- 1Department of Medicine and Health Sciences “Vincenzo Tiberio”, University of Molise, Campobasso, Italy
- 2School of Health and Life Sciences, University of the West of Scotland, Hamilton, United Kingdom
- 3Section of Human Anatomy, Department of Mental and Physical Health and Preventive Medicine, University of Campania “Luigi Vanvitelli”, Naples, Italy
- 4Department of Translational Medical Sciences, University of Naples “Federico II”, Naples, Italy
- 5Department of Advanced Biomedical Sciences, University of Naples “Federico II”, Naples, Italy
- 6Istituti Clinici Scientifici Maugeri SpA Società Benefit (ICS Maugeri SpA SB), Telese Terme, Italy
Background: Sarcopenia is a progressive loss of skeletal muscle mass whose pathophysiology has been proposed to possibly involve mechanisms of altered inflammatory status and endocrine function. Adiponectin has been shown to modulate inflammatory status and muscle metabolism. However, the possible association between adiponectin levels and sarcopenia is poorly understood. In order to fill this gap, in the present manuscript we aimed to summarize the current evidence with a systematic review and a meta-analysis of studies reporting serum adiponectin levels in patients with sarcopenia compared to non-sarcopenic controls.
Methods: An electronic search through Medline/PubMed, Cochrane Library, and Science Direct was performed till March 1, 2020. From the included papers, meta-analysis of cross-sectional studies comparing serum levels of adiponectin between patients with sarcopenia and controls was performed.
Results: Out of 1,370 initial studies, seven studies were meta-analyzed. Sarcopenic participants had significantly higher levels of adiponectin Hedges’ g with 95% confidence interval (CI): 1.20 (0.19–2.22), p = 0.02 than controls. Subgroup analysis, performed in Asian population and focused on identification of the condition based on AWGS criteria, reported higher adiponectin levels in sarcopenic population (2.1 (0.17–4.03), p = 0.03 and I2 = 98.98%. Meta-regression analysis revealed female gender to significantly influence the results as demonstrated by beta = 0.14 (95% CI (0.010–0.280), p = 0.040).
Conclusions: Our meta-analysis found evidence that sarcopenia is associated with higher adiponectin levels. However, caution is warranted on the interpretation of these findings, and future longitudinal research is required to disentangle and better understand the topic.
Introduction
Sarcopenia is a disease of massive concern in clinical epidemiology as a result of its rising prevalence inherently associated with the aging trends and the increasing elderly populations all over the world. It is common in men and women, with a prevalence of about 10% in those aged >65 years. Sarcopenia is clinically defined as a progressive and generalized musculoskeletal disease associated with increased likelihood of adverse health and functional outcomes including falls, fractures, physical disability and mortality (1). A few factors are recognized as possible pathophysiological mechanisms of sarcopenia and concurrent muscle loss, including among others neuromuscular aging, malnutrition, insufficient physical activity, alternations of inflammatory status and endocrine function (2, 3). The degenerative effects of malnutrition and insufficient physical activity on the neuromuscular function and functional capacity of the musculoskeletal system during aging have been object of extensive investigations, and are routinely acted upon to preserve muscle function, muscular strength, and muscle power for the health and wellbeing of older adults. Conversely, less and inconclusive evidence is available to elucidate the mediating role endocrine and inflammation homeostases have for the onset and degeneration of sarcopenia. Adiponectin is the most abundantly expressed human body adipokine, predominantly produced by bone marrow adipose tissue, that mediates inflammatory processes by inhibiting the synthesis of IL-6, IL-18, TNF-α, and blocking the activation NF-κB (4, 5). Furthermore, adiponectin plays a protective role in cardiovascular health by inhibiting foam cell formation and adhesion molecule expression (6) and facilitating the systemic insulin sensitivity (7). Recent studies have revealed that adiponectin signaling activation may have a protective role against muscle atrophy through binding to T-cadherin and consequently promoting muscle regeneration (8, 9). Importantly, the beneficial effects of physical exercise on muscle metabolism are also mediated via adiponectin signaling (10). In view of the effective role of physical activity in the prevention and treatment of sarcopenia (11, 12), clarifying the underpinning mechanisms in which adiponectin signaling concurs seems prudent. In fact, previous studies examining adiponectin circulating levels in sarcopenic population have reported contrasting results (13). This may be due to different clinical settings, population characteristics or, different tools used for sarcopenia definition.
Diagnosis and monitoring of sarcopenia is based on clinical, functional, and imaging evaluations. Muscle mass imaging assessments using magnetic resonance imaging (MRI), computed tomography (CT), or dual-energy x-ray absorptiometry (DXA) scan are validated methods for assessing muscle mass, but they are burdened by intrinsic limitations and high cost that confine their use to research activity rather than clinical practice. Physical performance assessment consists of standardized and low-cost tests, with relevant ability to predict the development of disability. Nevertheless, in clinical practice, their application may be altered by specific conditions or comorbidities, whose prevalence is higher in elderly (14).
Circulating adiponectin level measurements only require collection of blood samples and laboratory techniques, as enzyme linked immunosorbent assay (ELISA), which represents a valid widespread and low-cost method. It has been reported that circulating adiponectin levels are negatively associated with physical performance in adult population (15). Accordingly, exercise training and dietary interventions have been shown to modulate adiponectin levels, as emerged by cross-sectional studies and randomized controlled trials reporting that aerobic and resistance exercise training may influence the adipokine levels (16–18). Low protein intake enhanced adiponectin expression in experimental models (19); of note, a clinical trial focused on the effects of low caloric diet with different protein contents on adiponectin profile, showing that 35% protein diet regimen significantly modified adiponectin levels (20). Lifestyle interventions as resistance exercise training and adequate nutritional intake are the current approaches for the management and treatment of sarcopenia. In this context, adiponectin would be helpful to follow sarcopenia progression, also allowing to monitor response to treatment.
Therefore, we conducted a systematic review and meta-analysis of the studies that compared adiponectin levels between sarcopenic and non-sarcopenic subjects as to evaluate the association between adiponectin level and sarcopenia. In addition, we investigated whether this association is influenced by sarcopenia definition, tools used for its diagnosis, or population characteristics.
Materials and Methods
This systematic review was performed according to the Strengthening the Reporting of Observational Studies in Epidemiology (STROBE) (21) criteria and the recommendations in the Preferred Reporting Items for Systematic Reviews and Meta-Analyses (PRISMA) statement (22). The protocol has been registered in Prospero database (registration ID: CRD42020176530).
Data Sources and Searches
Two reviewers performed a systematic search from date of inception to 1 of march 2020 using four electronic databases: Medline/PubMed, Cochrane Library and Science Direct. Potentially relevant studies were subsequently screened by full text reading, independently by two reviewers. A third reviewer resolved any possible disagreement by discussion and reaching a consensus. Combination of the following free text terms and major medical subject headings adapted to the requirements of each database were used: “adiponectin,” “AdipoQ,” “ACRP30,” “sarcopenia,” “muscle mass.” Boolean Logical Operators AND, OR were used. As an example Medline/PubMed search: (“adiponectin”[MeSH Terms] OR “adiponectin”[All Fields] OR “adiponectin s”[All Fields] OR “adiponectine”[All Fields] OR “adiponectins”[All Fields] OR “AdipoQ”[All Fields] OR “ACRP30”[All Fields]) AND (“sarcopenia”[MeSH Terms] OR “sarcopenia”[All Fields]); (“adiponectin”[MeSH Terms] OR “adiponectin”[All Fields] OR “adiponectin s”[All Fields] OR “adiponectine”[All Fields] OR “adiponectins”[All Fields] OR “AdipoQ”[All Fields] OR “ACRP30”[All Fields]) AND ((“muscle s”[All Fields] OR “muscles”[MeSH Terms] OR “muscles”[All Fields] OR “muscle”[All Fields]) AND (“molecular weight”[MeSH Terms] OR (“molecular”[All Fields] AND “weight”[All Fields]) OR “molecular weight”[All Fields] OR “mass”[All Fields])) An additional reviewer was involved in the selection process, with particular task of solving conflicting opinions cases. Reference lists of retrieved papers and review articles were hand-searched for additional relevant citations. Only peer-reviewed articles published in English were included in the meta-analysis.
Study Selection
The studies initially obtained using the search strategy were assessed for eligibility by screening titles and abstracts, independently by two authors. Potentially relevant studies were subsequently screened by full text reading, independently by two reviewers and a third reviewer, which also resolved any possible disagreement by discussion and reaching a consensus. Studies included in the systematic review met the following inclusion criteria: a) diagnosis of sarcopenia based on European Working Group on Sarcopenia in Older People (EWGSOP), Asian Working Group for Sarcopenia (AWGS) (1, 23), consensuses, or any other definition used by original studies’ author; b) serum or plasmatic levels of adiponectin reported; c) included a control group (non-sarcopenic population). Studies were excluded if: a) not clear diagnosis of sarcopenia was reported; b) animal or in vitro studies; c) no report or measure of quantitative adiponectin levels was reported.
Data Extraction and Quality Assessment
Two reviewers independently extracted the following variables from the included studies: first author’s name, year of publication, total number of individuals included in the study, study design, mean or median age of individuals, mean or median Body Mass Index (BMI), percentage of males population, continent, applied definition(s) of sarcopenia, method used for sarcopenia identification, mean muscle mass and physical performance in sarcopenia and non-sarcopenia group, mean or median adiponectin levels in sarcopenia and non-sarcopenia population. Risk of bias of the included studies was assessed using the Newcastle Ottawa Scale (NOS) (24) for case–control and cohort studies and a modified version of the NOS for cross- sectional studies. A system of points was given to the eligible categories: (I) selection of the study population, (II) comparability, and (III) description of the outcome. A study was given a maximum of one point in each item within the Selection and Outcome categories and a maximum of two points was given for the Comparability category. The scale scores varied depending on the study design. For case–control and cohort studies, it ranged from 0 to 9 points with ≥7 points classified as high quality. For cross-sectional studies, it ranged from 0 to 7 points. A median of ≥4 points was considered as high quality for cross-sectional studies as described by other authors (25, 26).
Statistical Analysis
Analyses were performed using STATA SE 16.1. Adiponectin level was meta-analyzed when at least three studies contributed data. Hedges’ g was used for standardized mean differences calculation. Calculations of mean and standard deviation (SD) for different groups were performed according to Cochrane Handbook for Systematic Reviews of Interventions (27). As necessary estimation of mean and SD was calculated from sample size and inter quartile range (IQR) (28). Study heterogeneity was measured using the chi2 and I2 statistics (29). I2 values greater than 25% were considered to reflect low heterogeneity, 50% moderate, and 75% high heterogeneity. A random statistical analysis model was used. Given significant heterogeneity, a meta-regression analysis was performed using differences in mean age, BMI and percentage of female population as moderators. Moreover, subgroup analysis was conducted considering diagnosis of sarcopenia according to the definition of AWGS, and diagnostic methods used for sarcopenia confirmation such as (DXA) or Bioelectrical Impedance Analysis (BIA). Forest plots were used to visualize the results. Funnel plots were used initially to evaluate visually publication bias while Egger’s regression test and Begg’s test were used to inferentially evaluate publication bias (30, 31).
Results
Search Results
A total of 1370 studies were retrieved through electronic database searches and cross-references search. After removal of duplicates, 982 studies were identified for title and abstract screening. Review of the titles and abstracts yielded 52 relevant studies for full text screening. From these, seven studies were conducted on animal or in vitro models, 28 studies did not report quantitative expression of adiponectin level in sarcopenic and non-sarcopenic subjects, and in other 10 studies the reported diagnostic criteria for sarcopenia were not clear or absent. Finally, we included seven studies in the meta-analysis. Figure 1 shows the study flow-chart and searching results. Supplemental Table 2, reports the 982 screened articles with details regarding full-text reading or only title or abstract screening.
Study Characteristics
Study characteristics are described in Table 1. A total of 1,389 individuals, with a mean age ranging from 40.3 to 80. 2 years were included, and sample size ranged from 77 to 539 individuals. Six studies presented cross-sectional design (32–34, 36–38), while one study reported retrospective data (35). Five studies included community-dwelling individuals, while one study hospitalized patients prior to cardiac surgery and one study included data from both hospitalized and geriatric outpatients. Four studies used DXA to identify sarcopenia while two studies identified sarcopenia through BIA. The most common definition of sarcopenia was AWGS. Included studies reported serum measurements of adiponectin through ELISA or radioimmunoassay.
Adiponectin Expression and Meta-analysis Findings
Meta-analysis of seven included studies, revealed that individuals with sarcopenia (n= 557), compared to individuals without sarcopenia (n=832), were more likely to have significantly higher adiponectin levels: (Hedges’ g with 95% confidence interval [CI], 1.20; 0.19–2.22; p = 0.02). The studies were characterized by high heterogeneity (I2 = 98.36%, p < 0.0001) (Figure 2).
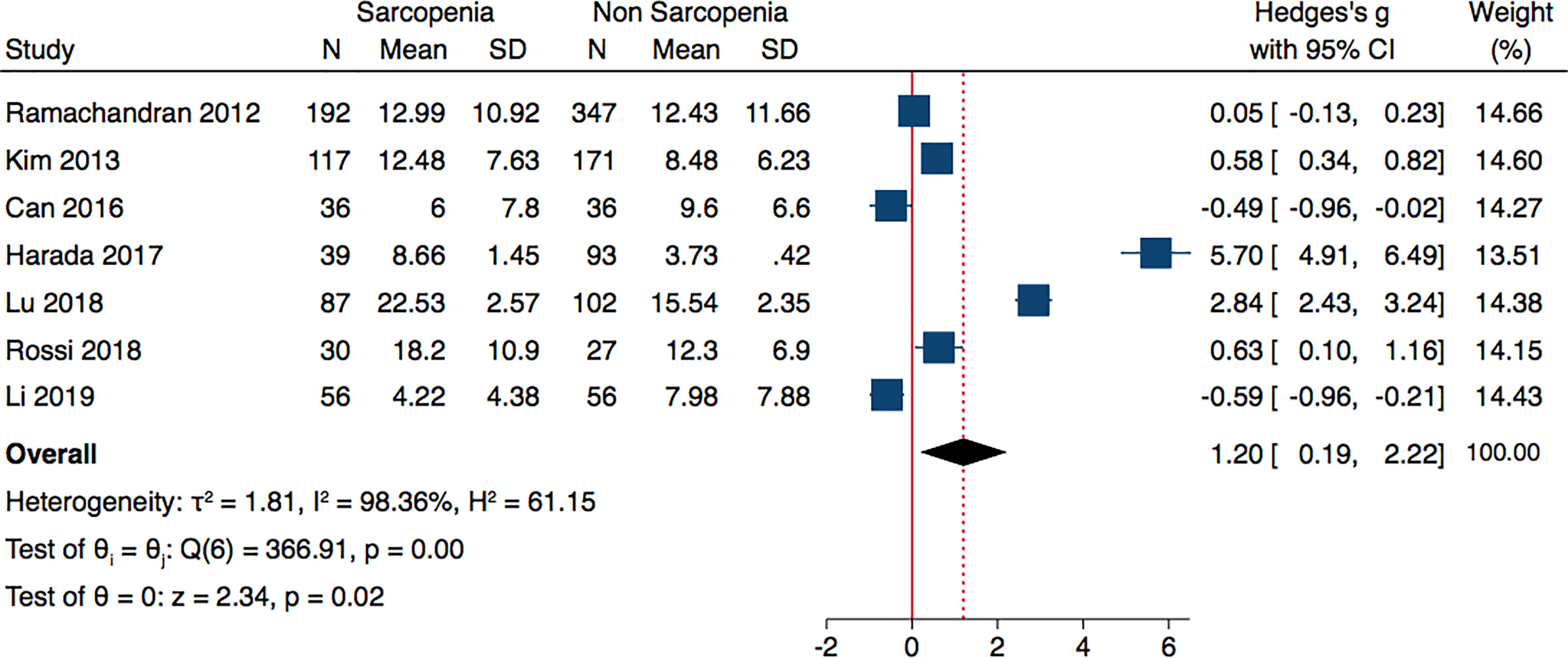
Figure 2 Forest plot of serum adiponectin levels in sarcopenic vs. no sarcopenic subjects. SD, standard deviation; CI, confidence interval.
Analyzing the data of four studies performed in Asian population and following AWGS criteria, sarcopenic individuals were characterized by higher levels of adiponectin: (Hedges’ g with 2.1; 0.17–4.03; p = 0.03 and I2 = 98.98%) (Figure 3). The subgroup analysis of studies based on DXA evaluation of sarcopenia, did not report significantly different adiponectin levels (Hedges’ g with 95% CI, 0.70; −0.22 to 1.61; p = 0.13; I2 = 97.83) (Figure 4).
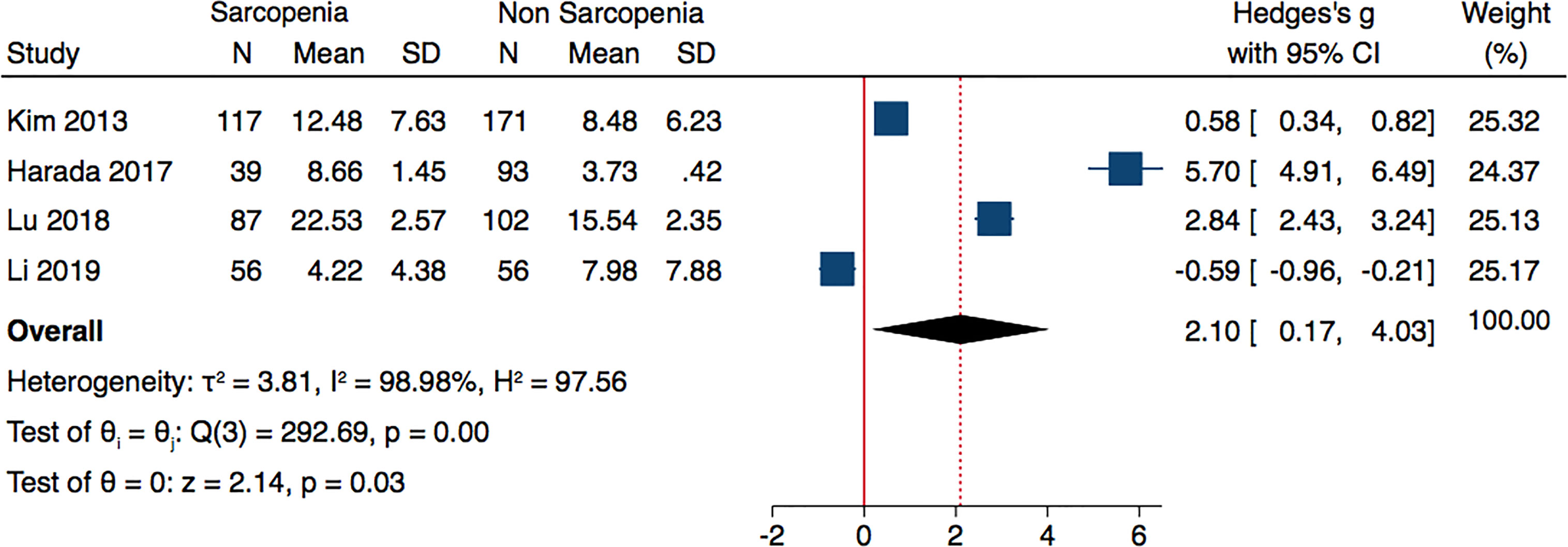
Figure 3 Forest plot of serum adiponectin levels in sarcopenic vs. no sarcopenic subjects. Studies performed on Asian population and or based on AWGS criteria. SD, standard deviation; CI, confidence interval.
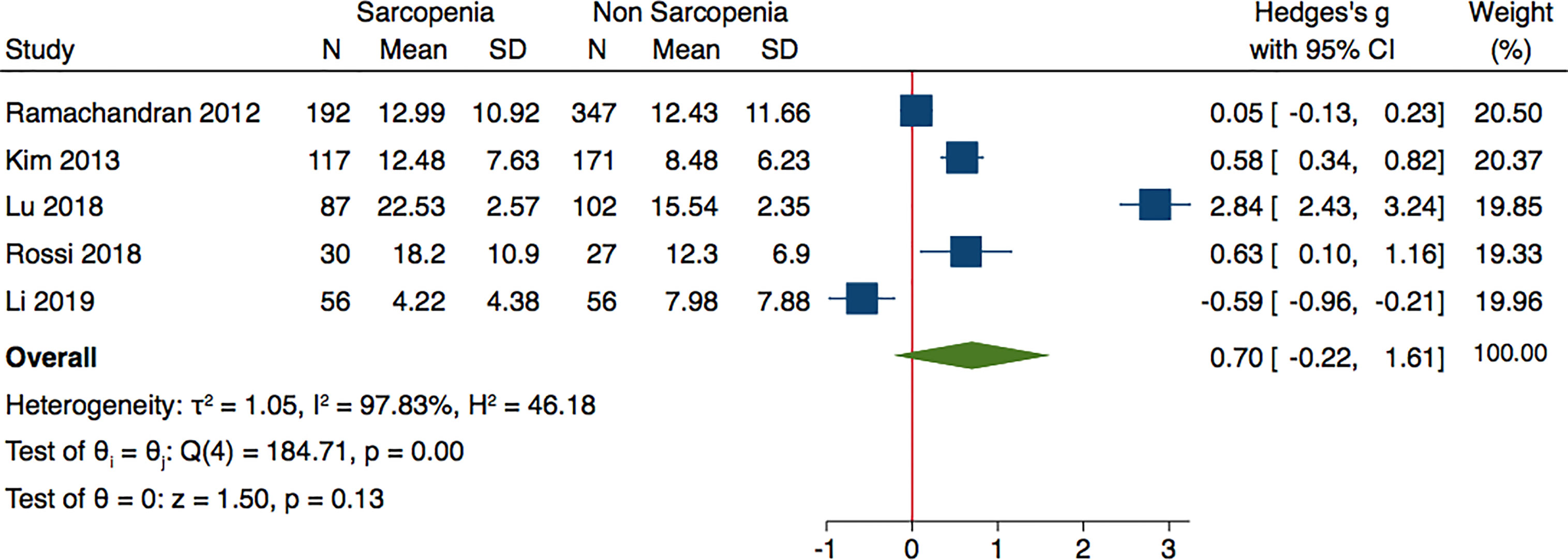
Figure 4 Forest plot of serum adiponectin levels in sarcopenic vs. no sarcopenic subjects. Studies applying DXA. SD, standard deviation; CI, confidence interval.
Meta-Regression Analysis
Since the differences in adiponectin levels between sarcopenic and non-sarcopenic population were characterized by a high heterogeneity (I2 ≥ 50%, p < 0.05), we ran a meta-regression analysis to seek potential moderators, such as age, BMI differences and percentage of female population. While age and BMI did not affect the findings (beta = 0.11; 95% CI, −1.40 to 1.63; p = 0.842; beta = 0.85; 95% CI, −1.76 to 3.47; p =0.416), respectively, gender was found to moderate the results (beta = 0.14; 95% CI, 0.010–0.280; p=0.040).
Study Quality and Publication Bias
The quality of studies evaluated by NOS was good. Results of the NOS quality assessment of the included studies are reported in Supplemental Material Table 1. Asymmetry was observed by visual inspection of funnel plots (Supplemental Figures 1–3). However, Egger’s regression test (p = 0.133) for all studies (p = 0.122), for AWGS and Asian population studies, and (p = 0.652) for studies using DEXA indicated no significant publication bias among the studies in this meta-analysis.
Discussion
From the overall meta-analysis on 1,389 individuals, 557 sarcopenic and 832 controls, it emerged that sarcopenic individuals are characterized by significantly higher adiponectin levels. The difference regarding the prevalence of females in sarcopenia and non-sarcopenia population resulted as a significant moderator and may explain, at least in part the heterogeneity found in our results. It should be mentioned that the included studies did not always report the same relationship between sarcopenia and adiponectin, and different results were described even in studies following AWGS criteria and performed on Asian population or using DEXA as a diagnostic method. Many studies emphasize the role of adiponectin on skeletal muscle metabolism and function (39–42). In vitro studies performed on primary human and animal skeletal muscle cells reported that adiponectin increased glucose up-take in muscle cells and this action is mediated by the translocation of the glucose transporter GLUT4 to the cell surface (43). Furthermore, adiponectin stimulates the interaction of adaptor protein containing pleckstrin homology domain (APPL1) with adiponectin receptors AdipoR1 and AdipoR2, leading to increased GLUT4 membrane translocation, and knock down of APPL1 resulted in significant reduction of glucose uptake and insulin sensitivity (44). At least in young individuals, insulin inhibits protein catabolism in muscle (45), and muscle accretion is mediated through activation of p38 MAPK signaling. The delivery of amino acids is related to enhanced perfusion which is impaired in aged muscle (46). With aging, insulin sensitivity decreases, whereas preservation of glucose homeostasis and insulin sensitivity is associated to longevity (47). Moreover, mice with fat-specific disruption of the insulin receptor gene (FIRKO) are characterized by lower fasting insulin levels, elevated serum adiponectin levels and enhanced longevity (48). Human studies including centenarians, report increased levels of adiponectin and inverse correlation with homeostasis model assessment for insulin resistance (HOMA-IR) or inflammatory markers (49, 50).
On the other hand, age- related reduction of protein metabolism and reduction of insulin mediated suppression of proteolysis leads to development of anabolic resistance and sarcopenia. Of interest, reduced adiponectin levels has been associated to activation of muscle protein degradation as ubiquitin-proteasome proteolytic pathway mediated by insulin resistance (51).
In contrasts, studies focused on chronic heart failure patients, reveal that high adiponectin levels are associated to HF severity and increased mortality (52). A recent meta-analysis also reported that high adiponectin levels increased the risk for both cardiovascular and all cause mortality, but this effect was attenuated by adjustment for natriuretic peptides levels (53).
Inflammation is considered another key factor in the genesis of sarcopenia (3). The mechanisms underpinning sarcopenia and chronic inflammation include NF-kB and TNF-alfa activation as primary mediators and principal pathway, and are characterized by increased muscle protein imbalance, alternation of mitochondrial function, cell death or apoptosis, and restricted muscle repair and regeneration (54). In animal models of low inflammation grade, an inverse correlation was found between cytokin levels and muscle wasting degree (55). Increased circulating levels of IL-1, IL-6, CRP, and tumor necrosis factor-alpha (TNF-alfa) are described in the elderly (56). Furthermore, a recent meta-analysis study reported that sarcopenic subjects are characterized by significantly elevated inflammatory markers (57). The cross-talk between adiponectin and inflammatory signaling is discussed in abundance. It has been suggested that adiponectin attenuates the inflammatory responses to multiple stimuli by modulating signaling pathways in a variety of cells (58). In experimental models of caloric restricted and aged animals, it has been reported a reduced secretion of IL-6, increased insulin sensitivity, amelioration of physical performance and at least in part these modifications are related to enhanced expression and secretion of adiponectin (59). Restoring adiponectin levels via modification of gene expression resulted in reduced TNFα, IL-1β, and CD68 levels, greater expression regeneration proteins like myogenin, Myh3, Myh7, and larger muscle fibers (60).
In our meta-analysis study, increased levels of adiponectin were associated with sarcopenia. Li et al. (38) reported that lower adiponectin levels were associated with high sarcopenia risk, but a 12-week intensive lifestyle intervention program led to significant improvements in muscle mass, muscle strength, and gait speed. Moreover, adiponectin levels considerably increased post-intervention, even above the levels registered in non-sarcopenic elderly subjects. Similar results regarding adiponectin levels between sarcopenic and non-sarcopenic population was reported also by Can et al. (34). However, the studies Lu et al. (36), Rossi et al. (37), Kim (32), and Harada (33) found opposite results.
Although, increased adiponectin levels in sarcopenia appears to be controversial, some possible mechanisms have been proposed to explain the detected peculiarity: a) down-regulation of adiponectin receptor signaling (61) b) deposition of adipose tissue in muscles that may influence adiponectin expression (62) c) activation of catabolism related to the presence of other comorbidities (63).
Discrepancies in the current literature may also be dependent on the confounding effect of adiponectin resistance and the resultant rise of adiponectin with ageing. However, in our meta-regression analysis, age difference between sarcopenic and non-sarcopenic populations was not a significant moderator of the results. It is worth mentioning that in some of these studies, population was heterogenous regarding gender, and meta-regression analysis found that the difference in female gender prevalence between sarcopenic and non-sarcopenic groups was a significant moderator, which in turn may at least in part explain the high heterogeneity rescued. In the study by Harada et al. (33), among non-sarcopenic population female prevalence was about 28%, whereas in sarcopenic population around 66.7%. This was a retrospective study performed in a cardiovascular setting, including patients undergoing cardiovascular surgery, and the later presentation of cardiovascular diseases and the higher comorbidity burden among females may explain these differences (64). While Li et al. (36), in a cross-sectional study reported that the sarcopenic group is characterized by higher age, more female and higher percentage of diabetic subjects. It has also been reported that the prevalence of sarcopenia differs between men and women (65).
We could not perform addition analysis regarding cardiovascular risk factor such as diabetes or dyslipidemia that could modify the results as these data were not available. In addition, subgroup analysis, performed only on Asian population diagnosed according to AWGS criteria, also reported significantly higher adiponectin levels in sarcopenic population. Subgroup analysis of studies using DXA failed to report any significant difference regarding adiponectin levels; however, this may be explained by different populations characterizing the included studies. We could not perform any additional subgroup analysis since the number of studies performed in U.S or Europe were not adequate to perform a meta-analysis.
In a study performed on community dwelling, older adults were found to have a significant and independent association between circulating adiponectin and frailty status: higher adiponectin levels in frail elderly than in their non-frail counterparts (66). It should be reminded that poor physical functioning, weight loss, and low muscle mass are the crucial components of frailty in older adults. Baker et al. (67) also uncovered a significant relationship between high adiponectin and weight loss, low muscle mass, and low muscle density. Furthermore, in this study, adiponectin was substantially associated with increased risk of incident disability and all-cause mortality. These findings are in agreement with the results of our meta-analysis, which points to sarcopenia and the associated catabolic stress as the pathophysiological background causing upregulation of adiponectin as part of compensatory mechanisms against inflammation and oxidative stress (68).
Moreover, our meta-regression analysis suggests an important role of female gender in explaining the association between sarcopenia and adiponectin levels. This finding seems to be pertinent with the current literature, suggesting that women present higher adiponectin levels than men. Indeed, women express greater plasma adiponectin than men, independent of fat mass and BMI, and there has been suggestion that this relationship is in part influenced by sex hormones (69, 70).
In addition to general obesity, abdominal obesity has been reported as an independent predictor of lower circulating adiponectin levels (71, 72). Furthermore, higher adiponectin levels have been described in obese individuals with higher subcutaneous to visceral adipose tissue ratio, thus allowing to speculate on the role of adipose tissue distribution in adipokine release (73). Indeed, adipose tissue sampling in individuals undergoing abdominal elective surgery reported decreased adiponectin secretion from central adipose tissue, while subcutaneous adipose tissue secretion remained unchanged (74).
From our meta-regression analysis, BMI did not significantly influence the results, and additional data as waist circumference or trunk fat could not be included. However, upregulation of adiponectin expression in muscles of obese and diabetic mice has been previously observed (75); the assessment of metabolic or inflammatory profile of subjects suffering from low muscle mass and impaired physical function revealed a more significant association with insulin resistance and elevated levels of C-reactive protein, IL-1, and IL-6 in obese patients than in subjects with normal or reduced BMI (76, 77). It is plausible that sarcopenic obesity is characterized by distinct metabolic and inflammatory profiles and adiponectin expression in this category deserves further investigation.
Considering that the current meta-analysis is the first of this kind, our findings should be interpreted with caution for a few reasons. First and utmost, we observed high heterogeneity in all the cross-sectional analyses conducted. Nevertheless, the Meta-analysis of Observational Studies in Epidemiology (MOOSE) guidelines state that when analyzing observational data, heterogeneity is to be expected. Second, we were not able to investigate the effects of physical exercise or nutritional intervention strategies on adiponectin levels and the indirect impact on outcome measures in sarcopenic populations. However, previous meta-analyses reported significant modifications of adiponectin levels in obese or diabetic population following physical exercise intervention, which presume similar effects also among sarcopenic people (12, 78). Thus, future longitudinal research should seek to investigate if adiponectin could be a potential biomarker of sarcopenia identification and prediction.
Conclusions
In conclusion, our meta-analysis found evidence that sarcopenia is associated with higher adiponectin levels. However, caution on the interpretation of these findings are warranted and future longitudinal research is required to disentangle and better understand the relationships that emerged.
Data Availability Statement
The raw data supporting the conclusions of this article will be made available by the authors, without undue reservation.
Author Contributions
Conceptualization, KK and GG. Methodology, KK and AI. Software, KK and FP. Validation, AL, AR, and LB. Formal analysis, KK, AR, and LB. Investigation, KK and AI. Resources, KK and GG. Data curation, KK, FP, GG, and AR. Writing original draft preparation, AI, KK, and GG. Supervision, GG and GR. All authors contributed to the article and approved the submitted version.
Conflict of Interest
The authors declare that the research was conducted in the absence of any commercial or financial relationships that could be construed as a potential conflict of interest.
Acknowledgments
LB has been supported by a research grant provided by the CardioPaTh PhD program.
Supplementary Material
The Supplementary Material for this article can be found online at: https://www.frontiersin.org/articles/10.3389/fendo.2021.576619/full#supplementary-material
Supplementary Table 1 | NOS quality assessment of the included study.
Supplementary Figure 1 | Funnel plot of serum adiponectin levels in sarcopenic vs. no sarcopenic subjects.
Supplementary Figure 2 | Funnel plot of serum adiponectin levels in sarcopenic vs. no sarcopenic subjects. Studies performed on Asian population and or based on AWGS criteria.
Supplementary Figure 3 | Funnel plot of serum adiponectin levels in sarcopenic vs. no sarcopenic subjects. Studies applying DXA.
References
1. Cruz-Jentoft AJ, Bahat G, Bauer J, Boirie Y, Bruyère O, Cederholm T, et al. Sarcopenia: revised European consensus on definition and diagnosis. Age Ageing (2019) 48(1):16–31. doi: 10.1093/ageing/afy169
2. Robinson S, Cooper C, Aihie Sayer A. Nutrition and sarcopenia: a review of the evidence and implications for preventive strategies. J Aging Res (2012) 2012:510801. doi: 10.1155/2012/510801
3. Marzetti E, Calvani R, Tosato M, Cesari M, Di Bari M, Cherubini A, et al. Sarcopenia: an overview. Aging Clin Exp Res (2017) 29(1):11–7. doi: 10.1007/s40520-016-0704-5
4. Chandrasekar B, Boylston WH, Venkatachalam K, Webster NJ, Prabhu SD, Valente AJ. Adiponectin blocks interleukin-18-mediated endothelial cell death via APPL1-dependent AMP-activated protein kinase (AMPK) activation and IKK/NF-kappaB/PTEN suppression. J Biol Chem (2008) 283:24889–98. doi: 10.1074/jbc.M804236200
5. Corbi G, Polito R, Monaco ML, Cacciatore F, Scioli M, Ferrara N, et al. Adiponectin Expression and Genotypes in Italian People with Severe Obesity Undergone a Hypocaloric Diet and Physical Exercise Program. Nutrients (2019) 11(9):2195. doi: 10.3390/nu11092195
6. Zhu W, Cheng KK, Vanhoutte PM, Lam KS, Xu A. Vascular effects of adiponectin: molecular mechanisms and potential therapeutic intervention. Clin Sci (2008) 114:361–74. doi: 10.1042/CS20070347
7. Kim JY, van de Wall E, Laplante M, Azzara A, Trujillo ME, Hofmann SM, et al. Obesity-associated improvements in metabolic profile through expansion of adipose tissue. J Clin Invest (2007) 117:2621–37. doi: 10.1172/JCI31021
8. Singh AK, Shree S, Chattopadhyay S, Kumar S, Gurjar A, Kushwaha S, et al. Small molecule adiponectin receptor agonist GTDF protects against skeletal muscle atrophy. Mol Cell Endocrinol (2017) 439:273–85. doi: 10.1016/j.mce.2016.09.013
9. Tanaka Y, Kita S, Nishizawa H, Fukuda S, Fujishima Y, Obata Y, et al. Adiponectin promotes muscle regeneration through binding to T-cadherin. Sci Rep (2019) 9:16. doi: 10.1038/s41598-018-37115-3
10. Inoue A, Cheng XW, Huang Z, Hu L, Kikuchi R, Jiang H, et al. Exercise restores muscle stem cell mobilization, regenerative capacity and muscle metabolic alterations via adiponectin/AdipoR1 activation in SAMP10 mice. J Cachexia Sarcopenia Muscle (2017) 8(3):370–85. doi: 10.1002/jcsm.12166
11. Papa EV, Dong X, Hassan M. Resistance training for activity limitations in older adults with skeletal muscle function deficits: a systematic review. Clin Interv Aging (2017) 12:955–61. doi: 10.2147/CIA.S104674
12. Beaudart C, Dawson A, Shaw SC, Harvey NC, Kanis JA, Binkley N, et al. Nutrition and physical activity in the prevention and treatment of sarcopenia: systematic review. Osteoporos Int (2017) 28:1817–33. doi: 10.1007/s00198-017-3980-9
13. Krause MP, Milne KJ, Hawke TJ. Adiponectin-Consideration for its Role in Skeletal Muscle Health. Int J Mol Sci (2019) 20(7):1528. doi: 10.3390/ijms20071528
14. Hughes VA, Frontera WR, Wood M, Evans WJ, Dallal GE, Roubenoff R, et al. Longitudinal muscle strength changes in older adults: influence of muscle mass, physical activity, and health. J Gerontol A Biol Sci Med Sci (2001) 56:B209–17. doi: 10.1093/gerona/56.5.B209
15. Huang C, Niu K, Momma H, Kobayashi Y, Guan L, Nagatomi R. Inverse association between circulating adiponectin levels and skeletal muscle strength in Japanese men and women. Nutr Metab Cardiovasc Dis (2014) 24(1):42–9. doi: 10.1016/j.numecd.2013.03.006
16. Simpson KA, Singh MA. Effects of exercise on adiponectin: a systematic review. Obesity (Silver Spring) (2008) 16(2):241–56. doi: 10.1038/oby.2007.53
17. Fatouros IG, Tournis S, Leontsini D, Jamurtas AZ, Sxina M, Thomakos P, et al. Leptin and adiponectin responses in overweight inactive elderly following resistance training and detraining are intensity related. J Clin Endocrinol Metab (2005) 90(11):5970–7. doi: 10.1210/jc.2005-0261
18. Silva FM, de Almeida JC, Feoli AM. Effect of diet on adiponectin levels in blood. Nutr Rev (2011) 69(10):599–612. doi: 10.1111/j.1753-4887.2011.00414.x
19. Yagi T, Toyoshima Y, Tokita R, Taguchi Y, Okamoto Y, Takahashi SI, et al. Low-protein diet enhances adiponectin secretion in rats. Biosci Biotechnol Biochem (2019) 83(9):1774–81. doi: 10.1080/09168451.2019.1621153
20. Mateo-Gallego R, Lamiquiz-Moneo I, Perez-Calahorra S, Marco-Benedí V, Bea AM, Baila-Rueda L, et al. Different protein composition of low-calorie diet differently impacts adipokine profile irrespective of weight loss in overweight and obese women. Nutr Metab Cardiovasc Dis (2018) 28(2):133–42. doi: 10.1016/j.numecd.2017.10.024
21. von Elm E, Altman DG, Egger M, Pocock SJ, Gøtzsche PC, Vandenbroucke JP, et al. The Strengthening the Reporting of Observational Studies in Epidemiology (STROBE) statement: guidelines for reporting observational studies. J Clin Epidemiol (2008) 61(4):344–9. doi: 10.1016/j.jclinepi.2007.11.008
22. Moher D, Liberati A, Tetzlaff J, Altman DG, PRISMA Group. Preferred reporting items for systematic reviews and meta-analyses: the PRISMA statement. Ann Intern Med (2009) 151(4):264–W64. doi: 10.7326/0003-4819-151-4-200908180-00135
23. Chen LK, Liu LK, Woo J, Assantachai P, Auyeung TW, Bahyah KS, et al. Sarcopenia in Asia: consensus report of the Asian Working Group for Sarcopenia. J Am Med Dir Assoc (2014) 15:95–101. doi: 10.1016/j.jamda.2013.11.025
24. Lo CK, Mertz D, Loeb M. Newcastle-Ottawa Scale: comparing reviewers’ to authors’ assessments. BMC Med Res Methodol (2014) 14:45. doi: 10.1186/1471-2288-14-45
25. Hermont AP, Oliveira PA, Martins CC, Paiva SM, Pordeus IA, Auad SM. Tooth erosion and eating disorders: a systematic review and meta-analysis. PloS One (2014) 9(11):e111123. doi: 10.1371/journal.pone.0111123
26. Yeung SSY, Reijnierse EM, Pham VK, Trappenburg MC, Lim WK, Meskers CGM, et al. Sarcopenia and its association with falls and fractures in older adults: A systematic review and meta-analysis. J Cachexia Sarcopenia Muscle (2019) 10(3):485–500. doi: 10.1002/jcsm.12411
27. Higgins JPT, Deeks JJ, Thomas J, Chandler J, Cumpston M, Li T, Page MJ, Welch VA eds. Chapter 6: Choosing effect measures and computing estimates of effect. In: Cochrane Handbook for Systematic Reviews of Interventions. 2nd Edition. Chichester (UK): John Wiley & Sons (2019).
28. Hozo SP, Djulbegovic B, Hozo I. Estimating the mean and variance from the median, range, and the size of a sample. BMC Med Res Methodol (2005) 5:13. doi: 10.1186/1471-2288-5-13
29. Higgins JP, Thompson SG, Deeks JJ, Altman DG. Measuring inconsistency in meta-analyses. BMJ (2003) 327(7414):557–60. doi: 10.1136/bmj.327.7414.557
30. Begg CB, Mazumdar M. Operating characteristics of a rank correlation test for publication bias. Biometrics (1994) 50(4):1088–101. doi: 10.2307/2533446
31. Egger M, Davey Smith G, Schneider M, Minder C. Bias in meta-analysis detected by a simple, graphical test. BMJ (1997) 315(7109):629–34. doi: 10.1136/bmj.315.7109.629
32. Ramachandran R, Gravenstein KS, Metter EJ, Egan JM, Ferrucci L, Chia CW. Selective contribution of regional adiposity, skeletal muscle, and adipokines to glucose disposal in older adults. J Am Geriatr Soc (2012) 60(4):707–12. doi: 10.1111/j.1532-5415.2011.03865.x
33. Kim TN, Won JC, Kim YJ, Lee EJ, Kim MK, Park MS, et al. Serum adipocyte fatty acid-binding protein levels are independently associated with sarcopenic obesity. Diabetes Res Clin Pract (2013) 101(2):210–7. doi: 10.1016/j.diabres.2013.05.014
34. Can B, Kara O, Kizilarslanoglu MC, Arik G, Aycicek GS, Sumer F, et al. Serum markers of inflammation and oxidative stress in sarcopenia. Aging Clin Exp Res (2017) 29(4):745–52. doi: 10.1007/s40520-016-0626-2
35. Harada H, Kai H, Shibata R, Niiyama H, Nishiyama Y, Murohara T, et al. New diagnostic index for sarcopenia in patients with cardiovascular diseases. PloS One (2017) 12(5):e0178123. doi: 10.1371/journal.pone.0178123
36. Lu Y, Karagounis LG, Ng TP, Carre C, Narang V, Wong G, et al. Systemic and Metabolic Signature of Sarcopenia in Community-Dwelling Older Adults. J Gerontol A Biol Sci Med Sci (2020) 75(2):309–17. doi: 10.1093/gerona/glz001
37. Rossi FE, Lira FS, Silva BSA, Freire APCF, Ramos EMC, Gobbo LA. Influence of skeletal muscle mass and fat mass on the metabolic and inflammatory profile in sarcopenic and non-sarcopenic overfat elderly. Aging Clin Exp Res (2019) 31(5):629–35. doi: 10.1007/s40520-018-1029-3
38. Li CW, Yu K, Shyh-Chang N, Li GX, Jiang LJ, Yu SL, et al. Circulating factors associated with sarcopenia during ageing and after intensive lifestyle intervention. J Cachexia Sarcopenia Muscle (2019) 10(3):586–600. doi: 10.1002/jcsm.12417
39. Fiaschi T, Cirelli D, Comito G, Gelmini S, Ramponi G, Serio M, et al. Globular adiponectin induces differentiation and fusion of skeletal muscle cells. Cell Res (2009) 19(5):584–97. doi: 10.1038/cr.2009.39
40. Gamberi T, Modesti A, Magherini F, D’Souza DM, Hawke T, Fiaschi T. Activation of autophagy by globular adiponectin is required for muscle differentiation. Biochim Biophys Acta (2016) 1863(4):694–702. doi: 10.1016/j.bbamcr.2016.01.016
41. Goto A, Ohno Y, Ikuta A, Suzuki M, Ohira T, Egawa T, et al. Up-regulation of adiponectin expression in antigravitational soleus muscle in response to unloading followed by reloading, and functional overloading in mice. PloS One (2013) 8(12):e81929. doi: 10.1371/journal.pone.0081929
42. Perrotta F, Nigro E, Mollica M, Costigliola A, D’Agnano V, Daniele A, et al. Pulmonary Hypertension and Obesity: Focus on Adiponectin. Int J Mol Sci (2019) 20(4):912. doi: 10.3390/ijms20040912
43. Chen MB, McAinch AJ, Macaulay SL, Castelli LA, O’brien PE, Dixon JB, et al. Impaired activation of AMP-kinase and fatty acid oxidation by globular adiponectin in cultured human skeletal muscle of obese type 2 diabetics. J Clin Endocrinol Metab (2005) 90(6):3665–72. doi: 10.1210/jc.2004-1980
44. Mao X, Kikani CK, Riojas RA, Langlais P, Wang L, Ramos FJ, et al. APPL1 binds to adiponectin receptors and mediates adiponectin signalling and function. Nat Cell Biol (2006) 8(5):516–23. doi: 10.1038/ncb1404. [published correction appears in Nat Cell Biol. 2006 Jun;8(6):642].
45. Abdulla H, Smith K, Atherton PJ, Idris I. Role of insulin in the regulation of human skeletal muscle protein synthesis and breakdown: a systematic review and meta-analysis. Diabetologia (2016) 59(1):44–55. doi: 10.1007/s00125-015-3751-0
46. Groen BB, Hamer HM, Snijders T, van Kranenburg J, Frijns D, Vink H, et al. Skeletal muscle capillary density and microvascular function are compromised with aging and type 2 diabetes. J Appl Physiol (1985) (2014) 116(8):998–1005. doi: 10.1152/japplphysiol.00919.2013
47. Wijsman CA, Rozing MP, Streefland TC, le Cessie S, Mooijaart SP, Slagboom PE, et al. Familial longevity is marked by enhanced insulin sensitivity. Aging Cell (2011) 10(1):114–21. doi: 10.1111/j.1474-9726.2010.00650.x
48. Blüher M, Kahn BB, Kahn CR. Extended longevity in mice lacking the insulin receptor in adipose tissue. Science (2003) 299(5606):572–4. doi: 10.1126/science.1078223
49. Arai Y, Kamide K, Hirose N. Adipokines and Aging: Findings From Centenarians and the Very Old. Front Endocrinol (Lausanne) (2019) 10:142. doi: 10.3389/fendo.2019.00142
50. Atzmon G, Pollin TI, Crandall J, Tanner K, Schechter CB, Scherer PE, et al. Adiponectin levels and genotype: a potential regulator of life span in humans. J Gerontol A Biol Sci Med Sci (2008) 63(5):447–53. doi: 10.1093/gerona/63.5.447
51. Wang X, Hu Z, Hu J, Du J, Mitch WE. Insulin resistance accelerates muscle protein degradation: Activation of the ubiquitin-proteasome pathway by defects in muscle cell signaling. Endocrinology (2006) 147(9):4160–8. doi: 10.1210/en.2006-0251
52. Kistorp C, Faber J, Galatius S, Gustafsson F, Frystyk J, Flyvbjerg A, et al. Plasma adiponectin, body mass index, and mortality in patients with chronic heart failure. Circulation (2005) 112(12):1756–62. doi: 10.1161/CIRCULATIONAHA.104.530972
53. Scarale MG, Fontana A, Trischitta V, Copetti M, Menzaghi C. Circulating adiponectin levels are paradoxically associated with mortality rate. A systematic review and meta-analysis. J Clin Endocrinol Metab (2018) 104:1357–68. doi: 10.1210/jc.2018-01501
54. Jo E, Lee SR, Park BS, Kim JS. Potential mechanisms underlying the role of chronic inflammation in age-related muscle wasting. Aging Clin Exp Res (2012) 24(5):412–22. doi: 10.3275/8464
55. Rieu I, Magne H, Savary-Auzeloux I, Averous J, Bos C, Peyron MA, et al. Reduction of low grade inflammation restores blunting of postprandial muscle anabolism and limits sarcopenia in old rats. J Physiol (2009) 587(Pt 22):5483–92. doi: 10.1113/jphysiol.2009.178319
56. Chung HY, Cesari M, Anton S, Marzetti E, Giovannini S, Seo AY, et al. Molecular inflammation: underpinnings of aging and age-related diseases. Ageing Res Rev (2009) 8(1):18–30. doi: 10.1016/j.arr.2008.07.002
57. Bano G, Trevisan C, Carraro S, Solmi M, Luchini C, Stubbs B, et al. Inflammation and sarcopenia: A systematic review and meta-analysis. Maturitas (2017) 96:10–5. doi: 10.1016/j.maturitas.2016.11.006
58. Ouchi N, Walsh K. Adiponectin as an anti-inflammatory factor. Clin Chim Acta (2007) 380(1-2):24–30. doi: 10.1016/j.cca.2007.01.026
59. Ding Q, Ash C, Mracek T, Merry B, Bing C. Caloric restriction increases adiponectin expression by adipose tissue and prevents the inhibitory effect of insulin on circulating adiponectin in rats. J Nutr Biochem (2012) 23(8):867–74. doi: 10.1016/j.jnutbio.2011.04.011
60. Abou-Samra M, Lecompte S, Schakman O, Noel L, Many MC, Gailly P, et al. Involvement of adiponectin in the pathogenesis of dystrophinopathy. Skelet Muscle (2015) 5:25. doi: 10.1186/s13395-015-0051-9
61. Kizer JR, Arnold AM, Strotmeyer ES, Ives DG, Cushman M, Ding J, et al. Change in circulating adiponectin in advanced old age: determinants and impact on physical function and mortality. The Cardiovascular Health Study All Stars Study. J Gerontol A Biol Sci Med Sci (2010) 65(11):1208–14. doi: 10.1093/gerona/glq122
62. Bucci L, Yani SL, Fabbri C, Bijlsma AY, Maier AB, Meskers CG, et al. Circulating levels of adipokines and IGF-1 are associated with skeletal muscle strength of young and old healthy subjects. Biogerontology (2013) 14(3):261–72. doi: 10.1007/s10522-013-9428-5
63. Baker JF, Von Feldt JM, Mostoufi-Moab S, Kim W, Taratuta E, Leonard MB. Insulin-like Growth Factor 1 and Adiponectin and Associations with Muscle Deficits, Disease Characteristics, and Treatments in Rheumatoid Arthritis. J Rheumatol (2015) 42(11):2038–45. doi: 10.3899/jrheum.150280
64. Jabagi H, Tran DT, Hessian R, Glineur D, Rubens FD. Impact of Gender on Arterial Revascularization Strategies for Coronary Artery Bypass Grafting. Ann Thorac Surg (2018) 105(1):62–8. doi: 10.1016/j.athoracsur.2017.06.054
65. Kirchengast S, Huber J. Gender and age differences in lean soft tissue mass and sarcopenia among healthy elderly. Anthropol Anz (2009) 67(2):139–51. doi: 10.1127/0003-5548/2009/0018
66. Nagasawa M, Takami Y, Akasaka H, Kabayama M, Maeda S, Yokoyama S, et al. High plasma adiponectin levels are associated with frailty in a general old-old population: The Septuagenarians, Octogenarians, Nonagenarians Investigation with Centenarians study. Geriatr Gerontol Int (2018) 18(6):839–46. doi: 10.1111/ggi.13258
67. Baker JF, Newman AB, Kanaya A, Leonard MB, Zemel B, Miljkovic I, et al. The Adiponectin Paradox in the Elderly: Associations With Body Composition, Physical Functioning, and Mortality. J Gerontol A Biol Sci Med Sci (2019) 74(2):247–53. doi: 10.1093/gerona/gly017
68. Arai Y, Nakazawa S, Kojima T, Takayama M, Ebihara Y, Shimizu K, et al. Total and high molecular weight adiponectin and level-modifying polymorphisms of ADIPOQ in centenarians. Endokrynol Pol (2012) 63(6):439–46.
69. Boöttner A, Kratzsch J, Müller G, Kapellen TM, Blüher S, Keller E, et al. Gender differences of adiponectin levels develop during the progression of puberty and are related to serum androgen levels. J Clin Endocrinol Metab (2004) 89(8):4053–61. doi: 10.1210/jc.2004-0303
70. Cnop M, Havel PJ, Utzschneider KM, Carr DB, Sinha MK, Boyko EJ, et al. Relationship of adiponectin to body fat distribution, insulin sensitivity and plasma lipoproteins: evidence for independent roles of age and sex. Diabetologia (2003) 46(4):459–69. doi: 10.1007/s00125-003-1074-z
71. Arita Y, Kihara S, Ouchi N, Takahashi M, Maeda K, Miyagawa J, et al. Paradoxical decrease of an adipose-specific protein, adiponectin, in obesity. Biochem Biophys Res Commun (1999) 257(1):79–83. doi: 10.1006/bbrc.1999.0255
72. Gavrila A, Chan JL, Yiannakouris N, Kontogianni M, Miller LC, Orlova C, et al. Serum adiponectin levels are inversely associated with overall and central fat distribution but are not directly regulated by acute fasting or leptin administration in humans: cross-sectional and interventional studies. J Clin Endocrinol Metab (2003) 88(10):4823–31. doi: 10.1210/jc.2003-030214
73. Guenther M, James R, Marks J, Zhao S, Szabo A, Kidambi S. Adiposity distribution influences circulating adiponectin levels. Transl Res (2014) 164(4):270–7. doi: 10.1016/j.trsl.2014.04.008
74. Reneau J, Goldblatt M, Gould J, Kindel T, Kastenmeier A, Higgins R, et al. Effect of adiposity on tissue-specific adiponectin secretion. PloS One (2018) 13(6):e0198889. doi: 10.1371/journal.pone.0198889
75. Delaigle AM, Senou M, Guiot Y, Many MC, Brichard SM. Induction of adiponectin in skeletal muscle of type 2 diabetic mice: In vivo and in vitro studies. Diabetologia (2006) 49(6):1311–23. doi: 10.1007/s00125-006-0210-y
76. Baek SJ, Nam GE, Han KD, Choi SW, Jung SW, Bok AR, et al. Sarcopenia and sarcopenic obesity and their association with dyslipidemia in Korean elderly men: the 2008-2010 Korea National Health and Nutrition Examination Survey. J Endocrinol Invest (2014) 37(3):247–60. doi: 10.1007/s40618-013-0011-3
77. Schrager MA, Metter EJ, Simonsick E, Ble A, Bandinelli S, Lauretani F, et al. Sarcopenic obesity and inflammation in the InCHIANTI study. J Appl Physiol (1985) (2007) 102(3):919–25. doi: 10.1152/japplphysiol.00627.2006
Keywords: adiponectin, sarcopenia, aging, elderly, muscle mass
Citation: Komici K, Dello Iacono A, De Luca A, Perrotta F, Bencivenga L, Rengo G, Rocca A and Guerra G (2021) Adiponectin and Sarcopenia: A Systematic Review With Meta-Analysis. Front. Endocrinol. 12:576619. doi: 10.3389/fendo.2021.576619
Received: 03 July 2020; Accepted: 17 March 2021;
Published: 15 April 2021.
Edited by:
Marc R. Blackman, Washington DC VA Medical Center, United StatesReviewed by:
Alex F. Bokov, The University of Texas Health Science Center at San Antonio, United StatesSara Tagliaferri, University of Parma, Italy
Copyright © 2021 Komici, Dello Iacono, De Luca, Perrotta, Bencivenga, Rengo, Rocca and Guerra. This is an open-access article distributed under the terms of the Creative Commons Attribution License (CC BY). The use, distribution or reproduction in other forums is permitted, provided the original author(s) and the copyright owner(s) are credited and that the original publication in this journal is cited, in accordance with accepted academic practice. No use, distribution or reproduction is permitted which does not comply with these terms.
*Correspondence: Klara Komici, a2xhcmEua29taWNpQHVuaW1vbC5pdA==
†These authors have contributed equally to this work