- 1Department of Clinical and Experimental Medicine, University of Messina, Messina, Italy
- 2Master Program on Childhood, Adolescent and Women’s Endocrine Health, University of Messina, Messina, Italy
- 3Interdepartmental Program of Molecular & Clinical Endocrinology and Women’s Endocrine Health, University Hospital, A.O.U. Policlinico G. Martino, Messina, Italy
Pharmacological interference on L-thyroxine (L-T4) therapy can be exerted at several levels, namely from the hypothalamus/pituitary through the intestine, where the absorption of exogenous L-T4 takes place. A number of medications interfere with L-T4 therapy, some of them also being the cause of hypothyroidism. The clinician should be aware that some medications simply affect thyroid function tests with no need of modifying the dose of L-T4 that the patient was taking prior to their prescription. Usually, the topic of pharmacological interference on L-T4 therapy addresses the patient with primary hypothyroidism, in whom periodic measurement of serum thyrotropin (TSH) is the biochemical target. However, this minireview also addresses the patient with central hypothyroidism, in whom the biochemical target is serum free thyroxine (FT4). This minireview also addresses two additional topics. One is the costs associated with frequent monitoring of the biochemical target when L-T4 is taken simultaneously with the interfering drug. The second topic is the issue of metabolic/cardiovascular complications associated with undertreated hypothyroidism.
Introduction
Prevalence of undiagnosed primary hypothyroidism (PH) in Europe is 4.76% (95% CI 2.98–6.79%), precisely 4.11% (3.05–5.31%) for subclinical hypothyroidism (SCH) and 0.65% (0.38–0.99%) for overt hypothyroidism (OH) (1), paralleling the American prevalence of 4.67, 4.34, and 0.3%, respectively (2). PH prevails in females, and in those aged ≥65 years (1). Central hypothyroidism (CH) was considered 1,000-fold rarer than PH, but it may be just 20-fold rarer (3). L-thyroxine (L-T4) monotherapy is the standard treatment for PH (4–6) and CH (4, 5, 7), and SCH patients candidate for replacement (4, 5, 8).
Thus, L-T4 is one top prescribed medication (9). However, ≈20% of patients with PH (10–13) and ≈20% (14) or 40% (15) of patients with CH are undertreated, co-administration of interfering medications (IM) being one major cause. Of 2,292 patients with PH (12), 42.7% had abnormal serum TSH (28.3% undertreated and 14.4% overtreated). Strikingly, only 52.1% of patients were told not to take L-T4 along with IM (12).
As mentioned below, there may be consequences on health from undertreated hypothyroidism (UTH).
The Pharmacological Interference on L-T4 Therapy
Such interference was covered by reviews (11, 16–19) chapters of books (10, 20–22), and guidelines (4, 5) with pertinent recommendations (4, 5) shown in Table 1.
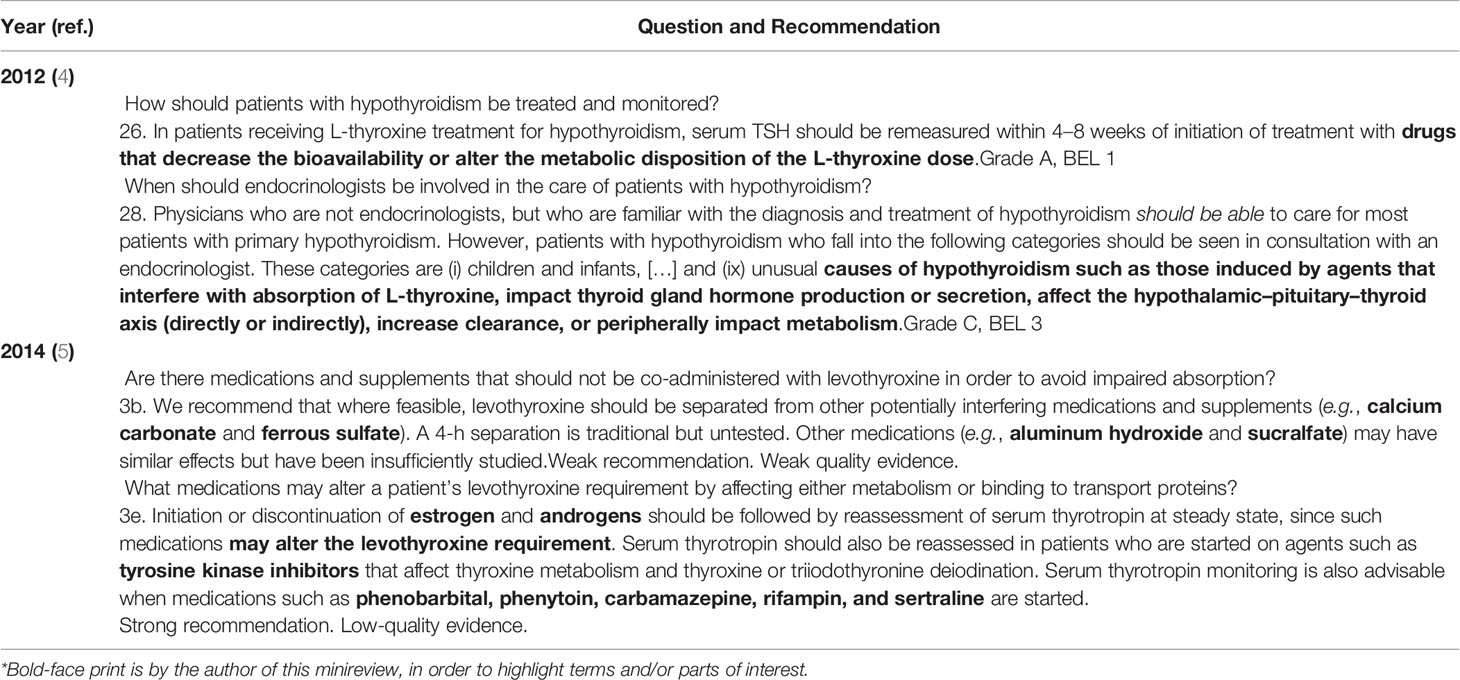
Table 1 Recommendations concerning medications that interfere with L-T4 therapy in the latest American Thyroid Association Guidelines on hypothyroidism*.
Table 2 summarizes the mechanism(s) of interference and suggestions. The next paragraphs illustrate the author’s experience. Of 210 L-T4-treated adult patients (tablet formulations) who were referred to SB in one year, 27 (13%) had UTH (11). In 8/27 (30%; 4% of 210), the explanation was pharmacological, all eight taking ≥1 drug that causes L-T4 malabsorption (proton-pump inhibitors [PPIs], calcium carbonate, ferrous sulfate) (11). A study with practitioners enrolled L-T4-treated PH (tablet formulations) that, for ≥2 years was not associated with drugs causing L-T4 malabsorption and that, for another ≥2 years, it was (23). Of 10,496 persons, 730 (7.0%) had L-T4-treated PH, of whom 391 (5.4%; 3.7% of 10,496) were taking those IM, with 114 enrolled (age 65.6 ± 12.7 years). PPIs were the leading IM (95/114 [83%]), either alone (n = 71 [62%]) or associated with other IM (n = 24 [21%]), followed by calcium salts (18/114 [16%]).
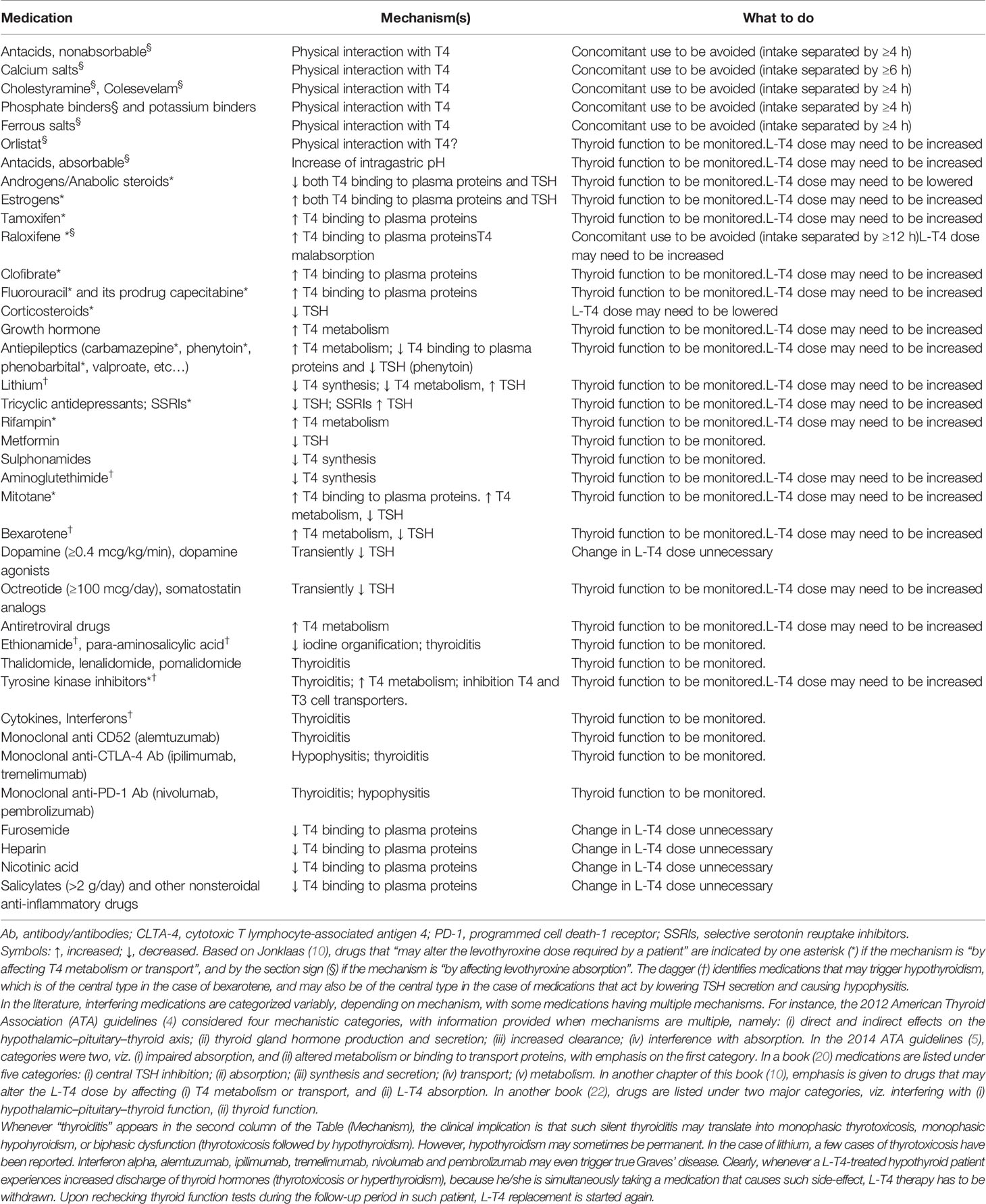
Table 2 Medications that may interfer on with L-T4 therapy and suggestions for management in addition to monitoring of thyroid function.
Another study evaluated changes in number of L-T4 prescriptions and dose of L-T4 before and during exposure to potential drug–drug interactions (DDIs) (24). In 5,426 L-T4 users aged ≥18 years (7.5% of persons under care), prescriptions and doses of L-T4 increased during exposure by 6 and 5%, respectively, suggesting that clinicians increase the number of L-T4 prescriptions to achieve TSH levels as low as those before DDIs (24).
A retrospective study evaluated TSH changes in 10,999 Scottish residents who were prescribed L-T4 before starting IM (25). Iron, calcium, PPI, and estrogens increased TSH significantly, with an increase >5 mU/L in 7.5, 4.4, 5.6, and 4.3% patients, respectively. TSH decreased significantly (0.17 mU/L) in patients on statins and changed insignificantly in patients on H2 receptor antagonists or glucocorticoids (25).
Before continuing, SB informs that this minireview disregards the endocrine disruptors, and does not emphasize clinically inconsequential IM. Such medications are those listed at the end of Table 2 (furosemide, heparin, salycylates, and other nonsteroidal anti-inflammatory drugs), which inhibit T4 binding to plasma proteins thus increasing serum FT4, and the unlisted propranolol (20). Also, a number of articles are dedicated to specific IM (26–40).
Thyroid Dysfunction and Suboptimal Treatment Caused by Interfering Medications Occur in a Proportion of Patients
The Scottish study (25) is important because it reminds that not all L-T4-replaced hypothyroid patients taking IM become undertreated. For instance, a 3-month supplementation of 1,200 mg/d calcium carbonate reversibly increased TSH in 13/20 patients (65%), with TSH >4.0 mU/L in four (20%) (41). Overall, TSH increased from 1.60 ± 0.22 to 2.71 ± 0.43 mU/L (+69%). Calcium carbonate adsorbed T4 dose-dependently at pH 2 but not pH 7.4, thus explaining L-T4 malabsorption (41), and reduced T4 pharmacokinetics (42). Lower L-T4 bioavailability applies to other calcium salts (43). Those data (41) match data of SB (44). Fifty postmenopausal women with L-T4-treated PH started taking 600–1000 mg/d calcium carbonate ≤2 h after L-T4. UTH (TSH >4.12 mU/L) occurred in 9/50 women (18%). Overall, TSH increased from 1.93 ± 0.51 mU/L to 3.33 ± 1.93 mU/L (+73%), but when all women took calcium 6–8 h after L-T4, all had TSH <4.12 mU/L (2.16 ± 0.54 mU/L) (44).
Confirming previous data (45), in 37 PH patients under stable L-T4 replacement and in whom PPIs were administered subsequently, TSH increased by 28%, with seven patients (19%) having post-PPI TSH levels >5 mU/L (46). A mean increase of 20 μg/d L-T4 (+35%) was necessary (46).
In 71 L-T4-treated PH patients who started the antituberculosis drug rifampin, an increased L-T4 dose was required for 50% of 46 patients (TSH-suppressive group) and 26% of 25 patients (replacement group) (47). Lack of thyroid remnant, time interval between starting rifampin and TSH measurement, and baseline L-T4 dose/kg body weight were significant risks for UTH (47). Ethionamide and para-aminosalycilic acid (PAS) are used in multidrug-resistant tuberculosis (MDR-TB). After initial reports (48–50), a meta-analysis on 6,241 MDR-TB patients (31) showed that PH prevalence in MDR-TB patients averaged 17.0%, with ethionamide and PAS were the most frequently reported drugs associated with hypothyroidism. Tubercolosis is a common opportunistic infection in HIV-seropositive persons, and antiretroviral therapy (ART) may induce PH. Of 69 HIV-infected MDR-TB patients under anti-TB and antiretroviral therapies, 37 (54%) had PH (51). Co-administration of PAS and ethionamide doubled the risk of hypothyroidism (RR = 1.93) (51). In MDR-TB patients receiving anti-TB, one-fourth developed PH: 32% in patients who received a regimen containing ethionamide, 35% in patients who received a regimen containing PAS, and 44% in HIV-positive patients on ART (52).
Immune reconstitution therapy (IRT)-induced either autoimmune hyperthyroidism with/without ophthalmopathy or autoimmune hypothyroidism may occur following: (i) highly active antiretroviral therapy (HAART) of HIV infection, (ii) alemtuzumab treatment for active relapsing-remitting multiple sclerosis, and (iii) allogeneic bone marrow transplantation or hematopoietic stem cell transplantation (30). No recommendation for managing IRT-related thyropathies addressed possible changes in L-T4 doses (30). Hypothyroid HAART-treated patients needing increased L-T4 doses were reported (53–55). HAART drugs were ritonavir (48), abacavir–lamivudine and lopinavir–ritonavir (54), and lopinavir/ritonavir plus zidovudine and lamivudine (55). Switch from lopinavir/ritonavir to nelfinavir failed to achieve euthyroidism (55), which was restored when dolutegravir was substituted for lopinavir–ritonavir (54). However, 826 well-treated HIV-infected persons, 95% of whom with undetectable viral replication, did not differ from 2,503 uninfected controls for prevalence of hyperthyroidism (0.8 vs. 0.8%) or hypothyroidism (3.8 vs. 4.6%) (56).
Hypothyroidism occurs in around 20 or 5–10% of thalidomide- or lenalomide-treated patients (57, 58). Of 170 patients under lenalidomide, 6% had thyroid dysfunction (hypothyroidism > thyrotoxicosis), which prevailed in patients with known vs. unknown thyroid dysfunction (17 vs 6%) (58). Unfortunately, the article fails to specify how many patients were under L-T4 and how many needed increased L-T4 doses (58). With just two cases reported (59, 60), the rate of pomalidomide-induced hypothyroidism is unknown. Interestingly, thyroid hormone autoantibodies appeared in 14% of thalidomide or lenalidomide-treated patients (61).
Autoimmune thyroiditis is also caused by monoclonal Ab to programmed cell death-1 receptor (nivolumab and pembrolizumab), and Ab to cytotoxic T lymphocyte-associated antigen-4 (ipilimumab, tremelimumab) (27, 37–39). Rates of hypothyroidism are 0–40% (nivolumab), 0–11.5% (pembrolizumab), 0–9% (ipilimumab), and 0–5% (tremelimumab) (38).
Tyrosine kinase inhibitors (TKI) may cause de novo hyperthyroidism or hypothyroidism, or worsen pre-existing hypothyroidism and increase L-T4 requirements (27–29). Upgraded titration of L-T4 was reported in athyreotic patients prescribed imatinib, motesanib, sorafenib, sunitinib, and vandetanib (5, 62, 63). Nilotinib and dasatanib rarely alter L-T4 requirements (27). One mechanism for the L-T4 increased requirements, further to T4 increased metabolism, can be inhibition of MCT8-dependent T3 and T4 uptake (64), since MCT8 is expressed by the gastrointestinal tract (65).
Other anti-cancer IM are mitotane (see below) and aminogluthetimide, a first-generation aromatase inhibitor used for advanced breast cancer (ABC), but whose side-effects and need for concomitant hydrocortisone limited its utility (66). Of 29 aminoglutethimide-treated patients with prostate cancer, nine (31%) developed hypothyroidism (TSH >10 mU/L) (67). Of 32 women under aminoglutethimide + hydrocortisone for ABC, 17 (53%) had hyperthyrotropinemia before treatment. In 15/17 patients, TSH increased further, with seven developing OH (68). Because aminoglutethimide blocks adrenal steroidogenesis, it is used to treat Cushing’s syndrome (69).
OH or SCH develop in up to 15 or 34% of lithum-treated patients (20), the annual rate of developing PH being 1.5%, (6.4% in thyroid antibody-positive and 0.8% in thyroid antibody-negative individuals) (70). Women <60-year-old are at greatest risk to develop thyroid disease (71). Indeed, women develop OH or SCH three times more frequently than men (25.8 vs. 8.7%), with prevalence among women exceeding 50% by the age of 65 years (35). During tricyclic antidepressant therapy, TSH levels are unchanged, and T4 and FT4 levels decrease within the euthyroid range, though other studies reported unchanged thyroid function tests (TFTs) (72). Selective serotonin reuptake inhibitors (SSRIs) affect TFTs variably, usually with no or downward changes of FT4 and FT3, and no or upward change of TSH within the corresponding reference range (72). L-T4 requirements increased in nine L-T4-treated hypothyroid patients under sertraline (73). Confirming the sertraline-T4 interaction, in two patients under TSH-suppressive L-T4 therapy, TSH levels rose into the normal range (73). A 3-month duration study was conducted in 57 patients with major depression and 10 control patients (72). The study patients were hypothyroid on adequate L-T4 therapy (n = 28) who were randomized to fluoxetine (n = 13) or sertraline (n = 15), and euthyroid (n = 29) who were treated with fluoxetine (n = 15) or sertraline (n = 14). Controls had hypothyroidism on adequate L-T4 therapy without depression (72). No changes occurred in the L-T4-replaced hypothyroids under either SSRI. In response to a letter (74) commenting that difference with the early study (73) could be that” many … patients … were athyreotic”, the authors admited that “in most [patients] the cause of hypothyroidism was autoimmune” (75). Noteworthy, a 41-year-old man with a history of bipolar disorder and schizophrenia had myxedema coma after therapy with sertraline and ariprazole (76). After discharge, TSH remained high (34 IU/ml) on sertraline, ariprazole and 200 µg/d L-T4.
SCH may occur reversibly following administration of antiepileptic drugs (AED), particularly carbamazepine, phenytoin, valproate (77), oxcarbazepine, but not lamotrigine, levitracetam, tiagabine and vigabatrine (36). AED administration in L-T4-replaced patients with either PH (78) or CH (32) requires higher L-T4 doses.
The anti-obesity drug orlistat inhibits gastro-intestinal lipases and is minimally absorbed. Based on data of the UK Medicines Information pharmacists (79), only two cases of interaction between orlistat and L-T4 were reported (80, 81), most likely via T4 malabsorption. It was recommended that L-T4 and orlistat “should be separated by 4 hours, and increased monitoring of […] thyroid hormone levels may be prudent” (79).
Through acceleration of both thyroid hormone and cortisol metabolism, initiation of growth hormone (GH) replacement may unmask CH and hypoadrenalism (82–84). Also, patients already under L-T4 replacement need increased L-T4 doses (82, 84). Unmasking of CH and increased L-T4 doses occurred in 30/84 (36%) and 25/159 (16%) patients, respectively (84).
Pharmacological Interference at Central Level
The antipsycotic dopamine antagonists (e.g., phenothiazines, haloperidol, domperidone, metoclopramide, cimetidine), antidepressive tricyclics and α-methyldopa slightly increase serum TSH without altering thyroid function (17). Though dopamine and its agonists (bromocriptine, cabergoline), somatostatin analogs (octreotide), dobutamine, amphetamines and corticosteroids inhibit TSH, patients taking chronically these medications have no sustained decreases of TFTs. Consequently, CH does not develop (34, 85). Reversible inhibition of TSH secretion is also observed with AED, particularly phenytoin (20).
Anti-CTLA-4 Ab and anti-PD-1 Ab induce autoimmune hypophysitis (27, 37–39), with rates of 0–17.4% (ipilimumab), 0–2.6% (tremelimumab), 0–0.9% (nivolumab), and 0–1.2% (pembrolizumab) (38). In anti-CTLA4-Ab-induced hypophysitis, ACTH- and TSH-deficiency are frequent (38).
Metformin lowers serum TSH in L-T4-treated OH and L-T4-untreated SCH patients, but not in euthyroid patients (33). After its chronic administration, FT4 was unchanged and TSH decreased in L-T4-treated or L-T4-untreated hypothyroid diabetics, but not in euthyroid subjects (86).
A single oral dose of clofibrate decreased significantly hyperthyrotropinemia of PH patients (87). Clofibrate did not change discernibly basal and TRH-induced TSH secretion in euthyroids. Similar results were given by meclofenoxate hydrochloride (87), suggesting that both drugs inhibit TSH secretion in PH patients possibly acting on the hypothalamus/pituitary (87). Clofibrate also increased serum T4-binding capacity of TBG, lowering serum FT4 in 11/12 hyperlipoproteinemic patients (92%) (88).
The adrenocytolytic drug mitotane is used to treat adrenocortical carcinoma (ACC) (89). In 17 patients with radically resected ACC, mitotane was administered associated with glucocorticoid replacement therapy (90). Excluding three patients under L-T4 treatment and one with SCH, during the first year, FT4 became subnormal in 12/13 evaluable patients (92%), with L-T4 replacement started after 9 months in four. At last follow-up, FT4 levels were unchanged compared with the 12 month-evaluation, but another three patients needed L-T4 replacement. Five women with mitotane-treated ACC showed features of CH, namely low FT4, normal FT3 and TSH, with impaired TSH response to TRH (91). Mitotane increased serum FT3/FT4 ratio, suggesting enhanced T4 to T3 conversion, a compensatory mechanism of hypothyroidism (91). CH was reported in a girl with mitotane-treated ACC (92); full restoration of FT4 required increasing the L-T4 dose. After completing chemotherapy, TFTs remained normal, and L-T4 replacement was discontinued.
The retinoid bexarotene causes CH in almost all patients (22), via TSH suppression (93) and non-deiodinase-mediated thyroid hormone increased metabolism (94).
Hypothyroid Patients Hospitalized in Intensive Care Units (ICU)
Among 133 such patients, TFTs were not performed in 29 (21.8%), replacement therapy was not prescribed for >7 days in 23 (17.3%) and was omitted in three (2.2%) (95). Nine hypothyroid patients who stayed at ICU for 12–45 days received H2-blockers as routine prophylaxis against gastroduodenal bleeding and were nasogastrically fed a calorically dense solution (96). This solution was stopped 2 h before and resumed 2 h after L-T4 administration (crushed tablets) nasogastrically. After the first 4–8 days at ICU, with L-T4 doses unchanged, TSH of all patients increased significantly to 5.69 mU/L (IQR = 3.87–6.83) from 1.52 mU/L (IQR = 0.79–3.8) at admission. L-T4 dose was increased in 8/9 patients (88.9%) from 86.1 ± 41.6 to 125 ± 39.5 µg/d (54.4 ± 31.6%). Upon discharge, TSH returned to levels (2.8 mU/L [IQR = 1.4–5.5]) insignificantly different from baseline. The increased L-T4 requirements were attributed to increased gastric pH by H2-blockers (and subsequent decreased L-T4 absorption) and increased T4 to reverse-T3 shunting (due to enhanced type-III deiodinase activity during critical illnesses) (96). In 320 ICU patients, the most frequent clinically significant drug–enteral nutrition interactions concerned phenytoin, warfarin and L-T4 (97).
Current Research Gaps
Concerning IM-induced UTH, few data are available on two issues (23, 44, 98, 99): (i) metabolic/cardiovascular complications, (ii) costs of frequent assays to monitor L-T4 therapy.
In the aforementioned study on 114 L-T4-treated PH patients (23), exposure to IM (32.1 ± 6.9 months) significantly increased TSH from 1.27 ± 1.34 to 2.81 ± 3.62 mU/L (+121%), and proportions of TSH >4.12 mU/L from 4.7 to 18.5% and >2.50 mU/L from 20.2 to 53.5% compared to the non-exposure period (35.4 ± 9.7 months). Some complications ensued: aggravation of pre-existing or de novo onset of any of metabolic syndrome (MS), impaired fasting glycemia (IFG), diabetes, dyslipidemia, hypertension, cardiovascular disease (CVD). Seventy-six patients (67%) had complications, whose rates of TSH >4.12 or >2.50 mU/L were significantly greater than in the 36 complication-free patients (22 vs. 11%, or 35 vs. 17%). TSH in patients with complications vs. complication-free patients were 118% greater during exposure (3.44 ± 4.08 vs. 1.58 ± 1.98 mU/L) (23). In the above study on 50 postmenopausal L-T4-treated PH women (44), TSH increased significantly from 1.93 ± 0.51 (before calcium; setting 1) to 3.33 ± 1.93 mU/L (calcium taken ≤2 h after L-T4; setting 2), and was significantly lower than 2.16 ± 0.54 mU/L (calcium taken 6–8 h after L-T4; setting 3). Total cholesterolemia (TC), fasting glycemia (FG), systolic and diastolic blood pressure (SBP and DBP) were also significantly higher in setting 2 vs. settings 1 and 3. For every 1.0 mU/L increase within the TSH range of 0.85–6.9 mU/L, TC, FG, SBP, and DBP increased by 12.1 mg/dl, 3.12 mg/dL, 2.31 mmHg, and 2.0 mmHg, respectively (44). As summarized elsewhere (100), our data (23, 44) agree with other data outside of the IM setting, which show that as TSH levels increase within its euthyroid range, metabolic and cardiovascular outcomes worsen progressively. For instance, comparing TSH of 3.0–3.5 mU/L with 0.50–0.99 mU/L, the OR for hypertension was 1.98 in men and 1.23 in women (101). Within the reference range of 0.50–3.5 mU/L, blood pressure increased linearly with increasing TSH (SBP by 2.0 mmHg in men and 1.8 mmHg in women, DBP increased by 1.6 and 1.1 mmHg) (101). In 12,584 adults with normal TSH levels, the frequency of diabetes, hypertension, and hypercholesterolemia, increased significantly across TSH tertiles (diabetes = 6.3, 7.7, and 9.1%; hypertension = 20.9, 24.8, and 29.8%; hypercholesterolemia = 13.2, 15.2, and 19.4%) (102). Moreover, SCH might favor diabetic complications with an OR of 1.74 (nephropathy), 1.42 (retinopathy), 1.85 (peripheral arterial disease), and 1.87 (peripheral neuropathy) (103).
High-normal TSH levels impact unfavorably on mortality. In 9,020 adults, SCH (TSH >5.60 mIU/L) and high-normal TSH (1.96–5.60 mIU/L) were associated with increased all-cause mortality (HR = 1.90 and 1.36) vs. the middle-normal TSH group (1.20–1.95 mIU/L) (104), with CVD mediating 14.3 and 5.9% of the association, respectively (104). A study on 611 hospitalized elderly patients evaluated all-cause mortality up to 66 months after discharge (105) and concluded that (i) in treated hypothyroid patients, median TSH levels of 5–10 IU/L associated with increased mortality, (ii) treatment should aim at achieving euthyroidism to improve survival (105).
Undertreated CH is associated with increased weight, BMI, larger waist circumference (106), and higher fat mass, worse lipid profile (total cholesterol, LDL-cholesterol, HDL-cholesterol, triglycerides) (107), with increased risk for cardiovascular morbidity (108).
Concerning costs, these were estimated by four simulations, based on 5% rate of PH (4.5% SCH, 0.5% OH), three rates (10, 50, and 80%) of L-T4-treated SCH patients, 440,000 to 3,608,000 UTH persons, and a total of 125,800 to 1,031,888 persons with calcium-related UTH and iron-related UTH (98). At euro 20 for one TSH test, the total cost for five TSH tests in these 125,840 or 1,031,888 patients ranged euro 12,584,000 to 103,188,800. Another study (99) analyzed the cost of resources consumed by frequent L-T4 dose changes over 24 months (laboratory testing, thyroid medications, general physician and specialist office visits, and emergency department visits/hospitalizations) in two groups of 227 hypothyroids each. Compared with the no-dose-adjustment patients (controls), significantly more patients needing dose adjustments (75 vs. 86%), were taking IM causing L-T4 malabsorption (PPIs, H2-receptor antagonists, calcium or iron supplements) (99). Overall resource utilization was higher in the ≥1-dose-adjustment group ($5,824/patient) vs. controls (USD 3,166/patient), peaking in the ≥3-dose-adjustment subgroup ($8,220/patient). The ≥1-dose-adjustment group experienced a 40.3% increase in lost productivity vs. controls ($1,381 vs. $984), with a peak in the ≥3-dose adjustment subgroup ($1,833). Compared to controls, patients requiring adjustments had significantly higher TSH (5.07 ± 11.04 vs. 2.57 ± 2.51 U/ml), and more frequent TSH tests (84 vs. 73%) (99).
Potential Future Developments
In the “Areas for future research” heading of the 2012 ATA guidelines (4), a section was devoted to “Agents and conditions having an impact on L-thyroxine therapy and interpretation of thyroid tests”. Except for reminding that the residual functioning thyroid tissue is a major factor for a given IM to cause thyroid dysfuntion and L-T4 dose adjustments, no ideas were presented (4).
Future developments have already occurred considering the availability of L-T4 formulations (liquid, softgel) that are refractory or much more resistant to IM than tablet L-T4 (9, 14, 98, 100, 109–114). In the author’s opinion, their use seems preferable to the strategies of (i) increasing stepwise the dose of L-T4 (with associated frequent monitoring of TFTs and risk of iatrogenic thyrotoxicosis if the IM is decreased in dose or withdrawn); (ii) adding supplementation with either 1 g/d (115) or 0.5 g/d (116) vitamin C to acidify the intragastric pH. In the Argentinian study, the 28 patients had no known cause for UTH (115), while in the Colombian study, the 31 patients had endoscopy/gastritis-proven gastritis (116). Both 2-month-long trials with vitamin C (115, 116) lack formal pharmacokinetics studies and challenge of patients with IM-associated UTH. Indeed, coadministration of acidic beverages is one way of solving the problem of decreased bioavailability of drugs whose bioavailability under conditions of increased intragastric pH (117). Further to T4, there are a number of other drugs with decreased absorption at high intragastric pH, such as ketoconazole, itraconazole, atazanavir, cefpodoxime, enoxacin, dipyridamole, raltegravir, alendronate, digoxin, and nifedipine, to name a few (117). The other way is the development of “formulations that can minimize or mitigate the effects of increased gastric pH on the bioavailability” (117).
Considering the magnitude of polypharmacy, particularly in the elderly (118), and the aforesaid unfavorable impact of UTH on metabolic and CVD outcomes, more research is needed to substantiate those outcomes in the setting of IM-associated UTH. Once pejorative outcomes are confimed, monitoring of hypothyroid patients under IM should be tightened, with the biochemical monitoring not restricted to TSH solely.
Author Contributions
SB made the work, drafted the article, revised it, and gave the final approval for the publication.
Conflict of Interest
IBSA Institut Biochimique SA (Lugano, Switzerland) and IBSA Farmaceutici Italia s.r.l. furnished the principal investigator (SB) with novel formulations to conduct studies cited in the reference list. However, IBSA had no role in any phase of the writing of the above studies and this manuscript. Furthermore, SB was an invited speaker at symposia organized by IBSA.
References
1. Mendes D, Alves C, Silverio N, Batel Marques F. Prevalence of undiagnosed hypothyroidism in Europe: A systematic review and meta-analysis. Eur Thyroid J (2019) 8:130–43. doi: 10.1159/000499751
2. Hollowell JG, Staehling NW, Flanders WD, Hannon WH, Gunter EW, Spencer CA, et al. T(4), and thyroid antibodies in the United States population (1988 to 1994): National Health and Nutrition Examination Survey (NHANES III). J Clin Endocrinol Metab (2002) 87:489–99. doi: 10.1210/jcem.87.2.8182
3. Benvenga S, Klose M, Vita R, Feldt-Rasmussen U. Less known aspects of central hypothyroidism: Part 1- Acquired etiologies. J Clin Transl Endocrinol (2018) 14:25–33. doi: 10.1016/j.jcte.2018.09.003
4. Garber JR, Cobin RH, Gharib H, Hennessey JV, Klein I, Mechanick JI, et al. American Association of Clinical Endocrinologists and American Thyroid Association Taskforce on Hypothyroidism in Adults. Clinical practice guidelines for hypothyroidism in adults: cosponsored by the American Association of Clinical Endocrinologists and the American Thyroid Association. Thyroid (2012) 22:1200–35. doi: 10.1089/thy.2012.0205
5. Jonklaas J, Bianco AC, Bauer AJ, Burman KD, Cappola AR, Celi FS, et al. American Thyroid Association Task Force on Thyroid Hormone Replacement. Guidelines for the treatment of hypothyroidism: prepared by the American Thyroid Association Task Force on Thyroid Hormone Replacement. Thyroid (2014) 24:1670–751. doi: 10.1089/thy.2014.0028
6. Wiersinga WM, Duntas L, Fadeyev V, Nygaard B, Vanderpump MP. ETA Guidelines: The Use of L-T4 + L-T3 in the Treatment of Hypothyroidism. Eur Thyroid J (2012) 2012) 1:55–71. doi: 10.1159/000339444
7. Persani L, Brabant G, Dattani M, Bonomi M, Feldt-Rasmussen U, Fliers E, et al. European Thyroid Association (ETA) Guidelines on the diagnosis and management of central hypothyroidism. Eur Thyroid J (2018) 2018) 7:225–37. doi: 10.1159/000491388
8. Pearce SH, Brabant G, Duntas LH, Monzani F, Peeters RP, Razvi S, et al. ETA Guideline: Management of Subclinical Hypothyroidism. Eur Thyroid J (2013) 2013) 2:215–28. doi: 10.1159/000356507
9. Virili C, Antonelli A, Santaguida MG, Benvenga S, Centanni M. Gastrointestinal Malabsorption of thyroxine. Endocr Rev (2019) 40:118–36. doi: 10.1210/er.2018-00168
10. Jonklaas J. Treatment of hypothyroidism. In: Braverman LE, Cooper DS, editors. Werner and Ingbar’s The Thyroid: A Fundamental and Clinical Text. Philadelphia: Wolters Kluwer, Lippincott Williams & Wilkins (2013). p. 611–28.
11. Benvenga S. When thyroid hormone replacement is ineffective? Curr Opin Endocrinol Diabetes Obes (2013) 20:467–77. doi: 10.1097/MED.0000000000000003
12. Vaisman F, Coeli CM, Ward LS, Graf H, Carvalho G, Montenegro jr R, et al. How good is the levothyroxine replacement in primary hypothyroidism patients in Brazil? Data of a multicentre study. J Endocrinol Invest (2013) 36:485–8. doi: 10.3275/8810
13. Taylor PN, Iqbal A, Minassian C, Sayers A, Draman MS, Greenwood R, et al. Falling threshold for treatment of borderline elevated thyrotropin levels-balancing benefits and risks: evidence from a large community-based study. JAMA Intern Med (2014) 174:32–9. doi: 10.1001/jamainternmed.2013.11312
14. Benvenga S, Capodicasa G. Perelli S. L-Thyroxine in an oral liquid or softgel formulation ensures more normal serum levels of free T4 in patients with central hypothyroidism. Front Endocrinol (2017) 8:321. doi: 10.3389/fendo.2017.00321
15. Koulouri O, Auldin MA, Agarwal R, Kieffer V, Robertson C, Falconer Smith J, et al. Diagnosis and treatment of hypothyroidism in TSH deficiency compared to primary thyroid disease: pituitary patients are at risk of under-replacement with levothyroxine. Clin Endocrinol (Oxf) (2011) 74:744–9. doi: 10.1111/j.1365-2265.2011.03984.x
16. Liwanpo L, Hershman JM. Conditions and drugs interfering with thyroxine absorption. Best Pract Res Clin Endocrinol Metab (2009) 23:781–92. doi: 10.1016/j.beem.2009.06.006
17. Barbesino G. Drugs affecting thyroid function. Thyroid (2010) 20:763–70. doi: 10.1089/thy.2010.1635
18. Ward LS. The difficult patient: drug interaction and the influence of concomitant diseases on the treatment of hypothyroidism. Arq Bras Endocrinol Metabol (2010) 54:435–42. doi: 10.1590/s0004-27302010000500002
19. Kundra P, Burman KD. The effect of medications on thyroid function tests. Med Clin North Am (2012) 96:283–95. doi: 10.1016/j.mcna.2012.02.001
20. Thalmann S, Meier CA. Braverman LE, Cooper DS, editors. Werner and Ingbar’s The Thyroid: A Fundamental and Clinical Text. Philadelphia: Wolters Kluwer, Lippincott Williams & Wilkins (2013). p. 187–202.
21. Singer PA. Primary hypothyroidism due to other causes. In: Braverman LE, Cooper DS, editors. Werner and Ingbar’s The Thyroid: A Fundamental and Clinical Text. Philadelphia: Wolters Kluwer, Lippincott Williams & Wilkins (2013). p. 552–60.
22. Montanelli L, Benvenga S, Hegedus L, Vitti P, Latrofa F, Duntas LH. Drugs and other substances interfering with thyroid function. In: Vitti P, Hegedus L, editors. Thyroid Diseases, Endocrinology 3. Cham, Switzerland: Springer (2018). p. 733–61.
23. Benvenga S, Pantano R, Saraceno G, lipari L, Alibrando A, Inferrera S, et al. Alecci U. A minimum of two years of undertreated primary hypothyroidism, as a result of drug-induced malabsorption of l-thyroxine, may have metabolic and cardiovascular consequences. J Clin Transl Endocrinol (2019) 16:100189. doi: 10.1016/j.jcte.2019.100189
24. Trifirò G, Parrino F, Sultana J, Giorgianni F, Ferrajolo C, Bianchini E, et al. Drug interactions with levothyroxine therapy in patients with hypothyroidism: observational study in general practice. Clin Drug Investig (2015) 35:187–95. doi: 10.1007/s40261-015-0271-0
25. Irving SA, Vadiveloo T, Leese GP. Drugs that interact with levothyroxine: an observational study from the Thyroid Epidemiology, Audit and Research Study (TEARS). Clin Endocrinol (Oxf) (2015) 82:136–41. doi: 10.1111/cen.12559
26. Hamnvik OP, Larsen PR, Marqusee E. Thyroid dysfunction from antineoplastic agents. J Natl Cancer Inst (2011) 103:1572–87. doi: 10.1093/jnci/djs011
27. Torino F, Barnabei A, Paragliola R, Baldelli R, Appetecchia M, Corsello SM. Thyroid dysfunction as an unintended side effect of anticancer drugs. Thyroid (2013) 23:1345–66. doi: 10.1089/thy.2013.0241
28. Fallahi P, Ferrari SM, Vita R, Di Domenicantonio A, Corrado A, Benvenga S, et al. Thyroid dysfunctions induced by tyrosine kinase inhibitors. Expert Opin Drug Saf (2014) 13:723–33. doi: 10.1517/14740338.2014.913021
29. Ferrari SM, Fallahi P, Elia G, Ragusa F, Ruffilli I, Patrizio A, et al. Autoimmune endocrine dysfunctions associated with cancer immunotherapies. Int J Mol Sci (2019) 20:2560. doi: 10.3390/ijms20102560
30. Muller I, Moran C, Lecumberri B, Decallonne B, Robertson N, Jones J, et al. European Thyroid Association Guidelines on the management of thyroid dysfunction following immune reconstitution therapy. Eur Thyroid J (2019) 2019) 8:173–85. doi: 10.1159/000500881
31. Tola HH, Holakouie-Naieni K, Lejisa T, Mansournia MA, Yaseri M, Tesfaye E, et al. Is hypothyroidism rare in multidrug resistance tuberculosis patients on treatment? A systematic review and meta-analysis. PloS One (2019) 14:e0218487. doi: 10.1371/journal.pone.0218487
32. Paragliola RM, Prete A, Kaplan PW, Corsello SM, Salvatori R. Treatment of hypopituitarism in patients receiving antiepileptic drugs. Lancet Diabetes Endocrinol (2015) 3:132–40. doi: 10.1016/S2213-8587(14)70081-6
33. Lupoli R, Di Minno A, Tortora A, Ambrosino P, Lupoli GA, Di Minno MN. Effects of treatment with metformin on TSH levels: a meta-analysis of literature studies. J Clin Endocrinol Metab (2014) 99:E143–8. doi: 10.1210/jc.2013-2965
34. Haugen BR. Drugs that suppress TSH or cause central hypothyroidism. Best Pract Res Clin Endocrinol Metab (2009) 23:793–800. doi: 10.1016/j.beem.2009.08.003
35. Lazarus JH. Lithium and thyroid. Best Pract Res Clin Endocrinol Metab (2009) 23:723–33. doi: 10.1016/j.beem.2009.06.002
36. Hamed SA. The effect of antiepileptic drugs on thyroid hormonal function: causes and implications. Expert Rev Clin Pharmacol (2015) 8:741–50. doi: 10.1586/17512433.2015.1091302
37. Corsello SM, Barnabei A, Marchetti P, De Vecchis L, Salvatori R, Torino F. Endocrine side effects induced by immune checkpoint inhibitors. J Clin Endocrinol Metab (2013) 98:1361–75. doi: 10.1210/jc.2012-4075
38. González-Rodríguez E, Rodríguez-Abreu D. Immune checkpoint inhibitors: review and management of endocrine adverse effects. Oncologist (2016) 21:804–16. doi: 10.1210/jc.2012-4075
39. Costa R, Carneiro BA, Agulnik M, Rademaker AW, Pai SG, Villaflor VM, et al. Toxicity profile of approved anti-PD-1 monoclonal antibodies in solid tumors: a systematic review and meta-analysis of randomized clinical trials. Oncotarget (2017) 8:8910–20. doi: 10.18632/oncotarget.13315
40. Bartalena L, Bogazzi F, Chiovato L, Hubalewska-Dydejczyk A, Links TP, Vanderpump M. European Thyroid Association (ETA) Guidelines for the Management of Amiodarone-Associated Thyroid Dysfunction. Eur Thyroid J (2018) 2018) 7:55–66. doi: 10.1159/000486957
41. Singh N, Singh PN, Hershman JM. Effect of calcium carbonate on the absorption of levothyroxine. JAMA (2000) 283:2822–5. doi: 10.1001/jama.283.21.2822
42. Singh N, Weisler SL, Hershman JM. The acute effect of calcium carbonate on the intestinal absorption of levothyroxine. Thyroid (2001) 11:967–71. doi: 10.1089/105072501753211046
43. Zamfirescu I, Carlson HE. Absorption of levothyroxine when coadministered with various calcium formulations. Thyroid (2001) 11:483–6. doi: 10.1089/thy.2010.0296
44. Morini E, Catalano A, Lasco A, Morabito N, Benvenga S. L-thyroxine malabsorption due to calcium carbonate impairs blood pressure, total cholesterolemia, and fasting glycemia. Endocrine (2019) 64:284–92. doi: 10.1007/s12020-018-1798-7
45. Centanni M, Gargano L, Canettieri G, Viceconti N, Franchi A, Delle Fave G, et al. Thyroxine in goiter, Helicobacter pylori infection, and chronic gastritis. N Engl J Med (2006) 354:1787–95. doi: 10.1056/NEJMoa043903
46. Sachmechi I, Reich DM, Aninyei M, Wibowo F, Gupta G, Kim PJ. Effect of proton pump inhibitors on serum thyroid-stimulating hormone levels in euthyroid patients treated with levothyroxine for hypothyroidism. Endocr Pract (2007) 13:345–9. doi: 10.4158/EP.13.4.345
47. Kim HI, Kim TH, Kim H, Kim YN, Jang HW, Chung JH, et al. Effect of Rifampin on Thyroid Function Test in Patients on Levothyroxine Medication. PloS One (2017) 12:e0169775. doi: 10.1371/journal.pone.0169775 eCollection 2017.
48. McDonnell ME, Braverman LE, Bernardo J. Hypothyroidism due to ethionamide. N Engl J Med (2005) 352:2757–9. doi: 10.1056/NEJM200506303522621
49. Drucker D, Eggo MC, Salit IE, Burrow GN. Ethionamide-induced goitrous hypothyroidism. Ann Intern Med (1984) 100:837–9. doi: 10.7326/0003-4819-100-6-837
50. MacGregor AG, Somner AR. The anti-thyroid action of para-aminosalicylic acid. Lancet (1954) 267:931–6. doi: 10.1016/s0140-6736(54)92552-0
51. Andries A, Isaakidis P, Das M, Khan S, Paryani R, Desai C, et al. High rate of hypothyroidism in multidrug-resistant tuberculosis patients co-infected with HIV in Mumbai, India. PloS One (2013) 8:e78313. doi: 10.1371/journal.pone.0078313
52. Munivenkatappa S, Anil S, Naik B, Volkmann T, Sagili KD, Akshatha JS, et al. Drug-Induced Hypothyroidism during anti-tuberculosis treatment of multidrug-resistant tuberculosis: Notes from the field. J Tuberc Res (2016) 4:105–10. doi: 10.4236/jtr.2016.43013
54. Sahajpal R, Ahmed RA, Hughes CA, Foisy MM. Probable interaction between levothyroxine and ritonavir: Case report and literature review. Am J Health Syst Pharm (2017) 74:587–92. doi: 10.2146/ajhp160200
55. Touzot M, Beller CL, Touzot F, Louet AL, Piketty C. Dramatic interaction between levothyroxine and lopinavir/ritonavir in a HIV-infected patient. AIDS (2006) 8:1210–2. doi: 10.1097/01.aids.0000226969.96880.3c
56. Harsløf M, Knudsen AD, Benfield T, Nordestgaard BG, Feldt-Rasmussen U, Nielsen SD. No evidence of increased risk of thyroid dysfunction in well treated people living with HIV. AIDS (2018) 32:2195–9. doi: 10.1097/QAD.0000000000001954
57. Badros AZ, Siegel E, Bodenner D, Zangari M, Zeldis J, Barlogie B, et al. Hypothyroidism in patients with multiple myeloma following treatment with thalidomide. Am J Med (2002) 112:412–3. doi: 10.1016/s0002-9343(01)01137-8
58. Figaro MK, Clayton W Jr, Usoh C, Brown K, Kassim A, Lakhani VT, et al. Thyroid abnormalities in patients treated with lenalidomide for hematological malignancies: Results of a retrospective case review. Am J Hematol (2011) 86:467–70. doi: 10.1002/ajh.22008
59. Hamadeh IS, Bourdage KL, Davis AC, Moreb JS. Pomalidomide induced hypothyroidism in a multiple myeloma patient: A case report. Ann Hematol Oncol (2015) 2:1057. doi: 10.1530/EDM-17-0110
60. Ali SH, Aljenaee K, Wan Mahmood WA, Hatunic M. Pomalidomide-induced hypothyroidism. Endocrinol Diabetes Metab Case Rep (2017) 2017:17–0110. doi: 10.1530/EDM-17-0110
61. Mondello P, Mian M, Pitini V, Cuzzocrea S, Sindoni A, Galletti M, et al. Thyroid hormone autoantibodies: are they a better marker to detect early thyroid damage in patients with hematologic cancers receiving tyrosine kinase inhibitor or immunoregulatory drug treatments? Curr Oncol (2016) 23:e165–70. doi: 10.3747/co.23.3026
62. Wong E, Rosen LS, Mulay M, Vanvugt A, Dinolfo M, Tomoda C. SugawaraM, Hershman JM 2007 Sunitinib induces hypothyroidism in advanced cancer patients and may inhibit thyroid peroxidase activity. Thyroid (2007) 17:351–5. doi: 10.1089/thy.2006.0308
63. de Groot JW, Zonnenberg BA, Plukker JT, van Der Graaf WT, Links TP. Imatinib induces hypothyroidism in patients receiving levothyroxine. Clin Pharmacol Ther (2005) 78:433–8. doi: 10.1016/j.clpt.2005.06.010
64. Braun D, Kim TD, le Coutre P, Köhrle J, Hershman JM, Schweizer U. Tyrosine kinase inhibitors noncompetitively inhibit MCT8-mediated iodothyronine transport. J Clin Endocrinol Metab (2012) 97:E100–5. doi: 10.1210/jc.2011-1837
65. Nishimura M, Naito S. Tissue-specific mRNA expression profiles of human solute carrier transporter superfamilies. Drug Metab Pharmacokinet (2008) 23:22–44. doi: 10.2133/dmpk.23.22
66. Shah PD, Dickler MN. Endocrine therapy for advanced breast cancer. Clin Adv Hematol Oncol (2014) 12:214–23.
67. Figg WD, Thibault A, Sartor AO, Mays D, Headlee D, Calis KA, et al. Hypothyroidism associated with aminoglutethimide in patients with prostate cancer. Arch Intern Med (1994) 154:1023–5.
68. Bruning PF, Bonfrèr JG, Engelsman E, Hamersma-vd Linden E, de Jong-Bakker M, Nooyen W. Pros and cons of aminoglutethimide for advanced postmenopausal breast cancer. Breast Cancer Res Treat (1984) 4:289–95. doi: 10.1007/BF01806041
69. Pozza C, Graziadio C, Giannetta E, Lenzi A, Isidori AM. Management strategies for aggressive Cushing’s Syndrome: From macroadenomas to ectopics. J Oncol (2012) 2012:685213. doi: 10.1155/2012/685213
70. Bocchetta A, Cocco F, Velluzzi F, Del Zompo M, Mariotti S, Loviselli A. Fifteen-year follow-up of thyroid function in lithium patients. J Endocrinol Investig (2007) 30:363–6. doi: 10.1007/BF03346311
71. Kirov G, Tredget J, John R, Owen MJ. Lazarus JH. A cross-sectional and a prospective study of thyroid disorders in lithium-treated patients. J Affect Disord (2005) 87:313–7. doi: 10.1016/j.jad.2005.03.010
72. de Carvalho GA, Bahls SC, Boeving A, Graf H. Effects of selective serotonin reuptake inhibitors on thyroid function in depressed patients with primary hypothyroidism or normal thyroid function. Thyroid (2009) 19:691–7. doi: 10.1089/thy.2008.0261
73. McCowen KC, Garber JR, Spark R. Elevated serum thyrotropin in thyroxine-treated patients with hypothyroidism given sertraline. N Engl J Med (1997) 337:1010–1. doi: 10.1056/NEJM199710023371415
74. McCowen KC, Garber JR. Effect of SSRI antidepressants on L-thyroxine requirements. Thyroid (2010) 20:937. doi: 10.1089/thy.2009.0387
75. de Carvalho GA, Boeving A. Response to McCowe and Geber. Thyroid (2010) 20(8):937–8. doi: 10.1089/thy.2009.0387
76. Church CO, Callen EC. Myxedema coma associated with combination aripiprazole and sertraline therapy. Ann Pharmacother (2009) 43:2113–6. doi: 10.1345/aph.1M369
77. Verrotti A, Scardapane A, Manco R, Chiarelli F. Antiepileptic drugs and thyroid function. J Pediatr Endocrinol Metab (2008) 21:401–8. doi: 10.1515/jpem.2008.21.5.401
78. De Luca F, Arrigo T, Pandullo E, Siracusano MF, Benvenga S, Trimarchi F. Changes in thyroid function tests induced by 2 month carbamazepine treatment in L-thyroxine-substituted hypothyroid children. Eur J Pediatr (1986) 145:77–9. doi: 10.1007/BF00441860
79. Medicines Q&As. Is there an interaction between orlistat and levothyroxine? Available at: https://www.surreyandsussex.nhs.uk/wp-content/uploads/2013/04/UKMi-Interaction-between-Orlistat-Thyroxine.pdf (Accessed June 1, 2020). Unknown author.
80. Madhava K, Hartley A. Hypothyroidism in thyroid carcinoma follow-up: Orlistat may inhibit the absoprtion of thyroxine. Clin Oncol (R Coll Radiol) (2005) 17:492. doi: 10.1016/j.clon.2005.05.001
81. Chiffoleau A, De Mallmann V, Lambert J, Bodin X. Interaction between orlistat and levothyroxine: a first French case report. Fundam Clin Pharmacol (2010) 24(1):1–1.
82. Feldt-Rasmussen U, Klose M, Benvenga S. Interactions between hypothalamic pituitary thyroid axis and other pituitary dysfunctions. Endocrine (2018) 62:519–27. doi: 10.1007/s12020-018-1738-6
83. Fierro G, Hoffman AR. Treatment of the adult growth hormone deficiency syndrome with growth hormone: What are the implications for other hormone replacement therapies for hypopituitarism? Growth Horm IGF Res (2020) 52:101316. doi: 10.1016/j.ghir.2020.101316
84. Agha A, Walker D, Perry L, Drake WM, Chew SL, Jenkins PJ, et al. Unmasking of central hypothyroidism following growth hormone replacement in adult hypopituitary patients. Clin Endocrinol (Oxf) (2007) 66:72–7. doi: 10.1111/j.1365-2265.2006.02688.x
85. Brabant A, Brabant G, Schuermeyer T, Ranft U, Schmidt FW, Hesch RD, et al. The role of glucocorticoids in the regulation of thyrotropin. Acta Endocrinol (1989) 121:95–100.
86. Cappelli C, Rotondi M, Pirola I, Agosti A, Gandossi E, Valentini U, et al. TSH-lowering effect of metformin in type 2 diabetic patients: differences between euthyroid, untreated hypothyroid, and euthyroid on L-T4 therapy patients. Diabetes Care (2009) 32:1589–90. doi: 10.2337/dc09-0273
87. Kobayashi I, Shimomura Y, Maruta S, Ohshima K, Mori M, Kamio N, et al. Clofibrate and a Related Compound Suppress TSH Secretion in Primary Hypothyroidism. Acta Endocrinol (Copenh) (1980) 94:53–7. doi: 10.1530/acta.0.1210095
88. McKerron CG, Scott RL, Asper SP, Levy RI. Effects of Clofibrate (Atromid S) on the thyroxine-binding capacity of thyroxine-binding globulin and free thyroxine. J Clin Endocrinol Metab (1969) 29:957–61. doi: 10.1210/jcem-29-7-957
89. Paragliola RM, Torino F, Papi G, Locantore P, Pontecorvi A, Corsello SM. Role of mitotane in adrenocortical carcinoma – Review and state of the art. Eur Endocrinol (2018) 14:62–6. doi: 10.17925/EE.2018.14.2.62
90. Daffara F, De Francia S, Reimondo G, Zaggi B, Aroasio E, Porpiglia F, et al. Prospective evaluation of mitotane toxicity in adrenocortical cancer patients treated adjuvantly. Endocr Relat Cancer (2008) 15:1043–53. doi: 10.1677/ERC-08-0103
91. Russo M, Scollo C, Pellegriti G, Cotta OR, Squatrito S, Frasca F, et al. Mitotane treatment in patients with adrenocortical cancer causes central hypothyroidism. Clin Endocrinol (Oxf) (2016) 84:614–9. doi: 10.1111/cen.12868
92. Oddie PD, Albert BB, Hofman PL, Jefferies C, Laughton S, Carter PJ. Mitotane in the treatment of childhood adrenocortical carcinoma: a potent endocrine disruptor. Endocrinol Diabetes Metab Case Rep (2018) 2018:18–0059. doi: 10.1530/EDM-18-0059
93. Sherman SI, Gopal J, Haugen BR, Chiu AC, Whaley K, Nowlakha P, et al. Central hypothyroidism associated with retinoid X receptor-selective ligands. N Engl J Med (1999) 340(14):1075–9. doi: 10.1056/NEJM199904083401404
94. Smit JWA, Stokkel MPM, Pereira AM, Romijn JA, Visser TJ. Bexarotene-induced hypothyroidism: Bexarotene stimulates the peripheral metabolism of thyroid hormones. J Clin Endocrinol Metab (2007) 92:2496–9. doi: 10.1210/jc.2006-2822
95. Barrett NA, Jones A, Whiteley C, Yassin S, McKenzie CA. Management of long-term hypothyroidism: a potential marker of quality of medicines reconciliation in the intensive care unit. Int J Pharm Pract (2012) 20:303–6.
96. Imberti R, Ferrari M, Albertini R, Rizzo V, Tinelli C, Iotti GA. Increased levothyroxine requirements in critically ill patients with hypothyroidism. Minerva Anestesiol (2010) 76:500–3. doi: 10.1111/j.2042-7174.2012.00205.x
97. Reis AM, de Carvalho RE, de Faria LM, de Oliveira RC, Zago KS, Cavelagna MF, et al. Prevalence and clinical significance of interactions drug-enteral nutrition in Intensive Care Units. Rev Bras Enferm (2014) 67:85–90. doi: 10.5935/0034-7167.20140011
98. Benvenga S, Di Bari F, Vita R. Undertreated hypothyroidism due to calcium or iron supplementation corrected by oral liquid levothyroxine. Endocrine (2017) 56:138–45. doi: 10.1007/s12020-017-1244-2
99. Ernst FR, Barr P, Elmor PR, Sandulli W, Thevathasan L, Sterman AB, et al. The economic impact of levothyroxine dose adjustments: The CONTROL HE Study. Clin Drug Investig (2017) 37:71–83. doi: 10.1007/s40261-016-0462-3
100. Morini E, Catalano A, Lasco A, Morabito N, Benvenga S. In thyroxine-replaced hypothyroid postmenopausal women under simultaneous calcium supplementation, switch to oral liquid or softgel capsule L-thyroxine ensures lower serum TSH levels and favorable effects on blood pressure, total cholesterolemia and glycemia. Endocrine (2019) 65:569–79. doi: 10.1007/s12020-019-01908-x [published correction appears in Endocrine. (2019) 65:580–1. doi: 10.1007/s12020-019-01979-w]
101. Asvold BO, Bjøro T, Nilsen TI, Vatten LJ. Association between blood pressure and serum thyroid-stimulating hormone concentration within the reference range: a population-based study. J Clin Endocrinol Metab (2007) 92:841–5. doi: 10.1210/jc.2006-2208
102. Inoue K, Tsujimoto T, Saito J, Sugiyama. Association between serum thyrotropin levels T. and mortality among euthyroid adults in the United States. Thyroid (2016) 26:1457–65. doi: 10.1089/thy.2016.0156
103. Han C, He X, Xia X, Li Y, Shi X, Shan Z, et al. Subclinical hypothyroidism and type 2 diabetes: A systematic review and meta-analysis. PloS One (2015) 10(8):e0135233. doi: 10.1371/journal.pone.0135233
104. Inoue K, Ritz B, Brent GA, Ebrahimi R, Rhee CM, Leung AM. Association of subclinical hypothyroidism and cardiovascular disease with mortality. JAMA Netw Open (2020) 2):e1920745. doi: 10.1001/jamanetworkopen.2019.20745
105. Akirov A, Gimbel H, Grossman A, Shochat T, Shimon I. Elevated TSH in adults treated for hypothyroidism is associated with increased mortality. Eur J Endocrinol (2017) 176:57–66. doi: 10.1530/EJE-16-0708
106. Filipsson NH, Feldt-Rasmussen U, Kourides I, Popovic V, Koltowska-Haggstrom M, Jonsson B, et al. The metabolic consequences of thyroxine replacement in adult hypopituitary patients. Pituitary (2012) 15:495–504. doi: 10.1007/s11102-011-0356-6
107. Klose M, Marina D, Hartoft-Nielsen ML, Klefter O, Gavan V, Hilsted L, et al. Central hypothyroidism and its replacement have a significant influence on cardiovascular risk factors in adult hypopituitary patients. J Clin Endocrinol Metab (2013) 98:3802–10. doi: 10.1210/jc.2013-1610
108. Feldt-Rasmussen U, Klose M. Central hypothyroidism and its role for cardiovascular risk factors in hypopituitary patients. Endocrine (2016) 54:15–23. doi: 10.1007/s12020-016-1047-x
109. Vita R, Saraceno G, Trimarchi F, Benvenga S. Switching levothyroxine from the tablet to the oral solution formulation corrects the impaired absorption of levothyroxine induced by proton-pump inhibitors. J Clin Endocrinol Metab (2014) 99:4481–6. doi: 10.1210/jc.2014-2684
110. Vita R, Benvenga S. Tablet levothyroxine (L-T4) malabsorption induced by proton pump inhibitor; a problem that was solved by switching to L-T4 in soft gel capsule. Endocr Pract (2014) 20(3):e38–41. doi: 10.4158/EP13316.CR
111. Vita R, Fallahi P, Antonelli A, Benvenga S. The administration of L-thyroxine as soft gel capsule or liquid solution. Expert Opin Drug Deliv (2014) 11:1103–11. doi: 10.1517/17425247.2014.918101
112. Benvenga S, Carlé A. Levothyroxine formulations: Pharmacological and clinical implications of generic substitution. Adv Ther (2019) 36(Suppl 2):59–71. doi: 10.1007/s12325-019-01079-1
113. Virili C, Trimboli P, Centanni M. Novel thyroxine formulations: a further step toward precision medicine. Endocrine (2019) 66:87–94. doi: 10.1007/s12020-019-02049-x
114. Brancato D, Scorsone A, Saura G, Ferrara L, Di Noto A, Aiello V, et al. Comparison of TSH levels with liquid formulation versus tablet formulations of levothyroxine in the treatment of adult hypothyroidism. Endocr Pract (2014) 20:657–62. doi: 10.4158/EP13418.OR
115. Antúnez PB, Licht SD. Vitamin C improves the apparent absorption of levothyroxine in a subset of patients receiving this hormone for primary hypothyroidism. Rev Argent Endocrinol Metab (2011) 48:16–24.
116. Jubiz W and Ramirez M. Effect of Vitamin C on the absorption of levothyroxine in patients with hypothyroidism and gastritis. J Clin Endocrinol Metab (2014) 99:E1031–4. doi: 10.1210/jc.2013-4360
117. Mitra A and Kesisoglou F. Impaired drug absorption due to high stomach pH: a review of strategies for mitigation of such effect to enable pharmaceutical product development. Mol Pharm (2013) 10:3970–9. doi: 10.1021/mp400256h
Keywords: levothyroxine, hypothyroidism, pharmacological interferents, metabolic complications, cardiovascular complications
Citation: Benvenga S (2020) L-T4 Therapy in the Presence of Pharmacological Interferents. Front. Endocrinol. 11:607446. doi: 10.3389/fendo.2020.607446
Received: 17 September 2020; Accepted: 17 November 2020;
Published: 22 December 2020.
Edited by:
Paolo Miccoli, University of Pisa, ItalyReviewed by:
Marco Centanni, Sapienza University of Rome, ItalySilvia Martina Ferrari, University of Pisa, Italy
Copyright © 2020 Benvenga. This is an open-access article distributed under the terms of the Creative Commons Attribution License (CC BY). The use, distribution or reproduction in other forums is permitted, provided the original author(s) and the copyright owner(s) are credited and that the original publication in this journal is cited, in accordance with accepted academic practice. No use, distribution or reproduction is permitted which does not comply with these terms.
*Correspondence: Salvatore Benvenga, cy5iZW52ZW5nYUBsaXZlLml0; c2JlbnZlbmdhQHVuaW1lLml0