- Departamento de Genética del Desarrollo y Fisiología Molecular, Instituto de Biotecnología, Universidad Nacional Autónoma de Mexico, Cuernavaca, Mexico
The activity of the hypothalamus-pituitary-thyroid (HPT) axis is inhibited by energy deficit, by acute or chronic stress, but activated by cold exposure or exercise. Because stress curtails acute cold induced activation of HPT, we evaluated the effect of chronic stress on HPT axis response to voluntary exercise, a persistent energy-demanding situation. Adult male and female Wistar rats were exposed to restraint stress, 30 min/day for 2 weeks, or to isolation (Iso) [post-natal day [PND] 30–63]. Exercise was performed (7 p.m.−7 a.m.) in a running wheel, sedentary controls stayed in individual cages (Sed); at 7 a.m. they were housed with their cage mate or individually (Iso); food intake by the exercised group was measured day and night to pair-fed Sed. At sacrifice, hormones, mRNA levels and tissue weights were quantified. Control or restrained adult rats had access to running wheel daily for 2 weeks. Compared to C, exercise decreased white adipose tissue (WAT) mass in females and males, increased hypothalamic paraventricular nucleus (PVN)-Trh expression in males proportionally to exercise performed, and increased TSH and T4 serum concentration in females. These changes were not detected in restrained groups. Starting at PND 63 control (2/cage) and isolated (1/cage) rats either exercised on 10 alternated nights or were sedentary. In control male animals, compared to Sed rats, exercise did not decrease WAT mass, nor changed HPT axis activity, but increased Pomc and deiodinase 2 (Dio2) expression in mediobasal hypothalamus (MBH), adrenergic receptor β3 and uncoupling protein-1 in brown adipose tissue. In control female animals, exercise decreased WAT mass, increased Pomc, Dio2, and Trhde expression in MBH, and TSH serum concentration. Iso females had lower TSH and T4 serum concentration, Dio2 and Trhde expression in MBH than controls. The stress response was higher in isolated males than females, but in males it did not alter the effects of exercise, in contrast to isolated females that had a blunted response to exercise compared to controls. In conclusion, chronic stress interferes with metabolic effects produced by exercise, such as loss of WAT mass, coincident with dampening of HPT activity.
Introduction
Physical activity and food intake are the two most important controllable variable in setting body weight. Energy expenditure is produced by basal metabolism and thermogenesis required for everyday living and physical activity. In concert with the sympathetic nervous system, thyroid hormones (TH) contribute to thermogenesis and basal metabolic rate, but they also participate in several aspects of exercise performance as they are directly involved in muscle activity, mitochondrial biogenesis, lipolysis, glycolysis, and gluconeogenesis, and mobilization of fuel to metabolically active tissues (1, 2). Patients with hypothyroidism, overt or subclinical, have reduced exercise tolerance, endurance, muscle strength, cardiovascular functions, deficient control of energy expenditure, fatigue, and poor quality of life (3–6).
Tissue levels of thyroid hormones are regulated by the activity of the hypothalamic-pituitary-thyroid (HPT) axis, and at target cells by transporters, deiodinases, and membrane or intracellular TH receptors, the latter acting as transcription factors (1, 7–9). The HPT axis is subject to multifactorial regulation, initiating with neurons of the hypothalamic paraventricular nucleus (PVN) that synthesize thyrotropin releasing hormone (TRH) and release it in the median eminence, in the vicinity of portal vessels and β2-tanycytes. These neurons receive multiple afferents from the arcuate nucleus and other brain areas that convey information of environmental, nutritional and metabolic status (10, 11). Tanycytes express deiodinase-2 (Dio2) that transforms T4 to T3, TH transporters, and TRH-degrading enzyme (Trhde) that inactivates released TRH (10). Once in portal vessels, TRH is transported to the adenohypophysis where it controls synthesis and release of thyrotropin (TSH). TSH activates synthesis of T4 in thyroid; some T4 is deiodinated to T3 in thyroid but most in target tissues (1, 12, 13). T3 is the active hormone at transcriptional level and responsible for feedback inhibition on TRH and TSH synthesis (7, 14, 15).
Multiple factors modulate the activity of the HPT axis; it is inhibited in situations of energy deficit as fasting, food restriction or chronic illness, and stimulated in certain conditions of energy excess (10, 16). Situations of energy demands as cold exposure and some types of exercise increase HPT axis activity (17–20). Rats running in a treadmill have increased serum concentration of T3 at the beginning of exercise (21) but lower after 120 min. In humans, contradictory results relate to the intensity and duration of exercise, nutritional status, and timing of blood samples; increased TSH serum concentrations are detected at short times after an exercise bout, and of T4 in some conditions although dehydration may give false results (22); increased TSH and TH concentration is detected after endurance training if measured before exhaustion but inhibited if energy reserves are temporarily depleted, or in conditions of high release of corticosterone or interleukins, for example (23). Research in animals has produced also inconsistent results; among the confounding factors, exercise usually diminishes food intake (19, 24), which by itself drives inhibition of HPT axis; likewise, for stress imposed by certain types of exercise (21). Access to a wheel provides rodents with the opportunity to exercise in voluntary non-stressed conditions (25). Using this paradigm we previously demonstrated that compared to sedentary animals exercised rats decrease their food intake and mass of white adipose tissue (WAT), and diminish the parameters that reflect HPT axis activity; however, if values are compared to a pair-fed group there is still a strong decrease in WAT mass, but PVN Trh expression, TSH and T3 serum concentrations are higher and proportional to the loss of WAT mass (19).
Another important regulator on HPT activity is stress, either acute or chronic, which may cause inhibition. Stress diminishes synthesis and release of TRH, TSH, and TH, and hepatic conversion of T4 into T3 (26–30); some effects are mimicked by acute or chronic corticosterone injections (31, 32). Not only basal HPT axis activity is affected by acute stress but also its response to an energy demanding situation as cold exposure; rats subjected to an acute stress or to corticosterone injection prior to cold exposure do not present the expected activation of HPT axis activity, and of a target organ as brown adipose tissue (BAT) (19, 32). The interference of glucocorticoids on neuronal activation of TRH neurons has been demonstrated at the level of Trh transcription in hypothalamic cells in vitro (33, 34); in vivo, corticosterone administration inhibits cold-induced CREB phosphorylation in TRH neurons (34). All these data pertain to male rodents as data on females are scarce.
Although chronic exposure to stress or to corticosterone inhibits PVN Trh expression and serum TSH concentration (31), the response of the HPT axis in chronically stressed animals exposed to energy demands is unknown. There are several models to induce chronic stress in rodents and depending on the type, the response of the HP-adrenal (HPA) axis (CRH:ACTH:glucocorticoids) can either subside (animals habituate) or intensify. Chronic stress induced by daily intermittent short periods of restraint (Res) is a model of psychological stress that produces habituation of the response of the HPA axis, but if rodents are submitted to a new stressor, response is enhanced (35, 36). After 30 min of acute restraint, PVN Trh expression and TSH serum concentration decrease but levels normalize after 14 daily sessions (19, 37). The cold-induced activation of the HPT axis is also curtailed in male rats submitted to 14 days of intermittent repeated restraint (unpublished). Another type of stressor is individual housing; isolation (Iso) constitutes a continuous chronic stress recognized as a strong psychosocial stressor that causes hyperactivation of the HPA axis, and the lack of social stimuli prevent habituation (38); animals isolated since puberty have behavioral and physiological malfunctions, as puberty and adolescence are critical developmental periods for circuit formation between limbic areas (39, 40).
As an adequate response of the HPT axis to exercise favors mobilization of energy reserves (19, 41), we hypothesized that chronic stress attenuates the response of the HPT axis to voluntary exercise and this contributes to long-term consequences in WAT depots. We selected these two paradigms to distinguish the effect of stress on animals that habituated (Res) and no longer have high basal corticosterone levels but may show an exaggerated response to a novel situation as exercise (35–37), compared to isolated animals that do not habituate and show disfunction of HPA axis (42). As an acute corticosterone increase blunts the increase in TRH synthesis induced by cold-induced neuronal stimulation (32–34), we may expect a different response if effects depend only in corticosterone variations or, if they depend on limbic circuitry (43). We studied the response of the HPT and HPA axes (the latter as control) and WAT depots to voluntary wheel running in male and female rats submitted to two types of chronic stress: (a) 2 weeks of intermittent restraint in adult animals, and (b) isolation caused by keeping rats in individual housing since puberty.
Materials and Methods
Animals
Male and female Wistar rats were raised at the Institute's Animal Facility, as described in Sotelo-Rivera et al. (32). Breeding colony management and care was carried out only by two technicians to avoid additional stress. The breeding procedure followed: an outbreed monogamous pair scheme in which the male is removed before the litter is born; litter is adjusted to 10 offspring at post-natal day 2 (PND 2) and culled if necessary. Animals are weaned at PND 21, placed by sex in groups of 2–4/cage and treated as described (44). Appropriate records assure non-inbreeding; colony breeders are renewed every 5 years. For these experiments, rats (PND 45–60) were placed in experimental independent rooms according to sex (2/cage). When males were near 350 g or 2.5 months, they were housed in groups of two per cage, in cages with a wire bar lid modified so that the rear 8.00″ of lid is ~3.00″ higher (total height of 11.00″), allowing rats to stand on their back legs (Y Corporation of America, INC.). Food (Teklad 2018SX, Envigo, USA) and water were offered ad libitum except where indicated otherwise. Maintenance and work with animals followed the Guide for the care and use of laboratory animals (8th ed.), as well as the Mexican norm NOM-062-ZOO-1999. These experiments were approved by the Bioethics Committee of the Institute, approval No. 273 and 318.
Experiment 1. Chronic Intermittent Restraint
Forty adult male rats at PND 86 (390 ± 6 g) were weighed and separated in five groups (eight rats per group). Two groups were taken to an empty room and introduced into a plexiglass tube (23.5 × 7 cm) with slots for ventilation and keeping tail out, in prone position during 30 min every day at 10:00 h for 2 weeks [restrained [Res] group]; two groups serving as experimental controls (C) were taken to a different room and placed in a small cage during 30 min (37). A group of 8 naïve (N) animals from the same cohort was kept undisturbed in the animal room to evaluate environmental conditions effect. Food intake was quantified by weight difference: A set measured amount of food was placed over the rack of the Res and N groups every 3 days, the amount left was weighted again after recovering small pieces from the bed. The mean of Res intake offered to controls (pair-fed).
At PND 101, rats of C and Res groups were divided; half of C (420 ± 13 g) and of Res (429 ± 14 g) animals were introduced every night (7:00 p.m.−7:00 a.m.), during 2 weeks, in a cage with a running wheel of 25 cm diameter (AccuScan Instruments INC) for voluntary exercise (exercised: Ex), the other two halves of animals stayed in individual cages (sedentary: Sed) (19). All rats rejoined their cage mate in the morning. Food was offered during dark or light cycles and intake registered at every food change (between 7:00 a.m. and 9:00 a.m. in the morning and 5:00 p.m.−7:00 p.m. in the night). Sedentary rats were pair-fed to the intake of Ex rats.
The same paradigm was used to study female rats starting at PND 95 (before Res: 248 ± 4 g; after Res, before Ex: 253 ± 3 g).
Experiment 2. Social Isolation
At PND 30 male (N = 32; 110 ± 2 g) and female (N = 32; 100 ± 2 g) Wistar rats were separated in two groups that were housed, either in individual cages (Iso) (N = 16) or in groups of 2/cage (C) in the same room to expose isolated rats to visual, auditive, and olfactory cues of other rats (45). Rats were maintained as described (32), in separate rooms according to sex. Food and water were offered ad libitum, food intake (FI) was quantified every 3 days from PND 30 to PND 63 and body weight measured every week in the adolescence period. At PND 63 C (Males: 379 ± 8 g; Females: 223 ± 6 g) or Iso (Males: 363 ± 7; Females: 224 ± 5 g) rats of each sex were further separated in two groups, one was left undisturbed (Sed) and the other exposed to a running wheel (Ex) (N = 8 each) as described above and in Uribe et al. (19), with some modifications. Due to limited amount of running cages, rats had alternate access during 16 days for a total of 10 running days. At light change (7:00 a.m.), C animals were housed with their cage-mate or in an isolated cage (Iso group); food intake, and number of revolutions were recorded daily and body weight every 2 days. Sedentary rats received the amount of food consumed by the exercised group as described previously.
Sacrifice and Tissue Collection
On the last day of exercise, 3 h after lights on and cage change, animals were decapitated with a sharp guillotine by an experienced technician as described (19), in a separate laboratory near the animal rooms, taking one animal at a time, cleaning the guillotine in-between and with care of diminishing stress. Trunk blood was collected, serum separated and stored in aliquots. Adipose tissues (gonadal, retroperitoneal and interscapular) and adrenals were extracted and weighed in fresh; brain and BAT were placed in dry ice and stored at −70°C. Several experienced persons participated to limit time between initiation and termination of sacrifice to a maximum of 2 h. Dissection of hypothalamic regions is described in Supplementary Figure 1.
Hormone Assays
Radioimmunoassays were utilized for TSH (NIDDK reagents and protocols) and corticosterone (CORT; reagents from Merck-Millipore, PerkinElmer, and Sigma) quantification. Total T3 and T4 were quantified with ELISA kits (Diagnóstica International Zapopan, JAL, México) adding to standard curves an aliquot of hypothyroid rat serum (46). All samples were measured in duplicate and the mean taken as one determination; intraassay and interassay coefficients of variation were <10%.
Semi-quantification of mRNA
Total RNA was extracted from frozen tissues according to Chomzynsky et al. (47) with some modifications for BAT, for which a centrifugation (870 g, 4°C, and 10 min) and an extra chloroform wash were performed to remove fat. Relative mRNA levels were measured by RT-PCR according to reported conditions (19); for those not previously reported by our group, linearity was verified and optimized conditions described in Supplementary Figure 2.
Statistical Analysis
The mean of duplicate biochemical measurements was considered as one determination; results were calculated as percent of each experiment's control group mean. Two-way ANOVA was performed to analyze the effect of chronic stress and sex before exercise, and to analyze the effect of chronic stress and physical activity on the different variables. Three-way ANOVA was performed to analyze effects of chronic stress, physical activity and sex, and interaction between these variables. All data were analyzed by Sigma plot 11.0 (Systat Software, Inc.) and GraphPad Prism 8 (GraphPad Software, Inc.). Results of statistical analysis are described in Supplementary Tables 1, 2. Post-hoc comparisons were carried out with either Bonferroni or Holm-Sidak tests, and difference considered to be significant when P < 0.05.
Results
Experiment 1. Effect of Chronic Restraint Stress on the Response to Voluntary Exercise
Chronic Restraint Decreased Food Intake and Body Weight Gain
During the period of intermittent restraint, there was no difference in the total food intake or body weight gain (BWg) of restrained male rats compared to naïve (Table 1). Res females ate 10% less than the naïve group and as controls were pair fed to Res, they ate all that was offered (Table 1). BWg was lowest in the pair-fed, followed by Res and highest by N. Restraint diminished voluntary food intake, pair-fed were probably stressed by hunger causing highest weight lost. Females ate less absolute food than males (Table 1) but ate more relatively to body weight, thus their food efficiency was lower (Supplementary Table 3A).
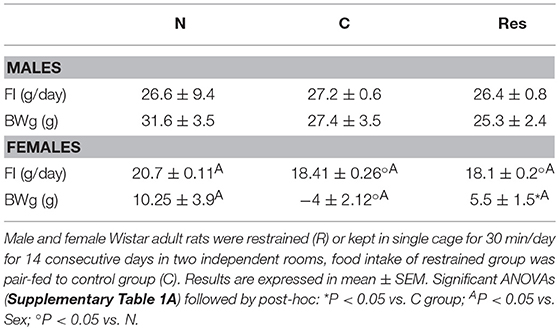
Table 1. Food intake (FI) and body weight gain (BWg) during restraint stress period of naive (N) pair fed controls (C) and restraint (R) male and female rats.
Chronic Restraint Diminished Motivation for Running Only in Males
Res males performed less exercise than controls (Figure 1A) whereas females were not affected as both groups gradually increased the total distance ran which were considerably higher than those ran by males (Figure 1B). As previously published (19), exercise diminished food intake more in C-Ex than in Res-Ex; body weight gain in Sed or Ex, C or Res was 5% lower than N but differences between C or Res groups were non-significant (Table 2). Compared to N, exercise decreased food intake in C or Res females but BWg was not significantly different (Table 2 and Supplementary Table 4A).
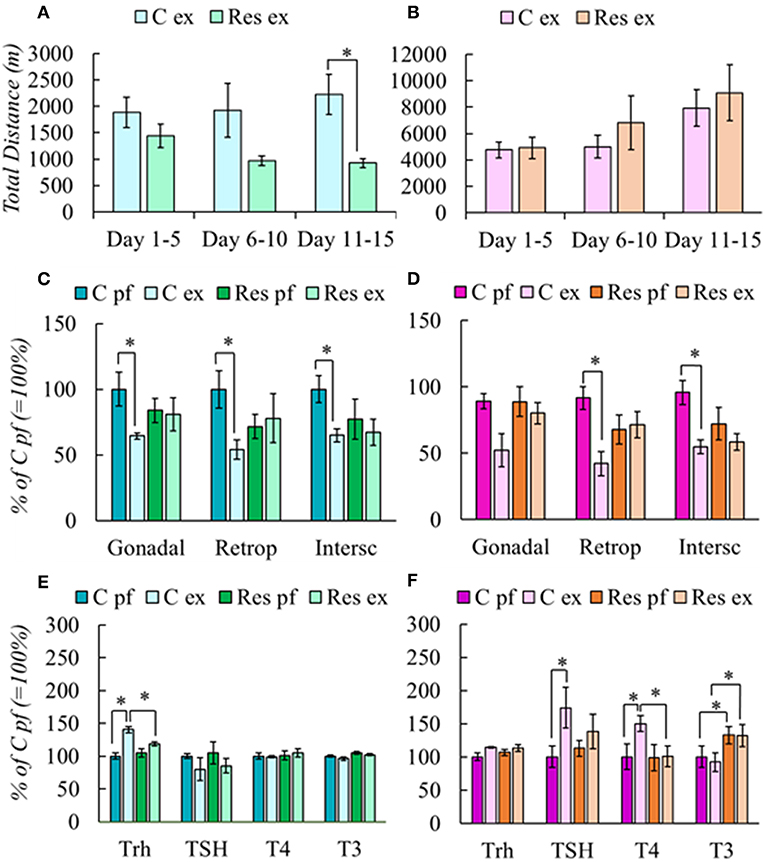
Figure 1. Effect of chronic restraint on physical activity (A,B), fat mass (C,D), and HPT axis response (E,F) of male and female rats. Stress protocol and exercise conditions as described in Tables 1, 2. respectively. Physical activity was expressed in total distance in meters at periods stated in the abscissa. Results are expressed in percent of mean values of C pf. Significant ANOVAs (Supplementary Table 2A), followed by post-hoc: *P < 0.05.
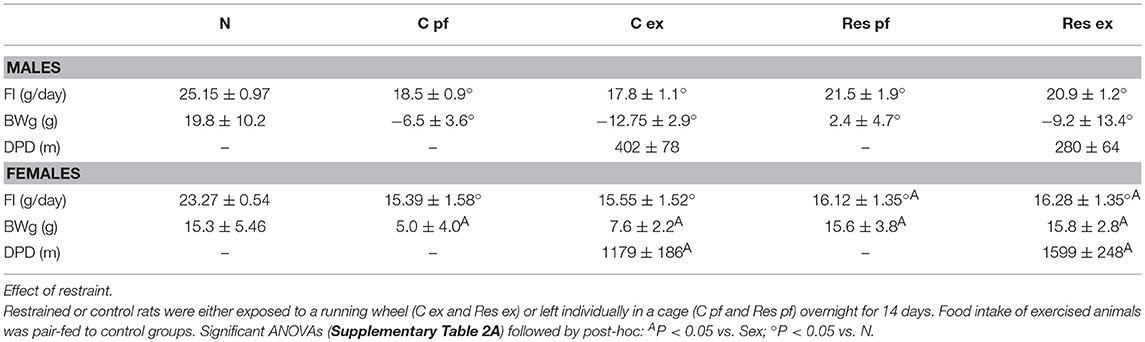
Table 2. Food intake (FI), Body weight gain (BWg) and distance per day (DPD) after exercise period of male and female rats.
Chronic Restraint Blunted Exercise-Induced Loss of Fat Mass and Activation of HPT Axis in a Sex-Dependent Manner
Exercise induces WAT mass loss even if it is not evident in body weight (19, 48). C males reduced gonadal, retroperitoneal, and interscapular fat depots after exercise, but Res group did not (Figure 1C). C-Ex females also reduced them, although gonadal WAT loss was not significant; as in males, Res females did not lose WAT after exercise (Figure 1D).
Because chronic stress affects the HPA response to new stressors and exercise has been considered either to increase or diminish HPA activity (19, 49), we measured gene expression profile of Crh and Gr in PVN, and corticosterone in serum. There were no significant differences in any of these variables in groups of either sex (not shown), except for an increase in right-adrenal weight of Res males (N, 27 ±3; C-Ex 31 ± 1; Res 31 ± 3; Res-Ex 39 ± 2*x mg, *P = 0.007 vs. N; xP = 0.02 vs. C-Ex) suggesting chronic stress (50). Females had higher basal corticosterone serum concentration (181 ± 7 ng/ml) than males (65 ± 4 ng/ml); but in contrast, values were increased similarly in all experimental groups compared to naïve (C-Sed 396 ± 56, C-ex 332 ± 19, Res-Sed 354 ± 30, Res-Ex 303 ± 16).
Trh expression was stimulated in C-Ex (Figure 1E) and values correlated negatively with WAT retroperitoneal mass (r = −0.832; P = 0.003). No other parameters of HPT axis varied among male groups. In females, Trh expression was not significantly different between C-Ex and C-Sed or between Res groups. In contrast to males, TSH serum concentration increased in C-Ex females as well as T4 (Figure 1F). In both sexes, activation of the HPT axis coincides with WAT mass decrease only in controls, but not in those previously stressed by daily restraint.
Experiment 2. Effect of Individual Housing on the Response to Voluntary Exercise
Social Isolation Did Not Affect Food Intake and Body Weight Gain During Adolescence
We chose to analyze social isolation since adolescence because this stressor prevents proper development of brain, causing behavioral and physiological alterations that can remain until adult stage (43, 51, 52). Social isolation in adulthood delays exercised-induced hippocampal neurogenesis in male and female rats, affecting more in males than in females (47, 53).
Rats were isolated at PND 30, as expected males grew faster than females; no difference was observed in body weight gain between C or Iso groups; by PND 60, body weight of C or Iso groups were similar so as their food intake (Table 3 and Supplementary Table 3B). The lack of effect of social isolation since weaning on ponderal variables during the growing phase has been previously reported (54, 55).
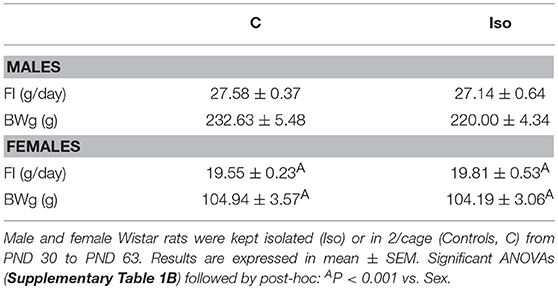
Table 3. Food intake (FI) and body weight gain (BWg) during adolescence-adulthood period of group-housed (C) and isolated (Iso) male and female rats.
Ponderal Changes and HPA Responses to Exercise Were Affected by Social Isolation Dependent on Sex
Exercise reduced their food intake 19% in C and 18% in Iso groups compared to average intake before exercise in both sexes (Tables 3, 4), but Iso-Ex ate more than exercised controls (Table 4), sedentary group were pair-fed accordingly.
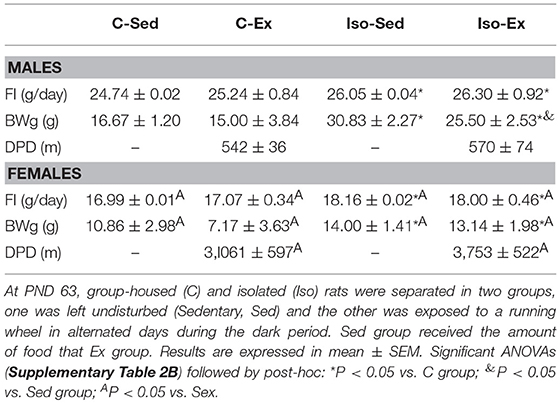
Table 4. Food intake (FI), body weight gain (BWg) and distance per day (DPD) after exercise period of group-housed (C) and isolated (Iso) male and female rats.
After exercise, Iso gained more weight than controls (Table 4). If food intake was calculated in relation to body weight, females ate more and their food efficiency was lower than males (Supplementary Table 4B). Isolation did not diminish the amount of running in males or females compared to group-housed controls (Figures 2A,B), and as in the previous experiments, females ran more than males (Table 4). Total distance ran by C males in this experiment was similar to controls of experiment 1, whereas females ran more, even though they had access to the wheel only in alternate days for a total of 10 nights, instead of 14 daily nights in the previous experiment (Supplementary Figure 3); probably be due to the lighter weight of the latter (56), and/or the form of exercise, on alternate days in Iso that avoided habituation (25) compared to every day in Res.
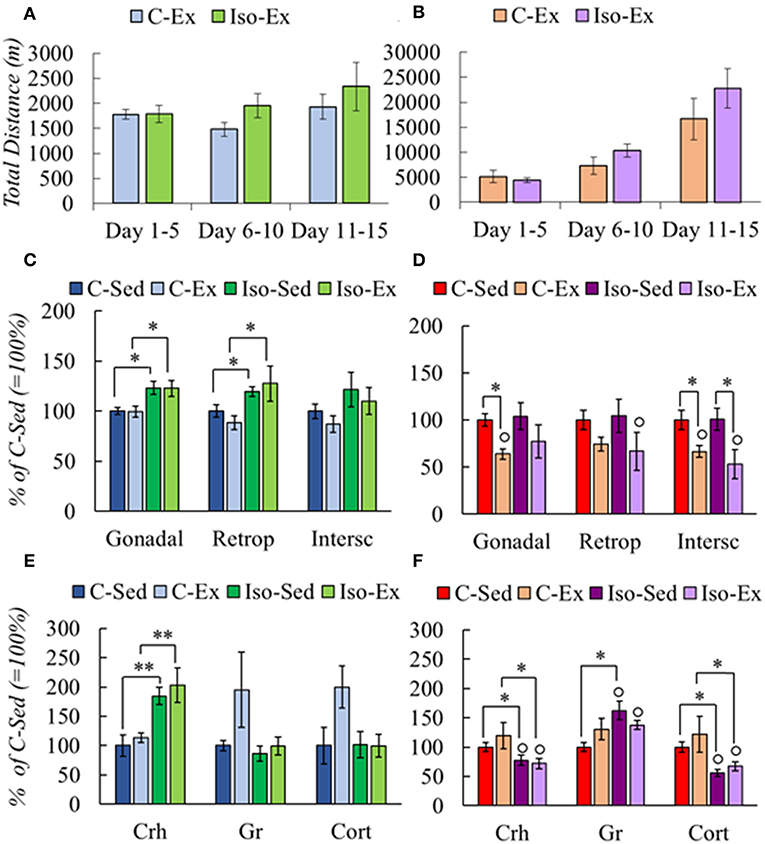
Figure 2. Effect of social isolation on physical activity (A,B), fat mass (C,D), and HPA axis response (E,F) of male and female rats. Rearing and exercise conditions described in tables 3 and 4, respectively. Significant ANOVAs (Supplementary Table 2B) followed by post-hoc: *P < 0.05; **P < 0.001; °P < 0.05 vs. Males of the same group.
C males had less gonadal and retroperitoneal WAT mass compared with Iso group, but there was no lost of any fat type by exercised (Figure 2C). C-Ex females lost gonadal and subcutaneous WAT mass albeit only subcutaneous in the Iso-Ex group (Figure 2D).
HPA response differed between groups. Isolation increased PVN Crh expression whether or not they exercised; there was a tendency to increase PVN Gr expression and Cort serum concentration in C-Ex but did not achieve significance (Figure 2E). Females in contrast, had lower PVN Crh expression and serum Cort concentration in Iso than C groups. PVN Gr mRNA levels increased although significant only in Iso-Sed (Figure 2F). Higher expression of Gr may contribute to overcome the reduced levels of corticosterone to lower Crh expression (57).
Social Isolation Altered the HPT Response to Voluntary Exercise in a Sex-Dependent Manner
Two important regions that regulate HPT axis activity reside in mediobasal hypothalamus (MBH), the arcuate nucleus and the median eminence. In the arcuate nucleus, neuronal populations that express anorexigenic (POMC/CART) and orexigenic (NPY/AgRP) peptides stimulate or inhibit Trh expression in PVN, respectively (10). Tanycytes in median eminence inhibit TRH expression through deiodinase 2 activity increasing T3 levels in the hypothalamus, and the activity of TRH-degrading enzyme controls TSH secretion (58). In the MBH, the expression of arcuate peptides varied due to rearing condition or exercise. Pomc expression increased in C-Ex males compared with C-Sed and Iso-Ex, and Npy expression decreased in Iso (Figure 3A). The pattern of Pomc expression was similar in female MBH, however Npy mRNA levels were higher in Iso than C groups (Figure 3B). Dio2 expression was higher in C-Ex than C-sed in males but Trhde was not modified (Figure 3A). In females, Dio2 was higher in C than in Iso; Trhde increased by exercise in C and slightly in Iso (Figure 3B).
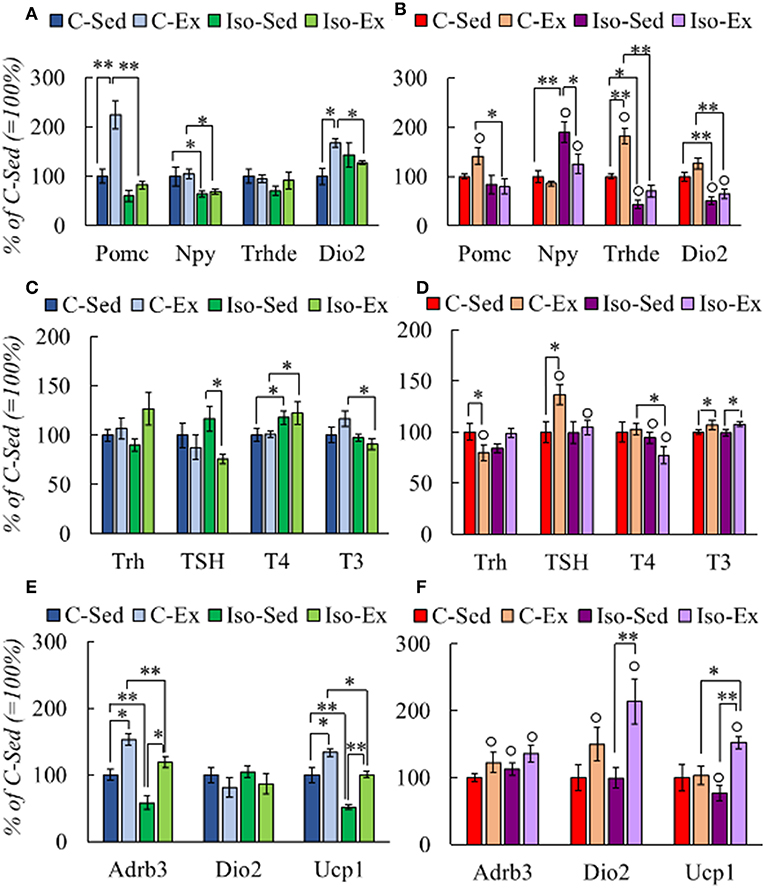
Figure 3. Effect of social isolation and alternate exercise on the expression of HPT status-related genes (A,B), HPT axis response (C,D), and genes that response to thyroid hormones in BAT (E,F) of male and female rats. Rearing and exercise conditions described in tables 3 and 4, respectively. Results are expressed in percent of mean values of C-Sed. Significant ANOVAs (Supplementary Table 2B) followed by post-hoc: *P < 0.05; **P < 0.001; °P < 0.05 vs. Males of the same group.
HPT axis was not stimulated by exercise in C-males. In contrast, isolated males had diminished TSH serum concentration after exercise; that of T4 augmented but in both Iso groups compared to their controls (Figure 3C). Negative correlation was observed between T4 and WAT retroperitoneal mass in C-Ex group (r = −0.856; P = 0.030) whereas positive, in Iso-Ex (r = 0.879; P = 0.009). In females, Trh mRNA levels were lower in C-Ex than in C-Sed, TSH serum concentration increased by exercise in C, and T4 decreased compared to Iso-sed, while T3 concentration increase in both exercised groups (Figure 3D). T4:T3 ratio was higher in Iso than C, and was higher in females than males (Supplementary Table 5).
Brown adipose tissue (BAT) is a target of thyroid hormones that in concert with the adrenergic system is responsible for thermogenesis, activity that contributes to energy expenditure (19, 59). Noradrenergic signaling stimulates Dio2 expression, rising T3 concentration in the tissue (60) and, in turn, stimulates the transcription of β-adrenergic receptors (β-AR) and UCP-1 (2, 61). We thus measured the expression of these molecules; mRNA levels of Adrb3 or Ucp1 were lower in Iso groups of males than C but increased in both by exercise, those of Dio2 were not changed (Figure 3E). Females showed no changes in Adrb3 expression, decreased that of Ucp1 in Iso-Sed compared to C-Sed while increased, as Dio2, in Iso-Ex (Figure 3F).
Discussion
Life of laboratory rodents, in controlled environments, is very distant from that in natural conditions. Experimental rats have little stimuli and space for physical activity, and usually become obese (62). Although distinct from training exercise, voluntary running for short bouts provides many benefits for animals' health (63), and allows comparing the physiology of a sedentary animal with an active one. In just 2 weeks of daily running during their active phase, male rats lose WAT mass proportionally to their physical activity and to the levels of serum thyroid hormones or PVN Trh expression (19). Stress inhibits the response of the HPT axis to the short-term energy needs of an acute exposure to cold [(32, 34) and unpublished], but its effect on long-term energy demands was not known. We considered voluntary running a suitable model of energy demand, to study the effects of two types of chronic stress on the responses of the HPT axis to exercise in both male and female rats, given the sex dimorphism in metabolic and stress responses. A short time of exercise was selected to avoid allostatic adjustments that could make it difficult to validate results with previously reported results (19).
The chronic stressors chosen were intermittent restraint at adulthood, and isolation from puberty to adulthood. Restraint is a well-studied model of psychological homotypic stress that causes habituation (35) as well as neurological changes that cause a hyper-reactive response to a new stressor, persistence of oxidative stress, modifications in immune responses, to name a few (64). In contrast, social isolation is a model of psychosocial stress that alters glucocorticoid feedback through changes in neurochemical functions of limbic and cortical systems, affecting the maturation of some of these circuits leading to long lasting alterations that induce depression and anxiety in adult rodents (38, 65, 66); isolation during adolescence produces HPA axis malfunction at adult stage, decreasing basal levels of serum corticosterone and producing a hyper-reactive response to acute stress (67–69). Voluntary exercise attenuates various deleterious effects produced by stress, and although it may cause increases in serum corticosterone concentration it is not considered a strong stressor, in contrast to forced training or exhaustive exercise (70, 71). The exercise paradigm utilized was not considered as a new stressor since variables of HPA axis were not different in exercised to those of sedentary rats. In our experiments, both types of stress affected feeding and exercise task; Res males exercised less and ate more, whereas Iso animals, especially females, increased food intake and body weight gain, suggesting stress-induced imbalances in eating behavior. Decreased fat mass was expected in exercised rats (19, 72, 73), and was detected in adult males and females that exercised every night (experiment 1). However, animals that exercised alternately (experiment 2) did not reduce food intake as did those running every night (this experiment, and 19). In males, lipolysis induced by daily exercise appeared to reduce hunger; signals required for this and for loosing WAT mass might not be enough if exercise is not done daily. Conversely, female controls of both experiments did loose WAT mass in response to voluntary exercise but also ran considerably more than males, as is generally recognized in rodents (74, 75). They lost subcutaneous WAT (interscapular) and only one of the abdominal types (gonadal or retroperitoneal) of WAT depending on the experiment. Previous Res-stress reduced the amount of exercise performed only in males that showed no WAT mass loss; less exercise may account for the lack of fat mass lost however, stressed females that ran the same amount as controls did not lose WAT mass either, despite not differing in food intake. In isolated females, exercise induced similar tendencies in WAT mass reduction, which was significant only in subcutaneous type. These results point for a negative effect of chronic stress on the adequate WAT-metabolic response to exercise.
Voluntary and forced exercise increase basal corticosterone serum concentration (47, 69, 76) without affecting the expression of Crh and Gr in PVN (77, 78). Adult control animals of both experiments showed no exercise-induced variations in any of the HPA axis parameters, and neither those that had been previously restrained. Isolation induced HPA malfunction differently in males and females; males had increased PVN Crh expression but no changes in serum corticosterone concentration, whereas females had decreased serum corticosterone concentration and Crh mRNA levels, suggesting hypoactivity of the axis (79). These findings agree with a higher susceptibility of females to stress (80), isolation (81) and depression (82, 83), the latter usually coincident with hypoactivity of HPA (79); this might be due to the effect of stress at adolescent stage (84), and exercise could reverse the deleterious effects of stress (53, 71).
Exercise promotes the expression of Pomc in arcuate nucleus (ARC) (85), in agreement with what we found in C male and female rats, albeit it was not according to the amount of exercise performed, as Iso males ran similar distances and their response was blunted. Isolation and not wheel running increased ARC Npy expression in females, coincident with higher food intake and some reports (86, 87).
The HPT axis is subject to multiple metabolic, hormonal and neuronal effectors that regulate its activity at multiple levels (8, 11, 56). Elements of the HPT axis showed a sex-dimorphic regulation (Figure 4). Change-dynamics of HPT axis parameters depend on the type of stimulus; PVN Trh expression changes can be rapid and transient (17, 18), half-lives of serum TSH and T3 are short (min to h) compared to that of serum T4 (8). Furthermore, since the activity of tissue deiodinases is also regulated, circulating thyroid hormones (TH) concentrations are difficult to interpret (12), making it necessary to evaluate as many variables as possible, if only one time point is studied. Exercise increased Dio2 expression in median eminence (ME) in C males, possibly because of increased serum corticosterone concentration (88). If DIO2 activity was indeed increased, it should have augmented local T3 concentration which in turn, would be expected to stimulate ME Trhde expression (58) or inhibit PVN Trh expression (10), albeit with unknown dynamics. None of these changes were found in males; since animals were sacrificed 3 h after switching to a wheel-free cage, and time since the last exercise boot was not recorded, it is possible that the time-point was inadequate. However, exercise did increase ME Trhde expression in C females, with only a slight non-significant tendency for Dio2 expression, and diminished PVN Trh expression. In females, isolation decreased ME Dio2 and Trhde expression, coincident only with decreased corticosterone serum concentration, which stimulates Dio2 expression (88); parallel changes in Dio2 and Trhde reflect the dependence of DIO2 activity to set the levels of T3 in tanycytes, which activate Trhde expression (58). However, despite increased Trhde expression in ME, TSH serum concentration was increased, most probably by an exercise-induced effect that could either increase TRH release in spite of not finding a higher expression or, increased TSH release due to higher expression of TRH-R1 or in response to events distinct from TRH.
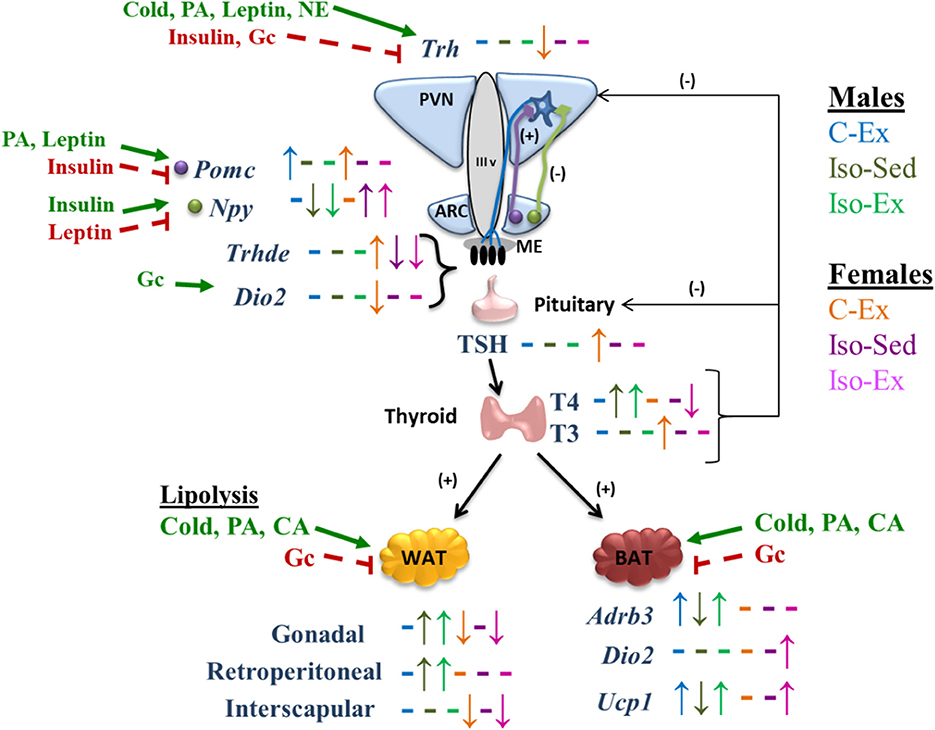
Figure 4. Schematic representation of the effect of social isolation and alternate voluntary exercise on HPT axis response, its regulation, and its effects in WAT and BAT. PA, physical activity; NE, norepinephrine; Gc, glucocorticoids; CA, catecholamines. Up and down arrows indicate changes and dashes indicate not changes of each experimental group compared with C-Sed group of each sex. Bold green arrows indicate activation and dashed red lines indicate inhibition.
Thyroid hormones regulate the activity of adipose tissue genes which constitute additional markers of HPT axis activity. BAT can promote energy expenditure through facultative thermogenesis induced by sympathetic nervous system activity (89). The impact of exercise on BAT is still not well-understood (90) since Adrb3 and Ucp1 regulation in BAT by exercise depends on species and training time; in mice, treadmill training increases Ucp1 expression but Adrb3 expression does not change (91), while in rat Ucp1 and Adbr3 mRNA levels do not change after one session of treadmill exercise (92), in contrast to an increase during progressive treadmill (93). The increase in the expression of Adrb3 and Ucp1 in Iso-Ex males, and of Dio2 and Ucp1 in Iso-Ex females, suggests that voluntary exercise promotes the effect of thyroid hormones on BAT, promoting energy expenditure as heat instead of muscle work, and being able to revert the effects of social isolation.
To conclude, present results demonstrate that a history of chronic stress curtails the adequate metabolic response to voluntary exercise characterized by loss of WAT depots, in male and female rats. Females ran much more than males, which allowed them to lose fat independent of schedule (daily or alternate days). Restraint stress reduced exercise in males, either due to fatigue or lack of motivation. Some changes in the HPT axis (PVN Trh expression or serum concentrations of TSH or TH) were related to WAT loss, but only in controls and not in stressed groups. We propose that in control animals the HPT axis is activated transiently at each exercise boot contributing to lipolysis, event inhibited by previous chronic stress, leading to problems with fuel availability.
Data Availability
The raw data generated are available on request to corresponding author.
Ethics Statement
The experiments were approved by the Bioethics Committee of the Institute, approval No. 273 and 318.
Author Contributions
MP-M, MG-M, and MS-J performed the experiments. MP-M, LJ-H, and PJ-B analyzed the data. MP-M and PJ-B wrote the manuscript draft. LJ-H and J-LC helped with manuscript edition. MP-M is a doctorate student and main contributor. PJ-B is principal investigator of grant and project director. J-LC and PJ-B are co-directors of the Neuroendocrinology group.
Funding
This work was supported by DGAPA IN204316 (PJ-B) and IA200417 (LJ-H); CONACYT 0284883 (PJ-B). MP-M is recipient of CONACYT 273496 post-graduate scholarship.
Conflict of Interest Statement
The authors declare that the research was conducted in the absence of any commercial or financial relationships that could be construed as a potential conflict of interest.
Acknowledgments
Authors express their gratitude to technicians QFB. Miguel Cisneros and Q. Fidelia Romero for their aid in experimental work, and to Roberto Rodríguez Bahena, Shirley Ainsworth, David Santiago Castañeda, and Eugenio López Bustos for providing reagents and computer assistance.
Supplementary Material
The Supplementary Material for this article can be found online at: https://www.frontiersin.org/articles/10.3389/fendo.2019.00418/full#supplementary-material
References
1. McAninch EA, Bianco A. Thyroid hormone signaling in energy homeostasis and energy metabolism. Ann N Y Acad Sci. (2014) 1311:77–87. doi: 10.1111/nyas.12374
2. Mullur R, Liu Y-Y, Brent GA. Thyroid hormone regulation of metabolism. Physiol Rev. (2014) 94:355–82. doi: 10.1152/physrev.00030.2013
3. Gonçalvez A, Resende ES, Fernandes MLMP, da Costa AM. Effect of thyroid hormones on cardiovascular and muscle systems on exercise tolerance: a brief review. Arq Bras Cardiol. (2006) 86:e42–4. doi: 10.1590/s0066-782x2006001600033
4. Louwerens M, Appelhof BC, Verloop H, Medici M, Peeters RP, Visser TJ, et al. Fatigue and fatigue-related symptoms in patients treated for different causes of hypothyroidism. Eur J Endocrinol. (2012) 167:809–15. doi: 10.1530/EJE-12-0501
5. Lankhaar JAC, de Vries WR, Jansen JACG, Zelissen PMJ, Backx FJG. Impact of overt and subclinical hypothyroidism on exercise tolerance: a systematic review. Res Q Exer Sport. (2014) 85:365–89. doi: 10.1080/02701367.2014.930405
6. Tanriverdi A, Ozcan Kahraman B, Ozsoy I, Bayraktar F, Ozgen Saydam B, Acar S, et al. Physical activity in women with subclinical hypothyroidism. J Endocrinol Inves. (2018) 1–7. doi: 10.1007/s40618-018-0981-2
7. Mendoza A, Hollenberg AN. New insight into thyroid hormone action. Pharmacol Ther. (2017) 173:135–45. doi: 10.1016/j.pharmthera.2017.02.012
8. Chatzitomaris A, Hoermann R, Midgley JE, Hering S, Urban A, Dietrich B, et al. Thyroid allostasis-adaptative responses of thyrotropic feedback control to conditions of strain, stress, and developmental programming. Front Endocrinol. (2017) 8:1–28. doi: 10.3389/fendo.2017.00163
9. Visser WE, Friesema EC, Visser TJ. Minireview: thyroid hormone transporters: the knowns and the unknowns. Mol Endocrinol. (2011) 25:1–14. doi: 10.1210/me.2010-0095
10. Fekete C, Lechan RM. Central regulation of hypothalamic-pituitary-thyroid axis under physiological and pathophysiological conditions. Endocr Rev. (2014) 35:159–94. doi: 10.1210/er.2013-1087
11. Joseph-Bravo P, Jaimes-Hoy L, Charli J-L. Advances in TRH signaling. Rev Endocr Metab Disord. (2016) 17:545–58. doi: 10.1007/s11154-016-9375-y
12. Bianco AC, Kim BW. Deiodinases: implications of the local control of thyroid hormone action. J Clin Invest. (2006) 116:2571–9. doi: 10.1172/JCI29812
13. Gereben B, Zavacki AM, Ribich S, Kim BW, Huang SA, Simonides WS, et al. Cellular and molecular basis of deiodinase-regulated thyroid hormone signaling. Endocr Rev. (2008) 29:898–938. doi: 10.1210/er.2008-0019
14. Dyess EM, Segerson TP, Liposits Z, Paull WK, Kaplan MM, Wu P, et al. Triiodothyronine exerts direct cell-specific regulation of thyrotropin-releasing hormone gene expression in the hypothalamic paraventricular nucleus. Endocrinology. (1988) 123:2291–7. doi: 10.1210/endo-123-5-2291
15. Chiamolera MI, Wondisford FE. Thyrotropin-releasing hormone and the thyroid hormone feedback mechanism. Endocrinology. (2009) 150:1091–6. doi: 10.1210/en.2008-1795
16. Fliers E, Kalsbeek A, Boelen A. Mechanisms in endocrinology: beyond the fixed setpoint of the hypothalamus–pituitary–thyroid axis. Eur J Endocrinol. (2014) 171: R197–208. doi: 10.1530/EJE-14-0285
17. Zoeller RT, Kabber N, Albers E. Cold exposure elevates cellular levels of messenger ribonucleic acid encoding thryrotripin-releasing hormone in paraventricular nucleus despite elevated levels of thyroid hormones. Endocrinology. (1990) 127:2955–62. doi: 10.1210/endo-127-6-2955
18. Uribe RM, Redondo JL, Charli JL, Joseph-Bravo P. Suckling and cold stress rapdly and transiently increase TRH mRNA in the paraventricular nucleus. Neuroendocrinology. (1993) 58:140–45. doi: 10.1159/000126523
19. Uribe RM, Jaimes-Hoy L, Ramírez-Martínez C, García-Vázquez A, Romero F, Cisneros M, et al. Voluntary exercise adapts the hypothalamus-pituitary-thyroid axis in male rats. Endocrinology. (2014) 155:2020–30. doi: 10.1210/en.2013-1724
20. Arkader R, Rosa MR, Moretti G. Physiological changes of exercise of thermogenesis, thyroid homeostasis and inflammation. Endocrinol Metab Int J. (2016) 3:85–8. doi: 10.15406/emij.2016.03.00055
21. Fortunato RS, Ignácio DL, Padron AS, Peçanha R, Marassi M P, Rosenthal D, et al. The effect of acute exercise session on thyroid hormone economy in rats. J Endocr. (2008) 198:347–53. doi: 10.1677/JOE-08-0174
22. Hackney AC, Dodbridge JD. Thyroid hormone and the interrelationship of cortisol and prolactin: influence of prolonged, exhaustive exercise. Pol J Endocrinol. (2009) 60:252–7.
23. Leal LG, Lopes MA, Batista ML Jr. Physical exercise-induced myokines and muscle-adipose tissue crosstalk: a review of current knowledge and the implications for health and metabolic diseases. Front Physiol. (2018) 9:1–17. doi: 10.3389/fphys.2018.01307
24. Afonso VM, Eikelboom R. Relationship between wheel running, feeding, drinking, and body weight in male rats. Physiol Behav. (2003) 80:19–26. doi: 10.1016/S0031-9384(03)00216-6
25. Basso JC, Morrell JI. Using wheel availability to shape running behavior of the rats towards improved behavioral and neurobiological outcomes. J Neurosci Methods. (2017) 290:13–23. doi: 10.1016/j.jneumeth.2017.07.009
26. Joseph-Bravo P, Jaimes-Hoy L, Uribe RM, Charli JL. TRH, the first hypophysiotropic releasing hormone isolated: control of the pituitary-thyroid axis. J Endocrinol. (2015) 226:T85–100. doi: 10.1530/JOE-15-0124
27. Olivares EL, Silva-Almeida C, Pestana FM, Sonoda-Cortes R, Araujo IG, Rodrigue NC, et al. Social stress-induced hypothyroidism is attenuated by antidepressant treatment in rats. Neuropharmacology. (2012) 62:446–56. doi: 10.1016/j.neuropharm.2011.08.035
28. Joseph-Bravo P, Jaimes-Hoy L, Charli JL. Regulation of TRH neurons and energy homeostasis-related signals under stress. J Endocrinol. (2015) 224:R139–59. doi: 10.1530/JOE-14-0593
29. Moog NK, Entringer S, Heim C, Wadhwa PD, Kathmann N, Buss C. Influence of maternal thyroid hormones during gestation on fetal brain development. Neuroscience. (2017) 342:68–100. doi: 10.1016/j.neuroscience.2015.09.070
30. Bianco AC, Nunes MT, Hell NS, Maciel RMB. The role of glucocorticoids in the stress-induced reduction of extrathyroidal 3,5,3'-triiodothyronine generation in rats. Endocrinology. (1987) 120:1033–8. doi: 10.1210/endo-120-3-1033
31. Kakucksa I, Qi Y, Lechan RM. Changes in adrenal status affect hypothalamic thyrotropin-releasing hormone gene expression in parallel with corticotropin-releasing hormone. Endocrinology. (1995) 136:2795–802. doi: 10.1210/endo.136.7.7789304
32. Sotelo-Rivera I, Jaimes-Hoy L, Cote-Vélez A, Espinoza-Ayala C, Charli J-L, Joseph-Bravo P. An acute injection of corticosterone increases thyrotrophin-releasing hormone in paraventricular nucleus of the hypothalamus but interferes with the rapid hypothalamus pituitary thyroid axis response to cold in male rats. J Endocr. (2014) 26:882–90. doi: 10.1111/jne.12224
33. Díaz-Gallardo MY, Cote-Vélez A, Charli JL, Joseph-Bravo P. A rapid interference between glucocorticoids and cAMP-activated signaling in hypothalamic neurons prevents binding of phosphorylated cAMP response element binding protein and glucocorticoid receptor at the CRE-like and composite GRE sites of thyrotropin-releasing hormone gene promoter. J Neuroendocrinol. (2010) 22:282–93. doi: 10.1111/j.1365-2826.2010.01966.x
34. Sotelo-Rivera I, Cote-Vélez A, Uribe RM, Charli JL, Joseph-Bravo P. Glucocorticoids curtail stimuli-induced CREB phosphorylation in TRH neurons through interaction of the glucocorticoid receptor with the catalytic subunit of protein kinase A. Endocrine. (2017) 55:861–71. doi: 10.1007/s12020-016-1223-z
35. Bhatnagar S, Dallman M. Neuroanatomical basis for facilitation of hypothalamic-pituitary-adrenal responses to a novel stressor after chronic stress. Neuroscience. (1998) 84:1025–39. doi: 10.1016/S0306-4522(97)00577-0
36. Herman JP, Tasker JG. Paraventricular hypothalamic mechanism of chronic stress adaptation. Front Endocrinol. (2014) 7:1–10. doi: 10.3389/fendo.2016.00137
37. Gutiérrez-Mariscal M, Sánchez E, García-Vázquez A, Rebolledo-Solleiro D, Charli JL, Joseph-Bravo P. Acute response of hypophysiotropic thyrotropin releasing hormone neurons and thyrotropin release to behavioral paradigms producing varying intensities of stress and physical activity. Regul Pept. (2012) 179:61–70. doi: 10.1016/j.regpep.2012.08.010
38. Filipović D, Todorovic N, Bernardi RE, Gass P. Oxidative and nitrosative stress pathways in the brain of socially isolated adult male rats demonstrating depressive- and anxiety-like symptoms. Brain Struc Funct. (2017) 222:1–20. doi: 10.1007/s00429-016-1218-9
39. Romeo RD, Patel R, Pham L, So VM. Adolescence and the ontogeny of the hormonal stress response in male and female rats and mice. Neurosci Biobehav Rev. (2016) 70:206–16. doi: 10.1016/j.neubiorev.2016.05.020
40. Burke AR, McCormick CM, Pellis SM, Lukkes JL. Impact of adolescent social experiences on behavior and neural circuits implicated in mental illnesses. Neurosci Biobehav Rev. (2017) 76:280–300. doi: 10.1016/j.neubiorev.2017.01.018
41. Ciloglu F, Peker I, Pehlivan A, Karacabey K, Ilan N, Saygin O, et al. Exercise intensity and its effects on thyroid hormones. Neuroendocrinol Lett. (2005) 26:830–4.
42. McCormick CM, Green MR, Simone JJ. Translational relevance of rodents models of hypothalamic-pitutitary-adrenal function and stressors in adolescence. Neurobiol Stress. (2017) 6:31–43. doi: 10.1016/j.ynstr.2016.08.003
43. Radley JJ, Sawchenco PE. Evidence of involvement of a limbic paraventricular hypothalamic inhibitory network in hypothalamic-pituitary-adrenal axis adaptations to repeated stress. J Comp Neurol. (2015) 523:2769–87. doi: 10.1002/cne.23815
44. Jaimes-Hoy L, Gutiérrez-Mariscal M, Vargas Y, Pérez-Maldonado A, Romero F, Sánchez-Jaramillo E, et al. Neonatal maternal separation alters, in a sex-specific manner, the expression of TRH, TRH-degrading ectoenzyme in the rat hypothalamus, and the response of the thyroid axis to starvation. Endocrinology. (2016) 157:3253–5. doi: 10.1210/en.2016-1239
45. Fone KCF, Porkess MV. Behavioral and neurochemical effects of post-weaning social isolation in rodents-Relevance to developmental neuropsychiatric disorders. Neurosci Behav Rev. (2008) 32:1087–102. doi: 10.1016/j.neubiorev.2008.03.003
46. Bianco AC, Anderson G, Forrest D, Galton VA, Gereben V, Kim BW, et al. American thyroid association guide to investigating thyroid hormone economy and action in rodent and cell models. Thyroid. (2014) 24:88–168. doi: 10.1089/thy.2013.0109
47. Chomzynsky P, Sacchi N. Single-step method of RNA isolation by acid guanidinium thiocyanate-phenol-chloroform extraction. Anal Biochem. (1987) 162:156–9. doi: 10.1006/abio.1987.9999
48. Horowitz JF. Fatty acid movilization from adipose tissue during exercise. Trends Endocrinol Metab. (2003) 14:386–92. doi: 10.1016/S1043-2760(03)00143-7
49. Stranahan AM, Khalil D, Gould E. Social isolation delays the positive effects of running on adult neurogenesis. Nat Neurosci. (2006) 9:526–33. doi: 10.1038/nn1668
50. Ulrich-Lai YM, Figueiredo HF, Ostrander MM, Choi DC, Engelan WC, Herman JP. Chronic stress induced adrenal hyperplasia and hypertrophy in a subregion-specific manner. Am JPhysiol Endocr Metab. (2006) 291:E965–73. doi: 10.1152/ajpendo.00070.2006
51. Lukkes JD, Watt MJ, Lowry CA, Forster GL. Consequences of post-weaning social isolation on anxiety behavior and related neural circuits in rodents. Front Behav Neurosci. (2009) 3:1–12. doi: 10.3389/neuro.08.018.2009
52. Arakawa H. Ethological approach to social isolation effects in behavioral studies of laboratory rodents. Behav Brain Res. (2018) 341:98–108. doi: 10.1016/j.bbr.2017.12.022
53. Leasure JL, Decker L. Social isolation prevents exercise-induced proliferation of hippocampal progenitor cells in female rats. Hippocampus. (2009) 19:907–12. doi: 10.1002/hipo.20563
54. Krolow R, Noschang C, Arcego DM, Huffell AP, Marcolin ML, Benitz AN, et al. Sex-specific effects of isolation stress and consumption of palatable diet during the prepubertal period on metabolic parameters. Metabolism. (2013) 62:1268–78. doi: 10.1016/j.metabol.2013.04.009
55. Arcego DM, Krolow R, Lampert C, Noschang C, Ferreira AGK, Scherer E, et al. Isolation during the pubertal period associated with chronic access to palatable diets: effects on plasma lipids profile and liver oxidative stress. Physiol Behav. (2014) 124:23–32. doi: 10.1016/j.physbeh.2013.10.029
56. Soch A, Bradburn S, Sominsky L, De Luca SN, Murgatroyd C, Spencer SJ. Effects of exercise on adolescent and adult hypothalamic and hippocampal neuroinflammation. Hippocampus. (2016) 26:1435–46. doi: 10.1002/hipo.22620
57. Jeanneteau FD, Lambert WM, Ismaili N, Bath KG, Lee FS, Garabedian MJ, et al. BDNF and glucocorticoids regulate corticotrophin releasing hormone (CRH) homeostasis in the hypothalamus. Proc Natl Acad Sci USA. (2012) 109:1305–10. doi: 10.1073/pnas.1114122109
58. Sánchez E, Vargas MA, Singru PF, Pascual I, Romero F, Fekete C, et al. Tanycyte pyroglutamyl peptidase II contributes to regulation of the hypothalamic-pituitary-thyroid axis through glial-axonal associations in the median eminence. Endocrinology. (2009) 150:2283–91. doi: 10.1210/en.2008-1643
59. Sánchez-Delgado G, Martínez-Téllez B, Olza J, Aguilera CM, Gil A, Ruiz JR. Role of exercise in the activation of brown adipose tissue. Ann Nutr Metab. (2015) 67:21–32. doi: 10.1159/000437173
60. Arrojo e Drigo R, Fonseca TL, Werneck-de-Castro JPS, Bianco AC. Role of the type 2 iodothyronine deiodinase (D2) in the control of thyroid hormone signaling. Biochim Biophys Acta Gen Subj. (2013) 1083:3956–64. doi: 10.1016/j.bbagen.2012.08.019
61. Rubio A, Raasmaja A, Silv JE. Thyroid hormone and norepinephrine signaling in Brown adipose tissue. II. Differential effects of thyroid hormone on beta 3-adrenergic receptors in brown and white adipose tissue. Endocrinology. (1995) 136:3277–84. doi: 10.1210/en.136.8.3277
62. Martin B, Ji S, Maudsley S, Mattson MP. “Control” laboratory rodents are metabolically morbid: why it matters. Proc Natl Acad Sci USA. (2010) 107:6127–33. doi: 10.1073/pnas.0912955107
63. Novak CM, Burghardt PR, Levine JA. The use of a running wheel to measure activity in rodents: relationship to energy balance, general activity, and reward. Neurosci Biobehav Rev. (2012) 36:1001–14. doi: 10.1016/j.neubiorev.2011.12.012
64. Buynitsky T, Mostofsky DI. Restrain stress in behavioral research: recent developments. Neurosci Behav Rev. (2009) 33:1089–98. doi: 10.1016/j.neubiorev.2009.05.004
65. Cacioppo JT, Cacioppo S, Capotanio JP, Cole SW. The neuroendocrinology of social isolation. Annu Rev Psycol. (2015) 66:733–67. doi: 10.1146/annurev-psych-010814-015240
66. Mumtaz F, Khan MI, Zubair M, Dehpour AR. Neurobiology and consequences of social isolation stressin animal model-A comprehensive review. Biomed Pharmacother. (2018) 105:1205–22. doi: 10.1016/j.biopha.2018.05.086
67. Biggio F, Pisu MG, Garau A, Boero G, Locci V, Mostallino MC, et al. Maternal separation attenuates the effect of adolescent social isolation on HPA axis responsiveness in adult rats. Eur Neuropsycopharm. (2014) 24:1152–61. doi: 10.1016/j.euroneuro.2014.03.009
68. Butler TR, Ariwodola OJ, Weiner JL. The impact of social isolation on HPA axis function, anxiety-like behavior, and ethanol drinking. Front Interg Neurosci. (2014) 7:102. doi: 10.3389/fnint.2013.00102
69. Pisu MG, Garau A, Boero G, Biggio F, Pibiri V, Dore R, et al. Sex differences in the outcome of juvenile social isolation on HPA axis function in rats. Neuroscience. (2016) 320:172–82. doi: 10.1016/j.neuroscience.2016.02.009
70. Stranahan AM, Lee K, Mattson MP. Central mechanisms of HPA axis regulation by voluntary exercise. Neuromolecular Med. (2008) 10:118–27. doi: 10.1007/s12017-008-8027-0
71. Chen C, Nakagawa S, An Y, Ito K, Kitaichi Y, Kusumi I. The exercise-glucocorticoid paradox: how exercise is benefical to cognition, mood, and the brain while increasing glucocorticoid levels. Front Neuroendocrinol. (2017) 44:83–102. doi: 10.1016/j.yfrne.2016.12.001
72. Gollisch KSC, Brandauer J, Jessen N, Toyoda T, Nayer A, Hirshman MF, et al. Effects of exercise training on subcutaneous and visceral adipose tissue in normal- and high-fat diet-fed rats. Am J Physiol Endocrinol Metab. (2009) 297:E495–504. doi: 10.1152/ajpendo.90424.2008
73. Stanford KI, Goodyear LJ. Exercise regulation of adipose tissue. Adipocyte. (2016) 5:153–62. doi: 10.1080/21623945.2016.1191307
74. Blizard DA. Sex differences in running-wheel behaviour in the rat: the inductive and activational effects of gonadal hormones. Anim Behav. (1983) 31:378–84. doi: 10.1016/S0003-3472(83)80056-6
75. Jones LC, Bellingham WP, Ward LC. Sex differences in voluntary locomotor activity of food-restricted and ad libitum-fed rats. Implications for the maintenance of a body weight set-point. Comp Biochem Physiol A Comp Physiol. (1990) 96:287–90. doi: 10.1016/0300-9629(90)90694-N
76. Branyan KW, DeVallance ER, Lemaster KA, Skinner RC, Bryner RW, Olfert IM, et al. Role of chronic stress and exercise on microvascular function in metabolic syndrome. Med Sci Sports. (2018) 50:957–66. doi: 10.1249/MSS.0000000000001531
77. Kawashima H, Saito T, Yoshizato H, Fujikawa T, Sato Y, McEwen BS, et al. Endurance treadmill training in rats alters CRH activity in the hypothalamic paraventricular nucleus at rest and during acute running according to its period. Life Sci. (2004) 76:763–74. doi: 10.1016/j.lfs.2004.09.014
78. Droste SK, Chandromohan Y, Hill LE, Linthorst ACE, Reul JMHM. Voluntary exercise impacts on the rat hypothalamic-pituitary-adrenocortical axis mainly at the adrenal level. Neuroendocrinology. (2007) 86:26–37. doi: 10.1159/000104770
79. Ostrander MM, Ulrich-Lai YM, Choi DC, Richtand NM, Herman JP. Hypoactivity of the hypothalamo-pituitary-adrenocortical axis during recovery from chronic variable stress. Endocrinology. (2006) 147:2008–17. doi: 10.1210/en.2005-1041
80. Heck AL, Handa RJ. Sex differences in the hypothalamic–pituitary–adrenal axis' response to stress: an important role for gonadal hormones. Neuropsychopharmacology. (2019) 44:45–58. doi: 10.1038/s41386-018-0167-9
81. Beery AK, Kaufer D. Stress, social behavior and resilience: insights for rodents. Neurobiol Stress. (2015) 1:116–27. doi: 10.1016/j.ynstr.2014.10.004
82. Weintraub A, Singaravelu J, Bhatnagar S. Enduring and sex-specific effects of adolecent social isolation in rats on adult stress reactivity. Brain Res. (2010) 1343:83–92. doi: 10.1016/j.brainres.2010.04.068
83. Janhg JW, Yoo SB, Ryu V, Lee J-H. Hyperphagia and depression-like behavior by adolescence social isolation in female rats. Int J Dev Neurosci. (2012) 30:47–53. doi: 10.1016/j.ijdevneu.2011.10.001
84. Bale TL, Epperson CN. Sex differences and stress across the lifespan. Nat Neurosci. (2015) 18:1413–20. doi: 10.1038/nn.4112
85. Jiaxu C, Weiyi Y. Influence of acute and chronic treadmill exercise on rat brain POMC gene expression. Med Sci Sport Exerc. (2000) 32:954–7. doi: 10.1097/00005768-200005000-00012
86. Bi S, Scott KA, Hyun J, Ladenheim EE, Moran TH. Running wheel activity prevents hyperphagia and obesity in Otsuka Long-Evans Tokushima Fatty rats: role of hypothalamic signaling. Endocrinology. (2005) 146:1676–85. doi: 10.1210/en.2004-1441
87. Chen J-X, Zhao X, Yue G-X, Wang Z-F. Influence of acute and chronic treadmill exercise on rat plasma lactate and brain NPY, L-ENK, DYN A1-13. Cell Mol Neurobiol. (2007) 27:1–10. doi: 10.1007/s10571-006-9110-4
88. Coppola A, Meli R, Diano S. Inverse shift in circulating corticosterone and leptin levels elevates hypothalamic deiodinase type 2 in fasted rats. Endocrinology. (2005) 146:2827–33. doi: 10.1210/en.2004-1361
89. Morrison SF, Madden CJ, Tupone D. Central neural regulation of brown adipose tissue thermogenesis and energy expenditure. Cell Metab. (2014) 19:741–56. doi: 10.1016/j.cmet.2014.02.007
90. Dewal RS, Stanford KI. Effects of exercise on brown and beige adipocytes. Biochim Biophys Acta Mol Cell Biol Lipids. (2019) 1864:71–8. doi: 10.1016/j.bbalip.2018.04.013
91. Slocum N, Durrant JR, Bailey D, Yoon L, Jordan H, Barton J. Responses of brown adipose tissue to diet-induce obesity, exercise, dietary restriction and ephendrine treatment. Exp Toxicol Pathol. (2013) 65:549–57. doi: 10.1016/j.etp.2012.04.001
92. De Matties R, Lucertini F, Guescini M, Polidori E, Zeppa S, Stocchi V, et al. Exercise as new physiological stimulus for brown adipose tissue activity. Nutr Metab Cardiovasc Dis. (2013) 23:582–90. doi: 10.1016/j.numecd.2012.01.013
93. Barbosa de Queiroz K, Rodovalho GV, Guimaraes JB, Carvalho de Lima D, Coimbra CC, Evangelista EA, et al. Endurance training blocks uncoupling protein 1 up-regulation in brown adipose tissue while increasing uncoupling protein 3 in the muscle tissue of rats fed with high-sugar diet. Nutr Res. (2012) 32:709–17. doi: 10.1016/j.nutres.2012.06.020
Keywords: TRH, WAT, HPT axis, restraint, social isolation, exercise
Citation: Parra-Montes de Oca MA, Gutiérrez-Mariscal M, Salmerón-Jiménez MF, Jaimes-Hoy L, Charli J-L and Joseph-Bravo P (2019) Voluntary Exercise-Induced Activation of Thyroid Axis and Reduction of White Fat Depots Is Attenuated by Chronic Stress in a Sex Dimorphic Pattern in Adult Rats. Front. Endocrinol. 10:418. doi: 10.3389/fendo.2019.00418
Received: 22 March 2019; Accepted: 11 June 2019;
Published: 26 June 2019.
Edited by:
Lee E. Eiden, National Institutes of Health (NIH), United StatesReviewed by:
Gábor B. Makara, Hungarian Academy of Sciences (MTA), HungaryJoao Pedro Werneck De Castro, University of Miami, United States
Copyright © 2019 Parra-Montes de Oca, Gutiérrez-Mariscal, Salmerón-Jiménez, Jaimes-Hoy, Charli and Joseph-Bravo. This is an open-access article distributed under the terms of the Creative Commons Attribution License (CC BY). The use, distribution or reproduction in other forums is permitted, provided the original author(s) and the copyright owner(s) are credited and that the original publication in this journal is cited, in accordance with accepted academic practice. No use, distribution or reproduction is permitted which does not comply with these terms.
*Correspondence: Patricia Joseph-Bravo, joseph@ibt.unam.mx