- 1Chongqing Public Health Medical Center, Chongqing, China
- 2Infectious Diseases and Immunity in Global Health Program, Research Institute, McGill University Health Centre, Montréal, QC, Canada
- 3Chronic Viral Illness Service, McGill University Health Centre, Montréal, QC, Canada
- 4CIHR Canadian HIV Trials Network, Vancouver, BC, Canada
- 5Department of Microbiology and Immunology, McGill University, Montréal, QC, Canada
- 6State Key Laboratory for Diagnosis and Treatment of Infectious Diseases, National Clinical Research Center for Infectious Diseases, Collaborative Innovation Center for Diagnosis and Treatment of Infectious Diseases, The First Affiliated Hospital, College of Medicine, Zhejiang University, Hangzhou, China
- 7Division of Hematology, McGill University Health Centre, Montréal, QC, Canada
Weight gain and obesity are global health concerns contributing to morbidity with increased risks of cardiovascular disease, diabetes, liver steatohepatitis and cancer. Pharmacological therapies or bariatric surgery are often required for those who fail to adhere to diet and lifestyle modifications. Metformin, a widely used antidiabetic agent, seems to have a health benefit beyond its anti-hyperglycemic properties, with few side effects. Emerging evidence shows weight loss to be associated with metformin in both diabetic and non-diabetic individuals. Recently, the growth differentiation factor 15 (GDF-15), a member of the transforming growth factor beta superfamily, has been identified as a key mediator of metformin-induced weight loss. Metformin increases the secretion of GDF-15, which binds exclusively to glial cell-derived neurotrophic factor family receptor alpha-like (GFRAL). This gut-brain cytokine works as a prominent player in reducing food intake and body weight in health and disease, like anorexia nervosa and cancer. Herein, we critically review advances in the understanding of the weight-reducing effects of metformin via the GDF-15 pathway.
Introduction
Weight gain and obesity represent the second most common causes of preventable mortalities worldwide, and promote the development of cardiovascular disease, hypertension, stroke, dyslipidemia, metabolic syndrome, liver steatohepatitis, and cancer (1, 2). Diet and lifestyle modifications are the first line interventions for the management of body weight, however long-term adherence remains difficult. As such, pharmacological interventions are needed. However, due to the cost and the risk of side effects, few drugs are currently available.
Isolated from French lilac in 1920s, metformin (dimethylbiguanide) has been widely used as a first-line treatment for type 2 diabetes mellitus (DM2), owing to its excellent tolerability, safety profile, and lack of hypoglycemic effect (3–6). Aside from its anti-hyperglycemic effects, metformin has an additional benefit for conditions like polycystic ovary syndrome, atherosclerosis, cancer, coronavirus disease 2019 (COVID-19), and obesity (7–14). Metformin was also reported to extend lifespan in some animal models, acting as a diet mimetic agent (15–17). In contrast to other antidiabetic drugs including insulin, metformin can lead independently to glycemic control and weight loss by decreasing food intake in both diabetic and non-diabetic individuals (18–20). Thus, these observations have driven metformin’s emergence as a research priority to counteract diseases associated with obesity and aging, evocating an immune-metabolic effect.
Bodyweight is usually maintained through central nervous system (CNS) circuitry integrating peripheral metabolic feedback signals of either energy surplus or deficit (21, 22). Studies have shown converging evidence that growth differentiation factor 15 (GDF-15) mediates metformin’s effect on the gut-nervous system axis to decrease body weight (23–26). GDF-15, a member of the transforming growth factor beta (TGF-β) superfamily, has many names indicating its multiple functions, including macrophage inhibitory cytokine (MIC)-1, non-steroidal anti-inflammatory drug-inducible gene (NAG)-1, placental TGF-β (PTGF), prostate-derived factor (PDF), and placental bone morphogenetic protein (PLAB) (27). GDF-15 is a stress-induced protein, produced by a variety of cells under stress conditions including tissue injury, anoxia, and inflammation (28). Elevated circulating GDF-15 levels have been associated with more severe disease or higher mortality in people with DM2, insulin resistance, hemodialysis, cachexia, cardiovascular diseases, chronic obstructive pulmonary disease (COPD), venous thromboembolism (VTE), cancer, or obesity (28–34). Husebø et al. reported that high levels of GDF15 were independently associated with a higher rate of COPD exacerbations, and impaired respiratory function (34). From a prospective cohort of 27,158 adults followed over 13 years, among 12 tested biomarkers, GDF-15 and D-dimer were independently associated with the occurrence of VTE in multivariable analyses. Importantly, the association between GDF-15 levels and VTE was independent of D-dimer and von Willebrand factor, which are well-established biomarkers for thrombosis (35). Therefore, the elevation of GDF-15 levels during those conditions are believed to be a compensatory mechanism, as numerous evidences proposed GDF-15 as a biomarker rather than an inducer of these diseases. GDF-15 has also been shown to inhibit apoptosis and inflammation, and protects the heart from injury (36–38). In addition to these functions, GDF-15 and its tissue-specific brainstem receptor, the glial cell-derived neurotrophic factor (GDNF) family receptor alpha-like (GFRAL), have emerged as regulators of energy balance and body weight (39–41). Higher circulating GDF-15 levels have been proven to be significantly associated with weight loss in cancer patients (42, 43). To review the influence of the GDF-15 pathway on weight loss induced by metformin, we searched for the keywords “GDF-15”, “metformin”, “weight”, “diabetes drugs” alone, or in combination in the public databases of PubMed, Google Scholar, and ClinicalTrials.gov. As the relationship between GDF-15 and anti-diabetic drugs is new, we were able to discuss all English publications. In this review, we critically discuss advances in the understanding of the weight-reducing effects of metformin through modulation of GDF-15 levels in diabetic and non-diabetic individuals.
Metformin Decreases Body Weight in Diabetic and Non-Diabetic Individuals
Dysglycemia is strongly associated with the development of overweight status or obesity, as most DM2 patients are overweight or obese (44–46). For diabetic patients, managing overweight or obesity is a crucial facet of diabetes management. However, the majority of antidiabetic agents, including insulin, thiazolidinediones, (TZDs) and insulin secretagogues, lead to body weight gain while controlling glycemia (47–49). Conversely, the first-line antidiabetic agent, metformin, has been shown to be able to decrease body weight (18, 19, 49–52). Kahn et al. (49) reported in a large randomized clinical trial (RCT) involving 4360 DM2 patients that participants lost a mean of 2.9 kg with metformin over a period of 5 years, while rosiglitazone and glyburide both induced weight gains of 4.8 and 1.6 kg, respectively. The Diabetes Prevention Program Research Group reported that metformin users had significantly reduced body weight and waist circumference compared with placebo in a 2-year RCT followed by a 8-year open-label extension. The magnitude of weight loss during the 2-year double-blind period was directly related to drug adherence, indicating a potential dose-effect (19). In 2016, a meta-analysis by Maruthur et al. showed that metformin decreased body weight more than dipeptidyl peptidase-4 (DPP-4) inhibitors which were expected to decrease body weight (18). Due to the effects of weight loss, metformin was recommended for obesity management in patients with evidence of prediabetes or insulin intolerance by AACE/ACE guidelines (53).
Aside from diabetic patients, metformin was also associated with a decrease of bodyweight in non-diabetic subjects (20, 54–57). Ejtahed et al. (20) demonstrated in a RCT that metformin induced significant weight loss compared with placebo, and this effect was associated with gut microbiota alteration in non-diabetic obese women. Furthermore, metformin has been used in obese children to promote weight loss in absence of DM2 (58–61). For adults and children with schizophrenia, metformin has been used to manage weight gain associated with anti-psychotic drugs, reducing risks of metabolic syndrome, diabetes and cardiovascular disorders (62–66). Moreover, obesity and insulin resistance are associated with pathogenesis of polycystic ovarian syndrome (PCOS), a condition characterized by a reduced frequency of ovulation, infertility, and hyperandrogenism in premenopausal women (67, 68). Metformin’s effect on weight in women with PCOS is not as well defined, depending on the population and study design (69–74). However, a meta-analysis showed that metformin contributed to a decrease of body mass index (BMI) and waist to hip ratio (WHR) in 11 and 7 RCTs of PCOS women respectively, compared to placebo (75). Finally, a 12-week metformin treatment decreased the weight of non-diabetic people living with HIV under antiretroviral therapy (25).
Metformin use has been associated with weight loss and it is noteworthy that metformin-associated weight loss was of a lesser extent in non-diabetic people. As such obese people may benefit from bariatric surgery more readily than from metformin (76). Although most studies have confirmed that metformin could decrease body weight in diabetic and non-diabetic subjects, the mechanism still remains unclear. Aside from the mechanisms summarized in two reviews (77, 78), recent study findings indicate that GDF-15 plays an independent role in body weight change in people taking metformin.
Metformin Induces GDF-15 Via ATF4 and CHOP
Converging findings showed that metformin induced gdf-15 gene expression and elevated the circulating GDF-15 level in animal and human models (Table 1). Metformin-induced expression was notably detected in gut and kidney epithelial cells (23). Metformin and other biguanides such as phenformin were shown to induce GDF-15 expression in murine and human hepatocytes (23), while the direct effect of other anti-diabetes drugs has not been studied yet. In vitro, 1mM of metformin upregulated GDF-15 gene expression in breast cancer cells to 26-fold compared with control (79). Higher concentrations of metformin (10-100 mM) in mesenchymal stem cells (MSCs) increased GDF-15 expression in a dose-dependent manner under normoglycemic conditions. Interestingly, this effect was hindered in hyperglycemic conditions (80). In vivo, Gerstein et al. assayed 237 biomarkers in baseline serum from 8,401 participants with dysglycemia of whom 2,317 received metformin and found that GDF-15 was linked to metformin treatment, in a dose dependent manner (1 per mg of metformin treatment led to 8.7 pg/ml of GDF-15 increased in plasma). Moreover, Coll et al. (23) reported in two independent RCT that metformin reduced food intake and lowered body weight in association with increasing levels of GDF-15. However, the same group showed that metformin retained its ability to lower circulating glucose and fasting insulin levels in GDF-15 knock-out mice. These findings suggest that GDF-15 mediates the beneficial effects of metformin on energy balance and weight loss, independently of insulin pathways. However, based on the negative effect of weight on insulin sensitivity, it was speculated that GDF-15-dependent weight loss contributed to enhance insulin sensitivity. We conducted a prospective study to examine the effect of metformin on body weight in non-diabetic, non-obese people living with HIV and receiving effective antiretroviral therapy (ART). We showed that metformin, independent of its glucose-lowering effect, increased plasma levels of GDF-15 and decreased weight, and its effects vanished upon discontinuation, establishing a direct cause-effect relationship between GDF-15 plasma level change and weight change during and after metformin discontinuation (25).
Although the mechanism responsible for metformin-induced GDF-15 expression is not yet deciphered, several pathways have been described to induce GDF-15 expression. In diabetic patients, hyperglycemia causes a stress condition leading to reactive oxygen species (ROS) overproduction, which further induces cellular apoptosis, cellular injury and cell death by inhibiting the PI3K/AKT/eNOS/NO pathway and activating the NF-κB/JNK/caspase-3 pathway (83–85). In vitro, Li et al. revealed that high glucose could induce GDF-15 expression and secretion in cultured human umbilical vein endothelial cells in a ROS- and p53-dependent manner (86). The transcriptional factor p53 regulates GDF-15 expression and was shown to link GDF-15 with obesity and insulin resistance (87). Obesity promotes p53 activation in adipose tissue and leads to increased production of proinflammatory cytokines and increased insulin resistance. When p53 was inhibited by RNA silencing, the effect of GDF-15 induction by high glucose vanished (86). In humans, high levels of plasma GDF-15 have been associated with type 2 diabetes and cardiovascular events (88–90). However, GDF-15 was shown to protect human islet cells from apoptosis and was suggested to have a protective role in diabetic mice (91). Metformin is also suggested to prevent cardiovascular diseases and to have anti-aging effects (15, 92–94), although these results need to be confirmed (95). As such, the clinical implication of metformin-induced GDF-15 increase will have to be assessed in future RCTs.
Aside from p53, several other factors have been implicated in the transcriptional regulation of GDF-15, including p63, Sp1, early growth response-1 (EGR-1), activating the integrated stress response transcription factor 4 (ATF4), C/EBP homologous protein (CHOP), and SMAD2/3 (96–103). Patel et al. reported that GDF-15 levels increased following sustained high-fat feeding or dietary amino acid imbalance in mice, and that GDF-15 expression is regulated by the integrated stress response, in which key transcriptional regulators like ATF4 and CHOP are involved (102, 104). Induced GDF-15 expression by the stressor tunicamycin was abolished in ATF4 knockout mouse embryonic fibroblasts and significantly reduced in CHOP-knockdown cells (102). Similarly, Chung et al. (100) showed that induction of GDF-15 upon mitochondrial unfolded protein response (UPRmt) activation was CHOP-dependent. Interestingly, metformin was reported to increase the expression of ATF4 and CHOP, further stimulating the secretion of GDF-15 in mouse and human hepatocytes (24). Therefore, current evidence indicates that metformin increases GDF-15 gene expression and stimulate GDF-15 secretion by direct induction of integrated stress response regulators ATF4 and CHOP.
GDF-15 and Weight Loss: Evidencesand Mechanism
A direct association between weight and GDF-15 was studied in animal and humans. Altered GDF-15 levels in comparison to matched lean controls have been frequently reported in obese mice, rats, and humans (32, 105). In addition, elevated GDF-15 expression and circulating levels correlate with further weight loss, reduce food intake and appetite (42, 43, 106). Patients with metastatic lung cancer who reported >5% weight loss were found to exhibit a twofold increase in GDF-15 plasma levels compared to those without weight loss (42). Tsai et al. (107) reported that GDF-15 gene knockout mice (Gdf-15 (-/-)) weighed more and had increased adiposity, which was associated with increased food intake, and that infusion of human recombinant GDF-15 was sufficient to raise serum levels in Gdf-15 (-/-) mice to within the normal human range, reducing body weight and food intake. On the contrary, overexpressing GDF-15 led to decreased body weight, fat mass and food intake, improving the glucose tolerance in mouse models (83, 108–110). GDF-15 has thus become an attractive target for reducing obesity. In line with these studies, exogenous administration of recombinant murine or human GDF-15 induced weight loss in different animal models (26, 32, 40, 41, 107). Xiong et al. (32) showed that Fc fusion GDF-15 molecules with extended half-life and increased efficacy in obese mice, rats, and cynomolgus monkeys was able to delay gastric emptying, change food preference, and activate area postrema neurons, confirming a role for GDF-15 in the gut-brain axis responsible for the regulation of body energy intake. Moreover, pharmacological recombinant human GDF-15 administration to mice can trigger conditioned-taste aversion, suggesting that GDF-15 may induce an aversive response to nutritional stimulation and may be associated with nausea in pregnancy (102). These results were further confirmed by Coll et al. as metformin did not induce weight loss when administered to gdf-15 knock out mice (23).
Mechanistically, the weight-related effect of GDF-15 is dependent on its receptor GFRAL and coreceptor tyrosine kinase RET (Figure 1). Unlike Gdf-15 which is expressed in diverse tissues, including kidney, liver, gut, muscle, adipose, and placenta, GFRAL expression is limited to the brainstem and Gfral mRNA is highly expressed in the area postrema of mouse, rat, monkey and human (39–41). GFRAL was previously considered as an orphan receptor with no endogenous ligand (111, 112). Recently, GDF-15 was validated as the only GFRAL ligand with a high-affinity. Flow cytometry analyses showed that GFRAL solely bound to GDF-15, but not to GDNF and its homologs neurturin, artemin, and persephin (26). Interestingly, GDF-15 also exclusively binds to GFRAL, and not to any other TGF-β receptors nor to other members of the GDNF family of receptors (39–41). In the brain, activation of the GFRAL receptor leads to a complex activation of a neuronal network involving the nucleus of the solitary tract, the hypothalamus, and the central amygdala, reducing food intake and appetite (Figure 1) (96). Gfral knockout mice are hyperphagic under stressed conditions and are resistant to chemotherapy-induced anorexia and body weight loss. Moreover, the effect of GDF-15 on body weight and food intake reduction in wild-type mice was completely lost in Gfral knockout mice (39). GFRAL antibody blocked GDF-15-induced body weight and food-intake suppression in rats (39). Additionally, GDF-15-induced cell signaling requires the interaction of GFRAL with the coreceptor RET (39). GDF-15 forms a complex with GFRAL and RET on the cell surface, then triggers an intracellular signaling cascade through the extracellular signal-related kinase (ERK) pathway (40, 113). Blocking RET by inhibitor or mRNA depletion could also prevent GDF-15-mediated signaling in neuroblastoma cells (41).
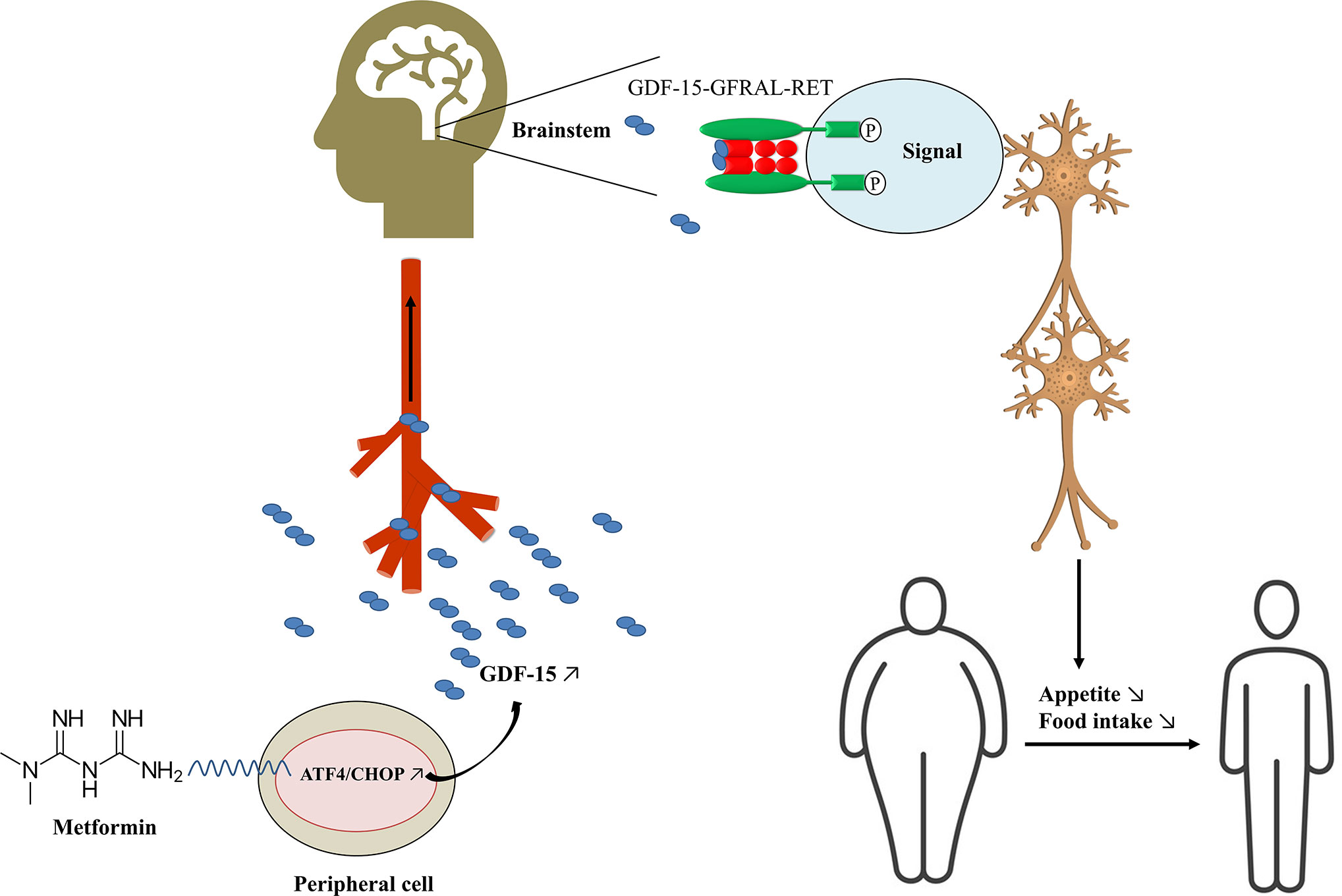
Figure 1 Potential mechanism of metformin decreasing body weight. GDF-15, growth differentiation factor 15; GFRAL, glial cell-derived neurotrophic factor (GDNF) family receptor alpha-like (GFRAL); ATF4, activating transcription factor 4; CHOP, C/EBP homologous protein.
Conclusion
Metformin has emerged as an effective weight-reducing medication in different animal and human models by increasing GDF-15 levels, which works as a “weight watcher” to maintain homeostasis. Metformin induces the expression of integrated stress response regulators ATF4 and CHOP, which stimulates the secretion of circulating GDF-15, which then binds to its exclusive receptor GFRAL and coreceptor RET in the brainstem. Intracellular signaling of the GDF-15/GFRAL/RET leads to feelings of satiety and control of appetite, resulting in decreased body weight (Figure 1). However, more evidence is needed to verify the role of GDF-15 as a biomarker of metformin’s weight-reducing effects in diabetic and non-diabetic individuals. In the future, metformin dose and duration should be well-defined, and use GDF-15 as a biomarker of metformin-induced weight loss will have to be confirmed. Moreover, the clinical implication and use of GDF-15 as a biomarker should be studied in large RCTs.
Author Contributions
JO and SI wrote the first draft of the manuscript. JL, BF, and XP provided critical revision of the manuscript. YC and J-PR conceived and designed the manuscript. All authors contributed to the article and approved the submitted version.
Funding
Our research is funded by the Fonds de la Recherche Québec-Santé (FRQ-S): Réseau SIDA/Maladies infectieuses and Thérapie cellulaire, the Canadian Institutes of Health Research (CIHR; grants MOP 103230 and PTJ 166049), the Vaccines & Immunotherapies Core of the CIHR Canadian HIV Trials Network (CTN; CTN PT027), the Canadian Foundation for AIDS Research (CANFAR; grant 02-512), and CIHR-funded Canadian HIV Cure Enterprise (CanCURE) Team Grant HB2-164064.
Conflict of Interest
The authors declare that the research was conducted in the absence of any commercial or financial relationships that could be construed as a potential conflict of interest.
Acknowledgments
We are highly grateful to Angie Massicotte, Josée Girouard, and Cezar Iovi for coordination and assistance.
References
1. Paccosi S, Cresci B, Pala L, Rotella CM, Parenti A. Obesity Therapy: How and Why? Curr Med Chem (2020) 27:174–86. doi: 10.2174/0929867326666190124121725
2. Nijhawans P, Behl T, Bhardwaj S. Angiogenesis in obesity. BioMed Pharmacother (2020) 126:110103. doi: 10.1016/j.biopha.2020.110103
3. American Diabetes Association. Standards of Medical Care in Diabetes-2019 Abridged for Primary Care Providers. Clin Diabetes Publ Am Diabetes Assoc (2019) 37:11–34. doi: 10.2337/cd18-0105
4. Yale JF, Paty B, Senior PA. Hypoglycemia. Can J Diabetes (2018) 42(Suppl 1):S104–s108. doi: 10.1016/j.jcjd.2017.10.010
5. Harper W, Clement M, Goldenberg R, Hanna A, Main A, Retnakaran R, et al. Pharmacologic management of type 2 diabetes. Can J Diabetes (2013) 37(Suppl 1):S61–8. doi: 10.1016/j.jcjd.2013.01.021
6. Bonadonna RC, Yale JF, Brulle-Wohlhueter C, Boëlle-Le Corfec E, Choudhary P, Bailey TS. Hypoglycaemia as a function of HbA1c in type 2 diabetes: Insulin glargine 300 U/mL in a patient-level pooled analysis of EDITION 1, 2 and 3. Diabetes Obes Metab (2019) 21:715–9. doi: 10.1111/dom.13578
7. Pollak M. The effects of metformin on gut microbiota and the immune system as research frontiers. Diabetologia (2017) 60:1662–7. doi: 10.1007/s00125-017-4352-x
8. Kim SA, Choi HC. Metformin inhibits inflammatory response via AMPK-PTEN pathway in vascular smooth muscle cells. Biochem Biophys Res Commun (2012) 425:866–72. doi: 10.1016/j.bbrc.2012.07.165
9. Hirsch HA, Iliopoulos D, Struhl K. Metformin inhibits the inflammatory response associated with cellular transformation and cancer stem cell growth. Proc Natl Acad Sci U.S.A. (2013) 110:972–7. doi: 10.1073/pnas.1221055110
10. Li SN, Wang X, Zeng QT, Feng YB, Cheng X, Mao XB, et al. Metformin inhibits nuclear factor kappaB activation and decreases serum high-sensitivity C-reactive protein level in experimental atherogenesis of rabbits. Heart Vessels (2009) 24:446–53. doi: 10.1007/s00380-008-1137-7
11. Victor VM, Rovira-Llopis S, Banuls C, Diaz-Morales N, Lopez-Domenech S, Escribano-Lopez I, et al. Metformin modulates human leukocyte/endothelial cell interactions and proinflammatory cytokines in polycystic ovary syndrome patients. Atherosclerosis (2015) 242:167–73. doi: 10.1016/j.atherosclerosis.2015.07.017
12. Arrieta O, Barron F, Padilla MS, Aviles-Salas A, Ramirez-Tirado LA, Arguelles Jimenez MJ, et al. Effect of Metformin Plus Tyrosine Kinase Inhibitors Compared With Tyrosine Kinase Inhibitors Alone in Patients With Epidermal Growth Factor Receptor-Mutated Lung Adenocarcinoma: A Phase 2 Randomized Clinical Trial. JAMA Oncol (2019), e192553. doi: 10.1001/jamaoncol.2019.2553
13. Luo P, Qiu L, Liu Y, Liu XL, Zheng JL, Xue HY, et al. Metformin Treatment Was Associated with Decreased Mortality in COVID-19 Patients with Diabetes in a Retrospective Analysis. Am J Trop Med Hyg (2020) 103:69–72. doi: 10.4269/ajtmh.20-0375
14. El-Arabey AA, Abdalla M. Metformin and COVID-19: A novel deal of an old drug. J Med Virol (2020) 92:2293–4. doi: 10.1002/jmv.25958
15. Onken B, Driscoll M. Metformin induces a dietary restriction-like state and the oxidative stress response to extend C. elegans Healthspan via AMPK, LKB1, and SKN-1. PloS One (2010) 5:e8758. doi: 10.1371/journal.pone.0008758
16. Martin-Montalvo A, Mercken EM, Mitchell SJ, Palacios HH, Mote PL, Scheibye-Knudsen M, et al. Metformin improves healthspan and lifespan in mice. Nat Commun (2013) 4:2192. doi: 10.1038/ncomms3192
17. Pryor R, Norvaisas P, Marinos G, Best L, Thingholm LB, Quintaneiro LM, et al. Host-Microbe-Drug-Nutrient Screen Identifies Bacterial Effectors of Metformin Therapy. Cell (2019) 178:1299–1312.e29. doi: 10.1016/j.cell.2019.08.003
18. Maruthur NM, Tseng E, Hutfless S, Wilson LM, Suarez-Cuervo C, Berger Z, et al. Diabetes Medications as Monotherapy or Metformin-Based Combination Therapy for Type 2 Diabetes: A Systematic Review and Meta-analysis. Ann Intern Med (2016) 164:740–51. doi: 10.7326/M15-2650
19. Diabetes Prevention Program Research Group. Long-term safety, tolerability, and weight loss associated with metformin in the Diabetes Prevention Program Outcomes Study. Diabetes Care (2012) 35:731–7. doi: 10.2337/dc11-1299
20. Ejtahed HS, Tito RY, Siadat SD, Hasani-Ranjbar S, Hoseini-Tavassol Z, Rymenans L, et al. Metformin induces weight loss associated with gut microbiota alteration in non-diabetic obese women: a randomized double-blind clinical trial. Eur J Endocrinol (2018) 180:165–76. doi: 10.1530/EJE-18-0826
21. Rebello CJ, Greenway FL. Obesity medications in development. Expert Opin Invest Drugs (2020) 29:63–71. doi: 10.1080/13543784.2020.1705277
22. Papathanasiou AE, Nolen-Doerr E, Farr OM, Mantzoros CS. GEOFFREY HARRIS PRIZE LECTURE 2018: Novel pathways regulating neuroendocrine function, energy homeostasis and metabolism in humans. Eur J Endocrinol (2019) 180:R59–r71. doi: 10.1530/EJE-18-0847
23. Coll AP, Chen M, Taskar P, Rimmington D, Patel S, Tadross JA, et al. GDF15 mediates the effects of metformin on body weight and energy balance. Nature (2020) 578:444–8. doi: 10.1038/s41586-019-1911-y
24. Day EA, Ford RJ, Smith BK, Mohammadi-Shemirani P, Morrow MR, Gutgesell RM, et al. Metformin-induced increases in GDF15 are important for suppressing appetite and promoting weight loss. Nat Metab (2019) 1:1202–8. doi: 10.1038/s42255-019-0146-4
25. Isnard S, Lin J, Fombuena B, Ouyang J, Varin TV, Richard C, et al. Repurposing metformin in non-diabetic people living with HIV: Influence on weight and gut microbiota. Open Forum Infect Dis (2020) 7. doi: 10.1093/ofid/ofaa338
26. Yang L, Chang CC, Sun Z, Madsen D, Zhu H, Padkjær SB, et al. GFRAL is the receptor for GDF15 and is required for the anti-obesity effects of the ligand. Nat Med (2017) 23:1158–66. doi: 10.1038/nm.4394
27. Wischhusen J, Melero I, Fridman WH. Growth/Differentiation Factor-15 (GDF-15): From Biomarker to Novel Targetable Immune Checkpoint. Front Immunol (2020) 11:951. doi: 10.3389/fimmu.2020.00951
28. Adela R, Banerjee SK. GDF-15 as a Target and Biomarker for Diabetes and Cardiovascular Diseases: A Translational Prospective. J Diabetes Res (2015) 2015:490842. doi: 10.1155/2015/490842
29. Benes J, Kotrc M, Wohlfahrt P, Conrad MJ, Franekova J, Jabor A, et al. The Role of GDF-15 in Heart Failure Patients With Chronic Kidney Disease. Can J Cardiol (2019) 35:462–70. doi: 10.1016/j.cjca.2018.12.027
30. Arkoumani M, Papadopoulou-Marketou N, Nicolaides NC, Kanaka-Gantenbein C, Tentolouris N, Papassotiriou I. The clinical impact of growth differentiation factor-15 in heart disease: A 2019 update. Crit Rev Clin Lab Sci (2020) 57:114–25. doi: 10.1080/10408363.2019.1678565
31. Spanopoulou A, Gkretsi V. Growth differentiation factor 15 (GDF15) in cancer cell metastasis: from the cells to the patients. Clin Exp Metastasis (2020) 37:451–64. doi: 10.1007/s10585-020-10041-3
32. Xiong Y, Walker K, Min X, Hale C, Tran T, Komorowski R, et al. Long-acting MIC-1/GDF15 molecules to treat obesity: Evidence from mice to monkeys. Sci Transl Med (2017) 9:eaan8732. doi: 10.1126/scitranslmed.aan8732
33. You AS, Kalantar-Zadeh K, Lerner L, Nakata T, Lopez N, Lou L, et al. Association of Growth Differentiation Factor 15 with Mortality in a Prospective Hemodialysis Cohort. Cardiorenal Med (2017) 7:158–68. doi: 10.1159/000455907
34. Husebø GR, Grønseth R, Lerner L, Gyuris J, Hardie JA, Bakke PS, et al. Growth differentiation factor-15 is a predictor of important disease outcomes in patients with COPD. Eur Respir J (2017) 49:1601298. doi: 10.1183/13993003.01298-2016
35. Hansen ES, Hindberg K, Latysheva N, Aukrust P, Ueland T, Hansen JB, et al. Plasma levels of growth differentiation factor 15 are associated with future risk of venous thromboembolism. Blood (2020) 136:1863–70. doi: 10.1182/blood.2019004572
36. Schlittenhardt D, Schober A, Strelau J, Bonaterra GA, Schmiedt W, Unsicker K, et al. Involvement of growth differentiation factor-15/macrophage inhibitory cytokine-1 (GDF-15/MIC-1) in oxLDL-induced apoptosis of human macrophages in vitro and in arteriosclerotic lesions. Cell Tissue Res (2004) 318:325–33. doi: 10.1007/s00441-004-0986-3
37. Kempf T, Zarbock A, Widera C, Butz S, Stadtmann A, Rossaint J, et al. GDF-15 is an inhibitor of leukocyte integrin activation required for survival after myocardial infarction in mice. Nat Med (2011) 17:581–8. doi: 10.1038/nm.2354
38. Kempf T, Eden M, Strelau J, Naguib M, Willenbockel C, Tongers J, et al. The transforming growth factor-beta superfamily member growth-differentiation factor-15 protects the heart from ischemia/reperfusion injury. Circ Res (2006) 98:351–60. doi: 10.1161/01.RES.0000202805.73038.48
39. Emmerson PJ, Wang F, Du Y, Liu Q, Pickard RT, Gonciarz MD, et al. The metabolic effects of GDF15 are mediated by the orphan receptor GFRAL. Nat Med (2017) 23:1215–9. doi: 10.1038/nm.4393
40. Hsu JY, Crawley S, Chen M, Ayupova DA, Lindhout DA, Higbee J, et al. Non-homeostatic body weight regulation through a brainstem-restricted receptor for GDF15. Nature (2017) 550:255–9. doi: 10.1038/nature24042
41. Mullican SE, Lin-Schmidt X, Chin CN, Chavez JA, Furman JL, Armstrong AA, et al. GFRAL is the receptor for GDF15 and the ligand promotes weight loss in mice and nonhuman primates. Nat Med (2017) 23:1150–7. doi: 10.1038/nm.4392
42. Lerner L, Gyuris J, Nicoletti R, Gifford J, Krieger B, Jatoi A. Growth differentiating factor-15 (GDF-15): A potential biomarker and therapeutic target for cancer-associated weight loss. Oncol Lett (2016) 12:4219–23. doi: 10.3892/ol.2016.5183
43. Lerner L, Hayes TG, Tao N, Krieger B, Feng B, Wu Z, et al. Plasma growth differentiation factor 15 is associated with weight loss and mortality in cancer patients. J cachexia sarcopenia Muscle (2015) 6:317–24. doi: 10.1002/jcsm.12033
44. Golay A, Ybarra J. Link between obesity and type 2 diabetes. Best practice & research. Clin Endocrinol Metab (2005) 19:649–63. doi: 10.1016/j.beem.2005.07.010
45. The Diabetes Prevention Program Research Group. The Diabetes Prevention Program: baseline characteristics of the randomized cohort. The Diabetes Prevention Program Research Group. Diabetes Care (2000) 23:1619–29. doi: 10.2337/diacare.23.11.1619
46. Daousi C, Casson IF, Gill GV, MacFarlane IA, Wilding JP, Pinkney JH. Prevalence of obesity in type 2 diabetes in secondary care: association with cardiovascular risk factors. Postgraduate Med J (2006) 82:280–4. doi: 10.1136/pmj.2005.039032
47. Zumla A, Niederman MS. The explosive epidemic outbreak of novel coronavirus disease 2019 (COVID-19) and the persistent threat of respiratory tract infectious diseases to global health security. Curr Opin Pulm Med (2020) 26:193–6. doi: 10.1097/MCP.0000000000000676
48. Fonseca V. Effect of thiazolidinediones on body weight in patients with diabetes mellitus. Am J Med (2003) 115(Suppl 8A):42s–8s. doi: 10.1016/j.amjmed.2003.09.005
49. Kahn SE, Haffner SM, Heise MA, Herman WH, Holman RR, Jones NP, et al. Glycemic durability of rosiglitazone, metformin, or glyburide monotherapy. N Engl J Med (2006) 355:2427–43. doi: 10.1056/NEJMoa066224
50. Saenz A, Fernandez-Esteban I, Mataix A, Ausejo M, Roque M, Moher D. Metformin monotherapy for type 2 diabetes mellitus. Cochrane Database Syst Rev (2005), Cd002966. doi: 10.1002/14651858.CD002966.pub3
51. Hermann LS, Karlsson JE, Sjöstrand A. Prospective comparative study in NIDDM patients of metformin and glibenclamide with special reference to lipid profiles. Eur J Clin Pharmacol (1991) 41:263–5. doi: 10.1007/BF00315441
52. DeFronzo RA, Goodman AM. Efficacy of metformin in patients with non-insulin-dependent diabetes mellitus. The Multicenter Metformin Study Group. N Engl J Med (1995) 333:541–9. doi: 10.1056/NEJM199508313330902
53. Garvey WT, Mechanick JI, Brett EM, Garber AJ, Hurley DL, Jastreboff AM, et al. American Association Of Clinical Endocrinologists And American College Of Endocrinology Comprehensive Clinical Practice Guidelines For Medical Care Of Patients With Obesity. Endocr Pract Off J Am Coll Endocrinol Am Assoc Clin Endocrinologists (2016) 22(Suppl 3):1–203. doi: 10.4158/EP161365.GL
54. Seifarth C, Schehler B, Schneider HJ. Effectiveness of metformin on weight loss in non-diabetic individuals with obesity. Exp Clin Endocrinol Diabetes (2013) 121:27–31. doi: 10.1055/s-0032-1327734
55. Björkhem-Bergman L, Asplund AB, Lindh JD. Metformin for weight reduction in non-diabetic patients on antipsychotic drugs: a systematic review and meta-analysis. J Psychopharmacol (Oxford England) (2011) 25:299–305. doi: 10.1177/0269881109353461
56. Hussain M, Atif MA, Ghafoor MB. Beneficial effects of sitagliptin and metformin in non-diabetic hypertensive and dyslipidemic patients. Pakistan J Pharm Sci (2016) 29:2385–9.
57. Rodríguez Y, Giri M, Feyen E, Christophe AB. Effect of metformin vs. placebo treatment on serum fatty acids in non-diabetic obese insulin resistant individuals. Prostagland Leukotrienes Essential Fatty Acids (2004) 71:391–7. doi: 10.1016/j.plefa.2004.08.004
58. Srinivasan S, Ambler GR, Baur LA, Garnett SP, Tepsa M, Yap F, et al. Randomized, controlled trial of metformin for obesity and insulin resistance in children and adolescents: improvement in body composition and fasting insulin. J Clin Endocrinol Metab (2006) 91:2074–80. doi: 10.1210/jc.2006-0241
59. Pastor-Villaescusa B, Cañete MD, Caballero-Villarraso J, Hoyos R, Latorre M, Vázquez-Cobela R, et al. Metformin for Obesity in Prepubertal and Pubertal Children: A Randomized Controlled Trial. Pediatrics (2017) 140:e20164285. doi: 10.1542/peds.2016-4285
60. Adeyemo MA, McDuffie JR, Kozlosky M, Krakoff J, Calis KA, Brady SM, et al. Effects of metformin on energy intake and satiety in obese children. Diabetes Obes Metab (2015) 17:363–70. doi: 10.1111/dom.12426
61. Freemark M, Bursey D. The effects of metformin on body mass index and glucose tolerance in obese adolescents with fasting hyperinsulinemia and a family history of type 2 diabetes. Pediatrics (2001) 107:E55. doi: 10.1542/peds.107.4.e55
62. Barton BB, Segger F, Fischer K, Obermeier M, Musil R. Update on weight-gain caused by antipsychotics: a systematic review and meta-analysis. Expert Opin Drug Saf (2020) 19:295–314. doi: 10.1080/14740338.2020.1713091
63. Bazo-Alvarez JC, Morris TP, Carpenter JR, Hayes JF, Petersen I. Effects of long-term antipsychotics treatment on body weight: A population-based cohort study. J Psychopharmacol (Oxford England) (2020) 34:79–85. doi: 10.1177/0269881119885918
64. de Silva VA, Suraweera C, Ratnatunga SS, Dayabandara M, Wanniarachchi N, Hanwella R. Metformin in prevention and treatment of antipsychotic induced weight gain: a systematic review and meta-analysis. BMC Psychiatry (2016) 16:341. doi: 10.1186/s12888-016-1049-5
65. Siskind DJ, Leung J, Russell AW, Wysoczanski D, Kisely S. Metformin for Clozapine Associated Obesity: A Systematic Review and Meta-Analysis. PloS One (2016) 11:e0156208. doi: 10.1371/journal.pone.0156208
66. Ellul P, Delorme R, Cortese S. Metformin for Weight Gain Associated with Second-Generation Antipsychotics in Children and Adolescents: A Systematic Review and Meta-Analysis. CNS Drugs (2018) 32:1103–12. doi: 10.1007/s40263-018-0571-z
67. Escobar-Morreale HF. Polycystic ovary syndrome: definition, aetiology, diagnosis and treatment. Nat Rev Endocrinol (2018) 14:270–84. doi: 10.1038/nrendo.2018.24
68. Naderpoor N, Shorakae S, de Courten B, Misso ML, Moran LJ, Teede HJ. Metformin and lifestyle modification in polycystic ovary syndrome: systematic review and meta-analysis. Hum Reprod Update (2015) 21:560–74. doi: 10.1093/humupd/dmv025
69. Javed Z, Papageorgiou M, Madden LA, Rigby AS, Kilpatrick ES, Atkin SL, et al. The effects of empagliflozin versus metformin on endothelial microparticles in overweight/obese women with polycystic ovary syndrome. Endocr Connect (2020) 9:563–9. doi: 10.1530/EC-20-0173
70. Preiss D, Sattar N, Harborne L, Norman J, Fleming R. The effects of 8 months of metformin on circulating GGT and ALT levels in obese women with polycystic ovarian syndrome. Int J Clin Pract (2008) 62:1337–43. doi: 10.1111/j.1742-1241.2008.01825.x
71. Jensterle M, Goricar K, Janez A. Metformin as an initial adjunct to low-dose liraglutide enhances the weight-decreasing potential of liraglutide in obese polycystic ovary syndrome: Randomized control study. Exp Ther Med (2016) 11:1194–200. doi: 10.3892/etm.2016.3081
72. Jensterle Sever M, Kocjan T, Pfeifer M, Kravos NA, Janez A. Short-term combined treatment with liraglutide and metformin leads to significant weight loss in obese women with polycystic ovary syndrome and previous poor response to metformin. Eur J Endocrinol (2014) 170:451–9. doi: 10.1530/EJE-13-0797
73. Al-Nozha O, Habib F, Mojaddidi M, El-Bab MF. Body weight reduction and metformin: Roles in polycystic ovary syndrome. Pathophysiology (2013) 20:131–7. doi: 10.1016/j.pathophys.2013.03.002
74. Aghahosseini M, Aleyaseen A, Safdarian L, Moddaress-Hashemi S, Mofid B, Kashani L. Metformin 2,500 mg/day in the treatment of obese women with polycystic ovary syndrome and its effect on weight, hormones, and lipid profile. Arch Gynecol Obstet (2010) 282:691–4. doi: 10.1007/s00404-010-1579-x
75. Patel R, Shah G. Effect of metformin on clinical, metabolic and endocrine outcomes in women with polycystic ovary syndrome: a meta-analysis of randomized controlled trials. Curr Med Res Opin (2017) 33:1545–57. doi: 10.1080/03007995.2017.1279597
76. Cheng J, Gao J, Shuai X, Wang G, Tao K. The comprehensive summary of surgical versus non-surgical treatment for obesity: a systematic review and meta-analysis of randomized controlled trials. Oncotarget (2016) 7:39216–30. doi: 10.18632/oncotarget.9581
77. Malin SK, Kashyap SR. Effects of metformin on weight loss: potential mechanisms. Curr Opin Endocrinol Diabetes Obes (2014) 21:323–9. doi: 10.1097/MED.0000000000000095
78. Yerevanian A, Soukas AA. Metformin: Mechanisms in Human Obesity and Weight Loss. Curr Obes Rep (2019) 8:156–64. doi: 10.1007/s13679-019-00335-3
79. Williams CC, Singleton BA, Llopis SD, Skripnikova EV. Metformin induces a senescence-associated gene signature in breast cancer cells. J Health Care Poor Underserved (2013) 24:93–103. doi: 10.1353/hpu.2013.0044
80. Zafarvahedian E, Roohi A, Sepand MR, Ostad SN, Ghahremani MH. Effect of metformin and celecoxib on cytotoxicity and release of GDF-15 from human mesenchymal stem cells in high glucose condition. Cell Biochem Funct (2017) 35:407–13. doi: 10.1002/cbf.3289
81. Gerstein HC, Pare G, Hess S, Ford RJ, Sjaarda J, Raman K, et al. Growth Differentiation Factor 15 as a Novel Biomarker for Metformin. Diabetes Care (2017) 40:280–3. doi: 10.2337/dc16-1682
82. Natali A, Nesti L, Venturi E, Shore AC, Khan F, Gooding K, et al. Metformin is the key factor in elevated plasma growth differentiation factor-15 levels in type 2 diabetes: A nested, case-control study. Diabetes Obes Metab (2019) 21:412–6. doi: 10.1111/dom.13519
83. Chrysovergis K, Wang X, Kosak J, Lee SH, Kim JS, Foley JF, et al. NAG-1/GDF-15 prevents obesity by increasing thermogenesis, lipolysis and oxidative metabolism. Int J Obes (Lond) (2014) 38:1555–64. doi: 10.1038/ijo.2014.27
84. Ho FM, Lin WW, Chen BC, Chao CM, Yang CR, Lin LY, et al. High glucose-induced apoptosis in human vascular endothelial cells is mediated through NF-kappaB and c-Jun NH2-terminal kinase pathway and prevented by PI3K/Akt/eNOS pathway. Cell Signal (2006) 18:391–9. doi: 10.1016/j.cellsig.2005.05.009
85. Afanas’ev I. Signaling of reactive oxygen and nitrogen species in Diabetes mellitus. Oxid Med Cell Longev (2010) 3:361–73. doi: 10.4161/oxim.3.6.14415
86. Li J, Yang L, Qin W, Zhang G, Yuan J, Wang F. Adaptive induction of growth differentiation factor 15 attenuates endothelial cell apoptosis in response to high glucose stimulus. PloS One (2013) 8:e65549. doi: 10.1371/journal.pone.0065549
87. Kelly JA, Lucia MS, Lambert JR. p53 controls prostate-derived factor/macrophage inhibitory cytokine/NSAID-activated gene expression in response to cell density, DNA damage and hypoxia through diverse mechanisms. Cancer Lett (2009) 277:38–47. doi: 10.1016/j.canlet.2008.11.013
88. Bao X, Borne Y, Muhammad IF, Nilsson J, Lind L, Melander O, et al. Growth differentiation factor 15 is positively associated with incidence of diabetes mellitus: the Malmo Diet and Cancer-Cardiovascular Cohort. Diabetologia (2019) 62:78–86. doi: 10.1007/s00125-018-4751-7
89. He X, Su J, Ma X, Lu W, Zhu W, Wang Y, et al. The association between serum growth differentiation factor 15 levels and lower extremity atherosclerotic disease is independent of body mass index in type 2 diabetes. Cardiovasc Diabetol (2020) 19:40. doi: 10.1186/s12933-020-01020-9
90. Wollert KC, Kempf T, Wallentin L. Growth Differentiation Factor 15 as a Biomarker in Cardiovascular Disease. Clin Chem (2017) 63:140–51. doi: 10.1373/clinchem.2016.255174
91. Nakayasu ES, Syed F, Tersey SA, Gritsenko MA, Mitchell HD, Chan CY, et al. Comprehensive Proteomics Analysis of Stressed Human Islets Identifies GDF15 as a Target for Type 1 Diabetes Intervention. Cell Metab (2020) 31:363–374 e6. doi: 10.1016/j.cmet.2019.12.005
92. Ouyang J, Isnard S, Lin J, Fombuena B, Marette A, Routy B, et al. Metformin effect on gut microbiota: insights for HIV-related inflammation. AIDS Res Ther (2020) 17:10. doi: 10.1186/s12981-020-00267-2
93. Han Y, Xie H, Liu Y, Gao P, Yang X, Shen Z. Effect of metformin on all-cause and cardiovascular mortality in patients with coronary artery diseases: a systematic review and an updated meta-analysis. Cardiovasc Diabetol (2019) 18:96. doi: 10.1186/s12933-019-0900-7
94. Kulkarni AS, Gubbi S, Barzilai N. Benefits of Metformin in Attenuating the Hallmarks of Aging. Cell Metab (2020) 32:15–30. doi: 10.1016/j.cmet.2020.04.001
95. Griffin SJ, Leaver JK, Irving GJ. Impact of metformin on cardiovascular disease: a meta-analysis of randomised trials among people with type 2 diabetes. Diabetologia (2017) 60:1620–9. doi: 10.1007/s00125-017-4337-9
96. Mullican SE, Rangwala SM. Uniting GDF15 and GFRAL: Therapeutic Opportunities in Obesity and Beyond. Trends Endocrinol Metab: TEM (2018) 29:560–70. doi: 10.1016/j.tem.2018.05.002
97. Baek SJ, Horowitz JM, Eling TE. Molecular cloning and characterization of human nonsteroidal anti-inflammatory drug-activated gene promoter. Basal transcription is mediated by Sp1 and Sp3. J Biol Chem (2001) 276:33384–92. doi: 10.1074/jbc.M101814200
98. Osada M, Park HL, Park MJ, Liu JW, Wu G, Trink B, et al. A p53-type response element in the GDF15 promoter confers high specificity for p53 activation. Biochem Biophys Res Commun (2007) 354:913–8. doi: 10.1016/j.bbrc.2007.01.089
99. Lim JH, Park JW, Min DS, Chang JS, Lee YH, Park YB, et al. NAG-1 up-regulation mediated by EGR-1 and p53 is critical for quercetin-induced apoptosis in HCT116 colon carcinoma cells. Apoptosis an Int J Programmed Cell Death (2007) 12:411–21. doi: 10.1007/s10495-006-0576-9
100. Chung HK, Ryu D, Kim KS, Chang JY, Kim YK, Yi HS, et al. Growth differentiation factor 15 is a myomitokine governing systemic energy homeostasis. J Cell Biol (2017) 216:149–65. doi: 10.1083/jcb.201607110
101. Jones JE, Cadena SM, Gong C, Wang X, Chen Z, Wang SX, et al. Supraphysiologic Administration of GDF11 Induces Cachexia in Part by Upregulating GDF15. Cell Rep (2018) 22:1522–30. doi: 10.1016/j.celrep.2018.01.044
102. Patel S, Alvarez-Guaita A, Melvin A, Rimmington D, Dattilo A, Miedzybrodzka EL, et al. GDF15 Provides an Endocrine Signal of Nutritional Stress in Mice and Humans. Cell Metab (2019) 29:707–718.e8. doi: 10.1016/j.cmet.2018.12.016
103. Ichikawa T, Suenaga Y, Koda T, Ozaki T, Nakagawara A. TAp63-dependent induction of growth differentiation factor 15 (GDF15) plays a critical role in the regulation of keratinocyte differentiation. Oncogene (2008) 27:409–20. doi: 10.1038/sj.onc.1210658
104. Suzuki T, Gao J, Ishigaki Y, Kondo K, Sawada S, Izumi T, et al. Mediates Insulin Resistance by Modulating Adipose Tissue Macrophage Polarity. Cell Rep (2017) 18:2045–57. doi: 10.1016/j.celrep.2017.01.076
105. Kempf T, Guba-Quint A, Torgerson J, Magnone MC, Haefliger C, Bobadilla M, et al. Growth differentiation factor 15 predicts future insulin resistance and impaired glucose control in obese nondiabetic individuals: results from the XENDOS trial. Eur J Endocrinol (2012) 167:671–8. doi: 10.1530/EJE-12-0466
106. Semba RD, Gonzalez-Freire M, Tanaka T, Biancotto A, Zhang P, Shardell M, et al. Elevated Plasma Growth and Differentiation Factor 15 Is Associated With Slower Gait Speed and Lower Physical Performance in Healthy Community-Dwelling Adults. J Gerontol Ser A Biol Sci Med Sci (2020) 75:175–80. doi: 10.1093/gerona/glz071
107. Tsai VW, Macia L, Johnen H, Kuffner T, Manadhar R, Jørgensen SB, et al. TGF-b superfamily cytokine MIC-1/GDF15 is a physiological appetite and body weight regulator. PloS One (2013) 8:e55174. doi: 10.1371/journal.pone.0055174
108. Johnen H, Lin S, Kuffner T, Brown DA, Tsai VW, Bauskin AR, et al. Tumor-induced anorexia and weight loss are mediated by the TGF-beta superfamily cytokine MIC-1. Nat Med (2007) 13:1333–40. doi: 10.1038/nm1677
109. Baek SJ, Okazaki R, Lee SH, Martinez J, Kim JS, Yamaguchi K, et al. Nonsteroidal anti-inflammatory drug-activated gene-1 over expression in transgenic mice suppresses intestinal neoplasia. Gastroenterology (2006) 131:1553–60. doi: 10.1053/j.gastro.2006.09.015
110. Macia L, Tsai VW, Nguyen AD, Johnen H, Kuffner T, Shi YC, et al. Macrophage inhibitory cytokine 1 (MIC-1/GDF15) decreases food intake, body weight and improves glucose tolerance in mice on normal & obesogenic diets. PloS One (2012) 7:e34868. doi: 10.1371/journal.pone.0034868
111. Hätinen T, Holm L, Airaksinen MS. Loss of neurturin in frog–comparative genomics study of GDNF family ligand-receptor pairs. Mol Cell Neurosci (2007) 34:155–67. doi: 10.1016/j.mcn.2006.10.009
112. Airaksinen MS, Holm L, Hätinen T. Evolution of the GDNF family ligands and receptors. Brain Behav Evol (2006) 68:181–90. doi: 10.1159/000094087
Keywords: metformin, body weight, obesity, GDF-15, GFRAL, diabetes
Citation: Ouyang J, Isnard S, Lin J, Fombuena B, Peng X, Chen Y and Routy J-P (2020) GDF-15 as a Weight Watcher for Diabetic and Non-Diabetic People Treated With Metformin. Front. Endocrinol. 11:581839. doi: 10.3389/fendo.2020.581839
Received: 22 July 2020; Accepted: 26 October 2020;
Published: 18 November 2020.
Edited by:
Stefan Zoltán Lutz, Bad Sebastiansweiler, GermanyReviewed by:
Britta Wilms, University of Lübeck, GermanyManjunath Ramanjaneya, Hamad Medical Corporation, Qatar
Copyright © 2020 Ouyang, Isnard, Lin, Fombuena, Peng, Chen and Routy. This is an open-access article distributed under the terms of the Creative Commons Attribution License (CC BY). The use, distribution or reproduction in other forums is permitted, provided the original author(s) and the copyright owner(s) are credited and that the original publication in this journal is cited, in accordance with accepted academic practice. No use, distribution or reproduction is permitted which does not comply with these terms.
*Correspondence: Jean-Pierre Routy, jean-pierre.routy@mcgill.ca; Yaokai Chen, yaokaichen@hotmail.com
†These authors have contributed equally to this work