- 1Department of Internal Medicine, Division of Endocrinology and Diabetes, University Hospital, University of Würzburg, Würzburg, Germany
- 2Department of General, Visceral, Transplant, Vascular and Pediatric Surgery, University Hospital, University of Würzburg, Würzburg, Germany
Background: Combination therapies of anorectic gut hormones partially mimic the beneficial effects of bariatric surgery. Thus far, the effects of a combined chronic systemic administration of Glucagon-like peptide-1 (GLP-1) and peptide tyrosine tyrosine 3-36 (PYY3-36) have not been directly compared to Roux-en-Y gastric bypass (RYGB) in a standardized experimental setting.
Methods: High-fat diet (HFD)-induced obese male Wistar rats were randomized into six treatment groups: (1) RYGB, (2) sham-operation (shams), (3) liraglutide, (4) PYY3-36, (5) PYY3-36+liraglutide (6), saline. Animals were kept on a free choice high- and low-fat diet. Food intake, preference, and body weight were measured daily for 4 weeks. Open field (OP) and elevated plus maze (EPM) tests were performed.
Results: RYGB reduced food intake and achieved sustained weight loss. Combined PYY3-36+liraglutide treatment led to similar and plateaued weight loss compared to RYGB. Combined PYY3-36+liraglutide treatment was superior to PYY3-36 (p ≤ 0.0001) and liraglutide (p ≤ 0.05 or p ≤ 0.01) mono-therapy. PYY3-36+liraglutide treatment and RYGB also reduced overall food intake and (less pronounced) high-fat preference compared to controls. The animals showed no signs of abnormal behavior in OF or EPM.
Conclusions: Liraglutide and PYY3-36 combination therapy vastly mimics reduced food intake, food choice and weight reducing benefits of RYGB.
Introduction
Obesity nowadays affects more than one-third of human individuals and represents a major escalating socioeconomic burden (1). Moreover, obesity correlates with elevated risk for several serious, life-shortening diseases, such as diabetes, chronic heart failure, cardiovascular disease, and several types of cancer (2).
Available non-invasive treatment options for severe obesity lack efficacy and result in long-term weight regain in the vast majority of cases. In contrast, bariatric surgery, in particular Roux-en-Y gastric bypass (RYGB), achieves marked and sustained weight loss but also improves metabolic health (3–6). The persistently negative energy balance after surgery is considered to be largely due to a decrease in energy intake (approximately 50%) (7–20), mostly from fat (7, 10, 12, 15, 17, 18, 20–22).
Circulating levels of the appetite-suppressing anorectic and glucoregulatory gut hormones derived from enteroendocrine L-cells such as glucagon-like peptide-1 (GLP-1) and peptide tyrosine tyrosine 3-36 (PYY3-36) are markedly increased after Roux-en-Y gastric bypass surgery (RYGB) (23–32). However, studies in which signaling of the GLP-1 receptor or the PYY3-36-preferring Y2 receptor are individually perturbed by genetic or pharmacologic means in RYGB-operated humans or rodents have generally shown little to no influence on food intake or body weight (33–37). It was consequently proposed that GLP-1 and PYY3-36 may compensate for each other when either one is blocked after RYGB, but this has not received support in mice with combined deletion of the GLP-1 receptor and the Y2 receptor (38). Thus, significant doubt has been cast on the causal roles of GLP-1 and PYY3-36 in the long-term negative energy balance induced by RYGB.
This does, however, not necessarily undermine the potential of GLP-1 and PYY3-36 as anti-obesity treatments. It has been shown that GLP-1 and PYY3-36 reduce food intake additively in rodents and humans when administered exogenously in an acute setting (39), while chronic administration of the clinically approved GLP-1 analogue liraglutide with PYY3-36 additively reduce body weight in diet-induced obese hamsters—similar to the patterns found after RYGB (40). We previously found that the anorectic gut hormones GLP-1 and PYY3-36 reduce high-fat food preference additively after RYGB in diet-induced obese rats (41).
These findings still suggest that GLP-1 + PYY3-36 combination therapy holds genuine promise as a noninvasive alternative to RYGB for treating obesity, although these peptides are probably not the only or main factors for the effects and actions of RYGB. However, the short half-life of PYY3-36 of only 4 h prevents it clinical use (42). GLP-1 is even more rapidly degraded to inactive metabolites, resulting in a half-life of only 10 min (43). Synthetic, long-acting GLP-1 receptor agonists like liraglutide (half-life in humans approximately 12 h), however, are used for the successful treatment of obesity (44, 45).
Thus, to formally test the combined effects of liraglutide and PYY3-36 in a standardized obesity model and, thereby, show the potential of a combinatory medical treatment for obesity, we directly compared the effects of RYGB with daily subcutaneous liraglutide injections in combination with PYY3-36 delivered by subcutaneous osmotic mini-pump on food intake, food choice, and body weight in diet-induced obese Wistar rats. To control for abnormal behavioral changes under these substances, which might especially occur during PYY3-36 treatment (46), open field and elevated plus maze test were performed. To assess the effects on body composition, we analyzed the weights of retroperitoneal and epididymal white adipose tissue (WAT) depots ex vivo.
Methods
Animals
As published before (41), adult male Wistar rats (Charles River Laboratories, n = 58) weighing 329.8 ± 2.2 g, 9–10 weeks old were initially group-housed in a dedicated facility with an ambient room temperature of 22°C and a 12 h light/dark cycle. They had free access to a High Fat Diet (C1090-60 HF diet, 5,228 kcal/kg; 60% calories from fat, 16% from protein, and 24% from carbohydrate; Altromin) for about 6 weeks to induce obesity. The animals were randomized into the following pharmaceutical/surgical treatment groups: Five animals received liraglutide s.c. (0.4 mg/kg/day, Victoza, Novo Nordisk Pharma) and isotonic saline (Braun) via osmotic minipump. Five animals received PYY3-36 (0.1 mg/kg/day, Hölzel diagnostika) via osmotic minipump and saline s.c. Eleven animals received a combination of liraglutide s.c. and PYY3-36 via osmotic minipump and nine animals received saline only (via osmotic minipump and s.c.). Fifteen animals underwent RYGB and thirteen animals a sham operation. Generally, we attended the principle of the 3Rs, that the number of animals necessary is the minimum required for statistical significance based on effect sizes and results. Based on our previous experiences (47), a number of 5–7 animals is necessary for this. Usually, we calculate for a dropout rate of 30% in our RYGB model and 5% in our sham model. For some reason, we experienced a far higher dropout rate (50%) during a previous study and thus we decided to strengthen these particular groups.
All animal procedures were approved by the local regulatory authority (Regierung von Unterfranken, Würzburg, Germany, AZ: 55.2-2532-2-467). All experiments were performed in accordance with German and European laws and regulations (TierSchG, TierSchVersV, Directive 2010/63/EU).
Drugs
Liraglutide was administered subcutaneously at a dose of 0.4 mg/kg/day once daily. PYY3-36 was given at a dose of 0.1 mg/kg/day (40, 48). To overcome the short half-life of PYY3-36 (42), it was administered via osmotic minipump (ALZET pump model 2004). Osmotic minipumps were implanted subcutaneously into the interscapular region of the animals under isoflurane anesthesia with butorphanol analgesia and postoperative carprofen analgesia (one injection of 5 mg/kg carprofen). Isotonic saline was administered at a dose of 1 µl/g body weight once daily subcutaneously (corresponding to the volume of liraglutide) and/or via osmotic minipump.
Surgeries
For RYGB and sham operation, the animals were isoflurane-anesthetized and under butorphanol (0.1 mg/kg) analgesia. Briefly, for the RYGB operation a small gastric pouch 5% of the original stomach size was created and the biliopancreatic and common limbs were made to measure 15 and 25 cm in length, respectively (41, 47). Animals were then transferred to individual cages and for the first 2 postoperative days were only given a liquid diet (chocolate-flavored Ensure, a nutrition drink, 0.93 kcal/ml; 22% calories from fat) and carprofen analgesia. Postoperatively, all animals received carprofen (5 mg/kg) for 5 days. From postoperative day 3 onwards, animals were maintained on a choice of C1090-10 LF (3,514 kcal/kg; 10% calories from fat, 24% from protein, and 66% from carbohydrate; Altromin) and HF diets. LF and HF diet intakes were measured daily until day 24 for each animal and HF/LF food preference was calculated by dividing the daily calories consumed from the HF/LF diet by the total daily calories consumed from both diets and expressed as percentage.
Open Field
The open field (OF) is used to test for locomotor activity, exploratory behavior, and anxiety (49). Normally, rodents avoid open areas and present thigmotaxis, the degree depending on their anxiety level. As OF, an apparatus of polyethylene with a square base (1 m²) and 60 cm high sidewalls was used. The animals were transferred to the center of this box individually and their activity was recorded for 10 min immediately after this. After each run, the OF was cleaned with Terralin liquid. At a later time point, the recordings were analyzed. The time in the central area (central square, 90 × 90 cm) of the OF, the number of entries in this area, as well as the rearing behavior was evaluated. Animals were tested at about 2 weeks after intervention.
Elevated Plus Maze
The elevated plus maze (EPM) is used for the evaluation of anxiety (50). As EPM, an elevated (1 m) apparatus of polyethylene with a central area (10 cm²) with four attached arms (50 × 10 cm, two opposing arms with 30 cm sidewalls) was used. The animals were transferred to the center of the EPM individually and their activity was recorded for 5 min immediately after this. After each run, the EPM was cleaned with Terralin liquid. At a later time point, the recordings were analyzed. The time in the open arms of the EPM and the number of entries in the open arms was measured. Animals were tested at about 2 weeks after intervention.
Ex Vivo White Adipose Tissue Analysis
After a midline laparotomy was performed and the intestine removed, the retroperitoneum was exposed. As described before, we chose the aorta as medial, the fascia transversalis as lateral and the psoas muscle as dorsal dissection border (51). Directly following dissection, retroperitoneal and epididymal (rWAT and eWAT) fat pads were weighed using a precision balance.
Statistical Analysis
Two-tailed unpaired t-test and two-way ANOVA with Tukey’s post-hoc comparison test where appropriate were used for statistical analysis using GraphPad Prism (Version 8.1.2) software. Due to inexplicably high weight loss, one RYGB treated animal had to be excluded from all analyses.
Results
Roux-En-Y Gastric Bypass and Peptide Tyrosine Tyrosine 3-36 +Liraglutide Lead to Similar Sustained Plateaued Body Weight
On the day of surgery and start of medical treatment, respectively, animals of the different treatment/control groups had similar body weights (477.7 ± 8.4 g for the RYGB group, 479.2 ± 11 g for the sham group, 547.8 ± 19.5 g for the PYY3-36 +liraglutide group, 502.4 ± 7.6 g for the PYY3-36 group, 487.6 ± 9.6 g for the liraglutide group, and 527.1 ± 18.6 g for the saline group). The weight course after intervention is shown in Figure 1. For better visualization of the differences, statistics were performed for weekly intervals. Post intervention, shams, saline, and PYY3-36 treated animals gained weight continuously, while RYGB, PYY3-36 +liraglutide and liraglutide treated animals started to lose weight. RYGB and PYY3-36 +liraglutide treated animals achieved sustained and plateaued weight loss, while the other two groups started to regain weight upon the second week during the further observation period of 4 weeks (effect of intervention: F (5, 255) = 221.6, p ≤ 0.0001; effect of time: F (4, 255) = 43.48, p ≤ 0.0001; interaction: F (20, 255) = 18.11, p ≤ 0.0001. Figure 1B).
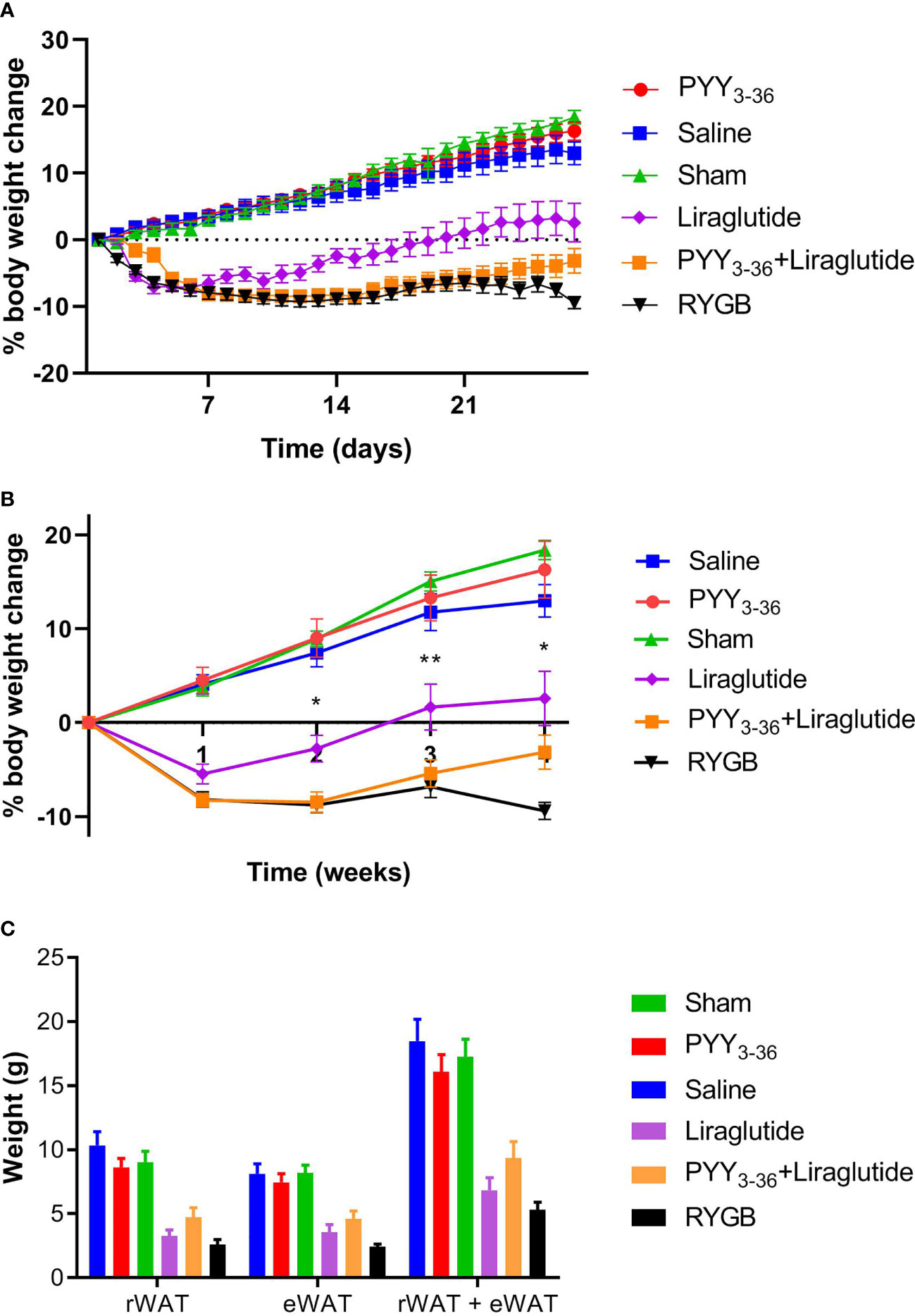
Figure 1 RYGB and PYY3-36+liraglutide lower body weight in diet-induced obese Wistar rats. Body weight in percent (%) weight change from baseline (intervention) of RYGB (n = 14), sham (n = 13), PYY3-36+liraglutide (n = 11), liraglutide (n = 5) and saline (n = 9) groups. (A) daily and (B) weekly. *p ≤ 0.05 and **p ≤ 0.01 for differences between PYY3-36+liraglutide and liraglutide as determined by two-way ANOVA with Tukey’s post-hoc. (C) Weights of rWAT, eWAT and sum of rWAT+eWAT. Significant differences between PYY3-36+liraglutide, liraglutide, RYGB, and their respective control group (not shown). (A–C) Data are presented as mean ± standard error of the mean.
The post-hoc test reveals a significant difference between RYGB and sham from the first week post intervention onwards (p ≤ 0.0001). A significant difference between PYY3-36+liraglutide and saline (p ≤ 0.0001), as well as between liraglutide and saline (p ≤ 0.0001) treated animals was also seen from the first week post intervention. A significant difference between PYY3-36 +liraglutide and liraglutide only was detected from the second week onwards (p ≤ 0.05 or p ≤ 0.01). Liraglutide treated animals also differed from RYGB treated from week two onwards (p ≤ 0.05 and p ≤ 0.0001). There was no significant difference between PYY3-36 and saline at any time point.
Roux-En-Y Gastric Bypass and Peptide Tyrosine Tyrosine 3-36 +Liraglutide Lower Overall Food Intake and Preference for High-Fat Diet
In order to study the effects of RYGB, PYY3-36 and liraglutide and a combination of both on food intake and food choice, animals from both surgical groups were given free access to LF (10% calories from fat) and HF (60% calories from fat) diets from the third postoperative day onwards. RYGB, PYY3-36+liraglutide, and liraglutide led to a reduced overall food intake (in kcal) in the observation period (effect of intervention: F (5, 300) = 75.2, p ≤ 0.0001; effect of time: F (5, 300) = 17.3, p ≤ 0.0001; interaction: F (25, 300) = 3.3, p ≤ 0.0001. Figure 2A). Post-hoc testing shows, that animals of the PYY3-36+liraglutide group consumed significantly less food than saline (p ≤ 0.05, p ≤ 0.01, p ≤ 0.001, and p ≤ 0.0001) and RYGB treated animals consumed significantly less than shams (p ≤ 0.001 and p ≤ 0.0001) throughout the whole observation period, while liraglutide treated animals only differed from saline treated in intervals 1 and 2. Moreover, there was a significant difference between PYY3-36+liraglutide and liraglutide regarding food intake at interval 2. Regarding food preference, the effects of the intervention were less pronounced (Figure 2B). RYGB treated animals showed a significantly reduced preference for 60% fat diet compared to sham treated animals in intervals 4, 5, and 6 (p ≤ 0.01 and p ≤ 0.05), PYY3-36 +liraglutide treated animals differed from saline treated in interval 5 and 6 (p ≤ 0.0001).
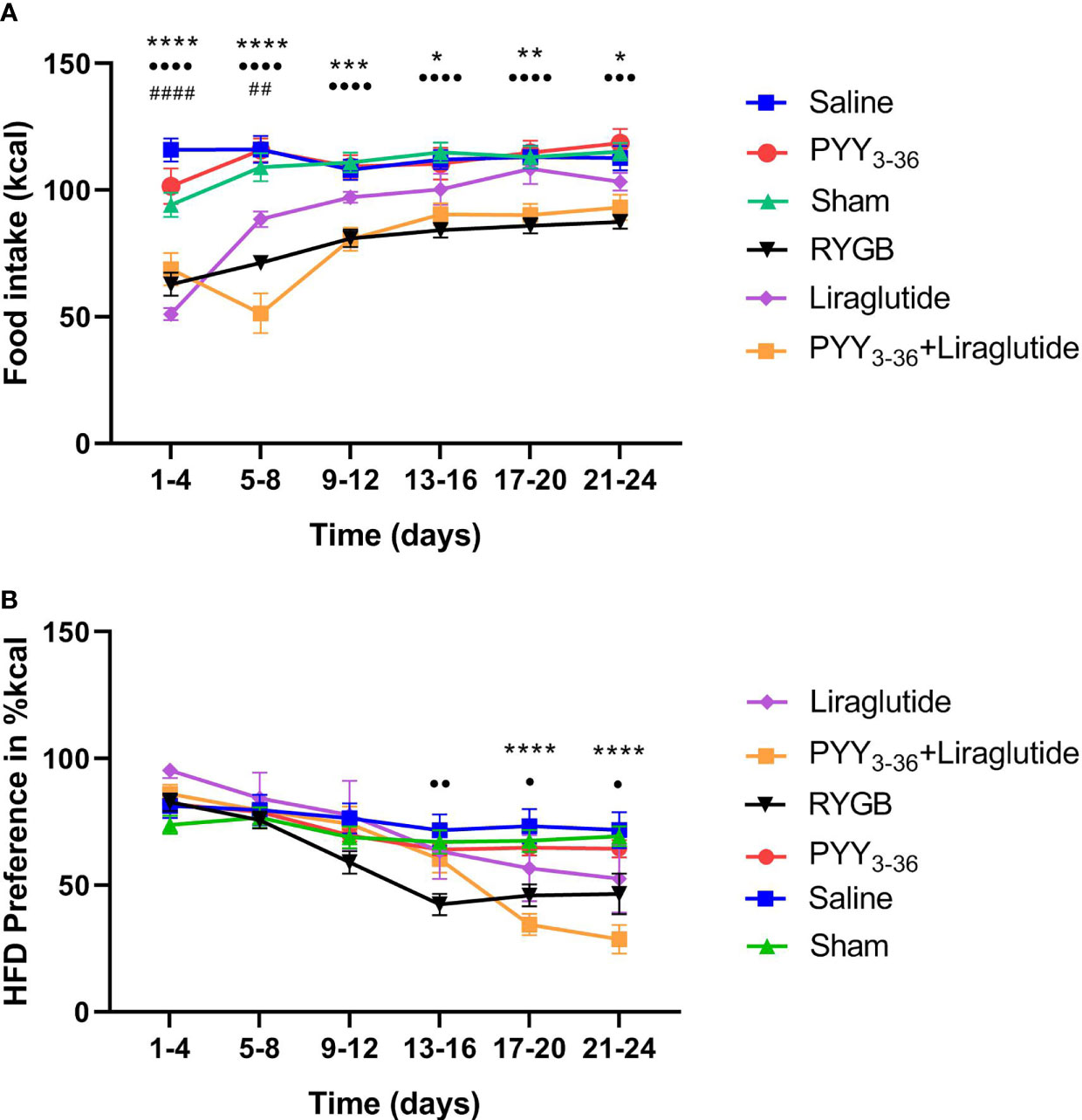
Figure 2 RYGB and PYY3-36+liraglutide lower overall food intake. (A) Food intake in kcal and (B) preference for high-fat diet in percent (kcal) in 4-day intervals of RYGB (n = 14), sham (n = 13), PYY3-36+liraglutide (n = 11), liraglutide (n = 5), PYY3-36 (n = 5), and saline (n = 9) groups post intervention. (A) ••••p ≤ 0.0001 and •••p ≤ 0.001 for differences between RYGB and Sham; ****p ≤ 0.0001, ***p ≤ 0.001, **p ≤ 0.01, *p ≤ 0.05 for differences between PYY3-36+liraglutide and saline; ####p ≤ 0.0001, ##p ≤ 0.01 for differences between liraglutide and saline as determined by two-way ANOVA with Tukey’s post-hoc. (B) •p ≤ 0.05 and ••p ≤ 0.01 for differences between RYGB and Sham and ****p ≤ 0.0001 for difference between PYY3-36+liraglutide and saline as determined by two-way ANOVA with Tukey’s post-hoc. (A, B) Data are presented as mean ± standard error of the mean.
No Abnormal Behavior Was Found Under Any Treatment
During open-field tests no significant differences between RYGB, sham, saline, and PYY3-36 +liraglutide treated animals were found in the open field regarding time in the central area (F (5, 35) = 0.65, p = 0.66; Figure 3) or entries into the central area (F (5, 35) = 1.28, p = 0.29).
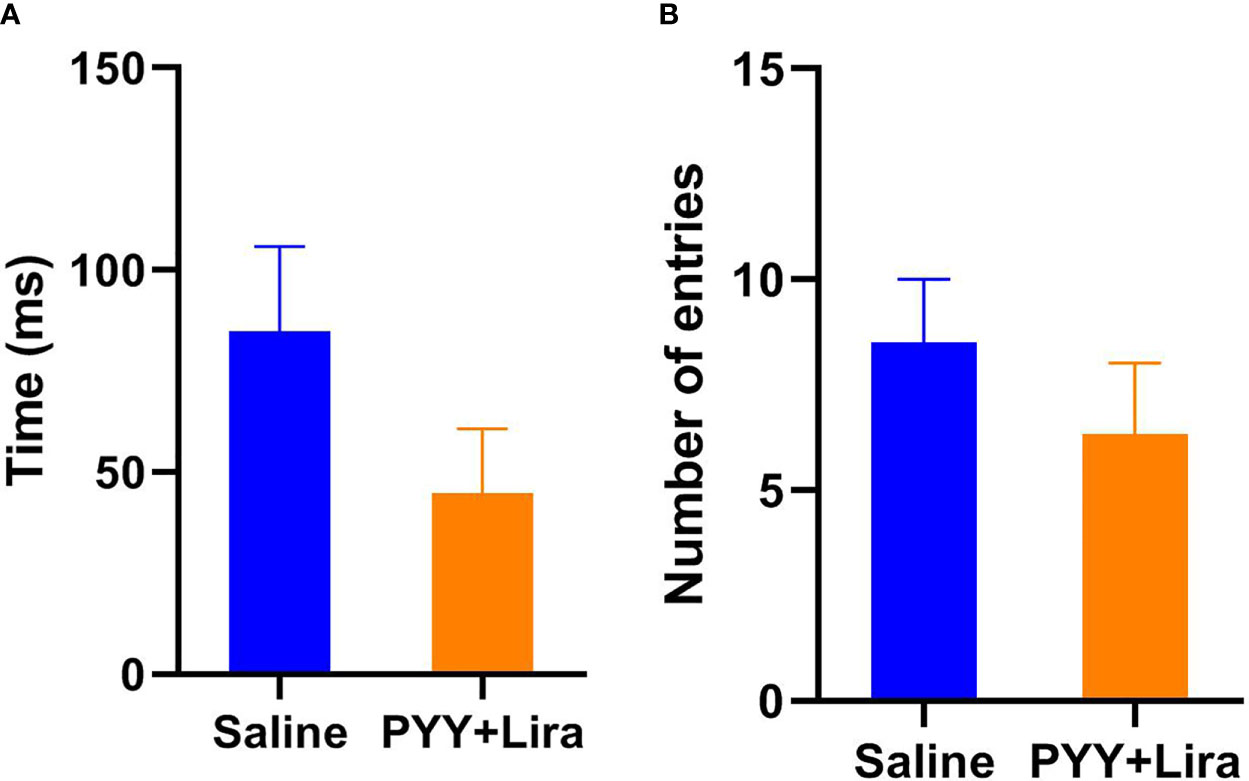
Figure 3 Open field, time in central area. (A) Time (in ms) of the animals in the central area of the open field. (B) Number of entries into the central area of the open field. PYY3-36+liraglutide n = 6, saline n = 4. Data are presented as mean ± standard error of the mean.
No significant differences between RYGB, sham, saline, and PYY3-36 +liraglutide treated animals were found in the elevated plus maze regarding time in the open arms (F (5, 35) = 3.62, p = 0.0096; no sig. difference between RYGB and sham or saline and PYY3-36 +liraglutide in the post-hoc test) or entries into the open arms (F (5, 35) = 1.02, p = 0.42).
Roux-En-Y Gastric Bypass and Peptide Tyrosine Tyrosine 3-36+Liraglutide Leads to Similar Reductions of Retroperitoneal and Epididymal Fat Pads
Weights of rWAT and eWAT were significantly lower in RYGB, PYY3-36 +liraglutide and liraglutide treated animals compared to their respective control group (F (5, 50) = 22.4, p ≤ 0.0001). Comparing amounts of eWAT+rWAT, no differences were found between RYGB, PYY3-36 +liraglutide and liraglutide treated animals (Figure 1C).
Discussion
The mechanisms behind the weight lowering effect of RYGB are not entirely understood. It has been repeatedly shown, however, that meal-stimulated levels of the enteroendocrine hormones peptide tyrosine tyrosine 3-36 (PYY3-36), glucagon-like peptide-1 (GLP-1), and other L-cell derived hormones are remarkably increased after RYGB (23–32). The relevance of their changed secretion for the effectivity of RYGB seems convincing but is still unclear, as well as their synergistic effectivity for the treatment of obesity, which we aimed to examine. Studies in which signaling of the GLP-1 receptor or the PYY3-36-preferring Y2 receptor are perturbed after RYGB have generally shown little to no influence on food intake or body weight (33–37). Additionally, RYGB increases plasma levels of various other anorexigenic gut hormones including oxyntomodulin (52, 53) and neurotensin (54–56), which also reduce food intake. In this sense, a combination therapy with GLP-1, oxyntomodulin and PYY has been shown to achieve superior glucose tolerance and reduced glucose variability compared with RYGB, while its effect on body weight was also less pronounced (52).
In the present study, we directly compared the effects of RYGB and liraglutide + PYY3-36 combination therapy on food intake, food choice, and body weight in diet-induced obese rats. We found further evidence that a combinatory treatment with PYY3-36 and a GLP-1 agonist (liraglutide) can exert an additive effect on body weight loss similar to that of RYGB, which further underlines their possible usability and efficacy for obesity treatment. The dose of PYY3-36 selected in the present study was the same as that used in a previous study that suppressed food intake in diet-induced obese rats (57). However, a 10-fold higher dose of PYY3-36 (in combination with liraglutide) was used before in hamsters (40). It would therefore be worth testing higher doses of PYY3-36 in combination with liraglutide in future head-to-head comparisons although any potentially adverse side effects would have to be carefully monitored.
As expected, RYGB treated animals lost weight after intervention reaching a stable plateau, while sham treated animals gained body weight continuously (Figures 1A, B), leading to highly significant differences over all weeks post intervention. PYY3-36+liraglutide treated animals showed a very similar food intake and body weight course. Showing the additive effect of the combinatory treatment, PYY3-36+liraglutide treated animals were significantly lighter than liraglutide-only treated animals from week 2 on. Instead, animals, who received PYY3-36 only, showed no significant differences compared to saline treated animals regarding food intake, preference, behavior, or metabolic parameters. Although, the mentioned superiority of PYY3-36+liraglutide over liraglutide strongly indicates that the route of the PYY3-36 application and the substance itself have worked. The detected additive effect might be caused by the manipulation of two different hormonal routes, which are known to reduce food intake independently. If only one route is addressed pharmaceutically, there might be an upregulation of the other and vice versa. Although, this hypothesis would need further confirmation.
The body weight course reflects in measured eWAT and rWAT weights. In this sense, the amount of eWAT+rWAT is significantly lower in PYY3-36+liraglutide, liraglutide, RYGB treated animals compared to their respective control group (Figure 1C). It has been shown, that measurements of fat pads ex-vivo correlate well with in-vivo methods like dual-energy X-ray absorptiometry (58). It has been repeatedly shown that RYGB leads to a reduced energy intake (7–20), as it changes food preference decreasing the intake of calories from fat (7, 10, 12, 15, 17, 18, 20–22). In accordance with this, we have recently found that GLP-1 and PYY3-36 reduce high-fat food preference additively after RYGB in diet-induced obese rats and that these animals had an increased meal-induced GLP-1 and PYY3-36 release (41). In this study, animals of the RYGB and PYY3-36+liraglutide group consumed significantly less food than sham treated animals over the whole observation period after intervention (Figure 2A). Moreover, RYGB treated animals showed a significantly reduced preference for 60% fat diet compared to sham treated animals (Figure 2B) which was also the case for PYY3-36+liraglutide treated animals compared to saline in the end of the observation period.
It would be interesting to assess if overlapping or different neural pathways are recruited by RYGB and PYY3-36+liraglutide combination therapy to reduce preference for high-fat food, which could include hypothalamic and striatal signaling in future studies. This would be even more interesting, as the onset of changes in HFD preference do not overlap in RYGB and PYY3-36+liraglutide treated animals, which might indicate different or additional neural mechanisms.
No significant differences were found in the behavioral tests performed, although, PYY3-36+liraglutide treated animals showed some reduced activity in the open field (Figure 3). This could be due to a known anxiogenic effect of NPY Y2 receptors, at which PYY3-36 mainly mediates its central effects (46). However, the more sensitive EPM did not confirm this observation, which might be therefore less relevant. Altogether, the behavioral tests show no relevant abnormal behavior during medical treatment. This is an important observation, as the fact, that a declining preference for HFD as well as a drop in overall food intake in the second interval (not accompanied by a reduced HFD preference) was shown in PYY3-36+liraglutide treated animals, could imply a certain sickness. All in all, however, we found no hints (especially not for visceral malaise), that this might have been the case over the whole observation period. In contrary, PYY3-36+liraglutide treated animals plateaued body weight and slightly regained weight in the end of the observation period, which contradicts general sickness as well as serious nausea or vomiting (which were not detectable clinically). Additionally, it is well described that malaise usually occurs early on during the treatment with liraglutide, possibly due to a strong activation of neurons in the area postrema. This in itself is not a learning process and is simply an acute pharmacological effect, which would be expected to desensitize with repeated treatments rather than worsen. To show the safety and tolerability of the treatment, additional behavioral tests at later stages of the treatment period should be performed in further studies. As a limitation, the groups liraglutide and PYY3-36 only consisted of only five animals each. Although it has to be mentioned, that the in-group consistency of the mentioned effects was strong. Moreover, our previous experience with RYGB studies clearly suggests that a number of five animals per group is generally sufficient (59–61). However, better-powered and equal group sizes in future studies will allow us to more definitively compare each pharmacological regimen to each other and to RYGB in a standardized setting. Due to unforeseen circumstances, it was not possible to start the individual treatment of all animals on the same day after randomization. This led to the limitation, that RYGB treated animals were significantly lighter than the animals of the PYY3-36+liraglutide group. Although this surely weakens the comparability of the groups to some extent, we still feel that the similar body weight change in percent shows the comparable effectivity of the treatments.
In summary, we demonstrated for the first time in a direct head-to-head comparison, that a combination of PYY3-36 and liraglutide had similar effects on body weight and food intake compared to RYGB. This strengthens their possible role as parts of a combinatory therapy for obesity. Chronic interventions with a combination of GLP-1 agonists and PYY3-36 seem to be very promising. From our observations we surely are not able to conclude, that GLP-1 and PYY3-36 are the main factors for the efficacy of RYGB.
Data Availability Statement
The datasets presented in this study can be found in online repositories. The names of the repository/repositories and accession number(s) can be found below: https://github.com/Dischinger/Towards-a-medical-gastric-bypass.git.
Ethics Statement
The animal study was reviewed and approved by Regierung von Unterfranken, Würzburg, Germany.
Author Contributions
UD, MH, FS, and MF planned the experiments. UD, MH, and FS conducted the experiments. FS and CC performed the surgeries. JH, MK, and CO supported the in vivo experiments. UD, MH, and FS wrote the manuscript. All authors discussed the results and commented on the manuscript. All authors contributed to the article and approved the submitted version.
Funding
The research in this manuscript was funded by the IZKF (grant number IZKF Z-2/71).
Conflict of Interest
The authors declare that the research was conducted in the absence of any commercial or financial relationships that could be construed as a potential conflict of interest.
References
1. Kim DD, Basu A. Estimating the Medical Care Costs of Obesity in the United States: Systematic Review, Meta-Analysis, and Empirical Analysis. Value Health (2016) 19(5):602–13. doi: 10.1016/j.jval.2016.02.008
2. Williams EP, Mesidor M, Winters K, Dubbert PM, Wyatt SB. Overweight and Obesity: Prevalence, Consequences, and Causes of a Growing Public Health Problem. Curr Obes Rep (2015) 4(3):363–70. doi: 10.1007/s13679-015-0169-4
3. Schauer PR, Bhatt DL, Kirwan JP, Wolski K, Aminian A, Brethauer SA, et al. Bariatric Surgery versus Intensive Medical Therapy for Diabetes - 5-Year Outcomes. N Engl J Med (2017) 376(7):641–51. doi: 10.1056/NEJMoa1600869
4. Mingrone G, Panunzi S, De Gaetano A, Guidone C, Iaconelli A, Nanni G, et al. Bariatric-metabolic surgery versus conventional medical treatment in obese patients with type 2 diabetes: 5 year follow-up of an open-label, single-centre, randomised controlled trial. Lancet (2015) 386(9997):964–73. doi: 10.1016/S0140-6736(15)00075-6
5. Cummings DE, Arterburn DE, Westbrook EO, Kuzma JN, Stewart SD, Chan CP, et al. Gastric bypass surgery vs intensive lifestyle and medical intervention for type 2 diabetes: the CROSSROADS randomised controlled trial. Diabetologia (2016) 59(5):945–53. doi: 10.1007/s00125-016-3903-x
6. Sjostrom L, Peltonen M, Jacobson P, Sjostrom CD, Karason K, Wedel H, et al. Bariatric surgery and long-term cardiovascular events. JAMA (2012) 307(1):56–65. doi: 10.1001/jama.2011.1914
7. Olbers T, Bjorkman S, Lindroos A, Maleckas A, Lonn L, Sjostrom L, et al. Body composition, dietary intake, and energy expenditure after laparoscopic Roux-en-Y gastric bypass and laparoscopic vertical banded gastroplasty: a randomized clinical trial. Ann Surg (2006) 244(5):715–22. doi: 10.1097/01.sla.0000218085.25902.f8
8. Coluzzi I, Raparelli L, Guarnacci L, Paone E, Del Genio G, le Roux CW, et al. Food Intake and Changes in Eating Behavior After Laparoscopic Sleeve Gastrectomy. Obes Surg (2016) 26(9):2059–67. doi: 10.1007/s11695-015-2043-6
9. Brown EK, Settle EA, Van Rij AM. Food intake patterns of gastric bypass patients. J Am Diet Assoc (1982) 80(5):437–43.
10. Laurenius A, Larsson I, Melanson KJ, Lindroos AK, Lonroth H, Bosaeus I, et al. Decreased energy density and changes in food selection following Roux-en-Y gastric bypass. Eur J Clin Nutr (2013) 67(2):168–73. doi: 10.1038/ejcn.2012.208
11. Kenler HA, Brolin RE, Cody RP. Changes in eating behavior after horizontal gastroplasty and Roux-en-Y gastric bypass. Am J Clin Nutr (1990) 52(1):87–92. doi: 10.1093/ajcn/52.1.87
12. Bavaresco M, Paganini S, Lima TP, Salgado W Jr., Ceneviva R, Dos Santos JE, et al. Nutritional course of patients submitted to bariatric surgery. Obes Surg (2010) 20(6):716–21. doi: 10.1007/s11695-008-9721-6
13. Coughlin K, Bell RM, Bivins BA, Wrobel S, Griffen WO Jr. Preoperative and postoperative assessment of nutrient intakes in patients who have undergone gastric bypass surgery. Arch Surg (1983) 118(7):813–6. doi: 10.1001/archsurg.1983.01390070025006
14. Giusti V, Theytaz F, Di Vetta V, Clarisse M, Suter M, Tappy L. Energy and macronutrient intake after gastric bypass for morbid obesity: a 3-y observational study focused on protein consumption. Am J Clin Nutr (2016) 103(1):18–24. doi: 10.3945/ajcn.115.111732
15. Kruseman M, Leimgruber A, Zumbach F, Golay A. Dietary, weight, and psychological changes among patients with obesity, 8 years after gastric bypass. J Am Diet Assoc (2010) 110(4):527–34. doi: 10.1016/j.jada.2009.12.028
16. Laurenius A, Larsson I, Bueter M, Melanson KJ, Bosaeus I, Forslund HB, et al. Changes in eating behaviour and meal pattern following Roux-en-Y gastric bypass. Int J Obes (Lond) (2012) 36(3):348–55. doi: 10.1038/ijo.2011.217
17. Miller GD, Norris A, Fernandez A. Changes in nutrients and food groups intake following laparoscopic Roux-en-Y gastric bypass (RYGB). Obes Surg (2014) 24(11):1926–32. doi: 10.1007/s11695-014-1259-1
18. Molin Netto BD, Earthman CP, Farias G, Landi Masquio DC, Grotti Clemente AP, Peixoto P, et al. Eating patterns and food choice as determinant of weight loss and improvement of metabolic profile after RYGB. Nutrition (2017) 33:125–31. doi: 10.1016/j.nut.2016.05.007
19. Sjostrom L, Lindroos AK, Peltonen M, Torgerson J, Bouchard C, Carlsson B, et al. Lifestyle, diabetes, and cardiovascular risk factors 10 years after bariatric surgery. N Engl J Med (2004) 351(26):2683–93. doi: 10.1056/NEJMoa035622
20. Brolin RE, Robertson LB, Kenler HA, Cody RP. Weight loss and dietary intake after vertical banded gastroplasty and Roux-en-Y gastric bypass. Ann Surg (1994) 220(6):782–90. doi: 10.1097/00000658-199412000-00012
21. le Roux CW, Bueter M, Theis N, Werling M, Ashrafian H, Lowenstein C, et al. Gastric bypass reduces fat intake and preference. Am J Physiol Regul Integr Comp Physiol (2011) 301(4):R1057–66. doi: 10.1152/ajpregu.00139.2011
22. Trostler N, Mann A, Zilberbush N, Avinoach E, Charuzi II. Weight Loss and Food Intake 18 Months following Vertical Banded Gastroplasty or Gastric Bypass for Severe Obesity. Obes Surg (1995) 5(1):39–51. doi: 10.1381/096089295765558141
23. Korner J, Bessler M, Cirilo LJ, Conwell IM, Daud A, Restuccia NL, et al. Effects of Roux-en-Y gastric bypass surgery on fasting and postprandial concentrations of plasma ghrelin, peptide YY, and insulin. J Clin Endocrinol Metab (2005) 90(1):359–65. doi: 10.1210/jc.2004-1076
24. Chan JL, Mun EC, Stoyneva V, Mantzoros CS, Goldfine AB. Peptide YY levels are elevated after gastric bypass surgery. Obesity (Silver Spring) (2006) 14(2):194–8. doi: 10.1038/oby.2006.25
25. Korner J, Inabnet W, Conwell IM, Taveras C, Daud A, Olivero-Rivera L, et al. Differential effects of gastric bypass and banding on circulating gut hormone and leptin levels. Obesity (Silver Spring) (2006) 14(9):1553–61. doi: 10.1038/oby.2006.179
26. le Roux CW, Aylwin SJ, Batterham RL, Borg CM, Coyle F, Prasad V, et al. Gut hormone profiles following bariatric surgery favor an anorectic state, facilitate weight loss, and improve metabolic parameters. Ann Surg (2006) 243(1):108–14. doi: 10.1097/01.sla.0000183349.16877.84
27. Morinigo R, Moize V, Musri M, Lacy AM, Navarro S, Marin JL, et al. Glucagon-like peptide-1, peptide YY, hunger, and satiety after gastric bypass surgery in morbidly obese subjects. J Clin Endocrinol Metab (2006) 91(5):1735–40. doi: 10.1210/jc.2005-0904
28. Morinigo R, Vidal J, Lacy AM, Delgado S, Casamitjana R, Gomis R. Circulating peptide YY, weight loss, and glucose homeostasis after gastric bypass surgery in morbidly obese subjects. Ann Surg (2008) 247(2):270–5. doi: 10.1097/SLA.0b013e31815f6e77
29. Olivan B, Teixeira J, Bose M, Bawa B, Chang T, Summe H, et al. Effect of weight loss by diet or gastric bypass surgery on peptide YY3-36 levels. Ann Surg (2009) 249(6):948–53. doi: 10.1097/SLA.0b013e3181a6cdb0
30. Bose M, Machineni S, Olivan B, Teixeira J, McGinty JJ, Bawa B, et al. Superior appetite hormone profile after equivalent weight loss by gastric bypass compared to gastric banding. Obesity (Silver Spring) (2010) 18(6):1085–91. doi: 10.1038/oby.2009.473
31. Jacobsen SH, Olesen SC, Dirksen C, Jorgensen NB, Bojsen-Moller KN, Kielgast U, et al. Changes in gastrointestinal hormone responses, insulin sensitivity, and beta-cell function within 2 weeks after gastric bypass in non-diabetic subjects. Obes Surg (2012) 22(7):1084–96. doi: 10.1007/s11695-012-0621-4
32. Yousseif A, Emmanuel J, Karra E, Millet Q, Elkalaawy M, Jenkinson AD, et al. Differential effects of laparoscopic sleeve gastrectomy and laparoscopic gastric bypass on appetite, circulating acyl-ghrelin, peptide YY3-36 and active GLP-1 levels in non-diabetic humans. Obes Surg (2014) 24(2):241–52. doi: 10.1007/s11695-013-1066-0
33. Ye J, Hao Z, Mumphrey MB, Townsend RL, Patterson LM, Stylopoulos N, et al. GLP-1 receptor signaling is not required for reduced body weight after RYGB in rodents. Am J Physiol Regul Integr Comp Physiol (2014) 306(5):R352–62. doi: 10.1152/ajpregu.00491.2013
34. Carmody JS, Munoz R, Yin H, Kaplan LM. Peripheral, but not central, GLP-1 receptor signaling is required for improvement in glucose tolerance after Roux-en-Y gastric bypass in mice. Am J Physiol Endocrinol Metab (2016) 310(10):E855–61. doi: 10.1152/ajpendo.00412.2015
35. Mokadem M, Zechner JF, Margolskee RF, Drucker DJ, Aguirre V. Effects of Roux-en-Y gastric bypass on energy and glucose homeostasis are preserved in two mouse models of functional glucagon-like peptide-1 deficiency. Mol Metab (2014) 3(2):191–201. doi: 10.1016/j.molmet.2013.11.010
36. Boland B, Mumphrey MB, Hao Z, Gill B, Townsend RL, Yu S, et al. The PYY/Y2R-Deficient Mouse Responds Normally to High-Fat Diet and Gastric. Nutrients (2019) 11(3):585. doi: 10.3390/nu11030585
37. Svane MS, Jorgensen NB, Bojsen-Moller KN, Dirksen C, Nielsen S, Kristiansen VB, et al. Peptide YY and glucagon-like peptide-1 contribute to decreased food intake after Roux-en-Y gastric bypass surgery. Int J Obes (Lond) (2016) 40(11):1699–706. doi: 10.1038/ijo.2016.121
38. Boland BB, Mumphrey MB, Hao Z, Townsend RL, Gill B, Oldham S, et al. Combined loss of GLP-1R and Y2R does not alter progression of high-fat diet-induced obesity or response to RYGB surgery in mice. Mol Metab (2019) 25:64–72. doi: 10.1016/j.molmet.2019.05.004
39. Neary NM, Small CJ, Druce MR, Park AJ, Ellis SM, Semjonous NM, et al. Peptide YY3-36 and glucagon-like peptide-17-36 inhibit food intake additively. Endocrinology (2005) 146(12):5120–7. doi: 10.1210/en.2005-0237
40. Dalboge LS, Pedersen PJ, Hansen G, Fabricius K, Hansen HB, Jelsing J, et al. A Hamster Model of Diet-Induced Obesity for Preclinical Evaluation of Anti-Obesity, Anti-Diabetic and Lipid Modulating Agents. PloS One (2015) 10(8):e0135634. doi: 10.1371/journal.pone.0135634
41. Dischinger U, Corteville C, Otto C, Fassnacht M, Seyfried F, Hankir MK. GLP-1 and PYY3-36 reduce high-fat food preference additively after Roux-en-Y gastric bypass in diet-induced obese rats. Surg Obes Relat Dis (2019) 15(9):1483–92. doi: 10.1016/j.soard.2019.04.008
42. Ortiz AA, Milardo LF, DeCarr LB, Buckholz TM, Mays MR, Claus TH, et al. A novel long-acting selective neuropeptide Y2 receptor polyethylene glycol-conjugated peptide agonist reduces food intake and body weight and improves glucose metabolism in rodents. J Pharmacol Exp Ther (2007) 323(2):692–700. doi: 10.1124/jpet.107.125211
43. Holst JJ. The physiology of glucagon-like peptide 1. Physiol Rev (2007) 87(4):1409–39. doi: 10.1152/physrev.00034.2006
44. Vilsboll T, Zdravkovic M, Le-Thi T, Krarup T, Schmitz O, Courreges JP, et al. Liraglutide, a long-acting human glucagon-like peptide-1 analog, given as monotherapy significantly improves glycemic control and lowers body weight without risk of hypoglycemia in patients with type 2 diabetes. Diabetes Care (2007) 30(6):1608–10. doi: 10.2337/dc06-2593
45. Wadden TA, Hollander P, Klein S, Niswender K, Woo V, Hale PM, et al. Weight maintenance and additional weight loss with liraglutide after low-calorie-diet-induced weight loss: the SCALE Maintenance randomized study. Int J Obes (Lond) (2013) 37(11):1443–51. doi: 10.1038/ijo.2013.120
46. Reichmann F, Holzer P. Neuropeptide Y: A stressful review. Neuropeptides (2016) 55:99–109. doi: 10.1016/j.npep.2015.09.008
47. Seyfried F, Bueter M, Spliethoff K, Miras AD, Abegg K, Lutz TA, et al. Roux-en Y gastric bypass is superior to duodeno-jejunal bypass in improving glycaemic control in Zucker diabetic fatty rats. Obes Surg (2014) 24(11):1888–95. doi: 10.1007/s11695-014-1301-3
48. Secher A, Jelsing J, Baquero AF, Hecksher-Sorensen J, Cowley MA, Dalboge LS, et al. The arcuate nucleus mediates GLP-1 receptor agonist liraglutide-dependent weight loss. J Clin Invest (2014) 124(10):4473–88. doi: 10.1172/JCI75276
49. Lipkind D, Sakov A, Kafkafi N, Elmer GI, Benjamini Y, Golani I. New replicable anxiety-related measures of wall vs center behavior of mice in the open field. J Appl Physiol (1985) (2004) 97(1):347–59. doi: 10.1152/japplphysiol.00148.2004
50. Walf AA, Frye CA. The use of the elevated plus maze as an assay of anxiety-related behavior in rodents. Nat Protoc (2007) 2(2):322–8. doi: 10.1038/nprot.2007.44
51. Miras AD, Seyfried F, Phinikaridou A, Andia ME, Christakis I, Spector AC, et al. Rats fed diets with different energy contribution from fat do not differ in adiposity. Obes Facts (2014) 7(5):302–10. doi: 10.1159/000368622
52. Behary P, Tharakan G, Alexiadou K, Johnson N, Wewer Albrechtsen NJ, Kenkre J, et al. Combined GLP-1, Oxyntomodulin, and Peptide YY Improves Body Weight and Glycemia in Obesity and Prediabetes/Type 2 Diabetes: A Randomized, Single-Blinded, Placebo-Controlled Study. Diabetes Care (2019) 42(8):1446–53. doi: 10.2337/dc19-0449
53. Perakakis N, Kokkinos A, Peradze N, Tentolouris N, Ghaly W, Pilitsi E, et al. Circulating levels of gastrointestinal hormones in response to the most common types of bariatric surgery and predictive value for weight loss over one year: Evidence from two independent trials. Metabolism (2019) 101:153997. doi: 10.1016/j.metabol.2019.153997
54. Holdstock C, Zethelius B, Sundbom M, Karlsson FA, Eden Engstrom B. Postprandial changes in gut regulatory peptides in gastric bypass patients. Int J Obes (Lond) (2008) 32(11):1640–6. doi: 10.1038/ijo.2008.157
55. Christ-Crain M, Stoeckli R, Ernst A, Morgenthaler NG, Bilz S, Korbonits M, et al. Effect of gastric bypass and gastric banding on proneurotensin levels in morbidly obese patients. J Clin Endocrinol Metab (2006) 91(9):3544–7. doi: 10.1210/jc.2006-0256
56. Ratner C, He Z, Grunddal KV, Skov LJ, Hartmann B, Zhang F, et al. Long-Acting Neurotensin Synergizes With Liraglutide to Reverse Obesity Through a Melanocortin-Dependent Pathway. Diabetes (2019) 68(6):1329–40. doi: 10.2337/db18-1009
57. Unniappan S, Kieffer TJ. Leptin extends the anorectic effects of chronic PYY(3-36) administration in ad libitum-fed rats. Am J Physiol Regul Integr Comp Physiol (2008) 295(1):R51–8. doi: 10.1152/ajpregu.00234.2007
58. Gerbaix M, Metz L, Ringot E, Courteix D. Visceral fat mass determination in rodent: validation of dual-energy X-ray absorptiometry and anthropometric techniques in fat and lean rats. Lipids Health Dis (2010) 9:140. doi: 10.1186/1476-511X-9-140
59. Arora T, Seyfried F, Docherty NG, Tremaroli V, le Roux CW, Perkins R, et al. Diabetes-associated microbiota in fa/fa rats is modified by Roux-en-Y gastric bypass. ISME J (2017) 11(9):2035–46. doi: 10.1038/ismej.2017.70
60. Hankir MK, Seyfried F, Hintschich CA, Diep TA, Kleberg K, Kranz M, et al. Gastric Bypass Surgery Recruits a Gut PPAR-alpha-Striatal D1R Pathway to Reduce Fat Appetite in Obese Rats. Cell Metab (2017) 25(2):335–44. doi: 10.1016/j.cmet.2016.12.006
Keywords: obesity, rygb, liraglutide, peptide tyrosine tyrosine (PYY), treatment, gastric bypass, peptide tyrosine tyrosine 3-36 (PYY3-36)
Citation: Dischinger U, Hasinger J, Königsrainer M, Corteville C, Otto C, Fassnacht M, Hankir M and Seyfried FJD (2021) Toward a Medical Gastric Bypass: Chronic Feeding Studies With Liraglutide + PYY3-36 Combination Therapy in Diet-Induced Obese Rats. Front. Endocrinol. 11:598843. doi: 10.3389/fendo.2020.598843
Received: 25 August 2020; Accepted: 07 December 2020;
Published: 22 January 2021.
Edited by:
Alexander Miras, Imperial College London, United KingdomReviewed by:
Zhipeng Tao, Massachusetts General Hospital and Harvard Medical School, United StatesDimitri Pournaras, North Bristol NHS Trust, United Kingdom
Brett Johnson, Imperial College, United Kingdom
Copyright © 2021 Dischinger, Hasinger, Königsrainer, Corteville, Otto, Fassnacht, Hankir and Seyfried. This is an open-access article distributed under the terms of the Creative Commons Attribution License (CC BY). The use, distribution or reproduction in other forums is permitted, provided the original author(s) and the copyright owner(s) are credited and that the original publication in this journal is cited, in accordance with accepted academic practice. No use, distribution or reproduction is permitted which does not comply with these terms.
*Correspondence: Ulrich Dischinger, dischinger_u@ukw.de
†These authors share last authorship