- 1Unidad de Gestión Clínica Endocrinología y Nutrición, Instituto de Investigación Biomédica de Málaga—IBIMA, Hospital Universitario Virgen de la Victoria, Universidad de Málaga, Málaga, Spain
- 2Centro de Investigación Biomédica en Red (CIBER) Fisiopatología de la Obesidad y Nutrición (CIBEROBN), Instituto de Salud Carlos III (ISCIII), Málaga, Spain
Obesity is one of the most serious worldwide epidemics of the twenty-first century according to the World Health Organization. Frequently associated with a number of comorbidities, obesity threatens and compromises individual health and quality of life. Bariatric surgery (BS) has been demonstrated to be an effective treatment to achieve not only sustained weight loss but also significant metabolic improvement that goes beyond mere weight loss. The beneficial effects of BS on metabolic traits are so widely recognized that some authors have proposed BS as metabolic surgery that could be prescribed even for moderate obesity. However, most of the BS procedures imply malabsorption and/or gastric acid reduction which lead to nutrient deficiency and, consequently, further complications could be developed in the long term. In fact, BS not only affects metabolic homeostasis but also has pronounced effects on endocrine systems other than those exclusively involved in metabolic function. The somatotropic, corticotropic, and gonadal axes as well as bone health have also been shown to be affected by the various BS procedures. Accordingly, further consequences and complications of BS in the long term in systems other than metabolic system need to be addressed in large cohorts, taking into account each bariatric procedure before making generalized recommendations for BS. In this review, current data regarding these issues are summarized, paying special attention to the somatotropic, corticotropic, gonadal axes, and bone post-operative health.
Introduction
Obesity is a chronic, progressive, and multifactorial disease involving genetic, metabolic, psychological, and endocrinology-related factors, among others. Obesity-associated comorbidities are numerous and are also related to higher mortality. Obesity is a risk factor for a number of other chronic illnesses related to metabolic syndrome including type 2 diabetes mellitus (T2DM), high blood pressure, dyslipidemia, cardiovascular diseases (CVD), respiratory disorders, joint diseases, psychosocial disorders, and even several types of cancer (including esophagus, colon, pancreas, prostate, and breast) (1).
The World Health Organization (WHO) has defined the term “obesity” as excessive fat accumulation that is harmful to health. Considered the twenty-first century epidemic, obesity is the main health problem in developed countries, reducing life expectancy and presenting a challenge for the global economy. This is a disease with exponential growth, and its rise and prevalence is also affecting the child population. In the last 40 years the proportion of the population that is obese has tripled. In 2016, 39% of adults aged 18 years and over were overweight and about 13% were obese. If nothing changes, the growing trend will continue and these numbers will increase in the coming years (2).
Bariatric Surgery (BS): A Treatment for Obesity
From the point of view of therapeutics, proper dietary re-education, together with lifestyle modification and physical exercise as well as psychological specialized support for obesity treatment are needed. However, studies such as Look AHEAD showed that weight loss and glycemic control is difficult to maintain in the long term, even with intensive lifestyle intervention (3). Thus, bariatric surgery (BS) is the most effective therapy in the long term for severely obese patients (grade II) with associated metabolic diseases and for morbidly and super morbidly obese patients (grades III-IV). In addition, there is increasing literature that supports the inclusion of BS for the treatment of T2DM and obese patients (1). In this context, a global approach developed by a multidisciplinary unit, which allows a personalized and comprehensive treatment for obese patients as well as the selection of those patients who can be benefit from surgical treatment is extremely relevant.
There are different effective surgical methods employed for obesity treatment and the majority of the most popular bariatric procedures such as Roux-en-Y gastric bypass (RYGB), sleeve gastrectomy (SG), mini-gastric bypass, and biliopancreatic diversion (BPD) are considered safe procedures that are efficient regarding obesity-associated comorbidities and weight loss (4). Nevertheless, there are some differences between bariatric procedures related to the magnitude of changes observed, complication rates or post-surgery morbidities in the short and long term (reintervention rates after surgery, gastroesophageal reflux disease). Due to these differences, an individualized treatment taking into account the characteristics of each patient is necessary (5–8).
After BS, many aspects are modified and imply a reduction in the risk of obesity-associated disorders as well as of all-cause mortality (9–11). Some of the beneficial effects of BS are improved physical function (12), sustained weight loss, reduction of comorbidities such as osteoarthritis and respiratory dysfunction (13), a more favorable metabolic profile, which implies an improvement in quality of life, and the resolution of cardiovascular risk factors (14–19) with lower triglyceride levels and higher HDL-C levels in most patients 1 year after surgery (20, 21).
Although there is extensive literature that claims beneficial health effects with BS, and the post-surgery mortality rate is <1%, these type of procedures are not exempt from long-term complications related to nutritional deficiencies. In general, mainly malabsorptive procedures, e.g., bypass procedures with duodenal exclusion of nutrients and a concomitant decrease in gastric acid, will have a higher risk of micronutrient and macronutrient deficiencies. Consequently, BS patients are at higher risk of developing nutrient deficiency-related disorders such as anemia, certain types of neuropathies or osteoporosis (22, 23).
Effects of BS on Glycemic Profile and Mechanisms Involved in T2DM Resolution
A number of studies have explored metabolic changes after BS, and the efficiency of BS for the treatment of T2DM is well-established. BS therefore offers a safe and more effective alternative to achieve sustained glycemic control in diabetic obese patients in comparison with intensive medical treatment (24–26), which involves a decrease in chronic micro- and macroangiopathic complications (27).
The various surgical procedures usually employed in BS lead to partial or total T2DM remission of about 34–85.3% depending on the criteria applied to define T2DM remission, and 95% global success in glycemic control (28). When the different bariatric procedures are compared, biliopancreatic diversion (BPD) is the most effective for T2DM treatment, but this is a more complex procedure with higher surgical adverse event rates compared to other bariatric procedures (29). Risstad et al. reported better outcomes related to sustained weight loss, glycemic control and improvement in lipid profile after BPD than RYGB 5 years after surgery (14). Garrido-Sanchez et al. concluded that BPD takes less time to achieve insulin resistance improvement than SG (30).
RYGB is also a highly effective option for T2DM remission. Most patients who underwent RYGB did not require hypoglycemic drugs 1 year post-surgery, and it has been reported 84–90% and 29–50% T2DM remission one and 5 years after RYGB, respectively (31–35). However, T2DM remission rates in the literature vary. Yan et al. reported 56.81% (36.8–90.3%) T2DM remission in their meta-analysis (36), while Chang et al. found 95.15% (88.38–98.8%) T2DM remission (37).
Sleeve gastrectomy has gained popularity and appears to achieve glycemic control rates similar to RYGB but with fewer surgery-associated complications. The randomized controlled trial SMBOSS reported 60% T2DM remission rates after SG compared to 77% remission after RYGB 3 years after surgery (15). The STAMPEDE study compared the efficacy of intensive medical therapy alone or intensive medical therapy plus RYGB or plus SG (5-year follow-up) finding that, 28.6% of patients who underwent RYGB achieved HbA1c ≤6% in comparison with 23.4% of patients who underwent SG and 5.3% of patients who only received intensive medical therapy. The average improvement in HbA1c was 2.1% in surgery cohorts vs. 0.3% in intensive medical treatment cohorts (25). The SLEEVEPASS study showed that the effects of T2DM remitted completely or partially in 49% of patients who underwent SG and in 57% who underwent RYGB with no significant differences between procedures (16). However, it should be mentioned that the comparison between these two procedures based on the current literature is difficult due to study heterogeneity and because some of them are biased. Thus, their findings should be interpreted cautiously and further randomized controlled trials with a careful design are necessary (38).
Mechanisms for T2DM Remission After BS
Many explanations have been put forth for the metabolic improvements after BS. Early and pronounced improvement in hepatic insulin sensitivity after RYGB, which could be related to caloric restriction in the short-term post-operative period and the reduction in intrahepatic fat, has been reported. Moreover, an improvement in peripheral insulin sensitivity, which occurs later, and its relationship with the sustained weight loss has been described (39, 40).
Nevertheless, it is well-known that the metabolic improvements after BS take place in the days or weeks after surgery even before significant weight loss occurs (21, 41, 42). Therefore, mechanisms other than weight loss that cannot be explained solely by caloric restriction are likely triggered after BS and could account for the beneficial metabolic changes observed. This has promoted the use of the term “metabolic surgery” for this type of procedures (43).
Several hypotheses have been put forth to explain the early improvement in carbohydrate metabolism after BS, but the precise mechanisms for T2DM resolution are not yet completely understood (Figure 1). Among the factors which could be determining these improvements, in addition to caloric and excess nutrient restriction and the weight loss associated with food malabsorption, are changes in nutrient detection, improvements in pancreatic islet function, modulation of neural mechanisms and the secretion of gastrointestinal hormones implicated in energy and glucose homeostasis (e.g., glucagon-like peptide-1 (GLP1), peptide YY (PYY), cholecystokinin (CKK), ghrelin (44), glucagon (45, 46), obestatin levels (47), alteration in circulating adipokines (leptin decrease and adiponectin increase) (48, 49), bile acid secretion, or gut microbiota modulation (50). Most of the mechanisms proposed to explain the early metabolic improvements that occur independently from weight loss are related to the anatomic remodeling of the gastrointestinal tract, which highlights the relevance of the intestine in carbohydrate metabolism regulation after BS (51, 52).
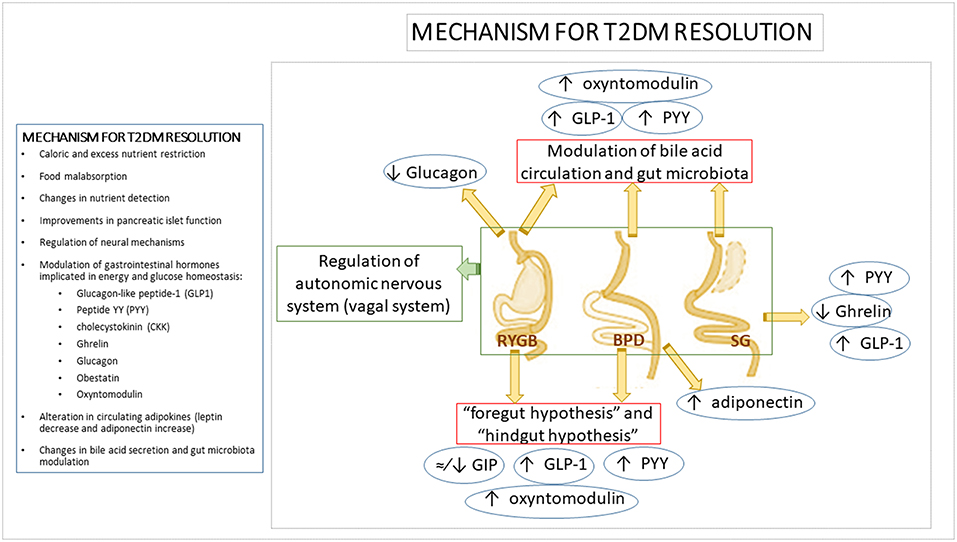
Figure 1. Mechanism for T2DM resolution. Mechanisms and modifications of main gastrointestinal hormones involved in T2DM resolution after bariatric surgery. Several mechanisms have been proposed to explain the metabolic improvement after bariatric surgery. However, due to the fact that each bariatric procedure does not involve the same gastrointestinal tract modifications, it has been suggested that each procedure acts by means of different mechanisms to achieve T2DM resolution, including differential shifts in gastrointestinal hormones levels. It has been proposed that the exclusion of the duodenum and proximal jejunum in bariatric procedures such as RYGB or BPD would inhibit the “anti-incretin” signaling (“foregut hypothesis”). This kind of remodeling would also reduce the time that nutrients take to reach distal jejunum which could imply an early activation of incretin-secreting L-cells in the distal ileum and proximal colon (“hindgut hypothesis”). Incretins such as GLP-1, PYY, or oxyntomodulin improve pancreatic insulin secretion and reduce glucagon release. By contrast, the main gastrointestinal hormonal shift expected after SG is the decrease in the levels of the orexigenic hormone ghrelin due to the removal of the gastric fundus and therefore, of the ghrelin-producing mucosa. However, it has also been described an increase in GLP-1 and PYY levels after SG likely due to a shorter intestinal transit after surgery. Apart from changes in gastrointestinal hormone patterns, alterations in bile acid metabolism, gut microbiota composition, modification in gastrointestinal vagal signaling or changes in adipokines levels described after the different bariatric procedures, could be also involved in the bariatric metabolic improvement and T2DM resolution after surgery. BPD, biliopancreatic diversion; GIP, gastric inhibitory polypeptide; GLP-1, Glucagon-like Peptide 1; PYY, Peptide YY; RYGB, Roux-en-Y gastric bypass; SG, sleeve gastrectomy; T2DM, Type 2 diabetes mellitus.
Several hypotheses have been raised regarding the implication of the gastrointestinal system as a metabolically active organ:
- The “foregut hypothesis” is based on the exclusion of the duodenum and proximal jejunum from the nutrient traffic across the gastrointestinal tract, which may inhibit “anti-incretin” signal production such as gastric inhibitory polypeptide (GIP). The removal of this signaling would favor direct antidiabetic effects with a reduction in insulin resistance (53–55).
- The “hindgut hypothesis” posits that nutrients reach the distal jejunum quickly, ensuring optimal digestion and nutrient absorption as well as metabolic improvement and weight loss through stimulation of L cells in the distal ileum and proximal colon which secrete GLP-1, PYY, oxyntomodulin, and other hormones (56). This response leads to an improvement in insulin secretion by pancreatic β cells and a reduction in glucagon production and hence, to a better post-prandial response to glucose and lower insulin resistance. By contrast, various BS procedures have shown heterogeneous effects on circulating GIP levels, ranging from moderate to unnoticeable and sometimes even leading to a decrease (46, 57–60).
- Other possible mechanisms have been elucidated from the results of SG. Although it would initially be considered a purely restrictive bariatric procedure, evidence has highlighted that its effects cannot be explained by caloric restriction alone. SG also leads to complex hormonal changes such as diminished ghrelin levels, which enhance weight loss and improve post-prandial insulin response together with lower post-prandial glucose levels (61, 62), as well as higher post-prandial GLP-1 and PYY responses induced by a short intestinal transit after surgery (63).
- The modulation of bile acid circulation has been postulated as a feasible mechanism to explain some of the metabolic effects of BS. Bile acids could be involved in both weight loss and the improvement in glycemic control by the stimulation of intestinal L-cell secretion of GLP-1, PYY, and oxyntomodulin which favors insulin response and the feeling of satiety (64, 65). Human and animal studies support the key role of bile acids in glucose metabolism regulation by means of pathways activated by the interaction of bile acid with the nuclear and membrane receptors farnesoid X receptor (FXR) and Takeda G protein-coupled receptor 5 (TGR-5), which are present in a number of tissues and organs such as the gut, liver, pancreas, adipose tissue, and skeletal muscle. By means of these receptors, bile acids lead to an increase in insulin secretion by pancreatic β cells, improvements in glucose tolerance, enhancement of glucose uptake by adipose tissue, decrease in gluconeogenesis, increase in glycogen synthesis and promotion of hepatic glucose catabolism (20, 66–68).
- Another intestine-related effect of BS which could be involved in the BS metabolic effects is the modulation of gut microbiota. Gut microbiota composition has previously been associated with obesity, T2DM and other metabolic diseases (55). Thus, it has been proposed that the modulation of gut microbiota composition by BS might be, at least in part, responsible for the post-surgical metabolic improvements. In agreement with this hypothesis, significant changes in gut microbiota composition have been reported after BS (50), but results from different studies are heterogeneous and no clear conclusions have been drawn (69). RYGB has been associated with an increase in Proteobacteria (70–72), while others described a concomitant decrease in Firmicutes and Bacteroidetes (70, 71). However, other studies found different shifts in gut microbiota profile after BS. Kong et al. showed a decrease in bacteria belonging to Firmicutes phyla at 3 and 6 months after RYGB in obese women (most of them without T2DM) (72) and a recent study found that RYGB lead to a decrease in Bacteroidetes 1 year post-operatively in obese diabetic patients (73). More detailed information regarding changes in specific bacterium groups have been recently reviewed elsewhere, giving an idea of the heterogeneous results (69, 74). Independently of the specific changes in bacterium groups, an increased bacterial diversity (previously related to the healthy status) post-surgery was reported (72, 75). Few studies have analyzed other BS procedures than RYGB regarding their effects on gut microbiota composition (73, 76–78). Similar changes in gut microbiota composition were found after RYGB and vertical banded gastroplasty (VBG) (76). However, recent studies reported differences in post-surgical gut microbiota profile between RYGB and SG (73) and between RYGB and laparoscopic adjustable gastric banding (LAGB) (77). The different design and participant inclusion criteria (gender, presence of T2DM and other obesity comorbidities or follow-up after surgery) of the current studies could explain the discrepancies found. Moreover, the gut microbiota profile after BS can be also dependent on post-surgery analysis time, omeprazole intake and diet (73, 79). Despite firm conclusions regarding the specific intestinal bacterial switch have not yet been drawn, recent studies suggest that gut microbiota composition is related to the success of surgical intervention in terms of excess weight loss or T2DM remission (73, 80). In view of these results, it can be hypothesized that the inter-individual response to BS might be, at least in part, dependent on gut microbiota composition. Furthermore, several studies have reported that BS is related to the modulation of the mechanisms presumably involved in the gut microbiota-host metabolism crosstalk such as LPS translocation and inflammatory markers (41, 70, 81, 82), regulation of GI hormone secretion (77, 83), influence on AT function (72, 84), bile acid metabolism (79), or short-fatty acid (SCFA) production (77). All these evidence point out that there is a relationship between BS consequences and gut microbiota, although further homogeneous studies are necessary in order to get a comprehensive overview of the involvement of gut microbiota in the metabolic improvement at both the short- and long-term after BS and the precise mechanisms by which it can take place.
- Changes in the neuroendocrine system have also been suggested to be involved in the beneficial effects of BS. As summarized above, the principal hypotheses about beneficial neuroendocrine effects of BS include the modification of gastrointestinal hormone release with well-recognized effects on food intake and energy equilibrium. Most of these hormones not only communicate with the brain in an endocrine manner, but they also act in a paracrine fashion by interacting with specific receptors located on vagal afferent nervous fibers which innervate the gastrointestinal tract and are in close proximity to gastrointestinal endocrine cells (85, 86). In addition, vagal afferent endings also respond to mechanical stimuli such as distension (86, 87). The nucleus tractus solitarius (NTS; vagal afferent nucleus) senses afferent inputs to regulate energy balance, including hunger and satiety feelings by the activation of brain regions involved in food intake regulation, as well as by vagal efferent inputs that regulates splanchnic organs such as liver and endocrine pancreas (87–89). These pathways seem to be altered in obesity with vagal afferent over-activation likely due to a decreased sensitivity to stimuli of vagal afferents which requires higher stimuli to activate proper response to feeding which leads to hyperphagia (87). Accordingly, interventions aimed at both depletion or stimulation of vagal afferent fibers have been shown to improve weight loss and food intake control (90, 91). The fact that these opposite interventions as well as the existence of contradictory results regarding vagotomy suggests that the effect depend on the site where vagal afferents are intervened (92). Animal studies analyzing vagal afferent restructuring after BS support this hypothesis. Different vagal pathway reorganizations are induced after each bariatric procedure, but behavioral reduced food intake and increased satiety feelings are common after BS regardless the procedure (93). Both RYGB and SG involves the damage of gastric vagal afferent fibers but in different gastric regions (94). RYGB damages gastric branches close to their origin resulting in a decreased density of vagal afferents in the NTS, reduction of synapses and NTS microglia activation (95). It is noteworthy that other vagal afferent endings to the rest of the intestine and other splanchnic organs are preserved (96). By contrast, SG only damages terminal gastric vagal branches which oppositely results in increased density of vagal afferent in the NTS and the number of synapses, which has been suggested to be linked to higher sensitivity to nutrients and satiety feelings, and then to lower food intake (95). The fact that blocking of vagal afferent fibers in the band site abolishes AGB effects on food intake and satiety, highlights the role of vagal afferent pathways in mediating AGB effects likely by means of mechanoreception (97). Evidence from human studies dealing with the involvement of vagal system in BS effects are limited. Indirect evidence regarding how distension stimuli, and then mechanosensitivity by vagal afferent fibers, might be involved in the voluntary food intake reduction after RYGB and AGB has been reported (98, 99). Sundbom et al. used pancreatic polypeptide (PP) as marker for vagal efferent function after RYGB, finding a post-operatively decrease in ghrelin and PP associated with weight loss that authors attribute to vagal dysfunction (100). Accordingly, Tamboli et al. found an attenuation in PP response to ghrelin after RYGB (101). By contrast, Perathoner et al. did not find significant differences in satiety score, weight loss, motility or ghrelin and gastrin levels in RYGB patients with or without vagal nerve dissection (102). Although not having being directly related to vagal pathways, significant changes in neural response (specifically reduction of the activation of brain areas linked to the mesolimbic reward pathway) to food cues have been reported in morbidly obese patients undergone RYGB (103). Ten Kulve et al. suggested that the reduction in brain activation to food pictures and to the intake of palatable food in patients who underwent RYGB could be mediated by the post-operatively GLP1 increase, although precise mechanism linking GLP1 and brain activity response to food remains unknown (104). In view of these results, neural modifications that affect patient behavior regarding feeding habits could be induced by the different bariatric procedures. Nevertheless, the limited evidence in humans make difficult to confirm the relevance of these neural remodeling and whether are cause or consequence of BS effects.
BS also alters hormone levels other than incretins such as glucagon. Patients who underwent RYGB had decreased glucagon levels (105). This decrease was similar to the reduction due to general weight loss. However, other studies did not find a significant decrease in glucagon levels after other surgical procedures (106).
Obestatin levels have also been described to be implicated not only in food intake and gastric emptying but also to have beneficial effects on pancreatic β cells by reducing apoptosis and promoting proliferation, increasing insulin secretion and decreasing insulin resistance, which have a positive effect on systemic glucose homeostasis. It has been suggested that obestatin may play a role in T2DM remission (47).
Increased adiponectin levels after BS have also been described. Adiponectin is an anti-inflammatory hormone that improves insulin sensitivity, and its rise after BS has been correlated with higher T2DM remission rates in patients who underwent BPD (48, 49).
Despite the general overview that BS improves carbohydrate metabolism, there are also studies that have reported T2DM recurrence 5 years after surgery in 50–95% of patients who had achieved T2DM remission. This recurrence was associated with weight regain and a longer history of T2DM previous to the surgery (107, 108). Thus, more studies are necessary in order to provide clinical recommendations on the use of oral antidiabetic drugs in patients who have undergone BS with anatomical remodeling and the consequent physiological changes. Another relevant aspect that should be considered is neuroglycopenic hypoglycemia in BS patients, which could be related to the foregut hypothesis and low production of “anti-incretin” signals needed to counteract the dominant incretin action after surgery together with the hyperinsulinemia triggered by the increased incretin response in the distal intestine (55).
Non-metabolic BS-Induced Endocrine Alterations
Aside from the well-known metabolic effects of BS, these surgical procedures also induce changes in other systems with further endocrine consequences (Table 1).
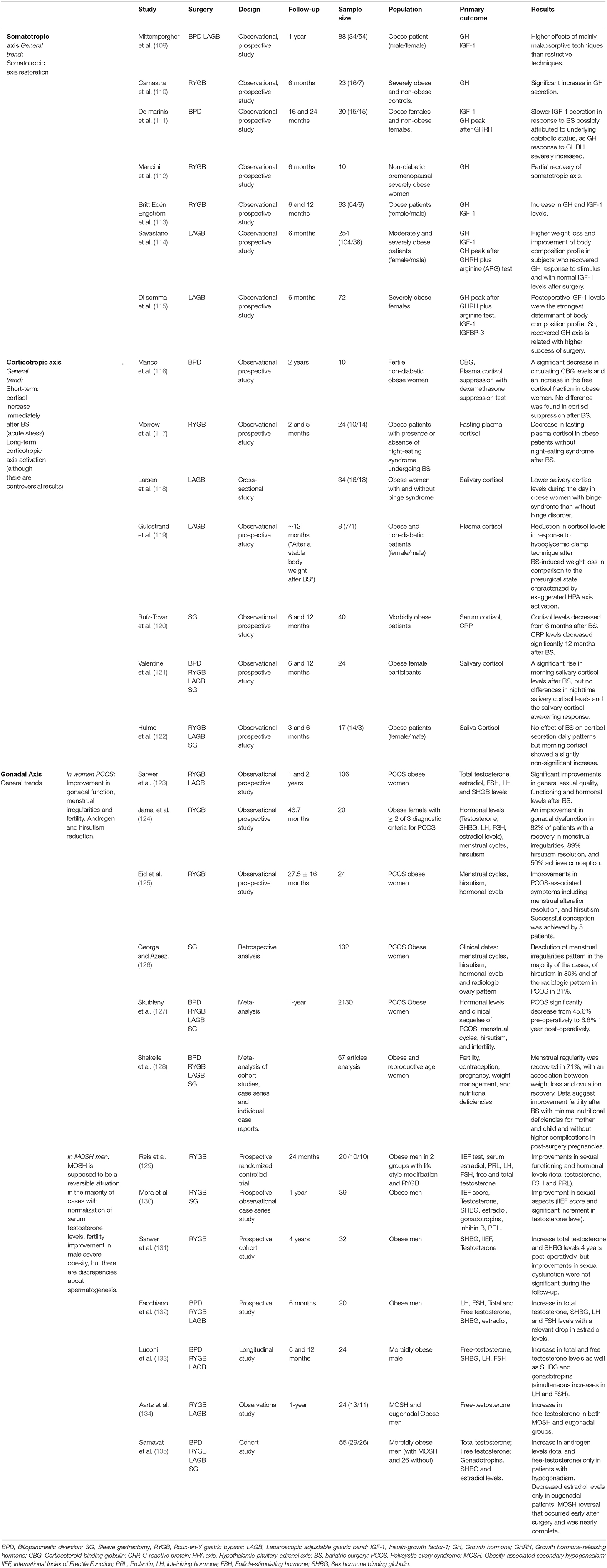
Table 1. Endocrine consequences of bariatric surgery on somatotropic, corticotropic, and gonadal axes.
Somatotropic Axis: Growth Hormone (GH)/Insulin-Growth Factor-1 (IGF-1) After BS
GH is mainly regulated by two hypothalamic peptide hormones: GH-releasing hormone (GHRH) and somatostatin. However, other brain signaling pathways such as those related to sleep regulation are also involved in the regulation of GH secretion (136). Obesity is associated with an acquired functional reduction in GH secretion and in GH response to stimulus (e.g., insulin-induced hypoglycemia, arginine, arginine-GHRH, sleep, or exercise), which is reversible after significant weight loss (137, 138). Likewise, adiposity acts as a negative determinant for GH secretion frequency and width, and has been associated with an increase in GH removal, which implies a lower GH half-life (109). A combination of multiple somatotropic axis alterations might be responsible for the different grades of GH and IGF-1 deficiency in obese subjects. However, the physiological role of GH in obesity and the mechanisms that lead to the perturbations in its levels are not completely clear. Among the underlying neuroendocrine alterations of low plasma GH levels in obesity, hormonal GHRH, somatostatin, or ghrelin dysregulation have been proposed (136, 139). It should be also noted that gender is a relevant regulatory factor of GH secretion in healthy subjects (113).
Reports regarding serum IGF-1 levels in obesity have shown contradictory results with both normal (109, 140, 141) and decreased IGF-1 levels in obese subjects having been described (142). Recent studies have suggested that inflammatory mediators could play a relevant role in the decrease in IGF-1 bioactivity (143), which could be related to obesity which is characterized by a chronic low-grade inflammation. Obesity, specifically abdominal obesity, appears to be associated with a decrease in free IGF-1 levels. This finding is highly interesting since visceral fat mass plays a key role in the development of obesity-related diseases, and low IGF-1 levels have also been related to T2DM and CVD (144, 145).
Somatotropic axis alterations in obese subjects, similar to those in patients who have a GH deficit, are associated with a higher prevalence of cardiovascular risk factors and alterations in body composition (muscle mass and bone density reduction and rise in fat mass) (146–148). Concordantly, Di Somma et al. found that obese patients with severe somatotropic axis alterations showed an exacerbated cardio-metabolic risk with more serious sequelae compared to obese subjects without GH deficiency (114).
Several studies have reported a restoration of somatotropic axis alterations in severely obese patients after BS. A significantly increased secretion of GH after BPD has been described (110), as well as partial recovery of the somatotropic axis after RYGB (112). Mittempergher et al. determined that the GH increase after BS was higher after mainly malabsorptive procedures (2.7-fold increase) than after mainly restrictive procedures (1.4-fold increase) with parallel improvements in cardiovascular profile and BMI reduction. Therefore, in view of these results, malabsorptive procedures might be better recommended for patients with somatotropic deficiencies (109). However, further research is necessary in order to confirm these recommendations.
Britt Edén Engström et al. determined that GH secretion and IGF-1 levels, previously compromised in severe obese subjects, were augmented at 6 months in women and at 12 months in both women and men after RYGB. A concomitant BMI reduction was reported in men and women at 6 months, which was further improved at 12 months after surgery (113). Savastano et al. and Di Somma et al. reported significant correlations between body composition and GH response to stimulus (GHRH + arginine) before and after surgery in subjects who underwent LAGB, and also found higher success with surgical treatment (weight loss and improved body composition profile) in those subjects without somatotropic axis alterations as well as in patients who recovered GH response to stimulus after surgery (114, 115). BS-derived benefits can be influenced by presurgery GH response to stimuli and by previous IGF-1 levels adjusted for sex and age. Thus, these factors could be interesting markers of surgery success in weight loss and body composition improvement. Likewise, a somatotropic axis study may constitute a useful tool for obese patient follow-up after BS (115).
Although some discrepancies have been found in the literature (109, 149), IGF-1 secretion displays a slower response to BS, similar to that of non-surgical weight loss, and this can be related to an underlying catabolic status induced by BS (malabsorptive effects, pronounced restriction in caloric intake, etc.) (111, 113). Few studies have analyzed tissue response to GH in obesity. Some have described a more pronounced IGF-1 response in obese individuals compared to lean subjects (150, 151). Nevertheless, these findings were not confirmed by others (152). Britt Edén Engström et al. observed that most obese subjects showed high IGF-1 levels with regard to their GH status. Altogether, these findings may indicate that there is a specific tissue adaptation in obesity with higher GH sensitivity, especially to its anabolic effects (113). In view of these results, the acquired dysfunction in GH secretion secondary to obesity appears to be reversed after BS. The somatotropic axis status could constitute a useful tool for initial and post-surgery follow-up of BS patients as a marker of surgery success in terms of weight loss and body composition.
Hypothalamic-Pituitary-Adrenal (HPA) or Corticotropic Axis: Corticotropin-Releasing Hormone (CRH)/Corticotropin (ACTH)/Cortisol After BS
The HPA axis is a relevant pathway in the response to physiological stress which regulates cortisol secretion. Cortisol has a number of effects on the organism, including energy reserve mobilization to promote survival and to comply with metabolic requirements during stress. Cortisol is secreted by the suprarenal cortex after HPA axis activation in response to physiological or psychological stress and high cortisol levels are associated with intense emotional responses to stressful stimuli (153). However, cortisol metabolism is not only centrally regulated, but type 1 and 2 11-β- hydroxysteroid dehydrogenase enzymes also regulate cortisol in tissues (154, 155). Food intake also increases cortisol levels and an individual may feel a decrease in anxiety with eating (156). Thus, both high cortisol levels and distressed emotions, appear to be modulated by calorie-rich food intake. Once this neuroendocrine-behavioral response to chronic stress is initiated, it could become a habit that favors weight gain. The non-pathological HPA axis is characterized by a distinctive circadian profile that is disrupted when the HPA axis is deregulated (157, 158). The declining curve of daily cortisol secretion is usually less pronounced in chronic health disorders such as obesity (159, 160). During chronic stress, HPA axis adaptation results in a higher and more prolonged cumulative exposure to glucocorticoids, which are associated with negative effects including metabolic disorders and cardiovascular mortality (161–166). Emerging data suggest that metabolic syndrome patients could have a hyperactive HPA axis which would lead to “functional hypercortisolism.” However, there are some controversial and heterogeneous findings in the studies analyzing this relationship (154, 155, 167). Moreover, there are disorders associated with HPA axis dysregulation that are more highly prevalent among obese subjects compared to the general population such as night-eating syndrome, binge eating disorder, sleep, and hormonal disorders as well as psychological symptoms (168–171). It is worth of mentioning that treatment for weight loss in obese subjects with these disorders is less successful than in obese individuals without these types of disorders (168, 172).
Very few studies have examined HPA axis regulation before and after BS in humans. The most commonly held idea is that weight loss tends to normalize cortisol levels and possible alterations in the HPA axis (173). Nevertheless, some studies suggest that long-term weight loss could be lessened when methods based on caloric restriction are employed, since caloric restriction could activate the HPA axis and increase circulating cortisol levels and these are associated with higher food intake and body weight (174–176). An increase in HPA axis tone during weight loss through food restriction can weaken behavioral modifications, which would make patient compliance to the prescribed diet and sustained weight loss in the long term difficult (177–179). Current evidence suggest that BS can affect HPA axis regulation, but controversial results have been found regarding the direction of this regulation (116, 119–121). Cortisol increases immediately after BS due to the acute stress caused by the surgery itself (180). However, the long-term effects of BS on cortisol have not yet been elucidated. Moreover, the few studies dealing with HPA axis after BS used different methodology for assessing HPA axis, which makes difficult to have clear conclusions regarding the effect of BS on HPA axis.
Morrow et al. studied HPA axis modulation in 24 severely obese participants (BMI = 40–70 Kg/m2) without associated comorbidities who underwent RYGB. Participants were classified according to the presence or absence of night-eating syndrome. A decrease in fasting plasma cortisol levels 5 months after surgery was reported in patients without night-eating syndrome, but an increase was seen in the subgroup of patients who had night-eating syndrome. However, the two groups did not differ in weight loss or waist circumference (117). By contrast, Hulme et al. found that BS did not affect daily cortisol secretion patterns during the first 6 months after surgery, although morning cortisol showed a slightly non-significant post-operative increase (122).
Valentine et al. also found a significant rise in morning saliva cortisol levels 6 and 12 months after BS (54.2% after SG; 16.7% after LAGB; 12.5% after RYGB, and 8.3% after duodenal switch) in 24 obese women, but no differences were found in nighttime saliva cortisol levels or in salivary cortisol awakening response (121). High morning cortisol could be associated with an improvement in physiological health after BS that is expressed by the narrowing of the decreasing curve of diurnal cortisol secretion in contrast to plainer curve characteristics in chronic health disorders.
Larsen et al. analyzed salivary cortisol levels in obese women after LAGB and found that neuroendocrine regulation after BS differs in these patients depending on the presence or absence of binge eating disorder. Obese women with binge eating disorder had significantly lower salivary cortisol levels during the day than patients without binge disorder. It is of note that a normal weight control group was not included in this study (118).
Manco et al. described a significant decrease in circulating corticosteroid-binding globulin (CBG) levels and a concomitant increase in the metabolically free cortisol fraction in obese women 2 years after BPD (116). The increase in free cortisol fraction may contribute to adaptive enterocyte hyperplasia in the long term, favoring better functional morphology as cortisol is a regulator of crypt enterocyte proliferation (181). Likewise, hypophyseal response, and/or suprarenal sensitivity, assessed by intravenous dexamethasone infusion, differed between the patients who underwent BPD and the normal weight control group. Nevertheless, there were no differences in cortisol suppression induced by dexamethasone before and after BS (116).
Guldstrand et al. studied HPA axis changes after LAGB in 8 non-diabetic severely obese patients. For this purpose, cortisol levels were analyzed in response to hypoglycemic clamp technique and a reduction in counter-regulatory hormone (cortisol among them) response was observed during the sustained hypoglycemic state after BS-induced weight loss in comparison to the pre-surgical state characterized by exaggerated HPA axis activation (119).
Ruiz-Tovar et al. reported sustained high serum cortisol levels up to 6 months after BS in 40 morbidly obese patients who underwent laparoscopic SG. However, cortisol levels decreased and were directly associated with the CVD risk predictor triglyceride/HDL ratio from 6 months after BS (120).
In view of these studies, obesity-induced corticotropic axis activation is reduced after BS and a normalization in HPA circadian rhythms might be also occurring. This effect has not been described for caloric restriction, which could add value to the use of BS for obesity treatment. However, although current evidence suggest that BS can affect HPA axis regulation with improvement in axis physiological health, controversial results have been found regarding the direction of this regulation. Therefore, more studies on this issue are needed to homogenize criteria concerning which variables should be used to monitor corticotropic axis status.
Modifications in Gonadal Axis or Hypothalamic/Pituitary/Gonadal Axis: Gonadotropin-Releasing Hormone (GnRH)/Follicle-Stimulating Hormone (FSH), Luteinizing Hormone (LH)/Testosterone, and Estradiol After BS
The incidence of sexual dysfunction, reproductive disorders, and infertility increase with age. The relationship between sexual dysfunction and other medical conditions such as obesity (182), diabetes (183, 184), high blood pressure (185, 186), metabolic syndrome, CVD or smoking (187) is widely accepted.
It is also well-known that adipose tissue plays a relevant role in the metabolism of hormones, including sex hormones, secreted by different glands (188). Obesity-associated gonadal dysfunction is one of the most prevalent comorbidities in obese subjects who seek to lose weight (29% for women and 45% for men) (189). These disorders are particularly common in severely obese subjects who have undergone BS (190). Similar to other obesity comorbidities, the higher the BMI, the higher the risk of gonadal dysfunction (191–193). A number of recent studies have dealt with this issue. Sarwer et al. studied gonadal dysfunction prevalence in 250 obese patients (141 who were interested in BS and 109 in lifestyle changes) finding differences according to the preferred treatment for weight loss achievement. Among individuals interested in BS, 51.4% of women (according to the Female Sexual Function Index) and 36.4% of men (according to the International Index of Erectile Function) had sexual dysfunction. By contrast, 40.9% of women and 20% of men who sought lifestyle modifications had sexual dysfunction. These differences were mainly related to BMI discrepancies. Sexual dysfunction was associated with quality life and other psychosocial functions, particularly in women (192). Interestingly, the significant improvement found in sexual functioning occurred mainly in women (130, 187, 194).
It has been widely described that BS leads to an improvement in sex hormone and sexual hormone binding globulin (SHBG) levels in morbidly obese patients (132, 133, 193, 195), even higher than the effect attributable to weight loss alone (133). Thus, this supports the hypothesis that factors derived from excess adipose tissue may modulate the gonadal axis (196). An increase in SHBG and a modification in serum estradiol levels are detected after BS in men and women. This could be due to the effect on estrogen production from testosterone and steroid precursors mediated by aromatase (an enzyme whose higher activity has been associated with gonadal dysfunction pathology in obesity) in reduced adipose tissue after BS (197).
The main evidence found in this context are due to: (1) excess androgen in obese women, mainly associated with polycystic ovary syndrome (PCOS) (36%) and with idiopathic hyperandrogenism (198), and (2) androgen deficiency in obese men that is termed male obesity-associated secondary hypogonadism (MOSH). A recent meta-analysis highlights that PCOS and MOSH are among the most common comorbidities in severely obese patients who have undergone BS (36 and 64%, respectively) that are usually resolved after BS (96% of women with PCOS and 87% of men with MOSH), as highlighted by a recent meta-analysis (191).
This improvement in gonadal profile occurs in parallel with insulin resistance improvement and metabolic disorder resolution (191).
Sex-specific changes induced by BS have been reported as described below.
Modifications in the Gonadal Axis in Obese Women After BS
Excess adipose tissue in obese women may contribute to excess androgens by stimulating both ovary and hormonal secretion by the suprarenal glands as secondary effects of insulin resistance and compensatory hyperinsulinism. Specifically, abdominal adiposity may feed a vicious circle in which excess androgens favors body fat deposition and this visceral fat promotes ovary and adrenal-derived excess androgens in PCOS. However, for PCOS to develop in response to obesity and visceral fat accumulation, women must have a primary defect in steroidogenesis that promotes excess androgen secretion, predisposing to androgen excess disorders (199). In contrast, women without this primary defect in androgenic secretion do not develop excess androgens or PCOS, even in the case of extreme obesity or insulin resistance (198). Renowned authors in the field consider that obesity is one of the secondary phenotypes of PCOS, and in these cases, gonadal dysfunction improves and even resolves with weight loss (200). A recent meta-analysis has demonstrated that PCOS significantly decreased from 45.6% pre-operatively to 6.8% 1 year after surgery (127). A decrease in serum androgen levels (total testosterone) associated with a reduction in androsterone and sulfate dehydroepiandrosterone (DHEA) as well as with resolution of hirsutism and menstrual irregularities (53 and 96% of cases, respectively) has been reported. Improvements in estradiol, FSH and SHBG have also been described (191, 193, 201–203).
Studies dealing with fertility recovery in women with PCOS that have assessed fertility before and after BS are scarce. The existing studies suggest that female fertility improves after bariatric procedures and intensive weight loss. However, most of these are observational studies with high variability in age, in the bariatric procedure employed and without a control group. This makes reach a consensus about the role of BS in fertility management difficult. Accordingly, smarter studies with a precise design comparing the different bariatric procedures are required (204). Among the studies dealing with fertility, Eid et al. demonstrated that RYGB-induced weight loss was associated with improvements in PCOS-associated symptoms including menstrual alteration resolution in all the participants and hirsutism in half of the participants. In addition, successful conception was achieved by five patients who previous to surgery failed to conceive (125).
George and Azeez carried out a study that included 132 women with PCOS who underwent SG in whom resolution was achieved in the majority of the cases of menstrual irregularities, hirsutism (80%) and radiologic irregularities (81%) (126).
Jamal et al. also observed improved gonadal dysfunction in 82% of RYGB PCOS patients, with decreased menstrual irregularities and resolution of hirsutism in 29% of the patients, and 50% of patients who were unable to conceive prior to surgery were successful after surgery (124).
Balen et al. based on low-quality evidence, recommended BS use in obese PCOS women with a BMI>35 kg/m2 and comorbidities such as gonadal dysfunction or BMI>40 kg/m2 who had infertility problems despite programmed intensive and structured treatment (based on dietary-hygienic habit changes) with no response for at least 6 months. They also recommended to avoiding pregnancy in the rapid post-operative weight loss period for at least 6–12 months after BS and at later times, recommended that these women should be attended in a specialized multidisciplinary unit due to the risk of BS-associated nutritional deficiencies (205).
Although PCOS is the most frequent reproductive disorder in obese women (191), idiopathic hypogonadotropic hypogonadism has also been associated with higher obesity prevalence in the female population (206). Several studies have reported improvements in the gonadotropic axis as well as sexual functioning after BS in obese women that are not necessarily related to PCOS. Sarwer et al. reported that after BS, 106 women (80% RYGB and 20% LAGB patients) showed significant improvements in general sexual quality and functioning as well as sexual desire and satisfaction 1 year after surgery. These improvements were maintained up to 2 years after BS. These changes took place in parallel with psychosocial improvements, significant weight reduction of 32.7% and significant improvement in hormonal profile: decreased total testosterone, estradiol and DHEAS and increased FSH, LH and SHGB levels (123).
Bond et al. described that in 68% of obese women with sexual dysfunction (defined by the Female Sexual Function Index score), this condition resolved after BS. This improvement in sexual function was not dependent on type of surgery or amount of weight loss and appears to be an additional benefit for women undergoing BS (194).
As detailed above, weight loss after lifestyle modification, and particularly after BS, is associated with significant improvements in sexual functioning and resolution of both menstrual and ovulatory disorders that occur in nearly all patients. Thus, weight loss could also contribute to fertility restoration (191, 194, 201). However, Shekelle et al. pointed out in their review that most of the reports are observational studies and data should be interpreted cautiously (128). Moreover, except for PCOS, the definition of pre-operative reproductive disorders in obese female patients having undergone BS is not clearly defined. It is of interest to understanding whether results of these reports are referring to PCOS or to different and independent reproductive anomalies to confirm whether BS is also effective for the resolution of additional reproductive disorders.
Modifications in Gonadal Axis in Obese Men After BS
In obese men, excess adipose tissue appears to contribute to androgenic deficiency by means of pituitary gonadotropin inhibition. This suggests central production deterioration together with reduced gonadal sensitivity to LH and increased peripheral androgen degradation by their conversion to estrogens (207, 208). MOSH is characterized by reduced serum testosterone together with high relative estradiol levels and inappropriately low or normal levels of LH and FSH (209). Although serum LH levels and the pulse frequency are similar in obese and lean subjects, LH pulse width is noticeably reduced in obesity (210). Among mechanisms involved in MOSH pathogenesis, gonadotropin inhibition by excess peripheral estrogen production in adipose tissue has been reported. However, insulin resistance and the secretion of inflammatory mediators and adipokines may play a relevant role in MOSH physiopathology with an inhibitory action at the hypothalamic-pituitary level (196, 211, 212). Recent data suggest that not only hyperinsulinemia but also excess liver fat are among the main inhibitory influences on SHBG secretion in obese subjects, in a process in which pro-inflammatory cytokines derived from ectopic adipose tissue are likely implicated (213). Likewise, hypophyseal dysfunction induced by obstructive sleep apnea syndrome may also participate in MOSH development (214).
Thus, abdominal adiposity could favor a vicious cycle in MOSH development by inhibiting hypophyseal gonadotropin secretion (215–217) and simultaneously, the resulting androgen deficiency promotes body fat accumulation in parallel to a decrease in muscle and lean mass (218).
Substantial fat mass loss after intensive weight loss will likely tend to reduce aromatase activity and regulate estradiol/testosterone equilibrium, resulting in decreased estradiol production, and will favor LH increase which contributes to stimulating testosterone production and substrate availability for estradiol production. It is thought that the differences between obese subjects with normal gonadal function and those with hypogonadism might be determined by aromatase expression levels: MOSH would be induced when aromatase gene expression is elevated. By contrast, testosterone levels would remain within the normal range when aromatase expression levels are low (134). Similar to PCOS in obese women, MOSH is not a generalized condition in male obesity and underlying mechanisms are largely unknown.
An increase in serum androgen levels (for both total testosterone and the free testosterone index), which was even higher than the increase in SHBG levels in men has been reported (134, 191). Normalization of serum testosterone levels after BS is associated with improved fertility in severely obese men. Nevertheless, more specific fertility studies focused on spermatogenesis and androgen levels are needed given the discrepancies between the studies published to date (191). Some studies suggest a deterioration in seminal parameters (219, 220), while others have observed no effect (202, 221) or even beneficial effects on sperm quality (222). It has also been postulated that fat mass reduction associated with weight loss might reduce abdominal, thigh and scrotal fat depots. This leads to a decrease in testicular temperature and, consequently, facilitate spermiogenesis (207).
Chronic testosterone deficiency could reduce post-operative fat loss and enhance the catabolic effects of BS on muscle and bone. This would attenuate the positive effects of BS on insulin sensitivity. A number of recent studies dealing with the changes and benefits in gonadal dysfunction due to BS in obese men, are in agreement with BS-induced improvements in testosterone, SHBG and LH levels (129, 223, 224). Although diet and lifestyle changes and BS are associated with a significant increase in hormone levels, MOSH normalization (increased testosterone and gonadotropin levels together with decreased estradiol levels) was more effective with BS than with low-caloric diets. By contrast, results regarding FSH levels are more controversial (129, 193, 223).
Reis et al. found significant improvements in sexual functioning and levels of hormones such as total testosterone, FSH and prolactin in 10 RYGB patients (129).
Mora et al. observed a pronounced weight loss (77.18% of excess weight), metabolic profile improvement together with improvements in sexual aspects such as an improved score on the International Index of Erectile Function and a significant increase in testosterone levels 1 year after surgery (RYGB and SG in 46.2 and 53.4%, respectively) in a prospective study carried out in 39 obese men (130).
In agreement, Sarwer et al. reported a concomitant decrease in patient weight by nearly one third and increase in total testosterone and SHBG levels 4 years after surgery in their cohort of 32 men who underwent RYGB and who showed deficient sexual functioning prior to surgery. Despite this finding and although improvements in sexual dysfunction were seen in the short term after surgery, these changes were not significant during the follow-up (131).
Facchiano et al. reported an increase in total testosterone, SHBG, LH, and FSH levels with a relevant drop in the initially elevated estradiol levels at 6 months after BS (10 with RYGB, 8 with LAGB and 2 with BPD) in 20 obese men (132).
The findings of Luconi et al. confirmed the already known relationship between excess adiposity and hypogonadism in morbidly obese men. In the same line as previous studies, patients showed increased total and free testosterone levels as well as gonadotropins (simultaneous increases in LH and FSH). The increased testosterone and SHBG levels induced by BS were higher than those expected for the weight loss alone. Likewise, the increase in total testosterone may be related to an SHBG rise and peripheral aromatization reduction that contributes to the observed fall in estradiol levels. Moreover, the direct effect of weight loss on Leydig cell function should not be discarded in the improvement of gonadal profile (133).
Aarts et al. included 24 severely obese men (BMI = 35–59 Kg/m2) in their study, 13 of them with MOSH and 11 eugonadal morbidly obese patients. One year after BS, an increase in free testosterone was observed in both MOSH and eugonadal groups. These levels were inversely related to weight loss. MOSH was a reversible situation in the majority of cases and this pre-operative condition was not adversely associated with BS efficiency in terms of weight, lipid and carbohydrate metabolism or with catabolic effects of surgery on muscle or bone mineral density (134).
Nevertheless, Samavat et al. in their study with morbidly obese BS patients (29 with hypogonadism and 26 eugonadal patients) observed that presurgery testosterone levels appear to moderate the BS effect on sex hormone recovery as an increase in androgen levels (total and free testosterone) only occurred in patients with hypogonadism. Preoperative estrogen levels were significantly lower in hypogonadal patients than in eugonadal patients. Moreover, estrogen levels only decreased after BS in eugonadal patients. Estradiol reduction in eugonadal patients was not related to testosterone level increment. As in the previous studies referenced above, Samavat et al. found a hypogonadism reversal that occurred early after surgery and was nearly complete. Taking into consideration these findings, the role of estradiol in obesity-related hypogonadism development might be limited (135).
All the revised studies, except for the study by Leenen et al. (225), reported a significant increase in total testosterone levels with weight loss and visceral fat reduction.
Few long-term studies have focused on the possible recurrence of gonadal dysfunction after weight regain. Rosenblatt et al. reported that patients who underwent RYGB from 6 to 16 years ago had high SHBG, total and free testosterone levels compared to non-surgical obese controls and these levels were comparable to those found in lean subjects. These findings agree with androgenic normalization in the long term. Nevertheless, the erectile function score was lower than in lean controls, which suggests incomplete functional restoration that may be related to weight gain and recurrent obesity comorbidities (226).
Recent research suggests reciprocal control between bone and testicles by means of osteocalcin (a peptide secreted by osteoblasts). It has been recently proposed that this peptide may be implicated in male fertility through direct stimulation of testosterone production by Leydig cells (227–231). Osteocalcin is decreased in obesity but it can also be produced by adipose tissue apart from bone production (232). Therefore, the inverse relationship between BMI and osteocalcin levels could indicate that only healthy adipose tissue may be involved in osteocalcin production (233). Weight loss induced by caloric restriction or BS is associated with the recovery of osteocalcin production. Samavat et al. analyzed 103 obese men, 76 patients who underwent RYGB and 27 controls who were waiting for surgery. The RYGB group showed an increase in osteocalcin, total testosterone and SHBG levels concomitant to a slight rise in gonadotropin levels, while estradiol levels fell likely due to a reduction in aromatase activity or expression. Osteocalcin levels were significantly associated with the restoration of testosterone levels in MOSH patients. Thus, osteocalcin may be an independent predictor for androgen restoration. It should be noted that no significant changes were seen in the non-surgical control group that had a slight weight loss. The osteocalcin levels increase might be explained by changes in leptin and adiponectin levels that regulate bone turnover in opposite ways. Likewise, insulin sensitivity improvement may enhance osteocalcin bone release (234). A recent report from Karsenty et al. supports the existence of a bone-testicle axis which is parallel to the pituitary-gonadal axis. In this way, osteocalcin and LH would act in parallel in both axes and osteocalcin would also be involved in human reproduction function (235).
In view of these results, BS is an effective method to treat gonadal dysfunction including recovery of fertility in comparison with lifestyle changes and diet-induced weight loss (200, 236, 237). Infertility or gonadal dysfunction could be used as criteria for indication for BS in obese patients with a BMI > 35 kg/m2. The considerable resolution rate of PCOS and MOSH, the most representative gonadal dysfunction disorders in obese individuals, after BS consistently supports the causal relationship of both obesity and adipose tissue dysfunction with gonadal dysfunction in predisposed subjects. However, more long-term studies focusing on the persistence of gonadal dysfunction resolution after BS are required.
Modifications in Bone Metabolism After BS
It has been widely described that obese patients are at higher risk of vitamin D deficiency pre-operatively, although it is not clear whether this association is more closely related to obesity per se or to obesity-related diseases such as T2DM (238). Consequently, secondary hyperparathyroidism due to vitamin D deficiency has also been extensively reported in obesity (239). Paradoxically, obese patients are generally characterized by higher bone mineral density (BMD) and bone mineral content (BMC), and it has even been suggested that obesity could be protective against osteoporosis (240). Bone remodeling implies a continuous process of bone replacement (formation) and bone removal (resorption). When these two processes are unbalanced, net bone formation or bone loss, respectively, will occur (241). Bone remodeling not only promotes bone repair, but also helps to mechanical stress adaptation. Thus, mechanical bone loading determines bone strength, size, and mass. This has led to the suggestion that mechanical bone loading could be responsible for the protective role of obesity in bone health. Larger bone size (that can also be due to increased mechanical loading) and enhanced aromatase activity from adipose tissue have also been suggested factors favoring BMD in obesity (240–242).
The most common nutritional deficiencies after BS include folate, iron, vitamin B12, calcium, vitamin D and zinc. Therefore, post-surgical changes in calcium and vitamin D can lead to bone loss resulting in higher fracture risk (243). In fact, it has generally been suggested that BS negatively affects bone health by diminishing BMD, enhancing bone resorption and impairing even further vitamin D levels and hyperparathyroidism (244). Most of the evidence comes from RYGB studies with fewer studies on other types of BS, but also finding negative effects from mainly restrictive procedures (245). One of the hypotheses posited to explain BMD and BMC after BS is mechanical unloading. A lower mechanical load due to weight loss can lead to less bone formation followed by a decrease in BMD. Concordantly, RYGB is associated with bone loss as reflected by BMD reduction at several sites 6 months (246), 1 year (247–251), and 2 years after surgery (22, 243, 252). Several studies have been carried out with longer follow-up periods up to 6 years finding a persistent bone loss (253–256). BPD has also been related to a higher incidence of metabolic bone disease 1–5 years after surgery (257) and Tsiftsis et al. reported decreased lumbar spine BMD 1 year after surgery (258). This BMD decrease could be site dependent since Marceau et al. found no changes in BMD in the hip but did find a decrease in the lumbar spine (259). Prospective studies comparing BMD at different sites between gastric bypass and SG, found that both procedures led to decreased BMD in the lumbar spine and femur. This decrease tended to be less pronounced after SG in most of the studies (260–262), but comparable in others (254, 263). Pluskiewicz et al. also reported a reduction in BMD in the lumbar spine, femoral neck and hip 6 months after SG (264). Results from LAGB are even more scarce. Decreased BMD and BMC have been reported 1 and 2 years after LAGB (265, 266). This negative effect seems to be smaller than that observed after RYGB (23). The effects of LAGB on BMC were compared to diet-induced weight loss and similar results were found (267). By contrast, Mach et al. did not find significant changes in BMC 1 year after LAGB (268).
BMD and BMC are determined by means of dual-energy X-ray absorptiometry (DXA), and technical limitations should be considered when data are interpreted. DXA are technically limited when applied to obese patients, particularly in severely obese subjects. Modifications in fat mass distribution due to drastic weight loss could influence measurement accuracy (22, 241, 269). Therefore, the controversial results regarding the effect of the different bariatric procedures on BMD and BMC could be due to technical issues.
In addition, it is unclear whether the reported BMD and BMC decrease after BS, is clinically relevant regarding osteoporosis incidence and fracture risk. As recently reviewed by Ben-Porat et al. different studies have found controversial results (245). In a study with a large sample size (n = 2079) in which most of the patients underwent LAGB, the risk for fracture was not increased 2 years after surgery (270). Nevertheless, when most of the population underwent RYGB, an increased fracture risk was observed in a retrospective study with a median follow-up of 7 years (271). These results were replicated after gastric bypass for a median follow-up time of 3.1 years (272). Studies that compared BS patients to obese and control patients, found higher fracture risk 4 years after surgery in the BS group than in the two control groups, significantly so with BPD. After 12 years there was also higher fracture risk after mainly malabsorptive procedures (273). A recent meta-analysis that included six studies concluded that BS is associated with increased fracture risk compared to control populations. However, the length of follow-up and the bariatric procedures were heterogeneous (274). Lack of long-term prospective studies does not help to elucidate the real effect of BS on osteoporosis development and fracture risk (245, 269).
The serum 25(OH)D form of vitamin D, calcium and parathyroid hormone (PTH) have also been measured to verify putative effects of BS on bone metabolism. As calcium is preferentially and actively absorbed in the proximal gut, and pancreatic secretion and bile acids are required for vitamin D absorption, bariatric procedures that bypass the duodenum and proximal jejunum could promote malabsorption of these micronutrients. Decreased stomach acid after SG and bypass of the proximal bowel would also reduce calcium absorption (22, 243). A meta-analysis analyzing 10 gastric bypass studies found, in addition to BMD reduction, a significant decrease in serum calcium levels (275). BPD was also associated with an increased prevalence of hypocalcemia (257). Gehrer et al. however, found no significant effect of RYGB or SG on serum calcium levels (276). Serum calcium levels are tightly regulated. While the intestine is responsible for calcium absorption from the diet, calcium reabsorption occurs in the kidneys. When these two mechanisms are insufficient to maintain serum calcium levels, calcium is released from the bones, which act as a calcium reservoir. Thus, despite normal calcium levels, low BMD could be present (22, 243). A more accurate way to evaluate calcium alteration after BS could be the direct determination of calcium absorption. Schafer et al. and Riedt et al. did this and found that gastric bypass led to a decline in calcium absorption 6 months after surgery (277, 278).
The tight regulation of serum calcium levels relies on PTH and the active form of Vitamin D (1,25(OH)2D). PTH stimulates osteoclast differentiation and survival and calcium release from the bone matrix when serum calcium levels are low. Negative feedback avoids uncontrolled PTH-induced bone loss, by stimulating 1,25(OH)2D synthesis. The active form of vitamin D inhibits PTH production and stimulates intestinal calcium absorption and FGF23 expression in bone (which leads to bone phosphorus loss) (241, 243). Although, it has been described as a pre-existing condition before BS, hyperparathyroidism has been reported after gastric bypass, LAGB, SG, and BPD (265, 275, 279–281). The prevalence of hyperparathyroidism increases with the time after BS, with higher prevalence after gastric bypass followed by RYGB, LAGB, and SG (281). Nevertheless, Tsiftsis et al. failed to find differences between pre-operative and post-operative PTH levels after BPD (258) and Guglielmi et al. found a decrease in PTH levels 6 months after SG (282). Likewise, hypovitaminosis D has been widely reported in obese patients prior to surgery. This status is sustained and even worsened after BS despite post-surgery vitamin D supplementation (283). It should be mentioned that BS patients usually receive vitamin D supplementation, but there is no consensus on the dose or duration of vitamin D supplementation. This should be taken into account for inter-study comparisons as this could be limiting conclusions regarding the magnitude of the effect of each BS procedure. Some studies compared 25(OH)D levels after different bariatric procedures in which patients were given the same vitamin D dose, and no differences were found between LAGB and RYGB on vitamin D deficiency incidence (284, 285). However, higher 25(OH)D levels were found after SG than RYGB after 2 years of follow-up (276, 286).
Vilarrasa et al. could not confirm these results when the administered doses of vitamin D were higher in the RYGB than in the SG group (254). This suggests that vitamin D supplementation should be adjusted depending on the bariatric procedure as 25(OH)D might be differentially affected depending on the technique. In addition, previous vitamin D deficiency and supplementation could be affecting post-surgical hypovitaminosis D. Furthermore, despite reaching normal 25(OH)D levels after BS, a higher incidence of bone diseases was reported after BPD which suggests that mechanisms other than PTH and vitamin D regulation could be affecting post-operative bone health (257).
Taking into account the limitation mentioned above regarding bone health assessment, bone turnover markers may also be an accurate approach to verify bone resorption and formation rates after BS. N-terminal propeptide of type 1 procollagen (P1NP) (cleaved from type 1 procollagen for collagen fiber assembling when incorporated into the bone matrix), bone-specific alkaline phosphatase (BAP) (a specific marker of bone formation osteoblast activity) and osteocalcin (a bone-specific protein produced by mature osteoblasts during bone matrix synthesis) are used as bone formation biomarkers. C-terminal and N-terminal telopeptides of type I collagen (CTX-1 and NTX-1, respectively; produced as a secondary product of collagen proteolytic cleavage during bone matrix degradation by osteoclasts) and tartrate-resistant acid phosphatase (TRACP; an enzyme highly expressed by osteoclasts) are considered bone resorption biomarkers (243, 287). SG and gastric bypass led to an increase in N-telopeptide but SG was also found to increase bone alkaline phosphatase (260). BPD also increased both bone formation and bone resorption markers. Despite this, BMD was reduced after surgery (258, 275). One year after LAGB, telopeptidases were reduced and correlated with bone loss (265). RYGB also decreased CTX 1 year after surgery (246). Crawford et al. recently analyzed the effect of weight loss through intensive medical therapy or by BS (RYGB or SG) on osteocalcin and CTX at 5 years. They found that surgical patients had increased levels of both CTX and osteocalcin, but no significant effect was seen with intensive medical therapy. A more noticeable effect was seen after RYGB than in SG (288).
Although some authors have questioned the mechanical unloading hypothesis (243), molecular mechanisms have been proposed to support this. Osteocytes act as mechanostat and comprise cellular response to environmental response to bone mechanical stress. Osteocytes sense mechanical bone deformation for mechanical loading and sclerostin production is inhibited. Sclerotin negatively regulates osteoblast differentiation and function and, conversely, sclerotin is upregulated under mechanical unloading. Thus, mechanical unloading could impair bone formation by impairing osteoblast function (241). Malnutrition, particularly after mainly malabsorptive techniques, has been also suggested as being responsible for bone metabolism deficiencies such as hypovitaminosis D or hyperparathyroidism as detailed above. But, further to mechanical stress and malnutrition, other hormonal mechanisms related to the well-described hormonal changes after the various bariatric procedures have been proposed (241). GLP-1, GIP, PYY, and ghrelin have been shown to act directly in bone (45, 46, 289). GIP inhibits osteoclast differentiation and its levels have been associated with bone formation markers (290, 291). Thus, the reported GIP decrease after BS procedures implies that proximal gut bypass could be associated with negative effects on bone. On the other hand, hormonal changes dependent on procedures involving stomach fundus removal such as SG, could also impair bone formation since ghrelin directly promotes osteoblast differentiation and proliferation (292), and its levels drop after this type of surgery. The relative function of GLP and PYY on bone metabolism and changes in their levels after BS do not correlate well, presupposing a harmful effect of BS on bone. PYY deficiency has been associated with low BMD (293), but its levels increase after RYGB, LAGB, SG, and BPD. Likewise, GLP-1 levels also rise after BS procedures such as BPD, but exogenous administration of GLP-1 and GLP-2 have been shown to improve BMD (294). Adipokines that decrease after BS, such as leptin, positively affect bone metabolism (295). On the other hand, adiponectin, which has been reported to raise after BS, has been negatively correlated with BMD (296). Moreover, adipose tissue is a source of estrogens which decrease bone resorption and enhance bone formation. Therefore, a weight loss-dependent estrogen decrease could also be associated with bone metabolism impairment after BS (223, 296).
All in all, there is sufficient evidence to assume that BS has an effect on bone metabolism. However, due to the fact that multiple mechanisms affected by BS could affect bone health in opposite ways, further research is needed to elucidate the net effect of each bariatric procedure on bone metabolism. Consensus on the best way to estimate bone health, taking into account technical limitations, should also be reached to obtain clear conclusions about what is really happening to bones in obese patients before and after surgery. Long-term prospective studies evaluating fracture risk and bone disease prevalence after BS, with large sample sizes and considering the type of post-surgery vitamin D and calcium supplementation, are further required to confirm BS effects on bone metabolism and to provide patients with accurate recommendations following each bariatric procedure.
Conclusion
Effects on metabolism and gastrointestinal hormones of BS are widely recognized, but the precise mechanisms are not yet completely understood. Moreover, it has been suggested that the different bariatric procedures are not equally effective in T2DM remission. However, heterogeneity regarding the criteria to define T2DM remission after BS makes difficult to state which surgical procedure is the most recommendable. Furthermore, other aspects than those exclusively metabolic should be taken into consideration since many other endocrine effects, but less explored up to date, are produced secondarily by the different bariatric procedures affecting corticotropic, somatotropic and gonadal axes as well as bone metabolism. Nevertheless, study design and criteria to evaluate the secondary consequences of BS are also heterogeneous which complicates the characterization of the precise effects of each bariatric procedure on these aspects. In addition, only few studies have evaluated BS consequences in the very long-term finding nutrient deficiency-related disorders, particularly after mainly malabsoprtive procedures which are at the same time considered as the most effective regarding T2DM resolution. Thus, although it is generally considered that benefits counteract by far the drawbacks of BS, further studies with homogeneous criteria and design, considering other bariatric consequences than merely metabolic effects, as well as the performance of a close long-term follow-up, are necessary in order to have a wider comprehensive overview of the consequences of each bariatric procedure. This would be useful for individualized surgical intervention recommendations depending on the different obesity co-morbidities previous to surgery of each patient as well as for preventing health-derived complications of BS.
Author Contributions
IC-P, MC-P, and FT contributed to the manuscript design, to researching the literature and to the discussion, drafted and wrote the manuscript, and approved the final version.
Funding
IC-P was recipient of a post-doctoral grant (Río Hortega CM 17/00169) from the Instituto de Salud Carlos III (ISCIII; Madrid, Spain) and MC-P was recipient of a post-doctoral grant Juan de la Cierva Formación (FJCI-2017-32194) from the Ministerio de Ciencia, Innovación y Universidades (Spain). This study was supported by Centros de Investigación Biomédica En Red (CIBER, CB06/03/0018) of the ISCIII, Madrid (Spain), RIC-0539-2018 and PI-0092-2017 from Consejería de Salud (Junta de Andalucía), PI15/01114 and PI18/01160 from the ISCIII (Madrid, Spain) and co-funded by the Fondo Europeo de Desarrollo Regional (FEDER).
Conflict of Interest Statement
The authors declare that the research was conducted in the absence of any commercial or financial relationships that could be construed as a potential conflict of interest.
Acknowledgments
The authors wish to thank FIMABIS and the help of Maria Repice for her language expertise in preparing this manuscript.
References
1. Salas-Salvadó J, Rubio MA, Barbany M, Moreno B, de la SEEDO GC. Consenso SEEDO 2007 para la evaluación del sobrepeso y la obesidad y el establecimiento de criterios de intervención terapéutica. Med Clin. (2007) 28:184–96. doi: 10.1016/S0025-7753(07)72531-9
2. WHO. Obesity and Overweight. Available online at: https://www.who.int/news-room/fact-sheets/detail/obesity-and-overweight
3. Look AHEAD Research Group, Wing RR, Bolin P, Brancati FL, Bray GA, Clark JM, et al. Cardiovascular effects of intensive lifestyle intervention in type 2 diabetes. N Engl J Med. (2013) 369:145–54. doi: 10.1056/NEJMoa1212914
4. Daigle CR, Brethauer SA, Tu C, Petrick AT, Morton JM, Schauer PR, et al. Which postoperative complications matter most after bariatric surgery? Prioritizing quality improvement efforts to improve national outcomes. Surg Obes Relat Dis. (2018) 14:652–7. doi: 10.1016/j.soard.2018.01.008
5. Magouliotis DE, Tasiopoulou VS, Svokos KA, Svokos AA, Sioka E, Tzovaras G, et al. Banded vs. non-banded Roux-en-Y gastric bypass for morbid obesity: a systematic review and meta-analysis. Clin Obes. (2018) 8:424–33. doi: 10.1111/cob.12274
6. Tice JA, Karliner L, Walsh J, Petersen AJ, Feldman MD. Gastric banding or bypass? A systematic review comparing the two most popular bariatric procedures. Am J Med. (2008) 121:885–93. doi: 10.1016/j.amjmed.2008.05.036
7. Colquitt JL, Pickett K, Loveman E, Frampton GK. Surgery for weight loss in adults. Cochrane Database Syst Rev. (2014). 8:CD003641. doi: 10.1002/14651858.CD003641.pub4
8. Rachlin E, Galvani C. Metabolic surgery as a treatment option for type 2 diabetes mellitus: surgical view. Curr Diab Rep. (2018) 18:113. doi: 10.1007/s11892-018-1094-1
9. Schauer PR, Kashyap SR, Wolski K, Brethauer SA, Kirwan JP, Pothier CE, et al. Bariatric surgery versus intensive medical therapy in obese patients with diabetes. N Engl J Med. (2012) 366: 1567–76. doi: 10.1056/NEJMoa1200225
10. Sjöström L. Bariatric surgery and reduction in morbidity and mortality: experiences from the SOS study. Int J Obes. (2008) 32(Suppl. 7):S93–7. doi: 10.1038/ijo.2008.244
11. Sjöström L, Narbro K, Sjöström CD, Karason K, Larsson B, Wedel H, et al. Swedish obese subjects study. Effects of bariatric surgery on mortality in Swedish obese subjects. N Engl J Med. (2007) 357:741–52. doi: 10.1056/NEJMoa066254
12. Adil MT, Jain V, Rashid F, Al-Taan O, Whitelaw D, Jambulingam P. Meta-analysis of the effect of bariatric surgery on physical function. Br J Surg. (2018) 105:1107–18. doi: 10.1002/bjs.10880
13. Leonetti F, Capoccia D, Coccia F, Casella G, Baglio G, Paradiso F, et al. Obesity, type 2 diabetes mellitus, and other comorbidities: a prospective cohort study of laparoscopic sleeve gastrectomy vs medical treatment. Arch Surg. (2012) 147:694–700. doi: 10.1001/archsurg.2012.222
14. Risstad H, Søvik TT, Engström M, Aasheim ET, Fagerland MW, Olsén MF, et al. Five-year outcomes after laparoscopic gastric bypass and laparoscopic duodenal switch in patients with body mass index of 50 to 60: a randomized clinical trial. JAMA Surg. (2015) 150:352–61. doi: 10.1001/jamasurg.2014.3579
15. Peterli R, Wölnerhanssen BK, Vetter D, Nett P, Gass M, Borbély Y, et al. Laparoscopic sleeve gastrectomy versus Roux-Y-gastric bypass for morbid obesity-3-year outcomes of the prospective randomized Swiss Multicenter Bypass Or Sleeve Study (SM-BOSS). Ann Surg. (2017) 265:466–73. doi: 10.1097/SLA.0000000000001929
16. Salminen P, Helmiö M, Ovaska J, Juuti A, Leivonen M, Peromaa-Haavisto P, et al. Effect of laparoscopic sleeve gastrectomy vs laparoscopic Roux-en-Y gastric bypass on weight loss at 5 years among patients with morbid obesity: the SLEEVEPASS randomized clinical trial. JAMA. (2018) 319:241–54. doi: 10.1001/jama.2017.20313
17. Hofsø D, Nordstrand N, Johnson LK, Karlsen TI, Hager H, Jenssen T, et al. Obesity related cardiovascular risk factors after weight loss: a clinical trial comparing gastric bypass surgery and intensive lifestyle intervention. Eur J Endocrinol. (2010) 163:735–45. doi: 10.1530/EJE-10-0514
18. Adams TD, Pendleton RC, Strong MB, Kolotkin RL, Walker JM, Litwin SE, et al. Health outcomes of gastric bypass patients compared to nonsurgical, nonintervened severely obese. Obesity. (2010) 18:121–30. doi: 10.1038/oby.2009.178
19. Heo YS, Park JM, Kim YJ, Kim SM, Park DJ, Lee SK, et al. Bariatric surgery versus conventional therapy in obese Korea patients: a multicenter retrospective cohort study. J Korean Surg Soc. (2012) 83:335–42. doi: 10.4174/jkss.2012.83.6.335
20. Noel OF, Still CD, Argyropoulos G, Edwards M, Gerhard GS. Bile Acids, FXR, and metabolic effects of bariatric surgery. J Obes. (2016) 2016:4390254. doi: 10.1155/2016/4390254
21. Tinahones FJ, Queipo-Ortuño MI, Clemente-Postigo M, Fernnadez-Garcia D, Mingrone G, Cardona F. Postprandial hypertriglyceridemia predicts improvement in insulin resistance in obese patients after bariatric surgery. Surg Obes Relat Dis. (2013) 9:213–8. doi: 10.1016/j.soard.2011.08.022
22. Kim J, Brethauer S, ASMBS Clinical Issues Committee American Society for Metabolic and Bariatric Surgery Clinical Issues Committee Position Statement. Metabolic bone changes after bariatric surgery. Surg Obes Relat Dis Off J Am Soc Bariatr Surg. (2015) 11:406–11. doi: 10.1016/j.soard.2014.03.010.
23. Rodríguez-Carmona Y, López-Alavez FJ, González-Garay AG, Solís-Galicia C, Meléndez G, Serralde-Zúñiga AE. Bone mineral density after bariatric surgery. A systematic review. Int J Surg Lond Engl. (2014) 12:976–82. doi: 10.1016/j.ijsu.2014.08.002
24. Mingrone G, Panunzi S, De Gaetano A, Guidone C, Iaconelli A, Nanni G, et al. Bariatric-metabolic surgery versus conventional medical treatment in obese patients with type 2 diabetes: 5 year follow-up of anopen-label, singlecentre, randomised controlled trial. Lancet. (2015) 386:964–73. doi: 10.1016/S0140-6736(15)00075-6
25. Schauer PR, Bhatt DL, Kirwan JP, Wolski K, Aminian A, Brethauer SA, et al. STAMPEDE Investigators. Bariatric surgery versus intensive medical therapy for diabetes 5-year outcomes. N Engl J Med. (2017) 376:641–51. doi: 10.1056/NEJMoa1600869
26. Schauer PR, Bhatt DL, Kirwan JP, Wolski K, Brethauer SA, Navaneethan SD, et al. STAMPEDE Investigators. Bariatric surgery versus intensive medical therapy for diabetes−3-year outcomes. N Engl J Med. (2014) 370:2002–13. doi: 10.1056/NEJMoa1401329
27. Iaconelli A, Panunzi S, De Gaetano A, Manco M, Guidone C, Leccesi L, et al. Effects of bilio pancreatic diversion on diabetic complications: a 10 year follow up. Diabetes Care. (2011) 34:56–7. doi: 10.2337/dc10-1761
28. Singh AK, Singh R, Kota SK. Bariatric surgery and diabetes remission: who would have thought it? Indian J Endocrinol Metab. (2015) 19:563–76. doi: 10.4103/2230-8210.163113
29. Benotti P, Wood GC, Winegar DA, Petrick AT, Still CD, Argyropoulos G, et al. Risk factors associated with mortality after Roux-en-Y gastric bypass surgery. Ann Surg. (2014) 259:123–30. doi: 10.1097/SLA.0b013e31828a0ee4
30. Garrido-Sanchez L, Murri M, Rivas-Becerra J, Ocaña-Wilhelmi L, Cohen RV, Garcia-Fuentes E, et al. Bypass of the duodenum improves insulin resistance much more rapidly than sleeve gastrectomy. Surg Obes Relat Dis. (2012) 8:145–50. doi: 10.1016/j.soard.2011.03.010
31. Buchwald H, Avidor Y, Braunwald E, Jensen MD, Pories W, Fahrbach K, et al. Bariatric surgery: a systematic review and meta-analysis. JAMA. (2004) 292.1724–37. doi: 10.1001/jama.292.14.1724
32. Pournaras DJ, Aasheim ET, Søvik TT, Andrews R, Mahon D, Welbourn R, et al. Effect of the definition of type II diabetes remission in the evaluation of bariatric surgery for metabolic disorders. Br J Surg. (2012) 99:100–3. doi: 10.1002/bjs.7704
33. Brethauer SA, Aminian A, Romero-Talam'as H, Batayyah E, Mackey J, Kennedy L, et al. Can diabetes be surgically cured? Long-term metabolic effects of bariatric surgery in obese patients with type 2 diabetes mellitus. Ann Surg. (2013) 258:628–36. Discussion 636–7. doi: 10.1097/SLA.0b013e3182a5034b
34. Buse JB, Caprio S, Cefalu WT, Ceriello A, Del Prato S, Inzucchi SE, et al. How do we define cure of diabetes? Diabetes Care. (2009) 32:2133–5. doi: 10.2337/dc09-9036
35. Mingrone G, Panunzi S, De Gaetano A, Guidone C, Iaconelli A, Leccesi L, et al. Bariatric surgery versus conventional medical therapy for type 2 diabetes. N Engl J Med. (2012) 366:1577–85. doi: 10.1056/NEJMoa1200111
36. Yan Y, Sha Y, Yao G, Wang S, Kong F, Liu H, et al. Roux-en-Y Gastric bypass versus medical treatment for type 2 diabetes mellitus in obese patients: a systematic review and meta-analysis of randomized controlled trials. Medicine. (2016) 95:e3462. doi: 10.1097/MD.0000000000003462
37. Chang SH, Stoll CR, Song J, Varela JE, Eagon CJ, Colditz GA. The effectiveness and risks of bariatric surgery: an updated systematic review and meta-analysis, 2003-2012. JAMA Surg. (2014) 149: 275–87. doi: 10.1001/jamasurg.2013.3654
38. Wang F-G, Yan W-M, Yan M, Song M-M. Outcomes of Mini vs Roux-en-Y gastric bypass: a meta-analysis and systematic review, Int J Surg. (2018) 56:7–14. doi: 10.1016/j.ijsu.2018.05.009
39. Bojsen Møller KN, Dirksen C, Jørgensen NB, Jacobsen SH, Serup AK, Albers PH, et al. Early enhancements of hepatic and later of peripheral insulin sensitivity combined with increased postprandial insulin secretion contribute to improved glycemic control after Roux en Y gastric bypass. Diabetes. (2014) 63:1725–37. doi: 10.2337/db13-1307
40. Camastra S, Gastaldelli A, Mari A, Bonuccelli S, Scartabelli G, Frascerra S, et al. Early and longer term effects of gastric bypass surgery on tissue specific insulin sensitivity and beta cell function in morbidly obese patients with and without type 2 diabetes. Diabetologia. (2011) 54:2093–102. doi: 10.1007/s00125-011-2193-6
41. Clemente-Postigo M, Roca-Rodriguez Mdel M, Camargo A, Ocaña-Wilhelmi L, Cardona F, Tinahones FJ. Lipopolysaccharide and lipopolysaccharide-binding protein levels and their relationship to early metabolic improvement after bariatric surgery. Surg Obes Relat Dis. (2015) 11:933–9. doi: 10.1016/j.soard.2014.11.030
42. Murri M, García-Fuentes E, García-Almeida JM, Garrido-Sánchez L, Mayas MD, Bernal R, et al. Changes in oxidative stress and insulin resistance in morbidly obese patients after bariatric surgery. Obes Surg. (2010) 20:363–8. doi: 10.1007/s11695-009-0021-6
43. Ashrafian H, le Roux CW. Metabolic surgery and gut hormones– a review of bariatric entero-humoral modulation. Physiol Behav. (2009)97:620–31. doi: 10.1016/j.physbeh.2009.03.012
44. Santiago-Fernández C, García-Serrano S, Tome M, Valdes S, Ocaña-Wilhelmi L, Rodríguez-Cañete A, et al. Ghrelin levels could be involved in the improvement of insulin resistance after bariatric surgery. Endocrinol Diabetes Nutr. (2017) 64:355–62. doi: 10.1016/j.endien.2017.08.011
45. Meek CL, Lewis HB, Reimann F, Gribble FM, Park AJ. The effect of bariatric surgery on gastrointestinal and pancreatic peptide hormones. Peptides. (2016) 77:28–37. doi: 10.1016/j.peptides.2015.08.013
46. Jacobsen SH, Olesen SC, Dirksen C, Jørgensen NB, Bojsen-Møller KN, Kielgast U, et al. Changes in gastrointestinal hormone responses, insulin sensitivity, and beta-cell function within 2 weeks after gastric bypass in non-diabetic subjects. Obes Surg. (2012) 22:1084–96. doi: 10.1007/s11695-012-0621-4
47. Wang JL, Xu XH, Zhang XJ, Li WH. The role of obestatin in roux-en-Y gastric bypass-induced remission of type 2 diabetes mellitus. Diabetes Metab Res Rev. (2016) 32:470–7. doi: 10.1002/dmrr.2735
48. Adami GF, Gradaschi R, Andraghetti G, Scopinaro N, Cordera R. Serum leptin and adiponectin concentration in type 2 diabetes patients in the short and long term following Biliopancreatic diversion. Obes Surg. (2016) 26:2442–8. doi: 10.1007/s11695-016-2126-z
49. Malin SK, Bena J, Abood B, Pothier CE, Bhatt DL, Nissen S, et al. Attenuated improvements in adiponectin and fat loss characterize type 2 diabetes non-remission status after bariatric surgery. Diabetes Obes Metab. (2014) 16:1230–8. doi: 10.1111/dom.12376
50. Guo Y, Huang ZP, Liu CQ, Qi L, Sheng Y, Zou DJ. Modulation of the gut microbiome: a systematic review of the effect of bariatric surgery. Eur J Endocrinol. (2018) 178:43–56. doi: 10.1530/EJE-17-0403
51. Haluzík M, Kratochvílová H, Haluzíková D, Mráz M. Gut as an emerging organ for the treatment of diabetes: focus on mechanism of action of bariatric and endoscopic interventions. J Endocrinol. (2018) 237:R1–17. doi: 10.1530/JOE-17-0438
52. Jackness C, Karmally W, Febres G, Conwell IM, Ahmed L, Bessler M, et al. Very low-calorie diet mimics the early beneficial effect of Roux-en-Y gastric bypass on insulin sensitivity and beta-cell function in type 2 diabetic patients. Diabetes. (2013) 62:3027–32. doi: 10.2337/db12-1762
53. Rubino F, Forgione A, Cummings DE, Vix M, Gnuli D, Mingrone G, et al. The mechanism of diabetes control after gastrointestinal bypass surgery reveals a role of the proximal small intestine in the pathophysiology of type 2 diabetes. Ann Surg. (2006) 244:741–9. doi: 10.1097/01.sla.0000224726.61448.1b
54. Pournaras DJ, Glicksman C, Vincent RP, Kuganolipava S, Alaghband-Zadeh J, Mahon D, et al. The role of bile after Roux-en-Y gastric bypass in promoting weight loss and improving glycaemic control. Endocrinology. (2012) 153:3613–9. doi: 10.1210/en.2011-2145
55. Batterham RL, Cummings DE. Mechanisms of diabetes improvement following bariatric/metabolic surgery. Diabetes Care. (2016) 39:893–901. doi: 10.2337/dc16-0145
56. Jirapinyo P, Jin DX, Qazi T, Mishra N, Thompson CC. A meta-analysis of GLP-1 after Roux-en-Y gastric bypass: impact of surgical technique andmeasurement strategy. Obes Surg. (2018) 28:615–26. doi: 10.1007/s11695-017-2913-1
57. Svane MS, Bojsen-Møller KN, Nielsen S, Jørgensen NB, Dirksen C, Bendtsen Fet al. Effects of endogenous GLP-1 and GIP on glucose tolerance after Roux-en-Y gastric bypass surgery. Am J Physiol Endocrinol Metab. (2016) 310:E505–14. doi: 10.1152/ajpendo.00471.2015
58. Mingrone G, Nolfe G, Gissey GC, Iaconelli A, Leccesi L, Guidone C, et al. Circadian rhythms of GIP and GLP1 in glucosetolerant and in type 2 diabetic patients after biliopancreatic diversion. Diabetologia. (2009) 52 873–81. doi: 10.1007/s00125-009-1288-9
59. Salinari S, Bertuzzi A, Asnaghi S, Guidone C, Manco M, Mingrone G. First-phase insulin secretion restoration and differential response to glucose load depending on the route of administration in type 2 diabetic subjects after bariatric surgery. Diabetes Care. (2009) 32:375–80. doi: 10.2337/dc08-1314
60. Oh TJ, Ahn CH, Cho YM. Contribution of the distal small intestine to metabolic improvement after bariatric/metabolic surgery: lessons from ileal transposition surgery. J Diabetes Invest. (2016) 7:94–101. doi: 10.1111/jdi.12444
61. Lo JC, Ljubicic S, Leibiger B, Kern M, Leibiger IB, Moede T, et al. Adipsin is an adipokine that improves β cell function in diabetes. Cell. (2014) 158:41–53. doi: 10.1016/j.cell.2014.06.005
62. Chronaiou A, Tsoli M, Kehagias I, Leotsinidis M, Kalfarentzos F, Alexandrides TK. Lower ghrelin levels and exaggerated postprandial peptide-YY, glucagon-like peptide-1, and insulin responses, after gastric fundus resection, in patients undergoing Roux-en-Y gastric bypass: a randomized clinical trial. Obes Surg. (2012) 22:1761–70. doi: 10.1007/s11695-012-0738-5
63. Still CD, Wood GC, Chu X, Erdman R, Manney CH, Benotti PN, et al. High allelic burden of four obesity SNPs is associated with poorer weight loss outcomes following gastric bypass surgery. Obesity. (2011):1676–83. doi: 10.1038/oby.2011.3
64. Flynn CR, Albaugh VL, Cai S, Cheung-Flynn J, Williams PE, Brucker RM, et al. Bile diversion to the distal small intestine has comparable metabolic benefits to bariatric surgery. Nat Commun. (2015) 6:7715. doi: 10.1038/ncomms8715
65. Shungin D, Winkler TW, Croteau-Chonka DC, Ferreira T, Locke AE, Mägi R, et al. New genetic loci link adipose and insulin biology to body fat distribution. Nature. (2015) 518:187–96. doi: 10.1038/nature14132
66. Kaska L, Sledzinski T, Chomiczewska A, Dettlaff-Pokora A, Swierczynski J. Improved glucose metabolism following bariatric surgery is associated with increased circulating bile acid concentrations and remodeling of the gut microbiome. World J Gastroenterol. (2016) 22:8698–719. doi: 10.3748/wjg.v22.i39.8698
67. Ryan KK, Tremaroli V, Clemmensen C, Kovatcheva-Datchary P, Myronovych A, Karns R, et al. FXR is a molecular target for the effects of vertical sleeve gastrectomy. Nature. (2014) 509:183–8. doi: 10.1038/nature13135
68. Schaap FG, Trauner M, Jansen PLM. Bile acid receptors as targets for drug development. Nat Rev Gastroenterol Hepatol. (2014) 11:55–67. doi: 10.1038/nrgastro.2013.151
69. Magouliotis DE, Tasiopoulou VS, Sioka E, Chatedaki C, Zacharoulis D. Impact of bariatric surgery on metabolic and gut microbiota profile: a systematic review and meta-analysis. Obes Surg. (2017) 27:1345–57. doi: 10.1007/s11695-017-2595-8
70. Graessler J, Qin Y, Zhong H, Zhang J, Licinio J, Wong M-L, et al. Metagenomic sequencing of the human gut microbiome before and after bariatric surgery in obese patients with type 2 diabetes: correlation with inflammatory and metabolic parameters. Pharmacogenomics J. (2013) 13:514–22. doi: 10.1038/tpj.2012.43
71. Zhang H, Di Baise JK, Zuccolo A, Kudrna D, Braidotti M, Yu Y, et al. Human gut microbiota in obesity and after gastric bypass. Proc Natl Acad Sci USA. (2009) 106:2365–70. doi: 10.1073/pnas.0812600106
72. Kong L-C, Tap J, Aron-Wisnewsky J, Pelloux V, Basdevant A, Bouillot J-L, et al. Gut microbiota after gastric bypass in human obesity: increased richness and associations of bacterial genera with adipose tissue genes. Am J Clin Nutr. (2013) 98:16–24. doi: 10.3945/ajcn.113.058743
73. Murphy R, Tsai P, Jüllig M, Liu A, Plank L, Booth M. Differential changes in gut microbiota after gastric bypass and sleeve gastrectomy bariatric surgery vary according to diabetes remission. Obes Surg. (2017) 27:917–25. doi: 10.1007/s11695-016-2399-2
74. Tabasi M, Ashrafian F, Khezerloo JK, Eshghjoo S, Behrouzi A, Javadinia SA, et al. Changes in gut microbiota and hormones after bariatric surgery: a bench-to-bedside review. Obes Surg. (2019) 29:1663–74. doi: 10.1007/s11695-019-03779-7
75. Palleja A, Kashani A, Allin KH, Nielsen T, Zhang C, Li Y, et al. Roux-en-Y gastric bypass surgery of morbidly obese patients induces swift and persistent changes of the individual gut microbiota. Genome Med. (2016) 8:67. doi: 10.1186/s13073-016-0312-1
76. Tremaroli V, Karlsson F, Werling M, Ståhlman M, Kovatcheva-Datchary P, Olbers T, et al. Roux-en-Y gastric bypass and vertical banded gastroplasty induce long-term changes on the human gut microbiome contributing to fat mass regulation. Cell Metab. (2015) 22:228–38. doi: 10.1016/j.cmet.2015.07.009
77. Ilhan ZE, DiBaise JK, Isern NG, Hoyt DW, Marcus AK, Kang D-W, et al. Distinctive microbiomes and metabolites linked with weight loss after gastric bypass, but not gastric banding. ISME J. (2017) 11:2047–58. doi: 10.1038/ismej.2017.71
78. Damms-Machado A, Mitra S, Schollenberger AE, Kramer KM, Meile T, Königsrainer A, et al. Effects of surgical and dietary weight loss therapy for obesity on gut microbiota composition and nutrient absorption. Biomed Res Int. (2015) 2015:806248. doi: 10.1155/2015/806248
79. Liu H, Hu C, Zhang X, Jia W. Role of gut microbiota, bile acids and their cross-talk in the effects of bariatric surgery on obesity and type 2 diabetes. J Diabetes Investig. (2018) 9:13–20. doi: 10.1111/jdi.12687
80. Gutiérrez-Repiso C, Moreno-Indias I, de Hollanda A, Martín-Núñez GM, Vidal J, Tinahones FJ. Gut microbiota specific signatures are related to the successful rate of bariatric surgery. Am J Transl Res. (2019) 11:942–52. Available online at: http://www.ajtr.org/files/ajtr0085422.pdf
81. Furet J-P, Kong L-C, Tap J, Poitou C, Basdevant A, Bouillot J-L, et al. Differential adaptation of human gut microbiota to bariatric surgery-induced weight loss: links with metabolic and low-grade inflammation markers. Diabetes. (2010) 59:3049–57. doi: 10.2337/db10-0253
82. Clemente-Postigo M, Queipo-Ortuño MI, Murri M, Boto-Ordoñez M, Perez-Martinez P, Andres-Lacueva C, et al. Endotoxin increase after fat overload is related to postprandial hypertriglyceridemia in morbidly obese patients. J Lipid Res. (2012) 53:973–8. doi: 10.1194/jlr.P020909
83. Cani PD, Possemiers S, Van de Wiele T, Guiot Y, Everard A, Rottier O, et al. Changes in gut microbiota control inflammation in obese mice through a mechanism involving GLP-2-driven improvement of gut permeability. Gut. (2009) 58:1091–103. doi: 10.1136/gut.2008.165886
84. Clemente-Postigo M, Oliva-Olivera W, Coin-Aragüez L, Ramos-Molina B, Giraldez-Perez RM, Lhamyani S, et al. Metabolic endotoxemia promotes adipose dysfunction and inflammation in human obesity. Am J Physiol Endocrinol Metab. (2019) 316:E319–32. doi: 10.1152/ajpendo.00277.2018
85. Shin AC, Zheng H, Berthoud H-R. Vagal innervation of the hepatic portal vein and liver is not necessary for Roux-en-Y gastric bypass surgery-induced hypophagia, weight loss, and hypermetabolism. Ann Surg. (2012) 255:294–301. doi: 10.1097/SLA.0b013e31823e71b7
86. Kentish SJ, Page AJ. The role of gastrointestinal vagal afferent fibres in obesity. J Physiol. (2015) 593:775–86. doi: 10.1113/jphysiol.2014.278226
87. Blasi C. The role of the vagal nucleus tractus solitarius in the therapeutic effects of obesity surgery and other interventional therapies on type 2 diabetes. Obes Surg. (2016) 26:3045–57. doi: 10.1007/s11695-016-2419-2
88. Murphy KG, Bloom SR. Gut hormones and the regulation of energy homeostasis. Nature. (2006) 444:854–9. doi: 10.1038/nature05484
89. Badman MK, Flier JS. The gut and energy balance: visceral allies in the obesity wars. Science. (2005) 307:1909–14. doi: 10.1126/science.1109951
90. Kassir R, Barthelemy J-C, Roche F, Blanc P, Zufferey P, Galusca B, et al. Bariatric surgery associated with percutaneous auricular vagal stimulation: a new prospective treatment on weight loss. Int J Surg Lond Engl. (2015) 18:55–6. doi: 10.1016/j.ijsu.2015.04.033
91. Papasavas P, El Chaar M, Kothari SN, American Society for Metabolic and Bariatric Surgery Clinical Issues Committee. American Society for Metabolic and Bariatric Surgery position statement on vagal blocking therapy for obesity. Surg Obes Relat Dis Off J Am Soc Bariatr Surg. (2016) 12:460–1. doi: 10.1016/j.soard.2015.12.004
92. de Lartigue G, Diepenbroek C. Novel developments in vagal afferent nutrient sensing and its role in energy homeostasis. Curr Opin Pharmacol. (2016) 31:38–43. doi: 10.1016/j.coph.2016.08.007
93. Dixon JB, Lambert EA, Lambert GW. Neuroendocrine adaptations to bariatric surgery. Mol Cell Endocrinol. (2015) 418(Pt 2):143–52. doi: 10.1016/j.mce.2015.05.033
94. Stefanidis A, Oldfield BJ. Neuroendocrine mechanisms underlying bariatric surgery: insights from human studies and animal models. J Neuroendocrinol. (2017) 29:1–7. doi: 10.1111/jne.12534
95. Ballsmider LA, Vaughn AC, David M, Hajnal A, Di Lorenzo PM, Czaja K. Sleeve gastrectomy and Roux-en-Y gastric bypass alter the gut-brain communication. Neural Plast. (2015) 2015:601985. doi: 10.1155/2015/601985
96. Gautron L, Zechner JF, Aguirre V. Vagal innervation patterns following Roux-en-Y gastric bypass in the mouse. Int J Obes 2005. (2013) 37:1603–7. doi: 10.1038/ijo.2013.48
97. Stefanidis A, Forrest N, Brown WA, Dixon JB, O'Brien PB, Juliane Kampe null, et al. An investigation of the neural mechanisms underlying the efficacy of the adjustable gastric band. Surg Obes Relat Dis Off J Am Soc Bariatr Surg. (2016) 12:828–38. doi: 10.1016/j.soard.2015.11.020
98. Dixon AFR, Dixon JB, O'Brien PE. Laparoscopic adjustable gastric banding induces prolonged satiety: a randomized blind crossover study. J Clin Endocrinol Metab. (2005) 90:813–9. doi: 10.1210/jc.2004-1546
99. Björklund P, Laurenius A, Een E, Olbers T, Lönroth H, Fändriks L. Is the Roux limb a determinant for meal size after gastric bypass surgery? Obes Surg. (2010) 20:1408–14. doi: 10.1007/s11695-010-0192-1
100. Sundbom M, Holdstock C, Engström BE, Karlsson FA. Early changes in ghrelin following Roux-en-Y gastric bypass: influence of vagal nerve functionality? Obes Surg. (2007) 17:304–10. doi: 10.1007/s11695-007-9056-8
101. Tamboli RA, Antoun J, Sidani RM, Clements A, Harmata EE, Marks-Shulman P, et al. Metabolic responses to exogenous ghrelin in obesity and early after Roux-en-Y gastric bypass in humans. Diabetes Obes Metab. (2017) 19:1267–75. doi: 10.1111/dom.12952
102. Perathoner A, Weiss H, Santner W, Brandacher G, Laimer E, Höller E, et al. Vagal nerve dissection during pouch formation in laparoscopic Roux-Y-gastric bypass for technical simplification: does it matter? Obes Surg. (2009) 19:412–7. doi: 10.1007/s11695-008-9657-x
103. Ochner CN, Kwok Y, Conceição E, Pantazatos SP, Puma LM, Carnell S, et al. Selective reduction in neural responses to high calorie foods following gastric bypass surgery. Ann Surg. (2011) 253:502–7. doi: 10.1097/SLA.0b013e318203a289
104. Ten Kulve JS, Veltman DJ, Gerdes VEA, van Bloemendaal L, Barkhof F, Deacon CF, et al. Elevated postoperative endogenous GLP-1 levels mediate effects of Roux-en-Y gastric bypass on neural responsivity to food cues. Diabetes Care. (2017) 40:1522–9. doi: 10.2337/dc16-2113
105. Korner J, Inabnet W, Conwell IM, Taveras C, Daud A, Olivero-Rivera L, et al. Differential effects of gastric bypass and banding on circulating gut hormone and leptin levels. Obesity. (2006) 14:1553–61. doi: 10.1038/oby.2006.179
106. Kalinowski P, Paluszkiewicz R, Wróblewski T, Remiszewski P, Grodzicki M, Bartoszewicz Z, et al. Ghrelin, leptin, and glycemic control after sleeve gastrectomy versus Roux-en-Y gastric bypass-results of a randomized clinical trial. Surg Obes Relat Dis Off J Am Soc Bariatr Surg. (2017) 13:181–8. doi: 10.1016/j.soard.2016.08.025
107. DiGiorgi M, Rosen DJ, Choi JJ, Milone L, Schrope B, Olivero-Rivera L, et al. Re-emergence of diabetes after gastric bypass in patients with mid- to long-term follow-up. Surg Obes Relat Dis. (2010) 6:249–53. doi: 10.1016/j.soard.2009.09.019
108. Chikunguwo SM, Wolfe LG, Dodson P, Meador JG, Baugh N, Clore JN, et al. Analysis of factors associated with durable remission of diabetes after Roux-en-Y gastric bypass. Surg Obes Relat Dis. (2010) 6:254–9. doi: 10.1016/j.soard.2009.11.003
109. Mittempergher F, Pat G, Crea N, Di Betta E, Vilardi A, Chiesa D, et al. Preoperative prediction of Growth Hormone (GH)/Insulin-Like Growth Factor-1 (IGF-1) axis modification and postoperative changes in candidates for bariatric surgery. Obes Surg. (2013) 23:594–601. doi: 10.1007/s11695-012-0820-z
110. Camastra S, Manco M, Frascerra S, Iaconelli A, Mingrone G, Ferrannini F. Daylong pituitary hormones in morbid obesity: effects of bariatric surgery. Int J Obes. (2009) 33:166–72. doi: 10.1038/ijo.2008.226
111. De Marinis L, Bianchi A, Mancini A, Gentilella R, Perrelli M, Giampietro A, et al. Growth hormone secretion and leptin in morbid obesity before and after biliopancreatic diversion: relationships with insulin and body composition. J Clin Endocrinol Metab. (2004) 89:174–80. doi: 10.1210/jc.2002-021308
112. Mancini MC, Costa AP, deMelo M-E, Cercato C, Giannella-Neto D, Garrido AB Jr, et al. Effect of gastric bypass on spontaneous growth hormone and ghrelin release profiles. Obesity. (2006) 14:383–7. doi: 10.1038/oby.2006.51
113. Edén Engström B, Burman P, Holdstock C, Ohrvall M, Sundbom M, Karlsson FA. Effects of gastric bypass on the GH/IGF-I axis in severe obesity–and a comparison with GH deficiency. Eur J Endocrinol. (2006) 154:53–9. doi: 10.1530/eje.1.02069
114. Savastano S, Angrisani L, Di Somma C, Rota F, Savanelli MC, Cascella T, et al. Relationship between growth hormone/insulin-like growth factor-1 axis integrity and voluntary weight loss after gastric banding surgery for severe obesity. Obes Surg. (2010) 20:211–20. doi: 10.1007/s11695-009-9926-3
115. Di Somma C, Angrisani L, Rota F, Savanelli MC, Cascella T, Belfiore A, et al. GH and IGF-I deficiency are associated with reduced loss of fat mass after laparoscopic-adjustable silicone gastric banding. Clin Endocrinol. (2008) 69:393–9. doi: 10.1111/j.1365-2265.2008.03183.x
116. Manco M, Fernández-Real JM, Valera-Mora ME, Déchaud H, Nanni G, Tondolo V, et al. Massive weight loss decreases corticosteroid-binding globulin levels and increases free cortisol in healthy obese patients: an adaptive phenomenon? Diabetes Care. (2007) 30:1494–500. doi: 10.2337/dc06-1353
117. Morrow J, Gluck M, Lorence M, Flancbaum L, Geliebter A. Night eating status and influence on body weight, body image, hunger, and cortisol pre- and post- Roux-en-Y Gastric Bypass (RYGB) surgery. Eat Weight Disord. (2008) 13:e96–9. doi: 10.1007/BF03327512
118. Larsen JK, van Ramshorst B, van Doornen LJ, Geenen R. Salivary cortisol and binge eating disorder in obese women after surgery for morbid obesity. Int J Behav Med. (2009) 16:311–5. doi: 10.1007/s12529-009-9036-6
119. Guldstrand M, Bo A, Wredling R, Backman L, Lins PE, Adamson U. Alteration of the counterregulatory responses to insulin-induced hypoglycemia and of cognitive function after massive weight reduction in severely obese subjects. Metabolism. (2003) 52:900–7. doi: 10.1016/S0026-0495(03)00103-3
120. Ruiz-Tovar J, Oller I, Galindo I, Llavero C, Arroyo A, Calero A, et al. Change in levels of C-reactive protein (CRP) and serum cortisol in morbidly obese patients after laparoscopic sleeve gastrectomy. Obes Surg. (2013) 23:764–9. doi: 10.1007/s11695-013-0865-7
121. Valentine AR, Raff H, Liu H, Ballesteros M, Rose JM, Jossart GH, et al. Salivary cortisol increases after bariatric surgery in women. Hormone Metab Res. (2011) 43:587–90. doi: 10.1055/s-0031-1279777
122. Hulme PA, McBride CL, Kupzyk KA, French JA. Pilot study on childhood sexual abuse, diurnal cortisol secretion, and weight loss in bariatric surgery patients. J Child Sex Abus. (2015) 24:385–400. doi: 10.1080/10538712.2015.1022293
123. Sarwer DB, Spitzer JC, Wadden TA, Mitchell JE, Lancaster K, Courcoulas A, et al. Changes in sexual functioning and sex hormone levels in women following bariatric surgery. JAMA Surg. (2014) 149:26–33. doi: 10.1001/jamasurg.2013.5022
124. Jamal M, Gunay Y, Capper A, Eid A, Heitshusen D, Samuel I. Roux-en-Y gastric bypass ameliorates polycystic ovary syndrome and dramatically improves conception rates: a 9-year analysis. Surg Obes Relat Dis. (2012) 8:440–4. doi: 10.1016/j.soard.2011.09.022
125. Eid GM, Cottam DR, Velcu LM, Mattar SG, Korytkowski MT, Gosman G, et al. Effective treatment of polycystic ovarian syndromewith Roux-en-Y gastric bypass. Surg Obes Relat Dis. (2005) 1:77–80. doi: 10.1016/j.soard.2005.02.008
126. George K, Azeez H. Resolution of gynaecological issues after bariatric surgery—a retrospective analysis. Obes Surg. (2013) 23:1043. doi: 10.1007/s11695-013-0986-z
127. Skubleny D, Switzer NJ, Gill RS, Dykstra M, Shi X, Sagle MA, et al. The impact of bariatric surgery on polycystic ovary syndrome: a systematic review and meta-analysis. Obesity Surg. (2016) 26:169–76. doi: 10.1007/s11695-015-1902-5
128. Shekelle PG, Newberry S, Maglione M, Li Z, Yermilov I, Hilton L, et al. Bariatric surgery in women of reproductive age: special concerns for pregnancy. Evid Rep Technol Assess. (2008) 1–51. Available online at: https://www.ncbi.nlm.nih.gov/books/NBK38559/
129. Reis LO, Favaro WJ, Barreiro GC, de Oliveira LC, Chaim EA, Fregonesi A, et al. Erectile dysfunction and hormonal imbalance in morbidly obese male is reversed after gastric bypass surgery: a prospective randomized controlled trial. Int J Androl. (2010) 33:736–44. doi: 10.1111/j.1365-2605.2009.01017.x
130. Mora M, Aranda GB, de Hollanda A, Flores L, Puig-Domingo M, Vidal J. Weight loss is a major contributor to improved sexual function after bariatric surgery. Surg Endosc. (2013) 27:3197–204. doi: 10.1007/s00464-013-2890-y
131. Sarwer DB, Spitzer JC, Wadden TA, Rosen RC, Mitchell JE, Lancaster K, et al. Sexual functioning and sex hormones in men who underwent bariatric surgery. Surg Obes Relat Dis. (2015) 11:643–51. doi: 10.1016/j.soard.2014.12.014
132. Facchiano E, Scaringi S, Veltri M, Samavat J, Maggi M, Forti G, et al. Age as a predictive factor of testosterone improvement in male patients after bariatric surgery: preliminary results of a monocentric prospective study. Obes Surg. (2013) 23:167–72. doi: 10.1007/s11695-012-0753-6
133. Luconi M, Samavat J, Seghieri G, Iannuzzi G, Lucchese M, Rotella C, et al. Determinants of testosterone recovery after bariatric surgery: is it only a matter of reduction of body mass index? Fertil Steril. (2013) 99:1872–9.e1. doi: 10.1016/j.fertnstert.2013.02.039
134. Aarts E, van Wageningen B, Loves S, Janssen I, Berends F, Sweep F, et al. Gonadal status and outcome of bariatric surgery in obese men. Clin Endocrinol. (2014) 81:378–86. doi: 10.1111/cen.12366
135. Samavat J, Facchiano E, Lucchese M, Forti G, Mannucci E, Maggi M, et al. Hypogonadism as an additional indication for bariatric surgery in male morbid obesity? Eur J Endocrinol. (2014) 171:555–60. doi: 10.1530/EJE-14-0596
136. Rasmussen MH. Obesity, growth hormone and weight loss. Mol Cell Endocrinol. (2010) 316:147–53. doi: 10.1016/j.mce.2009.08.017
137. Alvarez P, Isidro L, Leal-Cerro A, Casanueva FF, Dieguez C, Cordido F. Effect of withdrawal of somatostatin plus GH-releasing hormone stimulus of GH secretion in obesity. Clin Endocrinol. (2002) 56: 487–92. doi: 10.1046/j.1365-2265.2002.01487.x
138. Cordido F, Alvarez-Castro P, Isidro MI, Casanueva FF, Dieguez C. Comparison between insulin tolerance test, growth hormone releasing hormone (GHRH), GHRH plus acipimox and GHRH plus GH-releasing peptide-6 for the diagnosis of adult GH deficiency in normal subjects, obese and hypopituitary patients. Eur J Endocrinol. (2003) 149:117–22. doi: 10.1530/eje.0.1490117
139. Savastano S, Di Somma C, Barrea L, Colao A. The complex relationship between obesity and the somatropic axis: the long and winding road. Growth Horm IGF Res. (2014) 24:221–6. doi: 10.1016/j.ghir.2014.09.002
140. Park MJ, Kim HS, Kang JH, Kim DH, Chung CY. Serum levels of insulin-like growth factor (IGF)-I, free IGF-I, IGF binding (IGFBP)-1, IGFBP-3 and insulin in obese children. J Pediatr Endocrinol Metab. (1999) 12:139–44. doi: 10.1515/JPEM.1999.12.2.139
141. Ballerini MG, Ropelato MG, Domene HM, Pennisi P, Heinrich JJ, Jasper HG. Differential impact of simple childhood obesity on the components of the hormone-insulin-like growth factor (IGF)-IGF binding proteins axis. J Pediatr Endocrinol Metab. (2004) 17:749–57. doi: 10.1515/JPEM.2004.17.5.749
142. Attia N, Tamborlane WV, Heptulla R, Maggs D, Grozman A, Sherwin RS, et al. The metabolic syndrome and insulinlike growth factor I regulation in adult obesity. J Clin Endocrinol Metab. (1998) 83:1467–71. doi: 10.1210/jcem.83.5.4827
143. Street ME, Ziveri MA, Spaggiari C, Viani I, Volta C, Grzincich GL, et al. Inflammation is a modulator of the insulin-like growth factor (IGF)/IGF-binding protein system inducing reduced bioactivity of IGFs in cystic fibrosis. Eur J Endocrinol. (2006) 154:47–52. doi: 10.1530/eje.1.02064
144. Rasmussen MH, Juul A, Hilsted J. Effect of weight loss on free insulin-like growth factor-I in obese women with hyposomatotropism. Obesity. (2007) 15:879–86. doi: 10.1038/oby.2007.607
145. Johnsen SP, Hundborg HH, Sorensen HT, Schumacher H, Lichtinghagen R, Eschenfelder CC, et al. Insulin-like growth factor (IGF) I, -II, and IGF binding protein-3 and risk of ischemic stroke. J Clin Endocrinol Metab. (2005) 90: 5937–41. doi: 10.1210/jc.2004-2088
146. Maccario M, Grottoli S, Procopio M, Oleandri SE, Rossetto E, Gauna C, et al. The GH/IGF-I axis in obesity: influence of neuro-endocrine and metabolic factors. Int J. Obes Relat Metab Disord. (2000) 24:S96–9. doi: 10.1038/sj.ijo.0801289
147. Berryman DE, Gla CA, List EO, Johannsson G. The GH/IGF-1 axis in obesity: pathophysiology and therapeutic considerations, Nat Rev Endocrinol. (2013) 9:346–56. doi: 10.1038/nrendo.2013.64
148. Savastano S, Di Somma C, Belfiore A, Guida B, Orio F Jr, Rota F, et al. Growth hormone status in morbidly obese subjects and correlation with body composition. J Endocrinol Invest. (2006) 29:536–43. doi: 10.1007/BF03344144
149. Galli G, Pinchera A, Piaggi P, Fierabracci P, Giannetti M, Querci G, et al. Serum insulin-like growth factor-1 concentrations are reduced in severely obese women and raise after weight loss induced by laparoscopic adjustable gastric banding. Obes Surg. (2012) 22:1276–80. doi: 10.1007/s11695-012-0669-1
150. Maccario M, Tassone F, Gauna C, Oleandri SE, Aimaretti G, Procopio M, et al. Effects of short-term administration of low-dose rhGH on IGF-I levels in obesity and Cushing's syndrome: indirect evaluation of the sensitivity to GH. Eur J Endocrinol. (2001) 144:251–6. doi: 10.1530/eje.0.1440251
151. Gianotti L, Pivetti S, Lanfranco F, Tassone F, Vittori E, Rossetto R, et al. Concomitant impairment of growth hormone secretion and peripheral sensitivity in obese patients with obstructive sleep apnoea syndrome. J Clin Endocrinol Metab. (2002) 87:5052–7. doi: 10.1210/jc.2001-011441
152. Buijs MM, Romijn JA, Burggraaf J, De Kam MI, Cohen AF, Frolich M, et al. Growth hormone blunts protein oxidation and promotes protein turnover to a similar extent in abdominally obese and normal-weight women. J Clin Endocrinol Metab. (2002) 87:5668–74. doi: 10.1210/jc.2002-020927
153. Biondi M, Picardi A. Psychological stress and neuroendocrine function in humans: the last two decades of research. Psychother Psychosom. (1999) 68:114–50. doi: 10.1159/000012323
154. Anagnostis P, Athyros VG, Tziomalos K, Karagiannis A, Mikhailidis DP. Clinical review: the pathogenetic role of cortisol in the metabolic syndrome: a hypothesis. J Clin Endocrinol Metab. (2009) 94:2692–701. doi: 10.1210/jc.2009-0370
155. Constantinopoulos P, Michalaki M, Kottorou A, Habeos I, Psyrogiannis A, Kalfarentzos F, et al. Cortisol in tissue and systemic level as a contributing factor to the development of metabolic syndrome in severely obese patients. Eur J Endocrinol. (2015) 172:69–78. doi: 10.1530/EJE-14-0626
156. Rosmond R, Holm G, Bjorntorp P. Food-induced cortisol secretion in relation to anthropometric, metabolic and haemodynamic variables in men. Int J Obes Relat Metab Disord. (2000) 24:416–22. doi: 10.1038/sj.ijo.0801173
158. De Kloet ER. Hormones and the stressed brain. Ann N YAcad Sci. (2004) 1018:1–15. doi: 10.1196/annals.1296.001
159. Kumari M, Chandola T, Brunner E, Kivimaki M. A nonlinear relationship of generalized and central obesity with diurnal cortisol secretion in the Whitehall II study. J Clin Endocr Metab. (2010) 95:4415–23. doi: 10.1210/jc.2009-2105
160. Lasikiewicz N, Hendrickx H, Talbot D, Dye L. Exploration of basal diurnal salivary cortisol profiles in middle-aged adults: associations with sleep quality and metabolic parameters. Psychoneuroendocrinology. (2008) 33:143–51. doi: 10.1016/j.psyneuen.2007.10.013
161. De Kloet ER, Joels M, Holsboer F, Joëls M. Stress and the brain: from adaptation to disease. Nat Rev Neurosci. (2005) 6:463–75. doi: 10.1038/nrn1683
162. Kumari M, Shipley M, Stafford M, Kivimaki M. Association of diurnal patterns in salivary cortisol with all-cause and cardiovascular mortality: findings from the Whitehall II study. J Clin Endocrinol Metab. (2011) 96:1478–85. doi: 10.1210/jc.2010-2137
163. McEwen BS. The neurobiology of stress: from serendipity to clinical relevance. Brain Res. (2000) 886:172–89. doi: 10.1016/S0006-8993(00)02950-4
164. Dallman MF, Akana SF, Strack AM, Hanson ES, Sebastian RJ. The neural network that regulates energy balance is responsive to glucocorticoids and insulin and also regulates HPA axis responsivity at a site proximal to CRF neurons. Ann N Y Acad Sci. (1995) 771:730–42. doi: 10.1111/j.1749-6632.1995.tb44724.x
165. Jayo JM, Shively CA, Kaplan JR, Manuck SB. Effects of exercise and stress on body fat distribution in male cynomolgus monkeys. Int J Obes Relat Metab Disord. (1993) 17:597–604.
166. BjÖrntorp P, Rosmond R. Neuroendocrine abnormalities in visceral obesity. Int J Obes Relat Metab Disord. (2000) 24(Suppl. 2):S80–5. doi: 10.1038/sj.ijo.0801285
167. Reinehr T, Kulle A, Wolters B, Knop C, Lass N, Welzel M, et al. Relationships between 24-hour urinary free cortisol concentrations and metabolic syndrome in obese children. J Clin Endocrinol Metab. (2014) 99:2391–9. doi: 10.1210/jc.2013-4398
168. Geliebter A. Night-eating syndrome in obesity. Nutrition. (2001) 17:483–4. doi: 10.1016/S0899-9007(01)00550-0
169. Coutinho WF, Moreira RO, Spagnol C, Appolinario JC. Does binge eating disorder alter cortisol secretion in obese women? Eat Behav. (2007) 8:59–64. doi: 10.1016/j.eatbeh.2006.01.002
170. Monteleone P, Luisi M, De Filippis G, Colurcio B, Genazzani AR, Maj M. Circulating levels of neuroactive steroids in patients with binge eating disorder: a comparison with nonobese healthy controls and nonbinge eating obese subjects. Int J Eat Disord. (2003) 34:432–40. doi: 10.1002/eat.10199
171. Gluck ME, Geliebter A, Hung J, Yahav E. Cortisol, hunger, and desire to binge eat following a cold stress test in obese women with binge eating disorder. Psychosom Med. (2004) 66:876–81. doi: 10.1097/01.psy.0000143637.63508.47
172. Yanovski SZ. Binge eating disorder: current knowledge and future directions. Obes Res. (1993) 1:306–24. doi: 10.1002/j.1550-8528.1993.tb00626.x
173. Reinher T, Andler W. Cortisol and its relation to insulin resistance before and after weight loss in obese children. Horm Res. (2004) 62:107–12. doi: 10.1159/000079841
174. Flak JN, Jankord R, Solomon MB, Krause EG, Herman JP. Opposing effects of chronic stress and weight restriction on cardiovascular, neuroendocrine and metabolic function. Physiol Behav. (2011) 104:228–34. doi: 10.1016/j.physbeh.2011.03.002
175. Pankevich DE, Teegarden SL, Hedin AD, Jensen CL, Bale TL. Caloric restriction experience reprograms stress and orexigenic pathways and promotes binge eating. J Neurosci. (2010) 30:16399–407. doi: 10.1523/JNEUROSCI.1955-10.2010
176. Ulrich-Lai YM, Ryan KK. Neuroendocrine circuits governing energy balance and stress regulation: functional overlap and therapeutic implications. Cell Metab. (2014) 19:910–25. doi: 10.1016/j.cmet.2014.01.020
177. Witbracht MG, Laugero KD, Van Loan MD, Adams SH, Keim NL. Performance on the Iowa Gambling Task is related to magnitude of weight loss and salivary cortisol in a diet-induced weight loss intervention in overweight women. Physiol Behav. (2012) 106:291–7. doi: 10.1016/j.physbeh.2011.04.035
178. Tomiyama AJ, Mann T, Vinas D, Hunger JM, Dejager J, Taylor SE. Low calorie dieting increases cortisol. Psychosom Med. (2010) 72:357–64. doi: 10.1097/PSY.0b013e3181d9523c
179. Goodwin GM, Fairburn CG, Keenan JC, Cowen PJ. The effects of dieting and weight loss upon the stimulation of thyrotropin (TSH) by thyrotropin-releasing hormone (TRH) and suppression of cortisol secretion by dexamethasone in men and women. J Affect Disord. (1988) 14:137–44. doi: 10.1016/0165-0327(88)90056-0
180. Nguyen NT, Goldman CD, Ho HS, Gosselin RC, Singh A, Wolfe BM. Systemic stress response after laparoscopic and open gastric bypass. J Am Coll Surg. (2002) 194:557–66. doi: 10.1016/S1072-7515(02)01132-8
181. Quaroni A, Tian JQ, Goke M, Podolsky DK. Glucocorticoids have pleiotropic effects on small intestinal crypt cells. Am J Physiol. (1999) 277:G1027–40. doi: 10.1152/ajpgi.1999.277.5.G1027
182. Pasquali R. Obesity, fat distribution and infertility. Maturitas. (2006) 54:363–71. doi: 10.1016/j.maturitas.2006.04.018
183. Rosen RC, Wing RR, Schneider S, Wadden TA, Foster GD, West DS, et al. Erectile dysfunction in type 2 diabetic men: relationship to exercise fitness and cardiovascular risk factors in the Look AHEAD trial. J Sex Med. (2009) 6:1414–22. doi: 10.1111/j.1743-6109.2008.01209.x
184. De Berardis G, Franciosi M, Belfiglio M, Di Nardo B, Greenfield S, Kaplan SH, et al. Erectile dysfunction and quality of life in type 2 diabetic patients: a serious problem too often overlooked. Diabetes Care. (2002) 25:284–91. doi: 10.2337/diacare.25.2.284
185. Duncan LE, Lewis C, Jenkins P, Pearson TA. Does hypertension and its pharmacotherapy affect the quality of sexual function in women? Am J Hypertens. (2000) 13:640–7. doi: 10.1016/S0895-7061(99)00288-5
186. Grimm RH Jr, Grandits GA, Prineas RJ, McDonald RH, Lewis CE, Flack JM, et al. Long-term effects on sexual function of five antihypertensive drugs and nutritional hygienic treatment in hypertensive men and women. Treatment of Mild Hypertension Study (TOMHS). Hypertension. (1997) 29:8–14. doi: 10.1161/01.HYP.29.1.8
187. Dallal RM, Chernoff A, O'Leary MP, Smith JA, Braverman JD, Quebbemann BB. Sexual dysfunction is common in the morbidly obese male and improves after gastric bypass surgery. J Am Coll Surg. (2008) 207:859–64. doi: 10.1016/j.jamcollsurg.2008.08.006
188. Kirschner MA, Samojlik E. Sex hormone metabolism in upper and lower body obesity. Int J Obes. (1991) 15:101–8.
189. Moore RH, Sarwer DB, Lavenberg J, Lane IB, Evans JL, Volger S, et al. Relationship between sexual function and quality of life in persons seeking weight reduction. Obesity. (2013) 21:1966–74. doi: 10.1002/oby.20398
190. Steffen KJ, King WC, White GE, Subak LL, Mitchell JE, Courcoulas AP, et al. Sexual functioning of men and women with severe obesity before bariatric surgery. Surg Obes Relat Dis. (2017) 13:334–43. doi: 10.1016/j.soard.2016.09.022
191. Escobar-Morreale HF, Santacruz E, Luque-Ramírez M, Botella Carretero JI. Prevalence of “obesity-associated gonadal dysfunction” in severely obese men and women and its resolution after bariatric surgery: a systematic review and meta-analysis. Hum Reprod Update. (2017) 23:390–408. doi: 10.1093/humupd/dmx012
192. Sarwer DB, Spitzer JC, Wadden TA, Rosen RC, Mitchell JE, Lancaster K, et al. Sexual functioning and sex hormones in persons with extreme obesity and seeking surgical and nonsurgical weight loss. Surg Obes Relat Dis. (2013) 9:997–1007. doi: 10.1016/j.soard.2013.07.003
193. Corona G, Rastrelli G, Monami M, Saad F, Luconi M, Lucchese M, et al. Body weight loss reverts obesity-associated hypogonadotropic hypogonadism: a systematic review and meta-analysis. Eur J Endocrinol. (2013) 168:829–43. doi: 10.1530/EJE-12-0955
194. Bond DS, Wing RR, Vithiananthan S, Sax HC, Roye GD, Ryder BA, et al. Significant resolution of female sexual dysfunction after bariatric surgery. Surg Obes Relat Dis. (2011) 7:1–7. doi: 10.1016/j.soard.2010.05.015
195. Pellitero S, Olaizola I, Alastrue A, Martínez E, Granada ML, Balibrea JM, et al. Hypogonadotropic hypogonadism in morbidly obese males is reversed after bariatric surgery. Obes Surg. (2012) 22:1835–42. doi: 10.1007/s11695-012-0734-9
196. Saboor Aftab SA, Kumar S, Barber TM. The role of obesity and type 2 diabetes mellitus in the development of male obesity-associated secondary hypogonadism. Clin Endocrinol. (2013) 78:330–7. doi: 10.1111/cen.12092
197. Nelson LR, Bulun SE. Estrogen production and action. J Am Acad Dermatol. (2001) 45:S116–24. doi: 10.1067/mjd.2001.117432
198. Escobar-Morreale HF, Botella-Carretero JI, Alvarez-Blasco F, Sancho J, San Millan JL. The polycystic ovary syndrome associated with morbid obesity may resolve after weight loss induced by bariatric surgery. J Clin Endocrinol Metab. (2005) 90:6364–9. doi: 10.1210/jc.2005-1490
199. Escobar-Morreale HF, San Millan JL. Abdominal adiposity and the polycystic ovary syndrome. Trends Endocrinol Metab. (2007) 18:266–72. doi: 10.1016/j.tem.2007.07.003
200. Pasquali R, Diamanti-Kandarakis E, Gambineri A. Secondary polycystic ovary syndrome: theoretical and practical aspects. Eur J Endocrinol. (2016) 175:R157–69. doi: 10.1530/EJE-16-0374
201. Wing RR, Bond DS, Gendrano IN III, Wadden T, Bahnson J, Lewis CE, et al. Sexual Dysfunction Subgroup of the Look AHEAD Research Group. Effect of intensive lifestyle intervention on sexual dysfunction in women with type 2 diabetes: results from an ancillary Look AHEAD study. Diabetes Care. (2013) 36:2937–44. doi: 10.2337/dc13-0315
202. Reis LO, Zani EL, Saad RD, Chaim EA, de Oliveira LC, Fregonesi A. Bariatric surgery does not interfere with sperm quality–a preliminary long-term study. Reprod Sci. (2012) 19:1057–62. doi: 10.1177/1933719112440747
203. Kaukua J, Pekkarinen T, Sane T, Mustajoki P. Sex hormones and sexual function in obese men losing weight. Obes Res. (2003) 11:689–94. doi: 10.1038/oby.2003.98
204. Butterworth J, Deguara J, Borg CM. Bariatric surgery, polycystic ovary syndrome, and infertility. J Obes. (2016) 2016:1871594. doi: 10.1155/2016/1871594
205. Balen AH, Morley LC, Misso M, Franks S, Legro RS, Wijeyaratne CN, et al. The management of anovulatory infertility in women with polycystic ovary syndrome: an analysis of the evidence to support the development of global WHO guidance. Hum Reprod Update. (2016) 22:687–708. doi: 10.1093/humupd/dmw025
206. Bonomi M, Vezzoli V, Krausz C, Guizzardi F, Vezzani S, Simoni M, et al. Characteristics of a nationwide cohort of patients presenting with isolated hypogonadotropic hypogonadism (IHH). Eur J Endocrinol. (2018) 178:23–32. doi: 10.1530/EJE-17-0065
207. Rao SR, Kini S, Tamler R. Sex hormones and bariatric surgery in men. Gend Med. (2011) 8:300–11. doi: 10.1016/j.genm.2011.05.007
208. Tajar A, Forti G, O'Neill TW, Lee DM, Silman AJ, Finn JD, et al. Characteristics of secondary, primary, and compensated hypogonadism in aging men: evidence from the European Male Ageing Study. J ClinEndocrinol Metab. (2010) 95:1810–8. doi: 10.1210/jc.2009-1796
209. Hofstra J, Loves S, van Wageningen B, Ruinemans-Koerts J, Jansen I, de Boer H. High prevalence of hypogonadotropic hypogonadism in men referred for obesity treatment. Neth J Med. (2008) 66:103–109. Available online at: http://www.njmonline.nl/getpdf.php?id=637
210. Vermeulen A, Kaufman JM, Deslypere JP, Thomas G. Attenuated luteinizing hormone (LH) pulse amplitude but normal LH pulse frequency, and its relation to plasma androgens in hypogonadism of obese men. J Clin Endocrinol Metab. (1993) 76:1140–6. doi: 10.1210/jcem.76.5.8496304
211. Landry D, Cloutier F, Martin LJ. Implications of leptin in neuroendocrine regulation of male reproduction. Reprod Biol. (2013) 13:1–14. doi: 10.1016/j.repbio.2012.12.001
212. Bruning JC, Gautam D, Burks DJ, Gillette J, Schubert M, Orban PC, et al. Role of brain insulin receptor in control of body weight and reproduction. Science. (2000) 289:2122–5. doi: 10.1126/science.289.5487.2122
213. Simo R, Saez-Lopez C, Barbosa-Desongles A, Hernandez C, Selva DM. Novel insights in SHBG regulation and clinical implications. Trends Endocrinol Metab. (2015) 26:376–83. doi: 10.1016/j.tem.2015.05.001
214. Luboshitzky R, Aviv A, Hefetz A, Herer P, Shen-Orr Z, Lavie L, et al. Decreased pituitary-gonadal secretion in men with obstructive sleep apnea. J Clin Endocrinol Metab. (2002) 87:3394–8. doi: 10.1210/jc.87.7.3394
215. Michalakis K, Mintziori G, Kaprara A, Tarlatzis BC, Goulis DG. The complex interaction between obesity, metabolic syndrome and reproductive axis: a narrative review. Metabolism. (2013) 62:457–78. doi: 10.1016/j.metabol.2012.08.012
216. Burcelin R, Thorens B, Glauser M, Gaillard RC, Pralong FP. Gonadotropin-releasing hormone secretion from hypothalamic neurons: stimulation by insulin and potentiation by leptin. Endocrinology. (2003) 144:4484–91. doi: 10.1210/en.2003-0457
217. Dandona P, Dhindsa S. Update: hypogonadotropic hypogonadism in type 2 diabetes and obesity. J Clin Endocrinol Metab. (2011) 96: ,2643–51. doi: 10.1210/jc.2010-2724
218. Escobar-Morreale HF, Alvarez-Blasco F, Botella-Carretero JI, Luque-Ramirez M. The striking similarities in the metabolic associations of female androgen excess and male androgen deficiency. Hum Reprod. (2014) 29:2083–91. doi: 10.1093/humrep/deu198
219. Lazaros L, Hatzi E, Markoula S, Takenaka A, Sofikitis N, Zikopoulos K, et al. Dramatic reduction in sperm parameters following bariatric surgery: report of two cases. Andrologia. (2012) 44:428–32. doi: 10.1111/j.1439-0272.2012.01300.x
220. Sermondade N, Massin N, Boitrelle F, Pfeffer J, Eustache F, Sifer C, et al. Sperm parameters and male fertility after bariatric surgery: three case series. Reprod Biomed Online. (2012) 24:206–10. doi: 10.1016/j.rbmo.2011.10.014
221. Legro RS, Kunselman AR, Meadows JW, Kesner JS, Krieg EF, Rogers AM, et al. Time-related increase in urinary testosterone levels and stable semen analysis parameters after bariatric surgery in men. Reprod Biomed Online. (2015) 30:150–6. doi: 10.1016/j.rbmo.2014.10.014
222. El Bardisi H, Majzoub A, Arafa M, AlMalki A, Al Said S, Khalafalla K, et al. Effect of bariatric surgery on semen parameters and sex hormone concentrations: a prospective study. Reprod Biomed Online. (2016) 33:606–11. doi: 10.1016/j.rbmo.2016.08.008
223. Hammoud A, Gibson M, Hunt SC, Adams TD, Carrell DT, Kolotkin RL, et al. Effect of Roux-en-Y gastric bypass surgery on the sex steroids and quality of life in obese men. J Clin Endocrinol Metab. (2009) 94:1329–32. doi: 10.1210/jc.2008-1598
224. Hammoud AO, Gibson M, Peterson CM, Meikle AW, Carrell DT. Impact of male obesity on infertility: a critical review of the current literature. Fertil Steril. (2008) 90:897–904. doi: 10.1016/j.fertnstert.2008.08.026
225. Leenen R, van der Kooy K, Seidell JC, Deurenberg P, Koppeschaar HP. Visceral fat accumulation in relation to sex hormones in obese men and women undergoing weight loss therapy. J Clin Endocrinol Metab. (1994) 78:1515–20. doi: 10.1210/jcem.78.6.8200956
226. Rosenblatt A, Faintuch J, Cecconello I. Sexual hormones and erectile function more than 6 years after bariatric surgery. Surg Obes Relat Dis. (2013) 9: 636–40. doi: 10.1016/j.soard.2012.06.010
227. Oury F, Sumara G, Sumara O, Ferron M, Chang H, Smith CE, et al. Endocrine regulation of male fertility by the skeleton. Cell. (2011) 144: 796–809. doi: 10.1016/j.cell.2011.02.004
228. Smith LB, Saunders PT. The skeleton: the new controller of male fertility? Cell. (2011) 144: 642–3. doi: 10.1016/j.cell.2011.02.028
229. Kirmani S, Atkinson EJ, Melton LJ III, Riggs BL, Amin S, Khosla S. Relationship of testosterone and osteocalcin levels during growth. J Bone Miner Res. (2011) 26:2212–6. doi: 10.1002/jbmr.421
230. Bolland MJ, Grey A, Horne AM, Reid IR. Testosterone levels following decreases in serum osteocalcin. Calcif Tissue Int. (2013) 93: 133–6. doi: 10.1007/s00223-013-9730-x
231. Hannemann A, Breer S, Wallaschofski H, Nauck M, Baumeister SE, Barvencik F, et al. Osteocalcin is associated with testosterone in the general population and selected patients with bone disorders. Andrology. (2013) 1:469–74. doi: 10.1111/j.2047-2927.2012.00044.x
232. Foresta C, Strapazzon G, De Toni L, Gianesello L, Calcagno A, Pilon C, et al. Evidence for osteocalcin production by adipose tissue and its role in human metabolism. J Clin Endocrinol Metab. (2010) 95: 3502–6. doi: 10.1210/jc.2009-2557
233. Kim SH, Lee JW, Im JA, Hwang HJ. Serum osteocalcin is related to abdominal obesity in Korean obese and overweight men. Clin Chim Acta. (2010) 411:2054–7. doi: 10.1016/j.cca.2010.08.046
234. Samavat J, Facchiano E, Cantini G, Di Franco A, Alpigiano G, Poli G, et al. Osteocalcin increase after bariatric surgery predicts androgen recovery in hypogonadal obese males. Int J Obes. (2014) 38:357–63. doi: 10.1038/ijo.2013.228
235. Oury F, Ferron M, Huizhen W, Confavreux C, Xu L, Lacombe J, et al. Osteocalcin regulates murine and human fertility through a pancreas-bone-testis axis. J Clin Invest. (2013) 123:2421–33. doi: 10.1172/JCI65952
236. Rastrelli G, Carter EL, Ahern T, Finn JD, Antonio L, O'Neill TW, et al. Development of and recovery from secondary hypogonadism in aging men: prospective results from the EMAS. J Clin Endocrinol Metab. (2015) 100:3172–82. doi: 10.1210/jc.2015-1571
237. Hoeger KM. Role of lifestyle modification in the management of polycystic ovary syndrome. Best Pract Res Clin Endocrinol Metab. (2006) 20:293–310. doi: 10.1016/j.beem.2006.03.008
238. Clemente-Postigo M, Muñoz-Garach A, Serrano M, Garrido-Sánchez L, Bernal-López MR, Fernández-García D, et al. Serum 25-hydroxyvitamin D and adipose tissue vitamin D receptor gene expression: relationship with obesity and type 2 diabetes. J Clin Endocrinol Metab. (2015) 100:E591–5. doi: 10.1210/jc.2014-3016
239. Hamoui N, Kim K, Anthone G, Crookes PF. The significance of elevated levels of parathyroid hormone in patients with morbid obesity before and after bariatric surgery. Arch Surg Chic Ill. 1960. (2003) 138:891–7. doi: 10.1001/archsurg.138.8.891
240. Gómez-Ambrosi J, Rodríguez A, Catalán V, Frühbeck G. The bone-adipose axis in obesity and weight loss. Obes Surg. (2008) 18:1134–43. doi: 10.1007/s11695-008-9548-1
241. Folli F, Sabowitz BN, Schwesinger W, Fanti P, Guardado-Mendoza R, Muscogiuri G. Bariatric surgery and bone disease: from clinical perspective to molecular insights. Int J Obes. 2005. (2012) 36:1373–9. doi: 10.1038/ijo.2012.115
242. Gómez JM, Vilarrasa N, Masdevall C, Pujol J, Solano E, Soler J, et al. Regulation of bone mineral density in morbidly obese women: a cross-sectional study in two cohorts before and after bypass surgery. Obes Surg. (2009) 19:345–50. doi: 10.1007/s11695-008-9529-4
243. Corbeels K, Verlinden L, Lannoo M, Simoens C, Matthys C, Verstuyf A, et al. Thin bones: Vitamin D and calcium handling after bariatric surgery. Bone Rep. (2018) 8:57–63. doi: 10.1016/j.bonr.2018.02.002
244. Stein EM, Silverberg SJ. Bone loss after bariatric surgery: causes, consequences, and management. Lancet Diabetes Endocrinol. (2014) 2:165–74. doi: 10.1016/S2213-8587(13)70183-9
245. Ben-Porat T, Elazary R, Sherf-Dagan S, Goldenshluger A, Brodie R, Mintz Y, et al. Bone Health following Bariatric Surgery: implications for management strategies to attenuate bone loss. Adv Nutr Bethesda Md. (2018) 9:114–27. doi: 10.1093/advances/nmx024
246. Schafer AL, Kazakia GJ, Vittinghoff E, Stewart L, Rogers SJ, Kim TY, et al. Effects of gastric bypass surgery on bone mass and microarchitecture occur early and particularly impact postmenopausal women. J Bone Miner Res Off J Am Soc Bone Miner Res. (2018) 33:975–86. doi: 10.1002/jbmr.3371
247. Casagrande DS, Repetto G, Mottin CC, Shah J, Pietrobon R, Worni M, et al. Changes in bone mineral density in women following 1-year gastric bypass surgery. Obes Surg. (2012) 22:1287–92. doi: 10.1007/s11695-012-0687-z
248. Carrasco F, Ruz M, Rojas P, Csendes A, Rebolledo A, Codoceo J, et al. Changes in bone mineral density, body composition and adiponectin levels in morbidly obese patients after bariatric surgery. Obes Surg. (2009) 19:41–6. doi: 10.1007/s11695-008-9638-0
249. Mahdy T, Atia S, Farid M, Adulatif A. Effect of Roux-en Y gastric bypass on bone metabolism in patients with morbid obesity: mansoura experiences. Obes Surg. (2008) 18:1526–31. doi: 10.1007/s11695-008-9653-1
250. Vilarrasa N, Gómez JM, Elio I, Gómez-Vaquero C, Masdevall C, Pujol J, et al. Evaluation of bone disease in morbidly obese women after gastric bypass and risk factors implicated in bone loss. Obes Surg. (2009) 19:860–6. doi: 10.1007/s11695-009-9843-5
251. Fleischer J, Stein EM, Bessler M, Della Badia M, Restuccia N, Olivero-Rivera L, et al. The decline in hip bone density after gastric bypass surgery is associated with extent of weight loss. J Clin Endocrinol Metab. (2008) 93:3735–40. doi: 10.1210/jc.2008-0481
252. Shanbhogue VV, Støving RK, Frederiksen KH, Hanson S, Brixen K, Gram J, et al. Bone structural changes after gastric bypass surgery evaluated by HR-pQCT: a two-year longitudinal study. Eur J Endocrinol. (2017) 176:685–93. doi: 10.1530/EJE-17-0014
253. Rousseau C, Jean S, Gamache P, Lebel S, Mac-Way F, Biertho L, et al. Change in fracture risk and fracture pattern after bariatric surgery: nested case-control study. BMJ. (2016) 354:i3794. doi: 10.1136/bmj.i3794
254. Vilarrasa N, de Gordejuela AGR, Gómez-Vaquero C, Pujol J, Elio I, San José P, et al. Effect of bariatric surgery on bone mineral density: comparison of gastric bypass and sleeve gastrectomy. Obes Surg. (2013) 23:2086–91. doi: 10.1007/s11695-013-1016-x
255. Elias E, Casselbrant A, Werling M, Abegg K, Vincent RP, Alaghband-Zadeh J, et al. Bone mineral density and expression of vitamin D receptor-dependent calcium uptake mechanisms in the proximal small intestine after bariatric surgery. Br J Surg. (2014) 101:1566–75. doi: 10.1002/bjs.9626
256. Raoof M, Näslund I, Rask E, Szabo E. Effect of gastric bypass on bone mineral density, parathyroid hormone and vitamin D: 5 years follow-up. Obes Surg. (2016) 26:1141–5. doi: 10.1007/s11695-016-2114-3
257. Compston JE, Vedi S, Gianetta E, Watson G, Civalleri D, Scopinaro N. Bone histomorphometry and vitamin D status after biliopancreatic bypass for obesity. Gastroenterology. (1984) 87:350–6. doi: 10.1016/0016-5085(84)90712-1
258. Tsiftsis DDA, Mylonas P, Mead N, Kalfarentzos F, Alexandrides TK. Bone mass decreases in morbidly obese women after long limb-biliopancreatic diversion and marked weight loss without secondary hyperparathyroidism. A physiological adaptation to weight loss? Obes Surg. (2009) 19:1497–503. doi: 10.1007/s11695-009-9938-z
259. Marceau P, Biron S, Lebel S, Marceau S, Hould FS, Simard S, et al. Does bone change after biliopancreatic diversion? J Gastrointest Surg Off J Soc Surg Aliment Tract. (2002) 6:690–8. doi: 10.1016/S1091-255X(01)00086-5
260. Nogués X, Goday A, Peña MJ, Benaiges D, de Ramón M, Crous X, et al. [Bone mass loss after sleeve gastrectomy: a prospective comparative study with gastric bypass]. Cirugia Espanola. (2010) 88:103–9. doi: 10.1016/S2173-5077(10)70015-6
261. Ivaska KK, Huovinen V, Soinio M, Hannukainen JC, Saunavaara V, Salminen P, et al. Changes in bone metabolism after bariatric surgery by gastric bypass or sleeve gastrectomy. Bone. (2017) 95:47–54. doi: 10.1016/j.bone.2016.11.001
262. Carrasco F, Basfi-Fer K, Rojas P, Valencia A, Csendes A, Codoceo J, et al. Changes in bone mineral density after sleeve gastrectomy or gastric bypass: relationships with variations in vitamin D, ghrelin, and adiponectin levels. Obes Surg. (2014) 24:877–84. doi: 10.1007/s11695-014-1179-0
263. Bredella MA, Greenblatt LB, Eajazi A, Torriani M, Yu EW. Effects of Roux-en-Y gastric bypass and sleeve gastrectomy on bone mineral density and marrow adipose tissue. Bone. (2017) 95:85–90. doi: 10.1016/j.bone.2016.11.014
264. Pluskiewicz W, BuŽga M, Holéczy P, Bortlík L, Šmajstrla V, Adamczyk P. Bone mineral changes in spine and proximal femur in individual obese women after laparoscopic sleeve gastrectomy: a short-term study. Obes Surg. (2012) 22:1068–76. doi: 10.1007/s11695-012-0654-8
265. Pugnale N, Giusti V, Suter M, Zysset E, Héraïef E, Gaillard RC, et al. Bone metabolism and risk of secondary hyperparathyroidism 12 months after gastric banding in obese pre-menopausal women. Int J Obes Relat Metab Disord J Int Assoc Study Obes. (2003) 27:110–6. doi: 10.1038/sj.ijo.0802177
266. Giusti V, Gasteyger C, Suter M, Heraief E, Gaillard RC, Burckhardt P. Gastric banding induces negative bone remodelling in the absence of secondary hyperparathyroidism: potential role of serum C telopeptides for follow-up. Int J Obes 2005. (2005) 29:1429–35. doi: 10.1038/sj.ijo.0803040
267. Dixon JB, Strauss BJG, Laurie C, O'Brien PE. Changes in body composition with weight loss: obese subjects randomized to surgical and medical programs. Obes Silver Spring Md. (2007) 15:1187–98. doi: 10.1038/oby.2007.639
268. von Mach M-A, Stoeckli R, Bilz S, Kraenzlin M, Langer I, Keller U. Changes in bone mineral content after surgical treatment of morbid obesity. Metabolism. (2004) 53:918–21. doi: 10.1016/j.metabol.2004.01.015
269. Scibora LM, Ikramuddin S, Buchwald H, Petit MA. Examining the link between bariatric surgery, bone loss, and osteoporosis: a review of bone density studies. Obes Surg. (2012) 22:654–67. doi: 10.1007/s11695-012-0596-1
270. Lalmohamed A, de Vries F, Bazelier MT, Cooper A, van Staa T-P, Cooper C, et al. Risk of fracture after bariatric surgery in the United Kingdom: population based, retrospective cohort study. BMJ. (2012) 345:e5085. doi: 10.1136/bmj.e5085
271. Nakamura KM, Haglind EGC, Clowes JA, Achenbach SJ, Atkinson EJ, Melton LJ, et al. Fracture risk following bariatric surgery: a population-based study. Osteoporos Int. (2014) 25:151–8. doi: 10.1007/s00198-013-2463-x
272. Axelsson KF, Werling M, Eliasson B, Szabo E, Näslund I, Wedel H, et al. Fracture risk after gastric bypass surgery: a retrospective cohort study. J Bone Miner Res. (2018) 33:2122–31. doi: 10.1002/jbmr.3553
273. Lu C-W, Chang Y-K, Chang H-H, Kuo C-S, Huang C-T, Hsu C-C, et al. Fracture risk after bariatric surgery: a 12-year nationwide cohort study. Medicine. (2015) 94:e2087. doi: 10.1097/MD.0000000000002087
274. Zhang Q, Chen Y, Li J, Chen D, Cheng Z, Xu S, et al. A meta-analysis of the effects of bariatric surgery on fracture risk. Obes Rev. (2018) 19:728–36. doi: 10.1111/obr.12665
275. Liu C, Wu D, Zhang J-F, Xu D, Xu W-F, Chen Y, et al. Changes in bone metabolism in morbidly obese patients after bariatric surgery: a meta-analysis. Obes Surg. (2016) 26:91–7. doi: 10.1007/s11695-015-1724-5
276. Gehrer S, Kern B, Peters T, Christoffel-Courtin C, Peterli R. Fewer nutrient deficiencies after laparoscopic sleeve gastrectomy (LSG) than after laparoscopic Roux-Y-gastric bypass (LRYGB)-a prospective study. Obes Surg. (2010) 20:447–53. doi: 10.1007/s11695-009-0068-4
277. Schafer AL, Weaver CM, Black DM, Wheeler AL, Chang H, Szefc GV, et al. Intestinal calcium absorption decreases dramatically after gastric bypass surgery despite optimization of vitamin D status. J Bone Miner Res. (2015) 30:1377–85. doi: 10.1002/jbmr.2467
278. Riedt CS, Brolin RE, Sherrell RM, Field MP, Shapses SA. True fractional calcium absorption is decreased after Roux-en-Y gastric bypass surgery. Obes Silver Spring Md. (2006) 14:1940–8. doi: 10.1038/oby.2006.226
279. Youssef Y, Richards WO, Sekhar N, Kaiser J, Spagnoli A, Abumrad N, et al. Risk of secondary hyperparathyroidism after laparoscopic gastric bypass surgery in obese women. Surg Endosc. (2007) 21:1393–6. doi: 10.1007/s00464-007-9228-6
280. Tardio V, Blais J-P, Julien A-S, Douville P, Lebel S, Biertho L, et al. Serum parathyroid hormone and 25-hydroxyvitamin D concentrations before and after biliopancreatic diversion. Obes Surg. (2018) 28:1886–94. doi: 10.1007/s11695-017-3101-z
281. Wei J-H, Lee W-J, Chong K, Lee Y-C, Chen S-C, Huang P-H, et al. High incidence of secondary hyperparathyroidism in bariatric patients: comparing different procedures. Obes Surg. (2018) 28:798–804. doi: 10.1007/s11695-017-2932-y
282. Guglielmi V, Bellia A, Gentileschi P, Lombardo M, D'Adamo M, Lauro D, et al. Parathyroid hormone in surgery-induced weight loss: no glucometabolic effects but potential adaptive response to skeletal loading. Endocrine. (2018) 59:288–95. doi: 10.1007/s12020-017-1477-0
283. Chakhtoura MT, Nakhoul NN, Shawwa K, Mantzoros C, El Hajj Fuleihan GA. Hypovitaminosis D in bariatric surgery: a systematic review of observational studies. Metabolism. (2016) 65:574–85. doi: 10.1016/j.metabol.2015.12.004
284. DiGiorgi M, Daud A, Inabnet WB, Schrope B, Urban-Skuro M, Restuccia N, et al. Markers of bone and calcium metabolism following gastric bypass and laparoscopic adjustable gastric banding. Obes Surg. (2008) 18:1144–8. doi: 10.1007/s11695-007-9408-4
285. Fish E, Beverstein G, Olson D, Reinhardt S, Garren M, Gould J. Vitamin D status of morbidly obese bariatric surgery patients. J Surg Res. (2010) 164:198–202. doi: 10.1016/j.jss.2010.06.029
286. Lanzarini E, Nogués X, Goday A, Benaiges D, de Ramón M, Villatoro M, et al. High-dose vitamin D supplementation is necessary after bariatric surgery: a prospective 2-year follow-up study. Obes Surg. (2015) 25:1633–8. doi: 10.1007/s11695-015-1572-3
287. Shetty S, Kapoor N, Bondu JD, Thomas N, Paul TV. Bone turnover markers: emerging tool in the management of osteoporosis. Indian J Endocrinol Metab. (2016) 20:846–52. doi: 10.4103/2230-8210.192914
288. Crawford MR, Pham N, Khan L, Bena JF, Schauer PR, Kashyap SR. Increased bone turnover in type 2 diabetes patients randomized to bariatric surgery versus medical therapy at 5 years. Endocr Pract. (2018) 24:256–64. doi: 10.4158/EP-2017-0072
289. Rao RS, Kini S. GIP and bariatric surgery. Obes Surg. (2011) 21:244–52. doi: 10.1007/s11695-010-0305-x
290. Bollag RJ, Zhong Q, Phillips P, Min L, Zhong L, Cameron R, et al. Osteoblast-derived cells express functional glucose-dependent insulinotropic peptide receptors. Endocrinology. (2000) 141:1228–35. doi: 10.1210/en.141.3.1228
291. Zhong Q, Itokawa T, Sridhar S, Ding K-H, Xie D, Kang B, et al. Effects of glucose-dependent insulinotropic peptide on osteoclast function. Am J Physiol Endocrinol Metab. (2007) 292:E543–8. doi: 10.1152/ajpendo.00364.2006
292. Maccarinelli G, Sibilia V, Torsello A, Raimondo F, Pitto M, Giustina A, et al. Ghrelin regulates proliferation and differentiation of osteoblastic cells. J Endocrinol. (2005) 184:249–56. doi: 10.1677/joe.1.05837
293. Wong IP, Baldock PA, Herzog H. Gastrointestinal peptides and bone health. Curr Opin Endocrinol Diabetes Obes. (2010) 17:44–50. doi: 10.1097/MED.0b013e3283344a05
294. Nuche-Berenguer B, Moreno P, Esbrit P, Dapía S, Caeiro JR, Cancelas J, et al. Effect of GLP-1 treatment on bone turnover in normal, type 2 diabetic, and insulin-resistant states. Calcif Tissue Int. (2009) 84:453–61. doi: 10.1007/s00223-009-9220-3
295. Wucher H, Ciangura C, Poitou C, Czernichow S. Effects of weight loss on bone status after bariatric surgery: association between adipokines and bone markers. Obes Surg. (2008) 18:58–65. doi: 10.1007/s11695-007-9258-0
Keywords: bariatric surgery, somatotropic axis, corticotropic axis, gonadal axis, bone metabolism
Citation: Cornejo-Pareja I, Clemente-Postigo M and Tinahones FJ (2019) Metabolic and Endocrine Consequences of Bariatric Surgery. Front. Endocrinol. 10:626. doi: 10.3389/fendo.2019.00626
Received: 29 November 2018; Accepted: 29 August 2019;
Published: 19 September 2019.
Edited by:
Jennifer L. Miles-Chan, University of Auckland, New ZealandReviewed by:
Caterina Conte, Vita-Salute San Raffaele University, ItalySarah M. Turpin-Nolan, Monash University, Australia
Copyright © 2019 Cornejo-Pareja, Clemente-Postigo and Tinahones. This is an open-access article distributed under the terms of the Creative Commons Attribution License (CC BY). The use, distribution or reproduction in other forums is permitted, provided the original author(s) and the copyright owner(s) are credited and that the original publication in this journal is cited, in accordance with accepted academic practice. No use, distribution or reproduction is permitted which does not comply with these terms.
*Correspondence: Mercedes Clemente-Postigo, mer.cp@hotmail.com; Francisco J. Tinahones, fjtinahones@uma.es