- 1Psychiatry, Loma Linda University, Loma Linda, CA, United States
- 2Department of Psychiatry, Patton State Hospital, San Bernardino, CA, United States
- 3Department of Psychiatry, Loma Linda University, Loma Linda, CA, United States
- 4Department of Psychiatry, University of California, Riverside, Riverside, CA, United States
Chronic psychiatric patients with schizophrenia and related disorders are frequently treatment-resistant and may require higher doses of psychotropic drugs to remain stable. Prolonged exposure to these agents increases the risk of weight gain and cardiometabolic disorders, leading to poorer outcomes and higher medical cost. It is well-established that obesity has reached epidemic proportions throughout the world, however it is less known that its rates are two to three times higher in mentally ill patients compared to the general population. Psychotropic drugs have emerged as a major cause of weight gain, pointing to an urgent need for novel interventions to attenuate this unintended consequence. Recently, the gut microbial community has been linked to psychotropic drugs-induced obesity as these agents were found to possess antimicrobial properties and trigger intestinal dysbiosis, depleting Bacteroidetes phylum. Since germ-free animals exposed to psychotropics have not demonstrated weight gain, altered commensal flora composition is believed to be necessary and sufficient to induce dysmetabolism. Conversely, not only do psychotropics disrupt the composition of gut microbiota but the later alter the metabolism of the former. Here we review the role of gut bacterial community in psychotropic drugs metabolism and dysbiosis. We discuss potential biomarkers reflecting the status of Bacteroidetes phylum and take a closer look at nutritional interventions, fecal microbiota transplantation, and transcranial magnetic stimulation, strategies that may lower obesity rates in chronic psychiatric patients.
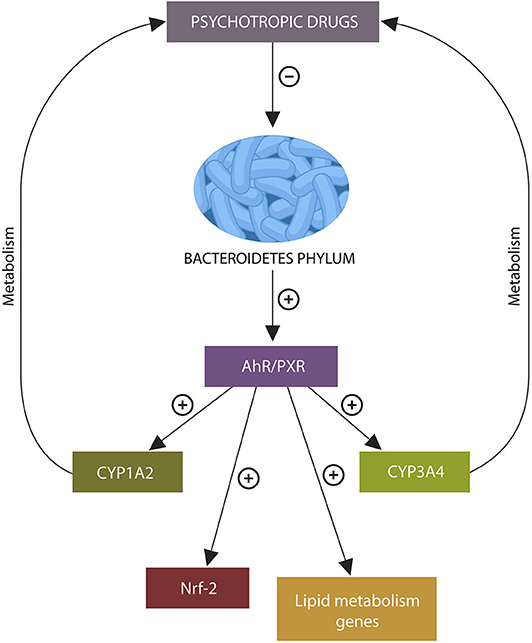
Graphical Abstract. Psychotropic drugs deplete intestinal Bacteroidetes phylum. The absence of metabolites generated by these microbes may under-activate xenobiotic sensors aryl hydrocarbon receptor (AhR) and pregnane X receptor (PXR), disrupting the transcription of genes related to psychotropic drug metabolism, lipid, and redox homeostasis, leading to altered bioavailability of antipsychotic agents, weight gain, and lowered body iron stores.
Introduction
Chronic psychiatric patients diagnosed with schizophrenia and schizophrenia-spectrum disorders (SSDs), are often treatment-resistant, requiring stabilization with above average doses of psychotropic drugs, especially olanzapine (OLZ), clozapine (CZP), risperidone (RSP), and quetiapine (QTP). Long-term exposure to these atypical antipsychotics (AAPs) increases the risk of metabolic adverse effects and weight gain (1, 2).
Novel studies have identified a bidirectional relationship between the gut bacterial community and psychotropic drugs in which these agents alter the composition of commensal flora while the later influences the pharmacokinetics of the former (3–5). For example, psychotropic drugs-induced depletion of Bacteroidetes phylum may under-activate xenobiotic sensors aryl hydrocarbon receptor (AhR) and pregnane X receptor (PXR), altering the expression of P450 isozymes CYP1A2 and CYP3A4 that are involved in the metabolism of these agents (6). Aside from the CYP system, gut microbes express numerous phase I and II enzymes that were shown to transform various drugs prior to absorption (7). On the other hand, agents conjugated in the liver and secreted in the bile can be deconjugated by gut microbes, increasing their toxicity (8). These novel findings led to the development of pharmacomicrobiomics, a new field investigating the complex interactions between the drugs and gut microbes (9).
Historically, psychotropic drugs have been known to possess antimicrobial properties but their role in causing dysbiosis was only appreciated after the microbiome discovery. For example, most studies in humans show that AAP-treated patients develop an obesogenic microbial pattern, marked by increased Firmicutes and Actinobacteria phyla and decreased Bacteroidetes (10, 11). Interestingly, AAP-upregulated phyla have the capability to synthesize heme, likely enabling them not only to survive but also thrive in iron-scarce conditions due to nutrients left behind by the non-surviving competitors.
Preclinical research offers more insight into the psychotropics-obesity link via germ-free animals that proved crucial for the study of AAP-related dysbiosis. Given that these studies are based on rodents, it is unclear at this time if the findings can be translated to humans, however body weight alteration have been minimal in germ-free rodents exposed to AAPs (12). Others have reported that prebiotic treatment can attenuate OLZ-induced weight gain in mice, further underlining the role of commensal flora in lipid homeostasis (13, 14). Another study has reported that antibiotic pretreatment prevented weight gain in OLZ-treated female rats, emphasizing once more the role of enteric microbiota in obesity (15). Several recent studies have found that, aside from antipsychotics and antidepressants, several other drugs possess antimicrobial functions and induce dysbiosis (16–19).
Taken together, there is a rapidly growing body of evidence showing that some psychiatric treatments can alter the composition of gut microbiota, triggering dysbiosis and dysmetabolism. On the other hand, intestinal microbes can alter the absorption and pharmacokinetics of psychotropic agents, impairing their bioavailability. Conversely, restoring microbial number and diversity by diet, pre or probiotics may attenuate or prevent psychotropic drugs-associated weight gain.
In this paper, we discuss the role of microbiota in the metabolism of psychotropic drugs as well as the dysbiosis associated with the antimicrobial properties of these agents. We suggest potential biomarkers, of Bacteroidetes phylum and take a closer look at various interventions, including dietary fiber, fecal microbiota transplant (FMT) and transcranial magnetic stimulation (tMS) in preventing weight gain associated with psychotropic agents.
Psychotropic Drugs and Obesity
There is little doubt that obesity has increased worldwide and reached epidemic proportions, engendering one of the most complex public health problems faced by the society today (20, 21). However, it has been less emphasized that another obesity epidemic of even greater proportions has been taking place silently in mentally ill patients, placing them at higher morbidity and mortality risk compared to the general population (22). Indeed, obesity rates and cardiovascular disease are 2–3 times higher in psychiatric patients, especially in women, children, and adolescents (23–26). Historically, reports of weight fluctuations and abnormal eating behavior in mentally ill individuals have been observed prior to the psychotropic drugs era, suggesting that either psychiatric disorders cause dysmetabolism or impaired metabolism leads to abnormal brain functioning (27).
There are several excellent reviews on weight gain and obesity in psychiatric patients, discussing receptor interactions, orexigenic peptides, insulin resistance, and reward mechanisms (28, 29). These topics are beyond the scope of this article that focuses primarily on the impact AAP drugs on gut microbial community.
Over the past few years, several studies have emphasized the role of gut microbial community in obesity and dysmetabolism as it was noted that germ-free animals exposed to psychotropics do not display weight fluctuations (12). This led to a heightened interest in microbial metabolites and their role in engendering an immunologically tolerant enteric environment optimal for nutrient harvesting. On the other hand, dysbiosis or selective elimination of microbiota by xenobiotics was linked to local pathology, and systemic disorders, including obesity and psychiatric conditions (30, 31). Interestingly, both psychotropic drugs-induced dysmetabolism and high fat diet-related weight gain present with a common enteric microbial pattern, depletion of Bacteroidetes phylum, suggesting an overlapping pathology. Others have opined that the loss of Bacteroidetes-generated metabolites is the common denominator of weight gain induced either by an unhealthy diet or psychotropics drugs (6, 32). Therefore, restoring the levels of these molecules may lead to novel weight loss strategies in chronic psychiatric patients.
The Holobiont, a Story of Two Kingdoms
The concept of the holobiont refers to a holistic model of two biological kingdoms living together: the eukaryote host and prokaryote microbes. This coexistence is made possible by the inter-kingdom cross talk via dietary molecules and microbial metabolites, enabling immunological tolerance of the microbial organ and food, while maintaining vigilance for pathogens, toxins, and pollutants (33–35). To accomplish all these tasks, nutrient harvesting is highly intertwined with immunity and xenobiotic metabolism through a system of promiscuous sensors expressed by intestinal epithelial cells (IECs) and enteric macrophages, such as AhR and PXR (36). These xenobiotic receptors activate innate immunity proportionally with the affinity of their binding ligands, rather than as binary “on-off” switches. For example, a strong agonist may trigger immune activation, while partial or weak agonists may induce immunosuppression (37). Indeed, high affinity AhR ligands have been canonically linked to the immune rejection of toxins and pollutants (38). However, the recent discovery of endogenous AhR and PXR ligands with weak or partial agonism synthesized by the gut microbes from dietary tryptophan (Trp) and undigestible fiber has shed some light on the role of xenobiotic sensors in both immune tolerance and energy metabolism (39). Indeed, loss of endogenous AhR ligands was associated with weight gain and decreased IL-10 that impaired commensals immune tolerance (40–43). By the same token, loss of microorganismal variety with depletion of microbial phyla was linked to both immune rejection of commensals and weight gain (44).
Of Kingdom Food Tasters and Cupbearers
Gut microbiota ensure their own acceptance into the GI habitat by generating weak AhR and PXR ligands, including indole-3-acetate and indole-3-propionate (IPA) that promote immunological tolerance by upregulating IL-10 (45–47). At the same time, microbiota assist the host by filtering xenobiotics, toxins, pollutants, and heavy metals as well as by denying nutrients to pathogenic bacteria (48). Therefore, gut microbes mediate not only their own immunological tolerance but also that of dietary molecules by converting them into AhR and PXR ligands (49).
The exact molecular underpinnings of immunological tolerance to microbes and dietary molecules are unclear at this time, however a recent study identified toll like receptor 9 (TLR9)-AhR signaling as the immunosuppressive switch that turns off innate immunity in the presence of apoptotic cells, suggesting that a similar mechanism may engender the tolerance of enteric microbes and food (50). Indeed, TLR9 is an established tolerogen that upregulates IL-10 during pregnancy, promoting fetal acceptance (51).
Taken together, these studies suggest an immunosuppressive TLR9-AhR-IL-10 axis of tolerance likely involved in the acceptance of gut microbes and food. Furthermore, TLR-9 was demonstrated to sense eukaryotic and prokaryotic DNA and contribute to lipid homeostasis, connecting genomic damage to obesity (52, 53). Indeed, it has been established that iron and related reactive oxygen species (ROS) induce DNA disruption with resultant dysmetabolism (54).
The Kingdom Messengers
Microbiota-derived molecules, including propionate, indole, neurotransmitters, and iron-related molecules, including bacterial siderophores and ROS were demonstrated to signal with the receptors expressed on the intestinal epithelial cells (IECs) and enteric macrophages, modulating both feeding and immunity. The following is a snapshot of inter-kingdom signals relevant for psychotropic drugs-induced weight gain.
Propionate
SCFAs, acetate, propionate, and butyrate, are generated by gut microbes via fermentation of dietary fiber. These molecules activate host intestinal G protein-coupled receptors (GPCRs), including GPR41 and GPR43, modulating numerous physiological functions, including feeding and immunity (55, 56). Bacteroides phylum is the major generator of enteric propionate, a SCFA that interacts with the Trp metabolite indole, engendering indole-3-propionic acid (IPA) a biomolecule involved in lipid homeostasis and acceptance of commensal microbes (57). In addition, propionate is a potent agonist at GPR41 and GPR43 expressed by enteric L-cells, enabling the release of anorexigenic hormones, glucagon-like peptide-1 (GLP-1), and peptide YY (PYY) (Figure 1). These bioactive molecules were demonstrated to lower appetite and feeding by activating the nutrient sensing neurons in brainstem nucleus tractus solitarius (NTS) (58) (Figure 1). Indeed, clinical trials with human subjects have demonstrated that propionate lowers food intake and increases energy expenditures via NTS (59, 60). As Bacteroidetes phylum generates most of the GI tract propionate, psychotropic drugs-associated depletion of these microbes may lower propionate, triggering dysmetabolism and weight gain. For example, a recent study in non-psychiatric, obese individuals associated propionate with weight loss (59). This study suggests that Bacteroidetes depletion, including that induced by psychotropic drugs, may trigger dysmetabolism and weight gain (59). It is, therefore realistic to expect that propionate fecal levels may accurately reflect the status Bacteroidetes phylum (Figure 3).
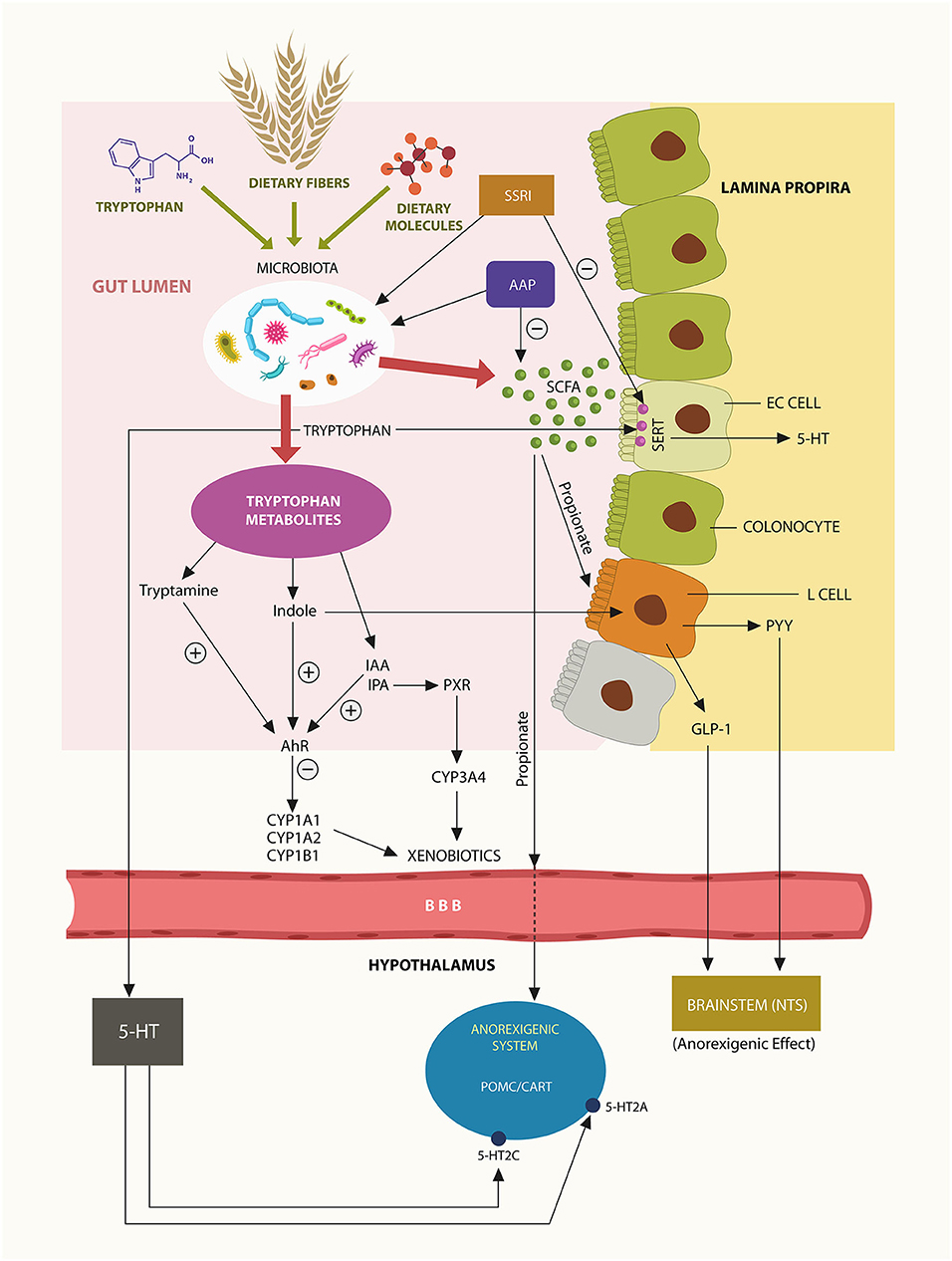
Figure 1. Trp, dietary fiber and food are converted by gut microbes into ligands at AhR and PXR. Bacteroidetes phylum generates most enteric propionate, inducing L-cells activation and release of anorexigenic peptides GLP-1 and PYY that lower appetite via NTS. Propionate may also cross the BBB and act on hypothalamic POMC/CART neurons, inhibiting feeding behavior. Trp metabolite, indole is an AhR agonist, while IPA is a PXR agonist. Activation of these xenobiotic sensors induces the transcription of psychotropic drugs metabolizing enzymes, including CYP1A2 and CYP3A4. A small fraction of gut Trp crosses into the brain, generating the central 5-HT.
Aside from its local physiological functions, propionate may cross the blood-brain barrier (BBB) to act on pro-opiomelanocortin (POMC) and cocaine-and amphetamine-regulated transcript (CART) hypothalamic neurons, suppressing food intake (61). Moreover, the serotonin (5-HT) precursor Trp was demonstrated to cross the BBB and, upon conversion to 5-HT, activate POMC/CART, 5-HT2A, and 5-HT2C receptors, modulating appetite (62) (Figure 1).
Tryptophan Metabolites
Trp is an essential amino acid that cannot be synthesized in the human body therefore it must be obtained entirely from the diet. In the gut, Trp is converted by microbes, including Bacteroidetes into AhR and PXR ligands that promote weight loss and immune tolerance to commensals (57, 63).
Under normal circumstances, Trp is converted in the GI tract to indole by tryptophanase-producing bacteria, including Bacteroidetes thetaiotaomicron (B. theta) a member of Bacteroidetes phylum. Since indole is a metabolite associated with weight loss, depletion of Bacteroidetes may lead to obesity (64, 65). Moreover, others have found that, like propionate, indole can also activate enteric L-cells, releasing the anorexigenic peptide hormones GLP-1 and PYY, further lowering body weight (66) (Figure 1).
The GI tract 5-HT does not cross the BBB but plays a major local role as it promotes intestinal motility (67). Interestingly, IECs express serotonin transporters (SERT), proteins inhibited by serotonin reuptake inhibitors (SSRI), explaining the beneficial role of these agents in Crohn's disease and ulcerative colitis (68–70). As shown above, Trp and propionate participate in the production of IPA, a PXR ligand associated with immune tolerance and weight loss (71–73).
Neurotransmitters
Aside from 5-HT, intestinal commensal flora is known for synthesizing many neurotransmitters, including dopamine (DA), norepinephrine (NE), and acetylcholine (ACh) that can act locally, but are also believed to reach the CNS and influence brain physiology (74–76). These biomolecules play a key role in energy metabolism and immune tolerance of gut microbes by upregulating IL-10 (77, 78). Interestingly, microbial DA and NE were demonstrated to influence iron absorption and metabolism by functioning as siderophores or bacterial iron scavengers (79, 80). Interestingly, a recent study found that enterobactin, an Escherichia coli siderophore, can be transferred to the host, revealing a novel form of inter-kingdom communication (81). On the other hand, Bacteroides phylum does not generate siderophores, but seems to “borrow” them from other microbes, including E. coli (82). Aside from enterobactin, E. coli was demonstrated to provide Bacteroidetes with porphyrins, emphasizing this phylum's dependence on iron and heme (83). This also demonstrates a special symbiosis and interdependence between Bacteroidetes and E. coli (83). Since psychotropic drugs were demonstrated to eliminate both microbial groups, the porphyrin exchange may be impaired, resulting in lower iron stores (83). Indeed, recent studies have indicated that enterobactin possesses antioxidant properties and may contribute to host redox homeostasis (84). Moreover, IPA was demonstrated to exert antioxidant properties against iron-generated ROS, reversing metabolic disturbances associated with genomic damage (54, 85). Taken together, it would be interesting to study if psychotropic drugs-induced oxidative stress may lead to weight gain associated with these agents.
Iron and ROS
The connection between psychotropic drugs and iron has been previously documented as iron deficiency anemia, restless leg syndrome, tardive dyskinesia, akathisia, and neuroleptic malignant syndrome were associated with iron dysmetabolism (86–89). Moreover, low iron levels were linked to the negative symptoms of schizophrenia, while the offspring of hypoferremic mothers were found to be at risk of developing this disorder (90). Nutritional immunity, the shifting of iron from the extracellular to the intracellular compartment to withhold it from pathogens during infection, may be the mechanism, linking the motor adverse effects of psychotropic drugs to low extracellular iron (86, 88, 91). Indeed, since nutritional immunity leads to excess intracellular iron, placing this biometal in close proximity to lipids, the risk of lipid peroxidation and ROS generation is increased (92). Moreover, psychotropic drugs modulate lipid metabolism, regulating iron absorption and ROS generation (93). For example, Iron depletion was demonstrated in RSP-treated children and adolescents, possibly indicating iron shifting from the extracellular to the intracellular compartment via nutritional immunity (94).
In humans, heme and non-heme iron are absorbed at different GI tract locations as the uptake of the later occurs in the proximal small bowel where it is regulated by hepcidin, while the former is very efficiently absorbed in the colon (95). Bacteroidetes-generated propionate facilitates the absorption of iron, linking depletion of this phylum with iron dysmetabolism (15, 96–98). Indeed, as mentioned above, decreased host iron stores were previously reported in connection with psychotropic drugs (86).
In summary, several psychiatric medications may act as indirect iron chelators as they lower the absorption of this micronutrient via Bacteroidetes phylum depletion.
Gut Microbes and Psychotropic Drugs Metabolism
Cytochrome P450 enzymes play a key role in the metabolism of xenobiotics. These iron-containing cytochromes are expressed in both intestinal tissue and the liver, however the enteric systems require more research as their specifics are not clear at this time. Compounding this problem is the fact that gut microbes express as many as 3000 cytochrome P450 (CYP450) enzymes that transform most drugs and dietary molecules the host consumes (99).
The major host intestinal cytochrome, CYP3A4 metabolizes over 50% of common pharmaceuticals, while about 20% of clinically used drugs are metabolized by CYP1A2 (100, 101). For example, CLZ and OLZ, are processed by CYP1A2, RSP is partially metabolized in CYP3A4, while QTP is converted to N-desalkylquetiapine in CYP3A4 (102–105). As xenobiotic sensors AhR and PXR control the expression of CYP1A2 and CYP3A4, respectively, the activation status of these receptors can alter the bioavailability of the drugs metabolized by these cytochromes.
AhR is a ligand-activated receptor, complexed in the IECs cytosol with HSP90 protein. Upon AhR ligand-binding, the entire complex migrates to the nucleus where it activates AhR nuclear translocator (ARNT), enabling the transcription of many genes, including CYP1A1, CYP1A2, and CYP1B1, several lipid homeostasis genes and the Nrf-2 gene (37, 106–109). Nrf-2, itself a transcription factor, controls the expression of numerous other genes, including those encoding for iron storage proteins and phase I and II drug-metabolizing enzymes, associating this redox protein with drug metabolism and iron dyshomeostasis (110, 111).
Bacteroidetes phylum activates AhR by generating propionate which forms AhR ligands with indole. Depletion of these microbes by psychotropic drugs may alter the bioavailability and metabolism of these agents (10). For example, OLZ-induced Bacteroidetes phylum depletion and lower propionate, may under-activate AhR, suppressing the expression of CYP1A2 gene. Since OLZ metabolism is dependent on the CYP1A2 gene, its inhibition can alter the metabolism and bioavailability of this drug (Figure 2) (105).
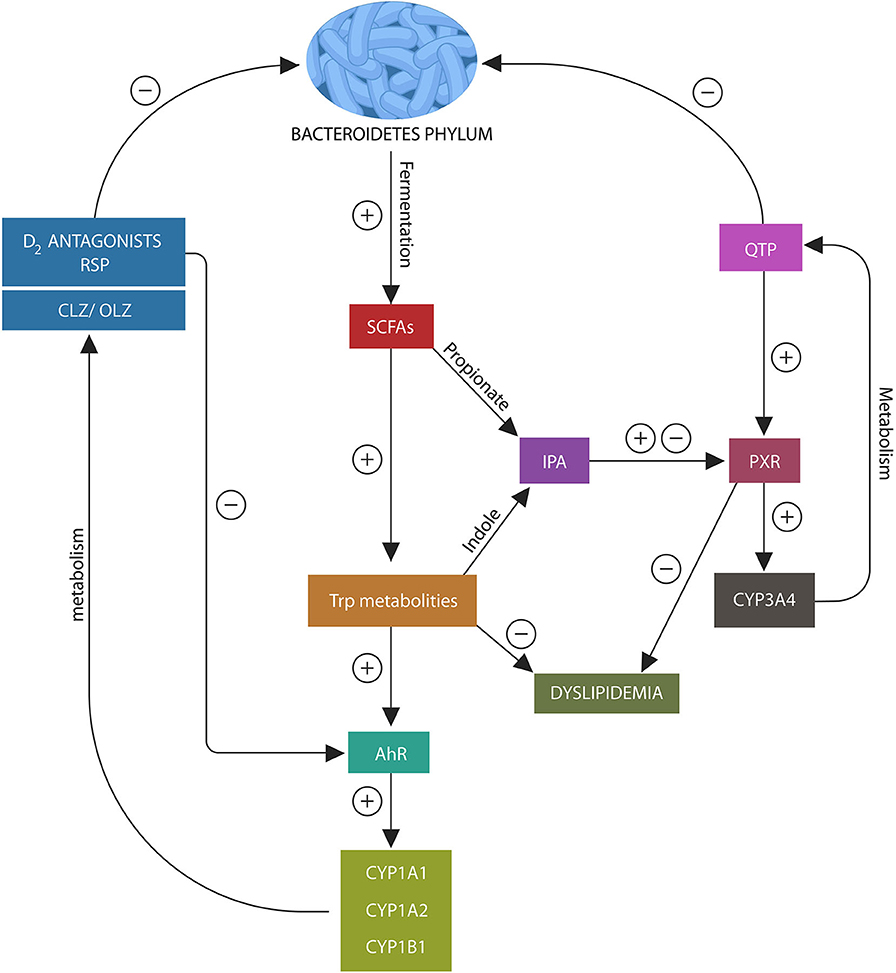
Figure 2. Psychotropic drugs deplete Bacteroidetes phylum as do most D2 blockers. This lowers the AhR and PXR ligands, propionate and indole, under-activating these xenobiotic sensors. This in turn, inhibits the expression of CYP1A1/A2, CYP1B1, and CYP3A4, impairing psychotropic drugs metabolism. In addition, loss of AhR and PXR ligands disrupts the expression of several lipid homeostasis genes and Nrf2 (not shown), leading to weight gain and impaired iron metabolism.
In the same manner, PXR receptor ligands enable the migration of this xenobiotic sensor to the nucleus from where it facilitates the expression of CYP3A4 and several lipid homeostasis genes (112–114) (Figure 3). Since QTP, metabolized by CYP3A4, is a potent PXR agonist, it may alter both its own and lipid metabolism, probably explaining the weight gain associated with this drug (115). Moreover, QTP, like other psychotropics, can induce gut dysbiosis and lower IPA biosynthesis, thus suppressing CYP3A4 gene and altering its own metabolism (Figure 2). A similar mechanism may be at work during the biotransformation of CLZ which is partially metabolized by CYP1A2. Bacteroidetes depletion and AhR under-activation may impair CLZ bioavailability and blood levels (105, 116). Moreover, recent studies in rodents found that psychotropic drugs can inhibit AhR directly by antagonizing D2 receptors, impairing the expression of CYP1A1, CYP1A2, and CYP1B1 (117) (Figure 2). Indeed, as most antipsychotic drugs are D2 blockers, they may lower their own metabolism both directly and via dysbiosis. Interestingly, valproic acid (VPA), a natural, branched short-chain fatty acid, was associated with intestinal dysbiosis as it also depletes Bacteroidetes, probably explaining the weight gain associated with this drug (118, 119). In addition, VPA was shown to augment the expression of intestinal and hepatic CYP3A4, indicating that this drug may alter its own metabolism via dysbiosis (120). These novel findings are relevant as they underline not only the importance of gut flora in drug metabolism but also the need for obtaining psychotropic blood levels in clinical practice.
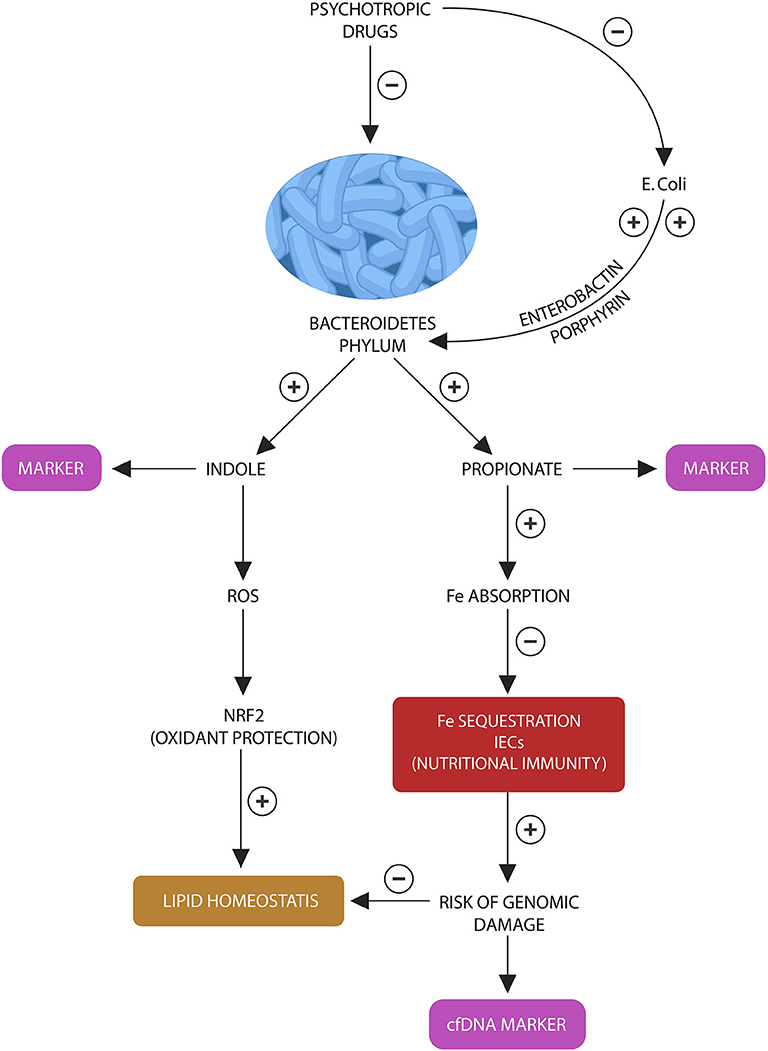
Figure 3. Bacteroidetes stress test: Bacteroidetes phylum and E. coli participate in a “special” symbiosis in which the later provides the former with iron-molecules enerobactin and porphyrins. Bacteroidetes in return generate indole and propionate (suggested as fecal biomarkers). In various combinations, indole and propionate are AhR and PXR ligands, contributing to lipid and redox homeostasis. Propionate also facilitates iron absorption, preventing the activation of nutritional immunity in intestinal macrophages and IECs. Lowered propionate (due to Bacteroidetes depletion) triggers nutritional immunity with iron sequestration, increasing the risk of ROS and genomic damage, measured by the cfDNA marker.
Taken together, the new science of pharmacomicrobiomics explains the role of the microbial organ in psychotropic drugs metabolism, underlining the interconnectedness between xenobiotic metabolism and nutrient harvesting.
Psychotropic Drugs As Antimicrobials
The awareness that psychotropic drugs have antimicrobial properties goes back to the nineteenth century when Paul Ehrlich observed the bactericidal action of methylene blue, a molecule that led the synthesis of chlorpromazine, ushering the development of antipsychotic agents (121). Moreover, antidepressant drugs can be traced back to the antimicrobial isoniazid which was found to induce euphoria in tuberculosis patients (122). For this reason, it should not be surprising that many psychotropic agents retain antimicrobial properties to this day. However, with the advent of the microbiome, these historical data have recaptured the attention of researchers and clinicians, prompting some to link chronic psychosis to infection, immunity, and antimicrobial treatments (123).
Most studies in humans have found that Bacteroidetes phylum (that together with Firmicutes comprise 90% of gut microbiota) is more vulnerable to psychotropic drugs than other phyla (6, 124). For example, chronic RSP treatment in children was associated with lower fecal Bacteroidetes compared to antipsychotic-naïve controls (97). This is surprising since Bacteroidetes are well-known for antibiotic-resistance by virtue of expressing numerous drug efflux pumps (DEPs) that facilitate the expulsion of drugs from the intracellular compartment (125). Interestingly, several psychotropic drugs, including the antipsychotic loxapine, phenothiazines, and selective serotonin reuptake inhibitors (SSRIs) were found to effectively block microbial DEPs, probably explaining their selective bactericidal action against Bacteroidetes phylum (126–128). Moreover, iron chelators were recently found to be effective DEP-inhibitors, a property that may explain their bactericidal action against the iron-dependent Bacteroides phylum (129). Indeed, another reason, Bacteroidetes phylum may be more susceptible to psychotropic drugs is that, unlike Firmicutes and Actinobacteria that can synthesize heme, Bacteroidetes, which lack the enzymatic machinery to synthesize this iron protein, depend on scavenging it from the colonic environment (82, 130, 131). Conversely, as Bacteroidetes phylum generates propionate that facilitates iron absorption, their depletion may impact host iron stores (12, 15, 97, 132). In addition, impaired iron absorption may activate nutritional immunity in IECs and macrophages to sequestrate this biometal and deny it to pathogens, exacerbating hypoferremia and metabolic syndrome (133, 134). On this point, a novel study in children and adolescents chronically treated with RSP found that iron status was inversely corelated with weight gain, contributing to dysmetabolic iron overload syndrome (DIOS) (135). This is significant as intracellular iron increases the risk of ROS generation that cannot be counteracted as Bacteroidetes depletion lowered IPA, a key gut antioxidant (98, 136, 137). Restoration of the adequate levels of AhR and PXR ligands via diet, pre, probiotics, or microbial transplant may upregulate Bacteroidetes phylum, attenuating psychotropic drugs-induced weight gain (138).
Taken together, recent studies seem to suggest that the anti-DEP properties of psychotropic drugs may selectively deplete Bacteroidetes phylum as these microorganisms express abundant DEPs and rely on these proteins for antibiotic defense. Conversely, depletion of Bacteroidetes phylum may impair iron and heme absorption, leading to DIOS.
Psychotropics, the New Antibiotics?
Animal studies have contributed further to the understanding of dysbiosis induced by the antimicrobial properties of psychotropic drugs. For example, a recent study reported that RSP-treated female mice exhibited significant weight gain and altered gut microbial diversity; however, when these rodents received a fecal transplant from RSP-naïve mice, the weight gain was reversed, underlining the role of commensals in obesity (97). Another preclinical study demonstrated that OLZ completely inhibited the growth of E. coli in vitro, emphasizing that the spectrum of this agent's antimicrobial properties extends beyond Bacteroidetes (12). On the other hand, antibiotics co-administered with OLZ, improved metabolic parameters, resulting in weight loss (15). It appears that a significantly reduced gut bacterial content, resembling germ-free status, is necessary to prevent psychotropics-induced weight gain, while a less drastic reduction in microbial number, has the opposite effect (19). A different study showed reduced gut commensal flora diversity in mice chronically treated with antidepressants. Interestingly, this study also found that excessive Ruminococcus flavefaciens can attenuate the efficacy of duloxetine, a serotonin-norepinephrine reuptake inhibitor (SNRI), suggesting microbiota involvement in the metabolism of this agent (139).
Other studies have reported that AADs are not the only psychotropics with antimicrobial activity as fluoxetine, sertraline, and escitalopram were reported to induce gut dysbiosis (6). For example, fluoxetine has demonstrated antimicrobial activity against Gram-positive microbes, including Staphylococcus, Enterococcus, and anaerobes such as Clostridium difficile and perfringens (140). Interestingly, as opposed to AAP drugs that affect primarily Gram negative Bacteroidetes, SSRIs appear to deplete Gram positive bacteria. Moreover, a novel study associated fluoxetine treatment with multi-antibiotic resistance via iron and ROS-induced DNA damage (141). Indeed, epidemiologic studies have associated long-term antidepressant treatment with ROS-induced obesity, connecting once more iron dysmetabolism with weight gain (142, 143).
The antimicrobial action of antipsychotic drugs against DEP-expressing bacterial strains has rendered these agents excellent candidates for antibiotic resistant bacteria (144). Indeed, a recent study found that phenothiazines, as a group are DEP-inhibitors, effective against several drug resistant bacterial strains, while another study found trifluoperazine beneficial to the treatment of sepsis (145, 146). In addition, QTP and OLZ were found effective against fungal DEPs and are currently being tested as anti-Cryptococcal drugs (147). Conversely, long-term treatment with antidepressant or antipsychotic drugs was associated with increased risk of methicillin-resistant Staphylococcus aureus (MRSA), further linking these agents to both the therapeutic and adverse effects of antibiotics (148). This is significant because chronic psychiatric patients with schizophrenia and SSD are frequently treatment-resistant and often require long-term use of high doses of psychotropic drugs to remain asymptomatic. Prolonged exposure to these agents increases the risk not only of weight gain and cardiometabolic disorders but also of antibiotic resistance, resulting in poorer outcomes and higher medical cost. Moreover, aside from psychotropics, several drug categories, including proton pump inhibitors (PPIs), histamine-2 (H2) blockers, antimitotic agents and non-steroidal anti-inflammatory drugs (NSAIDs) were reported to induce both dysbiosis and iron dysmetabolism, indicating the need for medical and psychiatric care integration (17, 86, 149, 150).
Markers and Interventions
Although life-changing for patients, psychotropic medications have been accompanied by an unintended consequence, an obesity epidemic that has increased the mortality, morbidity, and medical expenditures in this population (151). Fortunately, weight gain is a modifiable risk factor and a better understanding of its pathogenesis vis-à-vis psychotropic drugs may lead to the development of novel preventive strategies. Moreover, since preclinical studies have connected gut dysbiosis with the weight gain associated with psychotropic drugs, biological markers reflecting the health of gut microbes are of primary importance. For example, fecal propionate levels may mirror the status of Bacteroidetes phylum in general as these microbes are the major propionate producers. On the other hand, indole levels may be more species specific, reflecting Bacteroides thetaiotaomicron, that specializes in indole production. Since psychotropic drugs deplete the entire Bacteroidetes phylum, propionate, and indole levels may provide an index of microbial integrity. In addition, we propose a non-invasive peripheral blood marker, consisting of cell free DNA (cfDNA) with the specific Bacteroidetes motif, GTCGTT as an assessment tool for the psychotropic drugs-induced Bacteroidetes depletion. Aside from the markers of microbial loss, various nutrients and procedures, including fiber, probiotics, fecal microbial transplant, and vagus nerve manipulation were found helpful for preventing weight gain and restore physiological levels of Bacteroidetes phylum.
Beware of Stressed Bacteroidetes
Extracellular or cfDNA, a marker of eukaryotic and prokaryotic genomic damage, was demonstrated to strongly activate TLR9, an action that promptly turns off immunological tolerance to gut microbes and activates vigilance to potential pathogens (152). Recent studies have shown that cfDNA is released into the blood following eukaryotic or prokaryotic cell death, comprising a marker of microbial or host cells demise (153). Moreover, others have shown that bacterial DNA derived from Gram negative microbes, including Bacteroidetes, activates TLR9 more robustly than Gram positive DNA, suggesting that this receptor links immune activation to the body weight via AhR signaling (154). Furthermore, it has been established that Bacteroidetes DNA is marked by an overabundance of the genomic motifs GTCGTT, strong TLR9 activators, indicating that cfDNA carrying this sequence is a not only a hallmark of this phylum but also an influencer of body weight via TLR9-AhR-IL-10 signaling (155). For this reason, we construe that elevated GTCGTT cfDNA in peripheral blood may accurately reflect the risk of both psychotropic drugs-associated Bacteroidetes depletion and weight gain (156). Therefore, we propose a combined non-invasive peripheral blood test: real-time polymerase chain reaction (PCR) to detect cfDNA and CpG oligodeoxynucleotide (ODN) to identify GTCGTT motifs (157, 158).
We also believe that cfDNA alone, without the Bacteroidetes marker, may accurately mirror the activation of host nutritional immunity, iron sequestration and ROS-induced genomic damage in IECs and enteric macrophages. This probably takes place as a result of Bacteroidetes depletion as this phylum facilitates iron absorption by generating propionate (Figure 3). Insufficient iron may activate host nutritional immunity to preserve this biometal intracellularly, however chronic iron retention increases the risk of ROS and DNA damage, resulting in l cfDNA release. For this reason, we believe that cfDNA may accurately measure nutritional immunity, iron sequestration and genomic damage. Indeed, cfDNA is currently a marker of iron-induced DNA disruption in hemodialysis patients (159–161). Furthermore, since inter-kingdom signaling via ROS-Nrf2 mediate antioxidant and lipid homeostasis, deficient body iron stores may reflect the risk of weight gain (162, 163). Indeed, DIOS is encountered in many overweight individuals, indicating that excessive ROS generation may promote obesity (132). In addition, RSP-treated patients have demonstrated not only depleted iron stores but also weight gain proportional to hypoferremia (86).
In conclusion, to assess the integrity of host-microbiota interface, we propose a battery of four tests: peripheral blood cfDNA to reflect the status of host nutritional immunity, GTCGTT cfDNA to assess Bacteroides depletion along with fecal indole and propionate to further estimating the depletion of this phylum (Figure 3). We believe that the following laboratory assays can be optimal for detecting indole and propionate: hydroxylamine-based indole assay (HIA) as it was recently reported to accurately detect indole in complex clinical samples, including feces and liquid chromatography tandem mass spectrometry (LC-MS/MS) based on 3-nitrophenylhydrazone (3NPH) derivatization, an assay shown to accurately measure fecal propionate (164, 165).
Dietary Fiber as a Prebiotic
The hallmarks of Western diet, low fiber content, high refined sugars and fats, suggest that these nutrients, along with physical inactivity may, at least in part, account for the modern obesity epidemic (166). Novel studies have found that the general population of Western countries consumes an average of 10–20 g of dietary fiber per day (35–50 g being optimal), while much lower fiber intake characterizes the diet of chronic psychiatric patients (167–170). Both dietary fiber and fermenting microbes are necessary for the generation of indole and propionate. However, fiber was found to possess probiotic properties by promoting the growth of fermenting microbes, including Bacteroidetes (60, 171, 172). Aside from fiber, direct supplementation with sodium propionate was shown to increase energy expenditure and protect against high fat diet-induced obesity in rodents, suggesting that fiber or its derivative, propionate may reverse dyslipidemia in chronic psychiatric patients. However, prior to clinical use, more studies on sodium propionate are needed to assess its safety in humans. Fiber supplementation or high fiber diets, on the other hand, should be used routinely in chronic psychiatric patients (173).
Probiotics to Restore the Levels of Propionate
Probiotics or microbes beneficial to health have recently reawaken the interest of researchers and clinicians as several bacterial species, including Bacteroides fragilis and Bacteroides uniformis may selectively upregulate gut propionate levels (174, 175). For example, in preclinical studies, supplementation with B. uniformis was found to restore immunological and metabolic homeostasis caused by intestinal dysbiosis, indicating potential benefit for chronic psychiatric patients (176). Furthermore, indole-producing Bacteroides thetaiotaomicron has shown anti-obesity benefits in preclinical studies but to our knowledge, it has not been tested in humans (177). For this reason, more studies are needed as this microbe presents with the unique property of converting succinate to propionate (175). Since circulating succinate is increased in hypertension, ischemic heart disease, and type 2 diabetes, conditions prevalent in chronic psychiatric patients, a probiotic converting this molecule into the anorexigenic propionate is very promising (178).
Fecal Microbial Transplant (FMT) for Indole and Propionate Augmentation
FMT is an ancient procedure that has been practiced throughout the centuries to alter the balance of colonic microbes and protect against infections. FMT is commonly used in veterinary medicine, while in humans, it is currently approved for the treatment of Clostridium difficile infections (179, 180). The role of FMT in microbial dysbiosis-linked chronic disorders is still in the early stages and requires more studies in humans, specifically for developing adequate criteria for the identification of optimal donors (181). Based on findings from preclinical and small clinical studies, FMT may provide benefits in IBD and is currently being investigated for the treatment of several chronic conditions, including obesity and metabolic disorders (182, 183). We believe that when obtained from the adequate donors, FMT may have the potential to restore the physiological levels of propionate and indole in chronic psychiatric patients.
Deep Transcranial Magnetic Stimulation (dTMS) and Intermittent Vagal Blockade (vBloc)
Studies on dTMS, repetitive transcranial magnetic stimulation (rTMS) and transcranial direct current stimulation (tDCS) to decrease food intake and weight are currently ongoing but further data is needed before recommending these procedures to chronic psychiatric patients (180, 184, 185). In 2015, the U.S. Food and Drug Administration approved a vagal blocking device (vBloc) for the treatment of moderate to severe obesity, linking vagus nerve to feeding behavior (186). Interestingly, both intermittent vagal blockade and vagal stimulation were found effective for lowering body weight, suggesting an indirect effect on metabolism, probably mediated by gut microbes (187, 188). Moreover, a peripheral anti-inflammatory vagal system, operating via alpha 7-cholinergic nicotinic receptors on peripheral macrophages, has been known to dampen inflammation, suggesting that nicotinic signaling may also modulate the enteric microbes via a similar system (189, 190). Indeed, microbiota-derived acetylcholine (ACh) has been recently reported, suggesting ongoing cholinergic signaling between the microbial organ and vagus nerve (191). Interestingly, preclinical studies found a direct connection between enteric ACh and propionate, linking this neurotransmitter to lipid homeostasis (192). Recently, a study found higher levels of intestinal Bacteroidetes in smokers, suggesting that nicotinic cholinergic signaling may have trophic effects on this phylum (162). Since smoking and body weight are known to be inversely related, and smoking cessation has been associated weight gain, targeting nicotinic receptors may comprise a new strategy for Bacteroidetes phylum restoration (193). In addition, as weight loss is a reported adverse effect of cholinesterase inhibitors, including donepezil and rivastigmine, these agents may help restore physiological levels of Bacteroidetes in chronic psychiatric patients (194).
Conclusion
Psychotropic drugs-induced weight gain is marked by intestinal dysbiosis with Bacteroidetes phylum depletion. Propionate and indole, molecules that under normal circumstances are generated by Bacteroidetes, activate AhR and PXR xenobiotic receptors, modulating lipid and redox homeostasis. Bacteroidetes depletion by antibiotics, psychotropics or other drugs may deprive the host of these indispensable molecules, altering drug, lipid, and iron metabolism with subsequent weight gain. From this perspective, psychotropic drugs are indirect iron chelators as they lower the absorption of this biometal via gut microbes, decreasing body iron stores. The subsequent extracellular hypoferremia activates nutritional immunity with iron sequestration in enteric macrophages and IECs. Upregulated intracellular iron increases the risk of excessive ROS generation and the subsequent weight gain. Fortunately, obesity is a modifiable risk factor of general morbidity, therefore restoring the physiological levels of Bacteroidetes phylum by various strategies may attenuate or reverse the excess weight in chronic psychiatric patients. If validated, the biological markers described here, may offer the clinician an additional feed-back to estimate the imminence of weight-related complications.
Author Contributions
All authors listed have made a substantial, direct and intellectual contribution to the work, and approved it for publication.
Conflict of Interest
The authors declare that the research was conducted in the absence of any commercial or financial relationships that could be construed as a potential conflict of interest.
Abbreviations
SSD, schizophrenia-spectrum disorders; OLZ, olanzapine; CZP, clozapine; RSP, risperidone; QTP, quetiapine; AhR, aryl hydrocarbon receptor; PXR, pregnane X receptor; FMT, fecal microbiota transplant; tMS, transcranial magnetic stimulation; IECs, intestinal epithelial cells; Trp, tryptophan; TLR9, toll like receptor 9; NTS, nucleus tractus solitaries; POMC, pro-opiomelanocortin; CART, cocaine-and amphetamine-regulated transcript; B. theta, Bacteroidetes thetaiotaomicron; VPA, valproic acid; DEPs, drug efflux pumps; cfDNA, cell free DNA; HIA, hydroxylamine-based indole assay; vBloc, vagal blocking device.
References
1. Ojala K, Niskanen L, Tiihonen J, Paavola P, Putkonen A, Repo-Tiihonen E. Characterization of metabolic syndrome among forensic psychiatric inpatients. J Forens Psychiatry Psychol. (2008) 19:33–51. doi: 10.1080/14789940701562519
2. Hilton NZ, Ham E, Lang C, Harris GT. Weight gain and its correlates among forensic inpatients. Can J Psychiatry. (2015) 60:232–8. doi: 10.1177/070674371506000505
3. Basheer L, Kerem Z. Interactions between CYP3A4 and dietary polyphenols. Oxid Med Cell Longev. (2015) 2015:854015. doi: 10.1155/2015/854015
4. ElRakaiby M, Dutilh BE, Rizkallah MR, Boleij A, Cole JN, Aziz RK. Pharmacomicrobiomics: the impact of human microbiome variations on systems pharmacology and personalized therapeutics. OMICS. (2014) 18:402–14. doi: 10.1089/omi.2014.0018
5. Clarke G, Sandhu KV, Griffin BT, Dinan TG, Cryan JF, Hyland NP. Gut reactions: breaking down xenobiotic-microbiome interactions. Pharmacol Rev. (2019) 71:198–224. doi: 10.1124/pr.118.015768
6. Cussotto S, Clarke G, Dinan TG, Cryan JF. Psychotropics and the microbiome: a chamber of secrets. Psychopharmacology. (2019) 236:1411–32. doi: 10.1007/s00213-019-5185-8
7. Koppel N, Maini Rekdal V, Balskus EP. Chemical transformation of xenobiotics by the human gut microbiota. Science. (2017) 356:eaag2770. doi: 10.1126/science.aag2770
8. Wilson ID, Nicholson JK. Gut microbiome interactions with drug metabolism, efficacy, and toxicity. Transl Res. (2017) 179:204–22. doi: 10.1016/j.trsl.2016.08.002
9. Doestzada M, Vila AV, Zhernakova A, Doestzada M, Vila AV, Zhernakova A, et al. Pharmacomicrobiomics: a novel route towards personalized medicine?. Protein Cell. (2018) 9:432–45. doi: 10.1007/s13238-018-0547-2
10. Bretler T, Weisberg H, Koren O, Neuman H. The effects of antipsychotic medications on microbiome and weight gain in children and adolescents. BMC Med. (2019) 17:112. doi: 10.1186/s12916-019-1346-1
11. Flowers SA, Baxter NT, Ward KM, Kraal AZ, McInnis MG, Schmidt TM, et al. Effects of atypical antipsychotic treatment and resistant starch supplementation on gut microbiome composition in a cohort of patients with bipolar disorder or schizophrenia. Pharmacotherapy. (2019) 39:161–70. doi: 10.1002/phar.2214
12. Morgan AP, Crowley JJ, Nonneman RJ, Quackenbush CR, Miller CN, Ryan A, et al. The antipsychotic olanzapine interacts with the gut microbiome to cause weight gain in mouse. PLoS ONE. (2014) 9:e115225. doi: 10.1371/journal.pone.0115225
13. Luo C, Wang X, Huang H, Mao X, Zhou H, Liu Z. Effect of metformin on antipsychotic-induced metabolic dysfunction: the potential role of gut-brain axis. Front Pharmacol. (2019) 10:371. doi: 10.3389/fphar.2019.00371
14. Kao AC, Spitzer S, Anthony DC, Lennox B, Burnet PJ. Prebiotic attenuation of olanzapine-induced weight gain in rats: analysis of central and peripheral biomarkers and gut microbiota. Transl Psychiatry. (2018) 8:66. doi: 10.1038/s41398-018-0116-8
15. Davey KJ, Cotter PD, O'Sullivan O, Crispie F, Dinan TG, Cryan JF, et al. Antipsychotics and the gut microbiome: olanzapine-induced metabolic dysfunction is attenuated by antibiotic administration in the rat. Transl Psychiatry. (2013) 3:e309. doi: 10.1038/tp.2013.83
16. Maier L, Pruteanu M, Kuhn M, Zeller G, Telzerow A, Anderson EA, et al. Extensive impact of non-antibiotic drugs on human gut bacteria. Nature. (2018) 555:623–8. doi: 10.1038/nature25979
17. Le Bastard Q, Al-Ghalith GA, Grégoire M, Chapelet G, Javaudin F, Dailly E, et al. Systematic review: human gut dysbiosis induced by non-antibiotic prescription medications. Aliment Pharmacol Ther. (2018) 47:332–45. doi: 10.1111/apt.14451
18. Fung TC, Vuong HE, Luna CDG, Pronovost GN, Aleksandrova AA, Riley NG, et al. Intestinal serotonin and fluoxetine exposure modulate bacterial colonization in the gut. Nat Microbiol. (2019) 4:2064–73. doi: 10.1038/s41564-019-0540-4
19. Skonieczna-Zydecka K, Łoniewski I, Misera A, Stachowska E, Maciejewska D, Marlicz W, et al. Second-generation antipsychotics and metabolism alterations: a systematic review of the role of the gut microbiome. Psychopharmacology. (2019) 236:1491–512. doi: 10.1007/s00213-018-5102-6
20. Mitchell NS, Catenacci VA, Wyatt HR, Hill JO. Obesity: overview of an epidemic. Psychiatr Clin North Am. (2011) 34:717–32. doi: 10.1016/j.psc.2011.08.005
21. Bradshaw T, Mairs H. Obesity and serious mental ill health: a critical review of the literature. Healthcare. (2014) 2:166–82. doi: 10.3390/healthcare2020166
22. Mitchell AJ, Vancampfort D, Sweers K, van Winkel R, Yu W, De Hert M. Prevalence of metabolic syndrome and metabolic abnormalities in schizophrenia and related disorders–a systematic review and meta-analysis. Schizophr Bull. (2013) 39:306–18. doi: 10.1093/schbul/sbr148
23. Jonikas JA, Cook JA, Razzano LA, Steigman PJ, Hamilton MM, Swarbrick MA, et al. Associations between gender and obesity among adults with mental illnesses in a community health screening study. Community Ment Health J. (2016) 52:406–15. doi: 10.1007/s10597-015-9965-2
24. Katekaru M, Minn CE, Pobutsky AM. Weight reduction among people with severe and persistent mental illness after health behavior counseling and monitoring. Hawaii J Med Public Health. (2015) 74:146–9.
25. Mustillo S, Worthman C, Erkanli A, Keeler G, Angold A, Costello EJ. Obesity and psychiatric disorder: developmental trajectories. Pediatrics. (2003) 111(4 Pt 1):851–9. doi: 10.1542/peds.111.4.851
26. Ilyas A, Chesney E, Patel R. Improving life expectancy in people with serious mental illness: should we place more emphasis on primary prevention? Br J Psychiatry. (2017) 211:194–7. doi: 10.1192/bjp.bp.117.203240
27. Harris LW, Guest PC, Wayland MT, Umrania Y, Krishnamurthy D, Rahmoune H, et al. Schizophrenia: metabolic aspects of aetiology, diagnosis and future treatment strategies. Psychoneuroendocrinology. (2013) 38:752–66. doi: 10.1016/j.psyneuen.2012.09.009
28. Ventriglio A, Gentile A, Stella E, Bellomo A. Metabolic issues in patients affected by schizophrenia: clinical characteristics and medical management. Front Neurosci. (2015) 9:297. doi: 10.3389/fnins.2015.00297
29. Ryan MC, Collins P, Thakore JH. Impaired fasting glucose tolerance in first-episode, drug-naive patients with schizophrenia. Am J Psychiatry. (2003) 160:284–9. doi: 10.1176/appi.ajp.160.2.284
30. Gorbovskaya I, Kanji S, Liu JCW, MacKenzie NE, Agarwal SM, Marshe VS, et al. Investigation of the gut microbiome in patients with schizophrenia and clozapine-induced weight gain: protocol and clinical characteristics of first patient cohorts. Neuropsychobiology. (2019) 79:1–8. doi: 10.1159/000494696
31. Nishida A, Inoue R, Inatomi O, Bamba S, Naito Y, Andoh A. Gut microbiota in the pathogenesis of inflammatory bowel disease. Clin J Gastroenterol. (2018) 11:1–10. doi: 10.1007/s12328-017-0813-5
32. Nagpal R, Newman TM, Wang S, Jain S, Lovato JF, Yadav H. Obesity-linked gut microbiome dysbiosis associated with derangements in gut permeability and intestinal cellular homeostasis independent of diet. J Diabetes Res. (2018) 2018:3462092. doi: 10.1155/2018/3462092
33. Cryan JF, O'Riordan KJ, Cowan CSM, Sandhu KV, Bastiaanssen TFS, Boehme M, et al. The microbiota-gut-brain axis. Physiol Rev. (2019) 99:1877–2013. doi: 10.1152/physrev.00018.2018
34. van de Guchte M, Blottière HM, Doré J. Humans as holobionts: implications for prevention and therapy. Microbiome. (2018) 6:81. doi: 10.1186/s40168-018-0466-8
35. Postler TS, Ghosh S. Understanding the holobiont: how microbial metabolites affect human health and shape the immune system. Cell Metab. (2017) 26:110–30. doi: 10.1016/j.cmet.2017.05.008
36. Kim CH. Immune regulation by microbiome metabolites. Immunology. (2018) 154:220–9. doi: 10.1111/imm.12930
37. Murray IA, Perdew GH. Ligand activation of the Ah receptor contributes to gastrointestinal homeostasis. Curr Opin Toxicol. (2017) 2:15–23. doi: 10.1016/j.cotox.2017.01.003
38. Rannug A, Rannug U. The tryptophan derivative 6-formylindolo[3,2-b]carbazole, FICZ, a dynamic mediator of endogenous aryl hydrocarbon receptor signaling, balances cell growth and differentiation. Crit Rev Toxicol. (2018) 48:555–74. doi: 10.1080/10408444.2018.1493086
39. Hubbard TD, Murray IA, Perdew GH. Indole and tryptophan metabolism: endogenous and dietary routes to ah receptor activation. Drug Metab Dispos. (2015) 43:1522–35. doi: 10.1124/dmd.115.064246
40. Moyer BJ, Rojas IY, Kerley-Hamilton JS, Nemani KV, Trask HW, Ringelberg CS, et al. Obesity and fatty liver are prevented by inhibition of the aryl hydrocarbon receptor in both female and male mice. Nutr Res. (2017) 44:38–50. doi: 10.1016/j.nutres.2017.06.002
41. Alexeev EE, Lanis JM, Kao DJ, Campbell EL, Kelly CJ, Battista KD, et al. Microbiota-derived indole metabolites promote human and murine intestinal homeostasis through regulation of interleukin-10 receptor. Am J Pathol. (2018) 188:1183–94. doi: 10.1016/j.ajpath.2018.01.011
42. Esposito K, Pontillo A, Giugliano F, Giugliano G, Marfella R, Nicoletti G, et al. Association of low interleukin-10 levels with the metabolic syndrome in obese women. J Clin Endocrinol Metab. (2003) 88:1055–8. doi: 10.1210/jc.2002-021437
43. Wenninger J, Meinitzer A, Holasek S, Schnedl WJ, Zelzer S, Mangge H, et al. Associations between tryptophan and iron metabolism observed in individuals with and without iron deficiency. Sci Rep. (2019) 9:14548. doi: 10.1038/s41598-019-51215-8
44. Brown K, DeCoffe D, Molcan E, Gibson DL. Diet-induced dysbiosis of the intestinal microbiota and the effects on immunity and disease. Nutrients. (2012) 4:1095–119. doi: 10.3390/nu4081095
45. Larigot L, Juricek L, Dairou J, Coumoul X. AhR signaling pathways and regulatory functions. Biochim Open. (2018) 7:1–9. doi: 10.1016/j.biopen.2018.05.001
46. Wada T, Sunaga H, Miyata K, Shirasaki H, Uchiyama Y, Shimba S. Aryl hydrocarbon receptor plays protective roles against high fat diet (HFD)-induced hepatic steatosis and the subsequent lipotoxicity via direct transcriptional regulation of Socs3 gene expression. J Biol Chem. (2016) 291:7004–16. doi: 10.1074/jbc.M115.693655
47. Spruiell K, Jones DZ, Cullen JM, Awumey EM, Gonzalez FJ, Gyamfi MA. Role of human pregnane X receptor in high fat diet-induced obesity in pre-menopausal female mice. Biochem Pharmacol. (2014) 89:399–412. doi: 10.1016/j.bcp.2014.03.019
48. Defois C, Ratel J, Garrait G, Denis S, Le Goff O, Talvas J, et al. Food chemicals disrupt human gut microbiota activity and impact intestinal homeostasis as revealed by in vitro systems. Sci Rep. (2018) 8:11006. doi: 10.1038/s41598-018-29376-9
49. Kim, Chang H. Microbiota or short-chain fatty acids: which regulates diabetes? Cell Mol Immunol. (2018) 15:88–91. doi: 10.1038/cmi.2017.57
50. Shinde R, Hezaveh K, Halaby MJ, Kloetgen A, Chakravarthy A, da Silva Medina T, et al. Apoptotic cell-induced AhR activity is required for immunological tolerance and suppression of systemic lupus erythematosus in mice and humans. Nat Immunol. (2018) 19:571–82. doi: 10.1038/s41590-018-0107-1
51. Thaxton JE, Romero R, Sharma S. TLR9 activation coupled to IL-10 deficiency induces adverse pregnancy outcomes. J Immunol. (2009) 183:1144–54. doi: 10.4049/jimmunol.0900788
52. Nishimoto S, Fukuda D, Higashikuni Y, Higashikuni Y, Tanaka K, Kim-Kaneyama J-R, et al. Obesity-induced DNA released from adipocytes stimulates chronic adipose tissue inflammation and insulin resistance. Sci Adv. (2016) 2:e1501332. doi: 10.1126/sciadv.1501332
53. Hong CP, Yun CH, Lee GW, Park A, Kim YM, Jang MH. TLR9 regulates adipose tissue inflammation and obesity-related metabolic disorders. Obesity. (2015) 23:2199–206. doi: 10.1002/oby.21215
54. Rani V, Deep G, Singh RK, Palle K, Yadav UC. Oxidative stress and metabolic disorders: pathogenesis and therapeutic strategies. Life Sci. (2016) 148:183–93. doi: 10.1016/j.lfs.2016.02.002
55. Zhan K, Gong X, Chen Y, Jiang M, Yang T, Zhao G. Short-chain fatty acids regulate the immune responses via G protein-coupled receptor 41 in bovine rumen epithelial cells. Front Immunol. (2019) 10:2042. doi: 10.3389/fimmu.2019.02042
56. Chambers ES, Preston T, Frost G, Morrison DJ. Role of gut microbiota-generated short-chain fatty acids in metabolic and cardiovascular health. Curr Nutr Rep. (2018) 7:198–206. doi: 10.1007/s13668-018-0248-8
57. Gao J, Xu K, Liu H, Liu G, Bai M, Peng C, et al. Impact of the gut microbiota on intestinal immunity mediated by tryptophan metabolism. Front Cell Infect Microbiol. (2018) 8:13. doi: 10.3389/fcimb.2018.00013
58. Parkinson JR, Chaudhri OB, Bell JD. Imaging appetite-regulating pathways in the central nervous system using manganese-enhanced magnetic resonance imaging. Neuroendocrinology. (2009) 89:121–30. doi: 10.1159/000163751
59. Chambers ES, Viardot A, Psichas A, Morrison DJ, Murphy KG, Zac-Varghese SEK, et al. Effects of targeted delivery of propionate to the human colon on appetite regulation, body weight maintenance and adiposity in overweight adults. Gut. (2015) 64:1744–54. doi: 10.1136/gutjnl-2014-307913
60. Chambers ES, Byrne CS, Aspey K, Chen Y, Khan S, Morrison DL, et al. Acute oral sodium propionate supplementation raises resting energy expenditure and lipid oxidation in fasted humans. Diabetes Obes Metab. (2018) 20:1034–9. doi: 10.1111/dom.13159
61. Hoyles L, Snelling T, Umlai UK, Nicholson JK, Carding SR, Glen RC, et al. Microbiome-host systems interactions: protective effects of propionate upon the blood-brain barrier. Microbiome. (2018) 6:55. doi: 10.1186/s40168-018-0439-y
62. Halford JC, Harrold JA. 5-HT(2C) receptor agonists and the control of appetite. Handb Exp Pharmacol. (2012) 349–56. doi: 10.1007/978-3-642-24716-3_16
63. Taleb S. Tryptophan dietary impacts gut barrier and metabolic diseases. Front Immunol. (2019) 10:2113. doi: 10.3389/fimmu.2019.02113
64. Osadchiy V, Labus JS, Gupta A, Jacobs J, Ashe-McNalley C, Hsiao EY, et al. Correlation of tryptophan metabolites with connectivity of extended central reward network in healthy subjects. PLoS ONE. (2018) 13:e0201772. doi: 10.1371/journal.pone.0201772
65. Kumar A, Sperandio V. Indole signaling at the host-microbiota-pathogen interface. mBio. (2019) 10:e01031–19. doi: 10.1128/mBio.01031-19
66. Chimerel C, Emery E, Summers DK, Keyser U, Gribble FM, Reimann F. Bacterial metabolite indole modulates incretin secretion from intestinal enteroendocrine L cells. Cell Rep. (2014) 9:1202–8. doi: 10.1016/j.celrep.2014.10.032
67. Sumara G, Sumara O, Kim JK, Karsenty G. Gut-derived serotonin is a multifunctional determinant to fasting adaptation. Cell Metab. (2012) 16:588–600. doi: 10.1016/j.cmet.2012.09.014
68. Mawe GM, Hoffman JM. Serotonin signalling in the gut–functions, dysfunctions and therapeutic targets. Nat Rev Gastroenterol Hepatol. (2013) 10:473–86. doi: 10.1038/nrgastro.2013.105
69. Terry N, Margolis KG. Serotonergic mechanisms regulating the GI tract: experimental evidence and therapeutic relevance. Handb Exp Pharmacol. (2017) 239:319–42. doi: 10.1007/164_2016_103
70. Kristensen MS, Kjærulff TM, Ersbøll AK, Green A, Hallas J, Thygesen LC. The influence of antidepressants on the disease course among patients with Crohn's disease and ulcerative colitis-A danish nationwide register-based cohort study. Inflamm Bowel Dis. (2019) 25:886–93. doi: 10.1093/ibd/izy367
71. Venkatesh M, Mukherjee S, Wang H, Li H, Sun K, Benechet AP, et al. Symbiotic bacterial metabolites regulate gastrointestinal barrier function via the xenobiotic sensor PXR and Toll-like receptor 4. Immunity. (2014) 41:296–310. doi: 10.1016/j.immuni.2014.06.014
72. Wikoff WR, Anfora AT, Liu J, Schultz PG, Lesley SA, Peters EC, et al. Metabolomics analysis reveals large effects of gut microflora on mammalian blood metabolites. Proc Natl Acad Sci USA. (2009) 106:3698–703. doi: 10.1073/pnas.0812874106
73. Abdul Rahim MBH, Chilloux J, Martinez-Gili L, Neves AL, Myridakis A, Gooderham N, et al. Diet-induced metabolic changes of the human gut microbiome: importance of short-chain fatty acids, methylamines and indoles. Acta Diabetol. (2019) 56:493–500. doi: 10.1007/s00592-019-01312-x
74. Jones L, Kumar J, Mistry A, Chittoor Mana ST, Perry G, Reddy VP, et al. The transformative possibilities of the microbiota and mycobiota for health, disease, aging, and technological innovation. Biomedicines. (2019) 7:24. doi: 10.3390/biomedicines7020024
75. Obrenovich M, Rai H, Mana TS, Shola D, McCloskey B. Dietary co-metabolism within the microbiota-gut-brain-endocrine metabolic interactome. BAO Microbiol. (2017) 2:22.
76. Beck GCh, Brinkkoetter P, Hanusch C, Schulte J, van Ackern K, van der Woude FJ, et al. Clinical review: immunomodulatory effects of dopamine in general inflammation. Crit Care. (2004) 8:485–91. doi: 10.1186/cc2879
77. Roager HM, Licht TR. Microbial tryptophan catabolites in health and disease. Nat Commun. (2018) 9:3294. doi: 10.1038/s41467-018-05470-4
78. Melhem H, Kaya B, Ayata CK, Hruz P, Niess JH. Metabolite-sensing G protein-coupled receptors connect the diet-microbiota-metabolites axis to inflammatory bowel disease. Cells. (2019) 8:450. doi: 10.3390/cells8050450
79. Dichtl S, Demetz E, Haschka D, Tymoszuk P, Petzer V, Nairz M, et al. Dopamine is a siderophore-like iron chelator that promotes Salmonella enterica serovar typhimurium virulence in mice. MBio. (2019) 10:e02624–18. doi: 10.1128/mBio.02624-18
80. Freestone PP, Lyte M, Neal CP, Maggs AF, Haigh RD, Williams PH. The mammalian neuroendocrine hormone norepinephrine supplies iron for bacterial growth in the presence of transferrin or lactoferrin. J Bacteriol. (2000) 182:6091–8. doi: 10.1128/JB.182.21.6091-6098.2000
81. Sewell AK, Han M, Qi B. An unexpected benefit from E. coli: how enterobactin benefits host health. Microb Cell. (2018) 5:469–71. doi: 10.15698/mic2018.10.653
82. Rocha ER, Krykunivsky AS. Anaerobic utilization of Fe(III)-xenosiderophores among Bacteroides species and the distinct assimilation of Fe(III)-ferrichrome by bacteroides fragilis within the genus. Microbiologyopen. (2017) 6:e00479. doi: 10.1002/mbo3.479
83. Halpern D, Gruss A. A sensitive bacterial-growth-based test reveals how intestinal bacteroides meet their porphyrin requirement. BMC Microbiol. (2015) 15:282. doi: 10.1186/s12866-015-0616-0
84. Johnstone TC, Nolan EM. Beyond iron: non-classical biological functions of bacterial siderophores. Dalton Trans. (2015) 44:6320–39. doi: 10.1039/C4DT03559C
85. Karbownik M, Reiter RJ, Garcia JJ, Cabrera J, Burkhardt S, Osuna C, et al. Indole-3-propionic acid, a melatonin-related molecule, protects hepatic microsomal membranes from iron-induced oxidative damage: relevance to cancer reduction. J Cell Biochem. (2001) 81:507–13.
86. Calarge CA, Ziegler EE. Iron deficiency in pediatric patients in long-term risperidone treatment. J Child Adolesc Psychopharmacol. (2013) 23:101–9. doi: 10.1089/cap.2012.0046
87. Arinola G, Idonije B, Akinlade K, Ihenyen O. Essential trace metals and heavy metals in newly diagnosed schizophrenic patients and those on anti-psychotic medication. J Res Med Sci. (2010) 15:245–9.
88. Hofmann M, Seifritz E, Botschev C, Kräuchi K, Müller-Spahn F. Serum iron and ferritin in acute neuroleptic akathisia. Psychiatry Res. (2000) 93:201–7. doi: 10.1016/S0165-1781(00)00115-3
89. McCall WV, Riley MA, Hodges C, McCloud L, Phillips M, Rosenquist PB. Asenapine-induced restless legs syndrome: differentiation from akathisia. J Clin Sleep Med. (2014) 10:1341–2. doi: 10.5664/jcsm.4296
90. Kim S-W, Stewart R, Park W-O, Jhon M, Lee J-Y, Kim S-Y, et al. Latent iron deficiency as a marker of negative symptoms in patients with first-episode schizophrenia spectrum disorder. Nutrients. (2018) 10:1707. doi: 10.3390/nu10111707
91. Hennigar SR, McClung JP. Nutritional immunity: starving pathogens of trace minerals. Am J Lifestyle Med. (2016) 10:170–73. doi: 10.1177/1559827616629117
92. Lee WJ. Bacterial-modulated signaling pathways in gut homeostasis. Sci Signal. (2008) 1:pe24. doi: 10.1126/stke.121pe24
93. Jones RM, Mercante JW, Neish AS. Reactive oxygen production induced by the gut microbiota: pharmacotherapeutic implications. Curr Med Chem. (2012) 19:1519–29. doi: 10.2174/092986712799828283
94. Shin S, Wakabayashi N, Misra V, Biswal S, Lee GH, Agoston ES, et al. NRF2 modulates aryl hydrocarbon receptor signaling: influence on adipogenesis. Mol Cell Biol. (2007) 27:7188–97. doi: 10.1128/MCB.00915-07
95. Young I, Parker HM, Rangan A, Prvan T, Cook RL, Donges CE, et al. Association between haem and non-haem iron intake and serum ferritin in healthy young women. Nutrients. (2018) 10:81. Published 2018 Jan 12. doi: 10.3390/nu10010081
96. Adams S, Sello CT, Qin G-X, Che D, Han R. Does dietary fiber affect the levels of nutritional components after feed formulation? Fibers. (2018) 6:29. doi: 10.3390/fib6020029
97. Bahr SM, Tyler BC, Wooldridge N, Butcher BD, Burns TL, Teesch LM, et al. Use of the second-generation antipsychotic, risperidone, and secondary weight gain are associated with an altered gut microbiota in children. Transl Psychiatry. (2015) 5:e652. doi: 10.1038/tp.2015.135
98. Bouglé D, Vaghefi-Vaezzadeh N, Roland N, Bouvard G, Arhan P, Bureau F, et al. Influence of short-chain fatty acids on iron absorption by proximal colon. Scand J Gastroenterol. (2002) 37:1008–11. doi: 10.1080/003655202320378176
99. Nichols RG, Peters JM, Patterson AD. Interplay between the host, the human microbiome, and drug metabolism. Hum Genomics. (2019) 13:27. Published 2019 Jun 11. doi: 10.1186/s40246-019-0211-9
100. Teo YL, Ho HK, Chan A. Metabolism-related pharmacokinetic drug-drug interactions with tyrosine kinase inhibitors: current understanding, challenges and recommendations. Br J Clin Pharmacol. (2015) 79:241–53. doi: 10.1111/bcp.12496
101. Wang B, Zhou SF. Synthetic and natural compounds that interact with human cytochrome P450 1A2 and implications in drug development. Curr Med Chem. (2009) 16:4066–218. doi: 10.2174/092986709789378198
102. Bezirtzoglou EE. Intestinal cytochromes P450 regulating the intestinal microbiota and its probiotic profile. Microb Ecol Health Dis. (2012) 23:10. doi: 10.3402/mehd.v23i0.18370
103. Chetty M, Murray M. CYP-mediated clozapine interactions: how predictable are they? Curr Drug Metab. (2007) 8:307–13. doi: 10.2174/138920007780655469
104. Bakken GV, Molden E, Knutsen K, Lunder N, Hermann M. Metabolism of the active metabolite of quetiapine, N-desalkylquetiapine in vitro. Drug Metab Dispos. (2012) 40:1778–84. doi: 10.1124/dmd.112.045237
105. Prior TI, Baker GB. Interactions between the cytochrome P450 system and the second-generation antipsychotics. J Psychiatry Neurosci. (2003) 28:99–112.
106. Hankinson O. The role of AHR-inducible cytochrome P450s in metabolism of polyunsaturated fatty acids. Drug Metab Rev. (2016) 48:342–50. doi: 10.1080/03602532.2016.1197240
107. Kerley-Hamilton JS, Trask HW, Ridley CJ, Dufour E, Ringelberg CS, Nurinova N, et al. Obesity is mediated by differential aryl hydrocarbon receptor signaling in mice fed a Western diet. Environ Health Perspect. (2012) 120:1252–9. doi: 10.1289/ehp.1205003
108. Dietrich C. Antioxidant functions of the aryl hydrocarbon receptor. Stem Cells Int. (2016) 2016:7943495. doi: 10.1155/2016/7943495
109. Tanos R, Murray IA, Smith PB, Patterson A, Perdew GH. Role of the Ah receptor in homeostatic control of fatty acid synthesis in the liver. Toxicol Sci. (2012) 129:372–9. doi: 10.1093/toxsci/kfs204
110. Wu KC, Cui JY, Klaassen CD. Effect of graded Nrf2 activation on phase-I and -II drug metabolizing enzymes and transporters in mouse liver. PLoS ONE. (2012) 7:e39006. doi: 10.1371/journal.pone.0039006
111. Kerins MJ, Ooi A. The roles of NRF2 in modulating cellular iron homeostasis. Antioxid Redox Signal. (2018) 29:1756–73. doi: 10.1089/ars.2017.7176
112. Oladimeji PO, Lin W, Brewer CT, Chen T. Glucose-dependent regulation of pregnane X receptor is modulated by AMP-activated protein kinase. Sci Rep. (2017) 7:46751. doi: 10.1038/srep46751
113. Hassani-Nezhad-Gashti F, Kummu O, Karpale M, Rysä J, Hakkola J. Nutritional status modifies pregnane X receptor regulated transcriptome. Sci Rep. (2019) 9:16728. doi: 10.1038/s41598-019-53101-9
114. Helsley RN, Sui Y, Ai N, Park SH, Welsh WJ, Zhou C. Pregnane X receptor mediates dyslipidemia induced by the HIV protease inhibitor amprenavir in mice. Mol Pharmacol. (2013) 83:1190–9. doi: 10.1124/mol.113.085753
115. Meng Z, Gwag T, Sui Y, Park SH, Zhou X, Zhou C. The atypical antipsychotic quetiapine induces hyperlipidemia by activating intestinal PXR signaling. JCI Insight. (2019) 4:e125657. doi: 10.1172/jci.insight.125657
116. Doude van Troostwijk LJ, Koopmans RP, Vermeulen HD, Guchelaar HJ. CYP1A2 activity is an important determinant of clozapine dosage in schizophrenic patients. Eur J Pharm Sci. (2003) 20:451–7. doi: 10.1016/j.ejps.2003.09.010
117. Harkitis P, Daskalopoulos EP, Malliou F, Lang MA, Marselos M, Fotopoulos A, et al. Dopamine D2-receptor antagonists down-regulate CYP1A1/2 and CYP1B1 in the rat liver. PLoS ONE. (2015) 10:e0128708. doi: 10.1371/journal.pone.0128708
118. Liu F, Horton-Sparks K, Hull V, Li RW, Martínez-Cerdeño V. The valproic acid rat model of autism presents with gut bacterial dysbiosis similar to that in human autism. Mol Autism. (2018) 9:61. doi: 10.1186/s13229-018-0251-3
119. Chang P, Orabi B, Deranieh RM, Dham M, Hoeller O, Shimshoni JA, et al. The antiepileptic drug valproic acid and other medium-chain fatty acids acutely reduce phosphoinositide levels independently of inositol in dictyostelium. Dis Model Mech. (2012) 5:115–24. doi: 10.1242/dmm.008029
120. Cerveny L, Svecova L, Anzenbacherova E, Vrzal R, Staud F, Dvorak Z, et al. Valproic acid induces CYP3A4 and MDR1 gene expression by activation of constitutive androstane receptor and pregnane X receptor pathways. Drug Metab Dispos. (2007) 35:1032–41. doi: 10.1124/dmd.106.014456
121. Oz M, Lorke DE, Hasan M, Petroianu GA. Cellular and molecular actions of methylene blue in the nervous system. Med Res Rev. (2011) 31:93–117. doi: 10.1002/med.20177
122. Hillhouse TM, Porter JH. A brief history of the development of antidepressant drugs: from monoamines to glutamate. Exp Clin Psychopharmacol. (2015) 23:1–21. doi: 10.1037/a0038550
123. Klein-Petersen AW, Köhler-Forsberg O, Benros ME. Infections, antibiotic treatment and the mircrobiome in relation to schizophrenia. Schizophr Res. (2019) 31. doi: 10.1016/j.schres.2019.11.033
124. Rinninella E, Raoul P, Cintoni M, Franceschi F, Donato Miggiano GA, Gasbarrini A, et al. What is the healthy gut microbiota composition? A changing ecosystem across age, environment, diet, and diseases. Microorganisms. (2019) 7:14. doi: 10.3390/microorganisms7010014
125. Wexler HM. Pump it up: occurrence and regulation of multi-drug efflux pumps in bacteroides fragilis. Anaerobe. (2012) 18:200–8. doi: 10.1016/j.anaerobe.2011.12.017
126. Yang CY, Hsu CY, Fang CS, Shiau CW, Chen CS, Chiu HC. Loxapine, an antipsychotic drug, suppresses intracellular multiple-antibiotic-resistant salmonella enterica serovar typhimurium in macrophages. J Microbiol Immunol Infect. (2019) 52:638–47. doi: 10.1016/j.jmii.2019.05.006
127. Munoz-Bellido JL, Munoz-Criado S, Garcìa-Rodrìguez JA. Antimicrobial activity of psychotropic drugs: selective serotonin reuptake inhibitors. Int J Antimicrob Agents. (2000) 14:177–80. doi: 10.1016/S0924-85799900154-5
128. Amaral L, Fanning S, Pagès JM. Efflux pumps of gram-negative bacteria: genetic responses to stress and the modulation of their activity by pH, inhibitors, and phenothiazines. Adv Enzymol Relat Areas Mol Biol. (2011) 77:61–108. doi: 10.1002/9780470920541.ch2
129. Pal R, Hameed S, Fatima Z. Altered drug efflux under iron deprivation unveils abrogated MmpL3 driven mycolic acid transport and fluidity in mycobacteria. Biometals. (2019) 32:49–63. doi: 10.1007/s10534-018-0157-8
130. Dailey HA, Gerdes S. HemQ: an iron-coproporphyrin oxidative decarboxylase for protoheme synthesis in firmicutes and actinobacteria. Arch Biochem Biophys. (2015) 574:27–35. doi: 10.1016/j.abb.2015.02.017
131. Yilmaz B, Li H. Gut microbiota and iron: the crucial actors in health and disease. Pharmaceuticals. (2018) 11:98. doi: 10.3390/ph11040098
132. Deugnier Y, Bardou-Jacquet É, Lainé F. Dysmetabolic iron overload syndrome (DIOS). Presse Med. (2017) 46(12 Pt 2):e306–11. doi: 10.1016/j.lpm.2017.05.036
133. Hooda J, Shah A, Zhang L. Heme, an essential nutrient from dietary proteins, critically impacts diverse physiological and pathological processes. Nutrients. (2014) 6:1080–102. doi: 10.3390/nu6031080
134. de Oliveira Otto MC, Alonso A, Lee DH, Delclos GL, Bertoni AG, Jiang R, et al. Dietary intakes of zinc and heme iron from red meat, but not from other sources, are associated with greater risk of metabolic syndrome and cardiovascular disease. J Nutr. (2012) 142:526–33. doi: 10.3945/jn.111.149781
135. Calarge CA, Murry DJ, Ziegler EE, Arnold LE. Serum ferritin, weight gain, disruptive behavior, and extrapyramidal symptoms in risperidone-treated youth. J Child Adolesc Psychopharmacol. (2016) 26:471–7. doi: 10.1089/cap.2015.0194
136. den Besten G, van Eunen K, Groen AK, Venema K, Reijngoud DJ, Bakker BM. The role of short-chain fatty acids in the interplay between diet, gut microbiota, and host energy metabolism. J Lipid Res. (2013) 54:2325–40. doi: 10.1194/jlr.R036012
137. Wang ML, Lin SH, Hou YY, Chen YH. Suppression of lipid accumulation by indole-3-carbinol is associated with increased expression of the aryl hydrocarbon receptor and CYP1B1 proteins in adipocytes and with decreased adipocyte-stimulated endothelial tube formation. Int J Mol Sci. (2016) 17:1256. doi: 10.3390/ijms17081256
138. Konopelski P, Konop M, Gawrys-Kopczynska M, Podsadni P, Szczepanska A, Ufnal M. Indole-3-propionic acid, a tryptophan-derived bacterial metabolite, reduces weight gain in rats. Nutrients. (2019) 11:591. doi: 10.3390/nu11030591
139. Lukić I, Getselter D, Ziv O, Oron O, Reuveni E, Koren O, et al. Antidepressants affect gut microbiota and ruminococcus flavefaciens is able to abolish their effects on depressive-like behavior. Transl Psychiatry. (2019) 9:133. doi: 10.1038/s41398-019-0466-x
140. Waclawiková B, El Aidy S. Role of microbiota and tryptophan metabolites in the remote effect of intestinal inflammation on brain and depression. Pharmaceuticals. (2018) 11:63. doi: 10.3390/ph11030063
141. Jin M, Lu J, Chen Z, Nguyen SH, Mao L, Li J, et al. Antidepressant fluoxetine induces multiple antibiotics resistance in Escherichia coli via ROS-mediated mutagenesis. Environ Int. (2018) 120:421–30. doi: 10.1016/j.envint.2018.07.046
142. Savini I, Catani MV, Evangelista D, Gasperi V, Avigliano L. Obesity-associated oxidative stress: strategies finalized to improve redox state. Int J Mol Sci. (2013) 14:10497–538. doi: 10.3390/ijms140510497
143. Gafoor R, Booth HP, Gulliford MC. Antidepressant utilisation and incidence of weight gain during 10 years' follow-up: population based cohort study. BMJ. (2018) 361:k1951. doi: 10.1136/bmj.k1951
144. Nehme H, Saulnier P, Ramadan AA, Cassisa V, Guillet C, Eveillard M, et al. Antibacterial activity of antipsychotic agents, their association with lipid nanocapsules and its impact on the properties of the nanocarriers and on antibacterial activity. PLoS ONE. (2018) 13:e0189950. doi: 10.1371/journal.pone.0189950
145. Grimsey EM, Piddoc LJ. Do phenothiazines possess antimicrobial and efflux inhibitory properties? FEMS Microbiol Rev. (2019) 43:577–90. doi: 10.1093/femsre/fuz017
146. Denis K, Le Bris M, Le Guennec L, Barnier JP, Faure C, Gouge A, et al. Targeting type IV pili as an antivirulence strategy against invasive meningococcal disease. Nat Microbiol. (2019) 4:972–84. doi: 10.1038/s41564-019-0395-8
147. Ogundeji AO, Pohl CH, Sebolai OM. The repurposing of anti-psychotic drugs, quetiapine and olanzapine, as anti-cryptococcus drugs. Front Microbiol. (2017) 8:815. doi: 10.3389/fmicb.2017.00815
148. Köck R, Werner P, Friedrich AW, Becker K, Prevalence of Multiresistant Microorganisms (PMM) Study Group. Persistence of nasal colonization with human pathogenic bacteria and associated antimicrobial resistance in the German general population. N Microbes N Infect. (2015) 9:24–34. doi: 10.1016/j.nmni.2015.11.004
149. Goldstein JL, Chan FK, Lanas A, Wilcox CM, Peura D, Sands GH, et al. Haemoglobin decreases in NSAID users over time: an analysis of two large outcome trials. Aliment Pharmacol Ther. (2011) 34:808–16. doi: 10.1111/j.1365-2036.2011.04790.x
150. Lam JR, Schneider JL, Quesenberry CP, Corley DA. Proton pump inhibitor and histamine-2 receptor antagonist use and iron deficiency. Gastroenterology. (2017) 152:821–29.e1. doi: 10.1053/j.gastro.2016.11.023
151. Strassnig M, Brar JS, Ganguli R. Dietary intake of patients with schizophrenia. Psychiatry. (2005) 2:31–35.
152. Tchaptchet S, Gumenscheimer M, Kalis C, Freudenberg N, Hölscher C, Kirschning, et al. TLR9-dependent and independent pathways drive activation of the immune system by Propionibacterium acnes. PLoS ONE. (2012) 7:e39155. doi: 10.1371/journal.pone.0039155
153. Khier S, Lohan L. Kinetics of circulating cell-free DNA for biomedical applications: critical appraisal of the literature. Future Sci OA. (2018) 4:FSO295. doi: 10.4155/fsoa-2017-0140
154. Magnusson M, Tobes R, Sancho J, Pareja E. Cutting edge: natural DNA repetitive extragenic sequences from gram-negative pathogens strongly stimulate TLR9. J Immunol. (2007) 179:31–5. doi: 10.4049/jimmunol.179.1.31
155. Kant R, de Vos WM, Palva A, Satokari R. Immunostimulatory CpG motifs in the genomes of gut bacteria and their role in human health and disease. J Med Microbiol. (2014) 63(Pt 2):293–308. doi: 10.1099/jmm.0.064220-0
156. Vora NL, Johnson KL, Basu S, Catalano PM, Hauguel-De Mouzon S, Bianchi DW. A multifactorial relationship exists between total circulating cell-free DNA levels and maternal BMI. Prenat Diagn. (2012) 32:912–14. doi: 10.1002/pd.3919
157. Maron JL, Johnson KL, Bianchi DW. Cell-free fetal DNA plasma extraction and real-time polymerase chain reaction quantification. Methods Mol Med. (2007) 132:51–63. doi: 10.1007/978-1-59745-298-4_6
158. Krieg AM. Therapeutic potential of Toll-like receptor 9 activation. Nat Rev Drug Discov. (2006) 5:471–84. doi: 10.1038/nrd2059
159. Cichota LC, Bochi GV, Tatsch E, Torbitz VD, Agnol PR, Zanardo JC, et al. Circulating double-stranded DNA in plasma of hemodialysis patients and its association with iron stores. Clin Lab. (2015) 61:985–90. doi: 10.7754/Clin.Lab.2015.141239
160. Yi C, Yang CG, He C. A non-heme iron-mediated chemical demethylation in DNA and RNA. Acc Chem Res. (2009) 42:519–29. doi: 10.1021/ar800178j
161. Yara S, Lavoie J-C, Levy E. Oxidative stress and DNA methylation regulation in the metabolic syndrome. Epigenomics. (2015) 7:283–300. doi: 10.2217/epi.14.84
162. Lee SH, Yun Y, Kim SJ, Lee E-J, Chang Y, Ryu S, et al. Association between cigarette smoking status and composition of gut microbiota: population-based cross-sectional study. J Clin Med. (2018) 7:282. doi: 10.3390/jcm7090282
163. Shin S, Wakabayashi J, Yates MS, Wakabayashi N, Dolan PM, Aja S, et al. Role of Nrf2 in prevention of high-fat diet-induced obesity by synthetic triterpenoid CDDO-imidazolide. Eur J Pharmacol. (2009) 620:138–44. doi: 10.1016/j.ejphar.2009.08.022
164. Liebisch G, Ecker J, Roth S, Schweizer S, Öttl V, Schött H-F, et al. Quantification of fecal short chain fatty acids by liquid chromatography tandem mass spectrometry-investigation of pre-analytic stability. Biomolecules. (2019) 9:121. doi: 10.3390/biom9040121
165. Darkoh C, Chappell C, Gonzales C, Okhuysen P. A rapid and specific method for the detection of indole in complex biological samples. Appl Environ Microbiol. (2015) 81:8093–7. doi: 10.1128/AEM.02787-15
166. Joseph J, Depp C, Shih PB, Cadenhead KS, Schmid-Schönbein G. Modified mediterranean diet for enrichment of short chain fatty acids: potential adjunctive therapeutic to target immune and metabolic dysfunction in schizophrenia? Front Neurosci. (2017) 11:155. doi: 10.3389/fnins.2017.00155
167. Slavin J. Fiber and prebiotics: mechanisms and health benefits. Nutrients. (2013) 5:1417–35. doi: 10.3390/nu5041417
168. Henderson DC, Borba CP, Daley TB, Boxill R, Nguyen DD, Culhane MA, et al. Dietary intake profile of patients with schizophrenia. Ann Clin Psychiatry. (2006) 18:99–105. doi: 10.1080/10401230600614538
169. Eaton SB, Eaton SB III, Konner MJ. Paleolithic nutrition revisited: a twelve-year retrospective on its nature and implications. Eur J Clin Nutr. (1997) 51:207–16. doi: 10.1038/sj.ejcn.1600389
170. Natan Z, Tsodikov F, Chernyak R, Gimelfarb Y. Nutritional assessment of inpatients with schizophrenia. Harefuah. (2014) 153:573–8, 625.
171. Tirosh A, Calay ES, Tuncman G, Claiborn KC, Inouye KE, Eguchi K, et al. The short-chain fatty acid propionate increases glucagon and FABP4 production, impairing insulin action in mice and humans. Sci Transl Med. (2019) 11:eaav0120. doi: 10.1126/scitranslmed.aav0120
172. Chambers ES, Byrne CS, Morrison DJ, Murphy KG, Preston T, Tedford C, et al. Dietary supplementation with inulin-propionate ester or inulin improves insulin sensitivity in adults with overweight and obesity with distinct effects on the gut microbiota, plasma metabolome and systemic inflammatory responses: a randomised cross-over trial. Gut. (2019) 68:1430–38. doi: 10.1136/gutjnl-2019-318424
173. Aspey K, Chambers E, Frost G. The acute effects of propionate on resting energy expenditure and fat oxidation in healthy human volunteers. Endocrine Abstracts. (2018) 53:207–216. doi: 10.1530/endoabs.53.P01
174. Azad MAK, Sarker M, Li T, Yin J. Probiotic species in the modulation of gut microbiota: an overview. Biomed Res Int. (2018) 2018:9478630. doi: 10.1155/2018/9478630
175. El Hage R, Hernandez-Sanabria E, Calatayud Arroyo M, Props R, Van de Wiele T. Propionate-producing consortium restores antibiotic-induced dysbiosis in a dynamic in vitro model of the human intestinal microbial ecosystem. Front Microbiol. (2019) 10:1206. doi: 10.3389/fmicb.2019.01206
176. Gauffin Cano P, Santacruz A, Moya Á, Sanz Y. Bacteroides uniformis CECT 7771 ameliorates metabolic and immunological dysfunction in mice with high-fat-diet induced obesity. PLoS ONE. (2012) 7:e41079. doi: 10.1371/journal.pone.0041079
177. Wrzosek L, Miquel S, Noordine ML, Bouet S, Chevalier-Curt MJ, Robert V, et al. Bacteroides thetaiotaomicron and Faecalibacterium prausnitzii influence the production of mucus glycans and the development of goblet cells in the colonic epithelium of a gnotobiotic model rodent. BMC Biol. (2013) 11:61. doi: 10.1186/1741-7007-11-61
178. Serena C, Ceperuelo-Mallafré V, Keiran N, Queipo-Ortuño ME, Bernal R, Gomez-Huelgas R, et al. Elevated circulating levels of succinate in human obesity are linked to specific gut microbiota. ISME J. (2018) 12:1642–57. doi: 10.1038/s41396-018-0068-2
179. Pereira M, Chen TD, Buang N, Olona A, Ko J-H, Maria Prendecki M, et al. Acute iron deprivation reprograms human macrophage metabolism and reduces inflammation in vivo. Cell Rep. (2019) 28:498–511.e5. doi: 10.1016/j.celrep.2019.06.039
180. Kim KO, Gluck M. Fecal microbiota transplantation: an update on clinical practice. Clin Endosc. (2019) 52:137–43. doi: 10.5946/ce.2019.009
181. Wilson BC, Vatanen T, Cutfield WS, O'Sullivan JM. The super-donor phenomenon in fecal microbiota transplantation. Front Cell Infect Microbiol. (2019) 9:2. doi: 10.3389/fcimb.2019.00002
182. Leshem A, Horesh N, Elinav E. Fecal microbial transplantation and its potential application in cardiometabolic syndrome. Front Immunol. (2019) 10:1341. doi: 10.3389/fimmu.2019.01341
183. Zhang Z, Mocanu V, Cai C, Dang J, Slater L, Deehan EC, et al. Impact of fecal microbiota transplantation on obesity and metabolic syndrome-a systematic review. Nutrients. (2019) 11:2291. doi: 10.3390/nu11102291
184. Göbel CH, Tronnier VM, Münte TF. Brain stimulation in obesity. Int J Obes. (2017) 41:1721–7. doi: 10.1038/ijo.2017.150
185. Ferrulli A, Macrì C, Terruzzi I, Ambrogi F, Milani V, Adamo M, et al. High frequency deep transcranial magnetic stimulation acutely increases β-endorphins in obese humans. Endocrine. (2019) 64:67–74. doi: 10.1007/s12020-018-1791-1
186. Apovian CM, Shah SN, Wolfe BM, Ikramuddin S, Miller CJ, Tweden KS, et al. Two-year outcomes of vagal nerve blocking (vBloc) for the treatment of obesity in the recharge trial. Obes Surg. (2017) 27:169–76. doi: 10.1007/s11695-016-2325-7
187. Yao G, Kang L, Li J, Long Y, Wei H, Ferreira CA, et al. Effective weight control via an implanted self-powered vagus nerve stimulation device. Nat Commun. (2018) 9:5349. doi: 10.1038/s41467-018-07764-z
188. de Lartigue G. Role of the vagus nerve in the development and treatment of diet-induced obesity. J Physiol. (2016) 594:5791–815. doi: 10.1113/JP271538
189. Bonaz B, Bazin T, Pellissier S. The vagus nerve at the interface of the microbiota-gut-brain axis. Front Neurosci. (2018) 12:49. doi: 10.3389/fnins.2018.00049
190. Sfera A, Cummings M, Osorio C. Non-neuronal acetylcholine: the missing link between sepsis, cancer, and delirium? Front Med. (2015) 2:56. doi: 10.3389/fmed.2015.00056
191. Wall R, Cryan JF, Ross RP, Fitzgerald GF, Dinan TG, Stanton C. Bacterial neuroactive compounds produced by psychobiotics. Adv Exp Med Biol. (2014) 817:221–39. doi: 10.1007/978-1-4939-0897-4_10
192. Yajima T, Inoue R, Matsumoto M, Yajima M. Non-neuronal release of ACh plays a key role in secretory response to luminal propionate in rat colon. J Physiol. (2011) 589(Pt 4):953–62. doi: 10.1113/jphysiol.2010.199976
193. Harris KK, Zopey M, Friedman TC. Metabolic effects of smoking cessation. Nat Rev Endocrinol. (2016) 12:299–308. doi: 10.1038/nrendo.2016.32
Keywords: obesity, psychotropic drugs, Bacteroidetes phylum, xenobiotic sensors, antimicrobials
Citation: Sfera A, Osorio C, Diaz EL, Maguire G and Cummings M (2020) The Other Obesity Epidemic—Of Drugs and Bugs. Front. Endocrinol. 11:488. doi: 10.3389/fendo.2020.00488
Received: 11 January 2020; Accepted: 22 June 2020;
Published: 31 July 2020.
Edited by:
Katherine Samaras, St Vincent's Hospital Sydney, AustraliaReviewed by:
Scott B. Teasdale, University of New South Wales, AustraliaHui Han, Chinese Academy of Sciences (CAS), China
Copyright © 2020 Sfera, Osorio, Diaz, Maguire and Cummings. This is an open-access article distributed under the terms of the Creative Commons Attribution License (CC BY). The use, distribution or reproduction in other forums is permitted, provided the original author(s) and the copyright owner(s) are credited and that the original publication in this journal is cited, in accordance with accepted academic practice. No use, distribution or reproduction is permitted which does not comply with these terms.
*Correspondence: Adonis Sfera, dr.sfera@gmail.com