- 1Department of Endocrinology, Beijing Hospital, The Key Laboratory of Geriatrics, Beijing Institute of Geriatrics, National Center of Gerontology, National Health Commission, Institute of Geriatric Medicine, Chinese Academy of Medical Sciences, Beijing, China
- 2The Savaid School of Medicine, University of Chinese Academy of Sciences, Beijing, China
Background: Iron deficiency (ID) is concerned as the most common nutritional deficiency worldwide. The effects of ID on thyroid function and autoimmunity in pregnant women and reproductive-age women are controversial. The aim of the current study was to summarize the evidences and evaluate the relationship between ID and thyroid disorders.
Methods: In this systematic review and meta-analysis, studies published on the Cochrane, Embase, Medline, and PubMed databases by October 2020 were searched. A total of 636 studies which discussed the correlation between ID and thyroid disorders were eligible in the initial search. Pooled mean differences (MD) and 95% confidence intervals (CI) were calculated for the assessment of thyrotropin (TSH) and free thyroxine (FT4) levels. Combined odd ratios (OR) and 95% CI were calculated for the assessment of the prevalence of overt and subclinical hypothyroidism, positive thyroid peroxidase antibody (TPOAb), and thyroglobulin antibody (TgAb).
Results: For women of reproductive age, ID could significantly increase the risk of positive TPOAb (OR: 1.89; 95% CI: 1.17, 3.06: P = 0.01) and both positive TPOAb and TgAb (OR: 1.48; 95% CI: 1.03, 2.11: P = 0.03). The meta-analysis of pregnant women showed that pregnant women with ID had increased serum TSH levels (MD: 0.12; 95% CI: 0.07, 0.17; P < 0.00001) and decreased FT4 levels (MD: −0.73; 95% CI: −1.04, −0.41; P < 0.00001). Meanwhile, the prevalence of overt (OR: 1.60; 95% CI: 1.17, 2.19; P = 0.004) and subclinical (OR: 1.37; 95% CI: 1.13, 1.66; P = 0.001) hypothyroidism in pregnant women with ID was significantly increased.
Conclusions: ID may adversely affect thyroid function and autoimmunity of pregnant and reproductive-age women and it is very necessary for monitoring iron nutritional status and early treatment of ID for them.
Introduction
Thyroid dysfunction is a common endocrine disorder in pregnant women, and consists of imbalanced thyroid hormone levels (including overt and subclinical hypothyroidism, overt and subclinical hyperthyroidism) and positive thyroid antibody status. Studies have shown that about 0.3%–3% of pregnant women suffer from hypothyroidism (1). Hypothyroidism leads to multiple adverse outcomes such as premature delivery, miscarriage, neurodevelopmental dysplasia, and increased risk of autism and asthma in the offspring (1–5). In addition, more pregnant women (about 18%) are tested positive for thyroid autoantibodies (Thyroid peroxidase antibody, TPOAb or thyroglobulin antibody, TgAb), which will also adversely affect pregnant women and their offspring (1). A meta-analysis presented that positive TPOAb increased the risk of post-partum depression by 1.5 times (6). Pregnant women with positive thyroid antibodies have a higher risk of preterm birth and their infants are more likely to suffer from respiratory distress (7). Although the finding needs to be confirmed by more high-quality studies, thyroid disorders in pregnant women might be suggested to be prevented, diagnosed, and treated early.
Iron is an important micronutrient for maintaining cell energy and metabolism (8). Iron deficiency (ID) manifested as a decrease in the extracellular iron of the bone marrow and lower serum ferritin than normal, is concerned as the most common nutritional deficiency worldwide and can lead to adverse effects on thyroid metabolism in both reproductive-age women and pregnant women (9, 10). Some studies have shown that ID can negatively impact thyroid function by interfering with oxygen transport or affecting thyroid peroxidase activity (11, 12). N Okuroglu et al.’s study showed that there were no differences in the prevalence of positive TPOAb, thyrotropin (TSH), and free thyroxine (FT4) levels between reproductive-age women in ID group and control group (13). However, Zhang et al. hold the opposite view. They believed that the prevalence of positive TPOAb increased and serum FT4 levels decreased in women of reproductive age with ID (9). Veltri et al. and Yu et al. haven’t reached a consensus on whether serum TSH levels were elevated in pregnant women with ID (14, 15). Therefore, a meta-analysis was conducted to summarize the evidences and evaluate the relationship between ID and thyroid function and autoantibodies.
Methods
A systematic review and meta-analysis was conducted to answer the following PICO question: “Do pregnant or reproductive-age women with ID have a higher risk of thyroid function or autoimmune abnormalities than them without ID?” The Preferred Reporting Items for systematic review and meta-analysis protocols (PRISMA-P) were referenced in the process of carrying out our meta-analyses (16).
Literature Search Protocol
Literature published on the Cochrane, Embase, Medline and PubMed databases by October 2020 were searched. The search keywords used were: iron, pregnant, pregnancy, reproductive-age, thyroid function, thyroid dysfunction, thyroid disease, hypothyroidism, hyperthyroidism, thyroid peroxidase antibody, and thyroglobulin antibody. The search formula and the number of results in each database were shown in Supplementary Table 1.
Inclusion/Exclusion Criteria
The inclusion criteria were as follows: Reproductive-age women (Women aged 15–49 years old who can give birth to a baby) or pregnant women were considered as the research object; studies in English language; studies discussed the correlation between ID and thyroid function or autoantibodies; participants were divided into groups according to with ID or not; data on thyroid function indicators, autoantibodies, or the prevalence of overt hypothyroidism and subclinical hypothyroidism can be extracted. Books, conference abstracts, reports, comments, and animal experiments that could not be used for further data extraction and analyses were excluded.
Data Extraction
Two of the researchers extracted the following information of the included studies independently: first author, publication year, country of the population, pregnant stage, participants number in each group, diagnostic criteria for ID and thyroid disorders, study type, TSH and FT4 values from participants with or without ID, the events number of overt hypothyroidism, subclinical hypothyroidism, positive TPOAb and TgAb, and positive TPOAb, positive TgAb from participants with or without ID.
Quality Assessment
The Agency for Healthcare Research and Quality (AHRQ) scale was used for the assessment of the quality of the included studies. Studies with 0–3 points, 4–7 points, and 8–11 points were considered as low-, moderate-, and high-quality, respectively (17).
Statistical Analysis
We imported the data into the Review Manager software (Revman 5.4.1) to evaluate the differences between TSH and FT4 values of participants with or without ID, and to compare the prevalence of overt hypothyroidism, subclinical hypothyroidism, and positive thyroid autoantibodies. For dichotomous outcomes, the Mantel–Haenszel statistical method was used to calculate the significance of the pooled odds ratios (OR). For continuous outcomes, the Inverse-Variance statistical method was used to calculate the significance of the pooled mean differences (MD). Some studies provided data as median and interquartile range (or maximum and minimum), and we calculated their mean and standard deviation according to formulas (18, 19). Then we combined the estimated mean and standard deviation with data from other studies.
>I2 was used to estimate the level of study heterogeneity. I2 values of 25%, 50%, and 75% were identified as low, medium, and high heterogeneity, respectively. When I2 was greater than 50, the random-effects model was used to summarize the results. When I2 was less than 50, the fixed-effects model was used to summarize the results. Sensitivity analyses were conducted to interpret the source of heterogeneity. The protocol of the sensitivity analysis was to delete one study from each rotation and then re-calculated the pooled results of the remaining studies. P < 0.05 was considered to indicate statistical significance.
Begg’s tests and Egger’s tests were used to estimate the publication bias.
Results
Characteristics of the Included Studies
A total of 636 articles were searched from the Cochrane, Embase, Medline and PubMed databases based on the keywords. After removing the duplicate articles, 445 articles’ titles and abstracts were read. Sixteen of them were downloaded in full and read carefully. Eight articles were excluded because the data couldn’t be extracted (20, 21) or the research subjects were not grouped according to ID (10, 22–26). Finally, a total of eight articles were included in our meta-analysis (Figure 1). The quality of studies evaluated by AHRQ was shown in Supplementary Table 2. The basic information of the included articles was shown in Table 1.
Association Between ID and TSH Status in Reproductive-Age Women
The pooled results from the comparison of serum TSH levels between ID group and control group were shown in Figure 2A. There were no statistical differences in TSH levels between the two groups (MD: 0.06; 95% CI: −0.04, 0.16; P = 0.25; I2: 42%). Sensitivity analyses showed that the combined MD was stable after one research had been deleted. Begg’s test (P = 1.000) and Egger’s test (P = 0.753) did not indicate publication bias.
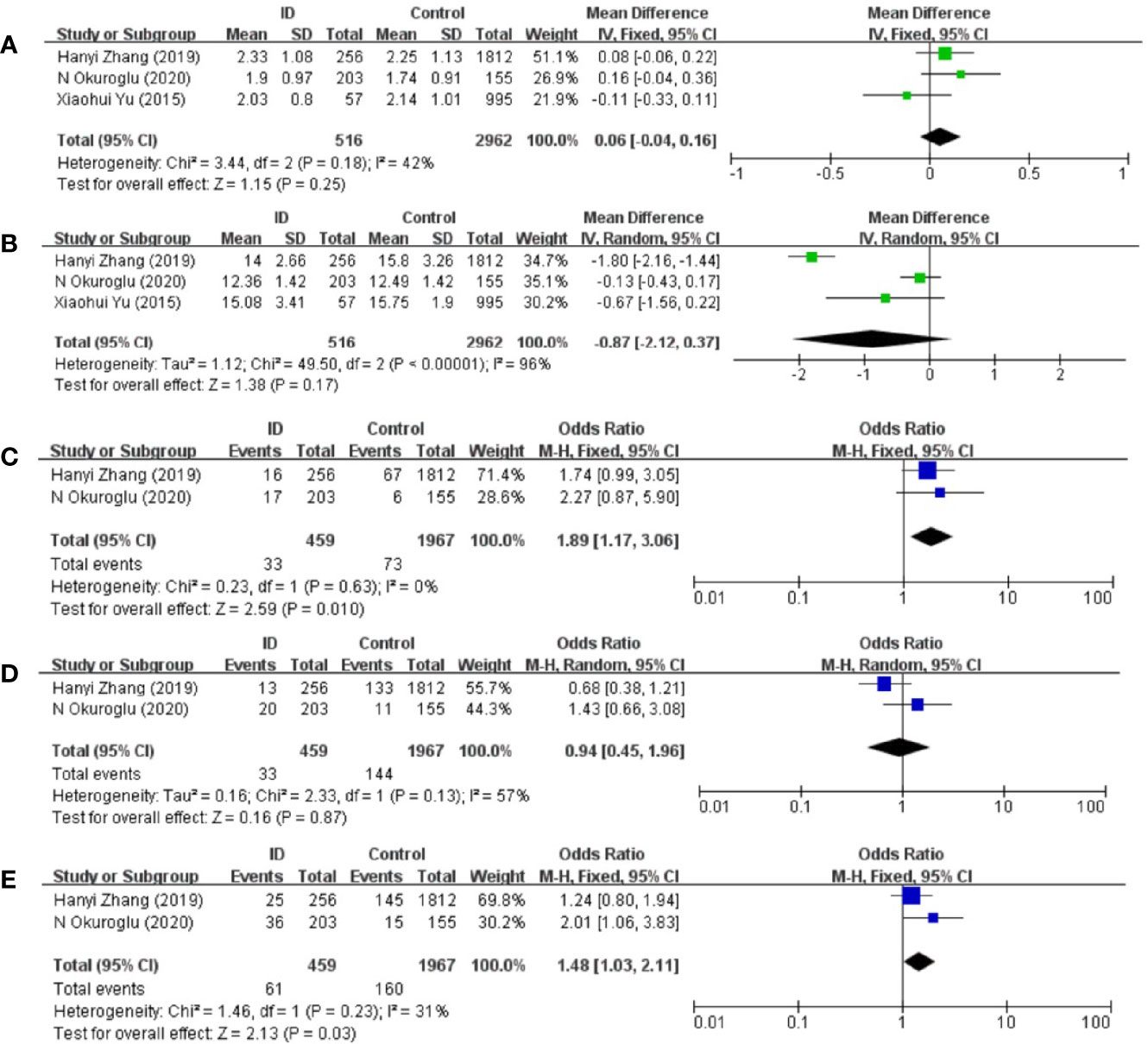
Figure 2 Forest plot of studies comparing reproductive-age women with iron deficiency (ID) to them without iron deficiency (Control) for (A) TSH, (B) FT4 and prevalence of (C) positive TPOAb, (D) positive TgAb, and (E) positive TPOAb and TgAb.
Association Between ID and FT4 Status in Reproductive-Age Women
The pooled results from the comparison of serum FT4 levels between ID group and control group were shown in Figure 2B. FT4 levels in ID group were lower than them in control group in the included articles. However, there was still no statistical difference in FT4 levels between the two groups (MD: −0.87; 95% CI: −2.12, 0.37; P = 0.17; I2: 96%). Heterogeneity decreased when Zhang et al.’s article was removed (I2: 21%). Begg’s test (P = 1.000) and Egger’s test (P = 0.462) did not indicate publication bias.
Association Between ID and TPOAb-Positive Status in Reproductive-Age Women
Compared with participants in control group, participants in ID group had higher risk of positive TPOAb (OR: 1.89; 95% CI: 1.17, 3.06: P = 0.01; I2: 0%; Figure 2C).
Association Between ID and TgAb-Positive Status in Reproductive-Age Women
There were no statistical differences in TgAb levels between ID group and control group (OR: 0.94; 95% CI: 0.45, 1.96: P = 0.87; I2: 57%; Figure 2D).
Association Between ID and Autoantibodies-Positive Status in Reproductive-Age Women
Compared with participants in control group, participants in ID group had higher risk of both positive TgAb and TPOAb (OR: 1.48; 95% CI: 1.03, 2.11; P = 0.03; I2: 31%; Figure 2E).
Association Between ID and TSH Status in Pregnant Women
Meta-analysis of the six articles using the pooled results from the comparison of serum TSH levels between ID group and control group were shown in Figure 3A. TSH levels were higher in ID group than them in control group (MD: 0.12; 95% CI: 0.07, 0.17; P < 0.00001; I2: 0%). Sensitivity analyses showed that the combined MD was stable after one research had been deleted. Begg’s test (P = 0.707) and Egger’s test (P = 0.910) did not indicate publication bias.
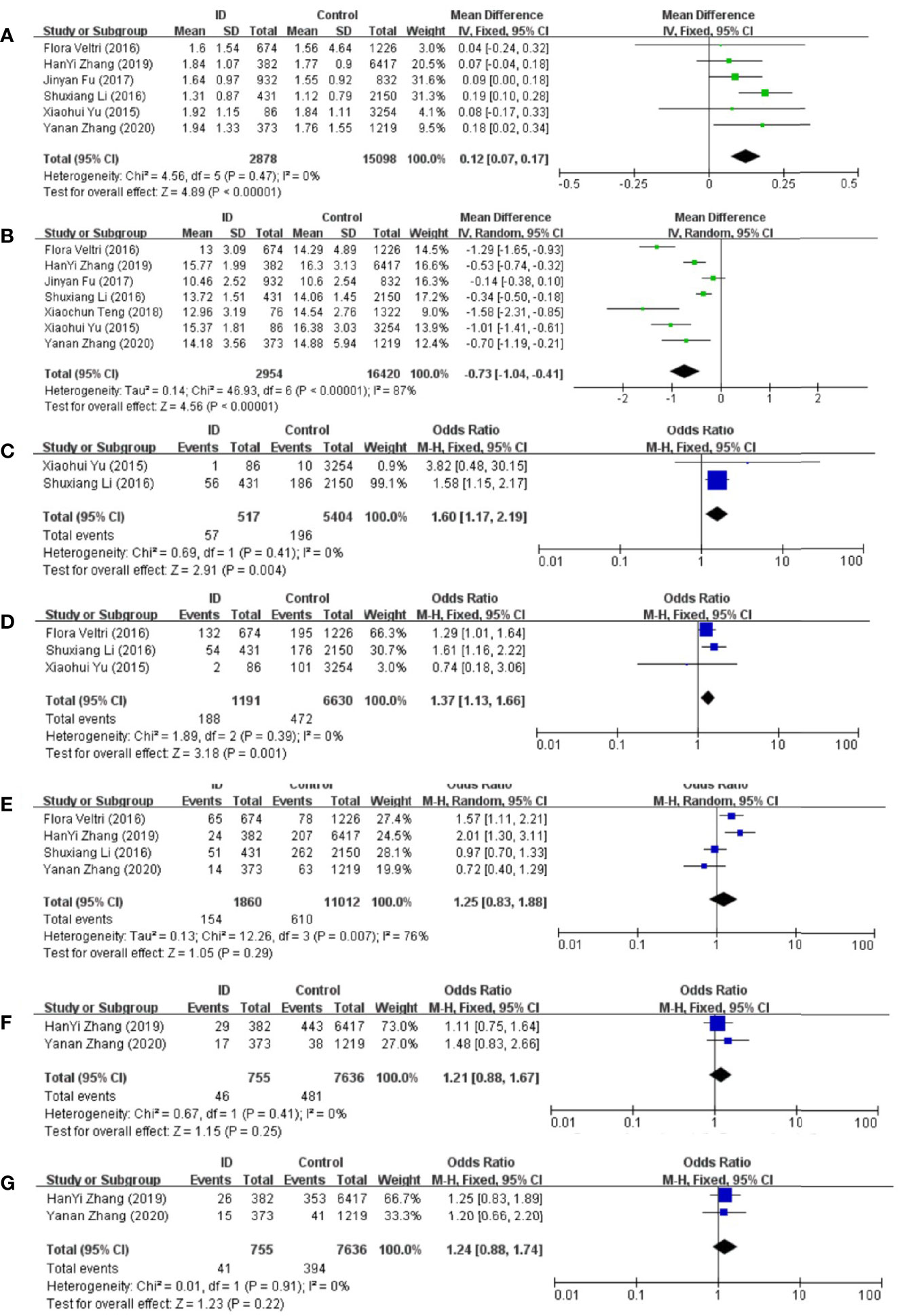
Figure 3 Forest plot of studies comparing pregnant women with iron deficiency (ID) to them without iron deficiency (Control) for (A) TSH, (B) FT4, and prevalence of (C) overt hypothyroidism, (D) subclinical hypothyroidism, (E) positive TPOAb, (F) positive TgAb, and (G) positive TPOAb and TgAb.
Association Between ID and FT4 Status in Pregnant Women
Meta-analysis of the six articles using the pooled results from the comparison of serum FT4 levels between ID group and control group were shown in Figure 3B. FT4 levels were lower in ID group than them in control group (MD: −0.73; 95% CI: −1.04, −0.41; P < 0.00001; I2: 87%). Sensitivity analysis showed that the combined MD was stable after one research had been deleted. In order to explore the source of heterogeneity, we conducted a subgroup analysis based on the different diagnostic criteria of ID. The heterogeneity was still high after the subgroup analysis, which might be due to the different time of the diagnosis of ID and the measurement of biochemical indicators in different articles. Begg’s test (P = 0.230) and Egger’s test (P = 0.152) did not indicate publication bias.
Association Between ID and Overt Hypothyroidism Prevalence in Pregnant Women
Compared with control group, subjects in ID group had higher risk of overt hypothyroidism (OR: 1.60; 95% CI: 1.17, 2.19; P = 0.004; I2: 0%; Figure 3C).
Association Between ID and Subclinical Hypothyroidism Prevalence in Pregnant Women
Compared with control group, subjects in ID group had higher risk of subclinical hypothyroidism (OR: 1.37; 95% CI: 1.13, 1.66; P = 0.001; I2: 0%; Figure 3D).
Association Between ID and TPOAb-Positive Status in Pregnant Women
The pooled results from the comparison of serum TPOAb levels between ID group and control group were shown in Figure 3E. There was no significant association in TPOAb levels between the two groups (OR: 1.25; 95% CI: 0.83, 1.88; P = 0.29; I2: 76%). Sensitivity analyses showed that the combined OR was stable after one research had been deleted. To explore the source of heterogeneity, a subgroup analysis was conducted based on the different diagnostic criteria of ID. The subgroup analysis showed that subjects in ID group had higher risk to have positive TPOAb (OR: 1.70; 95% CI: 1.30–2.24; P = 0.0001; I2: 0%; Figure 4). Begg’s test (P = 0.734) and Egger’s test (P = 0.851) did not indicate publication bias.
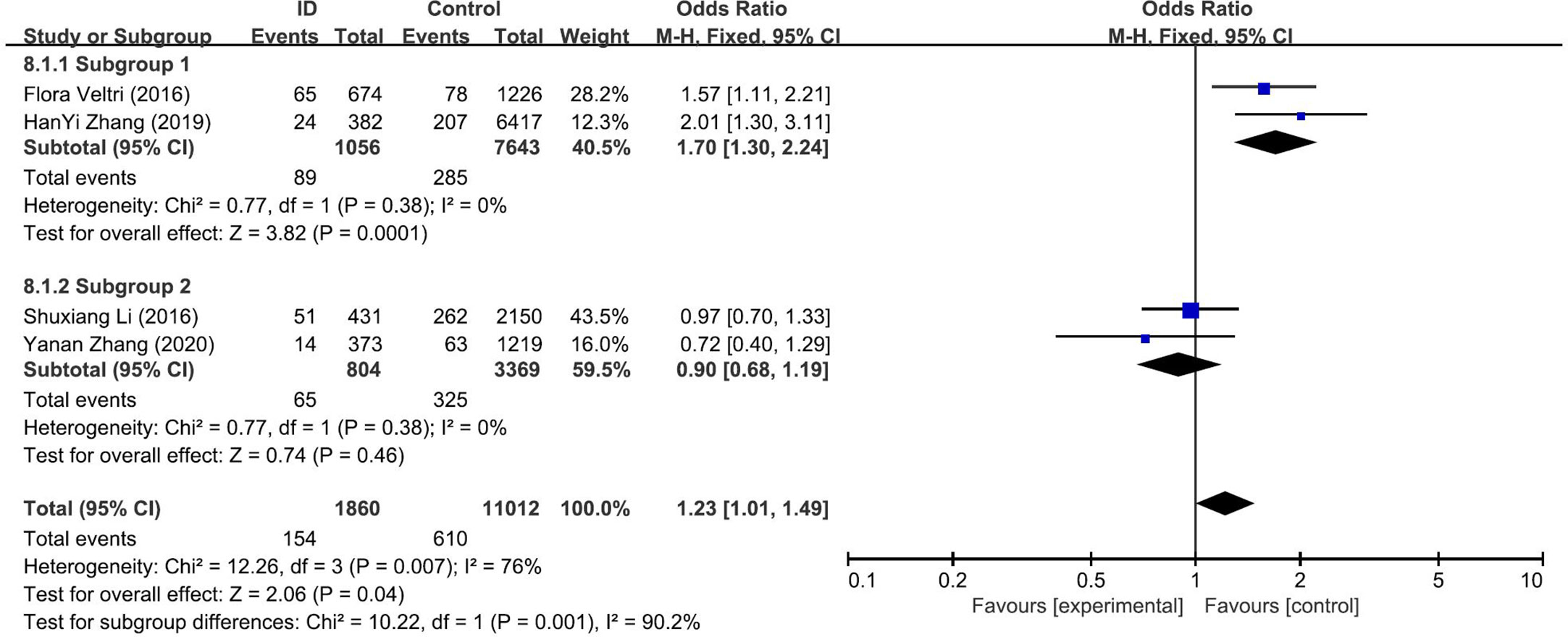
Figure 4 Forest plot of the subgroup analysis showing the comparison of prevalence of positive TPOAb in pregnant women with iron deficiency (ID) to those without iron deficiency (Control) according to different diagnostic crriteria for ID. Subgroup 1: <15ng/dl; Subgroup 2: <20ng/dl.
Association Between ID and TgAb-Positive Status in Pregnant Women
The pooled results from the comparison of serum TgAb levels between ID group and control group were shown in Figure 3F. There was no significant association in TgAb levels between the two groups (OR: 1.21; 95% CI: 0.88, 1.67; P = 0.25; I2: 0%).
Association Between ID and Autoantibodies-Positive Status in Pregnant Women
There was no significant association of autoantibodies-positive status between ID group and control group (OR: 1.24; 95% CI: 0.88, 1.74; P = 0.22; I2: 0%; Figure 3G).
Discussion
Iron is an essential element for the human system and ID has been a global health problem. The World Health Organization reports that the global prevalence of anemia for reproductive-age and pregnant women is 29.4% and 38.2%, respectively, and the main reason for anemia is ID (31). ID has adverse effects on both thyroid function and autoimmunity. Studies have shown that ID can reduce the conversion of T4 to T3 by interfering the activity of thyroxine deiodinase and also regulate thyroid metabolism through the central nervous system (32, 33). Severe ID will reduce thyroid peroxidase activity and interfere with thyroid hormone synthesis (34). ID or iron deficiency anemia during pregnancy has been reported to be associated with preeclampsia, fetal growth restriction, and low birth weight (35–38).
We tried to find evidences of the adverse effects of ID on thyroid function and autoimmunity through a meta-analysis. We divided the population into pregnant and reproductive-age groups. For women of reproductive age, ID could significantly increase the risk of positive TPOAb and both positive TPOAb and TgAb. Studies have shown that increased TPOAb and TgAb can increase the risk of miscarriage, premature rupture of fetal membrane, gestational hypothyroidism, and postpartum thyroiditis, even when the thyroid function is normal (39–42). During pregnancy, the mother’s demand for iron increases to expand women’s red blood cell content and ensure adequate iron supply for the growth of the fetus (43). Therefore, pregnant women as well as reproductive-age women are suggested to take adequate iron supplementation. The meta-analysis of pregnant women showed that compared with iron sufficient, pregnant women with ID had increased serum TSH levels, and decreased FT4 levels. Obviously, the prevalence of overt and subclinical hypothyroidism in pregnant women with ID was significantly increased. Maternal thyroid hormone levels are very important in the first trimester of gestation, because fetal thyroid hormone production is not self- sufficient until 18–20 weeks of gestation (44, 45). Compared with the control group, there were no statistical differences in the prevalence of TPOAb and TgAb in pregnant women with ID. However, we still see the potential harm of ID to thyroid autoimmunity from the subgroup analysis of TPOAb based on the different diagnosis criteria for ID.
ID is the feature of some other diseases such as celiac disease, atrophic gastritis, and H. Pylori infection (46). It’s noteworthy that celiac disease has been confirmed to be closely related to autoimmune thyroiditis (46). Therefore, the identification and treatment of diseases behind ID that may cause abnormal thyroid function cannot be ignored as well. Studies showed that co-presence of non-endocrine autoimmune disorders could increase the risk of recurrent pregnancy loss (47), suggesting that the negative effect of ID during pregnancy should be mentioned even in the frame of poly-autoimmunity.
The current article summarized and evaluated the effects of ID on thyroid function and autoimmunity using published studies. There are still some limitations in our article. Since some studies did not group the population according to whether with ID or not, we could not successfully extract relevant data. In addition, our inability to unify the time taken by different research teams for sampling and diagnosing diseases may have caused some differences in analyses. At the same time, we did not include studies in languages other than English, nor did we analyze them. Finally, most studies included in the current analysis were from China, but thyroid disorder prevalence and ID prevalence varied widely based on locale, which might also be a limitation.
In a conclusion, ID may adversely affect the thyroid function and autoimmunity of pregnant women and women of reproductive age and it is very necessary for monitoring iron nutritional status and early treatment of ID for them. Researchers from different regions were expected to pay more attention to the relationship between ID and thyroid disorders, so that the evidences in this field would be more comprehensive.
Data Availability Statement
The original contributions presented in the study are included in the article/Supplementary Material. Further inquiries can be directed to the corresponding authors.
Author Contributions
LG, LY, and JL conceived and designed the research. JL and XW searched articles and extracted data. JL and LG were responsible for data analyses and writing the manuscript. All authors contributed to the article and approved the submitted version.
Funding
The study was funded by Natural Science Foundation of China (Grant No. 81670763 and 81471050).
Conflict of Interest
The authors declare that the research was conducted in the absence of any commercial or financial relationships that could be construed as a potential conflict of interest.
Acknowledgments
We are grateful to all authors for their careful work and good cooperation.
Supplementary Material
The Supplementary Material for this article can be found online at: https://www.frontiersin.org/articles/10.3389/fendo.2021.629831/full#supplementary-material
References
1. Ge GM, Leung MTY, Man KKC, Leung WC, Ip P, Li GHY, et al. Maternal Thyroid Dysfunction During Pregnancy and the Risk of Adverse Outcomes in the Offspring: A Systematic Review and Meta-Analysis. J Clin Endocrinol Metab (2020) 105(12):3821–41. doi: 10.1210/clinem/dgaa555
2. Korevaar TIM, Derakhshan A, Taylor PN, Meima M, Chen L, Bliddal S, et al. Association of Thyroid Function Test Abnormalities and Thyroid Autoimmunity With Preterm Birth: A Systematic Review and Meta-analysis. Jama (2019) 322(7):632–41. doi: 10.1001/jama.2019.10931
3. Gietka-Czernel M, Glinicki P. Subclinical hypothyroidism in pregnancy: controversies on diagnosis and treatment. Pol Arch Internal Med (2020). doi: 10.20452/pamw.15626
4. Jansen TA, Korevaar TIM, Mulder TA, White T, Muetzel RL, Peeters RP, et al. Maternal thyroid function during pregnancy and child brain morphology: a time window-specific analysis of a prospective cohort. Lancet Diabetes Endocrinol (2019) 7(8):629–37. doi: 10.1016/s2213-8587(19)30153-6
5. Liu X, Andersen SL, Olsen J, Agerbo E, Schlünssen V, Dharmage SC, et al. Maternal hypothyroidism in the perinatal period and childhood asthma in the offspring. Allergy (2018) 73(4):932–9. doi: 10.1111/all.13365
6. Minaldi E, D’Andrea S, Castellini C, Martorella A, Francavilla F, Francavilla S, et al. Thyroid autoimmunity and risk of post-partum depression: a systematic review and meta-analysis of longitudinal studies. J Endocrinol Invest (2020) 43(3):271–7. doi: 10.1007/s40618-019-01120-8
7. Negro R, Schwartz A, Gismondi R, Tinelli A, Mangieri T, Stagnaro-Green A. Thyroid antibody positivity in the first trimester of pregnancy is associated with negative pregnancy outcomes. J Clin Endocrinol Metab (2011) 96(6):E920–4. doi: 10.1210/jc.2011-0026
8. Ponikowski P, Kirwan BA, Anker SD, McDonagh T, Dorobantu M, Drozdz J, et al. Ferric carboxymaltose for iron deficiency at discharge after acute heart failure: a multicentre, double-blind, randomised, controlled trial. Lancet (London England) (2020) 396(10266):1895–904. doi: 10.1016/s0140-6736(20)32339-4
9. Zhang HY, Teng XC, Shan ZY, Wang ZJ, Li CY, Yu XH, et al. Association between iron deficiency and prevalence of thyroid autoimmunity in pregnant and non-pregnant women of reproductive age: a cross-sectional study. Chin Med J (2019) 132(18):2143–9. doi: 10.1097/cm9.0000000000000409
10. He L, Shen C, Zhang Y, Chen Z, Ding H, Liu J, et al. Evaluation of serum ferritin and thyroid function in the second trimester of pregnancy. Endocr J (2018) 65(1):75–82. doi: 10.1507/endocrj.EJ17-0253
11. Surks MI. Effect of thyrotropin on thyroidal iodine metabolism during hypoxia. Am J Physiol (1969) 216(2):436–9. doi: 10.1152/ajplegacy.1969.216.2.436
12. Hess SY, Zimmermann MB, Arnold M, Langhans W, Hurrell RF. Iron deficiency anemia reduces thyroid peroxidase activity in rats. J Nutr (2002) 132(7):1951–5. doi: 10.1093/jn/132.7.1951
13. Okuroglu N, Ozturk A, Özdemir A. Is Iron Deficiency A Risk Factor For The Development Of Thyroid Autoantibodies In Euthyroid Women With Reproductive Ages? Acta Endocrinol (Bucharest Romania 2005) (2020) 16(1):49–52. doi: 10.4183/aeb.2020.49
14. Veltri F, Decaillet S, Kleynen P, Grabczan L, Belhomme J, Rozenberg S, et al. Prevalence of thyroid autoimmunity and dysfunction in women with iron deficiency during early pregnancy: is it altered? Eur J Endocrinol (2016) 175(3):191–9. doi: 10.1530/eje-16-0288
15. Yu X, Shan Z, Li C, Mao J, Wang W, Xie X, et al. Iron deficiency, an independent risk factor for isolated hypothyroxinemia in pregnant and nonpregnant women of reproductive age in China. J Clin Endocrinol Metab (2015) 100(4):1594–601. doi: 10.1210/jc.2014-3887
16. Moher D, Shamseer L, Clarke M, Ghersi D, Liberati A, Petticrew M, et al. Preferred reporting items for systematic review and meta-analysis protocols (PRISMA-P) 2015 statement. Syst Rev (2015) 4(1):1. doi: 10.1186/2046-4053-4-1
17. Hu J, Dong Y, Chen X, Liu Y, Ma D, Liu X, et al. Prevalence of suicide attempts among Chinese adolescents: A meta-analysis of cross-sectional studies. Compr Psychiatry (2015) 61:78–89. doi: 10.1016/j.comppsych.2015.05.001
18. Luo D, Wan X, Liu J, Tong T. Optimally estimating the sample mean from the sample size, median, mid-range, and/or mid-quartile range. Stat Methods Med Res (2018) 27(6):1785–805. doi: 10.1177/0962280216669183
19. Wan X, Wang W, Liu J, Tong T. Estimating the sample mean and standard deviation from the sample size, median, range and/or interquartile range. BMC Med Res Method (2014) 14:135. doi: 10.1186/1471-2288-14-135
20. Iqbal S, Rust P, Weitensfelder L, Ali I, Kundi M, Moshammer H, et al. Iron and Iodine Status in Pregnant Women from A Developing Country and Its Relation to Pregnancy Outcomes. Int J Environ Res Public Health (2019) 16(22):4414. doi: 10.3390/ijerph16224414
21. Rosario PW, Oliveira LFF, Calsolari MR. Maternal hypothyroxinemia in the first trimester of gestation and association with obstetric and neonatal outcomes and iron deficiency: a prospective Brazilian study. Arch Endocrinol Metab (2018) 62(3):332–6. doi: 10.20945/2359-3997000000043
22. Sitoris G, Veltri F, Kleynen P, Belhomme J, Rozenberg S, Poppe K. Screening for Thyroid Dysfunction in Pregnancy With Targeted High-Risk Case Finding: Can It Be Improved? J Clin Endocrinol Metab (2019) 104(6):2346–54. doi: 10.1210/jc.2018-02303
23. Joshi K, Nair S, Khade C, Rajan MG. Early gestation screening of pregnant women for iodine deficiency disorders and iron deficiency in urban centre in Vadodara, Gujarat, India. J Dev Origins Health Dis (2014) 5(1):63–8. doi: 10.1017/s2040174413000470
24. Zimmermann MB, Burgi H, Hurrell RF. Iron deficiency predicts poor maternal thyroid status during pregnancy. J Clin Endocrinol Metab (2007) 92(9):3436–40. doi: 10.1210/jc.2007-1082
25. Larsson A, Palm M, Hansson LO, Axelsson O. Reference values for clinical chemistry tests during normal pregnancy. BJOG (2008) 115(7):874–81. doi: 10.1111/j.1471-0528.2008.01709.x
26. Refaat B. Prevalence of pregnancy induced thyroid dysfunction and the characteristics of the associated anaemia in primigravida Saudi women during the first trimester: A cross-sectional study. Gazz Med Ital - Arch Sci Med (2014) 173:1–2. doi: 10.4103/2319-4170.151032
27. Li S, Gao X, Wei Y, Zhu G, Yang C. The Relationship between Iron Deficiency and Thyroid Function in Chinese Women during Early Pregnancy. J Nutr Sci Vitaminol (2016) 62(6):397–401. doi: 10.3177/jnsv.62.397
28. Fu J, Yang A, Zhao J, Zhu Y, Gu Y, Xu Y, et al. The relationship between iron level and thyroid function during the first trimester of pregnancy: A cross-sectional study in Wuxi, China. J Trace Elem Med Biol (2017) 43:148–52. doi: 10.1016/j.jtemb.2017.01.004
29. Teng X, Shan Z, Li C, Yu X, Mao J, Wang W, et al. Iron Deficiency May Predict Greater Risk for Hypothyroxinemia: A Retrospective Cohort Study of Pregnant Women in China. Thyroid (2018) 28(8):968–75. doi: 10.1089/thy.2017.0491
30. Zhang Y, Huang X, Chen Z, Yang Q, Li X, Zhang R, et al. Iron deficiency, a risk factor for thyroid autoimmunity during secomd trimester of pregnancy in China. Endocr Pract (2020) 26(6):595–603. doi: 10.4158/ep-2019-0220
31. Grabowski MK, Gray RH, Makumbi F, Kagaayi J, Redd AD, Kigozi G, et al. The global prevalence of anaemia in 2011. Geneva, Switzerland:WHO, 2015 (2011) 126(11), 5409–18. doi: 10.1016/S2214-109X(15)00086-8
32. Brigham DE, Beard JL. Effect of thyroid hormone replacement in iron-deficient rats. Am J Physiol (1995) 269(5 Pt 2):R1140–7. doi: 10.1152/ajpregu.1995.269.5.R1140
33. Beard JL, Brigham DE, Kelley SK, Green MH. Plasma thyroid hormone kinetics are altered in iron-deficient rats. J Nutr (1998) 128(8):1401–8. doi: 10.1093/jn/128.8.1401
34. Eftekhari MH, Simondon KB, Jalali M, Keshavarz SA, Elguero E, Eshraghian MR, et al. Effects of administration of iron, iodine and simultaneous iron-plus-iodine on the thyroid hormone profile in iron-deficient adolescent Iranian girls. Eur J Clin Nutr (2006) 60(4):545–52. doi: 10.1038/sj.ejcn.1602349
35. Mahmood T, Rehman AU, Tserenpil G, Siddiqui F, Ahmed M, Siraj F, et al. The Association between Iron-deficiency Anemia and Adverse Pregnancy Outcomes: A Retrospective Report from Pakistan. Cureus (2019) 11(10):e5854. doi: 10.7759/cureus.5854
36. Georgieff MK. Iron deficiency in pregnancy. Am J Obstetrics Gynecol (2020) 223(4):516–24. doi: 10.1016/j.ajog.2020.03.006
37. Ribot B, Aranda N, Viteri F, Hernández-Martínez C, Canals J, Arija V. Depleted iron stores without anaemia early in pregnancy carries increased risk of lower birthweight even when supplemented daily with moderate iron. Hum Reprod (Oxford England) (2012) 27(5):1260–6. doi: 10.1093/humrep/des026
38. Hernández-Martínez C, Canals J, Aranda N, Ribot B, Escribano J, Arija V. Effects of iron deficiency on neonatal behavior at different stages of pregnancy. Early Hum Dev (2011) 87(3):165–9. doi: 10.1016/j.earlhumdev.2010.12.006
39. Dhillon-Smith RK, Middleton LJ, Sunner KK, Cheed V, Baker K, Farrell-Carver S, et al. Levothyroxine in Women with Thyroid Peroxidase Antibodies before Conception. N Engl J Med (2019) 380(14):1316–25. doi: 10.1056/NEJMoa1812537
40. Chen LM, Zhang Q, Si GX, Chen QS, Ye EL, Yu LC, et al. Associations between thyroid autoantibody status and abnormal pregnancy outcomes in euthyroid women. Endocrine (2015) 48(3):924–8. doi: 10.1007/s12020-014-0420-x
41. Springer D, Jiskra J, Limanova Z, Zima T, Potlukova E. Thyroid in pregnancy: From physiology to screening. Crit Rev Clin Lab Sci (2017) 54(2):102–16. doi: 10.1080/10408363.2016.1269309
42. Benvenga S. Targeted Antenatal Screening for Predicting Postpartum Thyroiditis and Its Evolution Into Permanent Hypothyroidism. Front Endocrinol (2020) 11:220. doi: 10.3389/fendo.2020.00220
43. Milman N, Taylor CL, Merkel J, Brannon PM. Iron status in pregnant women and women of reproductive age in Europe. Am J Clin Nutr (2017) 106(Suppl 6):1655s–62s. doi: 10.3945/ajcn.117.156000
44. de Zegher F, Pernasetti F, Vanhole C, Devlieger H, Van den Berghe G, Martial JA. The prenatal role of thyroid hormone evidenced by fetomaternal Pit-1 deficiency. J Clin Endocrinol Metab (1995) 80(11):3127–30. doi: 10.1210/jcem.80.11.7593413
45. Vulsma T, Gons MH, de Vijlder JJ. Maternal-fetal transfer of thyroxine in congenital hypothyroidism due to a total organification defect or thyroid agenesis. N Engl J Med (1989) 321(1):13–6. doi: 10.1056/nejm198907063210103
46. Virili C, Antonelli A, Santaguida MG, Benvenga S, Centanni M. Gastrointestinal Malabsorption of Thyroxine. Endocr Rev (2019) 40(1):118–36. doi: 10.1210/er.2018-00168
Keywords: iron deficiency, thyroid function, pregnant, reproductive-age, thyroid autoantibody
Citation: Luo J, Wang X, Yuan L and Guo L (2021) Iron Deficiency, a Risk Factor of Thyroid Disorders in Reproductive-Age and Pregnant Women: A Systematic Review and Meta-Analysis. Front. Endocrinol. 12:629831. doi: 10.3389/fendo.2021.629831
Received: 16 November 2020; Accepted: 21 January 2021;
Published: 25 February 2021.
Edited by:
Alex Stewart Stagnaro-Green, University of Illinois at Chicago, United StatesReviewed by:
Allan Chen Dong, Advocate Lutheran General Hospital, United StatesMarco Centanni, Sapienza University of Rome, Italy
Sima Nazarpour, Islamic Azad University, Varamin, Iran
Copyright © 2021 Luo, Wang, Yuan and Guo. This is an open-access article distributed under the terms of the Creative Commons Attribution License (CC BY). The use, distribution or reproduction in other forums is permitted, provided the original author(s) and the copyright owner(s) are credited and that the original publication in this journal is cited, in accordance with accepted academic practice. No use, distribution or reproduction is permitted which does not comply with these terms.
*Correspondence: Lixin Guo, Z2x4d29yazIwMTZAMTYzLmNvbQ==; Li Yuan, eXVhbmxpQHVjYXMuYWMuY24=