- 1Department of Biomedical Science, Colorado State University, Fort Collins, CO, United States
- 2Department of Zoology and Physiology, University of Wyoming, Laramie, WY, United States
Gonadotropes cells located in the anterior pituitary gland are critical for reproductive fitness. A rapid surge in the serum concentration of luteinizing hormone (LH) secreted by anterior pituitary gonadotropes is essential for stimulating ovulation and is thus required for a successful pregnancy. To meet the requirements to mount the LH surge, gonadotrope cells display plasticity at the cellular, molecular and morphological level. First, gonadotrope cells heighten their sensitivity to an increasing frequency of hypothalamic GnRH pulses by dynamically elevating the expression of the GnRH receptor (GnRHR). Following ligand binding, GnRH initiates highly organized intracellular signaling cascades that ultimately promote the synthesis of LH and the trafficking of LH vesicles to the cell periphery. Lastly, gonadotrope cells display morphological plasticity, where there is directed mobilization of cytoskeletal processes towards vascular elements to facilitate rapid LH secretion into peripheral circulation. This mini review discusses the functional and organizational plasticity in gonadotrope cells including changes in sensitivity to GnRH, composition of the GnRHR signaling platform within the plasma membrane, and changes in cellular morphology. Ultimately, multimodal plasticity changes elicited by gonadotropes are critical for the generation of the LH surge, which is required for ovulation.
Introduction
The anterior pituitary is the body’s master endocrine gland and is, arguably, the most complex endocrine organ in the body. At maturity, this gland is responsible for the synthesis and secretion of hormones, which control homeostasis, growth, lactation, and reproduction in mammals (1). It is composed of five distinct endocrine cell types which secrete six different hormones: gonadotropes release luteinizing hormone (LH) and follicle-stimulating hormone (FSH); thyrotropes release thyroid-stimulating hormone (TSH); corticotropes release adrenocorticotropic hormone (ACTH); somatotropes release growth hormone (GH); and lactotropes release prolactin (Prl) (2). Collectively, anterior pituitary cells are under hypothalamic control and receive feedback from the periphery to adjust hormone secretion to keep the body in homeostatic balance (3).
In mammals, reproductive competence depends on the coordinated activation of gonadotrope cells in response to pulsatile hypothalamic gonadotropin releasing hormone (GnRH) (4, 5). Following release, GnRH is transported via the hypophysial portal vessels to the anterior pituitary where it binds to the GnRH receptor (GnRHR) on gonadotropes (6, 7). Stimulation of the GnRHR activates a complex array of intracellular signaling networks that culminates in the synthesis and secretion of the heterodimeric glycoproteins, LH and FSH, into systemic circulation where they regulate gonadal development and function (5, 8). The gonadal steroid hormones, estrogen, progesterone, and androgen, participate in a feedback loop with the hypothalamus and the anterior pituitary to regulate GnRH and gonadotropin secretion (9–11). Taken together, any substantive physiological response of gonadotrope cells represents a complex integration of multiple hormones and signaling pathways that culminate in the functional plasticity of distinct gene programs and protein expression over the course of the estrous cycle.
In females, a mid-cycle surge of LH is obligatory for ovulation. The “LH surge” is initiated by a rise in estradiol‐17β (E2) production by the ovarian follicles, triggering a switch from negative feedback on the hypothalamus and pituitary gland to transient positive feedback (5, 11). E2 positive feedback is driven by activation of ERα in kisspeptin neurons in the hypothalamus, which stimulate GnRH neurons to increase GnRH pulse frequency (12–14). In addition, rising E2 levels prior to ovulation stimulate GnRHR expression in multiple model systems (15–17). The dynamic increase in GnRHR expression represents an important priming event, which increases the sensitivity of gonadotropes to GnRH in preparation for the LH surge (18–20). During this critical pre-ovulatory window, gonadotrope cells demonstrate both functional and organizational plasticity to induce the LH surge. At the simplest level, gonadotrope functional plasticity is illustrated by the changes in the intracellular stores of LH and FSH and the relative abundance of secretory granules following GnRH stimulation (21). Collectively then, changes in gonadotrope synthesis and secretion of LH are not only dependent on changes in GnRH pulse frequency, but also the number of GnRH receptors available for binding and, consequently, the responsiveness to a given dose of GnRH. Finally, GnRH and, perhaps, E2, initiate dynamic organizational plasticity within the gonadotrope population that results in the development of cellular processes or projections containing LH that extend toward capillary sinusoids (22, 23). In this mini review we will address each of these dynamic changes that underlie gonadotrope plasticity including altered sensitivity to hypothalamic GnRH, the GnRHR signaling platform, and morphological alterations in the gonadotrope network.
Gonadotrope Functional Plasticity
The Gonadotrope Population
Gonadotropes are a minority population of cells representing between 5% and 15% of the anterior pituitary gland and are traditionally defined by a specific phenotype that includes expression of 4 unique genes, the common glycoprotein hormone α subunit, the unique LHβ and FSHβ subunits and the GnRHR (24). Gonadotropes are classically viewed as a single distinct cell lineage, however, as unbiased technological methods have advanced, it is increasingly clear that the gonadotrope population has greater complexity and plasticity with cell-type composition and hormonal expression than previously recognized (25). For example, gonadotropes can be classified as either mono or bihormonal based on their expression of LH and/or FSH (26, 27). Gonadotropes can further be classified by their size as “small”, “medium”, and “large” cells whose percentages display distinct changes during different stages of the reproductive cycle (28, 29).
Gonadotrope plasticity across the estrous cycle has been well documented (25, 30). For example, the percentage of bi-hormonal gonadotrope cells dynamically elevates during the pre-ovulatory period when large amounts of gonadotropin are released into systemic circulation (26, 31). Beyond changes in LH and FSH levels in gonadotropes, our current understanding of the pituitary expands beyond mutually exclusive cell lineages to include transdifferentiation. In support of this, somatotrope cells may transition into gonadotropes to support the LH surge by upregulating gonadotropin mRNA expression (32). Gonadotrope population plasticity is also supported by recent single cell RNA-seq analysis which described a unique cluster of cells containing Prl, Gh, Tshb, POMC, and Lhb mRNAs that represents approximately 5%–11% of the total anterior pituitary cell population in mice (33). This result is intriguing as traditionally gonadotropes and corticotropes derive as POU1F1 independent lineages during embryonic development (34). The developmental origins and transdifferentiation mechanisms of these multi-hormonal cell clusters is uncertain but clearly warrants further investigation especially considering mRNA levels do not necessarily correlate with protein expression.
Despite this unknown, it is interesting that the multi-hormonal pituitary cells represent a shift-able pool of cells that alter gene programs depending on the physiological state. For example, Prl expression in the population is significantly upregulated during pregnancy to initiate and sustain lactation postpartum. Concurrently, the multi-hormonal cells downregulate Lhb to impair ovulation and fertility during the lactation state (33). Thus, this multi-hormonal cell population is dynamic and functions to alter endocrine output to meet physiological demand. It is an intriguing possibility that these multi-hormonal cells may play a role in supporting the LH surge required for ovulation as estradiol has emerged as a stimulus for promoting transdifferentiation (35). Previous studies also found that multi-hormonal anterior pituitary cells are not only more abundant in females compared to males, but also display greater plasticity (33, 36, 37). Thus, female specific plasticity may underlie a broader mechanism under which clusters of multi-hormonal cells adjust their output (LH) based on hypothalamic (GnRH) and sex steroid (E2) hormonal input prior to ovulation to collectively maximize LH production necessary for the surge. Taken together, it is clear that the gonadotrope population represents a complex heterogeneous cell network that integrates multiple physiological queues to modulate gonadotropin secretion.
GnRH Receptor Expression
Ovulation is perhaps the most fundamental event in reproduction in virtually all female vertebrates. It requires a dramatic 20-30-fold surge of LH released by the anterior pituitary into the peripheral circulation to induce the release of the oocyte from the ovary (38). The dynamic change in GnRHR expression has received particular attention as an important mediator of the LH surge, since it functions to increase the sensitivity of gonadotropes to GnRH during the periovulatory period when serum concentrations of progesterone decline as a result of luteolysis and E2 levels rise with the development of the preovulatory follicle (39). The elevation of ovarian E2 leads to a 3–6 fold increase in GnRHR numbers in gonadotrope cells followed temporally by increased hypothalamic GnRHR secretion (20). It is also clear that the heightened expression of GnRHRs in response to E2 is a result of enhanced transcription of the Gnrhr gene (39). Although the mechanisms by which E2 stimulates Gnrhr gene expression are not clearly defined, it may be partially through a membrane-initiated signal proceeding through a cAMP-dependent mechanism (40).
Certainly, the loss or dysregulation of hypothalamic input to gonadotropes underlies a number of conditions of hypogonadotropic hypogonadism; however, it is becoming increasingly clear that diminished pituitary sensitivity to GnRH may also contribute to irregular ovulatory cycles. Of particular note is the inverse relationship between GnRHR levels and elevated BMI associated with obesity. Work in both humans and mice suggest that gonadotrope cells are regulated by metabolic signals, including the hormone leptin (41–43). More specifically, Gwen Childs research provides a direct link between leptin signaling and GnRHR expression in mice (41, 44). Leptin resistance, which is characteristic of an obese state, results in a loss of GnRHR expression on the plasma membrane that may reflect a post-transcriptional regulatory mechanism (41). Additional work by Nanette Santoro’s group and others highlights that obesity reduces LH pulse amplitude in women without a corresponding change in LH pulse frequency (43, 45, 46). These women present with a relative hypogonadotropic hypogonadal condition that appears to reflect diminished pituitary sensitivity to GnRH. If correct, then changes in the metabolic state may alter the ability of E2 to either elicit enhanced GnRHR expression or to activate the key gene programs that effectively couple GnRHR activation and increased gonadotropin production. Unfortunately, as gonadotropes only reflect approximately 5%–15% of the total population of endocrine cells in the pituitary, it is difficult to probe the unique transcriptional events evoked by E2 in gonadotropes (24). Recently, however, targeted expression of EGFP followed by Fluorescence Activated Cell Sorting (FACS) and RNA-seq allowed for the first characterization of global changes in gene expression in response to E2. This opens new avenues to explore the impact of pathological conditions (e.g. metabolic state) on E2 regulation of gonadotropes in a non-rodent species (sheep) that displays an estrous cycle that closely mimics the menstrual cycle of women (47).
GnRH Receptor Organization
Following plasma membrane expression, GnRH-occupied GnRHR couples to Gαq/11 leading to stimulation of phospholipase C, elevation of intracellular free calcium and activation of kinase C (PKC) isoforms (48, 49). These early events underlie GnRH activation of multiple mitogen activated protein kinase (MAPK) signaling cascades including extracellular signal regulated kinase (ERK) (50–52). The ERK signaling module has been studied extensively in gonadotropes and is linked to the transcriptional regulation of the lhb gene through induction of the immediate early gene Egr-1 (53–55). It is well documented that faster GnRH pulse frequencies generated during the pre-ovulatory period initiate distinct ERK activation patterns that are responsible for dynamic Egr-1 upregulation necessary for LHβ synthesis required for the LH surge (56, 57). In vivo work highlights that the lack of ERK signaling in gonadotrope cells results in an acyclic, anovulatory phenotype in female mice due to the loss of Egr-1 and LHβ subunit expression (55). Thus, GnRH induced activation of ERK is absolutely required for expression of LHβ and ovulation underscoring the importance of this signaling pathway within the female reproductive axis.
It is also clear that proper second messenger signaling in gonadotropes is dependent on GnRHR localization to highly compartmentalized microdomains in the plasma membrane (58). Membrane microdomains efficiently and spatially organize the initiation of intracellular signaling by co-localizing membrane receptors and their cognate downstream signaling components in complexes that, upon ligand activation, co-segregate to form transient signaling platforms (59, 60). Within gonadotropes, the GnRHR and downstream signaling intermediates including c-raf kinase, ERK, calmodulin and Gαq are detectable in microdomains in primary cultures of mouse pituitary cells (58). Additionally, the GnRHR co-immunoprecipitated with ERK from microdomain fractions prepared from mouse pituitary cells suggesting that GnRHR and ERK reside in the same microdomain (61). Functionally this is important because following disruption of gonadotrope microdomain organization, GnRHR is no longer capable of initiating ERK activation (58). Taken together, microdomains serve as a spatial platform that allows for precise organization of a multi-protein scaffold that underlies GnRH initiation of ERK activation. What awaits elucidation is the plasticity of the GnRHR signaling proteome. More specifically, how does composition of the GnRHR signaling platform dynamically change over the course of the estrous cycle?
Fluorophore-tagged GnRH receptors have allowed direct observations of the in-membrane behavior of unoccupied, agonist occupied, and antagonist-occupied receptors (62, 63). Fluorescence resonance energy transfer methods demonstrated that agonist but not antagonist leads to self-association of GnRH receptors in the plasma membrane (62). Thus, GnRH (agonist) induced receptor self-association in gonadotropes likely facilitates assembling multiple discrete GnRHR containing membrane microdomains to transduce a GnRH signal that leads to functional ERK activation and subsequent LH synthesis in gonadotropes.
Dynamic assembly of microdomains within the plasma membrane following GnRH activation suggests a role for the cytoskeleton in mediating their aggregation. Consistent with this, many of the molecular components regulating the actin cytoskeleton have been shown to associate with membrane microdomains (64, 65). An emerging concept is microdomains serve as centers for organizing ligand mediated communication between the plasma membrane and the actin cytoskeleton to control nucleation (66). The cytoskeleton has critical importance in gonadotropes as inhibition of actin remodeling effectively blocks GnRH signaling to ERK but also more acute signaling events such as the opening of L-type calcium channels in the plasma membrane (61, 67). Additionally, the disruption of GnRH-induced actin remodeling events blocks LH secretion from primary murine gonadotrope cells (68). Taken together, there appears to be interdependence between actin reorganization, compartmentalized GnRHR signaling to ERK and GnRH-mediated secretory events. Thus, understanding GnRH regulation of actin dynamics has clear implications for both LH biosynthesis and GnRH mediated release of LH into the bloodstream. Both of these pituitary events are critical for generating the large increase in LH secretion necessary for ovulation.
Gonadotrope Organizational Plasticity
The In Vivo Gonadotrope Network
In gonadotropes, the cytoskeleton plays a critical role in the regulated release of LH following GnRH stimulation (68, 69). Following ligand binding, the actin network reorganizes to allow vesicles to fuse with the plasma membrane to secrete hormone into the extracellular space. Reserve vesicles then move along microtubules and actin to replenish those vesicles that successfully docked with the plasma membrane (70, 71). In vivo and ex vivo evidence suggests that GnRH-stimulated gonadotropes are also capable of developing processes that extend directionally toward blood vessels during LH secretory episodes (22, 72, 73). Additionally, our group has demonstrated that primary pituitary cells concentrate LHβ into areas of dynamic membrane reorganization after GnRH treatment and, as previously discussed, disruption of the actin cytoskeleton inhibits LH release (68). These observations suggest that GnRHR engagement of the cytoskeleton not only facilitates the exocytosis of LH granules but also organizes these cells into a favorable spatial orientation to achieve a rapid and pronounced increase in circulating LH in vivo.
Towards this end, gonadotrope cells are organized in homotypic and heterotypic cellular networks that are often embedded in connective tissue surrounded by rich vascular networks (74–76). Capillary endothelia are fenestrated facilitating transfer of materials between the interstitium and the blood. Depending on the physiological state of the animal, such as estrous cycle stage, gonadotrope cells display considerable surface area apposed to capillary endothelium (73, 77). At the population level, gonadotropes can undergo plasticity changes that allow for coordination with one another for efficient and robust delivery of gonadotropin into the capillary (22, 74, 78). Previous work suggests that steroid hormones can regulate structural plasticity and cell-to cell connectivity of pituitary cells. For example, following castration, somatotropes within a living pituitary slice were found more clustered and responsive to growth hormone within hours (79). In gonadotropes, pretreatment of ex vivo murine pituitary slices with 10 nm E2 was capable of significantly enhancing gonadotrope responsiveness to GnRH with respect to cellular process generation and extension (22). The stimulatory E2 effect on gonadotropes was evident in experiments with long-term (14 h) but not short-term (1.5 h) exposure (22). The time dependence is consistent with upregulation of E2 mediated increases in GnRHR synthesis and increased responsiveness to GnRH necessary to generate the LH surge (80). The timing is also consistent with E2 upregulation of a number of genes involved in cell movement and the cytoskeleton (47).
Lastly, if gonadotropes position themselves to maximize their secretory surface to capillary sinusoids then LH secretory granules would need to be polarized to the side of the gonadotrope nearest to a vascular sinusoid during the preovulatory period. Importantly, this appears to be the case. In ovine pituitary cells, LH granules were polarized in about 20-30% of gonadotropes with this number increasing to 80% during the preovulatory LH surge (77, 81). Additionally, positive feedback from E2 can directly affect the mobilization of LH secretory granules (82). The mechanisms by which GnRH and E2 contribute to LH polarization are unclear but gonadotropes appear “primed” organizationally to support the LH surge. Collectively gonadotrope priming reflects multiple events that include enhanced GnRH responsiveness, transport and polarization of LH granules and increased apposition to the vascular system.
Conclusions
The dynamic changes exhibited by gonadotropes in preparation for the LH surge reflects a culmination of events following GnRH binding. These events include enhanced GnRHR responsiveness (driven by elevated E2), GnRHR aggregation, activation of ERK and upregulation of the immediate early gene Egr-1, synthesis and polarization of LH granules, engagement of the actin cytoskeleton, and increased apposition to pituitary vasculature. GnRH, in combination with E2, facilitates plasticity changes that not only underlie LH synthesis, but also to place the gonadotrope population containing LH secretory granules in close proximity to vasculature to maximize circulating LH concentrations. Thus, it is the collective functional and organizational plasticity induced by gonadotropes that mediate the preovulatory LH surge (Figure 1).
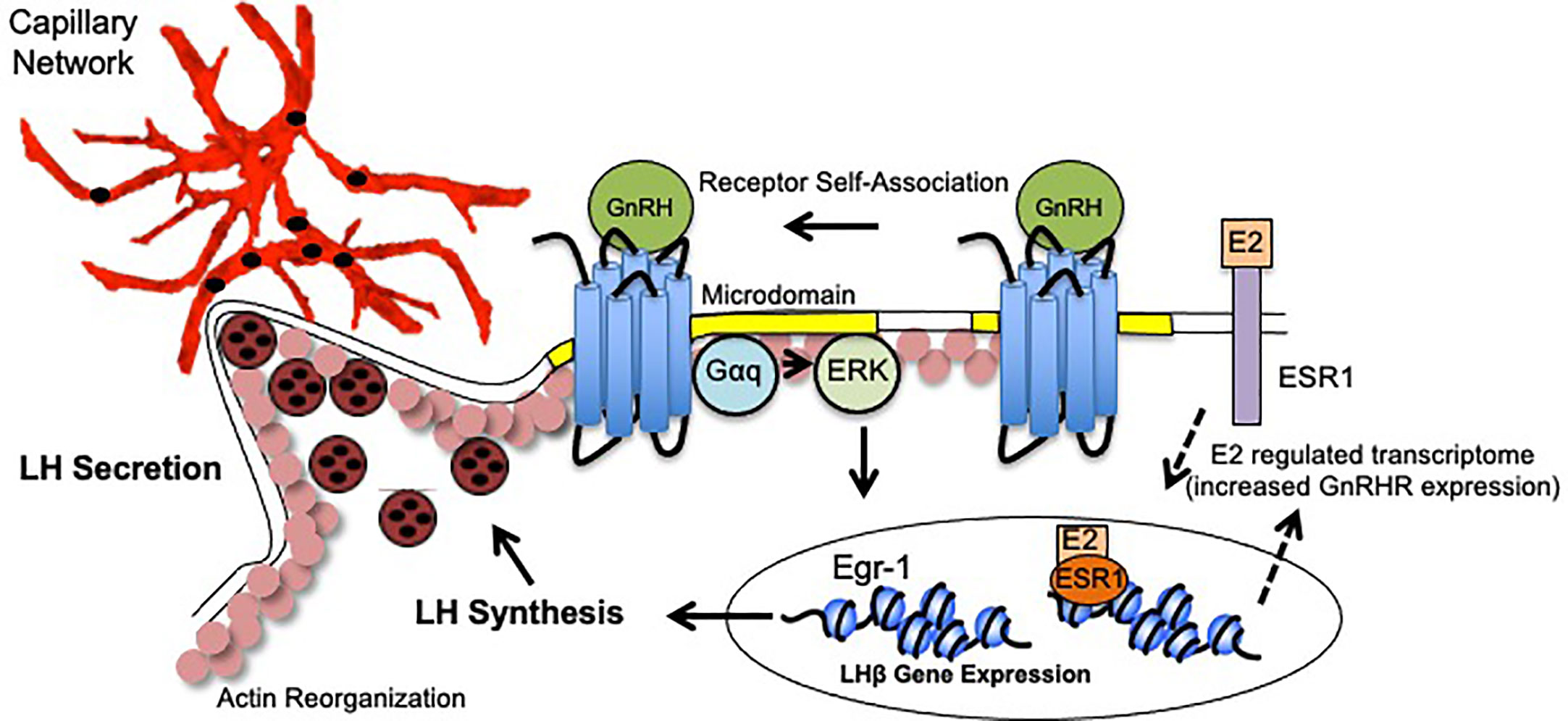
Figure 1 Model of Gonadotrope Dynamics Underlying the LH Surge. During the periovulatory period, ovarian estradiol-17(E2) levels rise. E2 activation of both plasma membrane associated and nuclear ESRI elicits expression of a unique gene program that includes enhanced expression of the GnRH Receptor (GnRHR), and therefore heightened sensitivity to hypothalamic GnRH (dashed arrows). Once expressed, the GnRHR is constitutively localized to microdomains in the plasma membrane (yellow). Following GnRH binding, the GnRHR self-associates to form dimers/oligomers. Second messengers including Gaq/1I and ERK are activated within the microdomains. Increased ERK activity leads to the activation of the immediate early gene Egr-1 which upregulates lhb gene expression. Functional LH is synthesized and packaged into secretory vesicles that embed in the cortical actin cytoskeleton (pink circles). GnRH activation also causes reorganization of the actin cytoskeleton which allows for the gonadotropes to appose the capillary endothelium (red) to release LH into circulation (black circles).
The mechanisms that underlie the surge in LH release from anterior pituitary gonadotropes have fascinated endocrinologists for decades. It is clear that GnRH and E2 are critical regulators of the LH surge, but mechanistic questions remain regarding how these and other hormones induce plasticity at the cellular, molecular, and gonadotrope network level. For example, how the gonadotrope network communicates in vivo to initiate directed movement towards vasculature is still unknown as is the potential role of pituitary cell transdifferentiation during the LH surge. Questions also remain about the role of other steroid regulators, such as progesterone (P4), in inducing the LH surge. Progesterone has historically been thought to suppress gonadotrope function and LH release, although some studies suggest that low P4 levels late in the follicular phase signal the hypothalamus to increase GnRH secretion (83). How this potential mechanism relates directly to gonadotropes requires further studies. Understanding the mechanism that control gonadotrope plasticity is not only of interest at a basic scientific level but also has profound clinical implications from the development of novel birth control and fertility treatments. Future studies will be critical to elucidate the combinatorial effects of endocrine, paracrine, autocrine, and metabolic stimuli on gonadotrope plasticity.
Author Contributions
All authors contributed to the article and approved the submitted version.
Funding
This publication was funded by National Institutes of Health, P20GM103432 and R21HD090541 (BC, AN) and R01HD065943 (CC).
Conflict of Interest
The authors declare that the research was conducted in the absence of any commercial or financial relationships that could be construed as a potential conflict of interest.
Acknowledgments
Our apologies to the many laboratories and investigators who have contributed to the field whose research we did not cite due to space limitations.
Abbreviations
GnRH, gonadotropin releasing hormone; GnRHR, gonadotropin releasing hormone receptor; LH, luteinizing hormone; FSH, follicle stimulating hormone; MAPK, mitogen activated protein kinase; ERK, extracellular signal regulated kinase.
References
1. Schwartz J, Cherny R. Intercellular communication within the anterior pituitary influencing the secretion of hypophysial hormones. Endocr Rev (1992) 13:453–75. doi: 10.1210/er.13.3.453
2. Burgess R, Lunyak V, Rosenfeld M. Signaling and transcriptional control of pituitary development. Curr Opin Genet Dev (2002) 12:534–9. doi: 10.1016/S0959-437X(02)00337-4
3. Maclean DB, Jackson IM. Molecular biology and regulation of the hypothalamic hormones. Baillieres Clin Endocrinol Metab (1988) 2:835–68. doi: 10.1016/S0950-351X(88)80021-1
4. Ordog T, Chen MD, Nishihara M, Connaughton MA, Goldsmith JR, Knobil E. On the role of gonadotropin-releasing hormone (GnRH) in the operation of the GnRH pulse generator in the rhesus monkey. Neuroendocrinology (1997) 65:307–13. doi: 10.1159/000127189
5. Karsch FJ, Bowen JM, Caraty A, Evans NP, Moenter SM. Gonadotropin-releasing hormone requirements for ovulation. Biol Reprod (1997) 56:303–9. doi: 10.1095/biolreprod56.2.303
6. Knobil E. On the control of gonadotropin secretion in the rhesus monkey. Recent Prog Horm Res (1974) 30:1–46. doi: 10.1016/B978-0-12-571130-2.50005-5
7. Belchetz PE, Plant TM, Nakai Y, Keogh EJ, Knobil E. Hypophysial responses to continuous and intermittent delivery of hypopthalamic gonadotropin-releasing hormone. Science (1978) 202:631–3. doi: 10.1126/science.100883
8. Fink G. Gonadotropin secretion and its control. In: Knobil E, Neill J, editors. The Physiology of Reproduction. New York: Raven Press (1988). p. 1349–78.
9. Terasawa E. Steroid modulation of pulsatile LHRH release in the rhesus monkey. Horm Behav (1994) 28:406–16. doi: 10.1006/hbeh.1994.1037
10. Clarke IJ. Evidence that the switch from negative to positive feedback at the level of the pituitary gland is an important timing event for the onset of the preovulatory surge in LH in the ewe. J Endocrinol (1995) 145:271–82. doi: 10.1677/joe.0.1450271
11. Clarke IJ. The preovulatory LH surge A case of a neuroendocrine switch. Trends Endocrinol Metab (1995) 6:241–7. doi: 10.1016/1043-2760(95)95218-T
12. Dungan HM, Clifton DK, Steiner RA. Minireview: kisspeptin neurons as central processors in the regulation of gonadotropin-releasing hormone secretion. Endocrinology (2006) 147:1154–8. doi: 10.1210/en.2005-1282
13. Adams C, Stroberg W, DeFazio RA, Schnell S, Moenter SM. Gonadotropin-Releasing Hormone (GnRH) Neuron Excitability Is Regulated by Estradiol Feedback and Kisspeptin. J Neurosci (2018) 38:1249–63. doi: 10.1523/JNEUROSCI.2988-17.2017
14. Dubois SL, Acosta-Martinez M, DeJoseph MR, Wolfe A, Radovick S, Boehm U, et al. Positive, but not negative feedback actions of estradiol in adult female mice require estrogen receptor alpha in kisspeptin neurons. Endocrinology (2015) 156:1111–20. doi: 10.1210/en.2014-1851
15. Sealfon SC, Laws SC, Wu JC, Gillo B, Miller WL. Hormonal regulation of gonadotropin-releasing hormone receptors and messenger RNA activity in ovine pituitary culture. Mol Endocrinol (1990) 4:1980–7. doi: 10.1210/mend-4-12-1980
16. Sakurai H, Adams BM, Oberbauer AM, Adams TE. Gonadotrope responsiveness in orchidectomized sheep. II. Effect of gonadotropin-releasing hormone amplitude shift during continuous infusion of estradiol. Biol Reprod (1993) 48:683–91. doi: 10.1095/biolreprod48.3.683
17. Nett TM, Crowder ME, Wise ME. Role of estradiol in inducing an ovulatory-like surge of luteinizing hormone in sheep. Biol Reprod (1984) 30:1208–15. doi: 10.1095/biolreprod30.5.1208
18. Gregg DW, Allen MC, Nett TM. Estradiol-induced increase in number of gonadotropin-releasing hormone receptors in cultured ovine pituitary cells. Biol Reprod (1990) 43:1032–6. doi: 10.1095/biolreprod43.6.1032
19. Gregg DW, Nett TM. Direct effects of estradiol-17 beta on the number of gonadotropin-releasing hormone receptors in the ovine pituitary. Biol Reprod (1989) 40:288–93. doi: 10.1095/biolreprod40.2.288
20. Wise ME, Nieman D, Stewart J, Nett TM. Effect of number of receptors for gonadotropin-releasing hormone on the release of luteinizing hormone. Biol Reprod (1984) 31:1007–13. doi: 10.1095/biolreprod31.5.1007
21. Lloyd JM, Childs GV. Changes in the number of GnRH-receptive cells during the rat estrous cycle: biphasic effects of estradiol. Neuroendocrinology (1988) 48:138–46. doi: 10.1159/000125001
22. Alim Z, Hartshorn C, Mai O, Stitt I, Clay C, Tobet S, et al. Gonadotrope plasticity at cellular and population levels. Endocrinology (2012) 153:4729–39. doi: 10.1210/en.2012-1360
23. Navratil AM, Knoll JG, Whitesell JD, Tobet SA, Clay CM. Neuroendocrine plasticity in the anterior pituitary: gonadotropin-releasing hormone-mediated movement in vitro and in vivo. Endocrinology (2007) 148:1736–44. doi: 10.1210/en.2006-1153
24. Scully KM, Rosenfeld MG. Pituitary development: regulatory codes in mammalian organogenesis. Science (2002) 295:2231–5. doi: 10.1126/science.1062736
25. Fontaine R, Ciani E, Haug TM, Hodne K, Ager-Wick E, Baker DM, et al. Gonadotrope plasticity at cellular, population and structural levels: A comparison between fishes and mammals. Gen Comp Endocrinol (2020) 287:113344. doi: 10.1016/j.ygcen.2019.113344
26. Molter-Gerard C, Caraty A, Guerin S, Fontaine J, Taragnat C. Dynamic changes in the gonadotrope cell subpopulations during an estradiol-induced surge in the ewe. Biol Reprod (2000) 63:1084–91. doi: 10.1095/biolreprod63.4.1084
27. Childs GV, Miller BT, Miller WL. Differential effects of inhibin on gonadotropin stores and gonadotropin-releasing hormone binding to pituitary cells from cycling female rats. Endocrinology (1997) 138:1577–84. doi: 10.1210/endo.138.4.5090
28. Childs GV, Unabia G, Lloyd J. Recruitment and maturation of small subsets of luteinizing hormone gonadotropes during the estrous cycle. Endocrinology (1992) 130:335–44. doi: 10.1210/endo.130.1.1727707
29. Childs GV, Unabia G, Lee BL, Rougeau D. Heightened secretion by small and medium-sized luteinizing hormone (LH) gonadotropes late in the cycle suggests contributions to the LH surge or possible paracrine interactions. Endocrinology (1992) 130:345–52. doi: 10.1210/endo.130.1.1727708
30. Qiao S, Nordstrom K, Muijs L, Gasparoni G, Tierling S, Krause E, et al. Molecular Plasticity of Male and Female Murine Gonadotropes Revealed by mRNA Sequencing. Endocrinology (2016) 157:1082–93. doi: 10.1210/en.2015-1836
31. Childs GV. Division of labor among gonadotropes. Vitam Horm (1995) 50:215–86. doi: 10.1016/S0083-6729(08)60657-3
32. Childs GV. Growth hormone cells as co-gonadotropes: partners in the regulation of the reproductive system. Trends Endocrinol Metab (2000) 11:168–75. doi: 10.1016/S1043-2760(00)00252-6
33. Ho Y, Hu P, Peel MT, Chen S, Camara PG, Epstein DJ, et al. Single-cell transcriptomic analysis of adult mouse pituitary reveals sexual dimorphism and physiologic demand-induced cellular plasticity. Protein Cell (2020) 11:565–83. doi: 10.1007/s13238-020-00705-x
34. Zhu X, Gleiberman AS, Rosenfeld MG. Molecular physiology of pituitary development: signaling and transcriptional networks. Physiol Rev (2007) 87:933–63. doi: 10.1152/physrev.00006.2006
35. Childs GV. Development of gonadotropes may involve cyclic transdifferentiation of growth hormone cells. Arch Physiol Biochem (2002) 110:42–9. doi: 10.1076/apab.110.1.42.906
36. Nunez L, Villalobos C, Senovilla L, Garcia-Sancho J. Multifunctional cells of mouse anterior pituitary reveal a striking sexual dimorphism. J Physiol (2003) 549:835–43. doi: 10.1113/jphysiol.2003.040758
37. Fletcher PA, Smiljanic K, Maso Previde R, Iben JR, Li T, Rokic MB, et al. Cell Type- and Sex-Dependent Transcriptome Profiles of Rat Anterior Pituitary Cells. Front Endocrinol (Lausanne) (2019) 10:623. doi: 10.3389/fendo.2019.00623
38. Legan SJ, Karsch FJ. A daily signal for the LH surge in the rat. Endocrinology (1975) 96:57–62. doi: 10.1210/endo-96-1-57
39. Turzillo AM, DiGregorio GB, Nett TM. Messenger ribonucleic acid for gonadotropin-releasing hormone receptor and numbers of gonadotropin-releasing hormone receptors in ovariectomized ewes after hypothalamic-pituitary disconnection and treatment with estradiol. J Anim Sci (1995) 73:1784–8. doi: 10.2527/1995.7361784x
40. Davis TL, Whitesell JD, Cantlon JD, Clay CM, Nett TM. Does a nonclassical signaling mechanism underlie an increase of estradiol-mediated gonadotropin-releasing hormone receptor binding in ovine pituitary cells? Biol Reprod (2011) 85:770–8. doi: 10.1095/biolreprod.111.091926
41. Odle AK, Benes H, Melgar Castillo A, Akhter N, Syed M, Haney A, et al. Association of Gnrhr mRNA With the Stem Cell Determinant Musashi: A Mechanism for Leptin-Mediated Modulation of GnRHR Expression. Endocrinology (2018) 159:883–94. doi: 10.1210/en.2017-00586
42. Kucherov A, Polotsky AJ, Menke M, Isaac B, McAvey B, Buyuk E, et al. Aromatase inhibition causes increased amplitude, but not frequency, of hypothalamic-pituitary output in normal women. Fertil Steril (2011) 95:2063–6. doi: 10.1016/j.fertnstert.2011.01.158
43. Jain A, Polotsky AJ, Rochester D, Berga SL, Loucks T, Zeitlian G, et al. Pulsatile luteinizing hormone amplitude and progesterone metabolite excretion are reduced in obese women. J Clin Endocrinol Metab (2007) 92:2468–73. doi: 10.1210/jc.2006-2274
44. Odle AK, Akhter N, Syed MM, Allensworth-James ML, Benes H, Melgar Castillo A II, et al. Leptin Regulation of Gonadotrope Gonadotropin-Releasing Hormone Receptors As a Metabolic Checkpoint and Gateway to Reproductive Competence. Front Endocrinol (Lausanne) (2017) 8:367. doi: 10.3389/fendo.2017.00367
45. Jones K, Ryan S, Carlson NE, Chosich J, Bradford AP, Santoro N, et al. Aromatase Inhibition Ameliorates Decreased LH Output Found in Obese Women. Reprod Sci (2020) 27:1018–23. doi: 10.1007/s43032-019-00105-5
46. Al-Safi ZA, Liu H, Carlson NE, Chosich J, Lesh J, Robledo C, et al. Estradiol Priming Improves Gonadotrope Sensitivity and Pro-Inflammatory Cytokines in Obese Women. J Clin Endocrinol Metab (2015) 100:4372–81. doi: 10.1210/jc.2015-1946
47. Murtazina DA, Arreguin-Arevalo JA, Cantlon JD, Ebrahimpour-Boroojeny A, Shrestha A, Hicks JA, et al. Enrichment of ovine gonadotropes via adenovirus gene targeting enhances assessment of transcriptional changes in response to estradiol-17 betadagger. Biol Reprod (2020) 102:156–69. doi: 10.1093/biolre/ioz166
48. Kaiser UB, Conn PM, Chin WW. Studies of gonadotropin-releasing hormone (GnRH) action using GnRH receptor-expressing pituitary cell lines. Endocr Rev (1997) 18:46–70. doi: 10.1210/edrv.18.1.0289
49. Stanislaus D, Janovick JA, Brothers S, Conn PM. Regulation of G(q/11)alpha by the gonadotropin-releasing hormone receptor. Mol Endocrinol (1997) 11:738–46. doi: 10.1210/mend.11.6.0005
50. Naor Z, Benard O, Seger R. Activation of MAPK cascades by G-protein-coupled receptors: the case of gonadotropin-releasing hormone receptor. Trends Endocrinol Metab (2000) 11:91–9. doi: 10.1016/S1043-2760(99)00232-5
51. Naor Z. Signaling by G-protein-coupled receptor (GPCR): studies on the GnRH receptor. Front Neuroendocrinol (2009) 30:10–29. doi: 10.1016/j.yfrne.2008.07.001
52. Kanasaki H, Bedecarrats GY, Kam KY, Xu S, Kaiser UB. Gonadotropin-releasing hormone pulse frequency-dependent activation of extracellular signal-regulated kinase pathways in perifused L{beta}T2 cells. Endocrinology (2005) 146:5503–13. doi: 10.1210/en.2004-1317
53. Bliss SP, Navratil AM, Xie J, Roberson MS. GnRH signaling, the gonadotrope and endocrine control of fertility. Front Neuroendocrinol (2010) 31(3):322–40. doi: 10.1016/j.yfrne.2010.04.002
54. Thackray VG, Mellon PL, Coss D. Hormones in synergy: regulation of the pituitary gonadotropin genes. Mol Cell Endocrinol (2010) 314:192–203. doi: 10.1016/j.mce.2009.09.003
55. Bliss SP, Miller A, Navratil AM, Xie J, McDonough SP, Fisher PJ, et al. ERK signaling in the pituitary is required for female but not male fertility. Mol Endocrinol (2009) 23:1092–101. doi: 10.1210/me.2009-0030
56. Dorn C, Ou Q, Svaren J, Crawford PA, Sadovsky Y. Activation of luteinizing hormone beta gene by gonadotropin-releasing hormone requires the synergy of early growth response-1 and steroidogenic factor-1. J Biol Chem (1999) 274:13870–6. doi: 10.1074/jbc.274.20.13870
57. Lee SL, Sadovsky Y, Swirnoff AH, Polish JA, Goda P, Gavrilina G, et al. Luteinizing hormone deficiency and female infertility in mice lacking the transcription factor NGFI-A (Egr-1). Science (1996) 273:1219–21. doi: 10.1126/science.273.5279.1219
58. Navratil AM, Bliss SP, Berghorn KA, Haughian JM, Farmerie TA, Graham JK, et al. Constitutive localization of the gonadotropin-releasing hormone (GnRH) receptor to low density membrane microdomains is necessary for GnRH signaling to ERK. J Biol Chem (2003) 278:31593–602. doi: 10.1074/jbc.M304273200
59. Helms JB, Zurzolo C. Lipids as targeting signals: lipid rafts and intracellular trafficking. Traffic (2004) 5:247–54. doi: 10.1111/j.1600-0854.2004.0181.x
60. Pike LJ. Lipid rafts: bringing order to chaos. J Lipid Res (2003) 44:655–67. doi: 10.1194/jlr.R200021-JLR200
61. Bliss SP, Navratil AM, Breed M, Skinner DC, Clay CM, Roberson MS. Signaling complexes associated with the type I gonadotropin-releasing hormone (GnRH) receptor: colocalization of extracellularly regulated kinase 2 and GnRH receptor within membrane rafts. Mol Endocrinol (2007) 21:538–49. doi: 10.1210/me.2006-0289
62. Horvat RD, Roess DA, Nelson SE, Barisas BG, Clay CM. Binding of agonist but not antagonist leads to fluorescence resonance energy transfer between intrinsically fluorescent gonadotropin-releasing hormone receptors. Mol Endocrinol (2001) 15:695–703. doi: 10.1210/mend.15.5.0633
63. Cornea A, Janovick JA, Maya-Nunez G, Conn PM. Gonadotropin-releasing hormone receptor microaggregation. Rate monitored by fluorescence resonance energy transfer. J Biol Chem (2001) 276:2153–8. doi: 10.1074/jbc.M007850200
64. Viola A, Gupta N. Tether and trap: regulation of membrane-raft dynamics by actin-binding proteins. Nat Rev Immunol (2007) 7:889–96. doi: 10.1038/nri2193
65. Chichili GR, Rodgers W. Cytoskeleton-membrane interactions in membrane raft structure. Cell Mol Life Sci (2009) 66:2319–28. doi: 10.1007/s00018-009-0022-6
66. Meiri KF. Membrane/cytoskeleton communication. Subcell Biochem (2004) 37:247–82. doi: 10.1007/978-1-4757-5806-1_8
67. Dang AK, Murtazina DA, Magee C, Navratil AM, Clay CM, Amberg GC. GnRH evokes localized subplasmalemmal calcium signaling in gonadotropes. Mol Endocrinol (2014) 28:2049–59. doi: 10.1210/me.2014-1208
68. Navratil AM, Dozier MG, Whitesell JD, Clay CM, Roberson MS. Role of cortactin in dynamic actin remodeling events in gonadotrope cells. Endocrinology (2014) 155:548–57. doi: 10.1210/en.2012-1924
69. Adams TE, Nett TM. Interaction of GnRH with anterior pituitary. III. Role of divalent cations, microtubules and microfilaments in the GnRH activated gonadotroph. Biol Reprod (1979) 21:1073–86. doi: 10.1095/biolreprod21.5.1073
70. Izumi T, Kasai K, Gomi H. Secretory vesicle docking to the plasma membrane: molecular mechanism and functional significance. Diabetes Obes Metab (2007) 9 Suppl 2:109–17. doi: 10.1111/j.1463-1326.2007.00789.x
71. Porat-Shliom N, Milberg O, Masedunskas A, Weigert R. Multiple roles for the actin cytoskeleton during regulated exocytosis. Cell Mol Life Sci (2013) 70:2099–121. doi: 10.1007/s00018-012-1156-5
72. Ibrahim SN, Moussa SM, Childs GV. Morphometric studies of rat anterior pituitary cells after gonadectomy: correlation of changes in gonadotropes with the serum levels of gonadotropins. Endocrinology (1986) 119:629–37. doi: 10.1210/endo-119-2-629
73. Childs GV. Functional ultrastructure of gonadotropes: a review. Curr Topics Neuroendocrinol (1986) 7:49–97. doi: 10.1007/978-3-642-71461-0_2
74. Le Tissier PR, Hodson DJ, Lafont C, Fontanaud P, Schaeffer M, Mollard P. Anterior pituitary cell networks. Front Neuroendocrinol (2012) 33:252–66. doi: 10.1016/j.yfrne.2012.08.002
75. Hodson DJ, Mollard P. Pituitary endocrine cell networks - 10 years and beyond. Ann Endocrinol (2012) 73:56–8. doi: 10.1016/j.ando.2012.03.033
76. Mollard P, Hodson DJ, Lafont C, Rizzoti K, Drouin J. A tridimensional view of pituitary development and function. Trends Endocrinol Metab (2012) 23:261–9. doi: 10.1016/j.tem.2012.02.004
77. McNeilly AS, Crawford JL, Taragnat C, Nicol L, McNeilly JR. The differential secretion of FSH and LH: regulation through genes, feedback and packaging. Reprod Suppl (2003) 61:463–76.
78. Hodson DJ, Mollard P. Navigating pituitary structure and function - defining a roadmap for hormone secretion. J Neuroendocrinol (2013) 25:674–5. doi: 10.1111/jne.12041
79. Schaeffer M, Hodson DJ, Meunier AC, Lafont C, Birkenstock J, Carmignac D, et al. Influence of estrogens on GH-cell network dynamics in females: a live in situ imaging approach. Endocrinology (2011) 152:4789–99. doi: 10.1210/en.2011-1430
80. Gregg DW, Schwall RH, Nett TM. Regulation of gonadotropin secretion and number of gonadotropin-releasing hormone receptors by inhibin, activin-A, and estradiol. Biol Reprod (1991) 44:725–32. doi: 10.1095/biolreprod44.4.725
81. Currie RJ, McNeilly AS. Mobilization of LH secretory granules in gonadotrophs in relation to gene expression, synthesis and secretion of LH during the preovulatory phase of the sheep oestrous cycle. J Endocrinol (1995) 147:259–70. doi: 10.1677/joe.0.1470259
82. Thomas SG, Clarke IJ. The positive feedback action of estrogen mobilizes LH-containing, but not FSH-containing secretory granules in ovine gonadotropes. Endocrinology (1997) 138:1347–50. doi: 10.1210/endo.138.3.5104
Keywords: gonadotrope, gonadotropin releasing hormone receptor, plasticity, luteinizing hormone, luteinizing hormone surge, fertility
Citation: Clay CM, Cherrington BD and Navratil AM (2021) Plasticity of Anterior Pituitary Gonadotrope Cells Facilitates the Pre-Ovulatory LH Surge. Front. Endocrinol. 11:616053. doi: 10.3389/fendo.2020.616053
Received: 10 October 2020; Accepted: 15 December 2020;
Published: 04 February 2021.
Edited by:
Finn-Arne Weltzien, Norwegian University of Life Sciences, NorwayReviewed by:
Philippa Melamed, Technion Israel Institute of Technology, IsraelTrude Marie Haug, University of Oslo, Norway
Copyright © 2021 Clay, Cherrington and Navratil. This is an open-access article distributed under the terms of the Creative Commons Attribution License (CC BY). The use, distribution or reproduction in other forums is permitted, provided the original author(s) and the copyright owner(s) are credited and that the original publication in this journal is cited, in accordance with accepted academic practice. No use, distribution or reproduction is permitted which does not comply with these terms.
*Correspondence: Amy M. Navratil, anavrati@uwyo.edu