- 1CEDOC, NOVA Medical School, Faculdade de Ciências Médicas, Universidade Nova de Lisboa, Lisbon, Portugal
- 2Faculty of Medicine, Institute of Physiology and Institute of Clinical and Biomedical Investigation of Coimbra (iCBR), University of Coimbra, Coimbra, Portugal
- 3Escola Superior de Tecnologia da Saúde, Departmento de Ciências Complementares, Instituto Politécnico de Coimbra, Coimbra, Portugal
- 4Departamento de Bioquímica y Biología Molecular y Fisiología, Facultad de Medicina, CSIC, Ciber de Enfermedades Respiratorias, CIBERES, Instituto de Biología y Genética Molecular, Instituto de Salud Carlos III, Universidad de Valladolid, Valladolid, Spain
Epidemiological studies showed that chronic caffeine intake decreased the risk of type 2 diabetes. Previously, we described that chronic caffeine intake prevents and reverses insulin resistance induced by hypercaloric diets and aging, in rats. Caffeine has several cellular mechanisms of action, being the antagonism of adenosine receptors the only attained with human coffee consumption. Here, we investigated the subtypes of adenosine receptors involved on the effects of chronic caffeine intake on insulin sensitivity and the mechanisms and sex differences behind this effect. Experiments were performed in male and female Wistar rats fed either a chow or high-sucrose (HSu) diet (35% of sucrose in drinking water) during 28 days, to induce insulin resistance. In the last 15 days of diet the animals were submitted to DPCPX (A1 antagonist, 0.4 mg/kg), SCH58261 (A2A antagonist, 0.5 mg/kg), or MRS1754 (A2B antagonist, 9.5 μg/kg) administration. Insulin sensitivity, fasting glycaemia, blood pressure, catecholamines, and fat depots were assessed. Expression of A1, A2A, A2B adenosine receptors and protein involved in insulin signaling pathways were evaluated in the liver, skeletal muscle, and visceral adipose tissue. UCP1 expression was measured in adipose tissue. Paradoxically, SCH58261 and MRS1754 decreased insulin sensitivity in control animals, whereas they both improved insulin response in HSu diet animals. DPCPX did not alter significantly insulin sensitivity in control or HSu animals, but reversed the increase in total and visceral fat induced by the HSu diet. In skeletal muscle, A1, A2A, and A2B adenosine receptor expression were increased in HSu group, an effect that was restored by SCH58261 and MRS1754. In the liver, A1, A2A expression was increased in HSu group, while A2B expression was decreased, being this last effect reversed by administration of MRS1754. In adipose tissue, A1 and A2A block upregulated the expression of these receptors. A2 adenosine antagonists restored impaired insulin signaling in the skeletal muscle of HSu rats, but did not affect liver or adipose insulin signaling. Our results show that adenosine receptors exert opposite effects on insulin sensitivity, in control and insulin resistant states and strongly suggest that A2 adenosine receptors in the skeletal muscle are the majors responsible for whole-body insulin sensitivity.
Introduction
In the past decades the prevalence of lifestyle diseases associated with metabolic disturbances like insulin resistance and obesity, core features in type 2 diabetes, has increased. Prediabetes and type 2 diabetes are characterized by defects in insulin secretion and insulin resistance, which leads to a decrease in whole-body glucose disposal (1). These diseases, seen as men's illness for decades, presently are more common in women than in men, and obesity has a higher association to insulin-resistance related risk factors in women than in men. Females tend to be more obese than men (2) with more women being overweight or obese after the age of 45 year, being this correlated with the higher incidence of insulin resistance and type 2 diabetes in females (2). Genetic effects and epigenetic mechanisms, nutritional factors, and sedentary lifestyle affect risk and complications differentially affect males and females and may be in the origin of sexes differences regarding metabolic diseases (3). Therefore, the study of the differential mechanisms on overall insulin sensitivity and metabolism between sexes may contribute to fill the gap in the current knowledge on sex-driven mechanisms, with a major impact for personalized medicine and societal strategies.
Caffeine is the most widely behaviorally active substance consumed in the world and when consumed chronically appears to have minor negative consequences on human health (4). Several epidemiological studies showed that chronic caffeine intake decreases the risk of type 2 diabetes in men and women (5–7). Additionally, our group described that chronic caffeine intake prevents the development of insulin resistance in female and male rats with prediabetes induced by the hypercaloric diets (8) and reversed insulin resistance in aged rats (9).
Caffeine has several mechanisms of action at a cellular level, however the only mechanism achieved with regular human caffeine consumption is the antagonism of adenosine receptors (4). Adenosine is a product of ATP catabolism, which can be recycled to re-synthesize ATP itself and exerts its action through four different G-protein coupled receptors, A1, A2A, A2B, and A3 (10). This mediator is involved in key pathways that regulate glucose homeostasis and insulin sensitivity, however its role remains controversial. Adenosine has been described to be associated with insulin sensitivity and glucose tolerance via action on adenosine A1 receptors (11). In contrast, Figler et al. (12) showed that adenosine through A2B adenosine receptors was involved in insulin resistance and inhibited whole body glucose disposal. In skeletal muscle, in vitro studies described an inhibitory effect of adenosine on glucose utilization and glucose transport induced by insulin (13–15), an effect that was shown to be mediated by A1 adenosine receptors (16). In contrast, other in vitro studies in skeletal muscle showed that adenosine has a stimulatory effect of insulin-induced glucose transport via A1 adenosine receptors (17–19).
In isolated rat hepatocytes, activation of A1 adenosine receptors triggers glycogenolysis, whereas the activation of adenosine A2A receptors increased gluconeogenesis (20). In contrast, other studies showed that the stimulation of adenosine A2B receptors augments glycogenolysis and gluconeogenesis (21, 22). In adipose tissue, it is consensual that adenosine inhibits lipolysis and stimulates lipogenesis through A1 adenosine receptors (23–27). This is in agreement with the increase in lipolysis, fat oxidation, and thermogenesis observed with caffeine intake and which contribute to its protective role in type 2 diabetes (28–30).
There is accumulating evidences from animal and human studies showing that central sympathetic overactivity plays a pivotal role in the etiology and complications of insulin resistance (31, 32). Activation of sympathetic nerves innervating the liver produce a rapid and marked production of glucose following a meal but promotes gluconeogenesis when fasted; and adrenal medulla activation can also stimulate the release of catecholamines to promote hepatic glucose production [for a review see Conde et al. (33)]. Sympathetic nerves innervating the skeletal muscle can promote glucose uptake independently of insulin through activation of β-adrenergic receptors, an effect counteracted by the neuronal stimulation of α-adrenergic receptors in arterioles, which elicits vasoconstriction (33). Acute caffeine has been shown to promote an increase in muscle sympathetic nervous activity (34). However, chronic caffeine administration has shown to normalize sympathetic activation and the levels of circulating catecholamines in rats (8), evidencing opposite roles for acute and chronic caffeine consumption.
Due to the contradictory findings regarding the role of adenosine receptors and the beneficial role of chronic caffeine on insulin sensitivity and glucose metabolism, herein, we explored the effect of 15 days administration of DPCPX, SCH58261, and MRS1754, an A1, A2A, and A2B adenosine receptor antagonists, in a rodent model of insulin resistance. Additionally, we investigated sex differences in the effects of these adenosine receptor antagonists on insulin sensitivity and signaling in insulin-sensitive tissues and on UCP1 expression in the visceral adipose tissue.
Materials and Methods
Animals and Experimental Procedures
Experiments were performed in Wistar rats (200–420 g) of both sexes, aged 3 months obtained from the vivarium of the NOVA Medical School|Faculdade de Ciências Médicas of the Universidade Nova de Lisboa, Lisboa, Portugal. Animals were kept under temperature and humidity control (21 ± 1°C; 55 ± 10% humidity) and a regular light (08.00–20.00 h) and dark (20.00–08.00 h) cycle, with food and water ad libitum. Animals were assigned to two groups: the control group that fed a standard diet (14.53% protein, 10% fat, 55.06% carbohydrates; RM3, SDS - Special Diet Services, UK) and the high-sucrose diet-fed (HSu) group, that fed the standard diet plus 35% (wt/vol.) sucrose (PanReac, Madrid, Spain) in drinking water for 28 days, representing a lean model of combined insulin resistance and hypertension (35, 36). In the last 15 days of the diet the animals were divided in 3 groups and submitted to the intraperitoneal administration of DPCPX (A1 antagonist, 0.4 mg/kg; Sigma, Madrid, Spain), SCH58261 (A2A antagonist, 0.5 mg/kg; Tocris Bioscience, UK), or MRS1754 (A2B antagonist, 9.5 μg/kg; Sigma, Madrid, Spain). All adenosine receptors antagonists were soluble in dimethyl sulfoxide (DMSO), and therefore to take into account the effects of DMSO on insulin sensitivity and glucose metabolism, a group of control and HSu rats was also submitted in the last 15 days of the diet to an intraperitoneal administration of DMSO (Vehicle, dilution 1:3). All test groups included males and females. Body weight, energy and liquid intake were monitored two times per week. At the end of 28 days of diet, animals were tested for insulin sensitivity through an insulin tolerance test (ITT) (8, 36). After the ITT, meaning 15 min post insulin administration, a heart puncture was performed to collect blood, the fat depots were collected and weighted, as well as the insulin sensitive tissues, the liver and skeletal muscle. The tissues were placed on liquid nitrogen and saved at −80°C, until posterior use. Laboratory care was in accordance with the European Union Directive for Protection of Vertebrates Used for Experimental and Other Scientific Ends (2010/63/ EU). Experimental protocols were approved by the NOVA Medical School|Faculdade de Ciências Médicas Ethics Committee.
Measurement of Insulin Sensitivity
The insulin sensitivity was evaluated by the ITT in animals under sodium pentobarbital (60 mg/kg, i.p.) anesthesia as previously described (8, 36). The ITT consists in the administration of an intravenous insulin (Humulin, 100 IU/ml, Lilly, Lisboa, Portugal) bolus of 0.1 U/kg body weight in the tail vein after an overnight fast, followed by measuring the decline in plasma glucose concentration over 15 min. The constant rate for glucose disappearance (KITT) was calculated using the formula 0.693/t1/2. Glucose half-time (t1/2) was calculated from the slope of the least square analysis of plasma glucose concentrations during the linear decay phase (8, 37). Blood samples were collected by tail snip tecnique and glucose levels were measured with a glucometer (Precision Xtra Meter, Abbott Diabetes Care, Portugal) and test strips (Abbott Diabetes Care, Portugal).
Measurement of Plasma Catecholamines Levels and Adenal Medulla Catecholamine Content
To quantify plasma catecholamines, 500 μl of plasma samples were purified and catecholamines were extracted using 30 mg OASIS Hlb Wat cartridges (Waters, Milford, MA, USA) and eluted in 500 μl of mobile phase as previously described (38). For quantification of catecholamine content in adrenal medulla, the organs previously frozen were homogenized in 0.6N perchloric acid, centrifuged at 13,000 g at 4°C and collected the supernatant. One hundred microliters of the samples were directly injected into a high-performance liquid chromatography system composed of a Waters 600 controller pump, a Waters C18 (particle size 4 μm) column, a Waters 717 plus autosampler, a Bioanalytical Systems LC-4A electrochemical detector (set at a holding potential of 0.65 mV and a sensitivity of 1 nA). An isocratic elution was used: the mobile phase consisted of a solution of Na2HP4 (25 mM) with 6% of methanol (pH 3.55), running at a flux of 1 ml/min. The signal coming out of the detector was fed to an analog to digital converter controlled by Peak Sample Chromatography System Software (Buck Scientific, East Norwalk, CT, USA). Identification and quantification of catecholamines were done against external standards.
Western Blot Analysis of Adenosine A1, A2A, and A2B Receptors, Insulin Receptor (IR), Protein Kinase B (Akt), Glucose Transporter Type 4 (GLUT4) or 2 (GLUT2), and Uncoupling Protein 1 (UCP1) in Skeletal Muscle, Liver, and Visceral Adipose Tissue
Skeletal muscle (50 mg), liver (50 mg), and visceral adipose tissue (100 mg) were homogenized in Zurich medium containing a cocktail of protease inhibitors (39). Samples were centrifuged (Eppendorf, Madrid, Spain) at 13,000 g for 20 min and the supernatant was collected and frozen at −80°C until further use. The evaluation of adenosine receptors A1, A2A, and A2B, UCP1, GLUT4, GLUT2, insulin receptor, insulin receptor phosphorylated at Tyr1361, Akt, and Akt phosphorylated at Ser473 was performed according to Sacramento et al. (39) and Matafome et al. (40). Briefly, after blocking for 1 h at room temperature with 5% non-fat milk in Tris-buffered saline (TBS), pH 7.4 containing 0.1% Tween 20 (TTBS) (BioRad, Spain), the membranes were incubated overnight at 4°C with the primary antibodies against A1 (1:200), A2A (1:200), A2B (1:200), GLUT4 (1:200), GLUT2 (1:200), insulin receptor (1:200) (Sta Cruz Biotechnology, USA), insulin receptor phosphorylated (phospho-Tyr1361, 1:500; Abcam, UK), Akt (1:1,000, Cell Signaling, USA), Akt phophorylated (phospho-Ser473, 1:1,000, Cell Signaling, USA), and UCP1 (1:1,000, Abcam, USA). The membranes were washed with Tris-buffered saline with 0.1% TBST and incubated with donkey anti-goat (1:2,000, Sta Cruz Biotechnology, USA) or goat anti-mouse (1:2,000, Sta Cruz Biotechnology, USA) or goat anti-rabbit (1:5,000, Rockland, USA) in TTBS for 2 h at room temperature and developed with enhanced chemiluminescence reagents according to the manufacturer's instructions (ClarityTM Western ECL substrate, BioRad, United States). Intensity of the signals was detected in a Chemidoc Molecular Imager (Chemidoc; BioRad, Madrid, Spain) and quantified using the Quantity-One software (BioRad, Madrid). We tested the expression of UCP1 in brown adipose tissue (BAT) as positive controls. The membranes were re-probed and tested for Calnexin (1:1,000, SicGen, Portugal), α-Tubulin (1:1,000, Sta Cruz Biotechnology, USA), or GAPDH (1:250, Sta Cruz Biotechnology, USA) immunoreactivity (bands in the 90, 55, and 37 kDa region, respectively) to compare and normalize the expression of proteins with the amount of protein loaded. Different loading proteins were used in accordance with the molecular weight of the protein to be studied or with the tissue in where protein expression was analyzed.
Data Analysis
Data were analyzed using GraphPad Prism Software, version 6 (GraphPad Software Inc., San Diego, CA, EUA) and were presented as mean ± SD. The significance of the differences between the means was calculated by One and Two-Way Analysis of Variance (ANOVA) with Dunnett's and Bonferroni multicomparison test, respectively. p-values of 0.05 or less were considered to represent significant differences.
Results
Liquid intake (milliliters/day) was similar in all groups of animals tested and the administration of the vehicle, DPCPX, SCH58261, and MRS1754 during 15 days did not modify liquid intake or animal behavior within groups (data not shown).
Effect of Chronic Administration of A1, A2A, and A2B Adenosine Receptor Antagonists on Insulin Sensitivity and Fasting Glycaemia
Figure 1 depicts the effect of chronic adenosine receptor antagonist administration on insulin sensitivity in control and HSu animals. Chronic blockade of A2A adenosine receptor by SCH58261 and A2B adenosine receptor by MRS1754 decreased significantly insulin sensitivity in control animals from a control value of 4.16 ± 0.83 to 3.31 ± 0.91% glucose/min and to 2.52 ± 0.47% glucose/min, respectively (Figure 1A). Administration of DPCPX, a selective A1 adenosine receptor antagonist, in control animals was unable to change insulin sensitivity (Figure 1A). As previously described by Conde et al. (8), ingestion of HSu diet during 28 days induced insulin resistance (KITT HSu = 2.41 ± 0.54% glucose/min) (Figure 1A). Chronic administration of DPCPX and SCH58261 improved insulin sensitivity by 23.24 and 36.93%, respectively (Figure 1A) in HSu animals. Moreover, blockade of A2B receptors almost restored insulin sensitivity induced by HSu diet (KITT HSu+MRS1754 = 3.84 ± 0.70% glucose/min). These effects of adenosine antagonists on insulin sensitivity in control and HSu groups followed the same pattern if animals were separated by sexes: females and males (Figures 1B,C). In control animals, chronic blockade of A1 receptors increased significantly by 16.25% fasting glycaemia (Table 1), whereas the blockade of A2A and A2B receptors did not produced any alteration. Chronic administration of HSu diet or of the different adenosine receptor antagonists did not modify significantly fasting glycaemia in HSu animals (Table 1).
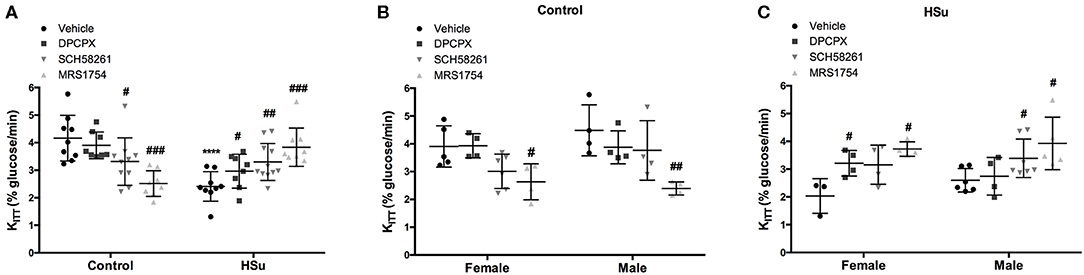
Figure 1. Effect of chronic administration of A1, A2A, and A2B adenosine receptor antagonists on insulin sensitivity in control and high-sucrose (HSu) animals. (A) Insulin sensitivity in animals of both sexes; (B,C) Gender differences in insulin sensitivity in control and HSu animals, respectively. Insulin sensitivity was determined by the insulin tolerance test (ITT) and expressed as constant for glucose disappearance (KITT). Vehicle (DMSO, dilution 1:3), DPCPX (A1 antagonist, 0.4 mg/kg), SCH58261 (A2A antagonist, 0.5 mg/kg), and MRS1754 (A2B antagonist, 9.5 μg/kg) were administered i.p. during 15 days. Values represent mean±SD of 8–11 animals of both sexes. One- and Two-Way ANOVA with Dunnett's and Bonferroni multicomparison tests, respectively: ****p < 0.0001 vs. vehicle (control); #p < 0.05, ##p < 0.01 and ###p < 0.001 comparing values with vehicle in the same group.
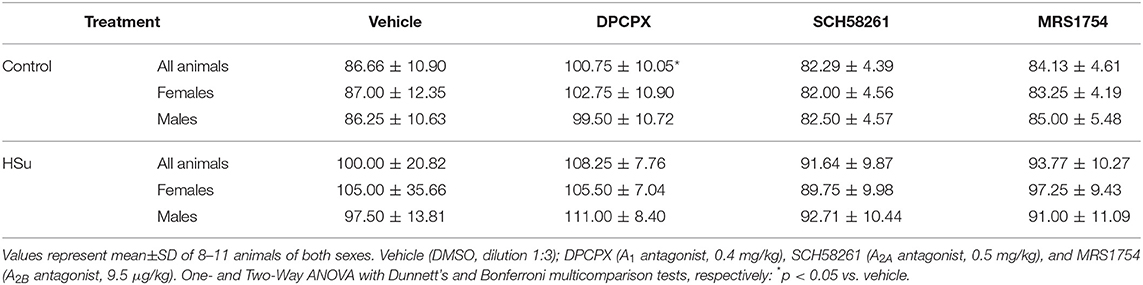
Table 1. Effect of chronic A1, A2A, and A2B adenosine receptor antagonist administration on fasting glycemia, in male and female rats submitted to a standard diet and to a high sucrose (HSu) diet.
Effect of Chronic Administration of A1, A2A, and A2B Adenosine Receptor Antagonists on Weight Gain and Fat Depots
HSu diet promoted an increase in weight gain (Figure 2), being the increase higher in males than in females (females = 2.27 ± 0.55 g/day; males = 3.96 ± 1.21 g/day). None of the adenosine antagonists tested altered weight gain in control or HSu animals, except the A2B antagonist, that increased by 211.90 and 244.19% weight gain in control female and male, respectively (Figure 2A).
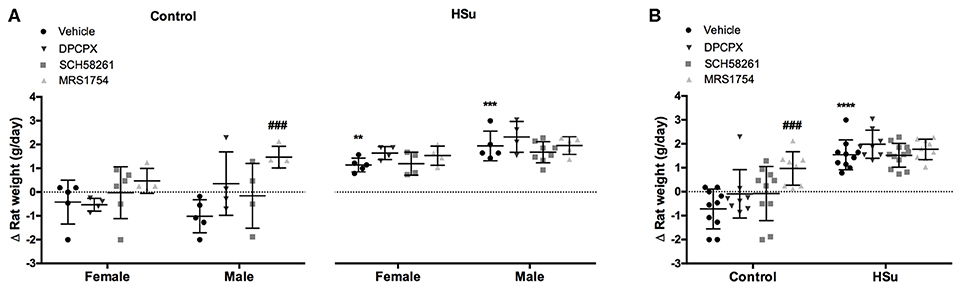
Figure 2. Effect of chronic A1, A2A and A2B adenosine receptor antagonist administration on body-weight increment, calculated as total weight variation during the experiment period. (A) Gender differences in weight increment in control and HSu female and male animals. (B) Weight gain in animals of both sexes. Vehicle (DMSO, dilution 1:3), DPCPX (A1 antagonist, 0.4 mg/kg), SCH58261 (A2A antagonist, 0.5 mg/kg), and MRS1754 (A2B antagonist, 9.5 μg/kg) were administered i.p. during 15 days. Values represent mean±SD of 8–12 animals of both sexes. One- and Two-Way ANOVA with Dunnett's and Bonferroni multicomparison tests, respectively: **p < 0.01, ***p < 0.001 and ****p < 0.0001 vs. vehicle (control); ###p < 0.001 comparing values with vehicle in the same group.
The effect of chronic administration of adenosine receptor antagonists on total, perienteric/visceral, genital, and perinephric fat in control and HSu males and females is shown in Table 2. Male control rats exhibit a higher total fat content than control females (Table 2). Administration of A1 and A2B blockers did not modify significantly nor the total fat amount neither the deposition of fat in the distinct fat depots, genital, perienteric, or perinephric in control males or females. Chronic administration of A2A blocker increased by 50.39% and decreased by 18.41% total fat amount, in control female and male animals, respectively (Table 2). These effects might be due to the 111.98% increase in the genital fat of female rats and to the 27.80% decrease in the perienephric fat of control males (Table 2). Interestingly, female HSu rats exhibit a higher total fat content than HSu males (Table 2). In HSu females, chronic A1 blockade decreased by 29.61, 22.10, 24.38, and 43.55% the total, perienteric, genital, and perinephric fat, respectively, while A2B blockade increased by 20.75% the total fat (Table 2). In male HSu animals, chronic administration of A2A and A2B antagonists increased by 24.19 and 20.10% the total fat, respectively, and by 25.32 and 22.59% the perinephric fat, respectively (Table 2). Concluding, male control rats exhibit higher total fat content than females, in contrast to what happens in HSu diet in which females exhibit more fat content than males. A1 receptor blockade in insulin resistant states lead to fat loss in female rats while the blockade of A2 receptors lead to an increase in total fat both in males and females.
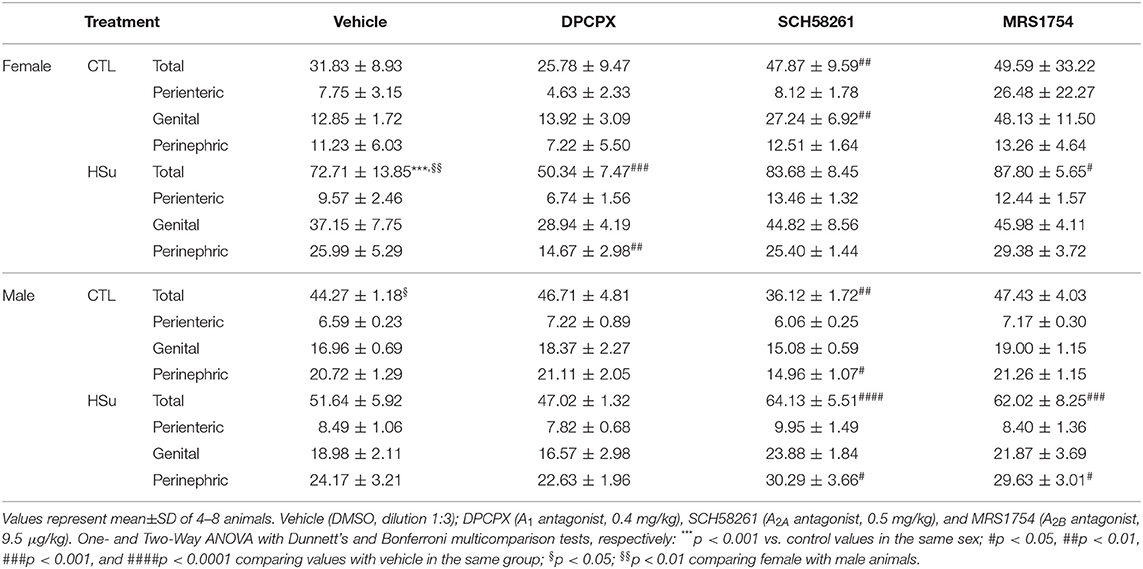
Table 2. Effect of chronic A1, A2A, and A2B adenosine receptor antagonist administration on total, visceral/perienteric, genital, and perinephric fat in control and high-sucrose (HSu) female and male animals.
Effect of Chronic Administration of A1, A2A, and A2B Adenosine Receptor Antagonists on Plasma and Adrenal Medulla Catecholamines
To evaluate the effect of chronic administration of A1, A2A, and A2B adenosine receptor antagonists on sympathetic nervous system activity, we measured both circulating and adrenal medulla catecholamines content in control and HSu animals (Table 3 and Figure 3). Values are presented in Table 3 separated by sexes and in Figure 3 plotted together. As expected and previously described (36) HSu diet increased plasma catecholamines and adrenal medulla Epi content (Figures 3A–C). Chronic administration of adenosine receptor antagonists did not modify circulating NE and Epi in both control and HSu animals (Figures 3A,B). Control animals submitted to chronic A1 and A2B adenosine receptor blockade exhibited significant increases of 252.07 and 209.68% in adrenal medulla NE, respectively, and of 172.22 and 128.30% in adrenal medulla Epi content, respectively, compared with the control animals (NE control vehicle = 2.17 ± 0.78 nmol/mg tissue; Epi control vehicle = 9.54 ± 2.76 nmol/mg tissue) (Figures 3C,D). Chronic A1 and A2B adenosine receptor blockade also increased significantly the adrenal medulla NE and Epi content in HSu animals (Figures 3C,D). SCH58261 chronic administration induced an increase of 37.52% in adrenal medulla Epi content in control animals (Figure 3D) and of 47.47% in adrenal medulla NE content in HSu animals (Figure 3C).
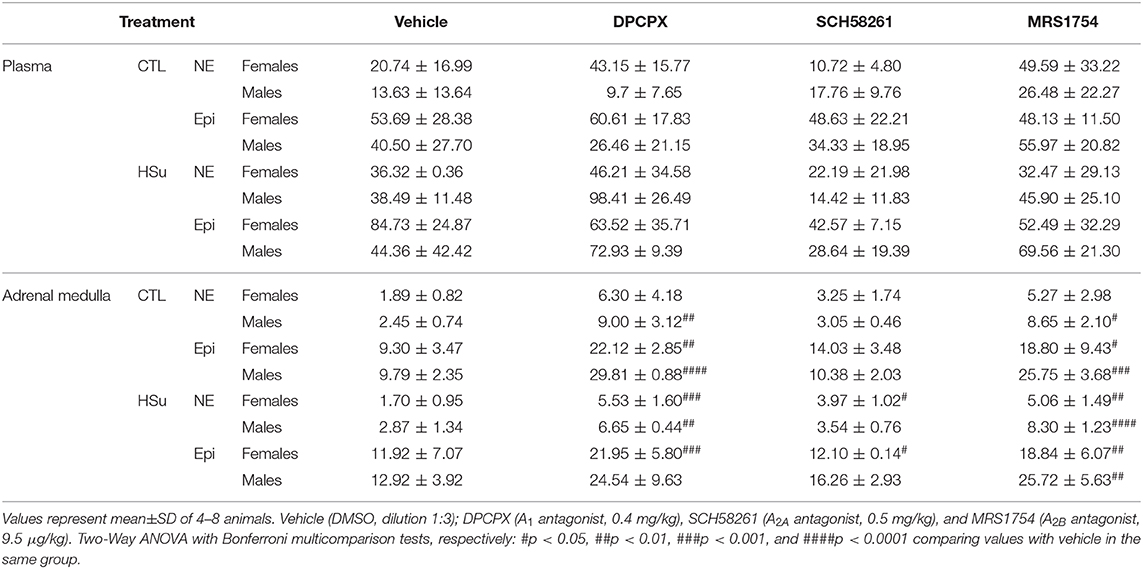
Table 3. Effect of chronic A1, A2A, and A2B adenosine receptor antagonist administration on circulating and adrenal medulla catecholamines, norepinephrine and epinephrine, in male and female rats submitted to a standard diet and to a high sucrose diet.
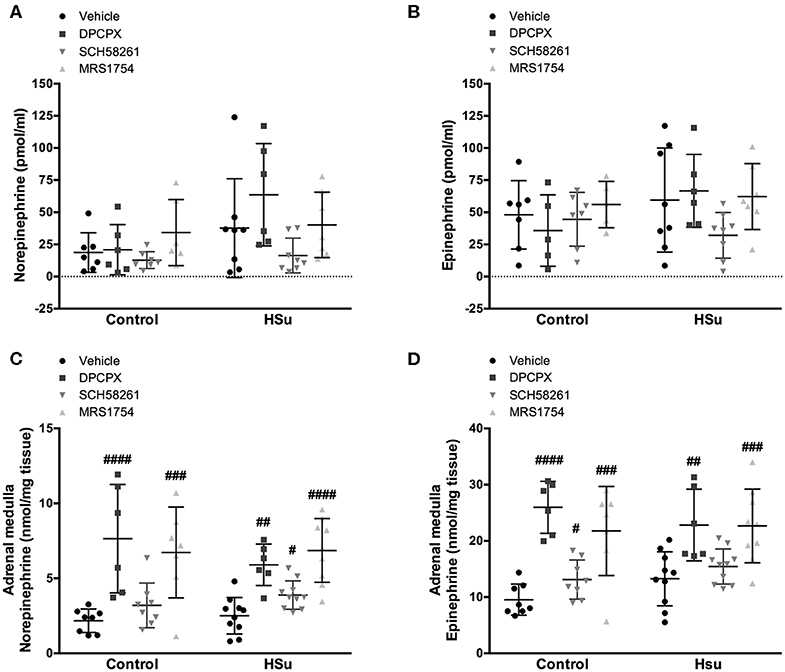
Figure 3. Effect of chronic A1, A2A, and A2B adenosine receptor antagonist administration on circulating catecholamines, norepinephrine and epinephrine, and on adrenal medulla norepinephrine and epinephrine content. (A,B) Shows respectively the impact of 15 days of A1, A2A, and A2B adenosine receptor antagonist administration on circulating catecholamines, norepinephrine, and epinephrine. (C,D) Shows respectively the impact of 15 days of A1, A2A, and A2B adenosine receptor antagonist administration on adrenal medulla norepinephrine and epinephrine content. Vehicle (DMSO, dilution 1:3), DPCPX (A1 antagonist, 0.4 mg/kg), SCH58261 (A2A antagonist, 0.5 mg/kg), and MRS1754 (A2B antagonist, 9.5 μg/kg) were administrated i.p. during 15 days. Values represent mean±SD of 6–11 animals. One- and Two-Way ANOVA with Dunnett's and Bonferroni multicomparison tests, respectively: #p < 0.05, ##p < 0.01, ###p < 0.001, ####p < 0.0001 comparing values with vehicle in the same group.
Effect of Chronic Administration of A1, A2A, and A2B Adenosine Receptor Antagonists on A1, A2A, and A2B Expression
No significant changes were observed for the effect of chronic DPCPX, SCH58261, and MRS1754 administration on the expression of A1, A2A, and A2B in insulin sensitive tissues expressed by sex (data not shown), thereby the results of female and male animals were plotted together (Figure 4). DMSO can interfere with various cellular processes (41), but herein DMSO did not modify the expression of A1, A2A, and A2B in skeletal muscle, liver, and adipose tissue (Figures 4A1,B1,C1). Chronic DPCPX and SCH58261 increased significantly by 44.72 and 65.82% the expression of A1, respectively, in the skeletal muscle from control animals (Figure 4A2). Additionally, chronic SCH58261 administration increased by 34.85% the expression of A2A receptors, but MRS1754 did not alter the expression of the different adenosine receptors in skeletal muscle (Figure 4A2). HSu diet itself caused a significant increase of 78.57 and 18.18% in the expression of A2A and A2B adenosine receptors, respectively, an effect that was restored by the chronic administration of SCH58261 and MRS1754, respectively (Figure 4A2). The expression of A1 adenosine receptors was also increased by 36.33% with the HSu diet, but chronic DPCPX administration did not altered A1 receptor expression in skeletal muscle (Figure 4A2).
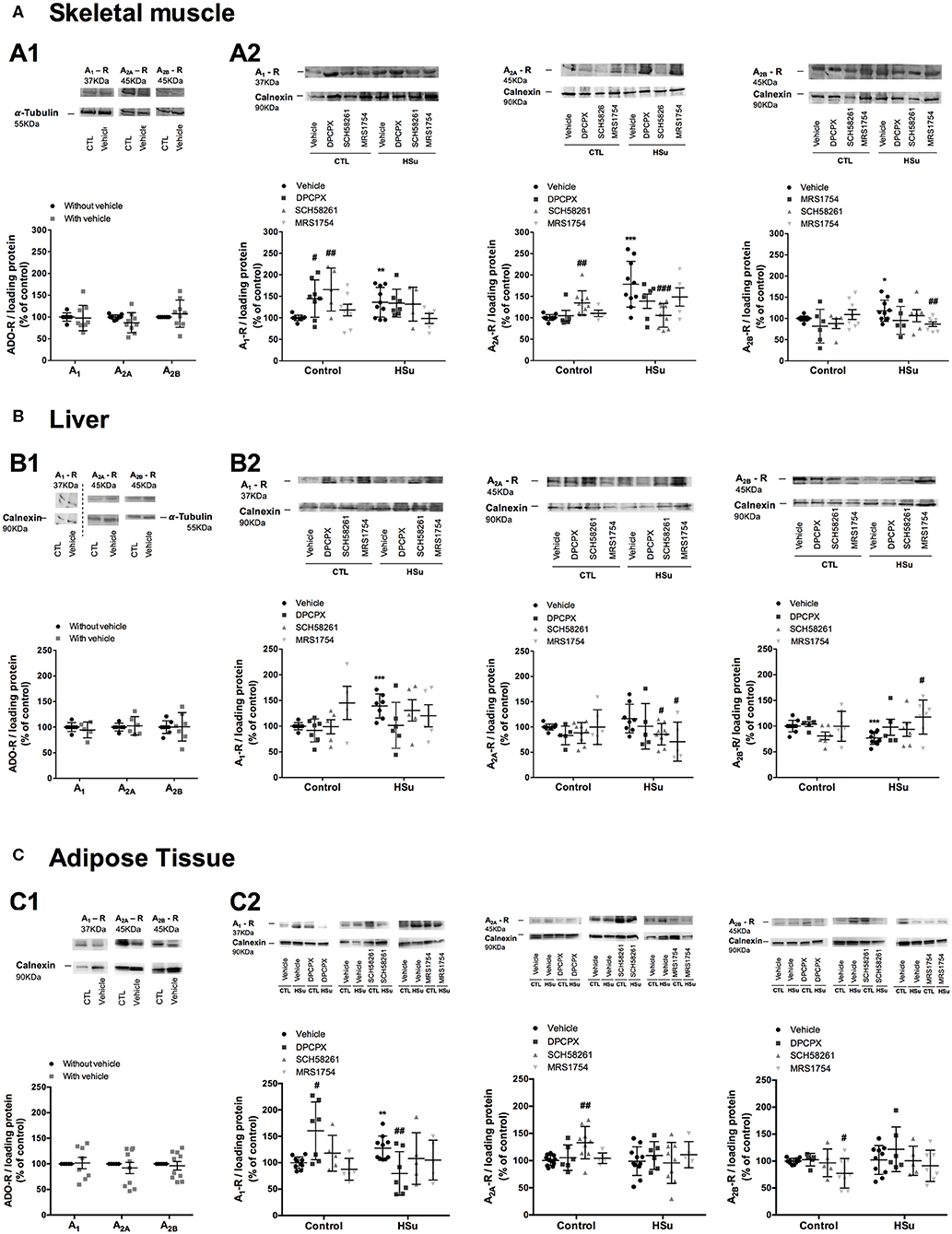
Figure 4. Effect of chronic A1, A2A, and A2B adenosine receptor antagonist administration on the expression of its receptors on the insulin sensitivity tissues, skeletal muscle, liver, and visceral fat. (A1,B1,C1) Shows respectively the impact of vehicle administration on the expression of the adenosine tested in the skeletal muscle, liver, and visceral fat of control animals. (A2,B2,C2) Shows respectively the impact of 15 days of A1, A2A, and A2B adenosine receptor antagonist administration on adenosine receptor expression in the skeletal muscle, liver, and visceral fat of control and HSu animals in relation to the expression of the loading protein. Vehicle (DMSO, dilution 1:3), DPCPX (A1 antagonist, 0.4 mg/kg), SCH58261 (A2A antagonist, 0.5 mg/kg), and MRS1754 (A2B antagonist, 9.5 μg/kg) were administrated i.p. during 15 days. Values represent mean±SD of 5–10 animals. One- and Two-Way ANOVA with Dunnett's and Bonferroni multicomparison tests, respectively: *p < 0.05, **p < 0.01, and ***p < 0.001, vs. vehicle (control); #p < 0.05, ##p < 0.01, and ###p < 0.001 comparing values with vehicle in the same group.
In the liver, chronic administration of DPCPX, SCH58261, and MRS1754 in control animals did not modify the expression of A1, A2A, and A2B adenosine receptors, respectively (Figure 4B2). HSu diet increased by 73.64 and 16.47% (p = 0.091) the expression of A1 and A2A adenosine receptors, respectively (Figure 4B2), but decreased by 23.17% the expression of adenosine A2B receptors, being this last effect restored by chronic MRS1754 administration (Figure 4B2).
Following the same profile than the skeletal muscle, in the adipose tissue from control animals, chronic administration of DPCPX and SCH58261 increased by 60.44 and 37.54% the expression of A1 and A2A adenosine receptors, respectively. Although in this tissue the administration of MRS1754 decreased by 22.80% the expression of A2B adenosine receptors (Figure 4C2). HSu diet or the administration of DPCPX, SCH58261, and MRS1754 in this insulin-resistant animal model did not modify the expression of A1, A2A, and A2B receptors in adipose tissue (Figure 4C2). Generally and as expected, the selective blockade of the different adenosine receptors produced an upregulation of these receptors in insulin sensitive tissues. HSu diet increased A1, A2A, and A2B receptor expression in the skeletal muscle, increased A1 receptor expression and decreased A2B in the liver, and increased A1 in the adipose tissue, effects that were rescued by the blockade of the respective adenosine receptors.
Effect of Chronic A1, A2A, and A2B Adenosine Receptor Antagonist Administration on Insulin Signaling Pathways
No sex differences were seen for effect of chronic DPCPX, SCH58261, and MRS1754 administration on insulin signaling pathways, and therefore results were expressed together (Figures 5–7). DMSO, the vehicle used in this study, did not modify the levels and activity of insulin receptor, Akt and GLUT4 levels in the skeletal muscle, liver, and adipose tissue (Figures 5–7A). In the skeletal muscle from control animals, chronic administration of SCH58261 and MRS1754 decreased significantly by 26.27 and 23.21% insulin receptor levels (Figure 5B). Insulin receptor phosphorylation, Akt levels and phosphorylation did not change with chronic administration of the different adenosine receptor antagonists in control animals (Figures 5C–E). GLUT4 levels in control animals decreased by 20.68, 17.29, and 16.40% with chronic DPCPX, SCH58261, and MRS1754 administration, respectively (Figure 5F). As expected and consistent with the development of insulin resistance, HSu diet decreased insulin receptor expression and GLUT4 levels by 23.89 and by 27.00%, respectively, without any effect on insulin receptor and Akt phosphorylation. In HSu animals, chronic administration of DPCPX, SCH58261, and MRS1754 increased by 40.81 (p = 0.066), 49.52 and 58.68% insulin receptor levels (Figure 5B). Chronic administration of the different adenosine receptor antagonists did not modify insulin receptor and Akt phosphorylation and GLUT4 levels (Figures 5C,E,F).
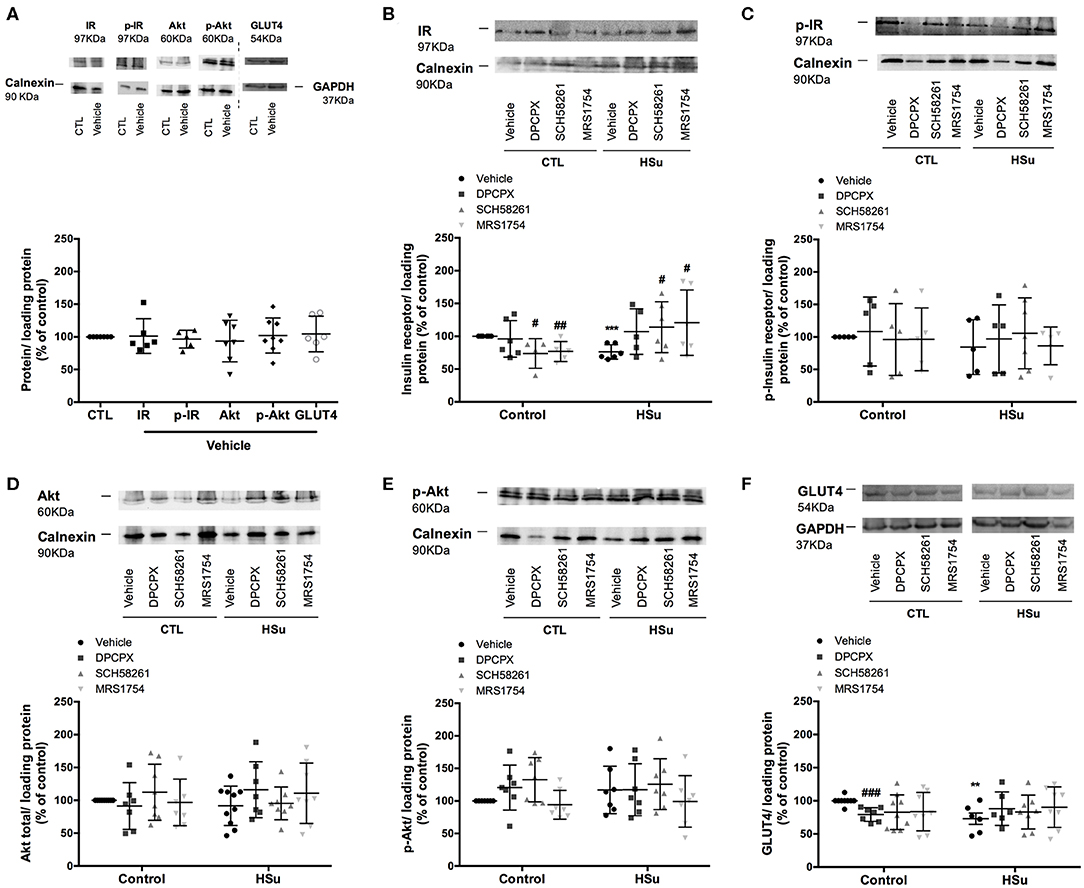
Figure 5. Effect of chronic A1, A2A, and A2B adenosine receptor antagonist administration on insulin signaling pathways in the skeletal muscle. (A) Effect of the vehicle, DMSO, on insulin signaling pathways in control animals. Average relative (B) insulin receptor levels (97 kDa band), (C) insulin receptor phosphorylation (97 kDa band), (D) Akt levels (60 kDa band), (E) Akt phosphorylation (60 kDa band), and (F) GLUT4 (54 kDa band) immunoreactivity in skeletal muscle from control and HSu animals with or without chronic A1, A2A, and A2B adenosine receptor antagonist administration in relation to the expression of the loading protein. Representative western blots for each protein studied are depicted above the respective graphs. Vehicle (DMSO, dilution 1:3), DPCPX (A1 antagonist, 0.4 mg/kg), SCH58261 (A2A antagonist, 0.5 mg/kg), and MRS1754 (A2B antagonist, 9.5 μg/kg) were administrated i.p. during 15 days. Values represent mean±SD of 5–10 animals. One- and Two-Way ANOVA with Dunnett's and Bonferroni multicomparison tests, respectively: **p < 0.01 and ***p < 0.001 vs. vehicle (control); #p < 0.05, ##p < 0.01, and ###p < 0.001 comparing values with vehicle in the same group.
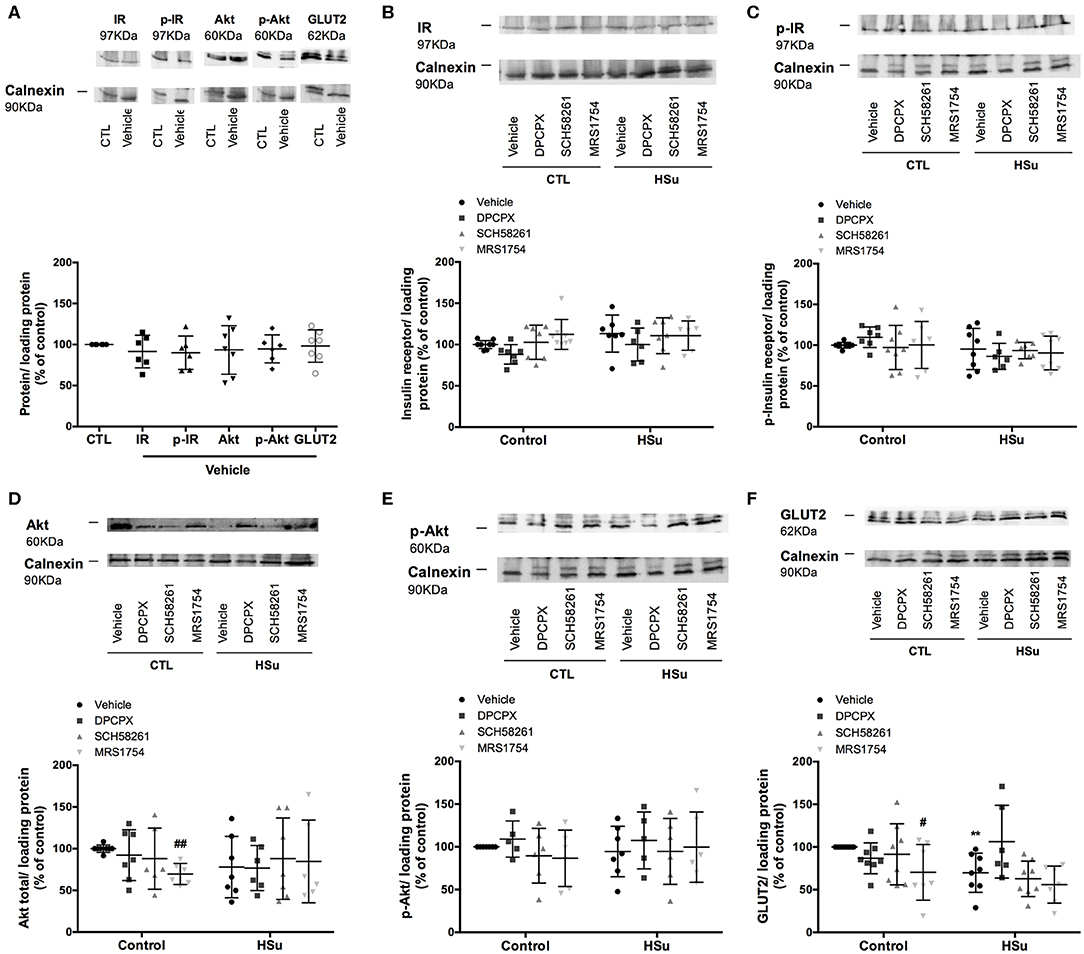
Figure 6. Effect of chronic A1, A2A, and A2B adenosine receptor antagonist administration on insulin signaling pathways in the liver. (A) Effect of the vehicle, DMSO, on insulin signaling pathways in control animals. Average relative (B) insulin receptor levels (97 kDa band), (C) insulin receptor phosphorylation (97 kDa band), (D) Akt levels (60 kDa band), (E) Akt phosphorylation (60 kDa band), and (F) GLUT2 (62 kDa band) immunoreactivity in the liver from control and HSu animals with or without chronic A1, A2A, and A2B adenosine receptor antagonist administration in relation to the expression of the loading protein. Representative western blots for each protein studied are depicted above the respective graphs. Vehicle (DMSO, dilution 1:3), DPCPX (A1 antagonist, 0.4 mg/kg), SCH58261 (A2A antagonist, 0.5 mg/kg), and MRS1754 (A2B antagonist, 9.5 μg/kg) were administrated i.p. during 15 days. Values represent mean±SD of 5–10 animals. One- and Two-Way ANOVA with Dunnett's and Bonferroni multicomparison tests, respectively: **p < 0.01 vs. vehicle (control); #p < 0.05 and ##p < 0.01 comparing values with vehicle in the same group.
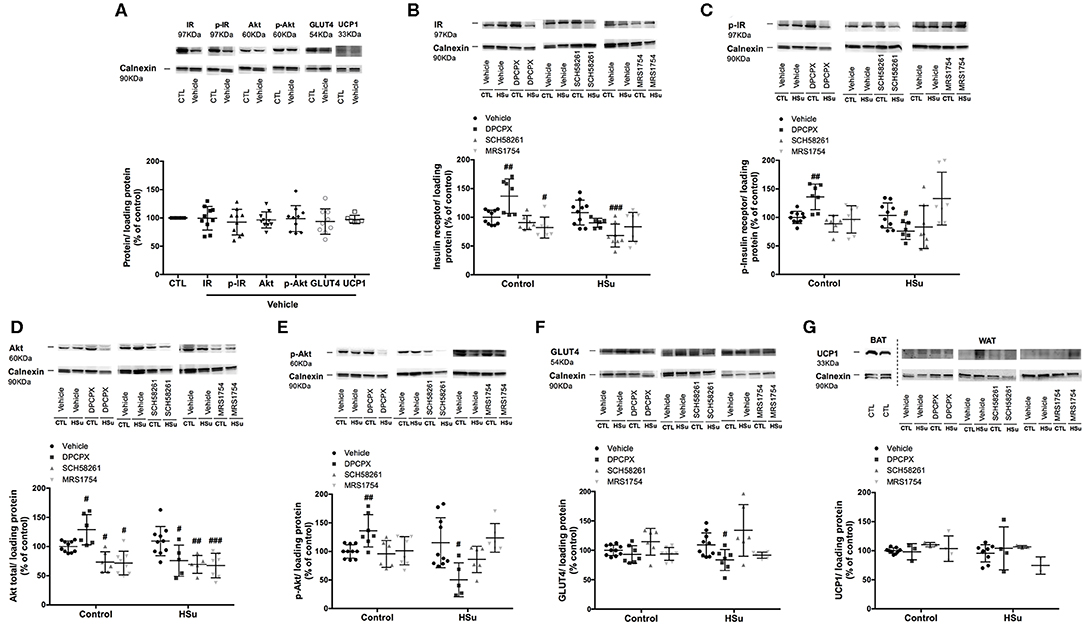
Figure 7. Effect of chronic A1, A2A, and A2B adenosine receptor antagonist administration on insulin signaling pathway and UCP1 expression in the visceral/perienteric adipose tissue. (A) Effect of the vehicle, DMSO, on insulin signaling pathways in control animals. Average relative (B) insulin receptor levels (97 kDa band), (C) insulin receptor phosphorylation (97 kDa band), (D) Akt levels (60 kDa band), (E) Akt phosphorylation (60 kDa band), (F) GLUT4 (54 kDa band), and (G) UCP1 (33 kDa band) immunoreactivity in white visceral adipose tissue (WAT) from control and HSu animals with or without chronic A1, A2A, and A2B adenosine receptor antagonist administration in relation to the expression of the loading protein. Representative western blots for each protein studied are depicted above the respective graphs. A positive control for UCP1 in brown adipose tissue (BAT) was used (G). Vehicle (DMSO, dilution 1:3), DPCPX (A1 antagonist, 0.4 mg/kg), SCH58261 (A2A antagonist, 0.5 mg/kg), and MRS1754 (A2B antagonist, 9.5 μg/kg) were administrated i.p. during 15 days. Values represent mean±SD of 5–10 animals. One- and Two-Way ANOVA with Dunnett's and Bonferroni multicomparison tests, respectively: #p < 0.05, ##p < 0.01, and ###p < 0.001, comparing values with vehicle in the same group.
In the liver in control animals, chronic administration of DPCPX and SCH58261 did not modify insulin receptor, Akt levels and their phosphorylation, whereas MRS1754 administration decreased by 30.31 and 29.66% Akt and GLUT2 levels, respectively (Figures 6B–F). HSu diet ingestion during 4 weeks did not alter levels and phosphorylation of insulin receptor and Akt, while decreased GLUT2 levels by 52.27% in liver, an effect restored with the chronic administration of DPCPX (p = 0.060) (Figures 6B–F).
In the visceral/perienteric adipose tissue, chronic administration of DPCPX in control animals increased insulin receptor levels and its phosphorylation, Akt levels and its phosphorylation by 36.81, 36.00, 28.86, and 36.10%, respectively, without altering Glut4 levels (Figures 7B–F). In contrast, chronic MRS1754 administration decreased by 17.92 and 28.28% insulin receptor values and Akt levels, respectively (Figures 7B,D). Moreover, chronic SCH58261 administration decreased by 26.60% Akt levels (Figure 7D). HSu diet per se was unable to alter the levels and activity of insulin receptor, Akt and GLUT4 levels (Figures 7B–F). In HSu animals, insulin receptor levels and its phosphorylation decreased by 36.91 and 26.31% with chronic administration of SCH58261 and DPCPX, respectively (Figures 7B,C). Chronic DPCPX and SCH58261 administration decreased by 30.71 and 36.55% Akt levels and by 64.83 and 33.48% Akt phosphorylation, respectively, although this last effect of SCH58261 on Akt phosphorylation was non–significant (Figures 7D,E). MRS1754 only decreased Akt values by 38.43% (Figures 7D,E). Also, in the adipose tissue from HSu animals, chronic DPCPX administration decreased by 23.56% GLUT4 levels (Figure 7F).
Effect of Selective Adenosine Receptor Blockade on UCP1 Expression in the Visceral Adipose Tissue
One of the mechanisms described to be associated with the beneficial caffeine consumption on metabolism is the increase in thermogenesis (29). The innervation of the brown adipose tissue by the sympathetic nervous system function as the principal stimulator of brown adipose tissue thermogenesis, being the activation of the sympathetic nervous system known also as one of the main triggers for the browning/beiging of the white adipose tissue (42). The beiging of the visceral white adipose tissue was evaluated as the expression of UCP1. From the observation of Figure 7G in the representative western blots and as expected, it is clear that the levels of UCP1 expression in the visceral adipose tissue are quite small in comparison with the levels of expression in brown adipose tissue. Also, HSu diet did not modify UCP1 expression in the visceral adipose tissue (p = 0.400) as well as none of the adenosine receptor antagonists changed the expression of UCP1 (Figure 7G).
Discussion
In the present study, we demonstrated that adenosine receptors exhibit opposite effects on insulin sensitivity, as chronic adenosine antagonists in control animals promote insulin resistance meaning that adenosine is an insulin-sensitizer and that in insulin-resistant conditions, in HSu animals, chronic antagonists rescue the insulin-resistance phenotype. Additionally, we showed that the role of adenosine receptors in whole-body insulin sensitivity is manly mediated by adenosine A2 receptors, with a contribution from adenosine A1 receptors, an effect similar between female and male animals. Consistent with these findings, A2A and A2B adenosine receptors antagonists rescued impaired insulin signaling pathways in the skeletal muscle. In contrast, the antagonists tested herein did not alter significantly insulin signaling in the liver, except the A2B antagonist that decreased Akt and GLUT2 expression in control animals, and contribute for the deregulation of insulin signaling pathways in the adipose tissue in HSu animals. In agreement with the improvement of insulin signaling pathways in the skeletal muscle of HSu animals, these animals showed increased expression of A2A and A2B receptor expression that was restored with the chronic blocking of these receptors.
We also show that the effect of chronic adenosine blockade on fat depots was different between females and males, with fat depots accumulation being decreased in female HSu animals submitted to the inhibition of A1 adenosine receptors, while increased with the inhibition of A2A and A2B adenosine receptors in male HSu animals. HSu hypercaloric diet promoted the overexpression of A1 receptors in the adipose tissue, an, effect rescued by A1 receptors blockade. Chronic A1 and A2B adenosine blocking induced an increase in sympatho-adrenal activity that did not correlate with the activation of the thermogenesis in the white adipose tissue.
All together, the results herein described strongly suggest that A2 adenosine receptors in the skeletal muscle and A1 receptors in the adipose tissue are majors contributors for the whole-body insulin sensitivity and that in the context of lean insulin resistance, as the HSu hypercaloric diet, the effect of adenosine on insulin action on skeletal muscle is more relevant than the effect of adenosine on adipose tissue.
Role of Adenosine Receptors on Whole-Body Insulin Sensitivity and Action
The present study was focused on adenosine A1, A2A, and A2B receptors, since the role of A3 adenosine receptors on insulin sensitivity and glucose homeostasis is not completely elucidated (43). Paradoxically, while in control animals, chronic blockade of A2A and A2B receptor induced insulin resistance in HSu animals, a model of insulin resistance, administration of these antagonists almost prevented insulin resistance, an effect similar between females and males. These results suggest that the beneficial effects of chronic caffeine consumption, a non-selective adenosine receptor antagonist, in lowering the risk of develop type 2 diabetes (5–7), might be mediated by adenosine A2 receptors, with a minor contribution from A1 adenosine receptors.
In control animals, chronic blockade of A1 receptor, showed a tendency to increase fasting glycaemia, which might suggest that in control animals, adenosine acting on A1 receptors might contribute to improve glucose metabolism. This is in agreement with the findings of Faulhaber-Walter et al. (11) evidencing that A1 receptor deletion impair glucose metabolism and with our results that show that blockade of A1 receptors worse insulin signaling dysfunction in the adipose tissue in HSu animals (Figure 7), but differ from the increase in insulin signaling pathways in control animals (Figure 7), reflecting once more the opposite effects in control vs. disease conditions. In contrast, blockade of A2A and A2B receptors showed a tendency to decrease the heightened levels of glucose induced by the HSu diet, which may be an indirect consequence of improved peripheral insulin sensitivity (Figure 1). We can conclude that adenosine receptors exert opposite actions in modulating insulin action in control and insulin resistant animals, with A2 chronic adenosine receptors antagonists promoting insulin resistance in control animals and rescuing this phenotype in insulin-resistance states.
Role of Adenosine Receptors in Weight, Fat Deposition, and Metabolism
The effect of adenosine and its receptors in weight and body fat deposition are not consensual, with some studies showing that the loss of adenosine A1 and A2B receptors promote an increase in weight gain and fat deposition (11, 44) and that long-term caffeine consumption is associated with weight loss in rodents and humans (45), effects that are not observed in other studies [e.g., Astrup et al. (29)]. Here, we show that in control animals, chronic blockade of A2B receptors increased weight gain with a higher impact in male animals (Figure 2), an effect that cannot be attributed to an increase in fat mass (Table 2). This effect is in agreement with the study by Csóka et al. (44), in where mice lacking A2B receptors increased weight gain, however due to an increase in retroperitoneal and epididymal fat mass. Surprisingly, chronic A2A blockade, increased total fat in female, while decreased total fat mass in male, which contrasts with the lack of effects of the deletion of A2A receptors in mice in body weight (46). As previously described, 4 weeks of HSu diet were unable to promote significant changes in weight gain (8, 47), being this effect also observed with the blockade of the adenosine receptors. However, even without any change in weight gain, chronic blockade of A1 receptors in HSu female animals decreased total fat mass, which was associated with a decrease in perienteric, genital, and perinephric fat mass, suggesting a redistribution of fat depots or an altered ratio lean/fat mass. This decrease in fat mass mediated by A1 adenosine receptors may be due to an increase in lipolysis or fat oxidation, effects that were described with chronic caffeine intake (45), but not to thermogenesis, since UCP1 expression in the adipose tissue was unaltered in these animals (Figure 7G). In male animals, blockade of A2A and A2B receptors increased total fat, which is associated with an increased in perinephric fat mass. These different effects of the adenosine receptor on weight gain and fat mass in female and male animals may be associated with hormonal and sex differences in body fat distribution and to a female higher lipolysis capacity (48, 49). Therefore, we can conclude that A2B receptors are involved in the control of weight, since the blockade of these receptors increases weigh gain, especially in males, an effect that is not correlated with an increase in fat mass. We can also conclude that A1 receptors are involved in fat metabolism in females and A2 receptors in males, as blockade of A1 receptors decreases fat mass deposition in females and A2A and A2B blockade increase fat deposition in males.
Role of Adenosine Receptors in the Control of Sympathetic Activity in Metabolic Diseases
One of the pathophysiological mechanisms described to be involved in the development of insulin resistance is the overactivation of the sympathetic nervous system (31). Herein, no sex differences were observed in the sympatho-adrenal activity in controls and HSu animals as well as in the effect of chronic administration of the different adenosine receptor antagonists in these parameters. We did not observe any significant change in circulating NE and Epi after the chronic administration of the different adenosine receptor antagonists, but the, chronic blockade of adenosine receptors increased adrenal medulla NE and Epi content in control and HSu animals (Figure 3). Under basal conditions, adenosine inhibited adrenal medulla catecholamine secretion, an effect partially achieved by the inhibitory effect of adenosine on the renin-angiotensin system and which is increased when sympathetic system is stimulated (50). Therefore, our results are in agreement with the data that shows that adenosine inhibits catecholamine secretion from adrenal medulla contrasting however with the findings that show that these effects are blunted with chronic caffeine consumption (50, 51), suggesting that the mechanisms of adaptation to caffeine are different or develop more faster than for A1 and A2B adenosine receptor blockers. Additionally, we can suggest that the increased content of catecholamines do not mean increased adrenal medulla secretion, as we did not observe any differences in catecholamines plasma levels (Figures 3A,B) and that is not key to the maintenance of insulin resistance, as HSu animals with adenosine receptors blocked exhibit high Epi/NE adrenal medulla content but normalized levels of insulin sensitivity.
Effect of Hypercaloric Hsu Diet on Insulin Sensitivity and Adenosine Receptor Expression
Herein we described for the first time that insulin resistance induced by HSu diet is associated with an increased expression of A1, A2A, and A2B receptors in the skeletal muscle, increased expression of A1 in the liver and in the adipose tissue and decreased expression of A2B adenosine receptors in the liver. The increase in A1 expression in the adipose tissue of HSu rats found in the present manuscript contrasts with the findings of Dhalla et al. (52) showing unaltered adenosine A1 receptor mRNA expression in Zucker diabetic fatty rat, but is consistent with the described role of A1 receptors in adipose tissue dysfunction (53). Also, in an obese mice model with insulin resistance induced by 16 weeks of high-fat diet, the expression of A2B adenosine receptor is increased in the liver, visceral fat, and gastrocnemius muscle (54). Based on this, we can postulate that the differences in the expression of adenosine receptors might be related with differences in the animal models studied, the HSu model studied in the present work is a lean model of insulin resistance (8, 47), or with the degree of insulin resistance and disease progression, as herein we used a 4 weeks model of diet and Johnston-Cox et al. (54) submitted the animals to 16 weeks of high-fat diet. Therefore, different metabolic disturbances and different stages of disease progression might contribute differently to the expression of adenosine receptor in the insulin sensitive tissues. As expected, and consistent with the application of chronic antagonists to a system, the chronic administration of DPCPX and SCH58261 increased A1 and A2A adenosine receptors in skeletal muscle and adipose tissue. Surprisingly, no alterations were found for the effect of adenosine antagonists in the liver and also for MRS1754, which decrease adenosine A2B receptor expression in adipose tissue, without any change in skeletal muscle and liver. In HSu animals, chronic administration of SCH58261 normalized the A2A adenosine receptor expression in skeletal muscle and in liver and the chronic MRS1754 administration normalized A2B adenosine receptors in skeletal muscle. Interestingly, in the liver, A2B adenosine receptors decreased with the HSu diet consumption, an effect that was normalized by the chronic MRS1754 administration.
We also found that the different insulin-sensitive tissues do not contribute equally or exhibit the same degree of impairment in insulin resistant states. In fact, it seems to be a notion that sometimes, and probably at the early stages of the disease, insulin resistance can be present in the absence of decreased insulin signaling [for a review see Fazakerley et al. (55)] with some authors showing evidences that the impairment in insulin action is independent of proximal elements of the insulin signaling pathway, but rather likely specific to the glucoregulatory branch of insulin signaling (55). In here, in the HSu model, obtained with 4 weeks of high-sucrose diet we found alterations in insulin signaling pathways only in the skeletal muscle, except for GLUT2 expression in the liver. One could expect also alterations in insulin signaling in the adipose tissue, but we can postulate that the mechanisms behind insulin resistance development might be different depending on the type of diet consumed and the time of exposure to the diets. In fact, it is consensual that the time-line for progression of insulin resistance in mice fed a high-fat diet starts with the development of insulin resistance in adipose tissue before the muscle (56, 57). However, the same might not be true for high sucrose diets at least when administrated during short periods of time. The liver is also another main insulin-sensitive tissue that is also involved in the development of insulin resistance (1). As previously described by our group, HSu diet did not modify extensively the expression of insulin signaling in the liver (39), since only decreased GLUT2. However, we cannot exclude possible major alterations in this tissue, as we know that insulin control of glycolysis and gluconeogenesis (1). Chronic administration of A2 adenosine antagonists produced the same effects in insulin signaling pathways, measured as insulin receptor expression and Glut4 transporters, in skeletal muscle, than in whole-body insulin sensitivity. Contrary results were found in the adipose tissue of control and HSu animals for chronic adenosine receptor antagonist, where the blockade of A1 receptors improved insulin signaling in control animals, but worsen insulin signaling in HSu animals. In contrast, no changes were found for the effect of adenosine antagonists in the liver in controls and HSu animals, suggesting that the role of adenosine in insulin action in the liver do not involve the pathways herein tested. Therefore, we can conclude that in HSu animals the effect of adenosine on insulin sensitivity is mainly mediated by A2 adenosine receptors in the skeletal muscle with a small contribution of A1 receptors in the adipose tissue.
Conclusion
In conclusion, all together, the results herein described suggest that A2 adenosine receptors in the skeletal muscle are the main responsibles for the whole-body insulin sensitivity, being therefore more relevant the effect of adenosine on skeletal muscle on insulin action than the effect of adenosine on adipose tissue in a context of lean insulin resistance. We can suggest that the targeting of A2 adenosine receptors might be useful to rescue insulin signaling pathways in insulin-resistant conditions.
Data Availability Statement
The datasets generated for this study are available on request to the corresponding author.
Ethics Statement
The animal study was reviewed and approved by NOVA Medical School Ethics Committee.
Author Contributions
JS and SC participated in research design and wrote or contributed to the writing of the manuscript. JS, FM, MR, and TR conducted experiments. JS, FM, PM, EO, and SC performed collection and data analysis. All the authors have approved the final version of the manuscript, and all persons designated as authors qualify for authorship, and all those who qualify for authorship are listed.
Funding
The present study was supported by the Portuguese Society of Diabetes. JS was supported by a PhD Grant from the Portuguese Foundation for Science and Technology, PD/BD/105890/2014.
Conflict of Interest
The authors declare that the research was conducted in the absence of any commercial or financial relationships that could be construed as a potential conflict of interest.
References
1. Bajaj M, Defronzo RA. Metabolic and molecular basis of insulin resistance. J Nucl Cardiol. (2003) 10:311–23. doi: 10.1016/s1071-3581(03)00520-8
2. Ng M, Fleming T, Robinson M, Thomson B, Graetz N, Margono C, et al. Global, regional, and national prevalence of overweight and obesity in children and adults during 1980–2013: a systematic analysis for the Global Burden of Disease Study 2013. Lancet. (2014) 384:766–81. doi: 10.1016/s0140-6736(14)60460-8
3. Kautzky-Willer A, Harreiter J, Pacini G. Sex and gender differences in risk, pathophysiology and complications of type 2 diabetes mellitus. Endocr Rev. (2016) 37:278–316. doi: 10.1210/er.2015-1137
4. Fredholm BB, Bättig K, Holmén J, Nehlig A, Zvartau EE. Actions of caffeine in the brain with special reference to factors that contribute to its widespreaduse. Pharmacol Rev. (1999) 51:83–133.
5. van Dam RM, Feskens EJ. Coffee consumption and risk of type 2 diabetes mellitus. Lancet. (2002) 360:1477–8. doi: 10.1016/S0140-6736(02)11436-X
6. Smith B, Wingard DL, Smith TC, Kritz-Silverstein D, Barrett-Connor E. Does coffee consumption reduce the risk of type 2 diabetes in individuals with impaired glucose? Diabetes Care. (2006) 29:2385–90. doi: 10.2337/dc06-1084
7. van Dam RM, Willett WC, Manson JE, Hu FB. Coffee, caffeine, and risk of type 2 diabetes: a prospective cohort study in younger and middle-aged U.S. women. Diabetes Care. (2006) 29:398–403. doi: 10.2337/diacare.29.02.06.dc05-1512
8. Conde SV, Nunes da Silva T, Gonzalez C, Mota Carmo M, Monteiro EC, Guarino MP. Chronic caffeine intake decreases circulating catecholamines and prevents diet-induced insulin resistance and hypertension in rats. Br J Nutr. (2012) 107:86–95. doi: 10.1017/S0007114511002406
9. Guarino MP, Ribeiro MJ, Sacramento JF, Conde SV. Chronic caffeine intake reverses age-induced insulin resistance in the rat: effect on skeletal muscle Glut4 transporters and AMPK activity. Age. (2013) 35:1755–65. doi: 10.1007/s11357-012-9475-x
10. Conde SV, Monteiro EC, Obeso A, Gonzalez C. Adenosine in peripheral chemoreception: new insights into a historically overlooked molecule–invited article. Adv Exp Med Biol. (2009) 648:145–59. doi: 10.1007/978-90-481-2259-2_17
11. Faulhaber-Walter R, Jou W, Mizel D, Li L, Zhang J, Kim SM, et al. Impaired glucose tolerance in the absence of adenosine A1 receptor signaling. Diabetes. (2011) 60:2578–87. doi: 10.2337/db11-0058
12. Figler RA, Wang G, Srinivasan S, Jung DY, Zhang Z, Pankow JS, et al. Links between insulin resistance, adenosine A2B receptors, and inflammatory markers in mice and humans. Diabetes. (2011) 60:669–79. doi: 10.2337/db10-1070
13. Espinal J, Challiss RA, Newsholme EA. Effect of adenosine deaminase and an adenosine analogue on insulin sensitivity in soleus muscle of the rat. FEBS Lett. (1983) 158:103–6. doi: 10.1016/0014-5793(83)80685-1
14. Leighton B, Lozeman FJ, Vlachonikolis IG, Challiss RA, Pitcher JA, Newsholme EA. Effects of adenosine deaminase on the sensitivity of glucose transport, glycolysis and glycogen synthesis to insulin in muscles of the rat. Int J Biochem. (1988) 20:23–7. doi: 10.1016/0020-711x(88)90005-5
15. Stace PB, Marchington DR, Kerbey AL, Randle PJ. Long term culture of rat soleus muscle in vitro. Its effects on glucose utilization and insulin sensitivity. FEBS Lett. (1990) 273:91–4. doi: 10.1016/0014-5793(90)81058-v
16. Challiss RA, Richards SJ, Budohoski L. Characterization of the adenosine receptor modulating insulin action in rat skeletal muscle. Eur J Pharmacol. (1992) 226:121–8. doi: 10.1016/0922-4106(92)90172-r
17. Vergauwen L, Hespel P, Richter EA. Adenosine receptors mediate synergistic stimulation of glucose uptake and transport by insulin and by contractions in rat skeletal muscle. J Clin Invest. (1994) 93:974–81. doi: 10.1172/JCI117104
18. Han DH, Hansen PA, Nolte LA, Holloszy JO. Removal of adenosine decreases the responsiveness of muscle glucose transport to insulin and contractions. Diabetes. (1998) 47:1671–5. doi: 10.2337/diabetes.47.11.1671
19. Thong FS, Lally JS, Dyck DJ, Greer F, Bonen A, Graham TE. Activation of the A1 adenosine receptor increases insulin-stimulated glucose transport in isolated rat soleus muscle. Appl Physiol Nutr Metab. (2007) 32:701–10. doi: 10.1139/H07-039
20. Gonzalez-Benitez E, Guinzberg R, Diaz-Cruz A, Pina E. Regulation of glycogen metabolism in hepatocytes through adenosine receptors. Role of Ca2+ and cAMP. Eur J Pharmacol. (2002) 437:105–11. doi: 10.1016/s0014-2999(02)01299-2
21. Harada H, Asano O, Hoshino Y, Yoshikawa S, Matsukura M, Kabasawa Y, et al. 2-Alkynyl-8-aryl-9- methyladenines as novel adenosine receptor antagonists: their synthesis and structure- activity relationships toward hepatic glucose production induced via agonism of the A2B receptor. J Med Chem. (2001) 44:170–9. doi: 10.1021/jm990499b
22. Yasuda N, Inoue T, Horizoe T, Nagata K, Minami H, Kawata T, et al. Functional characterization of the adenosine receptor contributing to glycogenolysis and gluconeogenesis in rat hepatocytes. Eur J Pharmacol. (2003) 459:159–66. doi: 10.1016/s0014-2999(02)02832-7
23. Xu B, Berkich DA, Crist GH, LaNoue KF. A1 adenosine receptor antagonism improves glucose tolerance in Zucker rats. Am J Physiol. (1998) 274:271–9. doi: 10.1152/ajpendo.1998.274.2.E271
24. Dong Q, Ginsberg HN, Erlanger BF. Overexpression of the A1 adenosine receptor in adipose tissue protects mice from obesity-related insulin resistance. Diabetes Obes Metab. (2001) 3:360–6. doi: 10.1046/j.1463-1326.2001.00158.x
25. Schoelch C, Kuhlmann J, Gossel M, Mueller G, Neumann-Haefelin C, Belz U, et al. Characterization of adenosine-A1 receptor-mediated antilipolysis in rats by tissue microdialysis, 1H-spectroscopy, and glucose clamp studies. Diabetes. (2004) 53:1920–6. doi: 10.2337/diabetes.53.7.1920
26. Dhalla AK, Santikul M, Smith M, Wong MY, Shryock JC, Belardinelli L. Antilipolytic activity of a novel partial A1 adenosine receptor agonist devoid of cardiovascular effects: comparison with nicotinic acid. J Pharmacol Exp Ther. (2007) 321:327–33. doi: 10.1124/jpet.106.114421
27. Johansson SM, Lindgren E, Yang JN, Herling AW, Fredholm BB. Adenosine A1 receptors regulate lipolysis and lipogenesis in mouse adipose tissue-interactions with insulin. Eur J Pharmacol. (2008) 597:92–101. doi: 10.1016/j.ejphar.2008.08.022
28. Acheson KJ, Zahorska-Markiewicz B, Pittet P, Anantharaman K, Jéquier E. Caffeine and coffee: their influence on metabolic rate and substrate utilization in normal weight and obese individuals. Am J Clin Nutr. (1980) 33:989–97.
29. Astrup A, Toubro S, Cannon S, Hein P, Breum L, Madsen J. Caffeine: a double-blind, placebo-controlled study of its thermogenic, metabolic, and cardiovascular effects in healthy volunteers. Am J Clin Nutr. (1990) 51:759–67. doi: 10.1093/ajcn/51.5.759
30. Lopez-Garcia E, van Dam RM, Rajpathak S, Willett WC, Manson JE, Hu FB. Changes in caffeine intake and long-term weight change in men and women. Am J Clin Nutr. (2006) 83:674–80. doi: 10.1093/ajcn.83.3.674
31. Esler M, Rumantir M, Wiesner G, Kaye D, Hastings J, Lambert G. Sympathetic nervous system and insulin resistance: from obesity to diabetes. Am J Hypertens. (2001) 14:304S−9S. doi: 10.1016/s0895-7061(01)02236-1
32. Thorp AA, Schlaich MP. Relevance of sympathetic nervous system activation in obesity and metabolic syndrome. J Diabetes Res. (2015) 2015:341583. doi: 10.1155/2015/341583
33. Conde SV, Ribeiro MJ, Melo BF, Guarino MP, Sacramento JF. Insulin resistance: a new consequence of altered carotid body chemoreflex? J Physiol. (2017) 595:31–41. doi: 10.1113/JP271684
34. Corti R, Binggeli C, Sudano I, Spieker L, Hänseler E, Ruschitzka F, et al. Coffee acutely increases sympathetic nerve activity and blood pressure independently of caffeine content: role of habitual versus nonhabitual drinking. Circulation. (2002) 106:2935–40. doi: 10.1161/01.cir.0000046228.97025.3a
35. Ribeiro RT, Lautt WW, Legare DJ, Macedo MP. Insulin resistance induced by sucrose feeding in rats is due to an impair- ment of the hepatic parasympathetic nerves. Diabetologia. (2005) 48:976–83. doi: 10.1007/s00125-005-1714-6
36. Ribeiro MJ, Sacramento JF, Gonzalez C, Guarino MP, Monteiro EC, Conde SV. Carotid body denervation prevents the development of insulin resistance and hypertension induced by hypercaloric diets. Diabetes. (2013) 62:2905–16. doi: 10.2337/db12-1463
37. Monzillo LU, Hamdy O. Evaluation of insulin sensitivity in clinical practice and in research settings. Nutr Rev. (2003) 61:397–412. doi: 10.1301/nr.2003.dec.397-412
38. Quintero M, Olea E, Conde SV, Obeso A, Gallego-Martin T, Gonzalez C, et al. Age protects from harmful effects produced by chronic intermittent hypoxia. J Physiol. (2016) 594:1773–90. doi: 10.1113/JP270878
39. Sacramento JF, Ribeiro MJ, Rodrigues T, Olea E, Melo BF, Guarino MP, et al. Functional abolishment of carotid body activity restores insulin action and glucose homeostasis: key roles for visceral adipose tissue and the liver. Diabetologia. (2017) 60:158–68. doi: 10.1007/s00125-016-4133-y
40. Matafome P, Rodrigues T, Pereira A, Letra L, Azevedo H, Paixão A, et al. Long-term globular adiponectin administration improves adipose tissue dysmetabolism in high-fat diet-fed Wistar rats. Arch Physiol Biochem. (2014) 120:147–57. doi: 10.3109/13813455.2014.950590
41. Tunçer S, Gurbanov R, Sheraj I, Solel E, Esenturk O, Banerjee S. Low dose dimethyl sulfoxide driven gross molecular changes have the potential to interfere with various cellular processes. Sci Rep. (2018) 8:14828. doi: 10.1038/s41598-018-33234-z
42. Bartness TJ, Vaughan CH, Song CK. Sympathetic and sensory innervation of brown adipose tissue. Int J Obes. (2010) 34(Suppl. 1):S36–42. doi: 10.1038/ijo.2010.182
43. Antonioli L, Blandizzi C, Csóka B, Pacher P, Haskó G. Adenosine signalling in diabetes mellitus-pathophysiology and therapeutic considerations. Nat Rev Endocrinol. (2015) 11:228–41. doi: 10.1038/nrendo.2015.10
44. Csóka B, Koscsó B, Töro G, Kókai E, Virág L, Németh ZH, et al. A2B adenosine receptors prevent insulin resistance by inhibiting adipose tissue inflammation via maintaining alternative macrophage activation Diabetes. (2014) 63:850–66. doi: 10.2337/db13-0573
45. Greenberg JA, Boozer CN, Geliebter A. Coffee, diabetes, and weight control. Am J Clin Nutr. (2006) 84:682–93. doi: 10.1093/ajcn/84.4.682
46. Gnad T, Scheibler S, von Kügelgen I, Scheele C, Kilić A, Glöde A, et al. Adenosine activates brown adipose tissue and recruits beige adipocytes via A2A receptors. Nature. (2014) 516:395–9. doi: 10.1038/nature13816
47. Melo BF, Sacramento JF, Ribeiro MJ, Prego CS, Correia MC, Coelho JC, et al. Evaluating the impact of different hypercaloric diets on weight gain, insulin resistance, glucose intolerance, and its comorbidities in rats. Nutrients. (2019) 11:1197. doi: 10.3390/nu11061197
48. Lladó I, Rodríguez-Cuenca S, Pujol E, Monjo M, Estrany ME, Roca P, et al. Gender effects on adrenergic receptor expression and lipolysis in white adipose tissue of rats. Obes Res. (2002) 10:296–305. doi: 10.1038/oby.2002.41
49. Shi H, Clegg DJ. Sex differences in the regulation of body weight. Physiol Behav. (2009) 97:199–204. doi: 10.1016/j.physbeh.2009.02.017
50. Tseng CJ, Chan JY, Lo WC, Jan CR. Modulation of catecholamine release by endogenous adenosine in the rat adrenal medulla. J Biomed Sci. (2001) 8:389–94. doi: 10.1007/bf02255947
51. Debrah K, Haigh R, Sherwin R, Murphy J, Kerr D. Effect of acute and chronic caffeine use on the cerebrovascular, cardiovascular and hormonal responses to orthostasis in healthy volunteers. Clin Sci. (1995) 89:475–80. doi: 10.1042/cs0890475
52. Dhalla AK, Santikul M, Chisholm JW, Belardinelli L, Reaven GM. Comparison of the antilipolytic effects of an A1 adenosine receptor partial agonist in normal and diabetic rats. Diabetes Obes Metab. (2008) 11:95–101. doi: 10.1111/j.1463-1326.2008.00902.x
53. Tozzi M, Novak I. Purinergic receptors in adipose tissue as potential targets in metabolic disorders. Front Pharmacol. (2017) 8:878. doi: 10.3389/fphar.2017.00878
54. Johnston-Cox H, Koupenova M, Yang D, Corkey B, Gokce N, Farb MG, et al. The A2b adenosine receptor modulates glucose homeostasis and obesity. PLoS ONE. (2012) 7:e40584. doi: 10.1371/journal.pone.0040584
55. Fazakerley DJ, Krycer JR, Kearney AL, Hocking SL, James DE. Muscle and adipose tissue insulin resistance: malady without mechanism? J Lipid Res. (2019) 60:1720–32. doi: 10.1194/jlr.R087510
56. Burchfield JG, Kebede MA, Meoli CC, Stöckli J, Whitworth PT, Wright AL, et al. High dietary fat and sucrose result in an extensive and time-dependent deterioration in health of multiple physiological systems in mice. J Biol Chem. (2018) 293:5731–45. doi: 10.1074/jbc.ra117.000808
Keywords: adenosine, adenosine receptors, insulin resistance, insulin signaling, adipose tissue, skeletal muscle, gender differences
Citation: Sacramento JF, Martins FO, Rodrigues T, Matafome P, Ribeiro MJ, Olea E and Conde SV (2020) A2 Adenosine Receptors Mediate Whole-Body Insulin Sensitivity in a Prediabetes Animal Model: Primary Effects on Skeletal Muscle. Front. Endocrinol. 11:262. doi: 10.3389/fendo.2020.00262
Received: 29 November 2019; Accepted: 08 April 2020;
Published: 28 April 2020.
Edited by:
Carolyn Mary Ecelbarger, Georgetown University, United StatesReviewed by:
Xavier Prieur, INSERM U1087 l'Unité de Recherche de l'Institut du Thorax, FranceAnne-Francoise Burnol, INSERM U1016 Institut Cochin, France
Copyright © 2020 Sacramento, Martins, Rodrigues, Matafome, Ribeiro, Olea and Conde. This is an open-access article distributed under the terms of the Creative Commons Attribution License (CC BY). The use, distribution or reproduction in other forums is permitted, provided the original author(s) and the copyright owner(s) are credited and that the original publication in this journal is cited, in accordance with accepted academic practice. No use, distribution or reproduction is permitted which does not comply with these terms.
*Correspondence: Silvia V. Conde, c2lsdmlhLmNvbmRlQG5tcy51bmwucHQ=