- 1Branislav Jankovic Immunology Research Centre, Institute of Virology, Torlak Vaccines and Sera, Belgrade, Serbia
- 2Department of Microbiology and Immunology, Faculty of Pharmacy, University of Belgrade, Belgrade, Serbia
- 3Department of Pathobiology, Faculty of Pharmacy, University of Belgrade, Belgrade, Serbia
The role of stress effector systems in the initiation and progression of multiple sclerosis (MS) and experimental autoimmune encephalomyelitis (EAE), the most commonly used experimental model of MS, has strongly been suggested. To corroborate this notion, alterations in activity of the sympathoadrenal and sympathoneural axes of sympathoadrenal system (a major communication pathway between the central nervous system and the immune system), mirrored in altered release of their end-point mediators (adrenaline and noradrenaline, respectively), are shown to precede (in MS) and/or occur during development of MS and EAE in response to immune cell activation (in early phase of disease) and disease-related damage of sympathoadrenal system neurons and their projections (in late phase of disease). To add to the complexity, innate immunity cells and T-lymphocytes synthesize noradrenaline that may be implicated in a local autocrine/paracrine self-amplifying feed-forward loop to enhance myeloid-cell synthesis of proinflammatory cytokines and inflammatory injury. Furthermore, experimental manipulations targeting noradrenaline/adrenaline action are shown to influence clinical outcome of EAE, in a disease phase-specific manner. This is partly related to the fact that virtually all types of cells involved in the instigation and progression of autoimmune inflammation and target tissue damage in EAE/MS express functional adrenoceptors. Although catecholamines exert majority of immunomodulatory effects through β2-adrenoceptor, a role for α-adrenoceptors in EAE pathogenesis has also been indicated. In this review, we summarize all aforementioned aspects of immunopathogenetic action of catecholamines in EAE/MS as possibly important for designing new strategies targeting their action to prevent/mitigate autoimmune neuroinflammation and tissue damage.
Introduction
Multiple sclerosis (MS) is one of the most common neurological disorders and cause of disability of young adults (1–3). Patogenetically, MS is the prototype of the autoimmune inflammatory diseases of the central nervous system (CNS), and is characterized by breakdown of the blood-brain barrier (BBB), neuroinflammation, and axonal damage (4, 5). Its pathogenesis is largely deciphered using experimental autoimmune encephalomyelitis (EAE), a group of neuroantigen-induced animal diseases (4). These models are mainly based on neuroinflammation induced by auto-reactive T helper (Th) cells (4, 6). Upon activation in draining lymph nodes (dLNs), neuroantigen-specific Th cells synthesize IL-17, IFN-γ and/or GM-CSF, and express chemokine receptors (CCR2, CCR6) to gain access in the CNS (4, 6, 7). In the CNS, upon reactivation by resident antigen-presenting cells (APCs), Th cells activate neighboring microglia and attract peripheral cells (T-cells, B-cells, inflammatory monocytes) to perpetuate neuroinflammation and cause demyelination (4, 7). With EAE development, apart from CD4+Foxp3+ regulatory T-cells (Tregs) (8), activated microglia may assume regulatory functions (through phagocytosis, anti-inflammatory mediator and growth factor release) to limit the CNS damage and promote recovery (9). Noteworthy, so far, no single experimental model covers the entire spectrum of MS immunopathological features (particularly role of CD8+ T-cells and B-cells in propagating inflammation and tissue damage in established MS), so the relevance of results from EAE models has to be critically validated (5).
MS is multifactorial disease involving genetic traits and non-genetic triggers (1, 2). Generally, physical and psychological stressors are important triggers of MS (10–12). To corroborate this notion, war veterans with stress-related disorders (associated with low levels of morning cortisol and elevated levels of noradrenaline), were found to exhibit the higher risk of being diagnosed with MS compared to those without any psychiatric disorders (13). However, not only does stress contribute to MS development, but the disease itself causes stress, creating a vicious cycle (10, 12, 14). Additionally, stress contributes to exacerbations of MS (12, 15). The pathogenetic role of stress has been ascribed not only to action of glucocorticoids, end-point mediators of hypothalamo-pituitary-adrenal system (16, 17), but also to catecholamines, end-point mediators of sympathoadrenal system consisting of sympathoneural (the key end-point mediator noradrenaline) and sympathoadrenal (the key end-point mediator adrenaline) axes (15, 16, 18). This review focuses the role of catecholamines in development of EAE/MS.
In MS, aside from sensory, motor and cognitive impairments, autonomic dysfunction (mirrored in fatigue, bladder, bowel, cardiovascular, and sexual disorders) considerably contributes to disability (19–21). It has been speculated that (i) altered sympathoadrenal system activity induced by various stressors, including the disease itself (in early phase and at the onset of exacerbations), and (ii) damage of central sympathoadrenal system neurons and their projections with the disease progression (12, 22–24) is not only consequence, but also mechanism involved in MS pathogenesis.
The sympathoadrenal system, a major communication pathway between the CNS and the immune system (25), originates from locus coeruleus (LC) (18, 26). Activation of LC leads to (i) central effects reflecting noradrenaline release (primarily via non-junctional varicosities to enable its action on non-neural cells) throughout the brain and spinal cord (SC), and (ii) peripheral effects due to release of catecholamines from adrenal medulla and sympathetic nerve fibers (18, 27–30). On the other hand, activation of peripheral immune cells activates sympathoadrenal system to secure control of the ongoing response (18, 31). Namely, cytokines released upon their activation signal to the sympathoadrenal system by stimulating proinflammatory mediator release from the CNS resident cells or by activation of afferent signaling pathways (32–36). In inflammatory autoimmune diseases, this activational effect is suggested to be superimposed on elevated sympathoadrenal system activity due to chronic stress and/or stressful adverse life events leading to its hyperactivity and proinflammatory action (31). In MS, the release of proinflammatory cytokines from activated immune cells in the CNS also contributes to sympathoadrenal hyperactivity (32, 33, 36). Stress-induced sympathoadrenal activation prior to these diseases is suggested to induce low grade self-perpetuating lymphoid tissue and systemic inflammation that further increases sympathoadrenal activity and alters immune system reactivity to enable autoreactive lymphocyte activation (31). This, in return, contributes to sympathoadrenal activation and the promotion of inflammation (31, 37). Adding to the complexity, innate and adaptive “catecholaminergic” immune cells also synthesize catecholamines (29, 30, 38) to regulate inflammatory/immune responses (39). However, in inflammatory autoimmune diseases, they may form an alternative catecholamine source with role in promotion of inflammation (40–43). Namely, activated immune cell-derived catecholamines are suggested to drive an autocrine/paracrine self-amplifying feed-forward loop to increase synthesis of proinflammatory cytokines in myeloid cells (41, 44). Thus, in early phases of the diseases neurocrine/endocrine- and autocrine/paracrine-derived catecholamines may synergistically act to promote inflammation.
Catecholamines exert immunomodulatory effects through β- and α-adrenoceptors expressed on almost all types of immune cells, but majority of their effects are β2-adrenoceptor-mediated (18, 25, 43, 45–60). Monocytes/macrophages, together with dendritic cells, constitute the mononuclear phagocyte system, which plays a key role in maintaining tissue integrity, its restoration after injury, and the initiation, direction and resolution of innate and adaptive immunity. Catecholamines modulate their activity in a context-dependent manner, so they exert both proinflammatory (61–63) and anti-inflammatory (64, 65) effects depending on a number of factors (25), including adrenoceptor subtype (66, 67), adrenoceptor agonist concentrations (68), and the timing of adrenoceptor engagement in relation to antigen stimulation (69). Thus, it seems obvious that their action in EAE/MS has to be disease phase-dependent. In this review, considering EAE/MS pathogenesis, catecholamine influence on microglia and Th17/Treg axis is focused. Several stress paradigms induce β-adrenoceptor antagonist (propranolol) preventable microglial activation and synthesis of inflammatory mediators exaggerating proinflammatory responses to subsequent immunological stimuli (70). β2-adrenoceptor activation in lipopolysaccharide-stimulated dendritic cells diminishes IL-12 secretion, leading to a shift in the IL-12/IL-23 ratio and thereby promotes the generation of CD4+ T cells that produce lower amounts of IFN-γ (Th1 signature cytokine) and higher levels of IL-17 (Th17 signature cytokine) (71).
Central Noradrenaline in Pathogenesis of EAE/MS
Human Data
It has been shown that in MS noradrenaline levels decrease in the tissue surrounding LC (72) reflecting the disease-induced neuronal damage in LC (72). At present, there is no data on effects of the disease on the other “descending catecholaminergic system neurons” projecting to the spinal cord. On the other hand, several studies showed that cerebrospinal fluid levels of noradrenaline metabolite 3-methoxy-4-hydroxyphenylglycol, a marker of central noradrenergic activity, do not change in MS (73, 74). Given that peripheral and central administration of cytokines to rodents increased noradrenaline synthesis and 3-methoxy-4-hydroxyphenylglycol levels in brain (75), it may be assumed that elevated noradrenaline synthesis and turnover in non-damaged brain structures overcame diminished noradrenaline synthesis in those affected by the disease. On the other hand, combination of lofepramine or maprotiline (noradrenaline reuptake inhibitors) with levodopa (after conversion to dopamine metabolizes to noradrenaline) exhibited therapeutic effects in MS (76). However, given that dopamine itself exerts beneficial effects on the disease (77), these effects cannot be ascribed to the rise in the central noradrenaline level. On the other hand, although combined treatment with lofepramine and phenylalanine (upstream noradrenaline precursor) was initially shown to moderate clinical symptoms of MS (78), follow-up rigorously controlled study put the benefits of this therapy into question (79). Additionally, there are limited and inconsistent data on the therapeutic effects of β2-adrenoceptor agonists, such as salbutamol (albuterol), in MS. Namely, depending on type and phase of MS both adverse and benefitial effects have been described (80–82). To potentiate need for further studies on role of central noradrenaline in MS, several studies provided evidence that anti-stress therapies, including exercise (83–85), mindfulness meditation (86–88), and yoga (89), moderate MS symptoms (depression, anxiety, fatigue, cognitive dysfunction). In the same line are data from a population-based study indicating that the incidence of MS was negatively associated with use of fenoterol, a β2-adrenoceptor agonist, but not salbutamol belonging to the same drug class (90). This was ascribed to differences in their functionality, as fenoterol differently from salbutamol significantly stimulates cyclic-adenosine monophosphate (90).
Animal Data
The study encompassing dogs suffering from EAE showed that cerebrospinal fluid and white matter noradrenaline levels rise early after the immunization, but decrease in the clinical phase of the disease (91). Consistently, decline in SC and/or brainstem noradrenaline concentration was found at the peak of EAE in Lewis and Dark Agouti (DA) rats (45, 92–94). Noradrenaline concentration in SC also decreased with EAE progression in C57BL/6 mice (72, 95). This was attributed to disease-related damage of LC noradrenergic neurons (72) and/or axonal damage in SC (92). Additionally, it was reported that electrolytic destruction of LC noradrenergic neurons attenuates the disease in Wistar rats (96). Furthermore, central noradrenaline depletion (decrease in noradrenaline level by ~85% without changes in dopamine) by intracisternal-ventricular 6-hydroxydopamine injections reduced motor deficit in Lewis EAE rats (97, 98). Conversely, in C57BL/6 mice developing chronic EAE, treatment with N-(2-chloroethyl)-N-ethyl-2 bromobenzylamine, selective neurotoxin for rodent LC neurons, exacerbated the disease (99). This discrepancy could be related to N-(2-chloroethyl)-N-ethyl-2 bromobenzylamine–induced increase in the central extraneuronal noradrenaline level due to its inflow from non-lesioned regions, so that noradrenaline levels were reduced by only 10–30% (100). Additionally, treatment with propranolol, a non-selective β-adrenoceptor antagonist, depending on its onset relative to immunization, produced different effects on clinical outcome of EAE in rats (101, 102). Propranolol treatment starting 3 days before immunization moderated clinical and histological picture of EAE in DA rats (102), whereas the treatment beginning at immunization prolonged the disease duration in Lewis rats (101). When administered over effector phase of EAE to Lewis rats, propranolol exacerbated the disease (103), or produced no effect (101), while propranolol treatment in DA rats starting before the onset of clinical EAE decreased the disease severity (45). Given that propranolol crosses the BBB (104), the latter findings were consistent with data indicating that chemical depletion of central noradrenaline starting before the effector phase of EAE may remove an effector amplification mechanism leading to suppression of the paralysis (97). The inconsistencies in data from different propranolol studies may be associated with differences in drug dose regimen and/or treatment onset/duration, as well as animal genetic makeup, immunization protocols (possibly affecting the kinetics in development of sympathoadrenal neuron damage).
The ameliorating effect of propranolol on the clinical outcome of EAE in DA rats was linked with upregulated expression of nuclear factor (erythroid-derived 2)-like 2 (Nrf2) and heme oxygenase-1, a Nrf2-regulated gene with a crucial role in the prevention of neuroinflammation (105, 106). This partly reflected propranolol-induced upregulation of CX3CR1, the receptor for fractalkine (CX3CL1), which activates the Nrf2 signaling in microglial cells to limit their activation (107). Nrf2 recognizes an enhancer sequence termed antioxidant response element that is present in the regulatory regions of over 250 genes (108), and is implicated in the modulation of inflammation through crosstalk with the transcription factor NF-κB, the principal regulator of inflammation (109). Consistently, compared with saline-injected controls, in propranolol-treated rats the frequencies of IL-1β- and IL-23-expressing cells among microglia, and microglia expression level of IL-6 and CCL-2, the chemokine recruiting inflammatory monocytes and T-cells to the sites of inflammation (110), was decreased (45). Additionally, in accordance with role of CX3CR1 in regulation of the expression of TAM receptors (111), which are essential in apoptotic cell phagocytosis (112), so that their deficiency is linked with autoimmune disease progression (110), the frequency of phagocytic cells among microglia was significantly increased in propranolol-treated rats (45). As expected (9, 113, 114), this correlated with the increased proportion of anti-inflammatory CD163- and IL-10-expressing microglia (45). In keeping with alterations in phenotypic and functional profile of microglia, in propranolol-treated EAE rats the infiltration of SC with blood-borne inflammatory monocytes and Th cells, their reactivation/proliferation and differentiation toward highly pathogenic IL-17/IFN-γ/GM-CSF co-producing Th17 cells was impaired (45).
On the other hand, administration of prazosin, an α1-adrenoceptor antagonist, throughout the disease or effector phase alone suppressed active and passively transferred EAE in rats (103, 115, 116). This was related to blockade of disease-promoting α1-adrenoceptor–mediated vascular action (115).
Putative Research Directions
Considering all the aforementioned, it is clear that many important issues still remain to be addressed to fully enlighten the role of sympathoadrenal system in EAE/MS pathogenesis, but to mention a few. To confirm changes in sympathoadrenal system reactivity during EAE development, noradrenaline concentration in SC along with development of sympathoadrenal neuron lesions, should be examined in distinct EAE models and distinct phases and types of MS. Additionally, considering that rodent microglia synthesize catecholamines (45), it should be investigated whether these cells, as macrophages (41), may enhance local inflammation by an autocrine/paracrine feedback mechanism. Furthermore, given that functional β1- and β2-adrenoceptors were revealed on microglia (46), further research to delineate β1-adrenoceptor-mediated from β2-adrenoceptor-mediated effects on microglia in this model is necessary. Moreover, given that microglia express α1-adrenoceptor (47), putative α1-adrenoceptor-mediated effects of catecholamines on microglia from EAE rats are also worth examining.
Peripheral Catecholamines in Pathogenesis of EAE/MS
Human Data
In favor of peripheral sympathoadrenal dysregulation in MS, in chronic progressive (CP) MS increase in circulating noradrenaline level was found (117). Differently, in relapsing-remitting (RR) MS its level is decreased (118). Additionally, in active RR MS, circulating levels of adrenaline and noradrenaline are lower than in stable disease (23). Alterations in lymphocyte catecholamine levels also occur in MS (119), so higher adrenaline in the first-attack MS patients and lower noradrenaline in RR MS were found (119). Higher noradrenaline level was also measured in peripheral blood mononuclear cells (PBMC) from MS patients (120). Additionally, upregulated β-adrenoceptor on T-lymphocytes from CP MS patients (121, 122), and on PBMC from RR and secondary progressive MS patients was reported (123–125). There is no data on the expression of α-adrenoceptors on peripheral immune cells from MS patients.
Animal Data
In Lewis EAE rats, splenic noradrenaline concentration decreased during the inductive phase of the disease (126). Our recent study demonstrated reduced noradrenaline concentration in dLNs from DA rats on the 7th day post-immunization (59). However, noradrenaline content was increased in dLN cells constituting, most likely, a compensatory mechanism (42, 127, 128). Early studies in active (129) and adoptively transferred (130) Lewis rat EAE showed more severe disease in adult rats subjected to 6-hydroxydopamine–induced sympathectomy at birth. These findings should be interpreted with caution, as neonatally administered 6-hydroxydopamine crosses the BBB (129), so aside from peripheral sympathectomy, it permanently increases noradrenergic innervation in hind brain (131). Administration of isoproterenol, a non-selective β-adrenergic agonist, during preclinical phase of EAE in Lewis rats suppressed the disease severity, while propranolol did not produce any effects (101). Conversely, we showed that propranolol administration throughout preclinical phase of EAE in DA rats moderated the disease severity (132). This discrepancy could be related to recent findings indicating that isoproterenol represents a novel type of α1A-adrenoceptor partial agonist (133), and differences in propranolol dose, particularly as in the rat there are strain differences in its metabolism. Furthermore, it has recently been reported that increased systemic noradrenaline levels due to sympathoneural system hyperactivity (134) in mice constitutively lacking α2a/c-adrenoceptor (constituting an important negative-feedback mechanism required for the presynaptic control of neurotransmitter release from sympathetic fibers) is associated with diminished pathogenic T-cell responses and CNS inflammation in EAE (135). These findings might be explained by data suggesting that prolonged sympathoneural activation (as it is in late phases of inflammatory autoimmune diseases) leads to anti-inflammatory sympathoneural action (31). The moderating effect of propranolol on clinical outcome of EAE in DA rat model was ascribed to diminished CD4+ T-cell activation/proliferation and Th17 cell generation in dLNs (132), due to impaired migration of neuroantigen-carrying APCs from the site of immunization to dLNs, reflecting decreased expression of CCL19/21, chemokines driving their migration in dLNs (132). On the other hand, study on propranolol effects on dLN cells recovered in the inductive phase of EAE in the presence of arterenol (synthetic noradrenaline) or its absence showed that it enhanced CD4+ cell IL-2 synthesis and proliferation (43). Additionally, propranolol augmented differentiation of Th17 cells in dLN cell cultures by increasing RORγt expression in CD4+ cells, and production of cytokines driving/maintaining Th17 cell differentiation (IL-1β and IL-23) by APCs (43). The discrepancy between the effects of propranolol in vivo and in vitro could be reconciled by data indicating that the number of autoantigen-carrying APCs in dLNs critically determines the magnitude of the primary (auto)reactive CD4+ T-cell response and clinical outcome of autoimmune responses (136, 137).
Until recently, role of α-adrenoceptor in EAE was exclusively related to the effector phase of the disease (103, 115, 138). Our recent study showed the expression of α1-adrenoceptor on Tregs (but not on effector CD4+ T-cells) and APCs from dLNs of DA rats in the inductive phase of EAE (59). More important, it showed that prazosin suppressed proliferation of neuroantigen-stimulated CD4+ T-cells in dLN cell cultures, by increasing the frequency of Tregs and their Foxp3 and TGF-β expression, and decreasing co-stimulatory molecule expression on APCs (59). Moreover, prazosin also decreased IL-1β and IL-23 production in EAE rat dLN cell cultures, and consequently the generation of Th17 cells, including the most pathogenic GM-CSF–producing ones (59).
Putative Research Directions
To fulfill composite picture of sympathoadrenal system modulation of EAE/MS development, several issues need to be resolved. In light of findings suggesting that sympathoneural changes in inflammatory autoimmune diseases may be organ-specific (31), it should be answered if sympathoadrenal influence on (auto)immune response in dLNs changes with progression of EAE, and at which time-point this change occurs, as well as to elucidate adrenoceptor types involved in immunoregulation. Furthermore, as human studies directly linking stress and (auto)immune response are lacking, owing to practical and ethical concerns (139), EAE models, despite limitations (5), should be considered for investigating role of stress in triggering MS, and particularly pharmacological treatments affecting catecholamine action trough distinct types of adrenoceptors. Considering that individual's elevated noradrenergic tone (e.g., due to genetics) may favor MS onset (140), such investigation should encompass animals of different genetic makeup.
Conclusions
Collectively, available data suggest that alterations in sympathoadrenal system activity due to premorbid/disease-induced stress and disease-associated sympathoneural damage contribute to EAE and possibly MS onset and development (affecting distinct types of immune cells, and particularly important microglia, as depicted in Figure 1), respectively. However, further research to elucidate noradrenaline/adrenaline immunomodulatory action in the target organ and lymphoid organs/blood, in distinct phases of EAE (and in distinct EAE models) and MS alike, is necessary to envisage significance of alterations in sympathoadrenal immunomodulatory action for susceptibility to/progression of EAE/MS, and consequently consider possibilities to manipulate catecholamine action to prevent/mitigate them. To emphasize significance of this research it should be pointed that adrenergic drugs are safe and cost-effective.
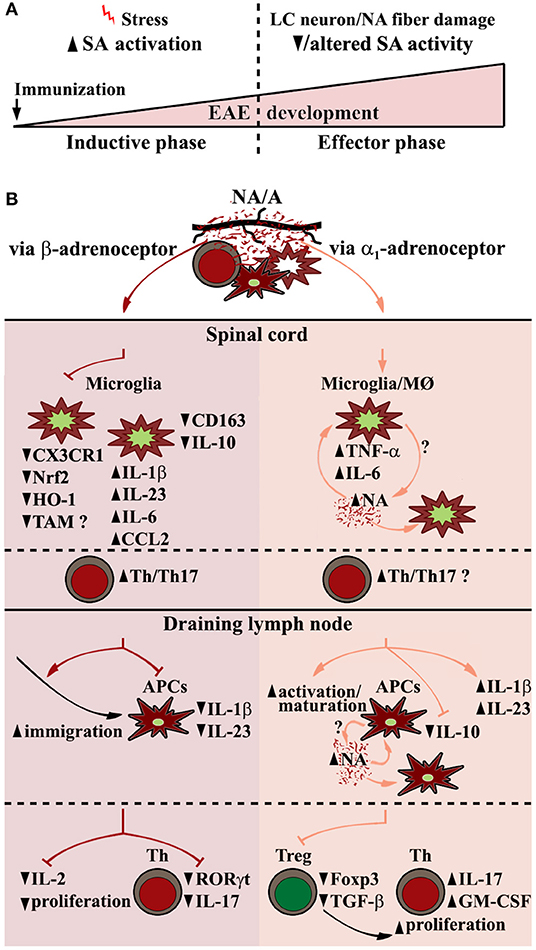
Figure 1. EAE-related alterations in sympathoadrenal system (SA) and putative central and peripheral effects of its key end-point mediators (noradrenaline and adrenaline) contributing to EAE pathogenesis. (A) Biphasic changes in SA over the course of EAE encompass SA overactivation in preclinical EAE stage (premorbid/disease-related stress), followed by its diminished and possibly qualitatively altered activity in clinical stage of the disease partly due to locus coeruleus (LC) neuron damage. (B) (Spinal cord) Central noradrenaline (NA)/adrenaline (A) acting through β-adrenoceptor in early phases of the disease downregulate microglial expression of nuclear factor (erythroid-derived 2)-like 2 (Nrf2), and its key anti-inflammatory downstream target genes, including those encoding heme oxygenase-1 (HO-1), and possibly TAM (Tyro3, Axl, and Mertk) receptors involved in phagocytosis via C-X3-C motif chemokine receptor 1 (CX3CR1)-dependent and CX3CR1-independent mechanisms. This leads to shift toward more proinflammatory microglial phenotype mirrored in increased expression of proinflammatory cytokines/chemokines (e.g., IL-1β, IL-23, IL-6, CCL2), followed by diminished expression of anti-inflammatory microglial markers (e.g., CD163, IL-10), and consequently, increased infiltration of spinal cord with Th cells, their reactivation/proliferation and differentiation toward pathogenic Th17 cells. (dLN, draining lymph node) NA/A acting through β-adrenoceptor enhance antigen-carrying antigen presenting cell (APC) migration to dLN, whereas impair their synthesis of Th17 polarizing cytokines (IL-1β, IL-23), and CD4+ cell expression of RORγt and their proliferation. On the other hand, α1-adrenoceptor–dependent stimulation leads to APC activation/maturation and augmented Th17-polarizing cytokine expression, followed by decrease in Foxp3+ Th cell (Treg) number and expression of Foxp3 and TGF-β leading to increased proliferation of Th cells and their IL-17/GM-CSF synthesis. Proinflammatory NA effects in myeloid cells, including microglia and macrophages (MØ) and peripheral APCs, may be self-amplified through a NA-α1-adrenoceptor loop.
Author Contributions
GL and IPi wrote the manuscript. All authors participated in data collection and interpretation, critically revised the manuscript, and approved the final version for submission.
Funding
This study was supported by the Ministry of Education, Science and Technological Development of the Republic of Serbia (Grant No. 175050).
Conflict of Interest
The authors declare that the research was conducted in the absence of any commercial or financial relationships that could be construed as a potential conflict of interest.
The reviewer DM declared a shared affiliation, with no collaboration, with the authors ZS-V and GL to the handling editor at the time of the review.
References
1. Filippi M, Bar-Or A, Piehl F, Preziosa P, Solari A, Vukusic S, et al. Multiple sclerosis. Nat Rev Dis Primers. (2018) 4:43. doi: 10.1038/s41572-018-0041-4
2. Al-Badri G, Castorina A. Insights into the role of neuroinflammation in the pathogenesis of multiple sclerosis. J Funct Morphol Kinesiol. (2018) 3:13. doi: 10.3390/jfmk3010013
3. Atlas of MS 2013: Mapping Multiple Sclerosis Around the World. London: Multiple Sclerosis International Federation (2013). Available online at: https://www.msif.org/about-us/who-we-are-and-what-we-do/advocacy/atlas/ (accessed September 6, 2019).
4. Constantinescu CS, Farooqi N, O'Brien K, Gran B. Experimental autoimmune encephalomyelitis (EAE) as a model for multiple sclerosis (MS). Br J Pharmacol. (2011) 164:1079–106. doi: 10.1111/j.1476-5381.2011.01302.x
5. Lassmann H, Bradl M. Multiple sclerosis: experimental models and reality. Acta Neuropathol. (2017) 133:223–44. doi: 10.1007/s00401-016-1631-4
6. Kurschus FC. T cell mediated pathogenesis in EAE: Molecular mechanisms. Biomed J. (2015) 38:183–93. doi: 10.4103/2319-4170.155590
7. Kara EE, McKenzie DR, Bastow CR, Gregor CE, Fenix KA, Ogunniyi AD, et al. CCR2 defines in vivo development and homing of IL-23-driven GM-CSF-producing Th17 cells. Nat Commun. (2015) 6:8644. doi: 10.1038/ncomms9644
8. Koutrolos M, Berer K, Kawakami N, Wekerle H, Krishnamoorthy G. Treg cells mediate recovery from EAE by controlling effector T cell proliferation and motility in the CNS. Acta Neuropathol Commun. (2014) 2:163. doi: 10.1186/s40478-014-0163-1
9. Thompson KK, Tsirka SE. The diverse roles of microglia in the neurodegenerative aspects of central nervous system (CNS) autoimmunity. Int J Mol Sci. (2017) 18:504. doi: 10.3390/ijms18030504
10. Briones-Buixassa L, Milà R, M Aragonès J, Bufill E, Olaya B, Arrufat FX. Stress and multiple sclerosis: a systematic review considering potential moderating and mediating factors and methods of assessing stress. Health Psychol Open. (2015) 2:2055102915612271. doi: 10.1177/2055102915612271
11. Stojanović L, Marisavljević D. Stress as a trigger of autoimmune disease. Autoimmun Rev. (2008) 7:209–13. doi: 10.1016/j.autrev.2007.11.007
12. Shepshelovich D, Shoenfeld Y. Prediction and prevention of autoimmune diseases: additional aspects of the mosaic of autoimmunity. Lupus. (2006) 15:183–90. doi: 10.1191/0961203306lu2274rr
13. O'Donovan A, Cohen BE, Seal KH, Bertenthal D, Margaretten M, Nishimi K, et al. Elevated risk for autoimmune disorders in iraq and afghanistan veterans with posttraumatic stress disorder. Biol Psychiatry. (2015) 77:365–74. doi: 10.1016/j.biopsych.2014.06.015
14. Carletto S, Borghi M, Scavelli F, Francone D, Perucchini ML, Cavallo M, et al. Prevalence of posttraumatic stress disorder in patients with multiple sclerosis. J Nerv Ment Dis. (2018) 206:149–51. doi: 10.1097/NMD.0000000000000780
15. Mohr DC, Hart SL, Julian L, Cox D, Pelletier D. Association between stressful life events and exacerbation in multiple sclerosis: a meta-analysis. BMJ. (2004) 328:731. doi: 10.1136/bmj.38041.724421.55
16. Gold SM, Mohr DC, Huitinga I, Flachenecker P, Sternberg EM, Heesen C. The role of stress-response systems for the pathogenesis and progression of MS. Trends Immunol. (2005) 26:644–52. doi: 10.1016/j.it.2005.09.010
17. Heesen C, Gold SM, Huitinga I, Reul JM. Stress and hypothalamic-pituitary-adrenal axis function in experimental autoimmune encephalomyelitis and multiple sclerosis - a review. Psychoneuroendocrinology. (2007) 32:604–18. doi: 10.1016/j.psyneuen.2007.05.002
18. Elenkov IJ, Wilder RL, Chrousos GP, Vizi ES. The sympathetic nerve–an integrative interface between two supersystems: the brain and the immune system. Pharmacol Rev. (2000) 52:595–638.
19. Haensch CA, Jörg J. Autonomic dysfunction in multiple sclerosis. J Neurol. (2006) 253:I3–9. doi: 10.1007/s00415-006-1102-2
20. Racosta JM, Kremenchutzky M. The role of autonomic dysregulation from pathophysiology to therapeutics of multiple sclerosis: a putative novel treatment target? J Neurol Neurophysiol. (2014) 5:212. doi: 10.4172/2155-9562.1000212
21. Pintér A, Cseh D, Sárközi A, Illigens BM, Siepmann T. Autonomic dysregulation in multiple sclerosis. Int J Mol Sci. (2015) 16:16920–52. doi: 10.3390/ijms160816920
22. Chelmicka Schorr E, Arnason BG. Nervous system-immune system interactions and their role in multiple sclerosis. Ann Neurol. (1994) 36(Suppl):S29–32. doi: 10.1002/ana.410360710
23. Flachenecker P, Reiners K, Krauser M, Wolf A, Toyka KV. Autonomic dysfunction in multiple sclerosis is related to disease activity and progression of disability. Mult Scler. (2001) 7:327–34. doi: 10.1177/135245850100700509
24. Merkelbach S, Haensch CA, Hemmer B, Koehler J, König NH, Ziemssen T. Multiple sclerosis and the autonomic nervous system. J Neurol. (2006) 253(Suppl 1):I21–5. doi: 10.1007/s00415-006-1105-z
25. Padro CJ, Sanders VM. Neuroendocrine regulation of inflammation. Semin Immunol. (2014) 26:357–68. doi: 10.1016/j.smim.2014.01.003
26. Samuels ER, Szabadi E. Functional neuroanatomy of the noradrenergic locus coeruleus: its roles in the regulation of arousal and autonomic function part I: principles of functional organisation. Curr Neuropharmacol. (2008) 6:235–53. doi: 10.2174/157015908785777229
27. Benarroch EE. The locus ceruleus norepinephrine system: functional organization and potential clinical significance. Neurology. (2009) 73:1699–704. doi: 10.1212/WNL.0b013e3181c2937c
28. O'Donnell J, Zeppenfeld D, McConnell E, Pena S, Nedergaard M. Norepinephrine: a neuromodulator that boosts the function of multiple cell types to optimize CNS performance. Neurochem Res. (2012) 37:2496–512. doi: 10.1007/s11064-012-0818-x
29. Deckx N, Lee WP, Berneman ZN, Cools N. Neuroendocrine immunoregulation in multiple sclerosis. Clin Dev Immunol. (2013) 2013:705232. doi: 10.1155/2013/705232
30. Miyake S. Mind over cytokines: crosstalk and regulation between the neuroendocrine and immune systems. Clin Exp Neuroimmunol. (2012) 3:1–15. doi: 10.1111/j.1759-1961.2011.00023.x
31. Bellinger DL, Lorton D. Sympathetic nerve hyperactivity in the spleen: causal for nonpathogenic-driven chronic immune-mediated inflammatory diseases (IMIDs)? Int J Mol Sci. (2018) 19:1188. doi: 10.3390/ijms19041188
32. Godinho-Silva C, Cardoso F, Veiga-Fernandes H. Neuro-immune cell units: a new paradigm in physiology. Annu Rev Immunol. (2019) 37:19–46. doi: 10.1146/annurev-immunol-042718-041812
33. Dantzer R. Neuroimmune interactions: from the brain to the immune system and vice versa. Physiol Rev. (2018) 98:477–504. doi: 10.1152/physrev.00039.2016
34. ThyagaRajan S, Priyanka HP. Bidirectional communication between the neuroendocrine system and the immune system: relevance to health and diseases. Ann Neurosci. (2012) 19:40–6. doi: 10.5214/ans.0972.7531.180410
35. Kaplin A, Bartner S. Reciprocal communication between the nervous and immune systems: crosstalk, back-talk and motivational speeches. Int Rev Psychiatry. (2005) 17:439–41. doi: 10.1080/02646830500381419
36. Besedovsky HO, del Rey A. Immune-neuro-endocrine interactions: facts and hypotheses. Endocr Rev. (1996) 17:64–102. doi: 10.1210/edrv-17-1-64
37. Racosta JM, Kimpinski K. Autonomic dysfunction, immune regulation, and multiple sclerosis. Clin Auton Res. (2016) 26:23–31. doi: 10.1007/s10286-015-0325-7
38. Jiang JL, Qiu YH, Peng YP, Wang JJ. Immunoregulatory role of endogenous catecholamines synthesized by immune cells. Sheng Li Xue Bao. (2006) 58:309–17.
39. Qiu YH, Peng YP, Jiang JM, Wang JJ. Expression of tyrosine hydroxylase in lymphocytes and effect of endogenous catecholamines on lymphocyte function. Neuroimmunomodulation. (2004) 11:75–83. doi: 10.1159/000075316
40. Kvetnansky R, Sabban EL, Palkovits M. Catecholaminergic systems in stress: structural and molecular genetic approaches. Physiol Rev. (2009) 89:535–606. doi: 10.1152/physrev.00042.2006
41. Flierl MA, Rittirsch D, Nadeau BA, Sarma JV, Day DE, Lentsch AB, et al. Upregulation of phagocyte-derived catecholamines augments the acute inflammatory response. PLoS ONE. (2009) 4:e4414. doi: 10.1371/journal.pone.0004414
42. Capellino S, Weber K, Gelder M, Härle P, Straub RH. First appearance and location of catecholaminergic cells during experimental arthritis and elimination by chemical sympathectomy. Arthritis Rheum. (2012) 64:1110–8. doi: 10.1002/art.33431
43. Vujnović I, Pilipović I, Jasnić N, Petrović R, Blagojević V, Arsenović-Ranin N, et al. Noradrenaline through β-adrenoceptor contributes to sexual dimorphism in primary CD4+ T-cell response in DA rat EAE model? Cell Immunol. (2019) 336:48–57. doi: 10.1016/j.cellimm.2018.12.009
44. Staedtke V, Bai RY, Kim K, Darvas M, Davila ML, Riggins GJ, et al. Disruption of a self-amplifying catecholamine loop reduces cytokine release syndrome. Nature. (2018) 564:273–7. doi: 10.1038/s41586-018-0774-y
45. Pilipović I, Stojić-Vukanić Z, Prijić I, Jasnić N, Leposavić G. Propranolol diminished severity of rat EAE by enhancing immunoregulatory/protective properties of spinal cord microglia. Neurobiol Dis. (2020) 134:104665. doi: 10.1016/j.nbd.2019.104665
46. Tanaka KF, Kashima H, Suzuki H, Ono K, Sawada M. Existence of functional beta1- and beta2-adrenergic receptors on microglia. J Neurosci Res. (2002) 70:232–7. doi: 10.1002/jnr.10399
47. Mori K, Ozaki E, Zhang B, Yang L, Yokoyama A, Takeda I, et al. Effects of norepinephrine on rat cultured microglial cells that express alpha1, alpha2, beta1 and beta2 adrenergic receptors. Neuropharmacology. (2002) 43:1026–34. doi: 10.1016/s0028-3908(02)00211-3
48. Maestroni GJ, Mazzola P. Langerhans cells beta 2-adrenoceptors: role in migration, cytokine production, Th priming and contact hypersensitivity. J Neuroimmunol. (2003) 144:91–9. doi: 10.1016/j.jneuroim.2003.08.039
49. Dimitrijević M, Pilipović I, Stanojević S, Mitić K, Radojević K, Pesić V, et al. Chronic propranolol treatment affects expression of adrenoceptors on peritoneal macrophages and their ability to produce hydrogen peroxide and nitric oxide. J Neuroimmunol. (2009) 211:56–65. doi: 10.1016/j.jneuroim.2009.03.014
50. Ramer-Quinn DS, Swanson MA, Lee WT, Sanders VM. Cytokine production by naive and primary effector CD4+ T cells exposed to norepinephrine. Brain Behav Immun. (2000) 14:239–55. doi: 10.1006/brbi.2000.0603
51. Loza MJ, Foster S, Peters SP, Penn RB. Beta-agonists modulate T-cell functions via direct actions on type 1 and type 2 cells. Blood. (2006) 107:2052–60. doi: 10.1182/blood-2005-08-3265
52. Guereschi MG, Araujo LP, Maricato JT, Takenaka MC, Nascimento VM, Vivanco BC, et al. Beta2-adrenergic receptor signaling in CD4+ Foxp3+ regulatory T cells enhances their suppressive function in a PKA-dependent manner. Eur J Immunol. (2013) 43:1001–12. doi: 10.1002/eji.201243005
53. Carvajal Gonczi CM, Tabatabaei Shafiei M, East A, Martire E, Maurice-Ventouris MHI, Darlington PJ. Reciprocal modulation of helper Th1 and Th17 cells by the β2-adrenergic receptor agonist drug terbutaline. FEBS J. (2017) 284:3018–28. doi: 10.1111/febs.14166
54. Liu Y, Rui XX, Shi H, Qiu YH, Peng YP. Norepinephrine inhibits Th17 cells via β2-adrenergic receptor (β2-AR) signaling in a mouse model of rheumatoid arthritis. Med Sci Monit. (2018) 24:1196–204. doi: 10.12659/msm.906184
55. Maestroni GJ. Dendritic cell migration controlled by alpha 1b-adrenergic receptors. J Immunol. (2000) 165:6743–7. doi: 10.4049/jimmunol.165.12.6743
56. Yanagawa Y, Matsumoto M, Togashi H. Enhanced dendritic cell antigen uptake via alpha2 adrenoceptor-mediated PI3K activation following brief exposure to noradrenaline. J Immunol. (2010) 185:5762–8. doi: 10.4049/jimmunol.1001899
57. Jetschmann JU, Benschop RJ, Jacobs R, Kemper A, Oberbeck R, Schmidt RE, et al. Expression and in-vivo modulation of alpha- and beta-adrenoceptors on human natural killer (CD16+) cells. J Neuroimmunol. (1997) 74:159–64. doi: 10.1016/S0165-5728(96)00221-4
58. Slota C, Shi A, Chen G, Bevans M, Weng NP. Norepinephrine preferentially modulates memory CD8 T cell function inducing inflammatory cytokine production and reducing proliferation in response to activation. Brain Behav Immun. (2015) 46:168–79. doi: 10.1016/j.bbi.2015.01.015
59. Pilipović I, Vujnović I, Stojić-Vukanić Z, Petrović R, Kosec D, Nacka-Aleksić M, et al. Noradrenaline modulates CD4+ T cell priming in rat experimental autoimmune encephalomyelitis: a role for the α1-adrenoceptor. Immunol Res. (2019) 67:223–40. doi: 10.1007/s12026-019-09082-y
60. Grisanti LA, Perez DM, Porter JE. Modulation of immune cell function by α(1)-adrenergic receptor activation. Curr Top Membr. (2011) 67:113–38. doi: 10.1016/B978-0-12-384921-2.00006-9
61. Torres MB, Vega VL, Bedri M, Saad D, Trentzsch H, Reeves RH, et al. IL-10 plasma levels are elevated after LPS injection in splenectomized A/J mice. J Surg Res. (2005) 129:101–6. doi: 10.1016/j.jss.2005.06.008
62. Flierl MA, Rittirsch D, Huber-Lang M, Sarma JV, Ward PA. Catecholamines-crafty weapons in the inflammatory arsenal of immune/inflammatory cells or opening pandora's box? Mol Med. (2008) 14:195–204. doi: 10.2119/2007-00105.Flierl
63. Grisanti LA, Evanson J, Marchus E, Jorissen H, Woster AP, DeKrey W, et al. Pro-inflammatory responses in human monocytes are β1-adrenergic receptor subtype dependent. Mol Immunol. (2010) 47:1244–54. doi: 10.1016/j.molimm.2009.12.013
64. Hu XX, Goldmuntz EA, Brosnan CF. The effect of norepinephrine on endotoxin-mediated macrophage activation. J Neuroimmunol. (1991) 31:35–42. doi: 10.1016/0165-5728(91)90084-k
65. van der Poll T. Effects of catecholamines on the inflammatory response. Sepsis. (2001) 4:159–67. doi: 10.1023/a:1011463006351
66. Hanke ML, Powell ND, Stiner LM, Bailey MT, Sheridan JF. Beta adrenergic blockade decreases the immunomodulatory effects of social disruption stress. Brain Behav Immun. (2012) 26:1150–9. doi: 10.1016/j.bbi.2012.07.011
67. Heijnen CJ, Rouppe van der Voort C, Wulffraat N, van der Net J, Kuis W, Kavelaars A. Functional alpha 1-adrenergic receptors on leukocytes of patients with polyarticular juvenile rheumatoid arthritis. J Neuroimmunol. (1996) 71:223–6. doi: 10.1016/s0165-5728(96)00125-7
68. Szelenyi I. Animal models of bronchial asthma. Inflamm Res. (2000) 49:639–54. doi: 10.1007/s000110050642
69. Sanders VM. The beta2-adrenergic receptor on T and B lymphocytes: do we understand it yet? Brain Behav Immun. (2012) 26:195–200. doi: 10.1016/j.bbi.2011.08.001
70. Wohleb ES, Hanke ML, Corona AW, Powell ND, Stiner LM, Bailey MT, et al. β-adrenergic receptor antagonism prevents anxiety-like behavior and microglial reactivity induced by repeated social defeat. J Neurosci. (2011) 31:6277–88. doi: 10.1523/JNEUROSCI.0450-11.2011
71. Takenaka MC, Araujo LP, Maricato JT, Nascimento VM, Guereschi MG, Rezende RM, et al. Norepinephrine controls effector T cell differentiation through β2-adrenergic receptor-mediated inhibition of NF-κB and AP-1 in dendritic cells. J Immunol. (2016) 196:637–44. doi: 10.4049/jimmunol.1501206
72. Polak PE, Kalinin S, Feinstein DL. Locus coeruleus damage and noradrenaline reductions in multiple sclerosis and experimental autoimmune encephalomyelitis. Brain. (2011) 134:665–77. doi: 10.1093/brain/awq362
73. Davidson D, Pullar IA, Mawdsley C, Kinloch N, Yates CM. Monoamine metabolites in cerebrospinal fluid in multiple sclerosis. J Neurol Neurosurg Psychiatry. (1977) 40:741–5. doi: 10.1136/jnnp.40.8.741
74. Markianos M, Koutsis G, Evangelopoulos ME, Mandellos D, Karahalios G, Sfagos C. Relationship of CSF neurotransmitter metabolite levels to disease severity and disability in multiple sclerosis. J Neurochem. (2009) 108:158–64. doi: 10.1111/j.1471-4159.2008.05750.x
75. Dunn AJ. Effects of cytokines and infections on brain neurochemistry. Clin Neurosci Res. (2006) 6:52–68. doi: 10.1016/j.cnr.2006.04.002
76. Berne-Fromell K, Fromell H, Lundkvist S, Lundkvist P. Is multiple sclerosis the equivalent of Parkinson's disease for noradrenaline? Med Hypotheses. (1987) 23:409–15. doi: 10.1016/0306-9877(87)90062-4
77. Pacheco R, Contreras F, Zouali M. The dopaminergic system in autoimmune diseases. Front Immunol. (2014) 5:117. doi: 10.3389/fimmu.2014.00117
78. Loder C, Allawi J, Horrobin DF. Treatment of multiple sclerosis with lofepramine, L-phenylalanine and vitamin B(12): mechanism of action and clinical importance: roles of the locus coeruleus and central noradrenergic systems. Med Hypotheses. (2002) 59:594–602. doi: 10.1016/s0306-9877(02)00261-x
79. Wade DT, Young CA, Chaudhuri KR, Davidson DLW. A randomised placebo controlled exploratory study of vitamin B-12, lofepramine, and L-phenylalanine (the “Cari Loder regime”) in the treatment of multiple sclerosis. J Neurol Neurosurg Psychiatry. (2002) 73:246–9. doi: 10.1136/jnnp.73.3.246
80. Axelrod S, Bielory L. Beta2-agonists and paresthesias in multiple sclerosis. Ann Allergy Asthma Immunol. (2007) 98:100. doi: 10.1016/S1081-1206(10)60871-X
81. Makhlouf K, Weiner HL, Khoury SJ. Potential of beta2-adrenoceptor agonists as add-on therapy for multiple sclerosis: focus on salbutamol (albuterol). CNS Drugs. (2002) 16:1–8. doi: 10.2165/00023210-200216010-00001
82. Khoury SJ, Healy BC, Kivisäkk P, Viglietta V, Egorova S, Guttmann CR, et al. A randomized controlled double-masked trial of albuterol add-on therapy in patients with multiple sclerosis. Arch Neurol. (2010) 67:1055–61. doi: 10.1001/archneurol.2010.222
83. Zimmer P, Bloch W, Schenk A, Oberste M, Riedel S, Kool J, et al. High-intensity interval exercise improves cognitive performance and reduces matrix metalloproteinases-2 serum levels in persons with multiple sclerosis: a randomized controlled trial. Mult Scler. (2018) 24:1635–44. doi: 10.1177/1352458517728342
84. Sandroff BM, Johnson CL, Motl RW. Exercise training effects on memory and hippocampal viscoelasticity in multiple sclerosis: a novel application of magnetic resonance elastography. Neuroradiology. (2017) 59:61–7. doi: 10.1007/s00234-016-1767-x
85. Feys P, Moumdjian L, Van Halewyck F, Wens I, Eijnde BO, Van Wijmeersch B, et al. Effects of an individual 12-week community-located “start-to-run” program on physical capacity, walking, fatigue, cognitive function, brain volumes, and structures in persons with multiple sclerosis. Mult Scler. (2019) 25:92–103. doi: 10.1177/1352458517740211
86. Gilbertson RM, Klatt MD. Mindfulness in motion for people with multiple sclerosis: a feasibility study. Int J MS Care. (2017) 19:225–31. doi: 10.7224/1537-2073.2015-095
87. Willekens B, Perrotta G, Cras P, Cools N. Into the moment: does mindfulness affect biological pathways in multiple sclerosis? Front Behav Neurosci. (2018) 12:103. doi: 10.3389/fnbeh.2018.00103
88. Pagnini F, Cavalera C, Rovaris M, Mendozzi L, Molinari E, Phillips D, et al. Longitudinal associations between mindfulness and well-being in people with multiple sclerosis. Int J Clin Health Psychol. (2019) 19:22–30. doi: 10.1016/j.ijchp.2018.11.003
89. Thakur P, Mohammad A, Rastogi YR, Saini RV, Saini AK. Yoga as an intervention to manage multiple sclerosis symptoms. J Ayurveda Integr Med. (2019). doi: 10.1016/j.jaim.2019.04.005. [Epub ahead of print].
90. Tsai CP, Lin FC, Lee CT. Beta2-adrenergic agonist use and the risk of multiple sclerosis: a total population-based case-control study. Mult Scler. (2014) 20:1593–601. doi: 10.1177/1352458514528758
91. Khoruzhaia TA, Saakov BA. Change in monoamine content and monoamine oxidase activity in brain structures during experimental allergic encephalomyelitis. Biull Eksp Biol Med. (1975) 79:80–2.
92. White SR, Bhatnagar RK, Bardo MT. Norepinephrine depletion in the spinal cord gray matter of rats with experimental allergic encephalomyelitis. J Neurochem. (1983) 40:1771–3. doi: 10.1111/j.1471-4159.1983.tb08156.x
93. Krenger W, Honegger CG, Feurer C, Cammisuli S. Changes of neurotransmitter systems in chronic relapsing experimental allergic encephalomyelitis in rat brain and spinal cord. J Neurochem. (1986) 47:1247–54. doi: 10.1111/j.1471-4159.1986.tb00747.x
94. Krenger W, Kabiersch A, Honegger CG. Monoamines and related substances in brainstem and spinal cord of Lewis rats during the attack and recovery of experimental autoimmune encephalomyelitis. Brain Res. (1989) 491:374–8. doi: 10.1016/0006-8993(89)90074-7
95. Musgrave T, Tenorio G, Rauw G, Baker GB, Kerr BJ. Tissue concentration changes of amino acids and biogenic amines in the central nervous system of mice with experimental autoimmune encephalomyelitis (EAE). Neurochem Int. (2011) 59:28–38. doi: 10.1016/j.neuint.2011.03.020
96. Jovanova-Nesic K, Nikolic V, Jankovic BD. Locus ceruleus and immunity. II. Suppression of experimental allergic encephalomyelitis and hypersensitivity skin reactions in rats with lesioned locus ceruleus. Int J Neurosci. (1993) 68:289–94. doi: 10.3109/00207459308994284
97. Karpus WJ, Konkol RJ, Killen JA. Central catecholamine neurotoxin administration. 1. Immunological changes associated with the suppression of experimental autoimmune encephalomyelitis. J Neuroimmunol. (1988) 18:61–73. doi: 10.1016/0165-5728(88)90135-X
98. Konkol RJ, Wesselmann U, Karpus WJ, Leo GL, Killen JA, Roerig DL. Suppression of clinical weakness in experimental autoimmune encephalomyelitis associated with weight changes, and post-decapitation convulsions after intracisternal-ventricular administration of 6-hydroxydopamine. J Neuroimmunol. (1990) 26:25–34. doi: 10.1016/0165-5728(90)90116-5
99. Simonini MV, Polak PE, Sharp A, McGuire S, Galea E, Feinstein DL. Increasing CNS noradrenaline reduces EAE severity. J Neuroimmune Pharmacol. (2010) 5:252–9. doi: 10.1007/s11481-009-9182-2
100. Ross SB, Stenfors C. DSP4, a selective neurotoxin for the locus coeruleus noradrenergic system. A review of its mode of action. Neurotox Res. (2015) 27:15–30. doi: 10.1007/s12640-014-9482-z
101. Chelmicka-Schorr E, Kwasniewski MN, Thomas BE, Arnason BGW. The β-adrenergic agonist isoproterenol suppresses experimental allergic encephalomyelitis in Lewis rats. J Neuroimmunol. (1989) 25:203–7. doi: 10.1016/0165-5728(89)90138-0
102. Dimitrijević M, Rauski A, Radojević K, Kosec D, Stanojević S, Pilipović I, et al. Beta-adrenoceptor blockade ameliorates the clinical course of experimental allergic encephalomyelitis and diminishes its aggravation in adrenalectomized rats. Eur J Pharmacol. (2007) 577:170–82. doi: 10.1016/j.ejphar.2007.08.021
103. Brosnan CF, Goldmuntz EA, Cammer W, Factor SM, Bloom BR, Norton WT. Prazosin, an alpha 1-adrenergic receptor antagonist, suppresses experimental autoimmune encephalomyelitis in the Lewis rat. Proc Natl Acad Sci USA. (1985) 82:5915–9. doi: 10.1073/pnas.82.17.5915
104. Neil-Dwyer G, Bartlett J, McAinsh J, Cruickshank JM. Beta-adrenoceptor blockers and the blood-brain barrier. Br J Clin Pharmacol. (1981) 11:549–53. doi: 10.1111/j.1365-2125.1981.tb01169.x
105. Lastres-Becker I, Innamorato NG, Jaworski T, Rábano A, Kügler S, Van Leuven F, et al. Fractalkine activates NRF2/NFE2L2 and heme oxygenase 1 to restrain tauopathy-induced microgliosis. Brain. (2014) 137:78–91. doi: 10.1093/brain/awt323
106. Lee H, Choi YK. Regenerative effects of heme oxygenase metabolites on neuroinflammatory diseases. Int J Mol Sci. (2019) 20:78. doi: 10.3390/ijms20010078
107. Castro-Sánchez S, García-Yagüe ÁJ, Kügler S, Lastres-Becker I. CX3CR1-deficient microglia shows impaired signalling of the transcription factor NRF2: implications in tauopathies. Redox Biol. (2019) 22:101118. doi: 10.1016/j.redox.2019.101118
108. Dinkova-Kostova AT, Kostov RV, Kazantsev AG. The role of Nrf2 signaling in counteracting neurodegenerative diseases. FEBS J. (2018) 285:3576–90. doi: 10.1111/febs.14379
109. Cuadrado A, Martin-Moldes Z, Ye J, Lastres-Becker I. Transcription factors NRF2 and NF-kappaB are coordinated effectors of the Rho family, GTP-binding protein RAC1 during inflammation. J Biol Chem. (2014) 289:15244–58. doi: 10.1074/jbc.M113.540633
110. Hinojosa AE, Garcia-Bueno B, Leza JC, Madrigal JL. CCL2/MCP-1 modulation of microglial activation and proliferation. J Neuroinflammation. (2011) 8:77. doi: 10.1186/1742-2094-8-77
111. Ji R, Meng L, Li Q, Lu Q. TAM receptor deficiency affects adult hippocampal neurogenesis. Metab Brain Dis. (2015) 30:633–44. doi: 10.1007/s11011-014-9636-y
112. Rothlin CV, Lemke G. TAM receptor signaling and autoimmune disease. Curr Opin Immunol. (2010) 22:740–6. doi: 10.1016/j.coi.2010.10.001
113. Zhang Z, Zhang ZY, Wu Y, Schluesener HJ. Lesional accumulation of CD163+ macrophages/microglia in rat traumatic brain injury. Brain Res. (2012) 1461:102–10. doi: 10.1016/j.brainres.2012.04.038
114. Ahn M, Yang W, Kim H, Jin JK, Moon C, Shin T. Immunohistochemical study of arginase-1 in the spinal cords of Lewis rats with experimental autoimmune encephalomyelitis. Brain Res. (2012) 1453:77–86. doi: 10.1016/j.brainres.2012.03.023
115. Brosnan CF, Sacks HJ, Goldschmidt RC, Goldmuntz EA, Norton WT. Prazosin treatment during the effector stage of disease suppresses experimental autoimmune encephalomyelitis in the Lewis rat. J Immunol. (1986) 137:3451–6.
116. White SR, Black PC, Samathanam GK, Paros KC. Prazosin suppresses development of axonal damage in rats inoculated for experimental allergic encephalomyelitis. J Neuroimmunol. (1992) 39:211–8. doi: 10.1016/0165-5728(92)90255-J
117. Karaszewski JW, Reder AT, Anlar B, Arnason GW. Increased high affinity beta-adrenergic receptor densities and cyclic AMP responses of CD8 cells in multiple sclerosis. J Neuroimmunol. (1993) 43:1–7. doi: 10.1016/0165-5728(93)90068-A
118. Mel'nikov MV, Belousova OO, Zhetishev RR, Pashchenkov MV, Boiko AN. Effects of catecholamines on Th17 cells in multiple sclerosis. Neurosci Behav Phys. (2018) 48:342–5. doi: 10.1007/s11055-018-0568-6
119. Rajda C, Bencsik K, Vécsei LL, Bergquist J. Catecholamine levels in peripheral blood lymphocytes from multiple sclerosis patients. J Neuroimmunol. (2002) 124:93–100. doi: 10.1016/S0165-5728(02)00002-4
120. Cosentino M, Zaffaroni M, Marino F, Bombelli R, Ferrari M, Rasini E, et al. Catecholamine production and tyrosine hydroxylase expression in peripheral blood mononuclear cells from multiple sclerosis patients: effect of cell stimulation and possible relevance for activation-induced apoptosis. J Neuroimmunol. (2002) 133:233–40. doi: 10.1016/S0165-5728(02)00372-7
121. Arnason BG, Brown M, Maselli R, Karaszewski J, Reder A. Blood lymphocyte beta-adrenergic receptors in multiple sclerosis. Ann N Y Acad Sci. (1988) 540:585–8. doi: 10.1111/j.1749-6632.1988.tb27181.x
122. Karaszewski JW, Reder AT, Maselli R, Brown M, Arnason BG. Sympathetic skin responses are decreased and lymphocyte beta-adrenergic receptors are increased in progressive multiple sclerosis. Ann Neurol. (1990) 27:366–72. doi: 10.1002/ana.410270404
123. Zoukos Y, Leonard JP, Thomaides T, Thompson AJ, Cuzner ML. beta-Adrenergic receptor density and function of peripheral blood mononuclear cells are increased in multiple sclerosis: a regulatory role for cortisol and interleukin-1. Ann Neurol. (1992) 31:657–62. doi: 10.1002/ana.410310614
124. Zoukos Y, Thomaides TN, Kidd D, Cuzner ML, Thompson A. Expression of beta2 adrenoreceptors on peripheral blood mononuclear cells in patients with primary and secondary progressive multiple sclerosis: a longitudinal six month study. J Neurol Neurosurg Psychiatry. (2003) 74:197–202. doi: 10.1136/jnnp.74.2.197
125. Zoukos Y, Kidd D, Woodroofe MN, Kendall BE, Thompson AJ, Cuzner ML. Increased expression of high affinity IL-2 receptors and beta-adrenoceptors on peripheral blood mononuclear cells is associated with clinical and MRI activity in multiple sclerosis. Brain. (1994) 117:307–15. doi: 10.1093/brain/117.2.307
126. Mackenzie FJ, Leonard JP, Cuzner ML. Changes in lymphocyte beta-adrenergic receptor density and noradrenaline content of the spleen are early indicators of immune reactivity in acute experimental allergic encephalomyelitis in the Lewis rat. J Neuroimmunol. (1989) 23:93–100. doi: 10.1016/0165-5728(89)90027-1
127. Huang HW, Fang XX, Wang XQ, Peng YP, Qiu YH. Regulation of differentiation and function of helper T cells by lymphocyte-derived catecholamines via α1-and β2-adrenoceptors. Neuroimmunomodulation. (2015) 22:138–51. doi: 10.1159/000360579
128. Huang HW, Zuo C, Chen X, Peng YP, Qiu YH. Effect of tyrosine hydroxylase overexpression in lymphocytes on the differentiation and function of T helper cells. Int J Mol Med. (2016) 38:635–42. doi: 10.3892/ijmm.2016.2639
129. Chelmicka-Schorr E, Checinski M, Arnason BG. Chemical sympathectomy augments the severity of experimental allergic encephalomyelitis. J Neuroimmunol. (1988) 17:347–50. doi: 10.1016/0165-5728(88)90125-7
130. Chelmicka-Schorr E, Kwasniewski MN, Wollmann RL. Sympathectomy augments adoptively transferred experimental allergic encephalomyelitis. J Neuroimmunol. (1992) 37:99–103. doi: 10.1016/0165-5728(92)90160-M
131. Konkol RJ, Bendeich EG, Breese GR. A biochemical and morphological study of the altered growth pattern of central catecholamine neurons following 6-hydroxydopamine. Brain Res. (1978) 140:125–35. doi: 10.1016/0006-8993(78)90242-1
132. Pilipović I, Vujnović I, Petrović R, Stojić-Vukanić Z, Leposavić G. Propranolol impairs primary immune responses in rat experimental autoimmune encephalomyelitis. Neuroimmunomodulation. (2019) 27:1–10. doi: 10.1159/000500094
133. Copik AJ, Baldys A, Nguyen K, Sahdeo S, Ho H, Kosaka A, et al. Isoproterenol acts as a biased agonist of the alpha-1a-adrenoceptor that selectively activates the MAPK/ERK pathway. PLoS ONE. (2015) 10:e0115701. doi: 10.1371/journal.pone.0115701
134. Hein L, Altman JD, Kobilka BK. Two functionally distinct alpha2-adrenergic receptors regulate sympathetic neurotransmission. Nature. (1999) 402:181–4. doi: 10.1038/46040
135. Araujo LP, Maricato JT, Guereschi MG, Takenaka MC, Nascimento VM, de Melo FM, et al. The sympathetic nervous system mitigates CNS autoimmunity via β2-adrenergic receptor signaling in immune cells. Cell Rep. (2019) 28:3120–30.e5. doi: 10.1016/j.celrep.2019.08.042
136. Allenspach EJ, Lemos MP, Porrett PM, Turka LA, Laufer TM. Migratory and lymphoid-resident dendritic cells cooperate to efficiently prime naive CD4 T cells. Immunity. (2008) 29:795–806. doi: 10.1016/j.immuni.2008.08.013
137. Ludewig B, Junt T, Hengartner H, Zinkernagel RM. Dendritic cells in autoimmune diseases. Curr Opin Immunol. (2001) 13:657–62. doi: 10.1016/S0952-7915(01)00275-8
138. Haerter K, Vroon A, Kavelaars A, Heijnen CJ, Limmroth V, Espinosa E, et al. In vitro adrenergic modulation of cellular immune functions in experimental autoimmune encephalomyelitis. J Neuroimmunol. (2004) 146:126–32. doi: 10.1016/j.jneuroim.2003.10.051
139. Padgett DA, Glaser R. How stress influences the immune response. Trends Immunol. (2003) 24:444–8. doi: 10.1016/S1471-4906(03)00173-X
Keywords: sympathoadrenal system, noradrenaline, β-adrenoceptor, α-adrenoceptor, experimental autoimmune encephalomyelitis, multiple sclerosis
Citation: Pilipović I, Stojić-Vukanić Z, Prijić I and Leposavić G (2020) Role of the End-Point Mediators of Sympathoadrenal and Sympathoneural Stress Axes in the Pathogenesis of Experimental Autoimmune Encephalomyelitis and Multiple Sclerosis. Front. Endocrinol. 10:921. doi: 10.3389/fendo.2019.00921
Received: 16 September 2019; Accepted: 17 December 2019;
Published: 14 January 2020.
Edited by:
Ana Rosa Pérez, National Council for Scientific and Technical Research (CONICET), ArgentinaReviewed by:
Djordje Miljkovic, Institute for Biological Research Sinisa Stankovic, University of Belgrade, SerbiaJames William Crane, University of Tasmania, Australia
Copyright © 2020 Pilipović, Stojić-Vukanić, Prijić and Leposavić. This is an open-access article distributed under the terms of the Creative Commons Attribution License (CC BY). The use, distribution or reproduction in other forums is permitted, provided the original author(s) and the copyright owner(s) are credited and that the original publication in this journal is cited, in accordance with accepted academic practice. No use, distribution or reproduction is permitted which does not comply with these terms.
*Correspondence: Gordana Leposavić, Z29yZGFuYS5sZXBvc2F2aWMmI3gwMDA0MDtwaGFybWFjeS5iZy5hYy5ycw==