- 1Department of Traditional Chinese Medical Gynecology, Hangzhou Hospital of Traditional Chinese Medicine Affiliated to Zhejiang Chinese Medical University, Hangzhou, China
- 2Second Clinical Medical College, Guangzhou University of Chinese Medicine, Guangzhou, China
- 3Dongfang Hospital, Beijing University of Chinese Medicine, Beijing, China
Background: Diminished ovarian reserve (DOR) significantly increases the risk of female infertility and contributes to reproductive technology failure. Recently, the role of melatonin in improving ovarian reserve (OR) has attracted widespread attention. However, details on the pharmacological targets and mechanisms of melatonin-improved OR remain unclear.
Objective: A systems pharmacology strategy was proposed to elucidate the potential therapeutic mechanism of melatonin on DOR at the molecular, pathway, and network levels.
Methods: The systems pharmacological approach consisted of target identification, data integration, network construction, bioinformatics analysis, and molecular docking.
Results: From the molecular perspective, 26 potential therapeutic targets were identified. They participate in biological processes related to DOR development, such as reproductive structure development, epithelial cell proliferation, extrinsic apoptotic signaling pathway, PI3K signaling, among others. Eight hub targets (MAPK1, AKT1, EGFR, HRAS, SRC, ESR1, AR, and ALB) were identified. From the pathway level, 17 significant pathways, including the PI3K-Akt signaling pathway and the estrogen signaling pathway, were identified. In addition, the 17 signaling pathways interacted with the 26 potential therapeutic targets to form 4 functional modules. From the network point of view, by regulating five target subnetworks (aging, cell growth and death, development and regeneration, endocrine and immune systems), melatonin could exhibit anti-aging, anti-apoptosis, endocrine, and immune system regulation effects. The molecular docking results showed that melatonin bound well to all hub targets.
Conclusion: This study systematically and intuitively illustrated the possible pharmacological mechanisms of OR improvement by melatonin through anti-aging, anti-apoptosis, endocrine, and immune system regulation effects.
Introduction
Infertility affects a significant proportion of humanity and is regarded as a global public health issue by the World Health Organization (1, 2). Diminished ovarian reserve (DOR), defined as a reduction in both oocyte quality and quantity, is one of the most common causes of female infertility and poor ovarian response to controlled ovarian stimulation with a rapidly increasing occurrence rate (3, 4). In addition, women with DOR have exceedingly high rates of recurrent pregnancy loss and no euploid embryos (5–7). Devine et al. reported that the prevalence of DOR increased from 19 to 26% in the past few years, representing a major challenge in reproductive medicine (8, 9). Despite its prevalence, its pathology remains unclear. Aging is the most common cause of DOR. Other influential factors for DOR include genetic predisposition, autoimmune diseases, chemotherapy, and psychological stress (10–13).
The decline of ovarian reserve (OR) is a continuous, gradual process starting from the oocyte death of embryos at 20 weeks of gestation until menopause (14). The premature depletion of OR eventually results in premature ovarian failure, a more severe condition, which might lead to a loss of reproductive capacity, seriously affecting women's quality of life (15, 16). Thus, early and active interventions should be implemented in women with DOR before it is too late. However, DOR treatment remains a significant challenge in reproductive medicine, although various treatment strategies are currently being used (9). For example, DHEA, as an adjuvant therapy in in vitro fertilization (IVF), might increase the number of retrieved oocytes (17); however, the true benefit is under active debate as DHEA has some side effects, including acne, sleep problems, and headaches (18).
Melatonin (5-methoxy-N-acetyl tryptamine), a pineal gland hormone, plays a significant role in regulating the circadian sleep-wake cycle, reproductive physiology, and immune functions (19). As a dietary supplement, it has gained widespread popularity globally. Lerner and colleagues' discovery of melatonin in 1958 presented a new research avenue in reproductive physiology (20, 21). Since Wurtman et al. reported that preovulatory follicles contain substantial amounts of melatonin, which may affect ovarian steroidogenesis, many studies have focused on the role of melatonin in OR (21). Morioka et al. conducted the first clinical trial to evaluate melatonin as a drug for improving oocyte quality in women who could not become pregnant because of poor-quality oocytes (22). The results showed that melatonin treatment increased oocyte quality. Interestingly, the melatonin-treated group's intrafollicular melatonin concentration was four times higher than that of the control group, consistent with Morioka's study. Similarly, several subsequent studies have confirmed that melatonin supplementation can ameliorate intrafollicular oxidative balance, improve the quantity and quality of oocytes, and improve IVF outcomes in women with DOR and infertility (23–27). Some experts have suggested that melatonin levels in the follicular fluid may serve as a biomarker for predicting OR (28, 29). In addition, animal experiments have also confirmed that melatonin can protect the quality of oocytes and improve OR through multiple mechanisms (30–35).
Although anti-DOR activities exerted by melatonin have been reported, in-depth mechanistic preclinical studies are currently limited. Moreover, details of biomarkers and the biological pathways through which melatonin exerts its effects in improving OR are yet to be completely elucidated. In a previous study, a network pharmacology-based approach was successfully used to uncover the target proteins and potential therapeutic mechanisms of drugs (36–38). Accordingly, this study was performed to reveal the predictive targets and therapeutic mechanisms underlying melatonin action against DOR using a systematic network pharmacology-based approach. Figure 1 illustrates the workflow of the study.
Materials and Methods
Identification of Putative Melatonin Targets
The PubChem database (https://pubchem.ncbi.nlm.nih.gov/) was used to obtain simplified molecular-input line-entry specification (SMILES) information and the 3D structure of melatonin (39). Melatonin's 3D structure was uploaded to the PharmMapper Server (http://www.lilab-ecust.cn/pharmmapper/), and the SMILES for melatonin was uploaded to the SwissTargetPrediction database (http://www.swisstargetprediction.ch/) to predict the potential melatonin targets (40, 41). DrugBank (https://go.drugbank.com/), SuperTarget (http://insilico.charite.de/supertarget/index.php), and TCMSP (https://tcmspw.com/tcmsp.php) databases were used to identify known melatonin targets (42, 43). All retrieved target names were corrected to official symbols using the UniProt database(https://www.uniprot.org/).
Selection of DOR-Associated Targets
DisGeNET (https://www.disgenet.org/), GeneCards (https://www.genecards.org/), and NCBI Gene databases (https://www.ncbi.nlm.nih.gov/gene/) were utilized to identify targets related to DOR (44). The keyword was "diminished ovarian reserve." To enhance the credibility of the results, DOR-related targets with a gene-disease score ≥0.1 were set in DisGeNET, and the threshold of relevance score was set at 10 in GeneCards.
Protein-Protein Interaction (PPI) Data
The protein-protein interaction data were integrated and obtained from the Search Tool for the Retrieval of Interacting Genes (STRING) platform (https://string-db.org/) (45). The species was limited to "Homo sapiens," and the interaction confidence score was set at 0.7, defined as high confidence on the STRING platform.
GO and KEGG Pathway Enrichment
To clarify the role of potential therapeutic targets in gene function and signaling pathways, the ClusterProfiler package of R 4.0.2 was used to perform GO and KEGG pathway enrichment analysis of the common genes for melatonin and DOR (46). The pathway class of every KEGG pathway was obtained from the KEGG PATHWAY database (https://www.kegg.jp/kegg/pathway.html) for further analysis.
Network Construction
Five networks were constructed: (1) the melatonin-putative target network was built by connecting melatonin and its targets; (2) the PPI network of DOR targets; (3) another PPI network was constructed using the intersected melatonin and DOR genes; (4) the melatonin-targets-pathways network was established by linking melatonin, its targets, and key pathways with literature support for DOR treatment. For further analysis, the network was divided into functional modules using the Community Cluster algorithm (Glay) of clustermaker2 (47) and (5) the sub-networks of the potential therapeutic targets that were enriched in different key pathway classes were constructed. All of the above networks were established using Cytoscape 3.8.0.
Molecular Docking Simulation
Target Protein Preparation
The crystal structures of the protein receptors were obtained from the RCSB Protein Data Bank (http://www.rcsb.org/). The downloaded protein structures were pretreated with PyMol 2.4.0 to remove the original ligand, solvent molecules, redundant protein chains and add polar hydrogen. Then, AutoDock Tools 1.5.6 was used to compute the Gasteiger and determine the docking box's center and size (48).
Ligand Preparation
The 3D structure of melatonin was treated by polarity hydrogenation and energy minimization using the MMFF94s force field.
Molecular Docking
AutoDock Vina was then used to evaluate melatonin binding and the hub targets by molecular docking (49). Prior to molecular docking, all protein and melatonin structures were converted to PDBQT format using AutoDock Tools 1.5.6. Melatonin was then docked onto the proteins using AutoDock Vina. Finally, the binding affinity calculated by AutoDock Vina was tallied, and the docking result was visualized using PyMol 2.4.0 software (Open-source version).
Results
Melatonin−Putative Target Network
A total of 206 melatonin targets were obtained after removing duplications from the PharmMapper, SuperTarget, DrugBank, SwissTargetPrediction, and TCMSP databases (Supplementary 1). Then, the melatonin-target network was constructed using Cytoscape 3.8.0 (Figure 2). As shown in Figure 2, there were 28 known targets, accounting for 13.6% of the total targets and 183 putative targets, accounting for 88.8% of the total targets. Furthermore, there were five intersecting targets between the potential and known targets.
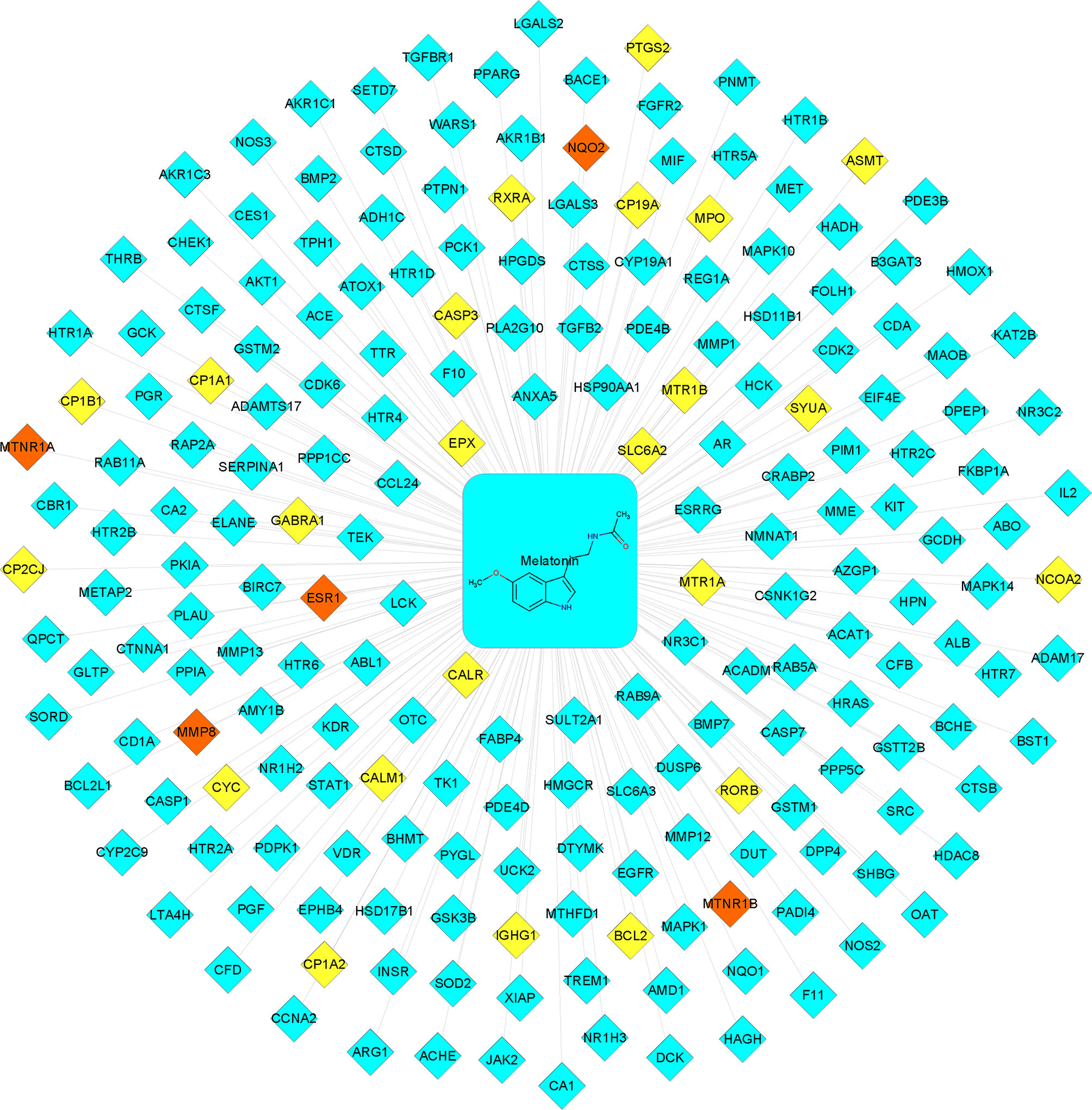
Figure 2 Melatonin−putative target network. The blue-colored nodes represent the potential targets. The yellow-colored nodes represent the known targets. The red-colored nodes represent the intersection of the potential and known targets.
PPI Network of DOR Targets
A total of 326 DOR-related targets were obtained from the DisGeNET, GeneCards, and NCBI Gene databases (Supplementary 2). A PPI network was constructed to demonstrate the interaction of DOR-related targets (Figure 3). Forty-five significant DOR-related targets were obtained according to the mean values for degree centrality (DC), betweenness centrality (BC), and closeness centrality (CC), which were 47.14553991, 0.004491643, and 0.527211414, respectively (Supplementary 3).
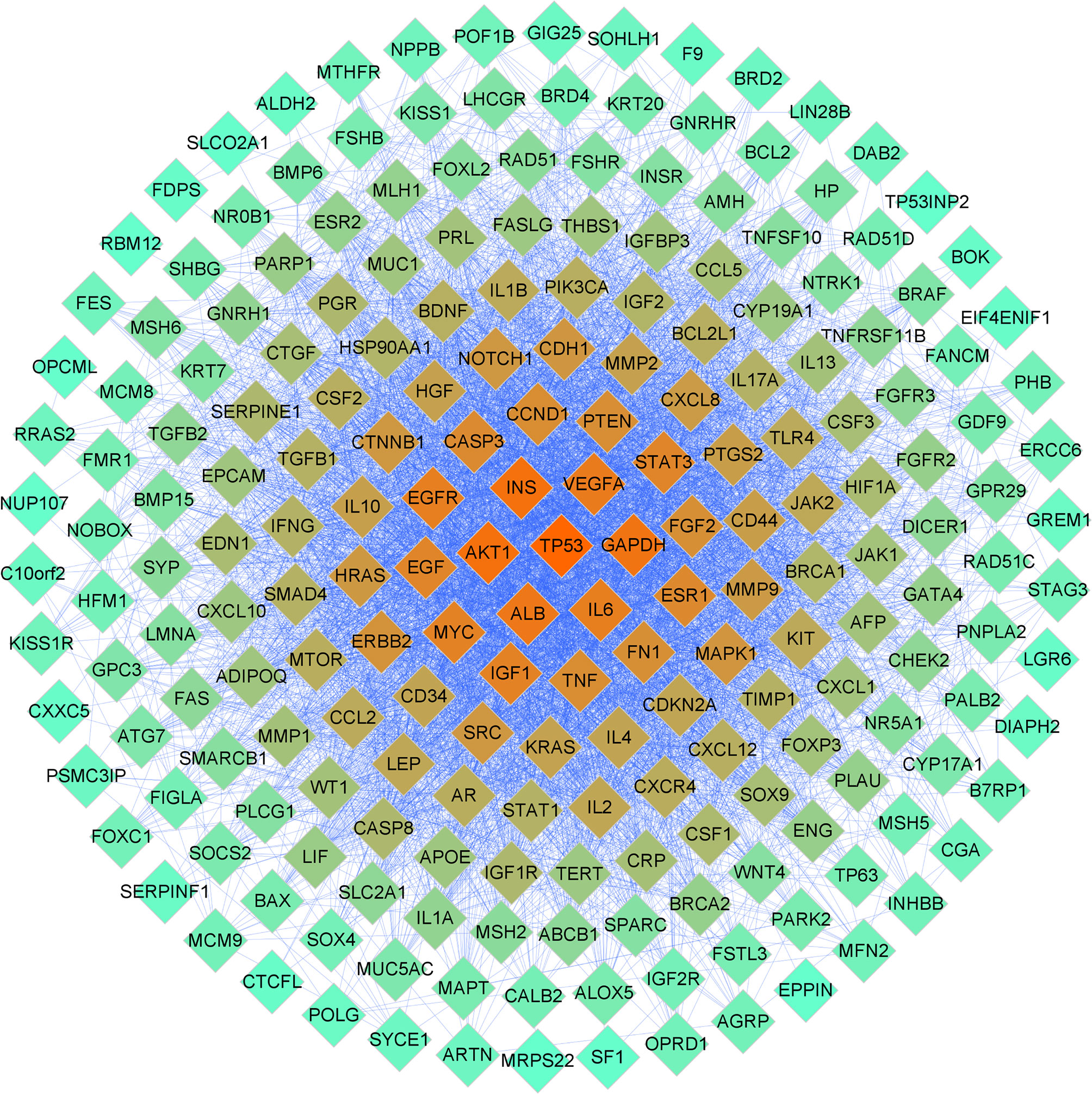
Figure 3 PPI network related to DOR. The color of the nodes is illustrated from red to cyan in descending order of degree values.
PPI Network of the Potential Therapeutic Targets
Based on the above results, 26 common melatonin and DOR targets (potential therapeutic targets) were obtained using the Venn Diagram tool (http://bioinformatics.psb.ugent.be/webtools/Venn/) (Figure 4A and Supplementary 4). Then, the PPI network of these 26 common targets was constructed (Figure 4B). To find the hub targets in this complex biological network, the topological parameters were analyzed. As a result, there are eight hub targets in this PPI network according to DC, BC, and CC mean values, including MAPK1, AKT1, EGFR, HRAS, SRC, ESR1, AR, and ALB (Supplementary 5). Meanwhile, as shown in Table 1, all eight hub targets were significant DOR-related targets. Therefore, these hub targets might play an essential role in OR improvement via melatonin and were used for the subsequent molecular docking study.
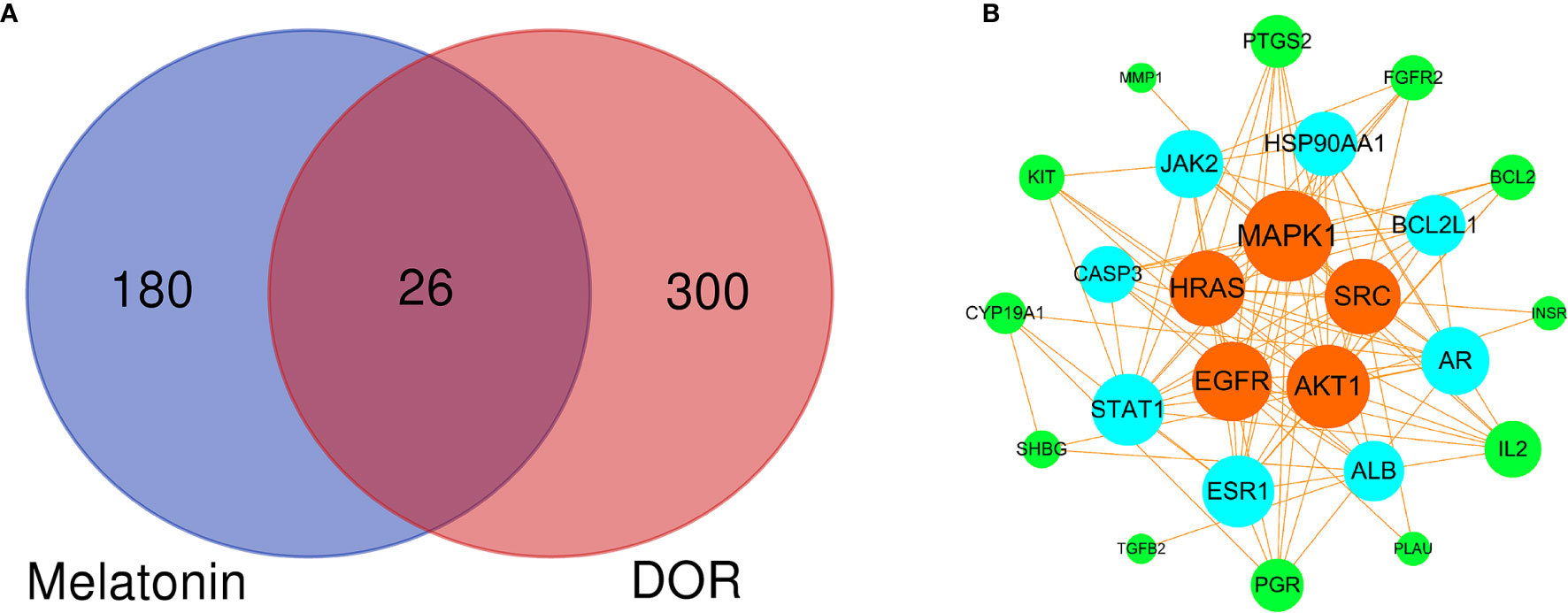
Figure 4 Venn diagram and PPI network of potential therapeutic targets. (A) Venn diagram of intersected targets of melatonin and DOR. (B) PPI network of potential therapeutic targets. The node sizes and colors are illustrated from large to small and orange to green in descending order of degree values.
GO and KEGG Enrichment Analysis
GO Enrichment Analysis
The 26 potential therapeutic targets were analyzed using the ClusterProfiler package of R 4.0.2. The top 10 terms of each part of the GO enrichment results were selected based on the counts of hit genes and the p-value. The results were visualized using the R package's ggplot2 and are shown in Figure 5A. After data screening, the top five enriched GO terms of biological processes (BP) are shown in Figure 5B. We could clearly identify that the top five enriched BPs of melatonin against DOR effects were mechanistically linked to reproductive structure development, epithelial cell proliferation, the extrinsic apoptotic signaling pathway, phosphatidylinositol 3-kinase signaling, and response to steroid hormones. Seven of the eight hub genes were also enriched in the top five enriched BPs, including MAPK1, AKT1, EGFR, SRC, HRAS, ESR1, and AR.
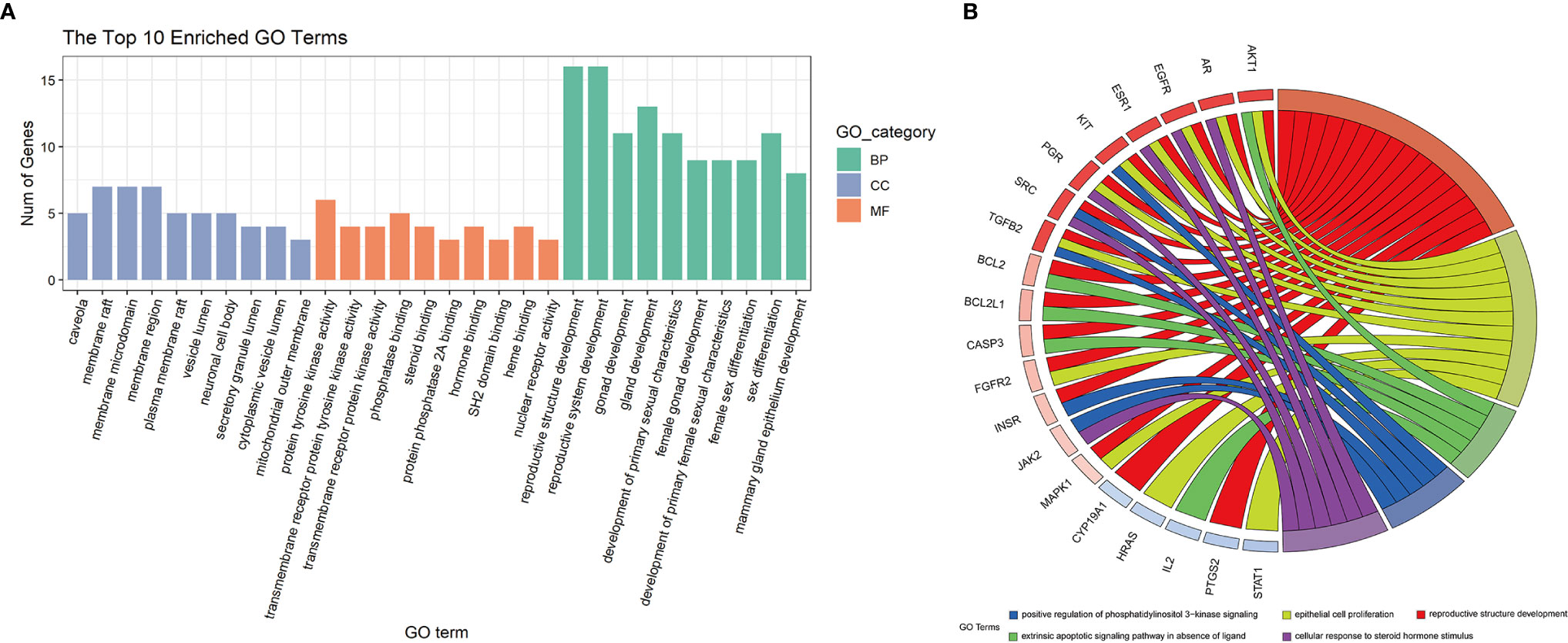
Figure 5 GO enrichment analysis and the top 5 enriched biological processes. (A) GO enrichment analysis. The top 10 significantly enriched terms of each part. BP, biological process; CC, cell component; MF, molecular function. (B) The top 5 enriched biological processes.
KEGG Enrichment Analysis of the Potential Therapeutic Targets
We carried out KEGG pathway enrichment analysis of the 26 therapeutic targets using the ClusterProfiler package of R 4.0.2 and obtained 123 pathways with a p-value <0.05. After data screening, 17 significant pathways were identified (Figure 6A and Table 1). Then, the genes enriched in each pathway were sorted, and a melatonin-target-pathway network was constructed (Figure 6B). In the network, the PI3K-Akt signaling pathway (hsa04151) and estrogen signaling pathway (hsa04915) were significantly enriched (Figure 7). To fully understand the mechanism of melatonin in treating DOR, the target-pathway network was decomposed into functional modules using the community cluster (Glay) algorithm of clustermaker2. As illustrated in Figure 6C, the target pathway network was divided into four modules. Module 1 contained six pathways, including the AGE-RAGE signaling pathway in diabetic complications (hsa04933), JAK-STAT signaling pathway (hsa04630), thyroid hormone signaling pathway (hsa04919), growth hormone synthesis, secretion, and action (hsa04935), progesterone-mediated oocyte maturation (hsa04914), and GnRH secretion (hsa04929). Module 2 consisted of four pathways, including the VEGF signaling pathway (hsa04370), apoptosis (hsa04210), IL-17 signaling pathway (hsa04657), and NF-κB signaling pathway (hsa04064). Module 3 comprised four pathways, including the PI3K-Akt signaling pathway (hsa04151), MAPK signaling pathway (hsa04010), FoxO signaling pathway(hsa04068), and ovarian steroidogenesis (hsa04913). Module 4 comprised three pathways, including the estrogen signaling pathway (hsa04915), GnRH signaling pathway (hsa04912), and progesterone-mediated oocyte maturation (hsa04914). In addition, the pathway class for each of the 123 pathways in the KEGG database was obtained, and the number of KEGG pathways classified in different biological systems is shown in Figure 8A. Meanwhile, according to the pathway class, five sub-networks were constructed to explain melatonin's multi-mechanism on DOR integrally.
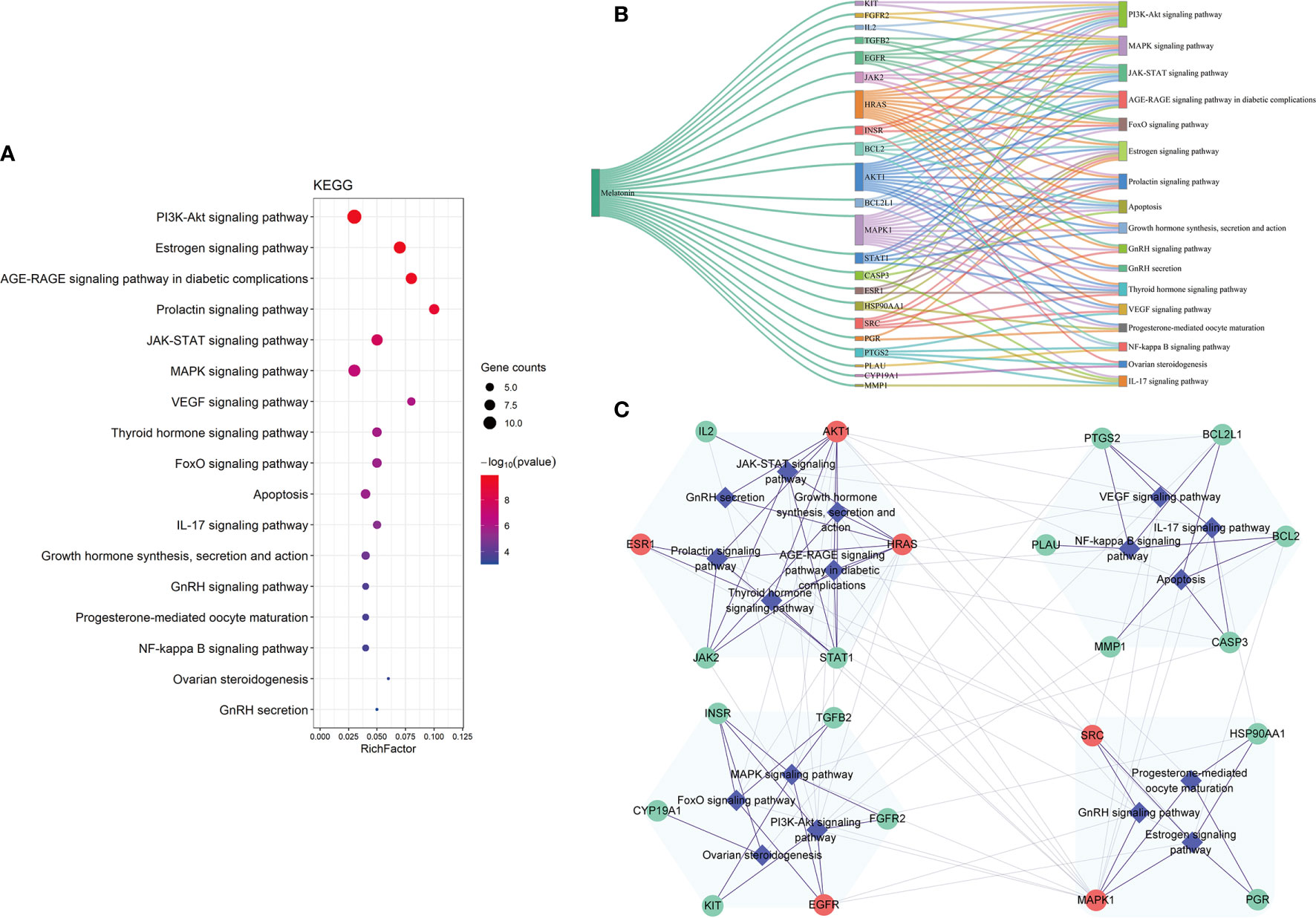
Figure 6 The KEGG pathway analysis of the 26 potential therapeutic targets. (A) The 17 significant pathways. The bubbles’ sizes are indicated from large to small in descending order of the count of the potential targets enriched in the pathways. The bubbles’ colors are indicated from red to blue in descending order of -lg (p-value). (B) Melatonin-targets-pathways network. The width of the line is proportional to the number of connected points. (C) Module analysis of the target-pathway network. The diamond nodes represent the pathways, and the circular nodes represent the targets. The red nodes represent the hub genes obtained from the PPI network of potential therapeutic targets.
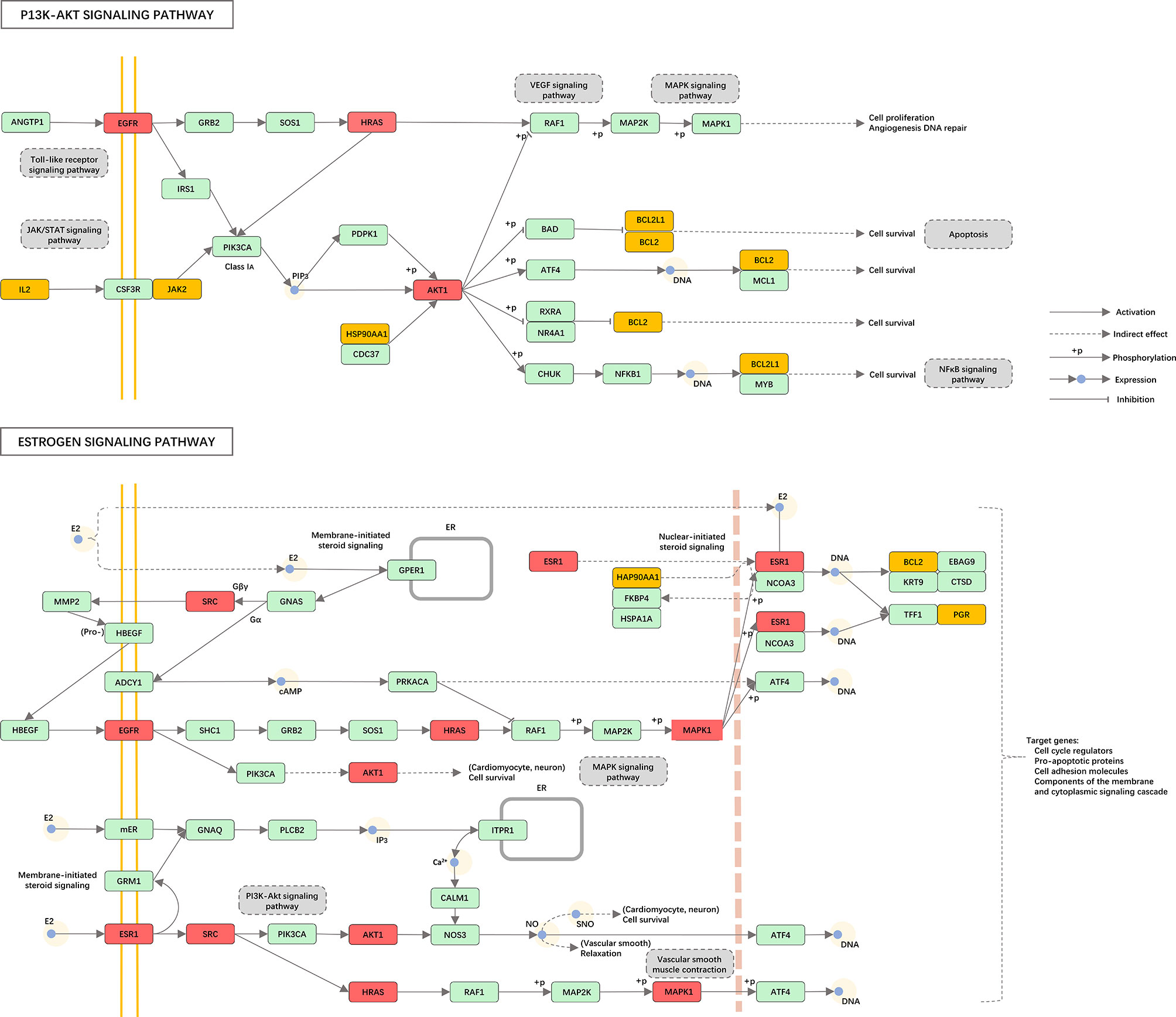
Figure 7 Distribution of the potential therapeutic targets on significantly enriched pathways. The red nodes represent key genes, the yellow nodes represent overlapping targets of Melatonin and DOR targets, and the green nodes represent the other targets in estrogen signaling pathway and PI3K-AKT signaling pathway.
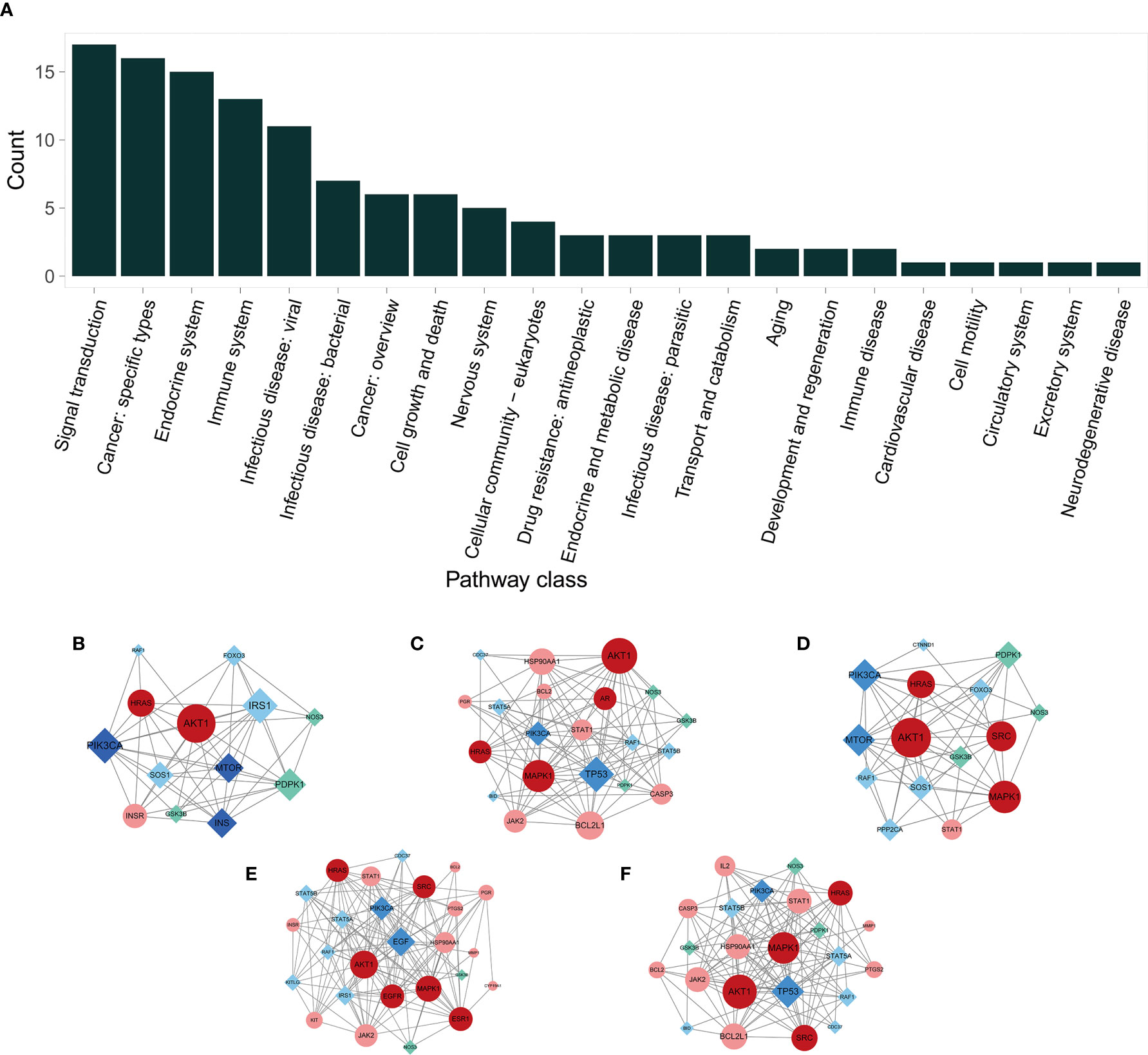
Figure 8 The KEGG pathway class analysis and sub-networks in different pathway classes. (A) The pathway class distribution. (B–F) Melatonin’s target sub-networks in different pathway classes. (B) Aging; (C) Cell growth and death; (D) Development and regeneration; (E) Endocrine system; and (F) Immune system. The circular nodes indicate the primary proteins, and the diamond nodes indicate secondary proteins. The pink nodes indicate the common targets of melatonin and DOR; the red nodes indicate the PPI network’s hub genes of potential therapeutic targets; the cyan nodes indicate the melatonin targets, and the deep blue nodes indicate the DOR targets.
Molecular Docking
Eight hub genes were selected for molecular docking analysis with melatonin. The active site parameters of each target were calculated and are listed in Table 2. The lower docking affinity reflects the stronger binding ability between melatonin and its targets, and the binding pose with the strongest affinity was selected to analyze the interaction between melatonin and its targets. As shown in Table 2, except for ALB, the affinity of the remaining targets and melatonin was lower than -5 kcal/mol, indicating a strong binding affinity. Therefore, melatonin may improve OR by regulating the activity of these proteins. Figure 9 shows the binding mode of melatonin with the hub targets. Taking Figure 9A as an example, melatonin completely entered the active site of AKT1 and formed hydrophobic interactions with residues T291(A) and V164(A). Moreover, the formation of three hydrogen bonds between melatonin and the active site residues of AKT1 involved residues E234 (A), L156 (A), and D292 (A).
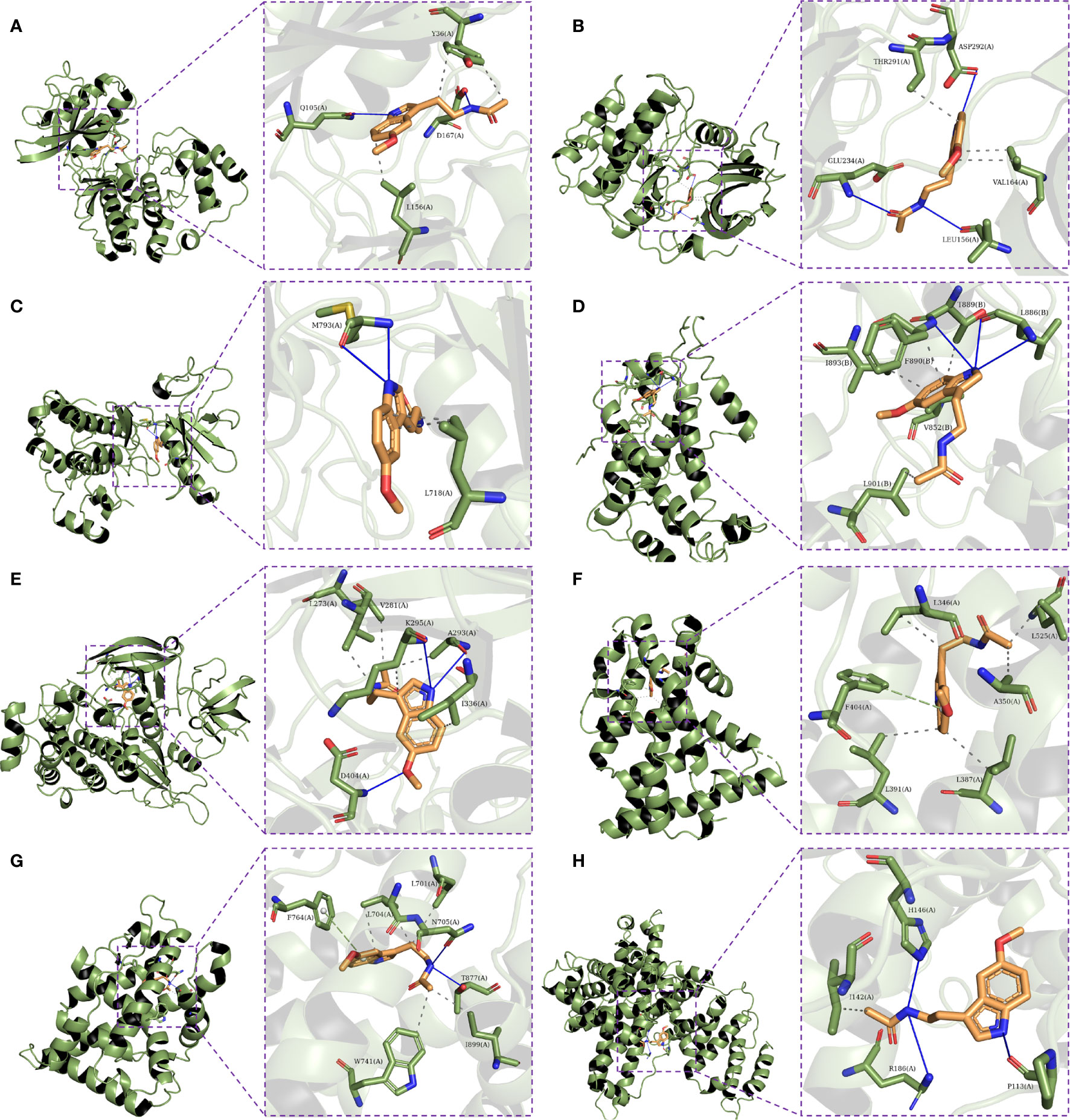
Figure 9 Molecular docking of the eight hub targets with Melatonin. (A) The binding poses of MAPK1 complexed with melatonin. (B) The binding poses of AKT1 complexed with melatonin. (C) The binding poses of EGFR complexed with melatonin. (D) The binding poses of HRAS complexed with melatonin. (E) The binding poses of SRC complexed with melatonin. (F) The binding poses of ESR1 complexed with melatonin. (G) The binding poses of AR complexed with melatonin. (H) The binding poses of ALB complexed with melatonin.
Discussion
Ovarian reserve plays a crucial role in reproductive potential and endocrine stability. Driven by societal trends, many young women choose to postpone marriage and childbirth. However, their OR sharply declines after the age of 35 years (7, 50). Besides leading to reproductive dysfunction, DOR has been associated with increased risk factors for cardiovascular disease and depression (51, 52). As mentioned previously, clinical findings have confirmed that melatonin effectively improves OR, but the therapeutic mechanism of action is still not fully understood. Therefore, in the present study, for the first time, systematic and comprehensive network pharmacology was utilized to reveal the mechanism of action of melatonin against DOR and to provide relevant information for further preclinical or clinical research. According to our network pharmacology results, AKT1, EGFR, MAPK1, HRAS, SRC, ESR1, AR, and ALB play vital roles in improving OR via melatonin. Interestingly, the molecular docking of the hub genes and melatonin exhibited high affinities, implying that the eight hub genes may be highly correlated in the treatment of DOR with melatonin.
Melatonin’s Eight Hub Targets in DOR
AKT1, which belongs to the AKT subfamily of serine/threonine kinases, is a multifunctional protein that regulates cell growth, survival, and proliferation (53). Emerging evidence has shown that melatonin can inhibit early follicle atresia and slow down the exhaustion of the ovarian follicle reserve by regulating the PI3K/AKT pathway in mice (54). Similarly, Leung et al. found that melatonin acts as a modulator of ovarian function and stimulates theca cell steroidogenesis by activating the PI3K/AKT pathway in bovine small follicles (55). Additionally, melatonin can ameliorate decreased embryo development caused by the AKT1 inhibitor SH6 during the in vitro maturation step by enhancing oocyte maturation, cumulus cell expansion, and protection from DNA fragmentation (56).
EGFR also plays an essential role in ovarian function (57–59). LH-induced EGFR activation is an essential component for the communication between the outer mural granulosa and theca cells and the inner cumulus cells and oocytes, leading to cumulus cell expansion and oocyte maturation (60). Interestingly, Tian et al. found that melatonin can upregulate the expression levels of EGFR and effectively improve the efficiency of oocyte maturation in vitro (61). Tian et al. further showed that melatonin enhances the expression of EGFR in cumulus cells and improves cumulus-oocyte complex maturation, mainly via melatonin receptor 1 (62). Moreover, several studies have shown that the activation of EGFR promotes several signaling pathways, including MAPK, PI3K/AKT, and JAK/STAT pathways, all of which play a crucial role in follicle recruitment, development, and maturation (63–66). These results suggest that melatonin enhances ovarian reserve variously by upregulating EGFR levels.
MAPK1, also known as extracellular signal-regulated kinase 2 (ERK2), is a downstream effector of the EGFR pathway. Activated ERK regulates the expression of LHβ and FSHβ, which are gonadotropin synthesis genes, and induces follicle growth and ovulation (67, 68). In addition, the activation of EGFR-ERK1/2 dependent gene transcription leads to the cascade of prostaglandin E2 and p38MAPK induction, which in turn stimulates the production of EGFR ligands (AREG, EREG, and BTC) in granulosa and cumulus cells, finally activating the entire EGF network (58). For the first time (2001), Leung et al. found that melatonin via the MAPK pathway regulates progesterone production, LH receptor, GnRH, and GnRH receptor gene expression in human granulosa-luteal cells, which play a direct role in regulating ovarian function (69). Furthermore, melatonin has been shown to enhance follicle growth and proliferation in cadmium-induced injury in rat ovaries via the ERK1/2 and mTOR pathways (70).
As the primary female sex hormones, estrogens are responsible for maturing and maintaining the female reproductive system and are also involved in gonadotropin secretion and ovarian follicle maturation. Estrogens exert their functions by binding to ERα and ERβ, encoded by ESR1 and ESR2, respectively. SRC and HRAS, the downstream proteins of ESR1 in the estrogen signaling pathway, participate in various cellular processes, including proliferation, differentiation, and adhesion (71). Many studies support the beneficial effects of androgens in follicular development, which may be related to AR upregulating FSH receptor expression, stimulating FSH activity in GCs, and promoting follicles from the anterior sinus phase to the anal phase (72–75). In addition, although the expression pattern and role of ALB in the ovaries have not been fully clarified, as a major serum protein, ALB plays a vital role in steroid hormone (SHs) carriers and acts as a regulator of SHs' access to their receptors (76, 77). At present, no animal or clinical studies have directly confirmed that melatonin can improve OR through the above five targets (ESR1, HRAS, SRC, AR, and ALB). However, based on the molecular docking results in the current study (as well as AKT1, MAPK1, and EGFR, the above five targets have a good binding ability with melatonin) and combining their physiological roles in the ovaries, we speculate that melatonin could play a beneficial role in ovaries via these targets. These results provide a preliminary basis and reference for future in-depth research on the mechanism of melatonin in animal models.
Important Pathways and Functional Modules of Melatonin’s Putative Targets
The pathophysiological mechanism of DOR is especially complicated, and various biological processes and pathways are involved in the DOR process. The 26 therapeutic targets screened in this study mainly participate in reproductive structure development, epithelial cell proliferation, the extrinsic apoptotic signaling pathway, PI3K signaling, and response to steroid hormones. Furthermore, the KEGG pathway analysis indicated that the PI3K-Akt signaling pathway (hsa04151) and the estrogen signaling pathway (hsa04915) were the two most enriched signaling pathways (Figure 6A). Accumulating evidence suggests that the PI3K-Akt signaling pathway plays a key role in folliculogenesis processes, including follicle recruitment, development, and maturation (63–65). The estrogen signaling pathway is vital for the maturation and maintenance of the female reproductive system (78).
To further understand melatonin mechanisms in improving OR, the target pathway network was divided into four densely linked functional modules, as shown in Figure 6C. The 1st module consists of pathways in the endocrine system and related signaling pathways. The 2nd module includes pathways in cell growth and death, the immune system, and related signaling pathways, and the 3rd module is related to signal transduction. The 4th module includes pathways in the endocrine system related to the regulation of ovarian function. According to the theory of network biology, the topology of a biological network is bridged to its function (79). These modules reflected melatonin's effects on endocrine and immune regulation, anti-apoptosis, and ovarian function improvement. In addition, exogenous growth hormone administration has been shown to improve oocyte and embryo quality in IVF treatment of women with poor OR (80, 81). The functional modules analysis showed that melatonin is closely related to the synthesis, secretion, and action of GH, which also supports the function of melatonin in improving OR.
Biological Processes and Organ Systems Regulated by Melatonin's Putative Targets
Importantly, in this study, to explain the multi-mechanism of melatonin on DOR, five sub-networks were constructed. The aging sub-network (Figure 8B) showed that melatonin targets AKT1, mTOR, and PIK3A, among others. The AKT/TOR pathway is a recognized central signaling pathway regulating lifespan, highlighting the anti-aging effect of melatonin (82, 83). Consistently, previous studies have shown that melatonin can prolong the lifespan and delay ovarian aging in mice (84, 85).
Apoptosis is a critical biological process that plays a vital role in germ cell depletion in mammalian ovaries (86). Follicular atresia caused by GC apoptosis is the primary process responsible for follicle loss (87–89). Bcl2-like-proteins are anti-apoptotic factors that may inhibit apoptosis. In the subnetwork of cell growth and death (Figure 8C), melatonin acts on BCL2, BCL2L1, BID, and CASP3, suggesting that melatonin exerts anti-apoptotic effects.
The sub-network of development and regeneration (Figure 8D) suggests the effect of melatonin in follicle development regulation. This network includes AKT, MAPK1, and HARS, which are involved in follicle growth and survival (90).
In addition to maintaining homeostasis, the immune system is associated with modulation at every level of the hypothalamic-pituitary-ovarian axis, as well as the regulation of proliferation and differentiation of ovarian germline stem cells (91). AKT1 and its interactions with MAPK1, JAK2, STAT1, etc., are involved in regulating melatonin in both the endocrine and immune systems (Figures 8E, F). Although they are not immune genes, they play an essential role in the division, differentiation, development, and function of various types of immune genes and immunomodulatory cytokines, including T-cells, IFN, Th17, and dendritic cells (92–94).
Conclusions
In summary, melatonin may improve OR by intervening in a series of targets (such as AKT1, EGFR, MAPK1, HRAS, SRC, ESR1, AR, and ALB), biological processes (reproductive structure development, epithelial cell proliferation, extrinsic apoptotic signaling pathway, PI3K signaling, and response to steroid hormone), and signaling pathways (such as PI3K-Akt and estrogen signaling pathways). Melatonin could exhibit anti-aging, anti-apoptosis, endocrine, and immune system regulation.
Data Availability Statement
The raw data supporting the conclusions of this article will be made available by the authors, without undue reservation.
Author Contributions
QZ conceptualized the manuscript. LY, HX, YC, YX, CM, and YZ collected the literature, wrote the manuscript, and made the figures. QZ edited and made significant revisions to the manuscript. All authors contributed to the article and approved the submitted version.
Funding
This work was financially supported through grants from the National Natural Science Foundation of China (81904241), the Program of Zhejiang Provincial TCM Sci-tech Plan (2020ZA078), the Medical and Health Science and Technology Plan of Zhejiang Province (2021KY920), and the Zhejiang Zhangqin Famous Traditional Chinese Medicine Expert Inheritance Studio Project (GZS2012014).
Conflict of Interest
The authors declare that the research was conducted in the absence of any commercial or financial relationships that could be construed as a potential conflict of interest.
Acknowledgments
We would like to thank Editage (www.editage.cn) for English language editing.
Supplementary Material
The Supplementary Material for this article can be found online at: https://www.frontiersin.org/articles/10.3389/fendo.2021.630504/full#supplementary-material
Abbreviations
AKT1, RAC-alpha serine/threonine-protein kinase; ALB, Albumin; AR, Androgen receptor; ART, Assisted reproductive technology; BC, Betweenness centrality; BP, Biological process; CC, Closeness centrality; DC,Degree centrality; DOR, Diminished ovarian reserve; EGFR, Epidermal growth factor receptor; ESR1, Estrogen receptor; GO, Gene Ontology; HRAS, GTPase HRas; KEGG, Kyoto Encyclopedia of Genes and Genomes; MAPK1, Mitogen-activated protein kinase 1; OR, Ovarian reserve; PPI, Protein–protein interaction; SMILES, Simplified molecular input entry specification; SRC, Proto-oncogene tyrosine-protein kinase Src; STRING, The Search Tool for the Retrieval of Interacting Genes; TCMSP, Traditional Chinese Medicine Systems Pharmacology Database and Analysis Platform; Uniprot, Universal Protein.
References
1. Inhorn MC, Patrizio P. Infertility around the globe: new thinking on gender, reproductive technologies and global movements in the 21st century. Hum Reprod Update (2015) 21(4):411–26. doi: 10.1093/humupd/dmv016
2. Macaluso M, Wright-Schnapp TJ, Chandra A, Johnson R, Satterwhite CL, Pulver A, et al. A public health focus on infertility prevention, detection, and management. Fertil Steril (2010) 93(1):16.e1–0. doi: 10.1016/j.fertnstert.2008.09.046
3. Pfister A, Crawford NM, Steiner AZ. Association between diminished ovarian reserve and luteal phase deficiency. Fertil Steril (2019) 112(2):378–86. doi: 10.1016/j.fertnstert.2019.03.032
4. Rasool S, Shah D. Fertility with early reduction of ovarian reserve: the last straw that breaks the Camel’s back. Fertil Res Pract (2017) 3(1):15. doi: 10.1186/s40738-017-0041-1
5. Bunnewell SJ, Honess ER, Karia AM, Keay SD, Al Wattar BH, Quenby S. Diminished ovarian reserve in recurrent pregnancy loss: a systematic review and meta-analysis. Fertil Steril (2020) 113(4):818–27.e3. doi: 10.1016/j.fertnstert.2019.11.014
6. Wald KA, Shahine LK, Lamb JD, Marshall LA, Hickok LR. High incidence of diminished ovarian reserve in young unexplained recurrent pregnancy loss patients. Gynecol Endocrinol (2020) 36(12):1079–81. doi: 10.1080/09513590.2020.1750001
7. Shahine LK, Marshall L, Lamb JD, Hickok LR. Higher rates of aneuploidy in blastocysts and higher risk of no embryo transfer in recurrent pregnancy loss patients with diminished ovarian reserve undergoing in vitro fertilization. Fertil Steril (2016) 106(5):1124–8. doi: 10.1016/j.fertnstert.2016.06.016
8. Devine K, Mumford SL, Wu M, DeCherney AH, Hill MJ, Propst A. Diminished ovarian reserve in the United States assisted reproductive technology population: diagnostic trends among 181,536 cycles from the Society for Assisted Reproductive Technology Clinic Outcomes Reporting System. Fertil Steril (2015) 104(3):612–19.e3. doi: 10.1016/j.fertnstert.2015.05.017
9. Romanski PA, Bortoletto P, Rosenwaks Z, Schattman GL. Delay in IVF treatment up to 180 days does not affect pregnancy outcomes in women with diminished ovarian reserve. Hum Reprod (2020) 35(7):1630–6. doi: 10.1093/humrep/deaa137
10. Eslami H, Eslami A, Favaedi R, Asadpour U, Zari Moradi S, Eftekhari-Yazdi P, et al. Epigenetic Aberration of FMR1 Gene in Infertile Women with Diminished Ovarian Reserve. Cell J (2018) 20(1):78–83. doi: 10.22074/cellj.2018.4398
11. Dong YZ, Zhou FJ, Sun YP. Psychological stress is related to a decrease of serum anti-müllerian hormone level in infertile women. Reprod Biol Endocrinol (2017) 15(1):51. doi: 10.1186/s12958-017-0271-4
12. Bahri S, Tehrani FR, Amouzgar A, Rahmati M, Tohidi M, Vasheghani M, et al. Overtime trend of thyroid hormones and thyroid autoimmunity and ovarian reserve: a longitudinal population study with a 12-year follow up. BMC Endocr Disord (2019) 19(1):47. doi: 10.1186/s12902-019-0370-7
13. Lee DY, Kim JY, Yu J, Kim SW. Prediction of Successful Ovarian Protection Using Gonadotropin-Releasing Hormone Agonists During Chemotherapy in Young Estrogen Receptor-Negative Breast Cancer Patients. Front Oncol (2020) 10:863. doi: 10.3389/fonc.2020.00863
14. Amanvermez R, Tosun M. An Update on Ovarian Aging and Ovarian Reserve Tests. Int J Fertil Steril (2016) 9(4):411–5. doi: 10.22074/ijfs.2015.4591
15. Karlberg S, Tiitinen A, Alfthan H, Lipsanen-Nyman M. Premature ovarian insufficiency and early depletion of the ovarian reserve in the monogenic Mulibrey nanism disorder. Hum Reprod (2018) 33(7):1254–61. doi: 10.1093/humrep/dey103
16. Bates GW Jr. Is hope on the horizon for premature ovarian insufficiency? Fertil Steril (2018) 109(5):800–1. doi: 10.1016/j.fertnstert.2018.02.129
17. Chen SN, Tsui KH, Wang PH, Chern CU, Wen ZH, Lin LT. Dehydroepiandrosterone Supplementation Improves the Outcomes of in vitro Fertilization Cycles in Older Patients With Diminished Ovarian Reserve. Front Endocrinol (Lausanne) (2019) 10:800. doi: 10.3389/fendo.2019.00800
18. Løvås K, Gebre-Medhin G, Trovik TS, Fougner KJ, Uhlving S, Nedrebø BG, et al. Replacement of dehydroepiandrosterone in adrenal failure: no benefit for subjective health status and sexuality in a 9-month, randomized, parallel group clinical trial. J Clin Endocrinol Metab (2003) 88(3):1112–8. doi: 10.1210/jc.2002-020769
19. Tordjman S, Chokron S, Delorme R, Charrier A, Bellissant E, Jaafari N, et al. Melatonin: Pharmacology, Functions and Therapeutic Benefits. Curr Neuropharmacol (2017) 15(3):434–43. doi: 10.2174/1570159x14666161228122115
20. Lerner AB, Case JD, Takahashi Y, Lee TH, Mori W. Isolation of melatonin, the pineal gland factor that lightens melanocytes1. J Am Chem Soc (1958) 80(10):2587–. doi: 10.1021/ja01543a060
21. Brzezinski A, Seibel MM, Lynch HJ, Deng M-H, Wurtman RJ. Melatonin in Human Preovulatory Follicular Fluid*. J Clin Endocrinol Metab (1987) 64(4):865–7. doi: 10.1210/jcem-64-4-865
22. Takasaki A, Nakamura Y, Tamura H, Shimamura K, Morioka H. Melatonin as a new drug for improving oocyte quality. Reprod Med Biol (2003) 2(4):139–44. doi: 10.1111/j.1447-0578.2003.00035.x
23. Tamura H, Nakamura Y, Korkmaz A, Manchester LC, Tan DX, Sugino N, et al. Melatonin and the ovary: physiological and pathophysiological implications. Fertil Steril (2009) 92(1):328–43. doi: 10.1016/j.fertnstert.2008.05.016
24. Jahromi BN, Sadeghi S, Alipour S, Parsanezhad ME, Alamdarloo SM. Effect of Melatonin on the Outcome of Assisted Reproductive Technique Cycles in Women with Diminished Ovarian Reserve: A Double-Blinded Randomized Clinical Trial. Iran J Med Sci (2017) 42(1):73–8.
25. Espino J, Macedo M, Lozano G, Ortiz Á, Rodríguez C, Rodríguez AB, et al. Impact of Melatonin Supplementation in Women with Unexplained Infertility Undergoing Fertility Treatment. Antioxid (Basel) (2019) 8(9):338. doi: 10.3390/antiox8090338
26. Olcese JM. Melatonin and Female Reproduction: An Expanding Universe. Front Endocrinol (Lausanne) (2020) 11:85. doi: 10.3389/fendo.2020.00085
27. Hu KL, Ye X, Wang S, Zhang D. Melatonin Application in Assisted Reproductive Technology: A Systematic Review and Meta-Analysis of Randomized Trials. Front Endocrinol (Lausanne) (2020) 11:160. doi: 10.3389/fendo.2020.00160
28. Tong J, Sheng S, Sun Y, Li H, Li WP, Zhang C, et al. Melatonin levels in follicular fluid as markers for IVF outcomes and predicting ovarian reserve. Reproduction (2017) 153(4):443–51. doi: 10.1530/rep-16-0641
29. Zheng M, Tong J, Li WP, Chen ZJ, Zhang C. Melatonin concentration in follicular fluid is correlated with antral follicle count (AFC) and in vitro fertilization (IVF) outcomes in women undergoing assisted reproductive technology (ART) procedures. Gynecol Endocrinol (2018) 34(5):446–50. doi: 10.1080/09513590.2017.1409713
30. He YT, Wang W, Shen W, Sun QY, Yin S. Melatonin protects against Fenoxaprop-ethyl exposure-induced meiotic defects in mouse oocytes. Toxicology (2019) 425:152241. doi: 10.1016/j.tox.2019.152241
31. Han L, Wang H, Li L, Li X, Ge J, Reiter RJ, et al. Melatonin protects against maternal obesity-associated oxidative stress and meiotic defects in oocytes via the SIRT3-SOD2-dependent pathway. J Pineal Res (2017) 63(3):e12431. doi: 10.1111/jpi.12431
32. Yang Q, Dai S, Luo X, Zhu J, Li F, Liu J, et al. Melatonin attenuates postovulatory oocyte dysfunction by regulating SIRT1 expression. Reproduction (2018) 156(1):81–92. doi: 10.1530/rep-18-0211
33. Nasheed Hamad Almohammed Z, Moghani-Ghoroghi F, Ragerdi-Kashani I, Fathi R, Tahaei LS, Naji M, et al. The Effect of Melatonin on Mitochondrial Function and Autophagy in In Vitro Matured Oocytes of Aged Mice. Cell J (2020) 22(1):9–16. doi: 10.22074/cellj.2020.6302
34. Guo Y, Sun J, Bu S, Li B, Zhang Q, Wang Q, et al. Melatonin protects against chronic stress-induced oxidative meiotic defects in mice MII oocytes by regulating SIRT1. Cell Cycle (2020) 19(13):1677–95. doi: 10.1080/15384101.2020.1767403
35. Li C, He X, Huang Z, Han L, Wu X, Li L, et al. Melatonin ameliorates the advanced maternal age-associated meiotic defects in oocytes through the SIRT2-dependent H4K16 deacetylation pathway. Aging (Albany NY) (2020) 12(2):1610–23. doi: 10.18632/aging.102703
36. Li R, Guo C, Li Y, Qin Z, Huang W. Therapeutic targets and signaling mechanisms of vitamin C activity against sepsis: a bioinformatics study. Brief Bioinform (2020). doi: 10.1093/bib/bbaa079
37. Xie B, Geng Q, Xu J, Lu H, Luo H, Hu Y, et al. The multi-targets mechanism of hydroxychloroquine in the treatment of systemic lupus erythematosus based on network pharmacology. Lupus (2020) 29(13):1704–11. doi: 10.1177/0961203320952541
38. Li R, Guo C, Li Y, Liang X, Yang L, Huang W. Therapeutic target and molecular mechanism of vitamin C-treated pneumonia: a systematic study of network pharmacology. Food Funct (2020) 11(5):4765–72. doi: 10.1039/d0fo00421a
39. Kim S, Thiessen PA, Bolton EE, Chen J, Fu G, Gindulyte A, et al. PubChem Substance and Compound databases. Nucleic Acids Res (2016) 44(D1):D1202–13. doi: 10.1093/nar/gkv951
40. Wang X, Shen Y, Wang S, Li S, Zhang W, Liu X, et al. PharmMapper 2017 update: a web server for potential drug target identification with a comprehensive target pharmacophore database. Nucleic Acids Res (2017) 45(W1):W356–w60. doi: 10.1093/nar/gkx374
41. Daina A, Michielin O, Zoete V. SwissTargetPrediction: updated data and new features for efficient prediction of protein targets of small molecules. Nucleic Acids Res (2019) 47(W1):W357–W64. doi: 10.1093/nar/gkz382
42. Wishart DS, Feunang YD, Guo AC, Lo EJ, Marcu A, Grant JR, et al. DrugBank 5.0: a major update to the DrugBank database for 2018. Nucleic Acids Res (2018) 46(D1):D1074–d82. doi: 10.1093/nar/gkx1037
43. Ru J, Li P, Wang J, Zhou W, Li B, Huang C, et al. TCMSP: a database of systems pharmacology for drug discovery from herbal medicines. J Cheminform (2014) 6:13. doi: 10.1186/1758-2946-6-13
44. Piñero J, Ramírez-Anguita JM, Saüch-Pitarch J, Ronzano F, Centeno E, Sanz F, et al. The DisGeNET knowledge platform for disease genomics: 2019 update. Nucleic Acids Res (2020) 48(D1):D845–d55. doi: 10.1093/nar/gkz1021
45. Szklarczyk D, Gable AL, Lyon D, Junge A, Wyder S, Huerta-Cepas J, et al. STRING v11: protein-protein association networks with increased coverage, supporting functional discovery in genome-wide experimental datasets. Nucleic Acids Res (2019) 47(D1):D607–d13. doi: 10.1093/nar/gky1131
46. Yu G, Wang LG, Han Y, He QY. clusterProfiler: an R package for comparing biological themes among gene clusters. Omics (2012) 16(5):284–7. doi: 10.1089/omi.2011.0118
47. Otasek D, Morris JH, Bouças J, Pico AR, Demchak B. Cytoscape Automation: empowering workflow-based network analysis. Genome Biol (2019) 20(1):185. doi: 10.1186/s13059-019-1758-4
48. Morris GM, Huey R, Lindstrom W, Sanner MF, Belew RK, Goodsell DS, et al. AutoDock4 and AutoDockTools4: Automated docking with selective receptor flexibility. J Comput Chem (2009) 30(16):2785–91. doi: 10.1002/jcc.21256
49. Trott O, Olson AJ. AutoDock Vina: improving the speed and accuracy of docking with a new scoring function, efficient optimization, and multithreading. J Comput Chem (2010) 31(2):455–61. doi: 10.1002/jcc.21334
50. Lyttle Schumacher BM, Jukic AMZ, Steiner AZ. Antimüllerian hormone as a risk factor for miscarriage in naturally conceived pregnancies. Fertil Steril (2018) 109(6):1065–71.e1. doi: 10.1016/j.fertnstert.2018.01.039
51. Quinn MM, Cedars MI. Cardiovascular health and ovarian aging. Fertil Steril (2018) 110(5):790–3. doi: 10.1016/j.fertnstert.2018.07.1152
52. Bleil ME, Bromberger JT, Latham MD, Adler NE, Pasch LA, Gregorich SE, et al. Disruptions in ovarian function are related to depression and cardiometabolic risk during premenopause. Menopause (2013) 20(6):631–9. doi: 10.1097/GME.0b013e31827c5c45
53. Kim MY, Park JY, Park HS. Akt1-Mediated Phosphorylation of RBP-Jk Controls Notch1 Signaling. Biochem (Moscow) (2019) 84(12):1537–46. doi: 10.1134/S0006297919120137
54. Yang C, Liu Q, Chen Y, Wang X, Ran Z, Fang F, et al. Melatonin as an endogenous hormone to slow down the exhaustion of ovarian follicle reserve in mice–a novel insight into its roles in early folliculogenesis and ovarian aging. Research Square (2020). doi: 10.21203/rs.3.rs-90252/v1
55. Wang X, Meng K, He Y, Wang H, Zhang Y, Quan F. Melatonin Stimulates STAR Expression and Progesterone Production via Activation of the PI3K/AKT Pathway in Bovine Theca Cells. Int J Biol Sci (2019) 15(2):404–15. doi: 10.7150/ijbs.27912
56. El Sheikh M, Mesalam A, Mesalam AA, Idrees M, Lee KL, Kong IK. Melatonin Abrogates the Anti-Developmental Effect of the AKT Inhibitor SH6 in Bovine Oocytes and Embryos. Int J Mol Sci (2019) 20(12):2956. doi: 10.3390/ijms20122956
57. Light A, Hammes SR. LH-Induced Steroidogenesis in the Mouse Ovary, but Not Testis, Requires Matrix Metalloproteinase 2- and 9-Mediated Cleavage of Upregulated EGF Receptor Ligands. Biol Reprod (2015) 93(3):65. doi: 10.1095/biolreprod.115.130971
58. Richani D, Gilchrist RB. The epidermal growth factor network: role in oocyte growth, maturation and developmental competence. Hum Reprod Update (2017) 24(1):1–14. doi: 10.1093/humupd/dmx029
59. Yong H, Oh HI, Lee SH, Cheong HT, Yang BK, Park CK. Treatment of Epidermal Growth Factor (EGF) enhances Nuclear Maturation of Porcine Oocytes and Stimulates Expression of ER/Golgi Transport Proteins. Dev Reprod (2017) 21(2):131–8. doi: 10.12717/dr.2017.21.2.131
60. Carbajal L, Biswas A, Niswander LM, Prizant H, Hammes SR. GPCR/EGFR Cross Talk Is Conserved in Gonadal and Adrenal Steroidogenesis but Is Uniquely Regulated by Matrix Metalloproteinases 2 and 9 in the Ovary. Mol Endocrinol (2011) 25(6):1055–65. doi: 10.1210/me.2010-0410
61. Tian X, Wang F, He C, Zhang L, Tan D, Reiter RJ, et al. Beneficial effects of melatonin on bovine oocytes maturation: a mechanistic approach. J Pineal Res (2014) 57(3):239–47. doi: 10.1111/jpi.12163
62. Tian X, Wang F, Zhang L, He C, Ji P, Wang J, et al. Beneficial Effects of Melatonin on the In Vitro Maturation of Sheep Oocytes and Its Relation to Melatonin Receptors. Int J Mol Sci (2017) 18(4):834. doi: 10.3390/ijms18040834
63. Zhang H, Qin F, Liu A, Sun Q, Wang Q, Li Q, et al. Kuntai capsule attenuates premature ovarian failure through the PI3K/AKT/mTOR pathway. J Ethnopharmacol (2019) 239:111885. doi: 10.1016/j.jep.2019.111885
64. Zhang H, Qin F, Liu A, Sun Q, Wang Q, Xie S, et al. Electro-acupuncture attenuates the mice premature ovarian failure via mediating PI3K/AKT/mTOR pathway. Life Sci (2019) 217:169–75. doi: 10.1016/j.lfs.2018.11.059
65. Grosbois J, Demeestere I. Dynamics of PI3K and Hippo signaling pathways during in vitro human follicle activation. Hum Reprod (2018) 33(9):1705–14. doi: 10.1093/humrep/dey250
66. Wee P, Wang Z. Epidermal Growth Factor Receptor Cell Proliferation Signaling Pathways. Cancers (Basel) (2017) 9(5):52. doi: 10.3390/cancers9050052
67. Kahnamouyi S, Nouri M, Farzadi L, Darabi M, Hosseini V, Mehdizadeh A. The role of mitogen-activated protein kinase-extracellular receptor kinase pathway in female fertility outcomes: a focus on pituitary gonadotropins regulation. Ther Adv Endocrinol Metab (2018) 9(7):209–15. doi: 10.1177/2042018818772775
68. Bliss SP, Miller A, Navratil AM, Xie J, McDonough SP, Fisher PJ, et al. ERK signaling in the pituitary is required for female but not male fertility. Mol Endocrinol (2009) 23(7):1092–101. doi: 10.1210/me.2009-0030
69. Woo MM, Tai CJ, Kang SK, Nathwani PS, Pang SF, Leung PC. Direct action of melatonin in human granulosa-luteal cells. J Clin Endocrinol Metab (2001) 86(10):4789–97. doi: 10.1210/jcem.86.10.7912
70. Kechiche S, Venditti M, Knani L, Jabłońska K, Dzięgiel P, Messaoudi I, et al. First evidence of the protective role of melatonin in counteracting cadmium toxicity in the rat ovary via the mTOR pathway. Environ Pollut (2021) 270:116056. doi: 10.1016/j.envpol.2020.116056
71. Ożegowska K, Brązert M, Ciesiółka S, Nawrocki MJ, Kranc W, Celichowski P, et al. Genes Involved in the Processes of Cell Proliferation, Migration, Adhesion, and Tissue Development as New Potential Markers of Porcine Granulosa Cellular Processes In Vitro: A Microarray Approach. DNA Cell Biol (2019) 38(6):549–60. doi: 10.1089/dna.2018.4467
72. Franks S, Hardy K. Androgen Action in the Ovary. Front Endocrinol (Lausanne) (2018) 9:452. doi: 10.3389/fendo.2018.00452
73. Laird M, Thomson K, Fenwick M, Mora J, Franks S, Hardy K. Androgen Stimulates Growth of Mouse Preantral Follicles In Vitro: Interaction With Follicle-Stimulating Hormone and With Growth Factors of the TGFβ Superfamily. Endocrinology (2017) 158(4):920–35. doi: 10.1210/en.2016-1538
74. Baba T, Ting AY, Tkachenko O, Xu J, Stouffer RL. Direct actions of androgen, estrogen and anti-Müllerian hormone on primate secondary follicle development in the absence of FSH in vitro. Hum Reprod (2017) 32(12):2456–64. doi: 10.1093/humrep/dex322
75. Fujibe Y, Baba T, Nagao S, Adachi S, Ikeda K, Morishita M, et al. Androgen potentiates the expression of FSH receptor and supports preantral follicle development in mice. J Ovarian Res (2019) 12(1):31. doi: 10.1186/s13048-019-0505-5
76. Smith P, Juengel J, Maclean P, Rand C, Stanton JL. Gestational nutrition 2: gene expression in sheep fetal ovaries exposed to gestational under nutrition. Reproduction (2019) 157(1):13–25. doi: 10.1530/rep-18-0358
77. Baker ME. Albumin, steroid hormones and the origin of vertebrates. J Endocrinol (2002) 175(1):121–7. doi: 10.1677/joe.0.1750121
78. Findlay JK, Liew SH, Simpson ER, Korach KS. Estrogen signaling in the regulation of female reproductive functions. Handb Exp Pharmacol (2010) 198):29–35. doi: 10.1007/978-3-642-02062-9_2
79. Barabási A-L, Oltvai ZN. Network biology: understanding the cell’s functional organization. Nat Rev Genet (2004) 5(2):101–13. doi: 10.1038/nrg1272
80. Hart RJ. Use of Growth Hormone in the IVF Treatment of Women With Poor Ovarian Reserve. Front Endocrinol (Lausanne) (2019) 10:500. doi: 10.3389/fendo.2019.00500
81. Gong Y, Zhang K, Xiong D, Wei J, Tan H, Qin S. Growth hormone alleviates oxidative stress and improves the IVF outcomes of poor ovarian responders: a randomized controlled trial. Reprod Biol Endocrinol (2020) 18(1):91. doi: 10.1186/s12958-020-00648-2
82. Na H-J, Park J-S, Pyo J-H, Jeon H-J, Kim Y-S, Arking R, et al. Metformin inhibits age-related centrosome amplification in Drosophila midgut stem cells through AKT/TOR pathway. Mech Ageing Dev (2015) 149:8–18. doi: 10.1016/j.mad.2015.05.004
83. Pan H, Finkel T. Key proteins and pathways that regulate lifespan. J Biol Chem (2017) 292(16):6452–60. doi: 10.1074/jbc.R116.771915
84. Tamura H, Kawamoto M, Sato S, Tamura I, Maekawa R, Taketani T, et al. Long-term melatonin treatment delays ovarian aging. J Pineal Res (2017) 62(2):e12381. doi: 10.1111/jpi.12381
85. Zhang L, Zhang Z, Wang J, Lv D, Zhu T, Wang F, et al. Melatonin regulates the activities of ovary and delays the fertility decline in female animals via MT1/AMPK pathway. J Pineal Res (2019) 66(3):e12550. doi: 10.1111/jpi.12550
86. Yadav PK, Tiwari M, Gupta A, Sharma A, Prasad S, Pandey AN, et al. Germ cell depletion from mammalian ovary: possible involvement of apoptosis and autophagy. J Biomed Sci (2018) 25(1):36. doi: 10.1186/s12929-018-0438-0
87. Regan SLP, Knight PG, Yovich JL, Stanger JD, Leung Y, Arfuso F, et al. The effect of ovarian reserve and receptor signalling on granulosa cell apoptosis during human follicle development. Mol Cell Endocrinol (2018) 470:219–27. doi: 10.1016/j.mce.2017.11.002
88. Krysko DV, Diez-Fraile A, Criel G, Svistunov AA, Vandenabeele P, D’Herde K. Life and death of female gametes during oogenesis and folliculogenesis. Apoptosis (2008) 13(9):1065–87. doi: 10.1007/s10495-008-0238-1
89. Regan SLP, Knight PG, Yovich JL, Leung Y, Arfuso F, Dharmarajan A. Granulosa Cell Apoptosis in the Ovarian Follicle-A Changing View. Front Endocrinol (Lausanne) (2018) 9:61. doi: 10.3389/fendo.2018.00061
90. Andrade GM, da Silveira JC, Perrini C, Del Collado M, Gebremedhn S, Tesfaye D, et al. The role of the PI3K-Akt signaling pathway in the developmental competence of bovine oocytes. PloS One (2017) 12(9):e0185045. doi: 10.1371/journal.pone.0185045
91. Ye H, Li X, Zheng T, Liang X, Li J, Huang J, et al. The effect of the immune system on ovarian function and features of ovarian germline stem cells. Springerplus (2016) 5(1):990. doi: 10.1186/s40064-016-2390-3
92. Kang YH, Biswas A, Field M, Snapper SB. STAT1 signaling shields T cells from NK cell-mediated cytotoxicity. Nat Commun (2019) 10(1):912. doi: 10.1038/s41467-019-08743-8
93. Mogensen TH. IRF and STAT Transcription Factors - From Basic Biology to Roles in Infection, Protective Immunity, and Primary Immunodeficiencies. Front Immunol (2019) 9:3047. doi: 10.3389/fimmu.2018.03047
Keywords: diminished ovarian reserve (DOR), ovarian reserve (OR), melatonin, potential therapeutic targets, signaling pathways, biological processes, network pharmacology
Citation: Yang L, Xu H, Chen Y, Miao C, Zhao Y, Xing Y and Zhang Q (2021) Melatonin: Multi-Target Mechanism Against Diminished Ovarian Reserve Based on Network Pharmacology. Front. Endocrinol. 12:630504. doi: 10.3389/fendo.2021.630504
Received: 17 November 2020; Accepted: 29 March 2021;
Published: 19 April 2021.
Edited by:
Nikolaos P. Polyzos, Dexeus University Hospital, SpainReviewed by:
Soledad Rossi, CONICET Instituto de Biología y Medicina Experimental (IBYME), ArgentinaJulio Martín Voget, Androfert, Andrology and Human Reproduction Clinic, Brazil
Copyright © 2021 Yang, Xu, Chen, Miao, Zhao, Xing and Zhang. This is an open-access article distributed under the terms of the Creative Commons Attribution License (CC BY). The use, distribution or reproduction in other forums is permitted, provided the original author(s) and the copyright owner(s) are credited and that the original publication in this journal is cited, in accordance with accepted academic practice. No use, distribution or reproduction is permitted which does not comply with these terms.
*Correspondence: Qin Zhang, zhaqin01@163.com
†These authors have contributed equally to this work