- 1Department of Emergency and Organ Transplantation, Section of Internal Medicine, Endocrinology, Andrology and Metabolic Diseases, University of Bari Aldo Moro, Bari, Italy
- 2Endocrinology Unit, IRCCS Ospedale Policlinico San Martino, Genova, Italy
- 3Department of Internal Medicine, University of Genova, Genova, Italy
- 4Neuroendocrine Unit, Department of Medical, Surgical and Experimental Sciences, University of Sassari—Endocrine Unit, Azienda Ospedaliera Universitaria Sassari, Sassari, Italy
- 5IRCCS Ospedale Policlinico San Martino, Genova, Italy
- 6Department of Experimental Medicine, “Sapienza” University of Rome, Rome, Italy
- 7Neuroendocrinology, Neuromed Institute, IRCCS, Pozzilli, Italy
- 8Department of Clinical Medicine and Surgery, Endocrinology Unit, University Federico II, Naples, Italy
- 9Endocrinology Unit, Department of Clinical and Molecular Medicine, Sant’Andrea Hospital, Sapienza University of Rome, Rome, Italy
Medullary thyroid carcinoma is a rare neuroendocrine neoplasm that originates from thyroid C cells. Surgery, with complete resection of the tumor, is the only curative approach. However, in most cases, the tumor recurs at locoregional or metastatic level. In this setting, the management remains challenging. In recent years, the immune checkpoint inhibitors have provided promise for changing the cancer treatment paradigm through the application of new approaches that enhance the body’s natural antitumor defenses. The aim of this review is to summarize and discuss available data on efficacy and safety of the Food and Drug Administration-approved immune checkpoint inhibitors in patients with medullary thyroid carcinoma. After an extensive search, we found 7 useful data sources (one single-case report, one short article with very preliminary data, five ongoing registered clinical trials). Despite the lack of published evidence regarding the use of immune check point inhibitors, it must be considered that all the ongoing registered clinical trials saw first light in the last three years, thus indicating a growing interest of researchers in this field. Results coming from these trials, and hopefully, in the next future, from additional trials, will help to clarify whether this class of drugs may represent a new weapon in favor of patients with medullary thyroid carcinoma.
Introduction
Medullary thyroid carcinoma (MTC) is a rare neuroendocrine neoplasm (NEN) that originates from thyroid C cells.
Surgery, with complete resection of the tumor, is the only curative approach (1). However, in many patients, the tumor displays an aggressive behavior, resulting in persistence or locoregional and distant disease recurrence. In this setting, the management remains challenging (1, 2).
Tyrosine kinase inhibitors (TKIs) vandetanib and cabozantinib have shown to improve progression-free survival (PFS), and are currently available as approved agents for the treatment of progressive MTC. However, both drugs may cause grade III or IV adverse events (AEs), classified according to the Common Terminology Criteria for Adverse Events of the National Cancer Institute (3, 4).
In 2020, the new generation TKIs selpercatinib and praseltinib gained the Food and Drug Administration (FDA) approval in patients with advanced/metastatic rearranged during transfection (RET) gene-mutant MTC who require systemic therapy, therefore widening the spectrum of available therapies. However, also for these drugs severe AEs have been reported (5, 6)
Therapeutic options also include radionuclide therapy, such as peptide receptor radionuclide therapy (i.e. lutetium-177 and yttrium-90 labeled somatostatin analogs) (7), and iodine-131-metaiodobenzylguanidine (8). However, radionuclide therapy is not approved for MTC treatment.
In recent years, immunotherapy has provided promise for changing the cancer treatment paradigm through the application of new approaches that enhance the body’s natural antitumor defenses.
One of the main mechanisms by which tumors escape host immune surveillance is the so-called cancer immunoediting. Acting on immune checkpoints, tumor cells promote the development of an immunosuppressive environment, to prevent the activation of T cell cytotoxicity. Thus the interfering with immune checkpoint signaling, to restore T cell functioning, is nowadays considered one of the most effective novel antitumor treatment goals (9). To date, antibodies targeted against the cytotoxic T-lymphocyte-associated protein 4 (CTLA-4) (i.e. ipilimumab), the programmed cell death protein-1 (PD-1) (i.e. cemiplimab, nivolumab, and pembrolizumab), and the programmed death-ligand-1 (PD-L1) (i.e. atezolizumab, avelumab, and durvalumab), have been approved by the FDA for human use with the aim to re-activate patient antitumor immunity (Figure 1). These drugs, referred as immune checkpoints inhibitors (ICIs), demonstrated significant clinical effectiveness in the treatment of advanced solid tumors and a favorable safety profile, so that entered in the standard clinical practice for several malignancies (10) (Table 1).
The inhibitory co-receptor CTLA-4 is constitutively expressed by immunosuppressive regulatory T cells (Tregs), but it can be induced in T cells when activated by antigen-presenting cells (APC). In resting T cells, CTLA-4 is intracellularly localized in endosomes, but, upon T cell receptor (TCR) and CD28 costimulatory signaling activation, CTLA-4 translocates to the cell membrane (11). When exposed on cell surface, CTLA-4 prevents CD28 binding to B7.1 and B7.2 on APCs, thus precluding activation and proliferation of T cells (12). In fact, CTLA-4 inhibition causes a major immunostimulation, as experimentally shown in CTLA-4 knock-out (KO) mice, which die after few months due to uncontrolled lymphoproliferative disorders (13) and, in a clinical setting, by the reactivation of T cell-mediated tumor rejection.
Binding of PD-1 to its ligands, PD-L1 and programmed death-ligand-2 (PD-L2), also prevents T cell activation. PD-L1 is an inducible protein expressed in innate and adaptive immune cells, mesenchymal cells, and cancer cells (14), while PD-L2 is mainly expressed by APCs. PD-1 binding to PD-L1 significantly prevents immune responses directed against cancer cells, thereby altering T cell cytokine release, inhibiting TCR signaling, and abridging T cells/APCs interactions (15). The relevant role of this system in controlling T cell activity was demonstrated in PD-1-KO mice, which develop a spontaneous lupus-like disease caused by unrestrained autoreactive T cells (16). On the other hand, the inhibition of PD-1/PD-L1 binding in cancer can promote T cell activation and proliferation, ultimately leading to cytotoxicity in tumors.
MTC is reported to exhibit low PD-L1 expression in both tumor cells and tumor-infiltrating immune cells (17–19) and no microsatellite instability, irrespective of the presence/absence of either desmoplasia, lymph node metastases and/or RET mutation (18, 20).
However, PD-L1 positivity is associated with aggressive clinicopathological features (e.g., larger tumor size, lymph node or distant metastasis and higher TNM stage) (18, 19) and accounted as a predictor of structural recurrence and biochemical recurrence/persistent disease (19), and CTLA-4 expression is also correlated with advanced staging and structural recurrence-free survival (21), thus suggesting a possible prognostic role in the management of MTC (22).
Aim
The aim of this review is to summarize and discuss available data on efficacy and safety of FDA-approved ICIs in patients with MTC.
Materials and Methods
Published Articles
We performed a literature search in the international online databases (PubMed, Web of Science, Scopus, and Embase) using the following terms: “immune checkpoint inhibitors”, CTLA-4, PD-L1, PD-1, atezolizumab, avelumab, cemiplimab, durvalumab, ipilimumab, nivolumab, pembrolizumab, “medullary thyroid cancer”, “medullary thyroid carcinoma”, “thyroid cancer”, “multiple endocrine neoplasia type 2”.
The search was last updated February 14, 2021.
Registered Clinical Trials
By using the same keywords adopted for reviewing published articles, we conducted an in-depth search in the ClinicalTrials.gov registry, European Clinical Trials Database, and China Clinical Trials Register.
The search was last updated February 14, 2021.
Results
Published Articles
The initial literature search revealed a total of 108 published articles, two of which were pertinent to the study objectives.
Del Rivero et al. have recently reported the case of a 61-year-old male with recurrent MTC (23) showing sharp decline in serum calcitonin level while on avelumab. The patient had been successfully treated with off-label sunitinib for 5 years, but he was forced to withdraw the drug due to relevant side effects. He was then enrolled on a clinical trial with a yeast-based, CEA-targeted, therapeutic cancer vaccine (GI-6207) (24), and his calcitonin doubling time improved in 3 months. He then chose to have elective surgery to remove a neck lymph node and, per protocol, the vaccine was discontinued. Three months after surgery, his calcitonin level was still rising and he was enrolled on a phase I, open-label, multiple-ascending dose trial of avelumab (Avelumab in Metastatic or Locally Advanced Solid Tumors [JAVELIN Solid Tumor], NCT01772004). Thereafter, his calcitonin level decreased > 40% on 5 consecutive evaluations, and response assessment by RECIST v1.1 criteria (25) reported stable disease. However, an immune-related AE (i.e., asymptomatic grade 3 rise in lipase) ultimately led to mandatory treatment discontinuation. A subsequent analysis of a patient’s lymph node (resected post-vaccination) revealed that the tumor was PD-L1 positive.
Very preliminary results of a phase II trial evaluating nivolumab plus ipilimumab in patients with aggressive thyroid cancer (NCT03246958) are also available (26). Indeed, 7 patients with progressive MTC and prior TKI failure were included in an exploratory cohort of the study and assessed for radiographic response based on RECIST v1.1 criteria. Lack of partial response is reported for all the 7 patients, without giving further detail. Also, no safety information is provided for MTC as a single cohort (please see the Registered Clinical Trials section for more comprehensive description of the trial design).
Registered Clinical Trials (RCTs)
We found 37 registered clinical trials (RCTs), five of which fully matched the aim of this review (Table 2).
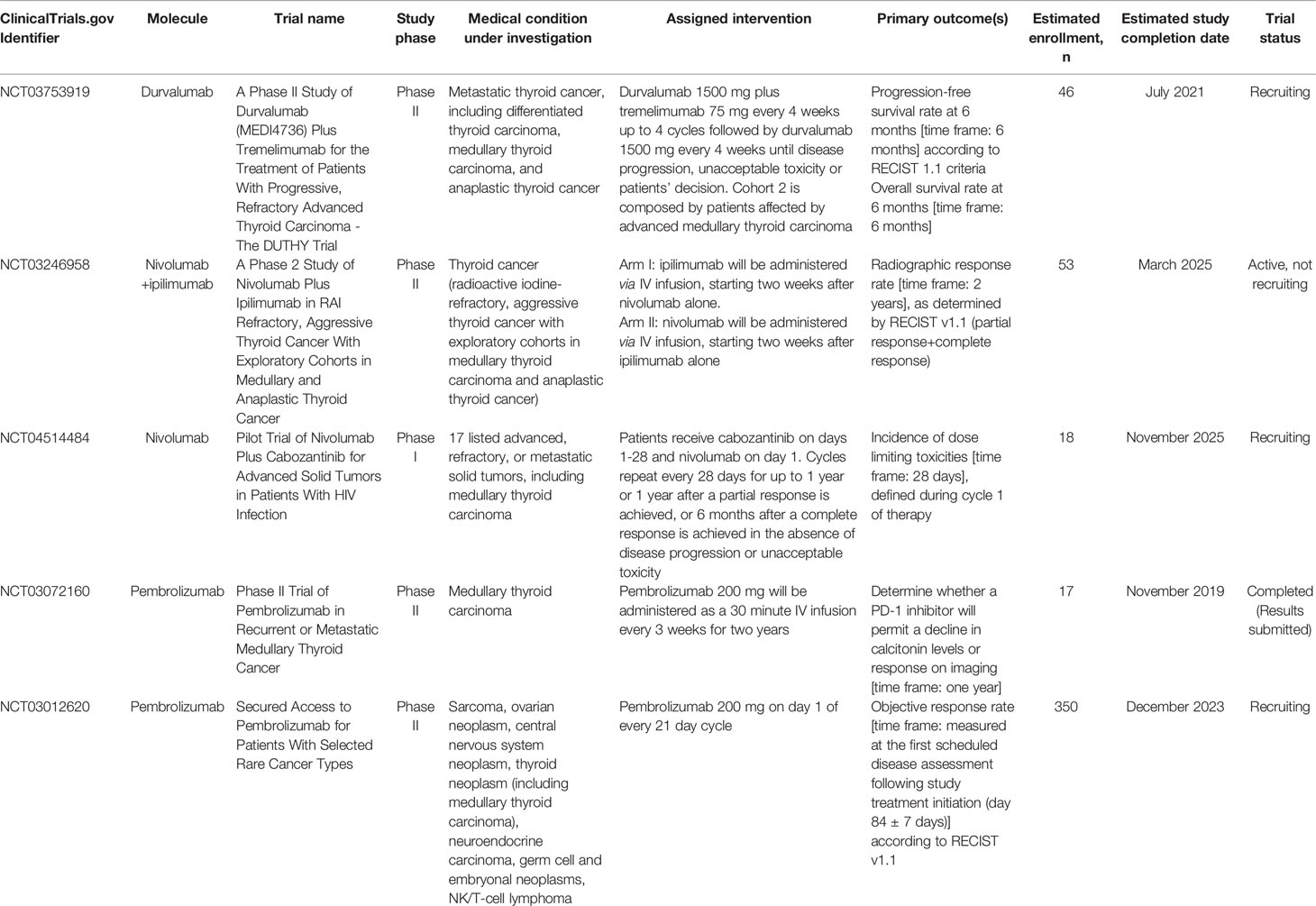
Table 2 Registered clinical trials evaluating FDA-approved immune checkpoint inhibitors in medullary thyroid carcinoma.
NCT03753919 (A Phase II Study of Durvalumab (MEDI4736) Plus Tremelimumab for the Treatment of Patients With Progressive, Refractory Advanced Thyroid Carcinoma - The DUTHY Trial) is a prospective, multi-center, open label, stratified, exploratory phase II study whose aim is to evaluate the following outcomes in patients affected by advanced thyroid cancer (estimated enrollment: 46 patients). Primary outcomes are PFS rate at 6 months and overall survival (OS) rate at 6 months; secondary outcomes comprise overall response rate (ORR), duration of response (DoR), median PFS, incidence of treatment-emergent AEs, median OS, and response status after start of study treatment. According to the primary histotype, patients are divided in three cohorts: i) advanced, radioiodine-refractory differentiated thyroid carcinoma (DTC), including papillary, follicular, Hürtle cell and poorly-differentiated thyroid carcinoma (Cohort 1); ii) advanced MTC (Cohort 2); iii) anaplastic thyroid cancer (ATC) (Cohort 3). Each cohort is planned to receive durvalumab plus tremelimumab (anti-CTLA-4 antibody, not yet approved by FDA) every 4 weeks up to 4 cycles followed by durvalumab alone every 4 weeks until progression, unacceptable toxicity or withdrawal. The study started in April 2019, with the estimated study completion date being July 2021. The present study status is “Recruiting”.
NCT03246958 (A Phase 2 Study of Nivolumab Plus Ipilimumab in RAI Refractory, Aggressive Thyroid Cancer With Exploratory Cohorts in Medullary and Anaplastic Thyroid Cancer) is a phase II clinical trial evaluating nivolumab in combination with ipilimumab, as a possible treatment for thyroid cancer, focusing on effectiveness (estimated enrollment: 53 patients). The primary endpoint is radiographic response rate as determined by RECIST v1.1 (i.e. partial response plus complete response), whereas secondary outcomes are PFS, OS, and tolerability at two years. This trial is designed to recruit patients with metastatic, progressive, RAI refractory DTC with exploratory cohorts in ATC (7 patients), and incurable, progressive MTC with prior TKI failure (10 patients). Participants aged ≥18 years are divided in two experimental arms: the first arm will be administered nivolumab alone for two weeks followed by nivolumab plus ipilimumab, whereas the second arm ipilimumab alone for two weeks followed by nivolumab/ipilimumab combination therapy. The study started in September 2017. The estimated study completion date is set for March 2025. The present study status is classified as “Active, not recruiting”. As above reported, very preliminary results of this trial have been recently published recently (26).
NCT04514484 (Pilot Trial of Nivolumab Plus Cabozantinib for Advanced Solid Tumors in Patients With HIV Infection) is a phase I trial that aims at defining in HIV-positive patients with advanced/metastatic solid cancer (estimated enrollment: 18 patients) the incidence of dose limiting toxicities during cycle 1 of therapy with cabozantinib and nivolumab (primary outcome). Secondary outcomes include the assessment of immune status (CD4 and CD8 cell counts) at each time point from baseline, HIV viral loads, changes in serum markers of immune activation, in immune checkpoint markers, in angiogenesis markers, and in infiltrating immune cell markers. According to the protocol, patients ≥18 years old receive cabozantinib on days 1-28 and nivolumab on day 1. Cycles repeat every 28 days for up to 1 year or 1 year after a partial response is achieved, or 6 months after a complete response is achieved in the absence of disease progression or unacceptable toxicity. The study started in November 2020. The estimated study completion date is November 2025. The present study status is “recruiting”.
NCT03072160 (Phase II Trial of Pembrolizumab in Recurrent or Metastatic Medullary Thyroid Cancer) is a phase II, open label, single center clinical trial aimed to determine, in patients having or not having undergone previous vaccine therapy (estimated enrollment: 15 patients in each cohort), whether a PD-1 inhibitor may allow for a decline in calcitonin levels or radiographic response (primary outcome); secondary outcomes include impact of previous therapeutic cancer vaccine on response rates, evaluation of immune responses in each cohort, changes in CEA and calcitonin kinetics, PFS, OS and safety. All patients will receive pembrolizumab 200 mg every 3 weeks. The study started in June 2017 and was completed in November 2019, and indeed the present study status is “completed”. On 11 February 2021, very preliminary results appeared in the Study Results section of the ClinicalTrials.gov registry. Thirteen patients were enrolled in the cancer vaccine arm (2/13 patients completed the trial), and 4 patients were enrolled in the control arm (none completed the trial). Disease progression was observed in 1/13 patients of the first arm, and in 1/4 patients of the second arm.
NCT03012620 (Secured Access to Pembrolizumab for Patients With Selected Rare Cancer Types) is a phase II, 2, non-randomized, open-label, multicenter study which aims to investigate the efficacy and safety of pembrolizumab in 7 different cohorts of patients with unresectable/locally advanced/metastatic rare cancers for which no other treatment options are available (estimated enrollment: 350 patients). Primary outcome is ORR, whereas secondary outcomes comprise PFS, OS, DoR, time to response, frequency and severity of AEs, and ORR/PFS/OS in subgroups of subjects with high versus low expression of PD-L1, CD4, FOX3 and other immune markers. According to the protocol, cohort 4 features rare thyroid cancer patients of ≥18 years, including MTC; these patients, same as for all other cohorts, are planned to receive pembrolizumab 200 mg on day 1 of every 21-day cycle. The study started in July 2017 and its estimated completion date is December 2023. The present study status is “recruiting”.
Discussion
Our review shows, despite very limited published evidence, an increasing attention to the possibility of treating MTC with ICIs, and indeed we found 5 ongoing RCTs with FDA-approved drugs that collectively involve nearly 500 patients with solid tumors, including MTC.
As an additional sign of interest, two trials investigating camrelizumab, a novel PD-1 inhibitor recently approved in China for the treatment of relapsed/refractory classical Hodgkin lymphoma (27, 28), are also intended to recruit patients with MTC, i.e. the NCT04612894 (The Efficacy and Safety of Anti-PD-1 Antibody Camrelizumab Combined With Apatinib for Neoadjuvant Therapy in Locally Advanced Thyroid Cancer: a Phase II Study), and NCT04521348 (A Phase II Study to Explore the Safety and Efficacy of Multiple Target Kinase Inhibitor (mTKI) Combined With Anti-Programmed Death-1(PD-1) Antibody in the Treatment of Advanced Thyroid Cancer) trials.
Notably, PD-1 and CTLA-4 have non-redundant immunosuppressive effects, paving the way for the development of clinical protocols with antibodies targeting the two pathways (29). Combination therapy with anti-PD-1 and anti-CTLA-4 drugs (durvalumab plus tremelimumab, NCT03753919 trial; nivolumab plus ipilimumab, NCT03246958 trial) is giving rise to great expectations in MTC. Overall, there is reliable evidence supporting a greater efficacy of the combined PD-1/CTLA-4 blockade over the two monotherapies in reversing tumor immune inhibition (30–32).
A number of different new scenarios could be opened by combinations or sequential schemes with other anti-tumor treatment modalities.
Systemic chemotherapy has been proposed to exert synergistic effects when combined with PD-1/PD-L1 blocking drugs in non-small-cell lung carcinoma (33). Indeed, chemotherapeutic agents may affect antitumor immunity both indirectly stimulating the immune system through immunogenic death of tumor cells, and directly regulating immune cell subsets, thereby reducing immunosuppression in the tumor microenvironment (TME) (34).
Second- or third-line treatment with ICIs has become increasingly common for patients with advanced disease who have already received other types of anticancer therapies (35).
It has been hypothesized that previous administration of cancer vaccines can drive immune cells to the TME and upregulate PD-L1 expression in the tumor cells due to cytokine release in the TME, thus giving a chance for anti-PD-L1/PD-1 drugs in patients who may not have otherwise benefited from such immunotherapies (36, 37). Interestingly, in the above-mentioned case report by Del Rivero et al. (23), the 61-year-old male showing >40% decrease in calcitonin level while on avelumab had previously undergone a 3-month trial with the GI-6207 cancer vaccine. Although a subsequent analysis of a patient’s lymph node (resected post-vaccination) revealed that the tumor was PD-L1 positive, no information about PD-L1 status before vaccination is available. As a further complication in this case’s assessment, the patient had been previously treated with the TKI sunitinib, which is acknowledged to deplete Tregs, and may have affected PD-L1 status as well (38).
The therapeutic potential of FDA-approved atezolizumab, avelumab, ipilimumab and pembrolizumab has also been investigated in NENs other than MTC (39, 40), thereby confirming a strong interest for ICI therapy in this subset of tumors.
Conclusion
Despite the lack of evidence regarding the use of ICIs in MTC, it should be considered that all the aforementioned RCTs saw first light in the last three years, thus indicating a growing interest of researchers in this field. Results coming from these trials, and hopefully from additional ones in the next future, will help clarify whether these drugs may represent a new weapon in favor of patients with MTC, and determine their position in the treatment algorithm.
Author Contributions
SDM, AD, GF, TFl, and TFe were responsible for the design, the methodology, the draft preparation, the reviewing and editing. AC and AF were responsible for the supervision. All authors contributed to the article and approved the submitted version.
Funding
This work was supported by the Italian Ministry of Education, University and Research (MIUR): PRIN 2017Z3N3YC.
Conflict of Interest
The authors declare that the research was conducted in the absence of any commercial or financial relationships that could be construed as a potential conflict of interest.
Acknowledgments
This review is part of the ‘Neuroendocrine Tumors Innovation Knowledge and Education’ project led by AC and AF, which aims at increasing the knowledge on NET. We would like to acknowledge all the Collaborators of the “NIKE” project: Manuela Albertelli—Genova; Barbara Altieri—Wurzburg; Luigi Barrea—Napoli; Filomena Bottiglieri—Napoli; Severo Campione—Napoli; Federica de Cicco—Napoli; Alessandra Dicitore—Milano; Diego Ferone—Genova; Francesco Ferraù—Messina; Erika Grossrubatscher—Milano; Marco Gallo—Torino; Elisa Giannetta—Roma; Federica Grillo—Genova; Elia Guadagno—Napoli; Valentina Guarnotta—Palermo; Andrea M. Isidori—Roma; Andrea Lania—Milano; Andrea Lenzi—Roma; Fabio Lo Calzo—Avellino; Pasquale Malandrino—Catania; Erika Messina—Messina; Roberta Modica—Napoli; Giovanna Muscogiuri—Napoli; Genoveffa Pizza—Avellino; Luca Pes—Sassari; Riccardo Pofi—Roma; Giulia Puliani—Roma; Carmen Rainone—Napoli; Paola Razzore—Torino; Laura Rizza—Roma; Manila Rubino —Milano; Rosa Maria Ruggieri—Messina; Emilia Sbardella—Roma; Franz Sesti—Roma; Mary Anna Venneri—Roma; Giovanni Vitale—Milano; Maria Chiara Zatelli—Ferrara.
Abbreviations
AEs, adverse events; APCs, antigen-presenting cells; ATC, anaplastic thyroid cancer; DTC, differentiated thyroid carcinoma; CTLA-4, cytotoxic T-lymphocyte-associated protein 4; DoR, duration of response; FDA, Food and Drug Administration; ICIs, immune checkpoints inhibitors; KO, knock-out; MTC, medullary thyroid carcinoma; NEN, neuroendocrine neoplasm; ORR, overall response rate; OS, overall survival; PD-1, programmed cell death protein-1; PD-L1, programmed death-ligand-1; PD-L2, programmed death-ligand-2; PFS, progression-free survival; RCTs, registered clinical trials; RET, rearranged during transfection; TME, tumor microenvironment; TCR, T cell receptor; TKIs, tyrosine kinase inhibitors; Tregs, regulatory T cells.
References
1. Ceolin L, Duval ,MAS, Benini AF, Ferreira CV, Maia AL. Medullary Thyroid Carcinoma beyond Surgery: Advances, Challenges, and Perspectives. Endocr Relat Cancer (2019) 26(9):R499–518. doi: 10.1530/ERC-18-0574
2. Viola D, Elisei R. Management of Medullary Thyroid Cancer. Endocrinol Metab Clin North Am (2019) 48(1):285–301. doi: 10.1016/j.ecl.2018.11.006
3. Wells SA, Robinson BG, Gagel RF, Dralle H, Fagin JA, Santoro M, et al. Vandetanib in Patients with Locally Advanced or Metastatic Medullary Thyroid Cancer: A Randomized, Double-Blind Phase III Trial. J Clin Oncol (2012) 30(2):134–41. doi: 10.1200/JCO.2011.35.5040
4. Elisei R, Schlumberger MJ, Müller SP, Schöffski P, Brose MS, Shah MH, et al. Cabozantinib in Progressive Medullary Thyroid Cancer. J Clin Oncol (2013) 31(29):3639–46. doi: 10.1200/JCO.2012.48.4659
5. U.S. Food and Drug Administration (FDA). Retevmo®. Highlights of Prescribing Information. Available at: https://www.accessdata.fda.gov/drugsatfda_docs/label/2020/213246s000lbl.pdf (Accessed on 13 March 2021).
6. U.S. Food and Drug Administration (FDA). FDA approves pralsetinib for RET-altered thyroid cancers. Available at: https://www.fda.gov/drugs/drug-approvals-and-databases/fda-approves-pralsetinib-ret-altered-thyroid-cancers (Accessed on 13 March 2021).
7. Grossrubatscher E, Fanciulli G, Pes L, Sesti F, Dolci C, de Cicco F, et al. Advances in the Management of Medullary Thyroid Carcinoma: Focus on Peptide Receptor Radionuclide Therapy. J Clin Med (2020) 9(11). doi: 10.3390/jcm9113507
8. Agrawal A, Rangarajan V, Shah S, Puranik A, Purandare N. MIBG (Metaiodobenzylguanidine) Theranostics in Pediatric and Adult Malignancies. Br J Radiol (2018) 91(1091):20180103. doi: 10.1259/bjr.20180103
9. Wilky BA. Immune Checkpoint Inhibitors: The Linchpins of Modern Immunotherapy. Immunol Rev (2019) 290(1):6–23. doi: 10.1111/imr.12766
10. Pardoll DM. The Blockade of Immune Checkpoints in Cancer Immunotherapy. Nat Rev Cancer (2012) 12(4):252–64. doi: 10.1038/nrc3239
11. Iida T, Ohno H, Nakaseko C, Sakuma M, Takeda-Ezaki M, Arase H, et al. Regulation of Cell Surface Expression of CTLA-4 by Secretion of CTLA-4-Containing Lysosomes upon Activation of CD4+ T Cells. J Immunol (2000) 165(9):5062–8. doi: 10.4049/jimmunol.165.9.5062
12. Teft WA, Kirchhof MG, Madrenas JA. Molecular Perspective of CTLA-4 Function. Annu Rev Immunol (2006) 24:65–97. doi: 10.1146/annurev.immunol.24.021605.090535
13. Tivol EA, Borriello F, Schweitzer AN, Lynch WP, Bluestone JA, Sharpe AH. Loss of CTLA-4 Leads to Massive Lymphoproliferation and Fatal Multiorgan Tissue Destruction, Revealing a Critical Negative Regulatory Role of CTLA-4. Immunity (1995) 3(5):541–7. doi: 10.1016/1074-7613(95)90125-6
14. Yi M, Niu M, Xu L, Luo S, Wu K. Regulation of PD-L1 Expression in the Tumor Microenvironment. J Hematol Oncol (2021) 14(1):10. doi: 10.1186/s13045-020-01027-5
15. Baumeister SH, Freeman GJ, Dranoff G, Sharpe AH. Coinhibitory Pathways in Immunotherapy for Cancer. Annu Rev Immunol (2016) 34:539–73. doi: 10.1146/annurev-immunol-032414-112049
16. Nishimura H, Nose M, Hiai H, Minato N, Honjo T. Development of Lupus-like Autoimmune Diseases by Disruption of the PD-1 Gene Encoding an ITIM Motif-Carrying Immunoreceptor. Immunity (1999) 11(2):141–51. doi: 10.1016/s1074-7613(00)80089-8
17. Bongiovanni M, Rebecchini C, Saglietti C, Bulliard J-L, Marino L, de Leval L, et al. Very Low Expression of PD-L1 in Medullary Thyroid Carcinoma. Endocr Relat Cancer (2017) 24(6):L35–8. doi: 10.1530/ERC-17-0104
18. Bi Y, Ren X, Bai X, Meng Y, Luo Y, Cao J, et al. PD-1/PD-L1 Expressions in Medullary Thyroid Carcinoma: Clinicopathologic and Prognostic Analysis of Chinese Population. Eur J Surg Oncol (2019) 45(3):353–8. doi: 10.1016/j.ejso.2018.10.060
19. Shi X, Yu P-C, Lei B-W, Li C-W, Zhang Y, Tan L-C, et al. Association Between Programmed Death-Ligand 1 Expression and Clinicopathological Characteristics, Structural Recurrence, and Biochemical Recurrence/Persistent Disease in Medullary Thyroid Carcinoma. Thyroid (2019) 29(9):1269–78. doi: 10.1089/thy.2019.0079
20. Ingenwerth M, Goetz M, Schmid KW, Theurer S. The Mismatch Repair System Is Not Affected in Medullary Thyroid Carcinoma Independent of Stromal Desmoplasia or Ret Proto-Oncogene Mutation. Ann Diagn Pathol (2020) 44:151445. doi: 10.1016/j.anndiagpath.2019.151445
21. Shi X, Li C-W, Tan L-C, Wen S-S, Liao T, Zhang Y, et al. Immune Co-Inhibitory Receptors PD-1, CTLA-4, TIM-3, LAG-3, and TIGIT in Medullary Thyroid Cancers: A Large Cohort Study. J Clin Endocrinol Metab (2021) 106(1):120–32. doi: 10.1210/clinem/dgaa701
22. Bai Y, Niu D, Yao Q, Lin D, Kakudo K. Updates in the Advances of Sporadic Medullary Thyroid Carcinoma: From the Molecules to the Clinic. Gland Surg (2020) 9(5):1847–56. doi: 10.21037/gs-2019-catp-21
23. Del Rivero J, Donahue RN, Marté JL, Gramza AW, Bilusic M, Rauckhorst M, et al. Madan RA. A Case Report of Sequential Use of a Yeast-CEA Therapeutic Cancer Vaccine and Anti-PD-L1 Inhibitor in Metastatic Medullary Thyroid Cancer. Front Endocrinol (Lausanne) (2020) 11:490. doi: 10.3389/fendo.2020.00490
24. Madan RA, Singh NK, Gramza AW, Fojo AT, Heery CR, Kim JW, et al. Phase II Study of a Yeast-Based Therapeutic Cancer Vaccine, GI-6207, Targeting CEA in Patients with Minimally Symptomatic, Metastatic Medullary Thyroid Cancer. JCO (2013) 31(15_suppl):TPS3127–TPS3127. doi: 10.1200/jco.2013.31.15_suppl.tps3127
25. Eisenhauer EA, Therasse P, Bogaerts J, Schwartz LH, Sargent D, Ford R, et al. New Response Evaluation Criteria in Solid Tumours: Revised RECIST Guideline (Version 1.1). Eur J Cancer (2009) 45(2):228–47. doi: 10.1016/j.ejca.2008.10.026
26. Lorch JH, Barletta JA, Nehs M, Uppaluri R, Alexander EK, Haddad RI, et al. Phase II Study of Nivolumab (N) plus Ipilimumab (I) in Radioidine Refractory Differentiated Thyroid Cancer (RAIR DTC) with Exploratory Cohorts in Anaplastic (ATC) and Medullary Thyroid Cancer (MTC). JCO (2020) 38(15_suppl):6513–3. doi: 10.1200/JCO.2020.38.15_suppl.6513
27. Markham A, Keam SJ. Correction to: Camrelizumab: First Global Approval. Drugs (2019) 79(13):1497. doi: 10.1007/s40265-019-01191-0
28. Nie J, Wang C, Liu Y, Yang Q, Mei Q, Dong L, et al. Addition of Low-Dose Decitabine to Anti–PD-1 Antibody Camrelizumab in Relapsed/Refractory Classical Hodgkin Lymphoma. JCO (2019) 37(17):1479–89. doi: 10.1200/JCO.18.02151
29. Schmidt C. The Benefits of Immunotherapy Combinations. Nature (2017) 552(7685):S67–9. doi: 10.1038/d41586-017-08702-7
30. Curran MA, Montalvo W, Yagita H, Allison JP. PD-1 and CTLA-4 Combination Blockade Expands Infiltrating T Cells and Reduces Regulatory T and Myeloid Cells within B16 Melanoma Tumors. Proc Natl Acad Sci USA (2010) 107(9):4275–80. doi: 10.1073/pnas.0915174107
31. Beavis PA, Henderson MA, Giuffrida L, Davenport AJ, Petley EV, House IG, et al. Dual PD-1 and CTLA-4 Checkpoint Blockade Promotes Antitumor Immune Responses through CD4+Foxp3- Cell-Mediated Modulation of CD103+ Dendritic Cells. Cancer Immunol Res (2018) 6(9):1069–81. doi: 10.1158/2326-6066.CIR-18-0291
32. Taggart D, Andreou T, Scott KJ, Williams J, Rippaus N, Brownlie RJ, et al. Anti-PD-1/Anti-CTLA-4 Efficacy in Melanoma Brain Metastases Depends on Extracranial Disease and Augmentation of CD8+ T Cell Trafficking. Proc Natl Acad Sci USA (2018) 115(7):E1540–9. doi: 10.1073/pnas.1714089115
33. Gadgeel SM, Stevenson J, Langer CJ, Gandhi L, Borghaei H, Patnaik A, et al. Pembrolizumab (Pembro) plus Chemotherapy as Front-Line Therapy for Advanced NSCLC: KEYNOTE-021 Cohorts A-C. JCO (2016) 34(15_suppl):9016–6. doi: 10.1200/JCO.2016.34.15_suppl.9016
34. Mathew M, Enzler T, Shu CA, Rizvi NA. Combining Chemotherapy with PD-1 Blockade in NSCLC. Pharmacol Ther (2018) 186:130–7. doi: 10.1016/j.pharmthera.2018.01.003
35. Evans S, Martini D, Magod B, Olsen T, Brown J, Yantorni L, et al. 255 Efficacy of Sequential Immune Checkpoint Inhibition (ICI) in Patients with Genitourinary Malignancies. J Immunother Cancer (2020) 8(Suppl 3). doi: 10.1136/jitc-2020-SITC2020.0255
36. Fu J, Malm I-J, Kadayakkara DK, Levitsky H, Pardoll D, Kim YJ. Preclinical Evidence That PD1 Blockade Cooperates with Cancer Vaccine TEGVAX to Elicit Regression of Established Tumors. Cancer Res (2014) 74(15):4042–52. doi: 10.1158/0008-5472.CAN-13-2685
37. Ribas A, Robert C, Hodi FS, Wolchok JD, Joshua AM, Hwu W-J, et al. Association of Response to Programmed Death Receptor 1 (PD-1) Blockade with Pembrolizumab (MK-3475) with an Interferon-Inflammatory Immune Gene Signature. JCO (2015) 33(15_suppl):3001–1. doi: 10.1200/jco.2015.33.15_suppl.3001
38. Farsaci B, Higgins JP, Hodge JW. Consequence of Dose Scheduling of Sunitinib on Host Immune Response Elements and Vaccine Combination Therapy. Int J Cancer (2012) 130(8):1948–59. doi: 10.1002/ijc.26219
39. Fanciulli G, Di Molfetta S, Dotto A, Florio T, Feola T, Rubino M, et al. Nike Group. Emerging Therapies in Pheochromocytoma and Paraganglioma: Immune Checkpoint Inhibitors in the Starting Blocks. J Clin Med (2020) 10(1). doi: 10.3390/jcm10010088
Keywords: medullary thyroid carcinoma, immune checkpoint inhibitors, avelumab, durvalumab, ipilimumab, nivolumab, pembrolizumab
Citation: Di Molfetta S, Dotto A, Fanciulli G, Florio T, Feola T, Colao A and Faggiano A (2021) Immune Checkpoint Inhibitors: New Weapons Against Medullary Thyroid Cancer? Front. Endocrinol. 12:667784. doi: 10.3389/fendo.2021.667784
Received: 14 February 2021; Accepted: 24 March 2021;
Published: 14 April 2021.
Edited by:
Michele Caraglia, University of Campania Luigi Vanvitelli, ItalyCopyright © 2021 Di Molfetta, Dotto, Fanciulli, Florio, Feola, Colao and Faggiano. This is an open-access article distributed under the terms of the Creative Commons Attribution License (CC BY). The use, distribution or reproduction in other forums is permitted, provided the original author(s) and the copyright owner(s) are credited and that the original publication in this journal is cited, in accordance with accepted academic practice. No use, distribution or reproduction is permitted which does not comply with these terms.
*Correspondence: Sergio Di Molfetta, s.dimolfetta@libero.it
†ORCID: Sergio Di Molfetta, orcid.org/0000-0003-3454-7330
Andrea Dotto, orcid.org/0000-0002-0140-7297
Giuseppe Fanciulli, orcid.org/0000-0002-8367-5649
Tullio Florio, orcid.org/0000-0002-2394-996X
Tiziana Feola, orcid.org/0000-0003-1066-0064
Annamaria Colao, orcid.org/0000-0001-6986-266X
Antongiulio Faggiano, orcid.org/0000-0002-9324-3946
‡These authors share first authorship