- 1Laboratory of Endocrinology and Receptor Biology, National Institute of Diabetes and Digestive and Kidney Diseases, National Institutes of Health Bethesda, MD, United States
- 2Metabolic Disease Branch, National Institutes of Diabetes and Digestive and Kidney Diseases, National Institutes of Health, Bethesda, MD, United States
Thyrotropin hormone (TSH) was reported to exhibit biphasic regulation of cAMP production in human thyroid slices; specifically, upregulation at low TSH doses transitioning to inhibition at high doses. We observed this phenomenon in HEK293 cells overexpressing TSH receptors (TSHRs) but in only 25% of human thyrocytes (hThyros) in vitro. Because TSHR expression in hThyros in vitro was low, we tested the hypothesis that high, in situ levels of TSHRs were needed for biphasic cAMP regulation. We increased expression of TSHRs by infecting hThyros with adenoviruses expressing human TSHR (AdhTSHR), measured TSH-stimulated cAMP production and TSHR homodimerization. TSHR mRNA levels in hThyros in vitro were 100-fold lower than in human thyroid tissue. AdhTSHR infection increased TSHR mRNA expression to levels found in thyroid tissue and flow cytometry showed that cell-surface TSHRs increased more than 15-fold. Most uninfected hThyro preparations exhibited monotonic cAMP production. In contrast, most hThyro preparations infected with AdhTSHR expressing TSHR at in vivo levels exhibited biphasic TSH dose responses. Treatment of AdhTSHR-infected hThyros with pertussis toxin resulted in monotonic dose response curves demonstrating that lower levels of cAMP production at high TSH doses were mediated by Gi/Go proteins. Proximity ligation assays confirmed that AdhTSHR infection markedly increased the number of TSHR homodimers. We conclude that in situ levels of TSHRs as homodimers are needed for hThyros to exhibit biphasic TSH regulation of cAMP production.
Introduction
The TSH receptor (TSHR) in human thyroid cells couples to G proteins of all four subfamilies (1), including the stimulatory G protein Gs, which activates adenylyl cyclase to produce cAMP, Gi, which inhibits cAMP production, G13, which activates p44/42 mitogen-activated protein kinase (MAPK) (2), and Gq/G11, which activate phospholipase C to produce inositol-1,4,5-trisphosphate, which is rapidly degraded to inositol monophosphate (IP-1; phosphoinositide signaling) (3).
TSHR oligomerization in primary cultures of thyrocytes and in cells overexpressing TSHRs has been demonstrated by several techniques including fluorescence resonance energy transfer (FRET) and bioluminescence resonance energy transfer (BRET) (4). Latif et al. demonstrated the existence of TSHR oligomers in intact cells using FRET (5). BRET experiments by Urizar et al. confirmed TSHR homodimerization and demonstrated that the serpentine domain of the TSHR is primarily involved in dimerization (6). Furthermore, this study showed that TSH binding induces negative cooperativity, a mechanism where TSH binding to one site reduces the binding affinity to another site on the TSHR homodimer (6). The potency for TSH to stimulate cAMP signaling is ~100-fold higher than for phosphoinositide signaling (3). We showed previously that the different potencies occurred because cAMP signaling is initiated by binding of one TSH to one protomer of a putative TSHR homodimer whereas phosphoinositide signaling requires binding of two TSH molecules to the TSHR homodimer (7).
We recently reported biphasic regulation of cAMP production by TSH in HEK293 cells overexpressing TSH receptors (HEK-TSHR cells) with upregulation at low doses and downregulation at high doses; that is, an inverted U-shaped dose-response curve (IUDRC) (8). The upregulation was consistent with Gs-mediated high potency cAMP signaling. The inhibition at high doses of TSH was shown to be mediated by Gi/Go proteins.
Dumont and co-workers had observed a biphasic response for TSH-mediated cAMP generation in thyroid tissue slices from 11 of 14 patients (9, 10). We also found biphasic cAMP production in hThyros but it occurred in only 25% of the tested hThyros in vitro. We had observed previously that the level of TSHRs rapidly decreased after hThyros were placed in cell culture suggesting a potential dependence of the biphasic response on the level of TSHR expression. Therefore, we tested the hypothesis that in vivo levels of TSHRs were needed for formation of TSHR homodimers allowing for biphasic cAMP regulation.
Materials and Methods
Primary Cultures of hThyros
Primary cultures of hThyros were established by isolating cells from normal thyroid tissue samples from patients undergoing surgery for thyroid tumors at the National Institutes of Health Clinical Center as described previously (11). The studies involving human participants were reviewed and approved by the NIDDK Institutional Review Board. Written informed consent was obtained from the participants of the study. Parts of the thyroid tissue specimens were frozen immediately in liquid nitrogen for subsequent mRNA measurement.
We routinely measure thyroglobulin (TG) as a marker of thyrocyte functionality. In general, TG expression remains detectable for up to 10 passages. In addition, we observe TSH-stimulated upregulation of the expression of other thyroid gene markers, such as thyroid peroxidase (TPO), sodium iodide symporter (NIS), and iodothyronine deiodinase 2 (DIO2).
Infection With AdhTSHR
Adenovirus expressing full-length human TSHR, TSHR-Ad-RGD, was kindly provided by Basil Rapoport and Sandra McLachlan from Cedars-Sinai Medical Center (12). We refer to this virus as AdhTSHR. We used adenoviral-mediated gene transfer to produce hThyros with markedly increased levels of TSHR. For infection, 2.5 ×104 cells were seeded in 48-well plates in growth medium (DMEM supplemented with 10% FBS (Hyclone Laboratories, Inc., Logan, UT, USA) and penicillin/streptomycin, Mediatech Inc, Manassas, VA, USA) and were incubated in a humidified atmosphere of 5% CO2 at 37°C. After cells attached (6–24 h), the medium was aspirated and replaced with growth medium containing 0, 1, 10, or 50 MOI/cell, and the cells were incubated at 37°C for 72 to 96 h. TSHR overexpression was confirmed by quantitative RT-PCR and by fluorescence-activated cell sorting (FACS) analysis (13). Homodimer TSHR formation was measured by proximity ligation assay (PLA). For PLA, cells were reseeded into MatTek glass bottom dishes (MatTek Corporation, Ashland, MA, USA) 48 h after infection with AdTSHR and 24 h before the experiment (see below).
Measurement of TSHR mRNA Expression
Levels of mRNA were measured in total RNA preparations using RNeasy Mini Kits (Qiagen, Hilden, Germany) followed by reverse transcription to synthesize first-strand cDNA using High Capacity cDNA Archive Kit (Applied Biosystems, Foster City, CA, USA). DNase was used to prevent Ad-TSHR genomic DNA contamination. Quantitative RT-PCR was performed using the prepared cDNA and iTaq™ Universal Probe Supermix (Bio-Rad Laboratories, Hercules, CA, USA) and primers and probes for TSHR were obtained from Taqman, Assay-on-Demand (Applied Biosystems). Quantitative RT-PCR results were normalized to GAPDH as described previously (14).
Measurement of TSHR Cell Surface Protein Expression
Mouse monoclonal anti-TSHR antibody, KSAb1, was kindly provided by Dr. Paul Banga, Kings College London (15). The human monoclonal TSHR antibodies M22 and 2C11 were purchased from Kronus (Star, ID, USA) and Thermo Fisher Scientific, Inc. (Waltham, MA, USA), respectively.
KSAb1, M22, and 2C11 were labeled with Alexa Fluor 647 using the Alexa Fluor 647 Antibody Labeling Kit (Thermo Fisher Scientific, Inc.) according to manufacturer's directions. 72–96 h post-infection, cells were harvested using Accutase (Innovative Cell Technologies, Inc., San Diego, CA, USA), washed twice, resuspended to 1 ×106 cells/ml in ice-cold HBSS with 2% FBS, and maintained at 4°C. Cells were incubated with KSAb1-647, M22-647, or 2C11-647 at a final concentration of 1 μg/ml. Following a 2 h antibody incubation, cells were washed and resuspended in ice-cold HBSS with 2% FBS. Flow cytometry was performed using a BD FACSAria II Cell Sorter (BD Biosciences, Franklin Lakes, NJ, USA), with a 100-μm nozzle and a sheath pressure of 20 psi. Cell gating was done with FACS Diva software. Debris and clustered cells were excluded from gated populations. Labeled cells were gated to exclude auto-fluorescence as defined by fluorescence of unlabeled cells.
cAMP Assay
cAMP production was measured in hThyros incubated in HBSS/10 mM HEPES, pH 7.4 containing 0.5 mM 3-isobutyl-1-methylxanthine (IBMX) (Sigma-Aldrich) with increasing doses of bovine TSH (bTSH) (Merck Millipore, Darmstadt, Germany) in a humidified 5% CO2 incubator at 37°C for 60 min as previously described (8). Following aspiration of the medium, cells were lysed using lysis buffer of the cAMP-Screen chemiluminescent immunoassay system (Thermo Fisher Scientific Inc.). The cAMP content of the cell lysate was determined using the method described in the manufacturer's protocol.
Inositol-1-phosphate (IP-1) Assay
IP-1 production, an index of phosphoinositide signaling, was measured as described previously under the same conditions as cAMP production except that we used the stimulation buffer provided with the kit containing 50 mM LiCl (3).
Measurement of TSHR Homodimer Expression
Proximity ligation assay (PLA) was used to quantify TSHR-TSHR interactions, that is, TSHR homodimers (16). KSAb1 was conjugated to either Duolink PLUS or MINUS oligo arms using Duolink® In Situ Probemaker kits (Sigma-Aldrich, St. Louis, MO, USA). Cells were fixed with 4% methanol-free formaldehyde solution for 15 min at room temperature, blocked with Duolink blocking solution for 1 h at 37°C and then incubated with 4 μg/ml KSAb1-PLUS and KSAb1-MINUS overnight at 4°C. PLA was performed according to the manufacturer's directions. Cells were counter-stained with SYTO9 and phalloidin (Thermo Fisher Scientific, Inc) and stored at 4°C prior to fluorescent microscopy. Cells were imaged on a Zeiss 510 NLO/Meta system using a Plan-Apochromat 63x/1.40 Oil DIC objective. Phalloidin fluorescence was used to confirm that Duolink signals were on cells. SYTO9 fluorescence was used to count the number of cells. Cells over-expressing thyrotropin-releasing hormone receptors using AdCMVmTRH-R1 adenovirus (17) were used as control and gave no signal (data not shown). Although PLA is commonly quantified by the number dots/cell, the high number of dots in the 10 and 50 MOI conditions resulted in a coalescence of signal, therefore, dots/cell could not be quantified. Instead, positive PLA signal was quantified by the area of pixels in the PLA channel (546 nm) with fluorescence intensity greater than background. Data were averaged over all images to determine mean ± SEM. Duolink signal per cell was quantified with ImageJ (18, 19).
Statistical Analysis
The potencies (i.e., EC50s) were calculated from the dose-response curves using GraphPad Prism Version 7 for Windows (GraphPad Software, La Jolla, CA, USA). Statistical analysis was performed by Student's t-test; statistical significance was defined as P <0.05.
Results
Comparison of TSHR Expression in Thyroid Tissue and in Primary Cultures of hThyros
We observed that levels of TSHR mRNA in thyroid tissue obtained immediately after thryroidectomy are 100-fold higher than in hThyros in vitro. Figure 1 illustrates TSHR mRNA levels in human thyroid tissues (n = 29) and in hThyros after at least 2 weeks in culture (n = 31), a time at which cells are used in studies of TSH signaling. As a fraction of GAPDH mRNA, thyroid tissue TSHR mRNA was 557 ± 66 ×103 and hThyro TSHR mRNA was 3.8 ± 0.78 ×103 (P <0.0001).
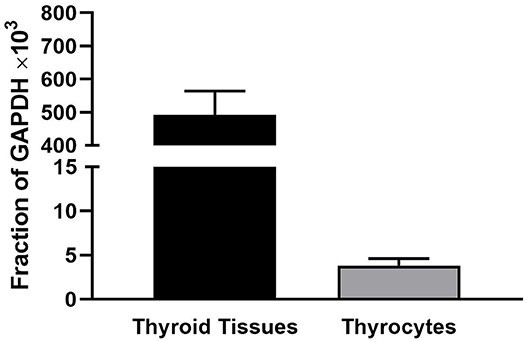
Figure 1. Comparison of the levels of TSHR mRNA in thyroid tissue and hThyros in vitro. There were 29 samples of thyroid tissue and 31 samples of hThyros. The mRNA levels were normalized to GAPDH mRNA. The values were markedly different (P <0.0001).
Figure 2A shows TSHR mRNA levels in hThyros infected with 0, 1, 10, and 50 MOI of AdhTSHR. As expected, there was a direct correlation of TSHR mRNA levels with increasing MOI. At AdhTSHR MOI of 10, the levels of TSHR mRNA in hThyros (1,700 ± 650 as a fraction of GAPDH x 103) were 3.0-fold higher than those in thyroid tissue. Measurement of cell surface TSHR protein expression in hThyros in culture from four different donors by flow cytometry with KSAb1-647 confirmed the correlation between increasing MOI and TSHR expression (Figure 2B). Similar increases in TSHR expression were found with two additional anti-TSHR antibodies M22-647 and 2C11-647 (data not shown). The increase of TSHR protein expression following infection with 50 MOI of AdhTSHR was donor dependent and ranged from 5 to 63-fold over control (0 MOI).
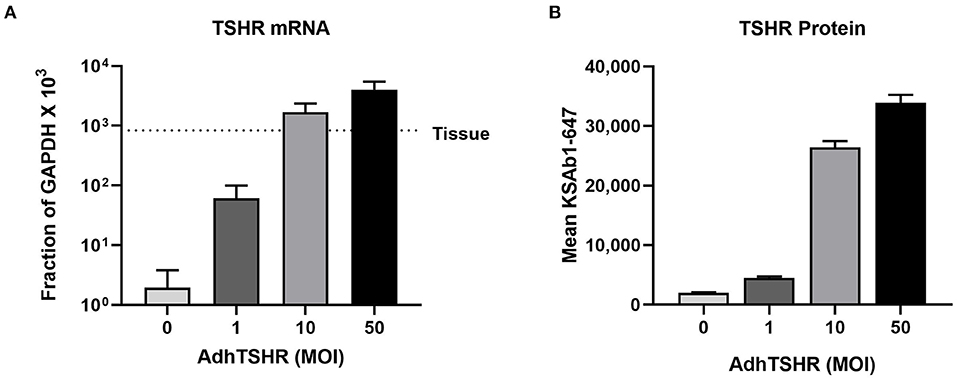
Figure 2. TSHR mRNA and protein expression in hThyros infected with AdhTSHR. (A) Effect of increasing AdhTSHR MOI on the level of TSHR mRNA in hThyros in culture. The measurements were performed in thyrocytes from four different donors in duplicate. Data are expressed as mean ± SD. (B) hThyros infected with increasing amounts of AdhTSHR were analyzed for KSAb1-647 fluorescence by flow cytometry. Bars depict mean KSAb1-647 fluorescence of labeled cells. Representative data from one thyrocyte strain are shown, and this experiment was repeated in thyrocytes from four different donors. Increase of TSHR protein expression following infection with 50 MOI was donor dependent and ranged from 5 to 63-fold over control (0 MOI).
in vivo TSHR Expression Is Crucial for Biphasic cAMP Regulation in hThyros
Infection of hThyros with increasing AdhTSHR MOI caused a progressive increase in basal cAMP levels that is due to constitutive signaling activity of TSHR (20), and concordantly increased cAMP production by 1 mU/ml TSH (Figure 3). In contrast, the effects of 100 mU/ml TSH were blunted compared to those of 1 mU/ml at 10 and 50 MOI with the decrease in cAMP greater at AdhTSHR of 50 MOI than at 10 MOI. These data indicate that the TSH-induced IUDCR for cAMP production may be dependent on the TSHR expression level. Therefore, we followed up by generating full dose response curves with TSH for cAMP production (Figure 4). In hThyros infected with AdhTSHR at 1 MOI, increasing doses of TSH stimulated a monotonic cAMP response that, as expected, was increased at high doses of TSH when the cells were pretreated with pertussis toxin, which relieves inhibition of adenylyl cyclase by Gi/Go proteins. In contrast, in hThyros infected with AdhTSHR at 50 MOI, increasing doses of TSH stimulated a biphasic cAMP response. As expected, because of the intrinsic constitutive activity of TSHR (20), the basal level and the response to low doses of TSH was greater and with higher potency than that in cells infected with 1 MOI AdhTSHR. More importantly, at high doses of TSH, cAMP production was inhibited exhibiting a classic IUDRC (21, 22). The decreasing response exhibited at high doses of TSH was abolished in cells pretreated with pertussis toxin. Thus, the biphasic response was dependent on high levels of TSHR expression, activation of Gs to stimulate adenylyl cyclase and activation of Gi/Go proteins to dampen adenylyl cyclase activity.
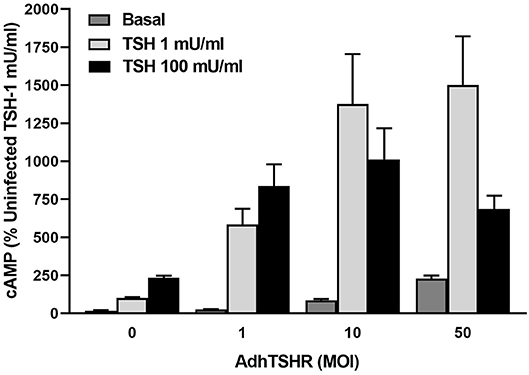
Figure 3. In vivo level expression of TSHR is required for biphasic cAMP production induced by TSH in hThyros. Cells were uninfected (0 MOI) or infected with AdhTSHR at 1, 10, and 50 MOI. Cells were not exposed to TSH (Basal) or were stimulated with TSH at 1 or 100 mU/ml. cAMP production was measured in cells incubated in buffer containing IBMX to inhibit cAMP degradation. Results shown are from 2 experiments performed in triplicate and are expressed as mean ± SEM. We observed statistically significant increase in constitutive levels of cAMP with increasing MOI of AdhTSHR (P <0.01 between 0 and 1 MOI and P <0.0001 between 0 and 10 and 50 MOI). The effects of 100 mU/ml TSH were reduced compared to those of 1 mU/ml at 10 and 50 MOI (P <0.045 between 1 and 100 mU/ml with 10 MOI and P <0.01 between 1 and 100 mU/ml with 50 MOI) with the decrease in cAMP greater at AdhTSHR of 50 MOI than at 10 MOI (P <0.04).
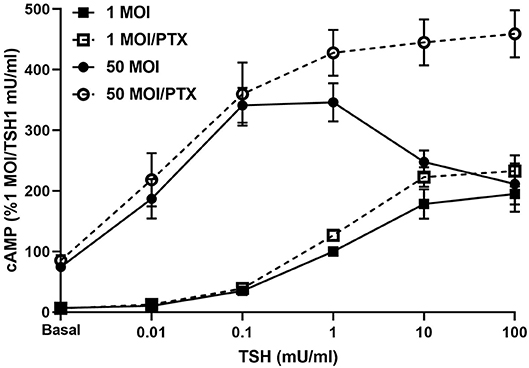
Figure 4. Biphasic cAMP production is stimulated by low TSH doses and inhibited by Gi/Go at high TSH doses. hThyros were infected with AdhTSHR at 1 MOI (squares) or 50 MOI (circles). Pertussis toxin (100 ng/ml) was added to cells 18 h prior to adding increasing doses of TSH. cAMP production was measured in cells incubated in buffer containing IBMX to inhibit cAMP degradation. Results shown are from 3 experiments performed in duplicate and presented as mean±SEM. In hThyros infected with AdhTSHR at 50 MOI and stimulated with high TSH doses, cAMP production exhibits a classic IUDRC. The decreasing response at 10 and 100 mU/ml TSH is abolished in cells pretreated with pertussis toxin (PTX) (P <0.01 and P <0.005, respectively).
Of note, the responses in IP-1 production to various doses of TSH was monotonic in hThyros infected with 1 or 50 MOI AdhTSHR (Supplemental Figure 1); there was little stimulation of IP-1 production in uninfected cells (data not shown) and low levels of IP-1 production in cells infected with 1 MOI AdhTSHR. As reported before, the potency for TSH in hThyros to stimulate IP-1 signaling is ~100-fold lower than for cAMP signaling. As expected, because of the increased TSHR levels and TSHR homodimer formation, the IP-1 response was greater in 50 MOI AdhTSHR infected cells. There was no effect of pertussis toxin pretreatment as the IP-1 response is mediated primarily by Gq/G11.
TSHR Levels Found in Thyroid Tissue Are Required for Homodimerization
PLA analysis was carried out to measure formation of TSHR homodimers. In uninfected hThyros, a few positive dots were detected which indicates homodimerization of TSHR at the endogenous expression level (Figure 5). hThyros infected with 1 MOI did not show a significant increase of TSHR homodimer expression compared to uninfected cells. In contrast, the Duolink signals in cells infected with 10 and 50 MOI AdhTSHR were very robust and demonstrated a strong increase in TSHR homodimer formation with correlates with an increase in TSHR expression (Figure 2). Figure 5 is representative of one of three donors. In this PLA, we used KSAb1, which is a monoclonal antibody that binds to a single epitope on TSHR, labeled with positive and negative adducts. Thus, the signal is from TSHR homodimers. We use the term homodimer to represent TSHR-TSHR dimers but recognize there may be higher order oligomers also. Moreover, although the PLA data demonstrate that two TSHR protomers are within 40 nanometers of each other, it does not prove that they are in direct contact.
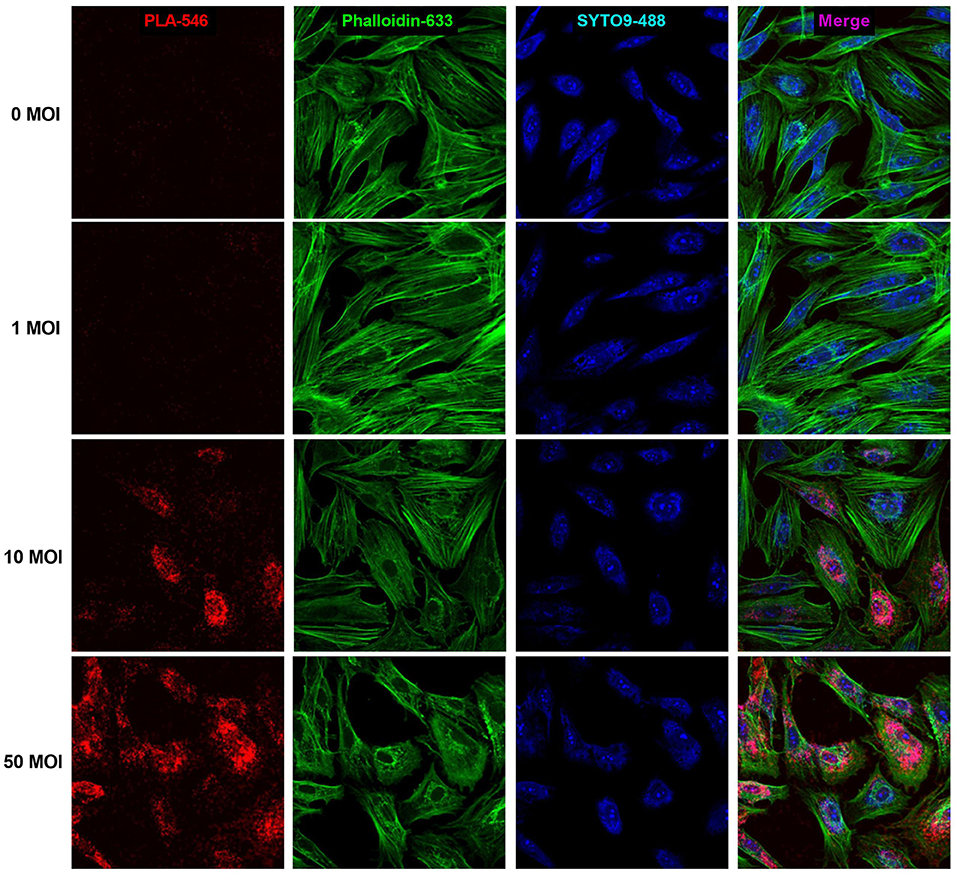
Figure 5. Formation of TSHR homodimers in hThyros infected with AdhTSHR. Increasing protein expression induces the formation of TSHR homodimers. Positive dots (red) in the proximity ligation assay decorate TSHR homodimers. Micrographs represent data from one of three thyrocyte strains from different donors. Cell boundaries were marked using Phalloidin Alexa 633 (green). Cell number was determined by SYTO9 staining (blue). We found no statistically significant difference between 0 and 1 MOI. The differences between uninfected cells and cells infected with 10 and 50 MOI were highly statistically significant (P <0.0001).
Discussion
Much of our understanding of TSHR signaling originated from in vitro studies. In those studies, TSH stimulation of cAMP production was monotonic. However, as mentioned above, Laurent et al. had observed a biphasic response for TSH-mediated cAMP generation in thyroid tissue slices from 11 of 14 patients (10). It is likely that TSHR levels in tissue slices were more similar to those present in vivo than those found in dispersed monolayer cultures in vitro that were employed in previous studies. Our recent report that TSH regulates cAMP in a biphasic manner in HEK 293 cells stably overexpressing the TSHR (HEK-TSHR cells) (8) implied that past investigations in monolayer cells may have missed an aspect of TSHR signaling that may be prevalent in vivo. We showed that TSHR expression is much higher in tissue obtained at thyroidectomy than in hThyros in vitro. We think that lower TSHR expression in vitro resulted in a blunted signaling response in primary cultures of hThyros rather than a different signaling profile. This possibility underscored the importance of formally testing the hypothesis that in vivo levels of TSHRs were needed for biphasic cAMP regulation. Previously, we reported that biphasic regulation of cAMP by TSH occurred robustly in HEK-TSHR cells (8) but we could only observe it in a small fraction of hThyros strains. Since the IUDRC was observed in hThyros from some donors, the findings in the HEK-TSHR cells could not be dismissed and motivated us to determine whether the IUDRC was an artifact of HEK-TSHR cells or a biologically relevant occurrence as it had also been described earlier by Dumont and co-workers for thyroid slices (9, 10). Here we found that in the majority of uninfected hThyros, cAMP production was monotonic with a plateau at TSH levels above 10 mU/ml. In contrast, hThyro strains from four different donors infected with AdhTSHR expressing TSHR mRNA at near in vivo levels exhibited higher levels of cAMP production that were biphasic with maximal levels with 1 mU/ml TSH and decreased production at higher TSH doses. We do not have a good measure of cell surface TSHR protein in vivo and, therefore, cannot conclude that we have replicated functional TSHRs in vitro at a level similar to that found in vivo. Nevertheless, we think we can conclude that near in vivo levels of TSHRs are needed for hThyros to exhibit biphasic TSH regulation of cAMP production.
Organization as dimers (or higher order oligomers) is a common feature of GPCRs that has been demonstrated for TSHR (4–6). It is well-documented that dimerization can have a major influence on signaling properties of interacting protomers in ligand binding, G protein coupling selectivity, and signal transduction mechanisms or cell surface expression (23). Here we show that TSHR dimers are likely involved in the mechanism of reduced cAMP production at high TSH doses. Because KSAb1 is a monoclonal antibody with a single epitope on TSHR that would not allow two KSAb1 antibodies to bind to a single receptor protomer, the PLA using KSAb1 with plus or minus arms allows us to positively identify TSHR homodimers. The PLA data presented herein (Figure 5) demonstrate that a high number of TSHR homodimers is present in hThyros expressing in vivo levels of TSHR. The decreasing phase of the biphasic dose response curve of TSH-stimulated cAMP production occurs at TSH doses similar to those needed for activation of Gq/G11. As we showed previously (7), signaling via cAMP production by activation of Gs requires occupancy of TSHR homodimers by one TSH molecule to one of the two protomers whereas activation of Gq/G11 leading to phosphoinositide signaling requires occupancy by two TSH molecules thereby explaining the ~100-fold lower TSH potency for phosphoinositide vs. cAMP signaling. Negative cooperativity was previously shown to be dependent on TSHR homodimerization (6), that is, on occupancy of the binding site on the second protomer of the homodimer. The dose dependency of TSH stimulation of inositol monophosphate (IP1) production was found similar to that of negatively cooperative 125I-TSH binding, which is known to require binding of unlabeled TSH to the low-affinity site on the second protomer (7). The need for the higher doses of TSH to bind to the unoccupied protomer is caused by the negative cooperativity since the occupied protomer induces the lower affinity exhibited by the second protomer. In this study, we show that the decreasing phase of cAMP production is reversed by pertussis toxin blocking of Gi/Go activation in hThyros as we demonstrated previously in HEK-TSHR cells (8). Since both high TSH doses and TSHR homodimers are necessary for cAMP inhibition, we conclude that Gi/Go activation by TSH requires binding of two TSH molecules, one to each TSHR protomer of TSHR homodimers as for Gq/G11 activation.
Hormesis, a biphasic response to increasing doses of a substance or condition, has been found in biology in general, especially in toxicology. The occurrence of U-shaped dose-response relationships has been documented in numerous biological, toxicological, and pharmacological investigations. Many of the endpoints studied are of considerable significance to public health (e.g., body weight, cholesterol levels, ethanol consumption, longevity, cancer incidence, cognitive functions and memory). An inverted IUDRC is a typical example of hormesis, and several hormetic dose-response relationships have been reported in receptor-mediated cell signaling (21). These findings suggest that hormetic responses may mediate specific cellular or physiological pathways. For stimulation of TSHRs, we suggest that the biphasic TSH dose response may be a regulatory mechanism to limit overstimulation of thyroid gland function. The mechanism we describe herein can serve as yet another fine regulator, in addition to the hypothalamic-pituitary-thyroid axis classical negative feedback, which emphasizes the importance of maintaining homeostasis in this axis controlling development, energy metabolism, growth, reproduction as well as heart and digestive function, muscle control, brain development, mood and bone maintenance.
In conclusion, we have shown that generation of a biphasic cAMP dose response by TSH in hThyros is dependent on the level of TSHR expression and the formation of TSHR homodimers. Given the likely higher TSHR expression in thyrocytes in the thyroid gland, signaling involving TSHR homodimers may have more biological relevance than previously appreciated.
Data Availability Statement
The datasets generated for this study are available on request to the corresponding author.
Ethics Statement
The studies involving human participants were reviewed and approved by the NIDDK Institutional Review Board. Written informed consent was obtained from the participants of the study.
Author Contributions
AB and CK: designed research, performed experiments, analyzed and interpreted the data, wrote the manuscript. BM-S: performed experiments, analyzed and interpreted the data. JK-G: provided thyroid tissue samples and facilitated collaboration with clinicians. SN: designed research, interpreted the data, wrote the manuscript. MG: designed and supervised the research, interpreted the data, wrote the manuscript.
Funding
This research was supported by the intramural research program (Z01 DK011006) of the National Institutes of Health.
Conflict of Interest
The authors declare that the research was conducted in the absence of any commercial or financial relationships that could be construed as a potential conflict of interest.
Acknowledgments
We would like to thank Oyeyemi Ruth Adewale and Stephanie Cardenas for providing us with patient's tissue samples.
Supplementary Material
The Supplementary Material for this article can be found online at: https://www.frontiersin.org/articles/10.3389/fendo.2020.00276/full#supplementary-material
Supplemental Figure 1. Effects of hThyros infection with 1 and 50 MOI AdhTSHR and pretreatment with pertussis toxin on TSH-stimulated IP-1 production. Cells were infected with AdhTSHR at 1 MOI (squares) or 50 MOI (circles). Pertussis toxin (100 ng/ml) was added 18 h prior to adding TSH. IP-1 production was measured in cells incubated in buffer containing LiCl to inhibit IP-1 degradation. Results shown are from 3 experiments performed in duplicate and presented as mean SEM. The IP-1 response was greater in 50 MOI AdhTSHR infected cells (P <0.006, P <0.002, and P <0.003 for 1, 10, and 100 mU/ml TSH). There was no effect of pertussis toxin (PTX) pretreatment.
References
1. Laugwitz KL, Allgeier A, Offermanns S, Spicher K, Van Sande J, Dumont JE, et al. The human thyrotropin receptor: a heptahelical receptor capable of stimulating members of all four G protein families. Proc Natl Acad Sci USA. (1996) 93:116–20. doi: 10.1073/pnas.93.1.116
2. Buch TR, Biebermann H, Kalwa H, Pinkenburg O, Hager D, Barth H, et al. G13-dependent activation of MAPK by thyrotropin. J Biol Chem. (2008) 283:20330–41. doi: 10.1074/jbc.M800211200
3. Corda D, Marcocci C, Kohn LD, Axelrod J, Luini A. Association of the changes in cytosolic Ca2+ and iodide efflux induced by thyrotropin and by the stimulation of alpha 1-adrenergic receptors in cultured rat thyroid cells. J Biol Chem. (1985) 260:9230–6.
4. Persani L, Calebiro D, Bonomi M. Technology insight: modern methods to monitor protein-protein interactions reveal functional TSH receptor oligomerization. Nat Clin Pract Endocrinol Metab. (2007) 3:180–90. doi: 10.1038/ncpendmet0401
5. Latif R, Graves P, Davies TF. Ligand-dependent inhibition of oligomerization at the human thyrotropin receptor. J Biol Chem. (2002) 277:45059–67. doi: 10.1074/jbc.M206693200
6. Urizar E, Montanelli L, Loy T, Bonomi M, Swillens S, Gales C, et al. Glycoprotein hormone receptors: link between receptor homodimerization and negative cooperativity. EMBO J. (2005) 24:1954–64. doi: 10.1038/sj.emboj.7600686
7. Allen MD, Neumann S, Gershengorn MC. Occupancy of both sites on the thyrotropin (TSH) receptor dimer is necessary for phosphoinositide signaling. FASEB J. (2011) 25:3687–94. doi: 10.1096/fj.11-188961
8. Neumann S, Malik SS, Marcus-Samuels B, Eliseeva E, Jang D, Klubo-Gwiezdzinska J, et al. Thyrotropin causes dose-dependent biphasic regulation of cAMP production mediated by Gs and Gi/o proteins. Mol Pharmacol. (2020) 97:2–8. doi: 10.1124/mol.119.117382
9. Van Sande J, Lamy F, Lecocq R, Mirkine N, Rocmans P, Cochaux P, et al. Pathogenesis of autonomous thyroid nodules: in vitro study of iodine and adenosine 3',5'-monophosphate metabolism. J Clin Endocrinol Metab. (1988) 66:570–9. doi: 10.1210/jcem-66-3-570
10. Laurent E, Van Sande J, Ludgate M, Corvilain B, Rocmans P, Dumont JE, et al. Unlike thyrotropin, thyroid-stimulating antibodies do not activate phospholipase C in human thyroid slices. J Clin Invest. (1991) 87:1634–42. doi: 10.1172/JCI115178
11. Morgan SJ, Neumann S, Gershengorn MC. Normal human thyrocytes in culture. Methods Mol Biol. (2018) 1817:1–7. doi: 10.1007/978-1-4939-8600-2_1
12. Chen CR, Pichurin P, Chazenbalk GD, Aliesky H, Nagayama Y, McLachlan SM, et al. Low-dose immunization with adenovirus expressing the thyroid-stimulating hormone receptor A-subunit deviates the antibody response toward that of autoantibodies in human graves' disease. Endocrinology. (2004) 145:228–33. doi: 10.1210/en.2003-1134
13. Krieger CC, Neumann S, Marcus-Samuels B, Gershengorn MC. TSHR/IGF-1R cross-talk, not IGF-1R stimulating antibodies, mediates graves' ophthalmopathy pathogenesis. Thyroid. (2017) 27:746–7. doi: 10.1089/thy.2017.0105
14. Jang D, Morgan SJ, Klubo-Gwiezdzinska J, Banga JPS, Neumann S, Gershengorn MC. Thyrotropin, but not thyroid-stimulating antibodies, induces biphasic regulation of gene expression in human thyrocytes. Thyroid. (2020) 30:270–6. doi: 10.1089/thy.2019.0418
15. Gilbert JA, Gianoukakis AG, Salehi S, Moorhead J, Rao PV, Khan MZ, et al. Monoclonal pathogenic antibodies to the thyroid-stimulating hormone receptor in graves' disease with potent thyroid-stimulating activity but differential blocking activity activate multiple signaling pathways. J Immunol. (2006) 176:5084–92. doi: 10.4049/jimmunol.176.8.5084
16. Bagchi S, Fredriksson R, Wallen-Mackenzie A. In situ proximity ligation assay (PLA). Methods Mol Biol. (2015) 1318:149–59. doi: 10.1007/978-1-4939-2742-5_15
17. Gershengorn MC, Heinflink M, Nussenzveig DR, Hinkle PM, Falck-Pedersen E. Thyrotropin-releasing hormone (TRH) receptor number determines the size of the TRH-responsive phosphoinositide pool. Demonstration using controlled expression of TRH receptors by adenovirus mediated gene transfer. J Biol Chem. (1994) 269:6779–83.
18. Schindelin J, Arganda-Carreras I, Frise E, Kaynig V, Longair M, Pietzsch T, et al. Fiji: an open-source platform for biological-image analysis. Nat Methods. (2012) 9:676–82. doi: 10.1038/nmeth.2019
19. Schneider CA, Rasband WS, Eliceiri KW. NIH Image to ImageJ: 25 years of image analysis. Nat Methods. (2012) 9:671–5. doi: 10.1038/nmeth.2089
20. Haas AK, Kleinau G, Hoyer I, Neumann S, Furkert J, Rutz C, et al. Mutations that silence constitutive signaling activity in the allosteric ligand-binding site of the thyrotropin receptor. Cell Mol Life Sci. (2011) 68:159–67. doi: 10.1007/s00018-010-0451-2
21. Calabrese EJ. Hormetic mechanisms. Crit Rev Toxicol. (2013) 43:580–606. doi: 10.3109/10408444.2013.808172
22. Vandenberg LN, Colborn T, Hayes TB, Heindel JJ, Jacobs DR Jr. Hormones and endocrine-disrupting chemicals: low-dose effects and nonmonotonic dose responses. Endocr Rev. (2012) 33:378–455. doi: 10.1210/er.2011-1050
Keywords: inverted U-shaped dose response curve, cAMP production, phosphoinositide signaling, thyrotropin receptor, TSHR, receptor homodimerization
Citation: Boutin A, Krieger CC, Marcus-Samuels B, Klubo-Gwiezdzinska J, Neumann S and Gershengorn MC (2020) TSH Receptor Homodimerization in Regulation of cAMP Production in Human Thyrocytes in vitro. Front. Endocrinol. 11:276. doi: 10.3389/fendo.2020.00276
Received: 09 March 2020; Accepted: 14 April 2020;
Published: 30 April 2020.
Edited by:
Noriyuki Koibuchi, Gunma University, JapanReviewed by:
Rauf Latif, Icahn School of Medicine at Mount Sinai, United StatesTakao Ando, Nagasaki University Hospital, Japan
Copyright © 2020 Boutin, Krieger, Marcus-Samuels, Klubo-Gwiezdzinska, Neumann and Gershengorn. This is an open-access article distributed under the terms of the Creative Commons Attribution License (CC BY). The use, distribution or reproduction in other forums is permitted, provided the original author(s) and the copyright owner(s) are credited and that the original publication in this journal is cited, in accordance with accepted academic practice. No use, distribution or reproduction is permitted which does not comply with these terms.
*Correspondence: Marvin C. Gershengorn, MarvinG@intra.niddk.nih.gov