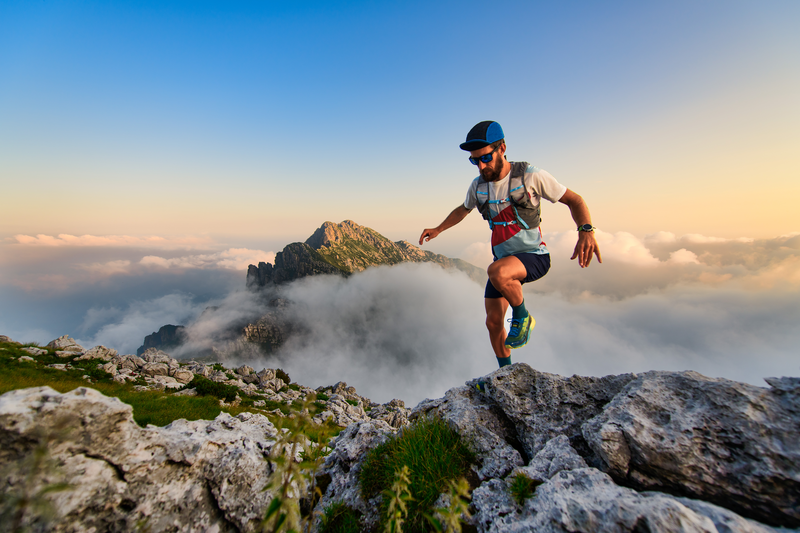
94% of researchers rate our articles as excellent or good
Learn more about the work of our research integrity team to safeguard the quality of each article we publish.
Find out more
REVIEW article
Front. Endocrinol. , 30 June 2020
Sec. Reproduction
Volume 11 - 2020 | https://doi.org/10.3389/fendo.2020.00373
This article is part of the Research Topic Bisphenols and Male Reproductive Health View all 10 articles
Over the last decades, the adverse effects of human exposure to the so-called “endocrine disruptors” have been a matter of scientific debate and public attention. Bisphenols are synthetic chemicals, widely used in the manufacture of hard plastic products. Bisphenol A (BPA) is one of the best-known environmental toxicants proven to alter the reproductive function in men and to cause other health problems. Consumer concern resulted in “BPA free” products and in the development of bisphenol analogs (BPA-A) to replace BPA in many applications. However, these other bisphenol derivatives seem to have effects similar to those of BPA. Although a number of reviews have summarized the effects of BPA on human reproduction, the purpose of this article is to review the effects of bisphenols on testicular steroidogenesis and to explore their mechanisms of action. Testicular steroidogenesis is a fine-regulated process, and its main product, testosterone (T), has a crucial role in fetal development and maturation and in adulthood for the maintenance of secondary sexual function and spermatogenesis. Contradictory outcomes of both human and animal studies on the effects of BPA on steroid hormone levels may be related to various factors that include study design, dosage of BPA used in in vitro studies, timing and route of exposure, and other confounding factors. We described the main possible molecular target of bisphenols on this complex pathway. We report that Leydig cells (LCs), the steroidogenic testicular component, are highly sensitive to BPA and several mechanisms concur to the functional impairment of these cells.
Over the last decades, the adverse effects of human exposure to the so-called “endocrine disruptors” have been a matter of deep debate by the scientific community and the layman. Particular attention has been paid to their toxicity on the reproductive function. Bisphenol A [2,2-bis(4-hydroxyphenyl)propane] (BPA) is among the most well-known endocrine disruptors proven capable of impairing the male reproductive function and to cause other health problems. BPA is an organic synthetic compound, including the group of dyphenylmenthane derivatives and bisphenols, widely used in the manufacture of hard plastic products. BPA has been used since the 1950s, in food packaging, industrial materials, dental sealants, personal hygiene products, and thermal receipts (1, 2). A significant exposure to BPA for children is given by toys, books, and feeding bottles (3, 4). BPA penetrates the body through the skin, inhalation, and the digestive system (5). Once adsorbed, BPA is then metabolized by the liver and excreted with the urine in 24 h (2). Despite the rapid metabolism, BPA can accumulate in different tissues (6). Consumer concern for BPA effects on health resulted in “BPA free” products and in the development of bisphenol analogs to replace BPA in many applications. However, these compounds seem to have endocrine disrupting capabilities similar to BPA and their impact on reproduction has been little investigated (7–9).
BPA seems to influence fetal testis development and predispose to the testicular dysgenesis syndrome (TDS). This syndrome may manifest itself not only at birth with cryptorchidism and hypospadias, but also in adulthood when it shows up with testicular tumors, hypogonadism, and/or infertility (10). Current evidence suggests that BPA can cause testicular histological abnormalities, which encompass dysregulated proliferation and apoptosis of Leydig cells (LCs) and alteration of steroidogenesis (11). In mice, pubertal exposure to high doses of BPA causes LC and germ cells apoptosis, resulting in underdeveloped testis with histopathological changes including atrophied seminiferous tubules, decreased number of late spermatids, and increased karyopyknotic cells (12). The reduction of testicular weight and the alteration of spermatogenesis persist till adulthood, long after the period of BPA exposure (12). The gestational period is a sensitive window of exposure to BPA. Male rats maternally exposed to BPA from gestation to the postnatal period have low testicular weight and testosterone (T) levels in the testicular interstitial fluid in adulthood (13). These effects may involve different molecular pathways discussed in section Bisphenol A Molecular Mechanisms of Action on Testicular Steroidogenesis.
Many studies have investigated the effects of BPA on human reproduction and extensive reviews have addressed the strength of the evidence on BPA toxicity (9, 10, 14, 15). Contradictory outcomes may depend on several factors including study design, BPA dose, timing, and route of exposure and other confounding factors (15). Several mechanisms of action have been described. First of all, BPA exhibits weak estrogenic and antiandrogenic proprieties. It binds to both estrogen receptors (ERs), ERα and ERβ (1, 10), and at high concentrations, BPA binds to the androgen receptor (AR) on which it acts as an antagonist (16). In addition to binding to the ARs, it disturbs the hypothalamic–pituitary–testicular axis and modulates gene expression and the enzymatic activity of testicular steroidogenesis (16). Furthermore, exposure to BPA is also associated with a decrease in the activity of the antioxidant system, resulting in increased oxidative stress, the most common cause of sperm damage (17, 18). Although several studies have supported the harmful effects of BPA on testicular function, its mechanism remains not fully understood.
The purpose of this article is to review the evidence on the relationship between bisphenols and testicular steroidogenesis, focusing on their mechanism(s) of action on LCs function.
The testis is a complex endocrine organ regulated by intra- and extra-testicular pathways that interact synergistically (19). LCs have a crucial role in the regulation of steroidogenesis and spermatogenesis. LCs produce testosterone (T), which has a main role in fetal development and maturation. During the masculinization programming window, the fetal testes begin to produce T, which allows male gonadal differentiation and development (20). Hence, T is necessary for the maintenance of secondary sexual function and spermatogenesis (21). Intratesticular T levels are approximately 100 times higher than the levels found in systemic circulation (22). The high local production rate of T implies the need for its intratesticular transport from LCs to Sertoli cells which nourish and support the development of the germinal cells during the various stages of spermatogenesis (23). LCs derive from mesenchymal cells located in the interstitial compartment of the testis. Their development occurs through three different stages during which they are called progenitor, immature, and adult LCs. Apoptosis seems to have a main role in maintaining a constant population of LCs, although other mechanisms may be involved (9).
LCs produce T in response to the luteinizing hormone (LH). LH binding to the LH receptors (LHR) on LCs activates Gs protein and adenylyl cyclase, increasing cAMP levels. cAMP acts as a key second messenger and upregulates the expression of genes related to the steroidogenesis (24). The steroidogenesis consists in a complex multi-enzyme process by which precursor cholesterol is converted to biologically active steroid hormones in a tissue-specific manner (Figure 1). Cholesterol can be synthesized in the endoplasmic reticulum but the first source of this precursor for steroidogenesis is via uptake of cholesteryl esters from high-density lipoprotein by the scavenger receptor SR-B1 (25). Therefore, SR-B1 has a key role for the maintenance of cholesterol balance. The first step in steroidogenesis takes place within mitochondria. The steroidogenic acute regulatory protein (StAR) mediates the transport of cholesterol from the outer to the inner mitochondrial membrane (26). The StAR-mediated transport of cholesterol is a crucial step for steroidogenesis (27, 28) and appropriate concentrations of cAMP are necessary for the regulation of StAR expression (29). However, cAMP/PKA is not the only pathway that regulates StAR expression. Other factors such as steroidogenic factor, activator protein, and cAMP-response element-binding protein are also associated with StAR regulation (30). Then, cholesterol is metabolized to pregnenolone into the smooth endoplasmic reticulum through a cascade of reactions that are catalyzed by the cytochrome P-450 proteins. Pregnenolone is then converted to T by 3β-hydroxysteroid dehydrogenase (3β-HSD), 17α-hydroxylase/17,20 lyase (CYP17A1), and 17β-hydroxysteroid dehydrogenase (17β-HSD). This complex process of steroidogenesis itself can be responsible for the increase of reactive oxygen species (ROS) (31). Thus, the normal products of steroidogenesis can act as pseudosubstrates and interact with P-450 enzymes, resulting in a pseudosubstrate–P-450–O2 complex, which is a source of dangerous free radicals (32).
Figure 1. Leydig cell steroidogenesis. LH binds to its receptors (LHR) on the Leydig cell (LC) membrane. This results in activation of Gs protein and adenylyl cyclase and increased concentration of intracellular cAMP. cAMP stimulates the mobilization and transport of cholesterol within the mitochondria in part by activating PKA and MAPK signaling. The first source of cholesterol for steroidogenesis is via uptake of cholesteryl esters from high-density lipoprotein (HDL) by the scavenger receptor SR-B1. Steroidogenic acute regulatory enzymes (StARs) regulate cholesterol transport from the outer to the inner mitochondrial membrane. At the inner mitochondrial membrane, cholesterol is converted into pregnenolone by CYP11A1 and pregnenolone is converted into testosterone by enzymes in the smooth endoplasmic reticulum (3β-HSD, CYP17A1, and 17β-HSD).
Experimental studies in male animals have shown that exposure to BPA is associated with altered hormone levels suggesting direct effects of BPA on LCs. However, these data are discordant. Low-dose BPA decreased T levels in CD-1 mice exposed during perinatal and postnatal periods (33), but not in adult C57BL/6 mice exposed in utero (34). In addition, low-dose BPA lowered T levels in Holtzman rats exposed during gestation or in the neonatal age (35, 36) and albino (37) and Wistar (38) rats exposed in adulthood. In contrast, by examining the gestational and neonatal exposure of low-dose BPA in Long–Evans (39) or Sprague–Dawley (SD) rats (40, 41), the levels of T did not change. Treatment with increasing concentrations of BPA (1 to 1,000 nM) did not significantly lower basal or hCG-stimulated T secretion by primary culture of LCs of young adult male rats (42). However, although Sánchez et al. reported that low-dose BPA did not decrease T levels in Wistar rats, dihydrotestosterone levels decreased (43). Gamez et al. reported that exposure to low-dose BPA led to an increase in serum LH and FSH levels in young Wistar rats (44). Nevertheless, another study in adult Wistar rats showed that exposure to BPA decreased serum T, LH, and FSH levels, but increased the levels of 17β-estradiol (E2) (45). In two studies in SD rats, postnatal exposure to low-dose BPA decreased serum T and E2 levels (46). BPA exposure lowered T levels in Swiss albino and C57BL/6 mice, but at variable dosage between 0.5 μg/kg and 100 mg/kg (47, 48). Sadowski et al. described a decrease in FSH concentrations in Long–Evans rats at weaning, after exposure to BPA at both 4 and 400 μg/kg/day (49). An in vitro study conducted on fetal testes explanted from mice, rats, and humans demonstrated that exposure to 10 nM of BPA was enough to decrease basal T secretion in human fetal testes, but higher concentrations were required in rats and mice (10 and 1 μM, respectively) (50).
The epidemiological studies evaluating the effects of BPA exposure on serum hormone levels in men have also shown conflicting results. In the INChianti adult population study, Galloway et al. found a correlation between higher urinary BPA concentrations and higher serum T, but not E2 levels in 307 Italian men living in Chianti, Italy (51). Another study, conducted on 308 young men from Denmark's general population, reported that higher urinary BPA concentration was associated with a significant increase of LH, T, and E2 levels (52). In contrast, in a cross-sectional study of 290 men, Zhou et al. found that increased serum BPA concentrations were statistically significantly associated with the reduction of androstenedione, free T and free androgen index (FAI) levels, and with the increase of sex hormone-binding globulin (SHBG) levels (53). Two cross-sectional studies, respectively, of 167 and 302 men, did not report any associations between BPA and T concentrations (54, 55). According to Meeker and colleagues, men with elevated urinary BPA concentrations had higher FSH and lower inhibin B levels with a higher FSH/inhibin B ratio and a lower E2/T ratio (54). Mendiola et al. found that higher urinary BPA levels were associated with lower FAI and FAI/LH and free T/LH ratios in fertile men (55). Two cross-sectional studies reported that urinary BPA levels were associated with higher SHBG in men occupationally exposed to BPA (56, 57). The NHANES 2011-2012 study showed an inverse correlation between urinary BPA levels and serum T concentrations in male adolescents (58). However, a retrospective cohort study did not find any effects on hormone levels in boys aged 8 to 14 years after prenatal or childhood exposure to BPA (59).
Although these results are controversial, they suggest that BPA alters steroid hormones pathways in men.
Although both animal and human studies support the harmful effects of BPA on steroid hormones, the mechanism of action of BPA in negatively interfering with testicular steroidogenesis remains unclear. Since LCs are the site of testicular steroidogenesis, several studies have been conducted on these cells to investigate the effects of BPA. In Wistar/ST pubertal rats, continuous exposure to BPA at high doses reduced the number of LCs and the expression of steroidogenic enzymes in these cells (60). In contrast, Long–Evans rats exposed to a low dose of BPA during gestation and at birth had an increase in the number of LCs in adulthood through the upregulation of mitogen factors. However, although a low dose of BPA increased LC proliferation, the expression of steroidogenic enzymes and T biosynthesis decreased (61). Chen et al. reported that BPA did not stimulate staminal LC proliferation, but it induced the differentiation of stem LCs into more mature LCs. They used an in vivo ethane dimethane sulfonate (EDS)-induced LC regeneration model to mimic the pubertal development of LCs. They treated rats with EDS to eliminate LCs and then they injected BPA within the testis. The intratesticular injection of BPA avoided possible interference of hypothalamus and pituitary. The results of this study showed that BPA significantly increased the number of 11β-HSD1-positive cells, which is a biomarker for LCs at an advanced stage. Thus, BPA promoted the differentiation of staminal LCs, increasing T production and upregulating LC-specific genes (LHCGR, StAR, CYP11A1, HSD3B1, CYP17A1, HSD17B3, and HSD11B1). These findings suggest a possible role of BPA in sexual precocious puberty in males (62). Exposure to high doses of BPA (480 and 960 mg/kg/day at postnatal days 31–44) has been reported to induce apoptosis in Leydig and germ cells via the upregulation of Fas, FasL, and caspase-3 (12). The apoptosis of LCs was associated with a decreased testicular testis weight and histopathological changes, which persisted into adulthood (12). In another study, Thuillier et al. reported that SD rats exposed in utero to BPA had an increase number of LCs but did not present significant change in serum T levels (63). Moreover, BPA can also induce Nur77 gene expression, an orphan nuclear receptor that plays an important role in the regulation of LH-mediated steroidogenesis, altering LC steroidogenesis (64). BPA induced Nur77 gene expression via PKA and MAPK signaling pathways in a time- and dose-dependent manner. BPA-mediated Nur77 expression resulted in the upregulation of steroidogenesis both in vitro and in vivo, with a significant increase of T synthesis (two-fold) (64).
The inhibition of testicular steroidogenesis by BPA can also be associated with a decreased LH secretion. Akingbemi et al. reported that Long–Evans rats exposed to low doses of BPA (2.4 μg/kg/day) from postnatal days 21–35, decreased both serum LH and T levels, downregulating pituitary LHβ expression but increasing ERβ pituitary mRNA levels (13).
The expression of LH and FSH receptors may also be altered by BPA. Li et al. showed that treatment of adult male zebrafish (Danio rerio) by 500 ng/L BPA for 7 weeks downregulated the expressions of FSHr and LHCGr (65). For the first time, Roelofs et al. demonstrated that BPA, BPF, and TBBPA showed clear glucocorticoid receptor antagonism, other than AR antagonism. They also found that bisphenol analogs upregulated the 5αRed1 gene expression, suggesting a redirection of steroidogenesis, which may have significant consequences for fetal testis development and function (7).
Within the steroid hormone biosynthetic pathway, steroidogenic enzymes are recognized as important targets for the actions of endocrine-disrupting chemicals. Several studies showed that BPA decreases the expression of steroidogenic enzymes (33, 41, 60, 61, 66, 67). Moreover, some compounds, including BPA, seem to disturb steroidogenesis by inhibiting the cAMP pathway. Nikula et al. analyzed the effects of BPA at micromolar concentration in cultured mouse Leydig tumor cells (mLTC-1). BPA did not have any effects on hCG binding to LH receptors, but it inhibited LH-receptor-mediated signal transduction by decreasing hCG-stimulated cAMP. Specifically, they found that after preincubation of mLTC-1 cells for 48 h with different doses of BPA, hCG-stimulated cAMP and progesterone production was inhibited. Whereas, preincubation with 17β-estradiol inhibited progesterone production but had no effect on cAMP. Thus, the effects of BPA did not seem to be estrogen-related (68). Moreover, the inhibitory effect of BPA could not be seen when cAMP formation was directly stimulated by forskolin (Fk) or through Gs protein by cholera toxin (CT), and when steroidogenesis was directly activated by 8-Br-cAMP, which can penetrate the plasma membranes and directly activate the protein kinase A. These results suggested that the negative effect of BPA is exerted between the LH receptor and the adenylate cyclase. Accordingly, Feng et al. found that BPA exposure inhibited progesterone secretion in hCG-stimulated mouse Leydig tumor cell line (mLTC-1) by decreasing SR-B1 and P450scc expression due to the adverse effects on cAMP. Moreover, lower SR-B1 levels cause a reduction in cholesterol levels within LCs that alters steroidogenesis (69). The role of StAR is instead controversial. According to Feng et al. (69), StAR seems not be the molecular target of BPA. Similarly, male rats exposed to BPA showed decreased T levels but did not exhibit significant changes in StAR expression (61). However, other previous studies have reported that BPA decreased StAR expression in cell culture in vitro (15, 33, 47), but, in contrast, other studies have shown that StAR expression is upregulated (41, 65). Takamiya et al. reported that StAR gene expression increased in the presence of both hCG (10 μg/L) plus BPA (10−5 M) or by hCG alone, but was not influenced by BPA alone. They found that BPA had only a weak modulating effect on gene expression of hCG-stimulated mLTC-1 cells (70). Li et al. showed that the exposure of adult male zebrafish to low doses (0.22–2.2 nM) of BPA for 7 weeks resulted in abnormal expression of genes involved in testicular steroidogenesis, specifically of 3β-HSD1, CYP17A1, and CYP11C1 (65). Samova et al. found that BPA significantly and dose-dependently affected the functions of 3β-HSD and 17β-HSD in the testis of inbred Swiss strain male albino mice (67). Ye et al. reported that BPA significantly inhibited 3β-HSD, CYP17A1, and 17β-HSD3 activities in both human and rat testis. However, the inhibition of 17β-HSD3 activity was much weaker compared with that on the other two enzymes. They also found that human enzymes were more sensitive to BPA (71). Specifically, their results suggested that BPA did not exert a competitive inhibition of 3β-HSD against its substrate (pregnenolone), but it competed with the cofactor NAD+ in the cofactor binding site of the enzyme, whereas BPA inhibition of CYP17A1 was mixed type for enzyme substrate progesterone, indicating a combination of two different types of reversible enzyme inhibition, both competitive and uncompetitive (71). Additionally, not only BPA, but also bisphenol S (BPS) and bisphenol F (BPF) exposure decreased T production in fetal mouse testis by inhibiting mRNA expression of StAR, 3β-HSD, and cytochrome P45017A1 (CYP17A1), but not of P450scc (72). Moreover, Dankers et al. suggested that the changes in T secretion after BPA or TBBPA exposure were only partly due to alterations of steroidogenic enzyme expression. These authors hypothesized that the inhibition of ATP-binding cassette (ABC) transporters, expressed in the blood–testis barrier (BTB), may play a role in this process. The BTB divides the seminiferous epithelium into a basal and an apical compartment and provides structural and protective support for the differentiation of spermatogonia into spermatocytes. It consists of tight junctions, testis-specific atypical adherent junctions, desmosomes, and gap junctions. In the active part of BTB, ABC transporters are present to allow the passage of endogenous molecules involved in cellular signaling and to block the passage of dangerous compounds within the testes and to protect germ cells. The cellular membranes of LCs, Sertoli cells, and capillary endothelial cells are provided of these transporters. For this reason, the association between endocrine disruptors and ABC transporters has a strong toxicological impact (23). The breast cancer resistance protein (BCRP/ABCG2), the P-glycoprotein (P-gp/ABCB1), and the multidrug resistance proteins 1 and 4 (MRP1, 4/ABCC1,4) are the major efflux transporters in the BTB with a differential expression in the various parts of the BTB (23). LCs express P-gp, MRP1, and MRP4, but not BCRP in adult human testis (73, 74). Dankers et al. investigated the effects of BPA and of TBBPA (tetrabromobisphenol A) on BCRP, MRP1, MRP4, and P-gp. They found that TBBPA inhibited all these transporters; thus, it is considered a non-competitive transporter inhibitor, whereas BPA inhibited only BCRP activity. They also showed that BPA, but not TBBPA, is transported by BCRP (23). Interestingly, they found that, although exposure to BPA and TBBPA significantly increased T level in MA-10 cells, the effects on steroidogenic genes were not so significant. Thus, these authors hypothesized that the changes in T levels upon BPA or TBBPA exposure were associated with the inhibition of efflux of T precursors. Increased availability of these precursors, such as androstenedione or DHEA, could be responsible for the increased T levels found.
Moreover, many compounds increase the levels of ROS in the testis, altering steroidogenesis. Oxidative stress has also been found to induce apoptosis in LCs and germ cells (64). Recent studies have reported an inverse relationship between NOS activity and StAR expression (47). Chouhan et al. exposed Swiss albino mice to BPA at concentrations of 0.5, 50, and 100 μg/kg body weight/day intraperitoneally for 60 days. They showed that BPA upregulated the expression of iNOS, downregulating the expression of StAR in mouse testis (47). It was also supposed that BPA impaired steroidogenesis by decreasing testicular glucose levels (38). Glucose homeostasis is crucial for testicular spermatogenesis and steroidogenesis. D'Cruz et al. reported that low-dose BPA exposure impaired insulin signaling interacting with GLUT-2 and GLUT-8 and inhibiting the uptake in the testis (38).
Recently, a number of studies suggest epigenetic effects of BPA, including DNA methylation, histone modifications, and non-coding RNAs. Epigenetic mechanisms can have long-term effects and may be transmitted across several generations (75). Specifically, Gao et al. (76) have recently investigated the epigenetic effects of BPA on the expression of non-coding RNAs (e.g., microRNAs) in the regulation of testicular steroidogenesis. They used both cell culture and in vivo mouse models and showed that miR-146a-5p was expressed only in LCs, and this expression was significantly induced by BPA. Consequently, the high miR-146a-5p expression intensifies the negative effects of BPA on testicular steroidogenesis by directly targeting the 3′ UTR of Mta3 gene (76). Mta3 is a subunit of the Mi-2/nucleosome remodeling and deacetylase (NuRD) protein complex that is exclusively expressed in LCs (77). Specifically, Mta3 role in the control of testicular steroidogenic function is proven by its negative regulation by the high levels of circulated insulin (77). He et al. showed that a deficiency of Mta3 in LCs of diabetic mice was associated with low serum T level, indicating that Mta3 expression in LCs may be associated with androgen deficiency (77). Thus, the downregulation of mir-146a-5p/Mta3 cascade seems to be involved in steroidogenic alterations caused by BPA (76).
DNA methylation is one of the best characterized epigenetic mechanisms. Liu et al. investigated the effects of BPA on DNA methylation in rare minnow Gobiocypris rarus. DNA hypermethylation consists of an addition of a methyl group to the cytosine bases of DNA to form 5-methylcytosine and it may be associated with changes in gene expression. In their study, Liu et al. found that the global DNA methylation level was significantly increased in testis of male G. rarus exposed to BPA for 7 days. Then, they specifically analyzed the change in DNA methylation in the 5′ flanking region of the cytochrome P450 aromatase (CYP19A1A) gene. After 35-day exposure, the DNA methylation levels of CYP19A1A did not have significant change in the testis, whereas they significantly increased in the ovary (78).
This review summarizes the current evidences on the association between BPA and testicular steroidogenesis. Altogether, these results show that LCs are very sensitive to BPA and that several mechanisms concur to the functional impairment of these cells. Testicular steroidogenesis is a complex and fine-regulated process and each component of this pathway may be the molecular target of BPA. The main possible sites of BPA action are summarized in Figure 2. The conflicting results of both human and animal studies may be related to various factors that include study design, dose of BPA, timing and route of exposure, and other confounding factors. This review confirms that the widespread use of bisphenols is certainly dangerous for testicular development and function and that a reduction of its use is necessary to preserve male sexual and reproductive health.
Figure 2. Mechanisms of action of bisphenol A on testicular steroidogenesis. Testicular steroidogenesis is a complex and fine-regulated process that bisphenol A (BPA) can perturb by acting with several mechanisms represented in this figure (circled in red).
FB, SL, and AC: concept and design. RAC, LM, and RC: articles research. FB: writing of the original draft. SL, AC, and AA: final approval. All authors contributed to the article and approved the submitted version.
The publication costs of this article are partially supported by the 2020–2022 grant Linea Open Access, University of Catania, Catania, Italy.
The authors declare that the research was conducted in the absence of any commercial or financial relationships that could be construed as a potential conflict of interest.
1. Rochester JR. Bisphenol a and human health: a review of the literature. Reprod Toxicol. (2013) 42:132–55. doi: 10.1016/j.reprotox.2013.08.008
2. Huo X, Chen D, He Y, Zhu W, Zhou W, Zhang J. Bisphenol-A and female infertility: a possible role of gene-environment interactions. Int J Environ Res Public Health. (2015) 12:11101–16. doi: 10.3390/ijerph120911101
3. Brede C, Fjeldal P, Skjevrak I, Herikstad H. Increased migration levels of bisphenol a from polycarbonate baby bottles after dishwashing, boiling and brushing. Food Addit Contam. (2003) 20:684–9. doi: 10.1080/0265203031000119061
4. Sajiki J, Yanagibori R, Kobayashi Y. Study of experiment on leaching of bisphenol a from infant books to artificial saliva. Nippon Eiseigaku Zasshi. (2010) 65:467–70. doi: 10.1265/jjh.65.467
5. Kang JH, Kondo F, Katayama Y. Human exposure to bisphenol A. Toxicology. (2006) 226:79–89. doi: 10.1016/j.tox.2006.06.009
6. Komarowska M, Hermanowicz A, Czyzewska U, Milewski R, Matuszczak E, Miltyk W, et al. Serum bisphenol a level in boys with cryptorchidism: a step to male infertility? Int J Endocrinol. (2015) 2015:973154. doi: 10.1155/2015/973154
7. Roelofs MJ, van den Berg M, Bovee TF, Piersma AH, van Duursen MB. Structural bisphenol analogues differentially target steroidogenesis in murine MA-10 leydig cells as well as the glucocorticoid receptor. Toxicology. (2015) 329:10–20. doi: 10.1016/j.tox.2015.01.003
8. Rochester JR, Bolden AL. Bisphenol S and F: a systematic review and comparison of the hormonal activity of bisphenol a substitutes. Environ Health Perspect. (2015) 123:643–50. doi: 10.1289/ehp.1408989
9. Siracusa JS, Yin L, Measel F, Liang S, Yu X. Effects of bisphenol a and its analogs on reproductive health: a mini review. Reprod Toxicol. (2018) 79:96–123. doi: 10.1016/j.reprotox.2018.06.005
10. Matuszczak E, Komarowska MD, Debek W, Hermanowicz A. The impact of bisphenol a on fertility, reproductive system, and development: a review of the literature. Int J Endocrinol. (2019) 2019:4068717. doi: 10.1155/2019/4068717
11. Williams C, Maria B, Dimitry KN, Cory T. Gestational bisphenol a exposure and testis development. Endocr Disruptors. (2014) 2:e29088. doi: 10.4161/endo.29088
12. Li Y, Song T, Cai Y, Zhou J, Song X, Zhao X, et al. Bisphenol a exposure induces apoptosis and upregulation of Fas/FasL and caspase-3 expression in the testes of mice. Toxicol Sci. (2009) 108:427–36. doi: 10.1093/toxsci/kfp024
13. Akingbemi Benson T, Sottas Chantal M, Koulova Anna I, Klinefelter Gary R, Hardy Matthew P. Inhibition of testicular steroidogenesis by the xenoestrogen bisphenol A is associated with reduced pituitary luteinizing hormone secretion and decreased steroidogenic enzyme gene expression in rat leydig cells. Endocrinology. (2004) 145:592–603. doi: 10.1210/en.2003-1174
14. Vom Saal FS, Akingbemi BT, Belcher SM, Birnbaum LS, Crain DA, Eriksen M, et al. Chapel hill bisphenol a expert panel consensus statement: integration of mechanisms, effects in animals and potential to impact human health at current levels of exposure. Reprod Toxicol. (2007) 24:131–8. doi: 10.1016/j.reprotox.2007.07.005
15. Peretz J, Vrooman L, Ricke WA, Hunt PA, Ehrlich S, Hauser R, et al. Bisphenol a and reproductive health: update of experimental and human evidence, 2007–2013. Environ Health Perspect. (2014) 122:775–86. doi: 10.1289/ehp.1307728
16. Hejmej A, Kotula-Balak M, Bilinsk B. Antiandrogenic and estrogenic compounds: effect on development and function of male reproductive system. In: Abduljabbar H, editor. Steroids – Clinical Aspect. London, UK: IntechOpen (2011). p. 57–9. doi: 10.5772/28538
17. Wang P, Luo C, Li Q, Chen S, Hu Y. Mitochondrion-mediated apoptosis is involved in reproductive damage caused by BPA in male rats. Environ Toxicol Pharmacol. (2014) 38:1025–33. doi: 10.1016/j.etap.2014.10.018
18. Francesco LM, Sandro LV, Enzo V, Aldo CE. Oxidative stress and medical antioxidant treatment in male infertility. Reprod Biomed Online. (2009) 19:638–59. doi: 10.1016/j.rbmo.2009.09.014
19. Tena-Sempere M, Barreiro ML, González LC, Gaytán F, Zhang FP, Caminos JE, et al. Novel expression and functional role of ghrelin in rat testis. Endocrinology. (2002) 143:717–25. doi: 10.1210/endo.143.2.8646
20. Scott HM, Mason JI, Sharpe RM. Steroidogenesis in the fetal testis and its susceptibility to disruption by exogenous compounds. Endocr Rev. (2009) 30:883–925. doi: 10.1210/er.2009-0016
21. Mathur PP, D'Cruz SC. The effect of environmental contaminants on testicular function. Asian J Androl. (2011) 13:585–91. doi: 10.1038/aja.2011.40
22. Ochsenkühn R, De Kretser DM. The contributions of deficient androgen action in spermatogenic disorders. Int J Androl. (2003) 26:195–201. doi: 10.1046/j.1365-2605.2003.00381.x
23. Dankers AC, Roelofs MJ, Piersma AH, Sweep FC, Russel FG, van den Berg M, et al. Endocrine disruptors differentially target ATP-binding cassette transporters in the blood-testis barrier and affect leydig cell testosterone secretion in vitro. Toxicol Sci. (2013) 136:382–91. doi: 10.1093/toxsci/kft198
24. Dufau ML. Endocrine regulation and communicating functions of the leydig cell. Annu Rev Physiol. (1988) 50:483–508. doi: 10.1146/annurev.ph.50.030188.002411
25. Shen WJ, Azhar S, Kraemer FB. Lipid droplets and steroidogenic cells. Exp Cell Res. (2016) 340:209–14. doi: 10.1016/j.yexcr.2015.11.024
26. Devoto L, Kohen P, Vega M, Castro O, González RR, Retamales I, et al. Control of human luteal steroidogenesis. Mol Cell Endocrinol. (2002) 186:137–41. doi: 10.1016/S0303-7207(01)00654-2
27. Stocco DM, Clark BJ. Regulation of the acute production of steroids in steroidogenic cells. Endocr Rev. (1996) 17:221–44. doi: 10.1210/er.17.3.221
28. Hasegawa T, Zhao L, Caron KM, Majdic G, Suzuki T, Shizawa S, et al. Developmental roles of the steroidogenic acute regulatory protein (StAR) as revealed by StAR knockout mice. Mol Endocrinol. (2000) 14:1462–71. doi: 10.1210/mend.14.9.0515
29. Stocco DM, Clark BJ. The role of the steroidogenic acute regulatory protein in steroidogenesis. Steroids. (1997) 62:29–36. doi: 10.1016/S0039-128X(96)00155-9
30. Stocco DM, Wang XJ, Jo Y, Manna PR. Multiple signaling pathways regulating steroidogenesis and steroidogenic acute regulatory protein expression: more complicated than we thought. Mol Endocrinol. (2005) 19:2647–59. doi: 10.1210/me.2004-0532
31. Hanukoglu I. Antioxidant protective mechanisms against reactive oxygen species (ROS) generated by mitochondrial P450 systems in steroidogenic cells. Drug Metab Rev. (2006) 38:171–96. doi: 10.1080/03602530600570040
32. Quinn PG, Payne AH. Steroid product-induced, oxygen-mediated damage of microsomal cytochrome P-450 enzymes in leydig cell cultures. Relationship to desensitization. J Biol Chem. (1985) 260:2092–9.
33. Xi W, Lee CKF, Yeung WSB, Giesy JP, Wong MH, Zhang X, et al. Effect of perinatal and postnatal bisphenol a exposure to the regulatory circuits at the hypothalamus-pituitary-gonadal axis of CD-1 mice. Reprod Toxicol. (2011) 31:409–17. doi: 10.1016/j.reprotox.2010.12.002
34. La Rocca J, Boyajian A, Brown C, Smith SD, Hixon M. Effects of in utero exposure to bisphenol a or diethylstilbestrol on the adult male reproductive system. Birth Defects Res B Dev Reprod Toxicol. (2011) 92:526–33. doi: 10.1002/bdrb.20336
35. Salian S, Doshi T, Vanage G. Neonatal exposure of male rats to bisphenol a impairs fertility and expression of sertoli cell junctional proteins in the testis. Toxicology. (2009) 265:56–67. doi: 10.1016/j.tox.2009.09.012
36. Salian S, Doshi T, Vanage G. Perinatal exposure of rats to bisphenol a affects the fertility of male offspring. Life Sci. (2009) 85:742–52. doi: 10.1016/j.lfs.2009.10.004
37. El-Beshbishy HA, Aly HAA, El-Shafey M. Lipoic acid mitigates bisphenol a-induced testicular mitochondrial toxicity in rats. Toxicol Ind Health. (2013) 29:875–87. doi: 10.1177/0748233712446728
38. D'Cruz SC, Jubendradass R, Jayakanthan M, Rani SJA, Mathur PP. Bisphenol a impairs insulin signaling and glucose homeostasis and decreases steroidogenesis in rat testis: an in vivo and in silico study. Food Chem Toxicol. (2012) 50:1124–33. doi: 10.1016/j.fct.2011.11.041
39. Howdeshell KL, Furr J, Lambright CR, Wilson VS, Ryan BC, Gray LE. Gestational and Lactational Exposure to Ethinyl Estradiol, but not Bisphenol A Decreases Androgen-Dependent Reproductive Organ Weights and Epididymal Sperm Abundance in the Male Long Evans Hooded Rat. Oxford University Press (2008) 371. doi: 10.1093/toxsci/kfm306
40. Kobayashi K, Kubota H, Ohtani K, Hojo R, Miyagawa M. Lack of effects for dietary exposure of bisphenol A during in utero and lactational periods on reproductive development in rat offspring. J Toxicol Sci. (2012) 37:565–73. doi: 10.2131/jts.37.565
41. Qiu L-L, Wang X, Zhang X-h, Zhang Z, Gu J, Liu L, et al. Decreased androgen receptor expression may contribute to spermatogenesis failure in rats exposed to low concentration of bisphenol A. Toxicol Lett. (2013) 219:116–24. doi: 10.1016/j.toxlet.2013.03.011
42. Murono EP, Derk RC, de León JH. Differential effects of octylphenol, 17beta-estradiol, endosulfan, or bisphenol a on the steroidogenic competence of cultured adult rat leydig cells. Reprod Toxicol. (2001) 15:551–60. doi: 10.1016/S0890-6238(01)00158-7
43. Sánchez P, Castro B, Torres JM, Olmo A, del Moral RG, Ortega E. Bisphenol a modifies the regulation exerted by testosterone on 5 α -reductase isozymes in ventral prostate of adult rats. Biomed Res Int. (2013) 2013:629235. doi: 10.1155/2013/629235
44. Gamez JM, Penalba R, Cardoso N, Ponzo O, Carbone S, Pandolfi M, et al. Low dose of bisphenol a impairs the reproductive axis of prepuberal male rats. J Physiol Biochem. (2014) 70:239–46. doi: 10.1007/s13105-013-0298-8
45. Wisniewski P, Romano RM, Kizys MML, Oliveira KC, Kasamatsu T, Giannocco G, et al. Adult exposure to bisphenol a (BPA) in wistar rats reduces sperm quality with disruption of the hypothalamic-pituitary-testicular axis. Toxicology. (2015) 329:1–9. doi: 10.1016/j.tox.2015.01.002
46. Gurmeet KSS, Rosnah I, Normadiah MK, Das S, Mustafa AM. Detrimental effects of bisphenol a on development and functions of the male reproductive system in experimental rats. Excli J. (2014) 13:151–60.
47. Chouhan S, Yadav SK, Prakash J, Westfall S, Ghosh A, Agarwal NK, et al. Increase in the expression of inducible nitric oxide synthase on exposure to bisphenol a: a possible cause for decline in steroidogenesis in male mice. Environ Toxicol Phar. (2015) 39:405–16. doi: 10.1016/j.etap.2014.09.014
48. Zang Z, Ji S, Xia T, Huang S. Effects of bisphenol a on testosterone levels and sexual behaviors of male mice. Adv Sex Med. (2016) 6:41–9. doi: 10.4236/asm.2016.64006
49. Sadowski RN, Park P, Neese SL, Ferguson DC, Schantz SL, Juraska JM. Effects of perinatal bisphenol a exposure during early development on radial arm maze behavior in adult male and female rats. Neurotoxicol Teratol. (2014) 42:17–24. doi: 10.1016/j.ntt.2014.01.002
50. N'Tumba-Byn T, Moison D, Lacroix M, Lecureuil C, Lesage L, Prud'homme SM, et al. Differential effects of bisphenol a and diethylstilbestrol on human, rat and mouse fetal leydig cell function. PLoS ONE. (2012) 7:e51579. doi: 10.1371/journal.pone.0051579
51. Galloway T, Cipelli R, Guralnik J, Ferrucci L, Bandinelli S, Corsi AM, et al. Daily bisphenol a excretion and associations with sex hormone concentrations: results from the InCHIANTI adult population study. Environ Health Perspect. (2010) 118:1603–8. doi: 10.1289/ehp.1002367
52. Lassen TH, Frederiksen H, Jensen TK, Petersen JH, Joensen UN, Main KM, et al. Urinary bisphenol a levels in young men: association with reproductive hormones and semen quality. Environ Health Persp. (2014) 122:478–84. doi: 10.1289/ehp.1307309
53. Zhou Q, Miao M, Ran M, Ding L, Bai L, Wu T, et al. Serum bisphenol-A concentration and sex hormone levels in men. Fertil Steril. (2013) 100:478–82. doi: 10.1016/j.fertnstert.2013.04.017
54. Meeker JD, Calafat AM, Hauser R. Urinary bisphenol a concentrations in relation to serum thyroid and reproductive hormone levels in men from an infertility clinic. Environ Sci Technol. (2010) 44:1458–63. doi: 10.1021/es9028292
55. Mendiola J, Jørgensen N, Andersson AM, Calafat AM, Ye X, Redmon JB, et al. Are environmental levels of bisphenol a associated with reproductive function in fertile men? Environ. Health Perspect. (2010) 118:1286–91. doi: 10.1289/ehp.1002037
56. Liu XQ, Miao MH, Zhou ZJ, Gao ES, Chen JP, Wang JT, et al. Exposure to bisphenol-A and reproductive hormones among male adults. Environ Toxicol Phar. (2015) 39:934–41. doi: 10.1016/j.etap.2015.03.007
57. Zhuang WL, Wu KS, Wang YK, Zhu HJ, Deng ZZ, Peng L, et al. Association of serum bisphenol-A concentration and male reproductive function among exposed workers. Arch Environ Contam Toxicol. (2015) 68:38–45. doi: 10.1007/s00244-014-0078-7
58. Scinicariello F, Buser MC. Serum testosterone concentrations and urinary bisphenol a benzophenone-3, triclosan, and paraben levels in male and female children and adolescents: NHANES 2011-2012. Environ Health Perspect. (2016) 124:1898–904. doi: 10.1289/EHP150
59. Ferguson KK, Peterson KE, Lee JM, Mercado-García A, Blank-Goldenberg C, Téllez-Rojo MM, et al. Prenatal and peripubertal phthalates and bisphenol a in relation to sex hormones and puberty in boys. Reprod Toxicol. (2014) 47:70–6. doi: 10.1016/j.reprotox.2014.06.002
60. Nakamura D, Yanagiba Y, Duan Z, Ito Y, Okamura A, Asaeda N, et al. Bisphenol a may cause testosterone reduction by adversely affecting both testis and pituitary systems similar to estradiol. Toxicol Lett. (2010) 194:16–25. doi: 10.1016/j.toxlet.2010.02.002
61. Nanjappa MK, Simon L, Akingbemi BT. The industrial chemical bisphenol a (BPA) interferes with proliferative activity and development of steroidogenic capacity in rat leydig cells. Biol Reprod. (2012) 86:135, 1–12. doi: 10.1095/biolreprod.111.095349
62. Chen L, Zhao Y, Li L, Xie L, Chen X, Liu J, et al. Bisphenol a stimulates differentiation of rat stem leydig cells in vivo and in vitro. Mol Cell Endocrinol. (2018) 474:158–67. doi: 10.1016/j.mce.2018.03.003
63. Thuillier R, Manku G, Wang Y, Culty M. Changes in MAPK pathway in neonatal and adult testis following fetal estrogen exposure and effects on rat testicular cells. Microsc Res Tech. (2009) 72:773–86. doi: 10.1002/jemt.20756
64. Song KH, Lee K, Choi HS. Endocrine disrupter bisphenol a induces orphan nuclear receptor Nur77 gene expression and steroidogenesis in mouse testicular leydig cells. Endocrinology. (2002) 143:2208–15. doi: 10.1210/endo.143.6.8847
65. Li X, Guo JY, Li X, Zhou HJ, Zhang SH, Liu XD, et al. Behavioural effect of low-dose BPA on male zebrafish: tuning of male mating competition and female mating preference during courtship process. Chemosphere. (2017) 169:40–52. doi: 10.1016/j.chemosphere.2016.11.053
66. Horstman KA, Naciff JM, Overmann GJ, Foertsch LM, Richardson BD, Daston GP. Effects of transplacental 17-α-ethynyl estradiol or bisphenol A on the developmental profile of steroidogenic acute regulatory protein in the rat testis. Birth Defects Res B Dev Reprod Toxicol. (2012) 95:318–25. doi: 10.1002/bdrb.21020
67. Samova S, Patel CN, Doctor H, Pandya HA, Verma RJ. The effect of bisphenol A on testicular steroidogenesis and its amelioration by quercetin: an in vivo and in silico approach. Toxicol Res. (2017) 7:22–31. doi: 10.1039/C7TX00161D
68. Nikula H, Talonpoika T, Kaleva M, Toppari J. Inhibition of hCG-stimulated steroidogenesis in cultured mouse leydig tumor cells by bisphenol a and octylphenols. Toxicol Appl Pharmacol. (1999) 157:166–73. doi: 10.1006/taap.1999.8674
69. Feng Y, Shi J, Jiao Z, Duan H, Shao B. Mechanism of bisphenol AF-induced progesterone inhibition in human chorionic gonadotrophin-stimulated mouse leydig tumor cell line (mLTC-1) cells. Environ Toxicol. (2018) 33:670–8. doi: 10.1002/tox.22554
70. Takamiya M, Lambard S, Huhtaniemi IT. Effect of bisphenol a on human chorionic gonadotrophin-stimulated gene expression of cultured mouse leydig tumour cells. Reprod Toxicol. (2007) 24:265–75. doi: 10.1016/j.reprotox.2007.07.003
71. Ye L, Binghai Z, Guoxin H, Yanhui C, Ren-Shan G. Inhibition of human and rat testicular steroidogenic enzyme activities by bisphenol A. Toxicol Lett. (2011) 207:137–42. doi: 10.1016/j.toxlet.2011.09.001
72. Eladak S, Grisin T, Moison D, Guerquin MJ, N'Tumba-Byn T, Pozzi-Gaudin S, et al. A new chapter in the bisphenol A story: bisphenol S and bisphenol F are not safe alternatives to this compound. Fertil Steril. (2015) 103:11–21. doi: 10.1016/j.fertnstert.2014.11.005
73. Bart J, Hollema H, Groen HJ, de Vries EG, Hendrikse NH, Sleijfer DT, et al. The distribution of drug-efflux pumps, P-gp, BCRP, MRP1 and MRP2, in the normal blood-testis barrier and in primary testicular tumours. Eur J Cancer. (2004) 40:2064–70. doi: 10.1016/j.ejca.2004.05.010
74. Morgan JA, Cheepala SB, Wang Y, Neale G, Adachi M, Nachagari D, et al. Deregulated hepatic metabolism exacerbates impaired testosterone production in Mrp4-deficient mice. J Biol Chem. (2012) 287:14456–66. doi: 10.1074/jbc.M111.319681
75. Kundakovic M, Champagne FA. Epigenetic perspective on the developmental effects of bisphenol A. Brain Behav Immun. (2011) 25:1084–93. doi: 10.1016/j.bbi.2011.02.005
76. Gao GZ, Zhao Y, Li HX, Li W. Bisphenol A-elicited miR-146a-5p impairs murine testicular steroidogenesis through negative regulation of Mta3 signaling. Biochem Biophys Res Commun. (2018) 501:478–85. doi: 10.1016/j.bbrc.2018.05.017
77. Ke H, Hu Q, Hui W, Shun Z, Xin-Hong Q, Wei L. Regulated and functional expression of the corepressor MTA3 in rodent testis. Endocrinology. (2016) 157:4400–10. doi: 10.1210/en.2016-1213
Keywords: bisphenols, BPA, endocrine disruptors, testicular steroidogenesis, spermatogenesis
Citation: Barbagallo F, Condorelli RA, Mongioì LM, Cannarella R, Aversa A, Calogero AE and La Vignera S (2020) Effects of Bisphenols on Testicular Steroidogenesis. Front. Endocrinol. 11:373. doi: 10.3389/fendo.2020.00373
Received: 01 January 2020; Accepted: 11 May 2020;
Published: 30 June 2020.
Edited by:
David H. Volle, Institut National de la Santé et de la Recherche Médicale (INSERM), FranceReviewed by:
Ren-Shan Ge, Wenzhou Medical University, ChinaCopyright © 2020 Barbagallo, Condorelli, Mongioì, Cannarella, Aversa, Calogero and La Vignera. This is an open-access article distributed under the terms of the Creative Commons Attribution License (CC BY). The use, distribution or reproduction in other forums is permitted, provided the original author(s) and the copyright owner(s) are credited and that the original publication in this journal is cited, in accordance with accepted academic practice. No use, distribution or reproduction is permitted which does not comply with these terms.
*Correspondence: Rosita A. Condorelli, cm9zaXRhLmNvbmRvcmVsbGlAdW5pY3QuaXQ=
Disclaimer: All claims expressed in this article are solely those of the authors and do not necessarily represent those of their affiliated organizations, or those of the publisher, the editors and the reviewers. Any product that may be evaluated in this article or claim that may be made by its manufacturer is not guaranteed or endorsed by the publisher.
Research integrity at Frontiers
Learn more about the work of our research integrity team to safeguard the quality of each article we publish.