term aromatase inhibitor treatment could induce a complete sex reversal in adult females of medaka (Oryzias latipes), tilapia (Oreochromis niloticus), and zebrafish (D. rerio) (43–45). This study reports that cyp19a1a exhibited a significantly higher level in female compared with male T. rubripes larvae (22). This is in accordance with previous studies. Treated with fadrozole from 19 to 100 dah also inhibited the ovarian cavity formation of all individuals, and induced the subsequently testicular differentiation in T. rubripes larvae (16). These results suggested that cyp19a1a may play a critical role in ovarian differentiation in T. rubripes as in other teleosts (38–45). Similarly, MET-induced masculinization may primarily occur through suppression of cyp19a1a and the production of E2. In zebrafish, hyperthyroid conditions that reduced male-biased sex ratios were accompanied by decreased expression of cyp191a and aromatase activity thereby generating male-biased gonadal sex ratios (11). In some mammals, hypothyroidism enhances aromatase activity and 17b-estradiol content in Sertoli cells of male rats (46, 47). Treatment with TH inhibits aromatase activity and estrogen production by the ovary (48, 49) and testicular Sertoli cells (46, 47). Therefore, these results indicate that the underlying mechanisms by which TH affects sex determination in T. rubripes and other teleosts may be related by intricate crosstalk between TH and the sex steroid hormones that appear to play a critical role in sex determination and differentiation in teleosts (50–53). In zebrafish, although MET delays testicular differentiation (ovary-to-testis transformation) in genetic males, and thyroid hormone induced masculinization of genetic females, both T4 and MET inhibited cyp19a1a expression (11). Such controversial results may point to an underlying mechanism of TH and MET on cyp19a1a expression and even sex differentiation. This effect may also be species-specific. One possible suggestion is that T4 may bind to the promoter of cyp19a1a to regulate its expression. Indeed, in silico analyses of the T. rubripes cyp19a1a promoter showed that there were three thyroid response elements bearing a palindromic sequence similar to what was identified as the thyroid binding site (5’-TGACCT-3’, 5’-TGTCCT-3’) in clawed frogs (Silurana tropicalis) (54).
Foxl2 has been demonstrated function in the transcriptional regulation of cyp19a1a in Nile tilapia. Knockout of foxl2 in XX fish by TALEN led to decreased cyp19a1a expression and serum E2 levels, resulting in sex reversal (55–57). Here, a higher expression of foxl2 in MET-treated XX T. rubripes was observed compared with control XX. This may explain the increase of cyp19a1a expression. Moreover, one thyroid response element (5’-TGTCCT-3’) identified in S. tropicalis, was found in the promoter of T. rubripes foxl2 by in silico analysis (54). Further experimental confirmation of putative thyroid response element in the promoter regions of foxl2 and cyp19a1a in T. rubripes is required. Additionally, it was also found that T4 treatment induced an increased expression of cyp19a1a in genetic XY gonads at 25 dat. This result suggests that T4 was able to induce the expression of cyp19a1a. Conversely, the increased expression of cyp19a1a did not continue to 40 and 55 dat. This may explain why sex-reversal (testis to ovary) in larvae was not obtained in the T4-treated group. These results reinforced that further study should be conducted in future experiments. Additionally, the transcriptional analysis revealed that although the expression levels of foxl2 and cyp19a1a of the T4-treated genetic XY were significantly lower than the genetic XX, levels were higher than genetic XY in the control group at 55 dat. This result differed from the results of qPCR experiment. This may be attributed to the tiny gonads of T. rubripes larvae that collected at 80 dah. This was due to the fact that different samples were used in the RNA-Seq and qPCR experiment.
In this study, treatment with MET increased the dmrt1 expression in genetic XX T. rubripes. In silico analyses of T. rubripes dmrt1 promoter showed that there were five thyroid response elements, which was identified as the thyroid binding site in clawed frogs (Silurana tropicalis) (54). In vertebrates, several DM domain genes (dmrt genes) have been shown to be required for gametogenesis and gonadal differentiation. Dmrt1 seems to have a more significant role and is likely involved in testicular differentiation in all vertebrates (58). Our previous study reported that XY T. rubripes showed higher expression levels of dmrt1 in undifferentiated gonads compared to XX individuals (22). During the early life stages of T. rubripes, 17-beta estradiol treatment induced a decrease of dmrt1 and feminization in XY individuals (18, 19). Therefore, dmrt1 may play a pivotal role during T. rubripes testicular differentiation. In addition to the negative regulation of foxl2 and cyp19a1a, induction of male-biased sex ratios by MET may require stimulation of dmrt1. dmrt1 has been shown to repress cyp19a1a transcription directly in tilapia. Gain-function analysis indicated that dmrt1 overexpression in XX tilapia was able to decrease cyp19a1a expression and serum E2 levels that resulted in sex reversal (55). In medaka, a duplicate of the autosomal gene dmrt1 encoding a DM-domain-containing transcriptional factor, dmy, may also be able to inhibit Ad4BP/SF-1 activated cyp19a1a gene transcription (55). Knockdown of dmy can also induce an increase of cyp19a1a expression and sex reversal (59). However, the relationship between dmrt1 and cyp19a1a needs to be confirmed by future studies.
Results of the transcriptomic analysis showed that MET treatment can downregulate the expression of estrogen receptor 1. Estrogenics exert their function by binding to a specific receptor, the nuclear estrogen receptors (ERs) (60, 61). However, single knockout of esr1, esr2a, or esr2b did not result in sex reversal in zebrafish. While, double knockout of esr2a/b induced an arrest of folliculogenesis at the previtellogenic stage and subsequently affected the female-to-male sex reversal (62). Although cyp19a1a and both esr2a/b mutants induced female-to-male sex reversal, lack of aromatase inhibited ovarian differentiation whereas the loss of ERs failed to maintain ovarian status resulting in sex reversal. Their mechanisms were suggested to be different. Taken together, cyp19a1a is a reliable early marker of ovarian differentiation and is indispensable for ovarian differentiation/maintenance in fish. Thus, it was uncertain whether the down-regulation of er1 was a cause or a consequence of MET-induced masculinization. gsdf, a new TGFB superfamily member found only in fish, has been cloned in many gonochoristic (63–66) and hermaphroditic fish (67–69). In Oryzias luzonensis, gsdf on the Y chromosome has been confirmed to be a sex-determining gene (65). Gain- and loss- functional analysis demonstrated that this gene essential for teleost sex determination and differentiation (70–72). In the male undifferentiated gonads of T. rubripes, higher expression levels of gsdf were observed, suggesting that it may have a similar role as described in other teleosts (22). In silico analyses of T. rubripes gsdf promoter showed that there was one thyroid response element (5’-TGTCCT-3’) was identified as the thyroid binding site (46). Yet, gsdf expression is not sensitive to either MET or TH exposure. This suggests that changes in gsdf expression are not necessary for, nor associated with, TH-dependent masculinization in T. rubripes.
Deiodinases are responsible for TH peripheral metabolism. Thyroid receptors mediate TH activity at sites of action, and both are present within gonadal tissues (52, 53). Generally, Dio1 and Dio2 are responsible for catalyzing the conversion of T4 into the more active T3, and Dio3 is responsible for the conversion of T3 and T4 into the inactive metabolites reverse T3 (rT3) and T2 respectively. Previous studies have demonstrated that the maintenance of a baseline level of active THs by deiodinases could be necessary for vertebrate testes development. In teleosts, dio2 and dio3 mRNA levels are higher in the testes than in the ovaries of the striped parrotfish Scarus iseri (73). In O. mykiss, testes are characterized by higher transcripts of dio2, and dio2 expression, which is dependent on spermatogenic stages, increasing at the beginning of spermatogenesis (74). In the present study, no sexual dimorphic expression of dio1, dio2, dio3a, trα, and trβ were found between testes and ovaries, Likewise, no changes in their expression levels were found after T4 or MET treatment by RNA-Seq. Yet, dio3 was identified as DEGs between C_XX and C_XY fugu. Both T4 and MET treatment downregulated its expression in XY fugu. These results suggest that T4 or MET treatment had an effect on thyroid hormone homeostasis. Previous studies have shown that the androgen axis directly regulates TH synthesis, and there is considerable crosstalk between the androgen and TH axes (52, 53). In teleost fish, the main endogenous androgen is 11-ketotestosterone (11-KT) (75), and the steroidogenic enzymes 11β-hydroxylase (cyp11c1) and 11β-hydroxysteroid dehydrogenase 2 (hsd11b2), are responsible for converting testosterone and androstenedione to 11-KT (76–78). Here, the expression of cyp11c1 was suppressed in the T4 and MET-treated XY fugu. Taken together, these results suggested that T4 and MET treatment downregulated the expression of dio3 through suppression the expression of cyp11c1. Further research on these potential regulatory and feedback mechanisms should be conducted. Since the amount of total RNA extracted from gonads is limited, qPCR only verified the DEGs in our study. Detailed expression profiles of dio1, dio2, dio3a, dio3, trα, and trβ in the XX and XY gonads should be examined, and the functional analysis of those genes in the process of sex differentiation in fugu should be conducted in the future.
In conclusion, the present study provides the first evidence that MET treatment causes masculinization in teleost fish. The effects of MET-induced masculinization in T. rubripes may act primarily via suppression of the expression of foxl2 and cyp19a1a, and stimulation of the expression of dmrt1. Moreover, effects of higher concentrations of T4, or different concentrations of T3, on sex differentiation should be tested in the near future.
Data Availability Statement
The datasets presented in this study can be found in online repositories. The names of the repository/repositories and accession number(s) can be found below: https://www.ncbi.nlm.nih.gov/, SRR12364833 https://www.ncbi.nlm.nih.gov/, SRR12358231 https://www.ncbi.nlm.nih.gov/, SRR12364848 https://www.ncbi.nlm.nih.gov/, SRR12364847 https://www.ncbi.nlm.nih.gov/, SRR12364850 https://www.ncbi.nlm.nih.gov/,SRR12364849.
Ethics Statement
The animal study was reviewed and approved by Care and Use of Laboratory Animals in Dalian Ocean University, Dalian, China and the animal study ethical committee of Dalian Ocean University and comply with Chinese laws. Written informed consent was obtained from the owners for the participation of their animals in this study.
Author Contributions
Conceived and designed the experiments: HY, QL, and YL. Performed the experiments: ZY, XS, and JJ. Collected sample and performed the analyses: ZY, YW, XS, JJ, BL, LZ, and H Y. Wrote the paper: ZY, XS, and HY. All authors contributed to the article and approved the submitted version.
Funding
This research was supported by National Key R&D Program of China (2019YFD0900503), Youth Program of National Natural Science Foundation of China (31902347), Projects for Dalian Youth Star of Science and Technology (2019R0), Key R&D Program of Liaoning Province (2019JH2/10200015), Scientific, Technological and Innovation Program of Dalian (2018J12SN069) and General Project of Education Department of Liaoning Province (JL201904).
Conflict of Interest
The authors declare that the research was conducted in the absence of any commercial or financial relationships that could be construed as a potential conflict of interest.
References
1. Devlin YN, Nagahama Y. Sex Determination and Sex Differentiation in Fish an Overview of Genetic, Physiological, and Environmental Influences. Aquaculture (2002) 208:191–364. doi: 10.1016/s0044-8486(02)00057-1
2. Kobayashi Y, Nagahama Y, Nakamura M. Diversity and Plasticity of Sex Determination and Differentiation in Fishes. Sex Dev (2013) 7:115–25. doi: 10.1159/000342009
3. Liu H, Todd EV, Lokman PM, Lamm MS, Godwin JR, Gemmell NJ. Sexual Plasticity: A Fishy Tale. Mol Reprod Dev (2016) 84:171–94. doi: 10.1002/mrd.22691
4. Hayasaka O, Takeuchi Y, Shiozaki K, Anraku K, Kotani T. Green Light Irradiation During Sex Differentiation Induces Female-to-Male Sex Reversal in the Medaka Oryzias latipes. Sci Rep (2019) 9:15264–8. doi: 10.1038/s41598-019-38908-w
5. Rajakumar A, Senthilkumaran B. Steroidogenesis and its Regulation in Teleost-a Review. Fish Physiol Biochem (2020) 46:803–18. doi: 10.1007/s10695-019-00752-0
6. Hattori RS, Fernandino JI, Kishii A, Kimura H, Kinno T, Oura M, et al. Cortisol Induced Masculinization: Does Thermal Stress Affect Gonadal Fate in Pejerrey, a Teleost Fish With Temperature Dependent Sex Determination? PloS One (2009) 4:e6548. doi: 10.1371/journal.pone.0006548
7. Yamaguchi T, Yoshinaga N, Yazawa T, Gen K, Kitano T. Cortisol is Involved in Temperature-Dependent Sex Determination in the Japanese Flounder. Endocrinology (2010) 151:3900–8. doi: 10.1210/en.2010-0228
8. Blanton ML, Specker JL. The Hypothalamic-Pituitary-Thyroid (HPT) Axis in Fish and Its Role in Fish Development and Reproduction. Crit Rev Toxicol (2007) 37:97–115. doi: 10.1080/10408440601123529
9. Mukhi S, Torres L, Patiño R. Effects of Larval–Juvenile Treatment With Perchlorate and Co-Treatment With Thyroxine on Zebrafish Sex Ratios. Gen Comp Endocrinol (2007) 150:486–94. doi: 10.1016/j.ygcen.2006.11.013
10. Sharma P, Patiño R. Regulation of Gonadal Sex Ratios and Pubertal Development by the Thyroid Endocrine System in Zebrafish (Danio rerio). Gen Comp Endocrinol (2013) 184:111–9. doi: 10.1016/j.ydbio.2006.10.001
11. Sharma P, Tang S, Mayer GD, Patiño R. Effects of Thyroid Endocrine Manipulation on Sex-Related Gene Expression and Population Sex Ratios in Zebrafish. Ge Comp Endocrinol (2016) 235:38–47. doi: 10.1016/j.ygcen.2016.05.028
12. Bernhardt RR, Von Hippel FA, Cresko WA. Perchlorate Induces Hermaphroditism in Threespine Sticklebacks. Environ Toxicol Chem (2006) 25:2087–96. doi: 10.1897/05-454R.1
13. Hayes TB. Sex Determination and Primary Sex Differentiation in Amphibians: Genetic and Developmental Mechanisms. J Exp Zool (1998) 281:373–99. doi: 10.1002/(sici)1097-010x(19980801)281:5<373::aid-jez4>3.0.co;2-l
14. Goleman WL, Carr JA, Anderson TA. Environmentally Relevant Concentrations of Ammonium Perchlorate Inhibit Thyroid Function and Alter Sex Ratios in Developing Xenopus laevis. Environ Toxicol Chem (2002) 21:590–7. doi: 10.1002/etc.5620210318
15. Aparicio S, Chapman J, Stupka E, Putnam N, Chia JM, Dehal P, et al. Whole Genome Shotgun Assembly and Analysis of the Genome of Fugu rubripes. Science (2002) 297:1301–10. doi: 10.1126/science.1072104
16. Kamiya T, Kai W, Tasumi S, Oka A, Matsunaga T, Mizuno N, et al. A Transspecies Missense SNP in Amhr2 is Associated With Sex Determination in the Tiger Pufferfish, Takifugu rubripes (fugu). PloS Genet (2012) 8:e1002798. doi: 10.1371/journal.pgen.1002798
17. Rashid H, Kitano H, Lee KH, Nii S, Shigematsu T, Kadomura K, et al. Fugu (Takifugu rubripes) Sexual Differentiation: CYP19 Regulation and Aromatase Inhibitor Induced Testicular Development. Sex Dev (2008) 1:311–22. doi: 10.1159/000108935
18. Lee KH, Yamaguchi A, Rashid H, Kadomura K, Yasumoto S, Matsuyama M. Estradiol-17beta Treatment Induces Intersexual Gonadal Development in the Pufferfish, Takifugu rubripes. Zool Sci (2009) 26:639–45. doi: 10.2108/zsj.26.639
19. Lee KH, Yamaguchi A, Rashid H, Kadomura K, Yasumoto S, Matsuyama M. Germ Cell Degeneration in High Temperature Treated Pufferfish, Takifugu rubripes. Sex Dev (2009) 3:225–32. doi: 10.1159/000228723
20. Hu P, Liu B, Ma Q, Liu SF, Liu XF, Zhuang ZM. Expression Profiles of Sex-Related Genes in Gonads of Genetic Male Takifugu rubripes after 17β-estradiol immersion. Chin J Oceanol Limnol (2019) 37:1113–24. doi: 10.1007/s00343-019-8060-x
21. Bergot P, Charlon N, Durante H. The Effect of Compound Diets Feeding on Growth Survival of Coregonid Larvae. Arch für Hydrobiol (1986) 22:265–72.
22. Yan HW, Shen XF, Cui X, Wu YM, Wang LS, Zhang L, et al. Identification of Genes Involved in Gonadal Sex Differentiation and the Dimorphic Expression Pattern in Takifugu rubripes gonad at the early stage of sex differentiation. Fish Physiol Biochem (2018) 44:1275–90. doi: 10.1007/s10695-018-0519-8
23. Bai DP, Chen Y, Hu YQ, He WF, Shi YZ, Fan QM, et al. Transcriptome Analysis of Genes Related to Gonad Differentiation and Development in Muscovy Ducks. BMC Genomics (2020) 21:438–55. doi: 10.1186/s12864-020-06852-z
24. Lam SH, Sin YM, Gong Z, Lam TJ. Effects of Thyroid Hormone on the Development of Immune System in Zebrafish. Gen Comp Endocrinol (2005) 142:325–35. doi: 10.1016/j.ygcen.2005.02.004
25. Crane HM, Pickford DB, Hutchinson TH, Brown JA. Effects of Ammonium Perchlorate on Thyroid Function in Developing Fathead Minnows, Pimephales promelas. Environ Health Perspect (2005) 113:396–401. doi: 10.1289/ehp.7333
26. Smith C, Reay P. Cannibalism in Teleost Fish. Rev Fish Biol Fisher (1991) 1:41–64. doi: 10.1007/BF00042661
27. Ruzzante DE. Domestication Effects on Aggressive and Schooling Behavior in Fish. Aquaculture (1994) 120:0–24. doi: 10.1016/0044-8486(94)90217-8
28. Ohgami H, Suzuki Y. The Influence of Rearing Condition on Survival and Cannibalism on Fingerlings of Tiger Puffer (Takifugu rubripes) (in Japanese). Bull Shizuoka Pref Fish Exp Stat (1982) 16:79–85.
29. Han KN, Matsui S, Furuichi M, Kitajima C. Effect of Stocking Density on Growth, Survival Rate, and Damage of Caudal Fin in Larval to Young Puffer Fish, Takifugu rubripes (in Japanese). Suisan Zoshoku (1994) 42:507–14. doi: 10.11233/aquaculturesci1953.42.507
30. Iwamoto A, Fujimoto H. Present Status of Production of Puffer Fry for Release. Fisheries Stock Managements Ocellate puffer Takifugu rubripes Japan (1997) 1:97–109.
31. Suzuki N, Okada K, Kamiya N. Organogenesis and Behavioral Changes During Development of Laboratory-Reared Tiger Puffer, Takifugu rubripes (in Japanese). Suisan Zoshoku (1995) 43:461–74. doi: 10.11233/aquaculturesci1953.43.461
32. Tao W, Yuan J, Zhou L, Sun L, Sun Y, Yang S, et al. Characterization of Gonadal Transcriptomes From Nile Tilapia (Oreochromis niloticus) Reveals Differentially Expressed Genes. PloS One (2013) 8:e63604. doi: 10.1371/journal.pone.0063604
33. Sun S, Cai J, Tao W, Wu L, Tapas C, Zhou L, et al. Comparative Transcriptome Profiling and Characterization of Gene Expression for Ovarian Differentiation Under RU486 Treatment. Gen Comp Endocrinol (2018) 261:166–73. doi: 10.1016/j.ygcen.2018.03.005
34. Takele Assefa A, Vandesompele J, Thas O. On the Utility of RNA Sample Pooling to Optimize Cost and Statistical Power in RNA Sequencing Experiments. BMC Genomics (2020) 21:312. doi: 10.1186/s12864-020-6721-y
35. Young G, Lokman PM, Kusakabe M, Nakamura I, Goetz FW. Gonadal Steroidogenesis in Teleost Fish. Mol Asp Fish Mar Biol (2005) 2:155–223. doi: 10.1142/9789812569189_0006
36. Nagahama Y, Yamashita M. Regulation of Oocyte Maturation in Fish. Dev Growth Differ (2010) 50:195–219. doi: 10.1111/j.1440-169X.2008.01019.x
37. Tokarz J, Möller G, Hrabě de Angelis M, Adamski J. Steroids in Teleost Fishes. A Functional Point of View. Steroids (2015) 103:123–44. doi: 10.1016/j.steroids.2015.06.011
38. Piferrer F, Zanuy S, Carrillo M, Solar I, Devlin RH, Donaldson EM. Brief Treatment With an Aromatase Inhibitor During Sex Differentiation Causes Chromosomally Female Salmon to Develop as Normal, Functional Males. J Exp Zool (2010) 270:255–62. doi: 10.1002/jez.1402700304
39. Kitano T, Takamune K, Nagahama Y, Abe SI. Aromatase Inhibitor and 17α- Methyltestosterone Cause Sex-Reversal From Genetical Females to Phenotypic Males and Suppression of P450 Aromatase Gene Expression in Japanese Flounder (Paralichthys olivaceus). Mol Reprod Dev (2000) 56:1–5. doi: 10.1002/(sici)1098-2795(200005)56:1<1:aid-mrd1>3.0.co;2-3
40. Fenske M, Segner H. Aromatase Modulation Alters Gonadal Differentiation in Developing Zebrafish (Danio Rerio). Aquat Toxicol (2004) 67:105–26. doi: 10.1016/j.aquatox.2003.10.008
41. Komatsu T, Nakamura S, Nakamura M. Masculinization of Female Golden Rabbitfish Siganus guttatus Using an Aromatase Inhibitor Treatment During Sex Differentiation. Comp Biochem Physiol (2006) 143:402–9. doi: 10.1016/j.cbpc.2006.04.015
42. Thresher R, Gurney R, Canning M. Effects of Lifetime Chemical Inhibition of Aromatase on the Sexual Differentiation, Sperm Characteristics and Fertility of Medaka (Oryzias latipes) and Zebrafish (Danio rerio). Aquat Toxicol (2011) 105:355–60. doi: 10.1016/j.aquatox.2011.07.008
43. Paul-Prasanth B, Bhandari RK, Kobayashi T, Horiguchi R, Kobayashi Y, Nakamoto M, et al. Estrogen Oversees the Maintenance of the Female Genetic Program in Terminally Differentiated Gonochorists. Sci Rep (2013) 3:2862. doi: 10.1038/srep02862
44. Takatsu K, Miyaoku K, Roy SR, Murono Y, Sago T, Itagaki H, et al. Induction of Female-to-Male Sex Change in Adult Zebrafish by Aromatase Inhibitor Treatment. Sci Rep (2013) 3:3400. doi: 10.1038/srep03400
45. Sun LN, Jiang XL, Xie QP, Yuan J, Huang BF, Tao WJ, et al. Transdifferentiation of Differentiated Ovary Into Functional Testis by Long Term Treatment of Aromatase Inhibitor in Nile Tilapia. Endocrinology (2014) 155:1476–88. doi: 10.1210/en.2013-1959
46. Panno ML, Beraldi E, Pezzi V, Salerno M, De LG, Lanzino M, et al. Influence of Thyroid Hormone on Androgen Metabolism in Peripuberal Rat Sertoli Cells. J Endocrinol (1994) 140:349–55. doi: 10.1677/joe.0.1400349
47. Panno ML, Sisci D, Salerno M, Lanzino M, Pezzi V, Morrone EG, et al. Thyroid Hormone Modulates Androgen and Oestrogen Receptor Content in the Sertoli Cells of Peripubertal Rats. J Endocrinol (1996) 148:43–50. doi: 10.1677/joe.0.1480043
48. Gregoraszczuk EL, Slomczynska M, Wilk R. Thyroid Hormone Inhibits Aromatase Activity in Porcine Thecal Cells Cultured Alone and in Coculture With Granulosa Cells. Thyroid (1998) 8:1157–63. doi: 10.1089/thy.1998.8.1157
49. Hatsuta M, Tamura K, Shimizu Y, Toda K, Kogo H. Effect of Thyroid Hormone on CYP19 Expression in Ovarian Granulosa Cells From Gonadotropin-Treated Immature Rats. J Pharmacol Sci (2004) 94:420–5. doi: 10.1254/jphs.94.420
50. Habibi HR, Nelson ER, Allan ERO. New Insights Into Thyroid Hormone Function and Modulation of Reproduction in Goldfish. Gen Comp Endocrinol (2012) 175:19–26. doi: 10.1016/j.ygcen.2011.11.003
51. Liu C, Zhang X, Deng J, Hecker M, Al-Khedhairy A, Giesy JP, et al. Effects of Prochloraz or Propylthiouracil on the Cross-Talk Between the HPG, HPA, and HPT Axes in Zebrafish. Environ Sci Technol (2011) 45:769–75. doi: 10.1021/es102659p
52. Flood D, Fernandino JI, Langlois VS. Thyroid Hormones in Male Reproductive Development: Evidence for Direct Crosstalk Between the Androgen and Thyroid Hormone Axes. Gen Comp Endocrinol (2013) 192:2–14. doi: 10.1016/j.ygcen.2013.02.038
53. Tovo-Neto A, Rodrigues S, Habibi HR, Rafael HN. Thyroid Hormone Actions on Male Reproductive System of Teleost Fish. Gen Comp Endocrinol (2018) 265:230–6. doi: 10.1016/j.ygcen.2018.04.023. S0016648017308171.
54. Campbell Diana EK, Langlois VS. Expression of Sf1 and Dax-1 are Regulated by Thyroid Hormones and Androgens During Silurana tropicalis Early Development. Gen Comp Endocrinol (2017) 259:33–4. doi: 10.1016/j.ygcen.2017.10.017
55. Wang DS, Zhou LY, Kobayashi T, Matsuda M, Shibata Y, Sakai F, et al. Doublesex- and Mab-3-related Transcription Factor-1 Repression of Aromatase Transcription, a Possible Mechanism Favoring the Male Pathway in Tilapia. Endocrinology (2010) 151:1331–40. doi: 10.1210/en.2009-0999
56. Li MH, Yang HH, Li MR, Sun YL, Jiang XL, Xie QP, et al. Antagonistic Roles of Dmrt1 and Foxl2 in Sex Differentiation via Estrogen Production in Tilapia as Demonstrated by Talens. Endocrinology (2013) 154:4814–25. doi: 10.1210/en.2013-1451
57. Zhang X, Li M, Ma H, Liu X, Shi H, Li M, et al. Mutation of Foxl2 or cyp19a1a Results in Female to Male Sex Reversal in XX Nile Tilapia. Endocrinology (2017) 158:2634–47. doi: 10.1210/en.2017-00127
58. Zarkower D. DMRT Genes in Vertebrate Gametogenesis. Curr Top Dev Biol (2013) 102:327–56. doi: 10.1016/B978-0-12-416024-8.00012-X
59. Chakraborty T, Zhou LY, Chaudhari A, Iguchi T, Nagahama Y. Dmy Initiates Masculinity by Altering Gsdf/Sox9a2/Rspo1 Expression in Medaka (Oryzias latipes). Sci Rep (2016) 6:19480. doi: 10.1038/srep19480
60. Klinge CM. Estrogen Receptor Interaction With Co-Activators and Co-Repressors. Steroids (2000) 65:227–51. doi: 10.1016/S0039-128X(99)00107-5
61. Hewitt SC, Korach KS. Estrogen Receptors: Structure, Mechanisms and Function. Rev Endocr Metab Disord (2002) 3:193–200. doi: 10.1023/A:1020068224909
62. Lu H, Cui Y, Jiang L, Ge W. Functional Analysis of Nuclear Estrogen Receptors in Zebrafish Reproduction by Genome Editing Approach. Endocrinology (2017) 158:2292–308. doi: 10.1210/en.2017-00215
63. Sawatari E, Shikina S, Takeuchi T, Yoshizaki G. A Novel Transforming Growth Factor-Beta Superfamily Member Expressed in Gonadal Somatic Cells Enhances Primordial Germ Cell and Spermatogonial Proliferation in Rainbow Trout (Oncorhynchns mykiss). Dev Biol (2007) 301:266–75. doi: 10.1016/j.ydbio.2006.10.001
64. Gautier A, Le GF, Lareyre JJ. The Gsdf Gene Locus Harbors Evolutionary Conserved and Clustered Genes Preferentially Expressed in Fish Previtellogenic Oocytes. Gene (2011) 472:7–17. doi: 10.1016/j.gene.2010.10.014
65. Myosho T, Otake H, Masuyama H, Matsuda M, Kuroki Y, Fujiyama A, et al. Tracing the Emergence of a Novel Sex-Determining Gene in Medaka, Oryzias luzonensis. Genetics (2012) 191:163–70. doi: 10.1534/genetics.111.137497
66. Kaneko H, Ijiri S, Kobayashi T, Izumi H, Kuramochi Y, Wang DS, et al. Gonadal Soma-Derived Factor (Gsdf), a TGF-beta Superfamily Gene, Induces Testis Differentiation in the Teleost Fish Oreochromis niloticus. Mol Cell Endocrinol (2015) 415:87–99. doi: 10.1016/j.mce.2015.08.008
67. Horiguchi R, Nozu R, Hirai T, Kobayashi Y, Nagahama Y, Nakamura M. Characterization of Gonadal Somaderived Factor Expression During Sex Change in the Protogjoious Wrasse, Halichoeres trimaculatus. Dev Dyn (2013) 242:388–99. doi: 10.1002/dvdy.23929
68. Chen Y, Hong WS, Wang Q, Chen SX. Cloning and Expression Pattern of Gsdf During the First Maleness Reproductive Phase in the Protandrous Acanthopagrus Latus. Gen Comp Endocrinol (2015) 217–218:71–80. doi: 10.1016/j.ygcen.2015.02.018
69. Zhu Y, Wang C, Chen X, Guan G. Identification of Gonadal Soma-Derived Factor Involvement in Monopterus albus (Protogynous Rice Field Eel) Sex Change. Mol Biol Rep (2016) 43:629–37. doi: 10.1007/s11033-016-3997-8
70. Imai T, Saino K, Matsuda M. Mutation of Gonadal Soma Derived Factor Induces Medaka XY Gonads to Undergo Ovarian Development. Biochem Biophys Res Commun (2015) 467:109–14. doi: 10.1016/j.bbrc.2015.09.112
71. Jiang DN, Yang HH, Li MH, Shi HJ, Zhang XB, Wang DS. Gsdf is a Downstream Gene of Dmrt1 That Functions in the Male Sex Determination Pathway of the Nile Tilapia. Mol Rep Dev (2016) 83:497–508. doi: 10.1002/mrd.22642
72. Zhang X, Guan GJ, Li MY, Zhu F, Liu QZ, Kiyoshi N, et al. Autosomal Gsdf Acts as a Male Sex Initiator in the Fish Medaka. Sci Rep (2016) 6:19738. doi: 10.1038/srep1973
73. Johnson KM, Lema SC. Tissue-Specific Thyroid Hormone Regulation of Gene Transcripts Encoding Iodothyronine Deiodinases and Thyroid Hormone Receptors in Striped Parrotfish (Scarus iseri). Gen Comp Endocrinol (2011) 172:505–17. doi: 10.1016/j.ygcen.2011.04.022
74. Sambroni E, Gutieres S, Cauty C, Guiguen Y, Breton B, Lareyre JJ. Type II Iodothyronine Deiodinase is Preferentially Expressed in Rainbow Trout (Oncorhynchus mykiss) Liver and Gonads. Mol Reprod Dev (2001) 60:338–50. doi: 10.1002/mrd.1096
75. Borg B. Androgens in Teleost Fishes. Comp Biochem Physiol C (1994) 109(3):219–45. doi: 10.1016/0742-8413(94)00063-G
76. Kusakabe M, Kobayashi T, Todo T, Mark Lokman P, Nagahama Y, Young G. Molecular Cloning and Expression During Spermatogenesis of a cDNA Encoding Testicular 11β-Hydroxylase (P45011β) in Rainbow Trout (Oncorhynchus mykiss). Mol Reprod Dev (2002) 62(4):456–69. doi: 10.1002/mrd.10145
77. Kusakabe M, Nakamura I, Young G. 11β-Hydroxysteroid Dehydrogenase Complementary Deoxyribonucleic Acid in Rainbow Trout: Cloning, Sites of Expression, and Seasonal Changes in Gonads. Endocrinology (2003) 144(6):2534–45. doi: 10.1210/en.2002-220446
Keywords: Takifugu rubripes, methimazole, thyroid hormone, sex differentiation, RNA-seq
Citation: Yuan Z, Shen X, Yan H, Jiang J, Liu B, Zhang L, Wu Y, Liu Y and Liu Q (2021) Effects of the Thyroid Endocrine System on Gonadal Sex Ratios and Sex-Related Gene Expression in the Pufferfish Takifugu rubripes. Front. Endocrinol. 12:674954. doi: 10.3389/fendo.2021.674954
Received: 02 March 2021; Accepted: 20 April 2021;
Published: 07 May 2021.
Edited by:
Antonio Brunetti, University of Catanzaro, ItalyReviewed by:
Gustavo M. Somoza, CONICET Instituto Tecnológico de Chascomús (INTECH), ArgentinaJuan Ignacio Fernandino, CONICET Institute of Biotechnological Research (IIB-INTECH), Argentina
Copyright © 2021 Yuan, Shen, Yan, Jiang, Liu, Zhang, Wu, Liu and Liu. This is an open-access article distributed under the terms of the Creative Commons Attribution License (CC BY). The use, distribution or reproduction in other forums is permitted, provided the original author(s) and the copyright owner(s) are credited and that the original publication in this journal is cited, in accordance with accepted academic practice. No use, distribution or reproduction is permitted which does not comply with these terms.
*Correspondence: Hongwei Yan, yanhongwei_1985@hotmail.com; Qi Liu, liuqisunson@163.com
†These authors have contributed equally to this work