- 1Department of Immunology and Oncology, Centro Nacional de Biotecnología/CSIC, Madrid, Spain
- 2Macromolecular X-Ray Crystallography Unit, Centro Nacional de Biotecnología/CSIC, Madrid, Spain
- 3Department Cell Biology, Research Institute Hospital 12 de Octubre (i+12), Complutense University of Madrid, Madrid, Spain
Chemokine receptors are members of the G protein-coupled receptor superfamily. These receptors are intimately involved in cell movement, and thus play a critical role in several physiological and pathological situations that require the precise regulation of cell positioning. CXCR4 is one of the most studied chemokine receptors and is involved in many functions beyond leukocyte recruitment. During embryogenesis, it plays essential roles in vascular development, hematopoiesis, cardiogenesis, and nervous system organization. It has been also implicated in tumor progression and autoimmune diseases and, together with CD4, is one of the co-receptors used by the HIV-1 virus to infect immune cells. In contrast to other chemokine receptors that are characterized by ligand promiscuity, CXCR4 has a unique ligand—stromal cell-derived factor-1 (SDF1, CXCL12). However, this ligand also binds ACKR3, an atypical chemokine receptor that modulates CXCR4 functions and is overexpressed in multiple cancer types. The CXCL12/CXCR4/ACKR3 axis constitutes a potential therapeutic target for a wide variety of inflammatory diseases, not only by interfering with cell migration but also by modulating immune responses. Thus far, only one antagonist directed against the ligand-binding site of CXCR4, AMD3100, has demonstrated clinical relevance. Here, we review the role of this ligand and its receptors in different autoimmune diseases.
Introduction
The human chemokine family is defined by almost 50 low-molecular-weight proteins, originally described as pro-inflammatory cytokines (1). By associating with and signaling through seven transmembrane G protein-coupled receptors (GPCRs), they activate multiple signaling pathways in target cells. Chemokines are essential for the spatio-temporal organization of leukocytes (2), and thereby ensure the correct organization and function of the immune system. Not surprisingly, dysregulation of chemokine production often results in disease. The chemokine system is complex and, in some cases, even redundant, with several chemokines that bind the same receptor with similar affinities and receptors that can bind the same ligand. Moreover, cells can express simultaneously or during different stages of their life several receptors at the cell membrane (3–7). This apparent promiscuity is, nonetheless, contentious and raises questions about the relevance of one or multiple chemokines or chemokine receptors in a determined pathological or physiological process. For example, through binding to CCR7, CCL19, and CCL21 are implicated in lymph node homeostasis and regulate naïve T cell encounters with mature antigen-presenting cells (8, 9). It is known, however, that both ligands promote differential G protein-coupled receptor kinase (GRK) recruitment to CCR7 and, in consequence, differentially activate β-arrestins (10, 11). These chemokines display differential interaction—and docking—modes for CCR7 leading to stabilization of different receptor conformations and hereby preferential activation of distinct intracellular signaling pathways (12).
Despite the crucial role of chemokines in immune function, efforts, and resources invested in the development of specific and efficient drugs have yielded poor results. Indeed, only two drugs targeting chemokine receptors have been approved for clinical use so far, and they apply to very specific situations: HIV-1 infection in the case of the CCR5 antagonist maraviroc (13) and stem cell mobilization from the bone marrow (BM) in the case of the CXCR4 antagonist AMD3100 (plerixafor) (14).
New findings have demonstrated that the chemokine/chemokine receptor system is even more complex. For instance, chemokines are capable of forming dimers and oligomers (15–17), and can interact with synergy-inducing molecules (18) and with glycosaminoglycans (GAGs) (17, 19, 20). It is also known that chemokine receptors are not only monomeric entities, but also exist as dimers and higher-order oligomers (21) that also interact with other membrane proteins (21–24) and exhibit signaling crosstalk with other proteins (25, 26).
A good example of the complex biology of chemokine signaling is the ubiquitously expressed CXCL12, which signals by binding to two receptors, CXCR4 and ACKR3. The CXCL12/CXCR4/ACKR3 axis plays key roles in many physiological and pathological processes, including embryogenesis, wound healing processes, angiogenesis, in the development and metastasis of tumors and during HIV-1 infection. The available evidence indicates that this signaling axis is also essential in maintenance of homesostasis and for host defense, and participates in progression of inflammation. The present review discusses the role of the CXCL12/CXCR4/ACKR3 axis in inflammation, focusing on its involvement in several autoimmune diseases.
CXCL12, the Ligand
Initially known as stromal cell-derived factor-1α (SDF-1 α) or preB-cell growth-stimulating factor (PBSF), CXCL12 is probably the most studied member of the chemokine family (27, 28). It is a homeostatic chemokine produced in multiple tissues including lymph nodes (LNs), brain, liver, colon, kidney, testis, lung, pancreas, skin and placenta, and in different cell types including stromal cells, osteoblasts, fibroblasts, dendritic cells and monocytes, among others. Data from Cxcl12-deficient mice, corroborated by those obtained in mice lacking the gene for its receptor, CXCR4, reveal its essential role in physiology. Both mouse models are embryonic lethal with severe defects in hematopoiesis and in the nervous and cardiovascular systems (29–31). In adults, CXCR4/CXCL12 participates in the retention of hematopoietic stem cells in BM, in the traffic of T cell-precursors to the thymus, and in the clearance of neutrophils in the BM. In cooperation with CCR7 ligands, CXCL12 participates in T lymphocyte homing, it assists lymphocyte trafficking across the high endothelial venules into LNs and Peyer's patches (32). CXCL12 directs T central memory cell homing to LNs (33). Interestingly, a role for CXCL12 in determining the location of metastasis in different tumors has been reported (34), and several studies point to its potential as a biomarker (35) in hepatocellular carcinoma (36), bladder cancer (37) and glioma recurrence (38), and as a predictor of poor survival in ovarian cancer (39). CXCL12 also has prognosis potential in non-tumoral processes such as cirrhosis (40), diabetes (41), and cardiovascular diseases (42).
CXCL12 also interacts with GAGs through a cluster of basic residues—the BBXB motif (43). The interaction with GAGs is necessary for the in vivo activity of certain chemokines (17), contributing to the complexity of the system (19). It increases the local concentration of chemokines, presents the ligand to the receptors, and allows the formation of chemokine gradients (17). The existence of partial overlap between GAG and receptor binding sites on CXCL12 suggests that chemokine oligomerization may allow simultaneous binding (15). However, recent data suggest that binding to CXCR4 competes with CXCL12 dimerization, which argues against GAG-mediated presentation (20). Although CXCR4 was initially described as the unique receptor for CXCL12, CXCL12 also binds the atypical receptor ACKR3, also known as CXCR7 (44). This receptor does not activate G proteins, but interacts with β-arrestins (45), indicating that it is likely to be more than just a scavenger receptor for CXCL12.
CXCR4/ACKR3, the Receptors
CXCR4 Expression and Function
Originally known as leukocyte-derived seven-transmembrane domain receptor (LESTR) or Fusin, CXCR4 was first described as an orphan GPCR that facilitates HIV-1 fusion with target cells—hence the name “Fusin” (46). CXCL12 is the unique and specific chemokine for CXCR4 (47). Its binding promotes the activation of heterotrimeric Gαβγ proteins, and the subsequent activation of multiple signaling pathways controlling calcium mobilization, actin polymerization, cytoskeletal rearrangements, gene transcription, and receptor internalization (48–51), cell proliferation, cell survival, and even apoptosis (52–55).
CXCR4 is an homeostatic receptor that is widely expressed both in embryonic and in adult tissues (1). As previously indicated, data from Cxcr4-deficient mice correlate with those observed in Cxcl12-knockout mice (29–31, 56), showing defects in hematopoiesis and in nervous and cardiovascular development. The importance of the CXCR4/CXCL12 axis is reflected not only by the fact that both knockout mice are embryonic lethal, but also because the ligand and the receptor are highly conserved members of chemokine and chemokine receptor families during evolution (57).
CXCR4 is ubiquitously expressed in the hematopoietic system, although its expression level is quite variable among cell types. As with other chemokine receptors, CXCR4 is important in leukocyte trafficking and arrest in specific niches, both under homeostatic and pathological conditions, by recruiting cells to sites of inflammation. During antigen-presenting cell/T cell contact, CXCR4, among other chemokine receptors, is recruited to the pSMAC (peripheral SupraMolecular Activation Cluster) where it contributes to the integrin activation needed to generate a productive immunological synapse and proper T cell activation (25). CXCL12/CXCR4 are thus key elements for the adaptive and innate immune response and also for BM organization and maintenance (58). Indeed, CXCR4 and CXCL12 are largely responsible for hematopoietic stem cell migration (59), homing (60–63), and survival (64, 65) in BM.
CXCR4 is also expressed in several non-hematopoietic tissues, such as lung, liver, kidney, gastrointestinal tract, adrenal gland, ovary, and brain. The importance of CXCR4 in adult tissues has been demonstrated using conditional Cxcr4-knockout mice, which have served to assign an important role of CXCR4 in regulating central nervous system development (66), and vasculature development in the gastrointestinal tract (30) and the kidney (67).
CXCR4 and CCR5, are the primary co-receptors for HIV-1 entry into target cells. Indeed, HIV strains are classified as X4- or T-tropic and R5- or M-tropic depending on the chemokine receptor used for cell infection (68). In newly infected individuals HIV-1 entry occurs mainly through CCR5 and CD4, they are M-tropic R5 strains which predominate in the acute and asymptomatic phases of HIV infection. CD4+ T helper type 1 (Th1) cells, which express high levels of CCR5 (69, 70), are implicated in maintaining the asymptomatic status (71, 72). The viral use of CXCR4 emerges later in disease and correlates with the phase of immunological deficiency and AIDS progression (73, 74).
Inherited heterozygous autosomal dominant mutations in the CXCR4 gene cause WHIM syndrome (75, 76), a severe combined immunodeficiency disease characterized by susceptibility to human papilloma virus infection, which causes warts, condyloma acuminata and carcinomas. These patients can suffer neutropenia, B cell lymphopenia, hypogammaglobulinemia which is related to recurrent infections and BM myelokathexis characterized by myeloid hyperplasia and increased numbers of mature, senescence neutrophils in the bone marrow (75). The mutations in CXCR4 result in a stop codon that eliminates the last 10–19 amino acids at the C-terminus, or alter specific key residues for receptor phosphorylation in this domain. In all cases, mutations impair CXCR4 internalization (48, 77), sustaining its activity and enhancing G protein- and β-arrestin-dependent signaling.
While considered a homeostatic receptor, the expression of CXCR4 can be modulated by different pathological conditions. For example, CXCR4 is overexpressed by many tumor types, including breast (34), ovarian (78), prostate (79), melanoma (80), and neuroblastoma (81), among others. Also, the elevated expression of CXCR4 in metastatic lesions correlates with tumor progression and with preferential metastatic sites of the primary tumor (82–84). Studies in mice show that CXCR4 is a good target in cancer as its blockade impairs the spread of cancer cells and metastasis in several cancer models (34, 85, 86). The CXCL12/CXCR4 axis is also involved in tumor growth, tumor cell interactions with the microenvironment (87), vasculogenesis and angiogenesis (88). In this setting, hypoxia has been related to the upregulation of CXCR4 expression, suggesting that this receptor is involved in tumor progression (89, 90).
Inflammation has also been identified as a relevant factor for CXCR4 modulation, as TGF-β1 (91), VGEF (90), and bFGF (92) are reported to upregulate CXCR4 expression, whereas other cytokines such as IL-5 (93), IFNα and IFNγ (94) downregulate its expression. Overall, these data illustrate the involvement of the CXCR4/CXCL12 axis in the development and progression of immunodeficiency and inflammatory diseases and cancer, and underline its interest as a target for therapeutic intervention.
ACKR3 Expression and Function
ACKR3, also known as RDC1 and CXCR7, was first identified as an orphan GPCR, and was later described as a high-affinity receptor for CXCL12 and CXCL11 (44, 95). Instead of having the canonical DRYLAIV motif, which is involved in coupling to G proteins, it contains the sequence DRYLSIT (96) and is, accordingly, included in the group of “atypical chemokine receptors” (ACKR)—hence the name “ACKR3.” It acts as a scavenger receptor for CXCL12 (97, 98), but also triggers the β-arrestin pathway (45), and can be also implicated in modulating CXCR4 functions by forming heterodimers (99, 100).
In adults and under homeostatic conditions, ACKR3 is not expressed at the cell surface of human or mouse leukocytes in peripheral blood (101), but is expressed in B cells and dendritic cells in secondary lymphoid organs (102). ACKR3 is also expressed in several non-hematopoietic cells, for example, in endothelial and mesenchymal cells and in neurons. Reflecting its importance in embryonic tissues, Ackr3-knockout mice die perinatally and show severe defects in cardiovascular, kidney, and brain development, but not in hematopoiesis (100).
In the presence and absence of ligands, ACKR3 continuously recycles between the cell membrane and the endosomal compartment. Ligands trigger a moderate increase of ACKR3 internalization which concours with a marked uptake and degradation of CXCL11 and CXCL12, thus supporting its scavenging role (98). As also occurs for CXCR4, ACKR3 surface expression is increased in pathological situations, both in leukocytes and endothelial cells. In a prostate cancer model, CXCL8 increases the expression of mRNA and protein levels of ACKR3 (103); IL-1β triggers ACKR3 expression in HUVEC cells (104); and other external factors such as lipopolysaccharides associate with ACKR3 upregulation in the pulmonary epithelium modulating microvascular permeability during acute pulmonary inflammation (105). Although the mechanisms are not fully elucidated, in vitro and in vivo studies have described that higher levels of ACKR3 correlate with increased cell proliferation and invasive migration, that is with tumor growth and metastasis (106–108).
Similar to CXCR4, ACKR3 is implicated in some autoimmune diseases, such as rheumatoid arthritis (104), inflammatory bowel disease (109), and experimental autoimmune encephalomyelitis (EAE) (110, 111).
CXCR4/CXCL12 Structure
While the sequence identity among chemokines varies greatly (15–90%), there is a high level of conservation in their tertiary structures, as revealed by nuclear magnetic resonance and X-ray crystallography (16). The structure of CXCL12 was first described in 1997 using nuclear magnetic resonance spectroscopy (112). Its 67 residues present a typical chemokine folding with an eight residue-long N-terminus followed by a central body assembled by three antiparallel β-strands forming a β-sheet covered by an α-helix. CXCL12 presents a high percentage of basic residues dispersed along the N-terminus and the main body that are important for the binding to specific residues in the receptor. A two-step binding site model has been proposed (112, 113) for the interaction between CXCL12 and CXCR4, with a first interaction of the central body of the chemokine with the N-terminus of CXCR4 in the Chemokine Recognition Site 1 (CRS1), allowing the ligand to obtain an optimal orientation on the receptor, which enables the N-terminus to penetrate deep into the receptor and bind to the CRS2 region. This structure may shift between monomers and dimers depending on the solution conditions, a fact that might affect CXCL12 activity. In addition, a non-dissociating CXCL12 dimer that binds CXCR4, induces Ca2+ mobilization, but does not mediate in vitro cell migration has been described (114), suggesting specific functions associated with distinct ligand conformations.
The first high-resolution structure of CXCR4 was published in 2010 (115), with five independent receptor structures reported in complex with two antagonists, the small molecule IT1t and the cyclic peptide CVX15; thus, all the structures presented an inactive state conformation. As described for other GPCRs (116, 117), CXCR4 displays the canonical 7-helix bundle arrangement crossing the membrane, linked by three extracellular loops (ECL1–3) and three intracellular loops (ICL1–3). Also, it contains a long N-terminus composed of 34 residues and a C-terminus where the VIII typical helix was not clearly defined. A more recent structure of CXCR4 has been reported in complex with the viral chemokine vMIP-II (118), with very similar features to those described previously.
CXCL12 binding to CXCR4 promotes conformational changes in the transmembrane domains governed by a chain of “signaling” residues present along the transmembrane α-helices identified by mutagenesis studies [(51); Figure 1A]. CXCL12 binding to the CRS2 region facilitates the interaction of the first two N-terminal residues of the ligand, K and P, with a group of residues mainly present at this domain in the receptor to engage and trigger signaling [Figure 1A, blue and green residues; (112)]. Next, eight residues present in the transmembrane (TM) segments TMVI and TMVII (Figure 1A, orange residues), which form a continuous rod through the receptor, connect the signal initiation with the next residues (Figure 1A, red). This connection is critical for signaling since these residues are components of the microswitch allowing G protein coupling. Of note, residues F248 to V242 in TMVI are in contact with almost all the conserved motifs present in the GPCRs that are crucial for signaling, including motifs CWxP in TMVI, NPxxY in TMVII, DRY in TMIII, and Y(x)5KL in TMV. This assigns a role for these residues in controlling the transition between active and inactive states, by enabling helix and side chain translations, as described using mutational studies of this hydrophobic bridge in different receptors (120–122).
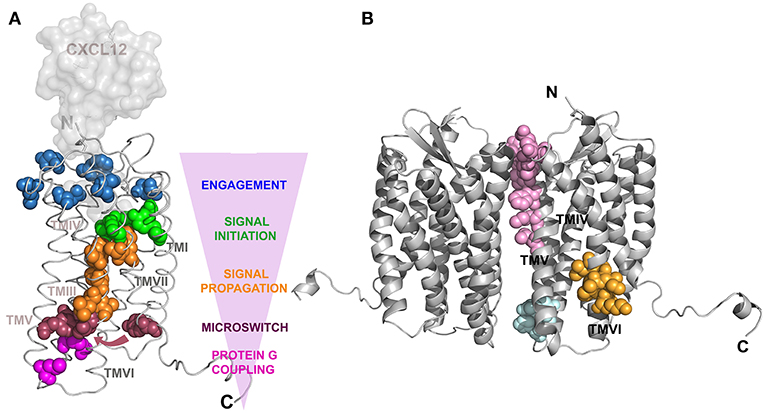
Figure 1. Structure of CXCR4. Models created using the Swiss-Model web server (119), with PDB 3oe8 as a reference for CXCR4 and PDB 1a15 for CXCL12, which was superposed to vMIP-II present in PDB 4 rws to build a CXCR4:CXCL12 complex. (A) Ribbon representation of a CXCR4 monomer in complex with a surface representation of CXCL12. Residues implicated in CXCL12 signal transmission are depicted as colored spheres corresponding to the specific state of signal transference showed by the pink arrowhead indicating the direction of the CXCR4:CXCL12 axis signal transit. Blue colored residues are part of the CXCL12 binding site that contact the green colored residues responsible for the initiation of the signal transmission toward the cytoplasm. Subsequent signal propagation through a hydrophobic bridge, in orange, allows conformational changes in TMVII inducing translation of specific residues (red spheres), depicted by an arrow, that will act like a microswitch and position the G-protein binding residues (pink) in an active conformation. [Adapted from Wescott et. al. (51)]. (B) Cartoon representation of the CXCR4 dimer. Residues contacting between monomers are shown by pink spheres for CXCR4:IT1t complex, cyan for CXCR4:CVX15 complex and orange for oligomerization inhibition after mutating residues 239–246 in TMVI (21).
The ability of GPCRs to homo- and heterodimerize has been described (123) and the crystallographic structures of CXCR4 highlight the regions implicated in these interactions (115, 118). The implicated residues are located mainly at the extracellular portion of helices V and VI in the case of the CXCR4:IT1t complex (Figure 1B, pink residues) and at the base of helices III and IV in the CXCR4:CVX15 complex (Figure 1B, cyan residues). These residues differ from those previously described in models of GPCR dimerization, where contacting residues were allocated in helices I and IV (124, 125). The functional implications of these differences for the CXCR4 life cycle remain unclear, but since there is a low sequence identity in the dimerization region among dimerizing GPCRs, it might be a feature specific to CXCR4. A novel region for allosteric regulation of CXCR4 oligomerization has also been described (21). Residues located at the N-terminus of TMVI (Figure 1B, orange residues) were mutated and the receptor remained able to dimerize but not to form large nanoclusters after ligand binding. This mutant receptor was able to trigger some calcium flux and to promote cell adhesion but could not trigger cell migration, suggesting that receptor nanoclustering is essential for complete CXCR4-mediated functions.
CXCL12-mediated Signaling Pathways
CXCR4 exists in the plasma membrane as a monomer, dimer, and higher-order oligomers. It can also interact with other chemokine receptors (i.e., ACKR3, CCR5, and CCR2) and other cell surface proteins such as the TCR, CD4, tetraspanins, and other GPCRs (21, 50). This wide variety of interactions predicts a high signaling potential and diversity for CXCR4.
Like most GPCRs, CXCR4 was initially believed to act as monomer. Resonance energy transfer techniques revealed that CXCR4, as with other chemokine receptors, forms homo- and heterodimers (21), even in the absence of ligand stimulation. Defining the contribution of different receptor conformations to functional response is a challenging endeavor. Recent studies using advanced microscopy techniques indicate that CXCR4 and also CCR7 form ligand-induced higher-order oligomers that are critical for receptor function (21, 126). Nevertheless, ligand-induced oligomerization is solely capable of mediating signaling, as blocking the formation of CXCR4 oligomers diminishes the functional response (21). Overall, these data indicate that CXCR4 exists in multiple conformations and highlight the importance of receptor oligomers for full receptor function. While binding experiments at equilibrium showed negative cooperativity between the subunits of a receptor dimer, supporting high-affinity binding of only a single chemokine molecule per receptor dimer (127), the stoichiometry of these receptor conformations with the ligands is still unclear.
As occurs with other GPCRs and their ligands, CXCL12 engagement with CXCR4 triggers conformational changes in the latter promoting the dissociation of GTP-loaded heterotrimeric G proteins (16); the GTP-loaded Gα subunit and the Gβγ heterodimer then activate downstream effectors. For CXCR4, as for chemokine receptors in general, signaling is abolished by pertussis toxin, indicating the involvement of Gαi in the signal cascade (128). However, chemokine receptors can be also coupled to Gαq or Gα11 in a cell- and stimulus-dependent manner (129–131).
Gαi inhibits adenylyl cyclase, promoting a reduction of cAMP and activating Src-related kinases, MAPK, PI3K, small GTPases and PLC-β (phospholipase C). In turn, PLC-β activation generates the second messengers diacylglycerol (DAG) and inositol 1,4,5-triphosphate (IP3), which are required for cell proliferation and migration. Some reports indicate that Gβγ also activates PLC-β (50, 132). Gαi also triggers biphasic PI3K activation, a pathway related to cell migration, proliferation, and survival (133). PI3K can also be activated directly by the Gβγ dimer (134), and PI3K catalytic subunits have a Ras-binding domain, which can be recognized by small GTPases (such as Ras). Both, the PI3K signaling cascade, which includes Akt and mTOR proteins, and the MAPK pathway, have important roles in actin reorganization and cell migration (53, 135, 136).
Other mechanisms activated by CXCR4/CXCL12 are G protein-independent, i.e., activation of GRK family proteins. It is known that GRK2, GRK3, and GRK6, and also PKC, are implicated in phosphorylation of serine/threonine residues in the CXCR4 C-terminus, which contributes to CXCR4 desensitization (137, 138). The phosphorylated residues of the CXCR4 C-tail trigger β-arrestin recruitment, thereby simultaneously promoting the uncoupling of G proteins (139), and resulting in β-arrestin-dependent receptor endocytosis.
Different regulatory mechanisms for CXCR4 have been also described (49). CXCL12 triggers a rapid ubiquitination and degradation of CXCR4 (140). The process requires a GRK6-mediated phosphorylation of several serine residues at the C terminal end of CXCR4 (141) and receptor internalization (142). Other regulatory mechanisms involve the ability of CXCR4 to associate with other receptors, and a role for ACKR3/CXCR4 heterodimers regulating CXCL12-mediated G protein signaling has been described (99). Indeed, in glioblastoma cells, ACKR3/CXCR4 crosstalk affects major signaling pathways related to cell survival, proliferation, and migration (143).
CXCR4 also interact with other chemokine receptors, with different functional consequences—as illustrated by its interaction with ACKR3. CXCR4 can form heterodimers with CCR5, and together with the TCR of CD4+ cells, modulates HIV infection (23, 144). Examples of other non-chemokine receptors known to interact with CXCR4 include the cannabinoid receptor CB2, with implications for tumor progression (145). Also, the delta opioid receptor forms heterodimers with CXCR4 and might modulate pathological processes (22).
CXCL12/CXCR4/ACKR3 in Autoimmune Diseases
Autoimmune diseases result from pathological immune responses against self-antigens. The clinical manifestations are initiated after tissue infiltration of immune cells, which trigger the uncontrolled attack. Because of its central role in the control of immune cell trafficking, the chemokines, produced by stromal cells, tissue cells, and activated cells of the innate immune system, are essential molecules in the development of autoimmune responses. Although CXCL12 was originally classified as a homeostatic chemokine, it also plays an important role during inflammation (see Table 1). Here, we review the involvement of the CXCL12/CXCR4/ACKR3 axis in some of the most prevalent autoimmune diseases: psoriasis (146), multiple sclerosis (161), rheumatoid arthritis (162), lupus (155), type I diabetes (T1D) (163), and inflammatory bowel disease (164, 165).
Psoriasis
Psoriasis is a chronic inflammatory disease affecting the skin. It affects 2 to 3% of the population worldwide (166). It is characterized by epidermal thickening linked to enhanced proliferation and aberrant terminal differentiation of epidermal keratinocytes. It also shows accumulation of inflammatory leukocytes—in particular dendritic cells, macrophages, and T cells (167)—and pronounced inflammatory angiogenesis, leading to vascular remodeling (168). Immune cell infiltration predicts an important role of chemokines in psoriasis as well as in other inflammatory skin diseases such as atopic dermatitis and mastocytosis. Indeed, comparative gene expression profiling showed significantly higher expression of CCL4, CCL20, CXCL8, and CXCL2 in skin lesions of patients with psoriasis as compared with atopic dermatitis, and the opposite was found for CCL13, CCL18, and CCL27, reflecting the distinct infiltrating cell types in the two conditions (169, 170). Especially relevant is the expression of CX3CL1 (fractalkine), whose receptor CX3CR1 has been identified as a psoriasis susceptibility gene (171), and is known to attract specific subtypes of T cells and immature mast cells to the inflamed skin (172).
The presence of angiogenesis in psoriatic lesions has led to a focus on chemokines with angiogenic properties, in particular CXCL12 (173), which is expressed in the skin (174) and is upregulated by the angiogenic factor VEGF-A (175). Elevated mRNA levels of both CXCL12 and CXCR4 have been found in lesions of psoriatic skin (176). In a model of imiquimod-induced skin inflammation in VEGF-A transgenic mice, blockade of CXCR4 inhibited skin inflammation, and was associated with reduced angiogenesis and inflammatory cell accumulation, including dermal CD4+ T cells, intra-epidermal CD8+ T cells and macrophages (146). Other reports have shown a beneficial role of the CXCL12/CXCR4 axis in psoriasis. For example, in a mouse model of IL-23-induced psoriasiform dermatitis, specific depletion of Cxcr4 in keratinocytes led to increased keratinocyte proliferation and enhanced the effects of proliferative Th17 cytokines (147). Psoriasis is nonetheless a human-specific disease and therefore most experimental mouse models do not fully summarize all the characteristics present in humans.
Multiple Sclerosis
Multiple sclerosis (MS) is the most common autoimmune disease affecting the central nervous system (CNS). It is characterized by chronic inflammation, demyelination, and neurodegeneration (177), triggering CNS dysfunction, visual disorders, and motor deficits. The prevalence of MS varies with country, but it is higher in Europe and North America (178). As in other autoimmune diseases, MS is a multifactorial disease caused by a combination of different factors, genetic predisposition, environment, viral infections that activate the immune system, and an uncontrolled autoimmune inflammation (179), but the pathogenesis is still not well-understood. Cell extravasation into the brain parenchyma is needed to provoke tissue damage; however, the existence of the blood brain barrier and the blood-cerebrospinal fluid (CSF) barrier makes the pathogenesis of CNS disease different from other inflammatory diseases (180). Immune cell activation occurs primarily in cervical lymph nodes (181), but autoimmune T cells require myeloid cell-mediated re-stimulation in the CNS to survive and trigger the disease (169, 182–184). The encounter between activated T cells and antigen-loaded myeloid cells along the abluminal surfaces of subpial vessels confers competence to T cells to invade the parenchyma and to mediate disease (184).
Chemokines, their receptors and adhesion molecules orchestrate leukocyte trafficking in the CNS (170). Ccr6-deficient mice did not develop experimental allergic encephalitis (EAE) although Ccr6-deficient T cells were efficiently primed in vivo, and their effector functions were unaffected (185). Studies have identified P-selectin, α4 integrins and CCR6 as the promoters of lymphocyte migration into the CSF (186). Accordingly, the ligand for CCR6, CCL20, is constitutively expressed by epithelial cells of the choroid plexus in mice and humans, indicating that T cells must cross the blood-CSF barrier to initiate EAE (181). However, a significant albeit minor fraction of cerebrospinal T cells in healthy humans express CCR6, suggesting that additional molecules are needed to support this trafficking (169).
CXCL12 is constitutively expressed in the adult CNS, and is rapidly up-regulated under some pathological situations such as HIV-1-associated dementia, brain tumor, or neuroinflammation (187–193). Due to the local hypoxia, during ischemia, the transcription factor hypoxia-inducible factor-1 (HIF-1), which regulates CXCL12 gene expression in endothelial cells is activated (194). CXCL12 is detected in the CSF of patients with MS (161, 195, 196), and its levels are high on astrocytes in active lesions, throughout the lesion areas as well as on some monocytes/macrophages within vessels (196, 197). During the induction of EAE, CXCR4 blockade promotes loss of the typical intense perivascular cuffs, which is then replaced with mononuclear cell infiltration of white matter (198). This observation concours with the increased levels of IFNγ and other cytokines associated with monocyte and microglial activation, demyelination, and with stronger clinical severity of the disease (199). The available evidence thus indicates that CXCL12 restricts the intraparenchymal migration of mononuclear cells during autoimmune disease at the CNS. A molecule derived from CXCL12, P2G-CXCL12, diminished the progression of EAE (200). A similar effect was obtained using another variant of the N-terminal domain of CXCL12, obtained as a result of phage display, LGGG-CXCL12 (201).
In EAE, ACKR3 expression increases at the infiltration sites on endothelial barriers, indicating that its scavenger role on CXCL12 is essential for leukocyte entry into the CNS parenchyma (111). A specific antagonist of ACKR3, CCX771, prevents immune cell infiltration into the CNS improving the clinical signs of EAE and preserves axonal integrity (202). ACKR3 has also been implicated in migration of activated microglia cells, an effect linked to amelioration of clinical severity (203).
Rheumatoid Arthritis
Rheumatoid arthritis (RA) is one of the most common autoimmune disorders and affects 1% of the population (204). It is characterized by persistent inflammation driven by proliferating fibroblasts in the synovial tissue, as well as T and B cell, neutrophil, and monocyte trafficking into the joint (205, 206). Cells invading RA pannus lesions express proinflammatory cytokines, chemokines, and matrix metalloproteinases that contribute to progressive cartilage and bone destruction (207). The RA pannus also shows strong neovascularization (208), particularly in the early stage of the disease (209), which might facilitate cell extravasation into the synovium. The etiopathology of RA has been difficult to define; however, as in other autoimmune processes, genetic predisposition, environmental factors, and an uncontrolled immune response are clearly implicated (206).
Chronically inflamed non-lymphoid tissues can host highly organized aggregates of ectopic lymphoid structures. These structures usually appear during the course of persistent infections, transplant rejection, cancer and autoimmune diseases such RA (210). The process occurs in response to mediators of inflammation such as chemokines, cytokines, and bioactive lipids produced by tissue-resident cells, and is able to regulate the recruitment and organization of lymphocytes.
Synovial fibroblasts and monocytes/macrophages are the main source of the inflammatory chemokines expressed in the inflamed RA joints (211, 212), of which CXCL8, CXCL5, CXCL10, CCL5, and CCL20 are primarily involved in the selective recruitment of immune cells. Especially significant to this process is CXCL8, which is released by osteoclasts in response to anti-citrullinated peptides antibodies, and attracts neutrophils, leading to bone erosion and pain (213). By acting in an autocrine or paracrine manner, the chemokines also activate fibroblasts in the RA synovium (214).
Synovial fibroblasts and endothelial cells also express CXCL12 (149), and the local presence of IL-15 and TGFβ enhances CXCR4 expression by immune cells (91). These observations support the hypothesis that the CXCL12/CXCR4 axis retains activated immune cells in the inflamed joint, thus contributing to the chronic inflammation. Indeed, CXCL12 expression correlates with bone erosion (215). CXCL12 attracts osteoclast precursors and stimulates their differentiation and bone-resorbing activity, upregulates the production of some metalloproteases, and confers resistance to apoptosis in osteoclasts (153, 216). In addition, in vitro experiments demonstrate that CXCL12 promotes chondrocytes necrosis, suggesting a role of this chemokine in cartilage damage.
CXCL12 has also been implicated in synovial neovascularization (91). As in tumors, the presence of hypoxia in inflamed joints activates HIF-1, inducing VEGF and CXCR4 expression (90). In addition, ACKR3 has been associated with angiogenic processes in RA synovium (104), although its role in the pathogenesis of RA has been less studied.
Systemic Lupus Erythematosus
Systemic lupus erythematosus (SLE) is a multisystem autoimmune disease characterized by circulating autoantibody-autoantigen complexes and infiltration of different types of leukocytes, promoting inflammation, and organ damage (217). Lupus nephritis is a major cause of morbidity and mortality in up to 60% of patients with SLE and is characterized by kidney inflammation (218). While the precise etiological mechanisms of SLE are unknown, genetic, hormonal, and environmental factors, as well as immune abnormalities, have been identified (219). Studies in animal models, also corroborated in humans point to essential roles of chemokines, including CXCL13, CXCL12, CXCL9, CXCL10, among others (220).
In several lupus models, CXCR4 expression is increased in B cells, plasma cells, T cells, neutrophils, and monocytes (221), which concurs with the increased CXCL12 expression in the kidney (222). CXCL12 also affects renal tissue cells. In glomerulonephritis, CXCR4 is overexpressed in parietal epithelial cells of the kidney, triggering their migration into the glomerular tuft where they form hyperplastic lesions (156). Administration of anti-CXCL12 neutralizing antibodies in NZB/WF1 mice increases their survival rate, and reduces IgG deposition and renal inflammation (222). In patients with lupus nephritis, CXCL12 expression is increased in the tubules and glomeruli of kidneys (223), and an accumulation of B cells is found in renal biopsies (224). In accordance, CXCR4 overexpression on B cells from these patients positively correlates with disease activity and kidney involvement (225).
Type I Diabetes
Type I diabetes (T1D) is an autoimmune disease caused by the selective destruction of insulin-producing pancreatic β-cells following infiltration of the islets of Langerhans by immune cells (insulitis) (226). This is accompanied by the progressive loss of glucose homeostasis that induces the classical early symptoms of the disease, polydipsia, polyphagia, and polyuria (211). As in other autoimmune diseases, genetic predisposition, environmental factors, and acute and/or chronic inflammation caused by acute and/or persistent pathogen infection are known factors in the development of T1D (212). Cardiovascular events, including myocardial infarction, stroke, angina, but also microvascular complications such as retinopathy, nephropathy, and neuropathy, all associate with T1D, and cardiovascular disease is the primary cause of death in patients (212).
Multiple chemokines have been associated with T1D and/or its complications. In particular, CXCL10 was identified as the central chemokine expressed in the islet environment of prediabetic animals and in patients with T1D (227), whereas CCL5, CCL8, CXCL9, and CX3CL1 are also present but at low levels (228). The role of chemokines is believed to be related to immune cell infiltration into the β-islets, which requires the coordinated action of many proteins involved in cell movement.
Blockade of CXCL12 inhibits insulitis and diabetes development (157, 163). It was proposed that the retention of Treg cells in the BM by CXCL12 alters the balance of T cell subpopulations in favor of autoreactive T cells. However, an opposite effect of CXCL12 inhibition was also reported, showing that a population of T cells attracted by CXCL12 protects recipient mice from the capacity of diabetogenic T cells to transfer diabetes (229). CXCL12 is also chemorepulsive on diabetogenic T cells, while mediating firm adhesion of normal T cells (158) and the retention of Treg cells (230). Pancreatic lymph nodes of NOD mice have reduced levels of Treg cells and this associates with decreased expression of CXCL12, whereas the recovery of normal levels of glucose in blood associates with the restoration of the Treg population in peripheral LNs (231). In addition, CXCL12/CXCR4 signaling is important for β-cell differentiation and the genesis of pancreatic islet (54). It also exerts anti-apoptotic and anti-necrotic effects on β-cells to protect from diabetogenic agents (159).
The function of CXCL12 as a proangiogenic factor together with its ability to recruit endothelial progenitor cells accelerates wound healing in diabetes (232, 233). But the same effects can promote the progression of retinopathy associated with T1D. Tissue ischemia and reduced blood flow stimulate aberrant neovascularization and normal retinal architecture damage, causing impaired vision. CXCL12 expression is controlled by HIF-1 (194), and its levels increase as diabetic retinopathy progresses, contributing to angiogenesis and recruitment of endothelial progenitor cells to the site of vascular injury (160). Similarly, during acute renal failure, CXCL12 expression in the kidney increases triggering progenitor cells homing to the injured kidney (234), a process that might also occur during the diabetic nephropathy.
Inflammatory Bowel Disease
Idiopathic Inflammatory Bowel Diseases (IBDs) comprise two chronic intestinal diseases, ulcerative colitis and Crohn's disease, triggered by a dysregulated immune system response to environmental in genetically susceptible individuals (235, 236). Evidence suggests that the disease is a consequence of the inappropriate control of the immune response against enteric microbiota, which promotes the influx of inflammatory cells directed by chemokines (237–239).
Both CXCL12 and CXCR4 are expressed by intestinal epithelial cells (240, 241) and CXCR4 is also expressed in cells of the lamina propria (242, 243). Both are upregulated in IBD patients (240, 243) and participate in T cell-recruitment, mainly of memory Th1 cells (109). Some polymorphisms of the CXCL12/CXCR4 axis have associated with IBD progression and disease severity (244). Administration of a CXCR4 antagonist ameliorated colonic inflammation in a dextran sulfate sodium-induced model of colitis. The same study showed that the CXCR4 antagonist decreased TNFα and IFNγ production by mesenteric lymph node cells, whereas IL-10 production was unaffected. The authors also indicated that these antagonists do not affect the percentage of mesenteric Foxp3+CD25+ T cells (164). Later, using the same murine model of colitis, it was demonstrated that AMD3100 also enhanced epithelial barrier integrity (245). A recent report has suggested a role of CXCR4 in mesenchymal stem cells (MSCs) homing to the inflamed intestinal tissues (246). MSCs play an important role in tissue repair and regeneration but also have immunoregulatory properties that might contribute to reduce inflammation (247). ACKR3 is also expressed in peripheral T cells as well as in those of the lamina propria, but only peripheral T cells show ACKR3 upregulation during IBD (248). Regarding the ability of ACKR3 to form heterodimers with CXCR4 and to modulate its function, it is possible that ACKR3 upregulation allows a rapid increase of the influx of T cells to inflamed mucosa. Nonetheless, a direct role of ACKR3 in modulating CXCL12 levels (249) or even in modulating cell survival should not be discarded (250), although this observation needs be confirmed.
Summary/Perspectives
CXCL12 is a ubiquitous chemokine whose expression is regulated by circadian signals (251) and inflammatory mediators. Based on its essential role in embryogenesis and in homeostasis, it has been traditionally classified as a homeostatic chemokine. CXCL12 and CXCR4 are essential during hematopoiesis, as they play an important role in hematopoietic stem cell retention in BM and in facilitating B cell differentiation. Plerixafor, a CXCR4 antagonist, is currently in use to mobilize hematopoietic stem and progenitor cells for autologous transplantation, and to increase the efficacy of chemotherapeutic agents in several hematological malignancies. CXCR4 and ACKR3 are also important for tumor growth and dissemination. Accordingly, CXCR4 antagonists, which impair tumor dissemination, are in clinical trials to evaluate their use in combined therapies with anti-cancer treatments. Many inflammatory and autoimmune diseases show increased expression of CXCL12, suggesting that it could also be considered an inflammatory chemokine or at least forms part of a mechanism to maintain the homeostatic balance in diseased tissues. Indeed, administration of CXCL12 antagonists delays inflammatory disease onset and/or retards disease progression.
CXCL12 and its cognate receptors are a paradigm of cell-to-cell signaling; however, their biology is more complex than originally anticipated. CXCL12 can associate with other chemokines, as well as with other inflammatory mediators that modulate its functionality, and is also a target for post-transductional modifications such as truncation, nitration or citrullination processes that alter its responses. Also, chemokine receptors adopt different conformations at the cell membrane: CXCR4 is present at the cell surface as monomers, dimers (both homo- and heterodimers), and oligomers. These conformations increase cell plasticity, and shape the responses to the biological environment; for example, by forming heterodimers, ACKR3 modulates CXCR4-mediated functions, thereby contributing to the functional plasticity of CXCL12.
Overall, the data outlined in this review reflect the relevance of CXCL12 in homeostasis as well as in several diseases such as cancer or AIDS where its role is well-known, but also highlight its participation in autoimmune diseases. There, CXCL12 shows several functions, mediates cell infiltration and retention in specific tissues, triggers angiogenesis and even, in some cases, promotes cell proliferation. These effects are sometimes essential for the development of the disease or for increasing its severity and therefore justify the search of new antagonists. Compounds that abolish CXCL12 binding or partially affect some of its functions without altering others, should be incorporated in the clinical armamentarium to treat these prevalent diseases.
Author Contributions
EG-C, CS, JV-D, YJ, JR-F, and MM wrote the manuscript. CS and JV-D performed the figures. MM completed the table and coordinated the manuscript. EG-C and JR-F coordinated the bibliography and the format of the manuscript.
Funding
We acknowledge support of the publication fee by the CSIC Open Access Publication Support Initiative through its Unit of Information Resources for Research (URICI). This work was supported in part by grants from the Spanish Ministry of Science, Innovation and Universities (SAF2017-82940-R), and the RETICS Program of ISCIII (RD12/0012/0006 and RD12/0012/0008; RIER).
Conflict of Interest Statement
The authors declare that the research was conducted in the absence of any commercial or financial relationships that could be construed as a potential conflict of interest.
Acknowledgments
We thank the people at the Chemokine Signaling Group for much of the work that contributed to this review, especially Pilar Lucas, Pablo Martínez, Sofía Gardeta, and Ricardo Villares, Ph.D.
References
1. Zlotnik A, Yoshie O. The chemokine superfamily revisited. Immunity. (2012) 36:705–16. doi: 10.1016/J.IMMUNI.2012.05.008
2. Barreiro O, Martin P, Gonzalez-Amaro R, Sanchez-Madrid F. Molecular cues guiding inflammatory responses. Cardiovasc Res. (2010) 86:174–82. doi: 10.1093/cvr/cvq001
3. Nagase H, Kudo K, Izumi S, Ohta K, Kobayashi N, Yamaguchi M, et al. Chemokine receptor expression profile of eosinophils at inflamed tissue sites: decreased CCR3 and increased CXCR4 expression by lung eosinophils. J Allergy Clin Immunol. (2001) 108:563–9. doi: 10.1067/mai.2001.118292
4. Sand KE, Rye KP, Mannsåker B, Bruserud Ø, Kittang AO. Expression patterns of chemokine receptors on circulating T cells from myelodysplastic syndrome patients. Oncoimmunology. (2013) 2:e23138. doi: 10.4161/onci.23138
5. Langenkamp A, Nagata K, Murphy K, Wu L, Lanzavecchia A, Sallusto F. Kinetics and expression patterns of chemokine receptors in human CD4+ T lymphocytes primed by myeloid or plasmacytoid dendritic cells. Eur J Immunol. (2003) 33:474–82. doi: 10.1002/immu.200310023
6. Yamamoto J, Adachi Y, Onoue Y, Adachi YS, Okabe Y, Itazawa T, et al. Differential expression of the chemokine receptors by the Th1- and Th2-type effector populations within circulating CD4+ T cells. J Leukoc Biol. (2000) 68:568–74. doi: 10.1189/jlb.68.4.568
7. Cravens PD, Hayashida K, Davis LS, Nanki T, Lipsky PE. Human peripheral blood dendritic cells and monocyte subsets display similar chemokine receptor expression profiles with differential migratory responses. Scand J Immunol. (2007) 65:514–24. doi: 10.1111/j.1365-3083.2007.01933.x
8. Ohl L, Mohaupt M, Czeloth N, Hintzen G, Kiafard Z, Zwirner J, et al. CCR7 governs skin dendritic cell migration under inflammatory and steady-state conditions. Immunity. (2004) 21:279–88. doi: 10.1016/j.immuni.2004.06.014
9. Moschovakis GL, Förster R. Multifaceted activities of CCR7 regulate T-cell homeostasis in health and disease. Eur J Immunol. (2012) 42:1949–55. doi: 10.1002/eji.201242614
10. Byers MA, Calloway PA, Shannon L, Cunningham HD, Smith S, Li F, et al. Arrestin 3 mediates endocytosis of CCR7 following ligation of CCL19 but Not CCL21. J Immunol. (2008) 181:4723–32. doi: 10.4049/jimmunol.181.7.4723
11. Kohout TA, Nicholas SL, Perry SJ, Reinhart G, Junger S, Struthers RS. Differential desensitization, receptor phosphorylation, beta-arrestin recruitment, and ERK1/2 activation by the two endogenous ligands for the CC chemokine receptor 7. J Biol Chem. (2004) 279:23214–22. doi: 10.1074/jbc.M402125200
12. Hauser MA, Kindinger I, Laufer JM, Späte A-K, Bucher D, Vanes SL, et al. Distinct CCR7 glycosylation pattern shapes receptor signaling and endocytosis to modulate chemotactic responses. J Leukoc Biol. (2016) 99:993–1007. doi: 10.1189/jlb.2VMA0915-432RR
13. Gilliam BL, Riedel DJ, Redfield RR. Clinical use of CCR5 inhibitors in HIV and beyond. J Transl Med. (2010) 9:S9. doi: 10.1186/1479-5876-9-S1-S9
14. Debnath B, Xu S, Grande F, Garofalo A, Neamati N. Small molecule inhibitors of CXCR4. Theranostics. (2013) 3:47–75. doi: 10.7150/thno.5376
15. Salanga CL, Handel TM. Chemokine oligomerization and interactions with receptors and glycosaminoglycans: the role of structural dynamics in function. Exp Cell Res. (2011) 317:590–601. doi: 10.1016/j.yexcr.2011.01.004
16. Allen SJ, Crown SE, Handel TM. Chemokine: receptor structure, interactions, and antagonism. Annu Rev Immunol. (2007) 25:787–820. doi: 10.1146/annurev.immunol.24.021605.090529
17. Proudfoot AEI, Handel TM, Johnson Z, Lau EK, LiWang P, Clark-Lewis I, et al. Glycosaminoglycan binding and oligomerization are essential for the in vivo activity of certain chemokines. Proc Natl Acad Sci USA. (2003) 100:1885–90. doi: 10.1073/pnas.0334864100
18. Cecchinato V, D'Agostino G, Raeli L, Uguccioni M. Chemokine interaction with synergy-inducing molecules: fine tuning modulation of cell trafficking. J Leukoc Biol. (2016) 99:851–5. doi: 10.1189/jlb.1MR1015-457R
19. Proudfoot A, Johnson Z, Bonvin P, Handel T. Glycosaminoglycan interactions with chemokines add complexity to a complex system. Pharmaceuticals. (2017) 10:70. doi: 10.3390/ph10030070
20. Graham GJ, Handel TM, Proudfoot AEI. Leukocyte adhesion: reconceptualizing chemokine presentation by glycosaminoglycans. Trends Immunol. (2019) 40:472–81. doi: 10.1016/j.it.2019.03.009
21. Martínez-Muñoz L, Rodríguez-Frade JM, Barroso R, Sorzano CÓS, Torreño-Pina JA, Santiago CA, et al. Separating actin-dependent chemokine receptor nanoclustering from dimerization indicates a role for clustering in CXCR4 signaling and function. Mol Cell. (2018) 70:106–19.e10. doi: 10.1016/J.MOLCEL.2018.02.034
22. Pello OM, Martínez-Muñoz L, Parrillas V, Serrano A, Rodríguez-Frade JM, Toro MJ, et al. Ligand stabilization of CXCR4/δ-opioid receptor heterodimers reveals a mechanism for immune response regulation. Eur J Immunol. (2008) 38:537–49. doi: 10.1002/eji.200737630
23. Martinez-Munoz L, Barroso R, Dyrhaug SY, Navarro G, Lucas P, Soriano SF, et al. CCR5/CD4/CXCR4 oligomerization prevents HIV-1 gp120IIIB binding to the cell surface. Proc Natl Acad Sci USA. (2014) 111:E1960–9. doi: 10.1073/pnas.1322887111
24. Barroso R, Muñoz LM, Barrondo S, Vega B, Holgado BL, Lucas P, et al. EBI2 regulates CXCL13-mediated responses by heterodimerization with CXCR5. FASEB J. (2012) 26:4841–54. doi: 10.1096/fj.12-208876
25. Cascio G, Martín-Cófreces NB, Rodríguez-Frade JM, López-Cotarelo P, Criado G, Pablos JL, et al. CXCL12 Regulates through JAK1 and JAK2 formation of productive immunological synapses. J Immunol. (2015) 194:5509–19. doi: 10.4049/jimmunol.1402419
26. Soriano SF, Serrano A, Hernanz-Falcón P, de Ana AM, Monterrubio M, Martínez-A C, et al. Chemokines integrate JAK/STAT and G-protein pathways during chemotaxis and calcium flux responses. Eur J Immunol. (2003) 33:1328–33. doi: 10.1002/eji.200323897
27. Nagasawa T, Kikutani H, Kishimoto T. Molecular cloning and structure of a pre-B-cell growth-stimulating factor. Proc Natl Acad Sci USA. (1994) 91:2305–9.
28. Bleul CC, Fuhlbrigge RC, Casasnovas JM, Aiuti A, Springer TA. A highly efficacious lymphocyte chemoattractant, stromal cell-derived factor 1 (SDF-1). J Exp Med. (1996) 184:1101–9.
29. Nagasawa T, Hirota S, Tachibana K, Takakura N, Nishikawa S, Kitamura Y, et al. Defects of B-cell lymphopoiesis and bone-marrow myelopoiesis in mice lacking the CXC chemokine PBSF/SDF-1. Nature. (1996) 382:635–8. doi: 10.1038/382635a0
30. Tachibana K, Hirota S, Iizasa H, Yoshida H, Kawabata K, Kataoka Y, et al. The chemokine receptor CXCR4 is essential for vascularization of the gastrointestinal tract. Nature. (1998) 393:591–4. doi: 10.1038/31261
31. Zou Y-R, Kottmann AH, Kuroda M, Taniuchi I, Littman DR. Function of the chemokine receptor CXCR4 in haematopoiesis and in cerebellar development. Nature. (1998) 393:595–9. doi: 10.1038/31269
32. Bai Z, Hayasaka H, Kobayashi M, Li W, Guo Z, Jang MH, et al. CXC chemokine ligand 12 promotes CCR7-dependent naive T cell trafficking to lymph nodes and Peyer's patches. J Immunol. (2009) 182:1287–95. doi: 10.4049/jimmunol.182.3.1287
33. Scimone ML, Felbinger TW, Mazo IB, Stein JV, Von Andrian UH, Weninger W. CXCL12 mediates CCR7-independent homing of central memory cells, but not naive T cells, in peripheral lymph nodes. J Exp Med. (2004) 199:1113–20. doi: 10.1084/jem.20031645
34. Müller A, Homey B, Soto H, Ge N, Catron D, Buchanan ME, et al. Involvement of chemokine receptors in breast cancer metastasis. Nature. (2001) 410:50–6. doi: 10.1038/35065016
35. Samarendra H, Jones K, Petrinic T, Silva MA, Reddy S, Soonawalla Z, et al. A meta-analysis of CXCL12 expression for cancer prognosis. Br J Cancer. (2017) 117:124–35. doi: 10.1038/bjc.2017.134
36. Semaan A, Dietrich D, Bergheim D, Dietrich J, Kalff JC, Branchi V, et al. CXCL12 expression and PD-L1 expression serve as prognostic biomarkers in HCC and are induced by hypoxia. Virchows Arch. (2017) 470:185–96. doi: 10.1007/s00428-016-2051-5
37. Yang DL, Xin MM, Wang JS, Xu HY, Huo Q, Tang ZR, et al. Chemokine receptor CXCR4 and its ligand CXCL12 expressions and clinical significance in bladder cancer. Genet Mol Res. (2015) 14:17699–707. doi: 10.4238/2015.December.21.43
38. Tang W, Wang X, Chen Y, Zhang J, Chen Y, Lin Z. CXCL12 and CXCR4 as predictive biomarkers of glioma recurrence pattern after total resection. Pathol Biol. (2015) 63:190–8. doi: 10.1016/j.patbio.2015.07.002
39. Popple A, Durrant LG, Spendlove I, Rolland P, Scott IV, Deen S, et al. The chemokine, CXCL12, is an independent predictor of poor survival in ovarian cancer. Br J Cancer. (2012) 106:1306–13. doi: 10.1038/bjc.2012.49
40. Chalin A, Lefevre B, Devisme C, Barget N, Amiot L, Samson M. Circulating levels of CXCL11 and CXCL12 are biomarkers of cirrhosis in patients with chronic hepatitis C infection. Cytokine. (2019) 117:72–8. doi: 10.1016/j.cyto.2019.02.006
41. Karimabad MN, Hassanshahi G. Significance of CXCL12 in type 2 diabetes mellitus and its associated complications. Inflammation. (2015) 38:710–7. doi: 10.1007/s10753-014-9981-3
42. Subramanian S, Liu C, Aviv A, Ho JE, Courchesne P, Muntendam P, et al. Stromal cell-derived factor 1 as a biomarker of heart failure and mortality risk. Arterioscler Thromb Vasc Biol. (2014) 34:2100–5. doi: 10.1161/ATVBAHA.114.303579
43. Amara A, Lorthioir O, Valenzuela A, Magerus A, Thelen M, Montes M, et al. Stromal cell-derived factor-1alpha associates with heparan sulfates through the first beta-strand of the chemokine. J Biol Chem. (1999) 274:23916–25.
44. Burns JM, Summers BC, Wang Y, Melikian A, Berahovich R, Miao Z, et al. A novel chemokine receptor for SDF-1 and I-TAC involved in cell survival, cell adhesion, and tumor development. J Exp Med. (2006) 203:2201–13. doi: 10.1084/jem.20052144
45. Rajagopal S, Kim J, Ahn S, Craig S, Lam CM, Gerard NP, et al. Beta-arrestin- but not G protein-mediated signaling by the “decoy” receptor CXCR7. Proc Natl Acad Sci USA. (2010) 107:628–32. doi: 10.1073/pnas.0912852107
46. Feng Y, Broder CC, Kennedy PE, Berger EA. HIV-1 entry cofactor: functional cDNA cloning of a seven-transmembrane, G protein-coupled receptor. Science. (1996) 272:872–7.
47. Oberlin E, Amara A, Bachelerie F, Bessia C, Virelizier J-L, Arenzana-Seisdedos F, et al. The CXC chemokine SDF-1 is the ligand for LESTR/fusin and prevents infection by T-cell-line-adapted HIV-1. Nature. (1996) 382:833–5. doi: 10.1038/382833a0
48. Haribabu B, Richardson RM, Fisher I, Sozzani S, Peiper SC, Horuk R, et al. Regulation of human chemokine receptors CXCR4. Role of phosphorylation in desensitization and internalization. J Biol Chem. (1997) 272:28726–31.
49. Busillo JM, Benovic JL. Regulation of CXCR4 signaling. Biochim Biophys Acta Biomembr. (2007) 1768:952–63. doi: 10.1016/j.bbamem.2006.11.002
50. Pozzobon T, Goldoni G, Viola A, Molon B. CXCR4 signaling in health and disease. Immunol Lett. (2016) 177:6–15. doi: 10.1016/J.IMLET.2016.06.006
51. Wescott MP, Kufareva I, Paes C, Goodman JR, Thaker Y, Puffer BA, et al. Signal transmission through the CXC chemokine receptor 4 (CXCR4) transmembrane helices. Proc Natl Acad Sci USA. (2016) 113:9928–33. doi: 10.1073/pnas.1601278113
52. Missé D, Cerutti M, Noraz N, Jourdan P, Favero J, Devauchelle G, et al. A CD4-independent interaction of human immunodeficiency virus-1 gp120 with CXCR4 induces their cointernalization, cell signaling, and T-cell chemotaxis. Blood. (1999) 93:2454–62.
53. Yin X, Liu Z, Zhu P, Wang Y, Ren Q, Chen H, et al. CXCL12/CXCR4 promotes proliferation, migration, and invasion of adamantinomatous craniopharyngiomas via PI3K/AKT signal pathway. J Cell Biochem. (2018) 120:9724–36. doi: 10.1002/jcb.28253
54. Kayali AG, Lopez AD, Hao E, Hinton A, Hayek A, King CC. The SDF-1α/CXCR4 axis is required for proliferation and maturation of human fetal pancreatic endocrine progenitor cells. PLoS ONE. (2012) 7:e38721. doi: 10.1371/journal.pone.0038721
55. Jacobson O, Weiss ID. CXCR4 chemokine receptor overview: biology, pathology and applications in imaging and therapy. Theranostics. (2013) 3:1–2. doi: 10.7150/thno.5760
56. Ma Q, Jones D, Borghesani PR, Segal RA, Nagasawa T, Kishimoto T, et al. Impaired B-lymphopoiesis, myelopoiesis, and derailed cerebellar neuron migration in CXCR4- and SDF-1-deficient mice. Proc Natl Acad Sci USA. (1998) 95:9448–53. doi: 10.1073/PNAS.95.16.9448
57. Nomiyama H, Osada N, Yoshie O. A family tree of vertebrate chemokine receptors for a unified nomenclature. Dev Comp Immunol. (2011) 35:705–15. doi: 10.1016/J.DCI.2011.01.019
58. Nie Y, Han Y-C, Zou Y-R. CXCR4 is required for the quiescence of primitive hematopoietic cells. J Exp Med. (2008) 205:777–83. doi: 10.1084/jem.20072513
59. Wright DE, Bowman EP, Wagers AJ, Butcher EC, Weissman IL. Hematopoietic stem cells are uniquely selective in their migratory response to chemokines. J Exp Med. (2002) 195:1145–54. doi: 10.1084/JEM.20011284
60. Lapidot T, Kollet O. The essential roles of the chemokine SDF-1 and its receptor CXCR4 in human stem cell homing and repopulation of transplanted immune-deficient NOD/SCID and NOD/SCID/B2mnull mice. Leukemia. (2002) 16:1992–2003. doi: 10.1038/sj.leu.2402684
61. Lapidot T, Pflumio F, Doedens M, Murdoch B, Williams D, Dick J, et al. Cytokine stimulation of multilineage hematopoiesis from immature human cells engrafted in SCID mice. Science. (1992) 255:1137–41. doi: 10.1126/science.1372131
62. Christopherson KW, Hangoc G, Mantel CR, Broxmeyer HE. Modulation of hematopoietic stem cell homing and engraftment by CD26. Science. (2004) 305:1000–3. doi: 10.1126/SCIENCE.1097071
63. Broxmeyer HE, Orschell CM, Clapp DW, Hangoc G, Cooper S, Plett PA, et al. Rapid mobilization of murine and human hematopoietic stem and progenitor cells with AMD3100, a CXCR4 antagonist. J Exp Med. (2005) 201:1307–18. doi: 10.1084/JEM.20041385
64. Broxmeyer HE, Kohli L, Kim CH, Lee Y, Mantel C, Cooper S, et al. Stromal cell-derived factor-1/CXCL12 directly enhances survival/antiapoptosis of myeloid progenitor cells through CXCR4 and Gαi proteins and enhances engraftment of competitive, repopulating stem cells. J Leukoc Biol. (2003) 73:630–8. doi: 10.1189/jlb.1002495
65. Broxmeyer HE, Cooper S, Kohli L, Hangoc G, Lee Y, Mantel C, et al. Transgenic expression of stromal cell-derived factor-1/CXC chemokine ligand 12 enhances myeloid progenitor cell survival/antiapoptosis in vitro in response to growth factor withdrawal and enhances myelopoiesis in vivo. J Immunol. (2003) 170:421–9. doi: 10.4049/JIMMUNOL.170.1.421
66. Cash-Padgett T, Sawa A, Jaaro-Peled H. Increased stereotypy in conditional Cxcr4 knockout mice. Neurosci Res. (2016) 105:75–9. doi: 10.1016/J.NEURES.2015.10.001
67. Takabatake Y, Sugiyama T, Kohara H, Matsusaka T, Kurihara H, Koni PA, Nagasawa Y, Hamano T, Matsui I, Kawada N, et al. The CXCL12 (SDF-1)/CXCR4 axis is essential for the development of renal vasculature. J Am Soc Nephrol. (2009) 20:1714–23. doi: 10.1681/ASN.2008060640
68. Berger EA, Murphy PM, Farber JM. Chemokine receptors as HIV-1 coreceptors: roles in viral entry, tropism, and disease. Annu Rev Immunol. (1999) 17:657–700. doi: 10.1146/annurev.immunol.17.1.657
69. Loetscher P, Uguccioni M, Bordoli L, Baggiolini M, Moser B, Chizzolini C, et al. CCR5 is characteristic of Th1 lymphocytes. Nature. (1998) 391:344–5. doi: 10.1038/34812
70. Bonecchi R, Bianchi G, Bordignon PP, D'Ambrosio D, Lang R, Borsatti A, et al. Differential expression of chemokine receptors and chemotactic responsiveness of type 1 T helper cells (Th1s) and Th2s. J Exp Med. (1998) 187:129–34.
71. Haynes BF, Pantaleo G, Fauci AS. Toward an understanding of the correlates of protective immunity to HIV infection. Science. (1996) 271:324–8.
72. Salk J, Bretscher PA, Salk PL, Clerici M, Shearer GM. Response. Science. (1993) 262:1075–6. doi: 10.1126/science.262.5136.1075
73. Tersmette M, de Goede RE, Al BJ, Winkel IN, Gruters RA, Cuypers HT, et al. Differential syncytium-inducing capacity of human immunodeficiency virus isolates: frequent detection of syncytium-inducing isolates in patients with acquired immunodeficiency syndrome (AIDS) and AIDS-related complex. J Virol. (1988) 62:2026–32.
74. Zhu T, Mo H, Wang N, Nam DS, Cao Y, Koup RA, et al. Genotypic and phenotypic characterization of HIV-1 patients with primary infection. Science. (1993) 261:1179–81.
75. Hernandez PA, Gorlin RJ, Lukens JN, Taniuchi S, Bohinjec J, Francois F, et al. Mutations in the chemokine receptor gene CXCR4 are associated with WHIM syndrome, a combined immunodeficiency disease. Nat Genet. (2003) 34:70–4. doi: 10.1038/ng1149
76. Kawai T, Malech HL. WHIM syndrome: congenital immune deficiency disease. Curr Opin Hematol. (2009) 16:20–26. doi: 10.1097/MOH.0b013e32831ac557
77. Marchese A, Raiborg C, Santini F, Keen JH, Stenmark H, Benovic JL. The E3 ubiquitin ligase AIP4 mediates ubiquitination and sorting of the G protein-coupled receptor CXCR4. Dev Cell. (2003) 5:709–22. doi: 10.1016/S1534-5807(03)00321-6
78. Hall JM, Korach KS. Stromal cell-derived factor 1, a novel target of estrogen receptor action, mediates the mitogenic effects of estradiol in ovarian and breast cancer cells. Mol Endocrinol. (2003) 17:792–803. doi: 10.1210/me.2002-0438
79. Taichman RS, Cooper C, Keller ET, Pienta KJ, Taichman NS, McCauley LK. Use of the stromal cell-derived factor-1/CXCR4 pathway in prostate cancer metastasis to bone. Cancer Res. (2002) 62:1832–7.
80. Scala S, Giuliano P, Ascierto PA, Ieranò C, Franco R, Napolitano M, et al. Human melanoma metastases express functional CXCR4. Clin Cancer Res. (2006) 12:2427–33. doi: 10.1158/1078-0432.CCR-05-1940
81. Geminder H, Sagi-Assif O, Goldberg L, Meshel T, Rechavi G, Witz IP, et al. A possible role for CXCR4 and its ligand, the CXC chemokine stromal cell-derived factor-1, in the development of bone marrow metastases in neuroblastoma. J Immunol. (2001) 167:4747–57. doi: 10.4049/jimmunol.167.8.4747
82. Bachelder RE, Crago A, Chung J, Wendt MA, Shaw LM, Robinson G, et al. Vascular endothelial growth factor is an autocrine survival factor for neuropilin-expressing breast carcinoma cells. Cancer Res. (2001) 61:5736–40.
83. Fingleton B. Molecular targets in metastasis: lessons from genomic approaches. Cancer Genomics Proteomics. (2007) 4:211–21.
84. Bohn OL, Nasir I, Brufsky A, Tseng GC, Bhargava R, MacManus K, et al. Biomarker profile in breast carcinomas presenting with bone metastasis. Int J Clin Exp Pathol. (2009) 3:139–46.
85. Kim SY, Lee CH, Midura B V, Yeung C, Mendoza A, Hong SH, et al. Inhibition of the CXCR4/CXCL12 chemokine pathway reduces the development of murine pulmonary metastases. Clin Exp Metastasis. (2008) 25:201–11. doi: 10.1007/s10585-007-9133-3
86. Porvasnik S, Sakamoto N, Kusmartsev S, Eruslanov E, Kim W-J, Cao W, et al. Effects of CXCR4 antagonist CTCE-9908 on prostate tumor growth. Prostate. (2009) 69:1460–9. doi: 10.1002/pros.21008
87. Orimo A, Gupta PB, Sgroi DC, Arenzana-Seisdedos F, Delaunay T, Naeem R, et al. Stromal fibroblasts present in invasive human breast carcinomas promote tumor growth and angiogenesis through elevated SDF-1/CXCL12 secretion. Cell. (2005) 121:335–48. doi: 10.1016/j.cell.2005.02.034
88. Liang Z, Brooks J, Willard M, Liang K, Yoon Y, Kang S, et al. CXCR4/CXCL12 axis promotes VEGF-mediated tumor angiogenesis through Akt signaling pathway. Biochem Biophys Res Commun. (2007) 359:716–22. doi: 10.1016/j.bbrc.2007.05.182
89. Schioppa T, Uranchimeg B, Saccani A, Biswas SK, Doni A, Rapisarda A, et al. Regulation of the chemokine receptor CXCR4 by hypoxia. J Exp Med. (2003) 198:1391–402. doi: 10.1084/jem.20030267
90. Zagzag D, Lukyanov Y, Lan L, Ali MA, Esencay M, Mendez O, et al. Hypoxia-inducible factor 1 and VEGF upregulate CXCR4 in glioblastoma: implications for angiogenesis and glioma cell invasion. Lab Investig. (2006) 86:1221–32. doi: 10.1038/labinvest.3700482
91. Buckley CD, Amft N, Bradfield PF, Pilling D, Ross E, Arenzana-Seisdedos F, et al. Persistent induction of the chemokine receptor CXCR4 by TGF-beta 1 on synovial T cells contributes to their accumulation within the rheumatoid synovium. J Immunol. (2000) 165:3423–9. doi: 10.4049/jimmunol.165.6.3423
92. Salcedo R, Wasserman K, Young HA, Grimm MC, Howard OM, Anver MR, et al. Vascular endothelial growth factor and basic fibroblast growth factor induce expression of CXCR4 on human endothelial cells: in vivo neovascularization induced by stromal-derived factor-1alpha. Am J Pathol. (1999) 154:1125–35.
93. Iikura M, Miyamasu M, Yamaguchi M, Kawasaki H, Matsushima K, Kitaura M, et al. Chemokine receptors in human basophils: inducible expression of functional CXCR4. J Leukoc Biol. (2001) 70:113–20. doi: 10.1189/JLB.70.1.113
94. Nagase H, Miyamasu M, Yamaguchi M, Imanishi M, Tsuno NH, Matsushima K, et al. Cytokine-mediated regulation of CXCR4 expression in human neutrophils. J Leukoc Biol. (2002) 71:711–7. doi: 10.1189/JLB.71.4.711
95. Balabanian K, Lagane B, Infantino S, Chow KYC, Harriague J, Moepps B, et al. The chemokine SDF-1/CXCL12 binds to and signals through the orphan receptor RDC1 in T lymphocytes. J Biol Chem. (2005) 280:35760–6. doi: 10.1074/jbc.M508234200
96. Cancellieri C, Vacchini A, Locati M, Bonecchi R, Borroni EM. Atypical chemokine receptors: from silence to sound. Biochem Soc Trans. (2013) 41:231–6. doi: 10.1042/BST20120246
97. Luker KE, Lewin SA, Mihalko LA, Schmidt BT, Winkler JS, Coggins NL, et al. Scavenging of CXCL12 by CXCR7 promotes tumor growth and metastasis of CXCR4-positive breast cancer cells. Oncogene. (2012) 31:4750–8. doi: 10.1038/onc.2011.633
98. Naumann U, Cameroni E, Pruenster M, Mahabaleshwar H, Raz E, Zerwes H-G, et al. CXCR7 Functions as a Scavenger for CXCL12 and CXCL11. PLoS ONE. (2010) 5:e9175. doi: 10.1371/journal.pone.0009175
99. Levoye A, Balabanian K, Baleux F, Bachelerie F, Lagane B. CXCR7 heterodimerizes with CXCR4 and regulates CXCL12-mediated G protein signaling. Blood. (2009) 113:6085–93. doi: 10.1182/BLOOD-2008-12-196618
100. Sierro F, Biben C, Martínez-Muñoz L, Mellado M, Ransohoff RM, Li M, et al. Disrupted cardiac development but normal hematopoiesis in mice deficient in the second CXCL12/SDF-1 receptor, CXCR7. Proc Natl Acad Sci USA. (2007) 104:14759–64. doi: 10.1073/pnas.0702229104
101. Berahovich RD, Zabel BA, Penfold MET, Lewén S, Wang Y, Miao Z, et al. CXCR7 protein is not expressed on human or mouse leukocytes. J Immunol. (2010) 185:5130–9. doi: 10.4049/jimmunol.1001660
102. Infantino S, Moepps B, Thelen M. Expression and regulation of the orphan receptor RDC1 and its putative ligand in human dendritic and B cells. J Immunol. (2006) 176:2197–207. doi: 10.4049/JIMMUNOL.176.4.2197
103. Singh RK, Lokeshwar BL. The IL-8-regulated chemokine receptor CXCR7 stimulates EGFR signaling to promote prostate cancer growth. Cancer Res. (2011) 71:3268–77. doi: 10.1158/0008-5472.CAN-10-2769
104. Watanabe K, Penfold MET, Matsuda A, Ohyanagi N, Kaneko K, Miyabe Y, et al. Pathogenic role of CXCR7 in rheumatoid arthritis. Arthritis Rheum. (2010) 62:3211–20. doi: 10.1002/art.27650
105. Ngamsri K-C, Müller A, Bösmüller H, Gamper-Tsigaras J, Reutershan J, Konrad FM. The pivotal role of CXCR7 in stabilization of the pulmonary epithelial barrier in acute pulmonary inflammation. J Immunol. (2017) 198:2403–13. doi: 10.4049/jimmunol.1601682
106. Li J-T, Jia L-T, Liu N-N, Zhu X-S, Liu Q-Q, Wang X-L, et al. MiRNA-101 inhibits breast cancer growth and metastasis by targeting CX chemokine receptor 7. Oncotarget. (2015) 6:30818–30. doi: 10.18632/oncotarget.5067
107. Gao W, Mei X, Wang J, Zhang X, Yuan Y. ShRNA-mediated knock-down of CXCR7 increases TRAIL-sensitivity in MCF-7 breast cancer cells. Tumour Biol. (2015) 36:7243–50. doi: 10.1007/s13277-015-3432-0
108. Miao Z, Luker KE, Summers BC, Berahovich R, Bhojani MS, Rehemtulla A, et al. CXCR7 (RDC1) promotes breast and lung tumor growth in vivo and is expressed on tumor-associated vasculature. Proc Natl Acad Sci USA. (2007) 104:15735–40. doi: 10.1073/pnas.0610444104
109. Werner L, Elad H, Brazowski E, Tulchinsky H, Vigodman S, Kopylov U, et al. Reciprocal regulation of CXCR4 and CXCR7 in intestinal mucosal homeostasis and inflammatory bowel disease. J Leukoc Biol. (2011) 90:583–90. doi: 10.1189/jlb.0111101
110. Banisadr G, Podojil JR, Miller SD, Miller RJ. Pattern of CXCR7 Gene expression in mouse brain under normal and inflammatory conditions. J Neuroimmune Pharmacol. (2016) 11:26–35. doi: 10.1007/s11481-015-9616-y
111. Cruz-Orengo L, Holman DW, Dorsey D, Zhou L, Zhang P, Wright M, et al. CXCR7 influences leukocyte entry into the CNS parenchyma by controlling abluminal CXCL12 abundance during autoimmunity. J Exp Med. (2011) 208:327–39. doi: 10.1084/jem.20102010
112. Crump MP. Solution structure and basis for functional activity of stromal cell-derived factor-1; dissociation of CXCR4 activation from binding and inhibition of HIV-1. EMBO J. (1997) 16:6996–7007. doi: 10.1093/emboj/16.23.6996
113. Gupta SK, Pillarisetti K, Thomas RA, Aiyar N. Pharmacological evidence for complex and multiple site interaction of CXCR4 with SDF-1alpha: implications for development of selective CXCR4 antagonists. Immunol Lett. (2001) 78:29–34. doi: 10.1016/S0165-2478(01)00228-0
114. Veldkamp CT, Seibert C, Peterson FC, De la Cruz NB, Haugner JC, Basnet H, et al. Structural basis of CXCR4 sulfotyrosine recognition by the chemokine SDF-1/CXCL12. Sci Signal. (2008) 1:ra4. doi: 10.1126/scisignal.1160755
115. Wu B, Chien EYT, Mol CD, Fenalti G, Liu W, Katritch V, et al. Structures of the CXCR4 chemokine GPCR with small-molecule and cyclic peptide antagonists. Science. (2010) 330:1066–71. doi: 10.1126/science.1194396
116. Schertler GFX, Villa C, Henderson R. Projection structure of rhodopsin. Nature. (1993) 362:770–2.
117. Palczewski K, Kumasaka T, Hori T, Behnke CA, Motoshima H, Fox BA, et al. Crystal structure of rhodopsin: a G protein-coupled receptor. (Illustration). Science. (2000) 289:739. doi: 10.1126/science.289.5480.739
118. Qin L, Kufareva I, Holden L, Wang C, Zheng Y, Zhao C, et al. Crystal structure of the chemokine receptor CXCR4 in complex with a viral chemokine. Science. (2015) 347:1117–22. doi: 10.1126/science.1261064
119. Waterhouse A, Bertoni M, Bienert S, Studer G, Tauriello G, Gumienny R, et al. SWISS-MODEL: Homology modelling of protein structures and complexes. Nucleic Acids Res. (2018) 46:W296–303. doi: 10.1093/nar/gky427
120. Deupi X, Standfuss J. Structural insights into agonist-induced activation of G-protein-coupled receptors. Curr Opin Struct Biol. (2011) 21:541–1. doi: 10.1016/j.sbi.2011.06.002
121. Greasley PJ, Fanelli F, Rossier O, Abuin L, Cotecchia S. Mutagenesis and modelling of the alpha(1b)-adrenergic receptor highlight the role of the helix 3/helix 6 interface in receptor activation. Mol Pharmacol. (2002) 61:1025–32. doi: 10.1124/mol.61.5.1025
122. Han X, Tachado SD, Koziel H, Boisvert WA. Leu128(3.43) (l128) and Val247(6.40) (V247) of CXCR1 are critical amino acid residues for g protein coupling and receptor activation. PLoS ONE. (2012) 7:e42765. doi: 10.1371/journal.pone.0042765
123. Kasai RS, Kusumi A. Single-molecule imaging revealed dynamic GPCR dimerization. Curr Opin Cell Biol. (2014) 27:78–86. doi: 10.1016/j.ceb.2013.11.008
124. Fanelli F, De Benedetti PG. Inactive and active states and supramolecular organization of GPCRs: insights from computational modeling. J Comput Aided Mol Des. (2006) 20:449–61. doi: 10.1007/s10822-006-9064-0
125. Vohra S, Chintapalli S V, Illingworth CJR, Reeves PJ, Mullineaux PM, Clark HSX, et al. Computational studies of Family A and Family B GPCRs. Biochem Soc Trans. (2007) 35:749–54. doi: 10.1042/BST0350749
126. Hauser MA, Schaeuble K, Kindinger I, Impellizzieri D, Krueger WA, Hauck CR, et al. Inflammation-induced CCR7 oligomers form scaffolds to integrate distinct signaling pathways for efficient cell migration. Immunity. (2016) 44:59–72. doi: 10.1016/j.immuni.2015.12.010
127. Springael J-Y, Urizar E, Parmentier M. Dimerization of chemokine receptors and its functional consequences. Cytokine Growth Factor Rev. (2005) 16:611–23. doi: 10.1016/j.cytogfr.2005.05.005
128. Murphy PM. The molecular biology of leukocyte chemoattractant receptors. Annu Rev Immunol. (1994) 12:593–633. doi: 10.1146/annurev.iy.12.040194.003113
129. Mellado M, Rodríguez-Frade JM, Vila-Coro AJ, Fernández S, Martín de Ana A, Jones DR, et al. Chemokine receptor homo- or heterodimerization activates distinct signaling pathways. EMBO J. (2001) 20:2497–507. doi: 10.1093/emboj/20.10.2497
130. Molon B, Gri G, Bettella M, Gómez-Moutón C, Lanzavecchia A, Martínez-A C, et al. T cell costimulation by chemokine receptors. Nat Immunol. (2005) 6:465–71. doi: 10.1038/ni1191
131. Shi G, Partida-Sánchez S, Misra RS, Tighe M, Borchers MT, Lee JJ, et al. Identification of an alternative Gα q -dependent chemokine receptor signal transduction pathway in dendritic cells and granulocytes. J Exp Med. (2007) 204:2705–18. doi: 10.1084/jem.20071267
132. Teicher BA, Fricker SP. CXCL12 (SDF-1)/CXCR4 pathway in cancer. Clin Cancer Res. (2010) 16:2927–31. doi: 10.1158/1078-0432.CCR-09-2329
133. Thorpe LM, Yuzugullu H, Zhao JJ. PI3K in cancer: divergent roles of isoforms, modes of activation and therapeutic targeting. Nat Rev Cancer. (2015) 15:7–24. doi: 10.1038/nrc3860
134. Murga C, Fukuhara S, Gutkind JS. A novel role for phosphatidylinositol 3-kinase beta in signaling from G protein-coupled receptors to Akt. J Biol Chem. (2000) 275:12069–73. doi: 10.1074/jbc.275.16.12069
135. Tian Y, Yin H, Deng X, Tang B, Ren X, Jiang T. CXCL12 induces migration of oligodendrocyte precursor cells through the CXCR4-activated MEK/ERK and PI3K/AKT pathways. Mol Med Rep. (2018) 18:4374–80. doi: 10.3892/mmr.2018.9444
136. Jiménez C, Portela RA, Mellado M, Rodríguez-Frade JM, Collard J, Serrano A, et al. Role of the PI3K regulatory subunit in the control of actin organization and cell migration. J Cell Biol. (2000) 151:249–61. doi: 10.1083/jcb.151.2.249
137. Balabanian K, Levoye A, Klemm L, Lagane B, Hermine O, Harriague J, et al. Leukocyte analysis from WHIM syndrome patients reveals a pivotal role for GRK3 in CXCR4 signaling. J Clin Invest. (2008) 118:1074–84. doi: 10.1172/JCI33187
138. Fong AM, Premont RT, Richardson RM, Yu Y-RA, Lefkowitz RJ, Patel DD. Defective lymphocyte chemotaxis in -arrestin2- and GRK6-deficient mice. Proc Natl Acad Sci USA. (2002) 99:7478–83. doi: 10.1073/pnas.112198299
139. Rajagopal S, Shenoy SK. GPCR desensitization: acute and prolonged phases. Cell Signal. (2018) 41:9–16. doi: 10.1016/j.cellsig.2017.01.024
140. Marchese A, Benovic JL. Agonist-promoted ubiquitination of the G protein-coupled receptor CXCR4 mediates lysosomal sorting. J Biol Chem. (2001) 276:45509–12. doi: 10.1074/jbc.C100527200
141. Caballero A, Mahn SA, Ali MS, Rogers MR, Marchese A. Heterologous regulation of CXCR4 lysosomal trafficking. J Biol Chem. (2019) 294:8023–36. doi: 10.1074/JBC.RA118.005991
142. Bhandari D, Robia SL, Marchese A. The E3 ubiquitin ligase atrophin interacting protein 4 binds directly to the chemokine receptor CXCR4 via a novel WW domain-mediated interaction. Mol Biol Cell. (2009) 20:1324–39. doi: 10.1091/mbc.e08-03-0308
143. Würth R, Bajetto A, Harrison JK, Barbieri F, Florio T. CXCL12 modulation of CXCR4 and CXCR7 activity in human glioblastoma stem-like cells and regulation of the tumor microenvironment. Front Cell Neurosci. (2014) 8:144. doi: 10.3389/fncel.2014.00144
144. Alkhabit G. The biology of CCR5 and CXCR4. Curr Opin HIV AIDS. (2009) 4:96–103. doi: 10.1016/j.freeradbiomed.2008.10.025
145. Coke CJ, Scarlett KA, Chetram MA, Jones KJ, Sandifer BJ, Davis AS, et al. Simultaneous activation of induced heterodimerization between CXCR4 chemokine receptor and cannabinoid receptor 2 (CB2) reveals a mechanism for regulation of tumor progression. J Biol Chem. (2016) 291:9991–10005. doi: 10.1074/jbc.M115.712661
146. Zgraggen S, Huggenberger R, Kerl K, Detmar M. An important role of the SDF-1/CXCR4 axis in chronic skin inflammation. PLoS ONE. (2014) 9:e93665. doi: 10.1371/journal.pone.0093665
147. Takekoshi T, Wu X, Mitsui H, Tada Y, Kao MC, Sato S, et al. CXCR4 negatively regulates keratinocyte proliferation in IL-23-mediated psoriasiform dermatitis. J Invest Dermatol. (2013) 133:2530–7. doi: 10.1038/jid.2013.151
148. Meiron M, Zohar Y, Anunu R, Wildbaum G, Karin N. CXCL12 (SDF-1alpha) suppresses ongoing experimental autoimmune encephalomyelitis by selecting antigen-specific regulatory T cells. J Exp Med. (2008) 205:2643–55. doi: 10.1084/jem.20080730
149. Nanki T, Hayashida K, El-Gabalawy HS, Suson S, Shi K, Girschick HJ, et al. Stromal cell-derived factor-1-CXC chemokine receptor 4 interactions play a central role in CD4+ T cell accumulation in rheumatoid arthritis synovium. J Immunol. (2000) 165:6590–8.
150. Pablos JL, Santiago B, Galindo M, Torres C, Brehmer MT, Blanco FJ, et al. Synoviocyte-derived CXCL12 is displayed on endothelium and induces angiogenesis in rheumatoid arthritis. J Immunol. (2003) 170:2147–52. doi: 10.4049/jimmunol.170.4.2147
151. Wei F, Moore DC, Wei L, Li Y, Zhang G, Wei X, et al. Attenuation of osteoarthritis via blockade of the SDF-1/CXCR4 signaling pathway. Arthritis Res Ther. (2012) 14:R177. doi: 10.1186/ar3930
152. Kanbe K, Takemura T, Takeuchi K, Chen Q, Takagishi K, Inoue K. Synovectomy reduces stromal-cell-derived factor-1 (SDF-1) which is involved in the destruction of cartilage in osteoarthritis and rheumatoid arthritis. J Bone Joint Surg Br. (2004) 86:296–300. doi: 10.1302/0301-620X.86B2.14474
153. Wright LM, Maloney W, Yu X, Kindle L, Collin-Osdoby P, Osdoby P. Stromal cell-derived factor-1 binding to its chemokine receptor CXCR4 on precursor cells promotes the chemotactic recruitment, development and survival of human osteoclasts. Bone. (2005) 36:840–53. doi: 10.1016/J.BONE.2005.01.021
154. De Klerck B, Geboes L, Hatse S, Kelchtermans H, Meyvis Y, Vermeire K, et al. Pro-inflammatory properties of stromal cell-derived factor-1 (CXCL12) in collagen-induced arthritis. Arthritis Res Ther. (2005) 7:R1208–20. doi: 10.1186/ar1806
155. Wang A, Fairhurst A-M, Tus K, Subramanian S, Liu Y, Lin F, et al. CXCR4/CXCL12 hyperexpression plays a pivotal role in the pathogenesis of lupus. J Immunol. (2009) 182:4448–58. doi: 10.4049/jimmunol.0801920
156. Rizzo P, Perico N, Gagliardini E, Novelli R, Alison MR, Remuzzi G, Benigni A. Nature and mediators of parietal epithelial cell activation in glomerulonephritides of human and rat. Am J Pathol. (2013) 183:1769–78. doi: 10.1016/j.ajpath.2013.08.008
157. Matin K, Salam MA, Akhter J, Hanada N, Senpuku H. Role of stromal-cell derived factor-1 in the development of autoimmune diseases in non-obese diabetic mice. Immunology. (2002) 107:222–32. doi: 10.1046/j.1365-2567.2002.01478.x
158. Sharp CD, Huang M, Glawe J, Patrick DR, Pardue S, Barlow SC, et al. Stromal cell derived factor-1/CXCL12 stimulates chemorepulsion of NOD/LtJ T-cell adhesion to islet microvascular endothelium. Diabetes. (2008) 57:102–12. doi: 10.2337/db07-0494
159. Liu Z, Habener JF. Stromal cell-derived factor-1 promotes survival of pancreatic beta cells by the stabilisation of beta-catenin and activation of transcription factor 7-like 2 (TCF7L2). Diabetologia. (2009) 52:1589–98. doi: 10.1007/s00125-009-1384-x
160. Butler JM, Guthrie SM, Koc M, Afzal A, Caballero S, Brooks HL, et al. SDF-1 is both necessary and sufficient to promote proliferative retinopathy. J Clin Invest. (2005) 115:86–93. doi: 10.1172/JCI22869
161. Pashenkov M, Söderström M, Link H. Secondary lymphoid organ chemokines are elevated in the cerebrospinal fluid during central nervous system inflammation. J Neuroimmunol. (2003) 135:154–60. doi: 10.1016/S0165-5728(02)00441-1
162. Hansen IB, Ellingsen T, Hornung N, Poulsen JH, Lottenburger T, Stengaard-Pedersen K. Plasma level of CXC-chemokine CXCL12 is increased in rheumatoid arthritis and is independent of disease activity and methotrexate treatment. J Rheumatol. (2006) 33:1754–9.
163. Leng Q, Nie Y, Zou Y, Chen J. Elevated CXCL12 expression in the bone marrow of NOD mice is associated with altered T cell and stem cell trafficking and diabetes development. BMC Immunol. (2008) 9:51. doi: 10.1186/1471-2172-9-51
164. Mikami S, Nakase H, Yamamoto S, Takeda Y, Yoshino T, Kasahara K, et al. Blockade of CXCL12/CXCR4 axis ameliorates murine experimental colitis. J Pharmacol Exp Ther. (2008) 327:383–92. doi: 10.1124/jpet.108.141085
165. Nagafuchi Y, Shoda H, Sumitomo S, Nakachi S, Kato R, Tsuchida Y, et al. Immunophenotyping of rheumatoid arthritis reveals a linkage between HLA-DRB1 genotype, CXCR4 expression on memory CD4(+) T cells, and disease activity. Sci Rep. (2016) 6:29338. doi: 10.1038/srep29338
166. Perera GK, Di Meglio P, Nestle FO. Psoriasis. Annu Rev Pathol. (2012) 7:385–422. doi: 10.1146/annurev-pathol-011811-132448
167. Lowes MA, Bowcock AM, Krueger JG. Pathogenesis and therapy of psoriasis. Nature. (2007) 445:866–73. doi: 10.1038/nature05663
168. Huggenberger R, Detmar M. The cutaneous vascular system in chronic skin inflammation. J Investig dermatology Symp Proc. (2011) 15:24–32. doi: 10.1038/jidsymp.2011.5
169. Ransohoff RM, Engelhardt B. The anatomical and cellular basis of immune surveillance in the central nervous system. Nat Rev Immunol. (2012) 12:623–35. doi: 10.1038/nri3265
170. Ransohoff RM. Chemokines and chemokine receptors: standing at the crossroads of immunobiology and neurobiology. Immunity. (2009) 31:711–21. doi: 10.1016/j.immuni.2009.09.010
171. Raychaudhuri SP, Jiang WY, Farber EM. Cellular localization of fractalkine at sites of inflammation: antigen-presenting cells in psoriasis express high levels of fractalkine. Br J Dermatol. (2001) 144:1105–13. doi: 10.1046/j.1365-2133.2001.04219.x
172. Aung G, Niyonsaba F, Ushio H, Kajiwara N, Saito H, Ikeda S, et al. Catestatin, a neuroendocrine antimicrobial peptide, induces human mast cell migration, degranulation and production of cytokines and chemokines. Immunology. (2011) 132:527–39. doi: 10.1111/j.1365-2567.2010.03395.x
173. Mirshahi F, Pourtau J, Li H, Muraine M, Trochon V, Legrand E, et al. SDF-1 activity on microvascular endothelial cells: consequences on angiogenesis in in vitro and in vivo models. Thromb Res. (2000) 99:587–94. doi: 10.1016/S0049-3848(00)00292-9
174. Pablos JL, Amara A, Bouloc A, Santiago B, Caruz A, Galindo M, et al. Stromal-cell derived factor is expressed by dendritic cells and endothelium in human skin. Am J Pathol. (1999) 155:1577–86. doi: 10.1016/S0002-9440(10)65474-0
175. Petit I, Jin D, Rafii S. The SDF-1–CXCR4 signaling pathway: a molecular hub modulating neo-angiogenesis. Trends Immunol. (2007) 28:299–307. doi: 10.1016/j.it.2007.05.007
176. Suárez-Fariñas M, Fuentes-Duculan J, Lowes MA, Krueger JG. Resolved psoriasis lesions retain expression of a subset of disease-related genes. J Invest Dermatol. (2011) 131:391–400. doi: 10.1038/jid.2010.280
177. Nylander A, Hafler DA. Multiple sclerosis. J Clin Invest. (2012) 122:1180–8. doi: 10.1172/JCI58649
178. Kingwell E, Marriott JJ, Jetté N, Pringsheim T, Makhani N, Morrow SA, et al. Incidence and prevalence of multiple sclerosis in Europe: a systematic review. BMC Neurol. (2013) 13:128. doi: 10.1186/1471-2377-13-128
179. Hemmer B, Archelos JJ, Hartung H-P. New concepts in the immunopathogenesis of multiple sclerosis. Nat Rev Neurosci. (2002) 3:291–301. doi: 10.1038/nrn784
180. Perry VH, Anthony DC, Bolton SJ, Brown HC. The blood-brain barrier and the inflammatory response. Mol Med Today. (1997) 3:335–41. doi: 10.1016/S1357-4310(97)01077-0
181. Goverman J. Autoimmune T cell responses in the central nervous system. Nat Rev Immunol. (2009) 9:393–407. doi: 10.1038/nri2550
182. Kawakami N, Lassmann S, Li Z, Odoardi F, Ritter T, Ziemssen T, et al. The activation status of neuroantigen-specific T cells in the target organ determines the clinical outcome of autoimmune encephalomyelitis. J Exp Med. (2004) 199:185–97. doi: 10.1084/jem.20031064
183. Greter M, Heppner FL, Lemos MP, Odermatt BM, Goebels N, Laufer T, et al. Dendritic cells permit immune invasion of the CNS in an animal model of multiple sclerosis. Nat Med. (2005) 11:328–34. doi: 10.1038/nm1197
184. Hickey WF, Kimura H. Perivascular microglial cells of the CNS are bone marrow-derived and present antigen in vivo. Science. (1988) 239:290–2.
185. Reboldi A, Coisne C, Baumjohann D, Benvenuto F, Bottinelli D, Lira S, et al. C-C chemokine receptor 6–regulated entry of TH-17 cells into the CNS through the choroid plexus is required for the initiation of EAE. Nat Immunol. (2009) 10:514–23. doi: 10.1038/ni.1716
186. Wilson EH, Weninger W, Hunter CA. Trafficking of immune cells in the central nervous system. J Clin Invest. (2010) 120:1368–79. doi: 10.1172/JCI41911
187. Rempel SA, Dudas S, Ge S, Gutiérrez JA. Identification and localization of the cytokine SDF1 and its receptor, CXC chemokine receptor 4, to regions of necrosis and angiogenesis in human glioblastoma. Clin Cancer Res. (2000) 6:102–11.
188. Rostasy K, Egles C, Chauhan A, Kneissl M, Bahrani P, Yiannoutsos C, et al. SDF-1α is expressed in astrocytes and neurons in the AIDS dementia complex: an in vivo and in vitro study. J Neuropathol Exp Neurol. (2003) 62:617–26. doi: 10.1093/jnen/62.6.617
189. Hill WD, Hess DC, Martin-Studdard A, Carothers JJ, Zheng J, Hale D, et al. SDF-1 (CXCL12) is upregulated in the ischemic penumbra following stroke: association with bone marrow cell homing to injury. J Neuropathol Exp Neurol. (2004) 63:84–96. doi: 10.1093/jnen/63.1.84
190. Metelitsa LS, Wu H-W, Wang H, Yang Y, Warsi Z, Asgharzadeh S, et al. Natural killer T cells infiltrate neuroblastomas expressing the chemokine CCL2. J Exp Med. (2004) 199:1213–21. doi: 10.1084/jem.20031462
191. Miller JT, Bartley JH, Wimborne HJ, Walker AL, Hess DC, Hill WD, et al. The neuroblast and angioblast chemotaxic factor SDF-1 (CXCL12) expression is briefly up regulated by reactive astrocytes in brain following neonatal hypoxic-ischemic injury. BMC Neurosci. (2005) 6:63. doi: 10.1186/1471-2202-6-63
192. Peng H, Erdmann N, Whitney N, Dou H, Gorantla S, Gendelman HE, et al. HIV-1-infected and/or immune activated macrophages regulate astrocyte SDF-1 production through IL-1β. Glia. (2006) 54:619–29. doi: 10.1002/glia.20409
193. Tabatabai G, Frank B, Möhle R, Weller M, Wick W. Irradiation and hypoxia promote homing of haematopoietic progenitor cells towards gliomas by TGF- -dependent HIF-1 -mediated induction of CXCL12. Brain. (2006) 129:2426–35. doi: 10.1093/brain/awl173
194. Ceradini DJ, Kulkarni AR, Callaghan MJ, Tepper OM, Bastidas N, Kleinman ME, et al. Progenitor cell trafficking is regulated by hypoxic gradients through HIF-1 induction of SDF-1. Nat Med. (2004) 10:858–64. doi: 10.1038/nm1075
195. Corcione A, Casazza S, Ferretti E, Giunti D, Zappia E, Pistorio A, et al. Recapitulation of B cell differentiation in the central nervous system of patients with multiple sclerosis. Proc Natl Acad Sci USA. (2004) 101:11064–9. doi: 10.1073/pnas.0402455101
196. Krumbholz M, Theil D, Cepok S, Hemmer B, Kivisäkk P, Ransohoff RM, et al. Chemokines in multiple sclerosis: CXCL12 and CXCL13 up-regulation is differentially linked to CNS immune cell recruitment. Brain. (2006) 129:200–11. doi: 10.1093/brain/awh680
197. Calderon TM, Eugenin EA, Lopez L, Kumar SS, Hesselgesser J, Raine CS, et al. A role for CXCL12 (SDF-1α) in the pathogenesis of multiple sclerosis: regulation of CXCL12 expression in astrocytes by soluble myelin basic protein. J Neuroimmunol. (2006) 177:27–39. doi: 10.1016/j.jneuroim.2006.05.003
198. Li M, Ransohoff RM. Multiple roles of chemokine CXCL12 in the central nervous system: a migration from immunology to neurobiology. Prog Neurobiol. (2008) 84:116–31. doi: 10.1016/j.pneurobio.2007.11.003
199. McCandless EE, Wang Q, Woerner BM, Harper JM, Klein RS. CXCL12 limits inflammation by localizing mononuclear infiltrates to the perivascular space during experimental autoimmune encephalomyelitis. J Immunol. (2006) 177:8053–64. doi: 10.4049/jimmunol.177.11.8053
200. Kohler RE, Comerford I, Townley S, Haylock-Jacobs S, Clark-Lewis I, McColl SR. Antagonism of the chemokine receptors CXCR3 and CXCR4 reduces the pathology of experimental autoimmune encephalomyelitis. Brain Pathol. (2008) 18:504–16. doi: 10.1111/j.1750-3639.2008.00154.x
201. Hanes MS, Salanga CL, Chowdry AB, Comerford I, McColl SR, Kufareva I, et al. Dual targeting of the chemokine receptors CXCR4 and ACKR3 with novel engineered chemokines. J Biol Chem. (2015) 290:22385–97. doi: 10.1074/jbc.M115.675108
202. Cruz-Orengo L, Chen Y-J, Kim J, Dorsey D, Song S-K, Klein RS. CXCR7 antagonism prevents axonal injury during experimental autoimmune encephalomyelitis as revealed by in vivo axial diffusivity. J Neuroinflammation. (2011) 8:170. doi: 10.1186/1742-2094-8-170
203. Bao J, Zhu J, Luo S, Cheng Y, Zhou S. CXCR7 suppression modulates microglial chemotaxis to ameliorate experimentally-induced autoimmune encephalomyelitis. Biochem Biophys Res Commun. (2016) 469:1–7. doi: 10.1016/j.bbrc.2015.11.059
204. Firestein GS. Evolving concepts of rheumatoid arthritis. Nature. (2003) 423:356–61. doi: 10.1038/nature01661
205. Brennan FM, McInnes IB. Evidence that cytokines play a role in rheumatoid arthritis. J Clin Invest. (2008) 118:3537–3545. doi: 10.1172/JCI36389
206. McInnes IB, Schett G. Cytokines in the pathogenesis of rheumatoid arthritis. Nat Rev Immunol. (2007) 7:429–42. doi: 10.1038/nri2094
207. McInnes IB, Buckley CD, Isaacs JD. Cytokines in rheumatoid arthritis — shaping the immunological landscape. Nat Rev Rheumatol. (2016) 12:63–8. doi: 10.1038/nrrheum.2015.171
208. Szekanecz Z, Koch AE. Vascular involvement in rheumatic diseases: “Vascular rheumatology.” Arthritis Res Ther. (2008) 10:224. doi: 10.1186/ar2515
209. Taylor PC, Sivakumar B. Hypoxia and angiogenesis in rheumatoid arthritis. Curr Opin Rheumatol. (2005) 17:293–8. doi: 10.1097/01.bor.0000155361.83990.5b
210. Pitzalis C, Jones GW, Bombardieri M, Jones SA. Ectopic lymphoid-like structures in infection, cancer and autoimmunity. Nat Rev Immunol. (2014) 14:447–62. doi: 10.1038/nri3700
211. Atkinson MA, Eisenbarth GS, Michels AW. Type 1 diabetes. Lancet. (2014) 383:69–82. doi: 10.1016/S0140-6736(13)60591-7
212. Todd JA. Etiology of type 1 diabetes. Immunity. (2010) 32:457–67. doi: 10.1016/j.immuni.2010.04.001
213. Catrina AI, Svensson CI, Malmström V, Schett G, Klareskog L. Mechanisms leading from systemic autoimmunity to joint-specific disease in rheumatoid arthritis. Nat Rev Rheumatol. (2017) 13:79–86. doi: 10.1038/nrrheum.2016.200
214. Nanki T, Nagasaka K, Hayashida K, Saita Y, Miyasaka N. Chemokines regulate IL-6 and IL-8 production by fibroblast-like synoviocytes from patients with rheumatoid arthritis. J Immunol. (2001) 167:5381–5.
215. Yu X, Huang Y, Collin-Osdoby P, Osdoby P. Stromal cell-derived factor-1 (SDF-1) recruits osteoclast precursors by inducing chemotaxis, matrix metalloproteinase-9 (MMP-9) activity, and collagen transmigration. J Bone Miner Res. (2003) 18:1404–18. doi: 10.1359/jbmr.2003.18.8.1404
216. Grassi F, Cristino S, Toneguzzi S, Piacentini A, Facchini A, Lisignoli G. CXCL12 chemokine up-regulates bone resorption and MMP-9 release by human osteoclasts: CXCL12 levels are increased in synovial and bone tissue of rheumatoid arthritis patients. J Cell Physiol. (2004) 199:244–51. doi: 10.1002/jcp.10445
217. Tsokos GC. Systemic lupus erythematosus. N Engl J Med. (2011) 365:2110–21. doi: 10.1056/NEJMra1100359
218. Saxena R, Mahajan T, Mohan C. Lupus nephritis: current update. Arthritis Res Ther. (2011) 13:240. doi: 10.1186/ar3378
219. Mok CC, Lau CS. Pathogenesis of systemic lupus erythematosus. J Clin Pathol. (2003) 56:481–90. doi: 10.1136/jcp.56.7.481
220. Liao X, Pirapakaran T, Luo XM. Chemokines and chemokine receptors in the development of lupus nephritis. Mediators Inflamm. (2016) 2016:6012715. doi: 10.1155/2016/6012715
221. Badr G, Sayed A, Abdel-Maksoud MA, Mohamed AO, El-Amir A, Abdel-Ghaffar FA, et al. Infection of female BWF1 lupus mice with malaria parasite attenuates B cell autoreactivity by modulating the CXCL12/CXCR4 axis and its downstream signals PI3K/AKT, NFκB and ERK. PLoS ONE. (2015) 10:e0125340. doi: 10.1371/journal.pone.0125340
222. Balabanian K, Couderc J, Bouchet-Delbos L, Amara A, Berrebi D, Foussat A, et al. Role of the chemokine stromal cell-derived factor 1 in autoantibody production and nephritis in murine lupus. J Immunol. (2003) 170:3392–400. doi: 10.4049/jimmunol.170.6.3392
223. Wang A, Guilpain P, Chong BF, Chouzenoux S, Guillevin L, Du Y, et al. Dysregulated expression of CXCR4/CXCL12 in subsets of patients with systemic lupus erythematosus. Arthritis Rheum. (2010) 62:3436–46. doi: 10.1002/art.27685
224. Hanaoka H, Okazaki Y, Hashiguchi A, Yasuoka H, Takeuchi T, Kuwana M. Overexpression of CXCR4 on circulating B cells in patients with active systemic lupus erythematosus. Clin Exp Rheumatol. (2015) 33:863–70.
225. Zhao L, Liang D, Wu X, Li Y, Niu J, Zhou C, et al. Contribution and underlying mechanisms of CXCR4 overexpression in patients with systemic lupus erythematosus. Cell Mol Immunol. (2017) 14:842–9. doi: 10.1038/cmi.2016.47
226. Bluestone JA, Herold K, Eisenbarth G. Genetics, pathogenesis and clinical interventions in type 1 diabetes. Nature. (2010) 464:1293–300. doi: 10.1038/nature08933
227. Antonelli A, Ferrari SM, Corrado A, Ferrannini E, Fallahi P. CXCR3, CXCL10 and type 1 diabetes. Cytokine Growth Factor Rev. (2014) 25:57–65. doi: 10.1016/j.cytogfr.2014.01.006
228. Overbergh L, Gysemans C, Mathieu C. Quantification of chemokines by real-time reverse transcriptase PCR: applications in type 1 diabetes. Expert Rev Mol Diagn. (2006) 6:51–64. doi: 10.1586/14737159.6.1.51
229. Aboumrad E, Madec AM, Thivolet C. The CXCR4/CXCL12 (SDF-1) signalling pathway protects non-obese diabetic mouse from autoimmune diabetes. Clin Exp Immunol. (2007) 148:432–9. doi: 10.1111/j.1365-2249.2007.03370.x
230. Papeta N, Chen T, Vianello F, Gererty L, Malik A, Mok Y-T, et al. Long-term survival of transplanted allogeneic cells engineered to express a T cell chemorepellent. Transplantation. (2007) 83:174–83. doi: 10.1097/01.tp.0000250658.00925.c8
231. Nti BK, Markman JL, Bertera S, Styche AJ, Lakomy RJ, Subbotin VM, et al. Treg cells in pancreatic lymph nodes: the possible role in diabetogenesis and β cell regeneration in a T1D model. Cell Mol Immunol. (2012) 9:455–63. doi: 10.1038/cmi.2012.36
232. Feng G, Hao D, Chai J. Processing of CXCL12 impedes the recruitment of endothelial progenitor cells in diabetic wound healing. FEBS J. (2014) 281:5054–62. doi: 10.1111/febs.13043
233. Gallagher KA, Liu Z-J, Xiao M, Chen H, Goldstein LJ, Buerk DG, et al. Diabetic impairments in NO-mediated endothelial progenitor cell mobilization and homing are reversed by hyperoxia and SDF-1α. J Clin Invest. (2007) 117:1249–59. doi: 10.1172/JCI29710
234. Tögel F, Isaac J, Hu Z, Weiss K, Westenfelder C. Renal SDF-1 signals mobilization and homing of CXCR4-positive cells to the kidney after ischemic injury. Kidney Int. (2005) 67:1772–84. doi: 10.1111/j.1523-1755.2005.00275.x
235. Wallace KL, Zheng L-B, Kanazawa Y, Shih DQ. Immunopathology of inflammatory bowel disease. World J Gastroenterol. (2014) 20:6. doi: 10.3748/wjg.v20.i1.6
236. Scharl M, Rogler G. Inflammatory bowel disease pathogenesis. Curr Opin Gastroenterol. (2012) 28:301–9. doi: 10.1097/MOG.0b013e328353e61e
237. Shen Z-H, Zhu C-X, Quan Y-S, Yang Z-Y, Wu S, Luo W-W, et al. Relationship between intestinal microbiota and ulcerative colitis: mechanisms and clinical application of probiotics and fecal microbiota transplantation. World J Gastroenterol. (2018) 24:5–14. doi: 10.3748/wjg.v24.i1.5
238. MacDermott RP. Chemokines in the inflammatory bowel diseases. J Clin Immunol. (1999) 19:266–72.
239. Papadakis KA, Targan SR. The role of chemokines and chemokine receptors in mucosal inflammation. Inflamm Bowel Dis. (2000) 6:303–13. doi: 10.1002/ibd.3780060408
240. Agace WW, Amara A, Roberts AI, Pablos JL, Thelen S, Uguccioni M, et al. Constitutive expression of stromal derived factor-1 by mucosal epithelia and its role in HIV transmission and propagation. Curr Biol. (2000) 10:325–8. doi: 10.1016/S0960-9822(00)00380-8
241. Katsuta T, Lim C, Shimoda K, Shibuta K, Mitra P, Banner BF, et al. Interleukin-8 and SDF1-alpha mRNA expression in colonic biopsies from patients with inflammatory bowel disease. Am J Gastroenterol. (2000) 95:3157–64. doi: 10.1111/j.1572-0241.2000.03289.x
242. Jordan NJ, Kolios G, Abbot SE, Sinai MA, Thompson DA, Petraki K, et al. Expression of functional CXCR4 chemokine receptors on human colonic epithelial cells. J Clin Invest. (1999) 104:1061–9. doi: 10.1172/JCI6685
243. Dotan I, Werner L, Vigodman S, Weiss S, Brazowski E, Maharshak N, et al. CXCL12 is a constitutive and inflammatory chemokine in the intestinal immune system. Inflamm Bowel Dis. (2010) 16:583–92. doi: 10.1002/ibd.21106
244. Mrowicki J, Przybylowska-Sygut K, Dziki L, Sygut A, Chojnacki J, Dziki A, et al. The role of polymorphisms of genes CXCL12/CXCR4 and MIF in the risk development IBD the Polish population. Mol Biol Rep. (2014) 41:4639–52. doi: 10.1007/s11033-014-3335-y
245. Xia X-M, Wang F-Y, Xu W-A, Wang Z-K, Liu J, Lu Y-K, et al. CXCR4 antagonist AMD3100 attenuates colonic damage in mice with experimental colitis. World J Gastroenterol. (2010) 16:2873. doi: 10.3748/wjg.v16.i23.2873
246. Zheng X-B, He X-W, Zhang L-J, Qin H-B, Lin X-T, Liu X-H, et al. Bone marrow-derived CXCR4-overexpressing MSCs display increased homing to intestine and ameliorate colitis-associated tumorigenesis in mice. Gastroenterol Rep. (2019) 7:127–38. doi: 10.1093/gastro/goy017
247. Wang M, Yuan Q, Xie L. Mesenchymal stem cell-based immunomodulation: properties and clinical application. Stem Cells Int. (2018) 2018:3057624. doi: 10.1155/2018/3057624
248. Werner L, Guzner-Gur H, Dotan I. Involvement of CXCR4/CXCR7/CXCL12 interactions in inflammatory bowel disease. Theranostics. (2013) 3:40–6. doi: 10.7150/thno.5135
249. Hartmann TN, Grabovsky V, Pasvolsky R, Shulman Z, Buss EC, Spiegel A, et al. A crosstalk between intracellular CXCR7 and CXCR4 involved in rapid CXCL12-triggered integrin activation but not in chemokine-triggered motility of human T lymphocytes and CD34+ cells. J Leukoc Biol. (2008) 84:1130–40. doi: 10.1189/jlb.0208088
250. Wang H, Tao L, Qi KE, Zhang H, Feng D, Wei W, et al. CXCR7 functions in colon cancer cell survival and migration. Exp Ther Med. (2015) 10:1720–4. doi: 10.3892/etm.2015.2748
Keywords: chemokines/chemokine receptors, inflammation, autoimmunity, CXCL12 chemokine, CXCR4 = chemokine receptor 4, ACKR3
Citation: García-Cuesta EM, Santiago CA, Vallejo-Díaz J, Juarranz Y, Rodríguez-Frade JM and Mellado M (2019) The Role of the CXCL12/CXCR4/ACKR3 Axis in Autoimmune Diseases. Front. Endocrinol. 10:585. doi: 10.3389/fendo.2019.00585
Received: 17 June 2019; Accepted: 09 August 2019;
Published: 27 August 2019.
Edited by:
Alain Couvineau, Institut National de la Santé et de la Recherche Médicale (INSERM), FranceReviewed by:
Francoise Bachelerie, Institut National de la Santé et de la Recherche Médicale (INSERM), FranceTullio Florio, University of Genoa, Italy
Copyright © 2019 García-Cuesta, Santiago, Vallejo-Díaz, Juarranz, Rodríguez-Frade and Mellado. This is an open-access article distributed under the terms of the Creative Commons Attribution License (CC BY). The use, distribution or reproduction in other forums is permitted, provided the original author(s) and the copyright owner(s) are credited and that the original publication in this journal is cited, in accordance with accepted academic practice. No use, distribution or reproduction is permitted which does not comply with these terms.
*Correspondence: Mario Mellado, bW1lbGxhZG8mI3gwMDA0MDtjbmIuY3NpYy5lcw==