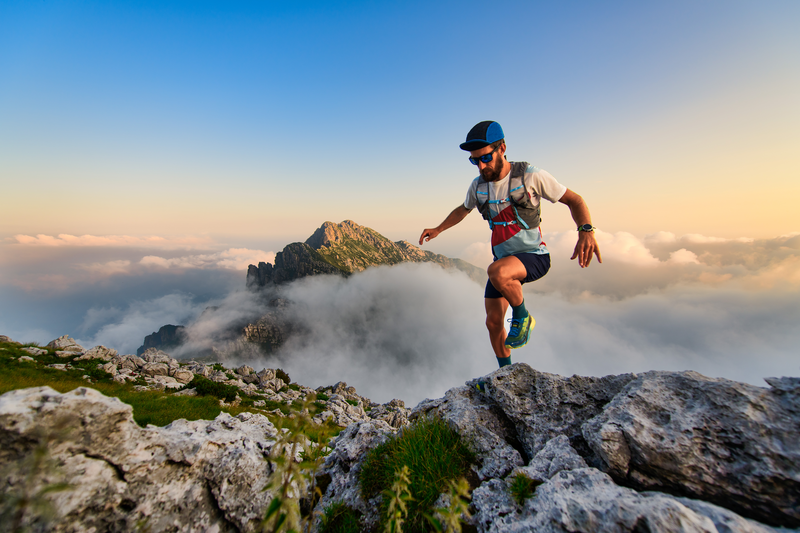
94% of researchers rate our articles as excellent or good
Learn more about the work of our research integrity team to safeguard the quality of each article we publish.
Find out more
ORIGINAL RESEARCH article
Front. Endocrinol. , 11 September 2018
Sec. Thyroid Endocrinology
Volume 9 - 2018 | https://doi.org/10.3389/fendo.2018.00527
Background: Protective effects of vitamin D have been reported in autoimmune and malignant thyroid diseases, though little is known about the underlying mechanism. Sirtuin 1 histon deacethylase (SIRT1) links the vitamin D pathway with regulation of transcription factor FOXO3a, a key player in cell cycle regulation and apoptosis. Aim of the present study was to investigate common single nucleotide polymorphisms (SNP's) in FOXO3a gene in respect to thyroid diseases, as well as to evaluate the hypothesis of Sirtuin1-FOXO3a interaction being a mediator of anti-proliferative vitamin D effects.
Methods: The SNP's FOXO3a rs4946936/rs4945816/rs9400239 were genotyped in 257 patients with differentiated thyroid carcinoma (DTC), 139 patients with Hashimoto thyroiditis (HT) and 463 healthy controls (HC). Moreover, T-helper cells of HC and papillary thyroid cancer cell line BCPAP were incubated with 1,25(OH)2D3 and/or SIRT1 inhibitor Ex-527 in order to elucidate SIRT1- dependent vitamin D effects on cell proliferation and FOXO3a gene expression in vitro.
Results: Patients with DTC tended to carry more often allele C in FOXO3a rs4946936 in comparison to HC (pcorrected = pc = 0.08). FOXO3a rs9400239T and rs4945816C was more frequent in HT in comparison to HC (pc = 0.02 and pc = 0.01, respectively). In both DTC and HT, we could not find a correlation of FOXO3a SNP's with vitamin D status. However, on in vitro level, 1,25(OH)2D3 showed an anti-proliferative effect in both T-helper cells and BCPAP, that was blocked by SIRT1 inhibition (T-helper cells: p = 0.0059, BCPAP: p = 0.04) and accompanied by elevated FOXO3a gene expression in T-helper cells (p = 0.05).
Conclusions: FOXO3a rs9400239T and rs4945816C may constitute risk factors for HT, independent of the vitamin D status.This indicates the implication of FOXO3a in pathogenesis of autoimmune thyroid diseases. The dependency of anti-proliferative vitamin D effects on SIRT1 activity further suggests a key role of vitamin D-SIRT1-FOXO3a axis for protective vitamin D effects.
Hashimoto's thyroiditis (HT) and differentiated thyroid cancer (DTC) are the most common autoimmune and malignant thyroid diseases. Although they differ by pathophysiology, both diseases manifest on the background of genetic susceptibility and either triggering or causal environmental factors (1, 2). These etiological characteristics become apparent in the frequent coincidence of HT with DTC, as well as in the familial clustering of the disease (3, 4).
Vitamin D (VD) deficiency represents a joint risk factor for HT (5) and DTC (6) and affects ~50% of the global population (7). Thus, low levels of vitamin D have been found in HT (5) and DTC (8). Notably, an inverse correlation of VD levels was found with the malignancy rate of thyroid nodules (6) as well as with thyroid antibody titers (1, 9) suggesting a causal link.
Whereas 25(OH)D3 is the stable inactive metabolite and used to assess the VD status in humans, 1,25(OH)2D3 is the final active form of VD, interacting with the VD receptor (VDR). By targeting various transcription factors and cell cycle proteins, the VDR mediates anti-proliferative, pro-differentiating and immune-modulating effects (10). Thus, VD was reported to inhibit cell growth of thyroid cancer (TC) cells (11). Moreover, VD has been shown to regulate type 1 and 17 T helper cells (12, 13), that are involved in the pathogenesis of HT (14, 15). Appropriately, VD treatment in patients with HT has been shown to decrease levels of thyroid antibodies (Ab's) (16). Various associations have been reported for single nucleotide polymorphisms (SNP's) of the VDR or VD metabolizing enzymes with both DTC (17, 18) and HT (19, 20). Therefore VD may further impact on autoimmune and malignant thyroid diseases through genetic susceptibility. However, until now the exact molecular mechanisms of VD's preventive potential in HT and DTC are still unclear.
Sirtuins are histone deacetylases, which are involved in various metabolic pathways, including aging and stress response (21). As NAD dependent metabolic sensors, sirtuins adjust posttranslational modification of cellular regulator proteins on energy status of the cell (22). Sirtuin 1 (SIRT1) represents a subform located in the nucleus that has lately not only been shown to be regulated by the VDR, but also been interpreted as a mediator of anti-proliferative VD signals by deacetylation of forkhead box protein O3a (FOXO3a) (23). SNP's of the FOXO3a related transcription factor forkhead box protein E1 (FOXE1) have only recently been reported as important risk factors for DTC (24). Interestingly, posttranslational modification of FOXO3a by SIRT1 antagonizes the phosphatidylinositol-4,5-bisphosphate 3-pathway (25). This signal cascade is activated in T-helper cells (Thc) upon T cell receptor activation (26) and has been found to be oncogenic in thyroid carcinoma (27). This suggests a potential key role of VD-SIRT1-FOXO3a signaling in immune regulation and DTC.
To the best of our knowledge, the VD-SIRT1-FOXO3a interaction has never been investigated in relation to autoimmune and malignant thyroid diseases. In this study, we aimed to examine common FOXO3a SNP's for association with autoimmune or malignant thyroid diseases. Moreover we questioned the SIRT1-dependency of anti-proliferative VD effects on immune cells and malignant thyroid cells as an indication of VD-SIRT1-FOXO3a interaction constituting a molecular pathway of protective VD effects.
In total 257 patients (172 females and 85 males, median age: 55 years) with pathologically confirmed diagnosis of DTC (208 of papillary differentiation and 49 of follicular differentiation) as well as 139 patients with HT (116 females and 23 males, median age: 40 years) were recruited from the Department of Medicine 1 and the Department of Nuclear Medicine at the University Hospital Frankfurt am Main, Germany as well as from the Department of Surgery at the Bürgerhospital Frankfurt am Main, Germany. Furthermore 463 healthy controls (HC, 228 females and 235 males, median age: 37 years) were volunteer blood donors.
The study protocol was approved by the Ethics Committee of the University Hospital Frankfurt am Main. Informed and written consent was obtained from all participants.
The 25(OH)D3 and 1,25(OH)2D3 plasma levels were measured by radioimmunoassay (DiaSorin, Stillwater, Minnesota, USA and IDS, Frankfurt am Main, Germany) in blood samples of DTC, HT, and HC. The expected normal range (95% reference interval) in healthy adults using this method is 9.0–37.6 ng/ml for 25(OH)D3 and 18.06–70.56 pg/ml for 1,25 (OH)2D3 level with a reported coefficient of variance of < 20% and < 11%, respectively. An enzyme-linked immunosorbent assay (Phadia, Freiburg, Germany) was used to determine serum levels of thyroglobulin (Tg)- and thyroperoxidase (TPO)- Ab's in patients with HT (n = 91).
The FOXO3a gene is located on chromosome 6 at the gene locus q21 (6q21). Three single nucleotide polymorphisms (SNP's) within FOXO3a gene were studied in DTC, HT and HC. The SNPs rs4946936 and rs4945816 are located at the 3′ untranslated (UTR) region of FOXO3a. The third SNP rs9400239 is located at the 5′ end of FOXO3a.
Genomic DNA from all blood samples was isolated by Miller and Dakes salting out procedure and subsequently amplified by polymerase chain reaction. The SNP's within the FOXO3a gene rs4946936 and rs4945816 (both specifically designed by Applied Biosystems) and rs9400239 (Assay-ID: C__11904122_10, Applied Biosystem) were analyzed using Taqman assays in an ABI 7300 system with conditions, recommended by the manufacturer. Random samples of the FOXO3a rs4946936 and FOXO3a 9400239 were analyzed twice in order to confirm the accuracy of the applied method. The results revealed a concordance rate of 100%.
Ten HC, consisting of 5 men and 5 women (median age: 26 years) were recruited for analysis of physiological VD–SIRT1-FOXO3a pathway in immune cells. Therefore, Thc were isolated from peripheral blood mononuclear cells of the subjects by using the CD4 T cell Isolation Kit II (Miltenyi Biotec, Bergisch Gladbach, Germany). During cell cultivation in basic cell medium, all cells were supplemented with the T cell mitogen phytohemagglutinin (PHA, Sigma-Aldrich) in concentrations of 50 μg/ml.
Human papillary thyroid cancer cell line BCPAP (RRID:CVCL_0153) was obtained from Leibniz Institute DSMZ, German Collection of Microorganisms and Cell Cultures (Braunschweig, Germany). This cell line was authenticated by DSMZ using multiplex PCR of minisatellite markers. The cells were processed immediately upon receipt and cells of passage four were used for five repetitions of the following cell culture approaches.
All cells were cultivated in RPMI-1640, that was supplemented with 10% FCS, 1% penicillin-streptomycin and 25 mM Hepes (basic cell medium) and were seeded out in volumes of 5 × 105 cells/ml in 6 well plates, in case of BCPAP cells (Sigma-Aldrich, munich, Germany, growth area: 9.5 cm2) or 24 well plates in case of Thc (Sigma-Aldrich, growth area: 1.9 cm2). Furthermore, either 10 nM 1,25(OH)2D3 or 2.4 μM of the SIRT1 inhibitor Ex-527 [that have been reported to abolish SIRT1 activity by virtually 100%, respectively (28)] or the combination of 1,25(OH)2D3 and Ex-527 were added.
The cells were incubated at 37°C. Forty-eight and ninety-six hours after isolation, cells were detached from the plates, requiring trypsinization in case of thyroid cells. Finally all cells were counted using hemocytometer.
A total of 18 ng RNA was extracted from BCPAP cells and Thc for reverse transcription into cDNA (Affinity Script QPCR, Agilent Technologies, Waldbronn, Germany). Quality control of RNA-integrity was performed previous to reverse transcription, using 2100 Bioanalyzer (Agilent Technologies). Subsequently, gene expression of FOXO3a (Hs00921424_m1) and the endogenous control 18s (Hs99999901_s1) were measured by Taqman assay (Applied Biosystems). Gene expression were compared as relative levels of cycle threshold (CT) normalized to the endogenous controls, calculated by 2−[CTtarget(t)−CT18s(t)] × 106.
The statistical analyses were performed using BiAS statistic software package 10.0 (Epsilon, Weinheim, Germany). Differences in genotype and allele distributions between all groups were evaluated by chi-square test. The odds ratio (OR) and its 95% confidence interval (CI) were estimated by unconditional logistic regression. CT-values, levels of thyroid Ab's, and cell proliferation were analyzed by non-parametric tests (Willcoxon-Mann-Whitney-U-test, Kruskall-Wallis-test and Wilcoxon matched pairs test).
In case of multiple comparisons, p values were corrected by multiplying them with the number of comparisons tested (Bonferroni correction). P-values (p or pc, respectively) < 0.05 were considered significant, p-values (p or pc, respectively) < 0.10 were considered as a trend.
DTC and HC were genotyped for the SNP's FOXO3a rs4946936, rs4945816, and rs9400239. The genotypes of all groups were in Hardy Weinberg equilibrium (p > 0.05).
The genotype analyses of all three SNP's did not show any differences between DTC and HC (Supplemental Table 1). However allele analysis of FOXO3a rs4946936 revealed a tendency of a higher frequency of allele “C” in DTC in comparison to HC (“C”: DTC vs. HC = 72.4% vs. 66.7%, OR: 1.28[CI 0.99–1.66], pc = 0.08, Supplemental Table 1). Allele and genotype distributions did not show association with markers of malignancy in DTC (tumor grade, TNM classification). Moreover, no correlation was found between genotypes of all investigated SNP's and VD status (DTC: Median level: 25(OH)D3 = 17.0 ng/ml and 1,25(OH)2D3 = 36.0 pg/ml; HC: 25(OH)D3 = 19.95 ng/ml and 1,25(OH)2D3 = 52.7 pg/ml, consistent within all genotypes, data not shown).
FOXO3a rs4946936, rs4945816, and rs9400239 were analyzed in 139 HT. Genotype analysis showed elevated frequency of “TT” in rs9400239 and “CC” in rs4945816 in patients with HT (FOXO3a rs9400239: “TT”: HT vs. HC = 16.5 vs. 9.3%, pc = 0.06; FOXO3a rs4945816: “CC”: HT vs. HC = 15.1 vs. 8.8%, pc = 0.06, Table 1). Moreover, HT carried allele T in rs9400239 and allele C in rs4945816 significantly more often than HC (FOXO3a rs9400239: “T”: HT vs. HC = 39.9 vs. 31.4%, OR: 1.45 [CI 1.10–1.91], pc = 0.02; FOXO3a rs4945816: “C”: HT vs. HC = 39.6 vs. 30.5%, OR: 1.5 [CI 1.12–2.00], pc = 0.01). These associations were especially strong in males (FOXO3a rs9400239: “T”: HT♂ vs. HC♂ = 52.2 vs. 30.9%, OR: 2.05 [CI 1.12–3.78], pc = 0.04, FOXO3a rs4945816: “C”: HT♂ vs. HC♂ = 47.8 vs. 29.9%, OR: 2.15 [CI 1.15–4.04], pc = 0.04, Table 1). We also analyzed the Tg- and TPO Ab's levels in relation to FOXO3a rs9400239 and rs4945816 genotypes. However we could not find significant differences (data not shown).
Table 1. Distribution of FOXO3a single nucleotide polymorphisms in Hashimoto thyroiditis and healthy controls.
The genotypes and alleles of FOXO3a rs4946936 did not differ between HT and HC.
No correlation was found between genotypes of all investigated SNP's and VD status (HT: Median level: 25(OH)D3 = 18.35 ng/ml and 1,25(OH)2D3 = 51.0 pg/ml, consistent within all genotypes, data not shown).
Since FOXO3a was reported as a target of the VD cascade by mediation of SIRT1, the effects of VD on cell proliferation were analyzed in relation to SIRT1 activity. Hereby, Thc of HC served as a model of physiologic VD-SIRT1-FOXO3a interaction.
We observed a significant cell suppressive effect of 1,25(OH)2D3 on Thc after 48 and 96 h (Median cell count(48 h): 1,25(OH)2D3 vs. Co = 2.48 × 105 vs. 4.16 × 105 cells/ml, p = 0.002, Figure 1A, 96 h: 1,25(OH)2D3 vs. Co = 6.86 × 105 vs. 1.15 × 106, p = 0.02 Figure 1B). Interestingly the cell suppressive effect of VD was blocked in cells that were additionally supplemented with the SIRT1 inhibitor Ex-527 after 48 h (Ex-527+1,25(OH)2D3 vs. 1,25(OH)2D3 = 2.81 × 105 vs. 2.48 × 105 cells/ml, p = 0.0059, Figure 1A).
Figure 1. Effect of vitamin D supplementation and SIRT1 inhibition on proliferation on T-helper cells. Thc of 10 HC were supplemented with the following reagents in cell counts of 1 x 105 cells/ml: 10 nM 1,25(OH)2D3 or 2.4 μM Ex-527, or 10 nM 1,25(OH)2D3+2.4 μM Ex-527. After 48 h (A) and 96 h (B) of cell cultivation, cell proliferation was measured by hemocytometer. Figure shows the mean value (bar level), the median (bold line), the standard deviation and maximum level of the measured cell counts in the corresponding groups of cell approaches.
Supplementation with Ex-527 alone did not affect cell count after 48 and 96 h of cell cultivation (Figures 1A,B).
Based on the insights of physiological VD/SIRT1/FOXO3a interplay in immune cells, a similar experimental setup was carried out in papillary thyroid cancer cells. None of the reagents affected BCPAP cell proliferation within 48 h of cultivation (1,25(OH)2D3 vs. Co; 1,25(OH)2D3+Ex-527 vs. 1,25(OH)2D3, Ex-527 vs. Co, peach>0.05, Figure 2A). However, as seen for Thc, we observed a significant inhibitory effect of 1,25(OH)2D3 on cell proliferation after 96 h (1,25(OH)2D3 vs. Co = 5.6 × 105 vs. 1.2 × 106 cells/ml, p = 8 × 10−3). Moreover, once again addition of SIRT1 inhibitor Ex-527 to 1,25(OH)2D3 treated cells reduced the anti-proliferative effect of 1,25(OH)2D3 (1,25(OH)2D3+Ex-527 vs. 1,25(OH)2D3 = 9.5 × 105 vs. 5.6 × 105 cells/ml, p = 0.04, Figure 2B). SIRT1 inhibition by Ex-527 alone did not affect cell proliferation of BCPAP after 48 and 96 h of cell cultivation (Figures 2A,B).
Figure 2. Effect of vitamin D supplementation and SIRT1 inhibition on proliferation on papillary thyroid cancer cells. Papillary thyroid cancer cells of cell line BCPAP were supplemented with the following reagents in cell counts of 1 × 105 cells/ml: 10 nM 1,25(OH)2D3 or 2.4 μM Ex-527, or 10 nM 1,25(OH)2D3+2.4 μM Ex-527. After 48 h (A) and 96 h (B) of cell cultivation, cell proliferation was measured by hemocytometer. Figure shows the mean value (bar level), the median (bold line), the standard deviation and maximum level of the measured cell counts in the corresponding groups of cell approaches.
Thc and BCPAP were further analyzed for SIRT1 dependent VD effects on FOXO3a gene expression level. Interestingly, VD supplemented Thc showed significant increase in FOXO3a gene expression after 48h (1,25(OH)2D3 vs. Co: 20.52 vs. 13.28 2−(CT FOXO3a−CT 18s) × 106, p = 0.05, Table 2). Remarkably, Thc cultivated with VD+Ex-527 did not differ in FOXO3a gene expression levels from Thc supplemented with 1,25(OH)2D3 alone (1,25(OH)2D3 vs. 1,25(OH)2D3+Ex-527: 20.52 vs. 21.66 2−(CTFOXO3a−CT 18s)x106, p > 0.05, Table 2). After 96 h of cultivation no effects were seen on FOXO3a gene expression for neither VD supplementation, nor the combination of VD and Ex-527 (data not shown).
Table 2. Effect of vitamin D stimulation and SIRT1 inhibition on FOXO3a gene expression in T-helper cells and BCPAP cells.
In contrast to Thc, VD supplementation did not affect FOXO3a gene expression in BCPAP. Also the combination of VD and SIRT1 inhibitor did not alter FOXO3a gene expression in papillary TC cells (Table 2).
Protective effects of vitamin D have been reported in several autoimmune (29) and malignant diseases (30), though little is known about the underlying molecular mechanisms.
In thyroid diseases, out of the VD pathway SNP's in the VDR are the best-studied genetic associations with HT and DTC. Four polymorphisms detected by restriction enzymes FokI, BsmI, ApaI, and TaqI have been investigated extensively in relation to thyroid diseases. Changes of the predicted VDR protein sequence at the FokI and ApaI sites have been associated with DTC (18) whereas FokI, BsmI, and TaqI have been related to autoimmune thyroid diseases (31–33).
New aspects of VD's mode of action affect SIRT1, a histone deacetylase implicated in cancer formation (34, 35) and autoimmune mechanisms (36). Enzymatic activation of SIRT1 by the VDR mediates deacetylation and hereby reactivation of the transcription factor FOXO3a (23). Interestingly, this forkheadbox protein is also found to be inhibited in DTC (37) and Thc upon stimulation (26). This concurrence suggests that FOXO3a could be a key mediator of VD's anti-proliferative and immune regulatory effects, also affecting the pathogenesis of DTC and HT. Since genetic variations of other members of the forkhead box protein family have already been identified as important risk factors for thyroid diseases (24), we questioned the association of FOXO3a SNP's with DTC and HT. Thus, FOXO3a rs4946936T has already been reported to be associated with vitiligo, an autoimmune disease that often occurs together with HT (38). In fact, FOXO3a rs9400239T and rs4945816C were associated with HT in our study group. To our knowledge, this is the first report of FOXO3a SNP's showing associations with autoimmune thyroid disease. Due to the limited number of patients, these observations need to be confirmed in a larger cohort. Since, rs9400239T or rs4945816C did not correlate with the Tg- or TPO-Ab level in HT, the underlying pathomechanism may not directly impact on antibody related immunological mechanisms.
Consistent with recent analyses of FOXO3a SNP's in cancers of other origin (39), we only found tendencies toward a higher frequency of FOXO3a rs4946936C in DTC. Future studies with more probands should elucidate the relevance of the observed distributions.
Despite its function as a target of the VD cascade, the association of FOXO3a SNP's with HT did not correlate with the VD status of the patients. In line with this observation, the FOXO3a gene itself does not harbor any VDRE's and FOXO3a transcriptional control is thought to occur via a VDRE in a neighboring gene (40).
A molecular explanation for the functional relevance of those non-coding SNP's could be an interaction with micro RNA's (41) affecting FOXO3a mRNA expression levels (42). Consistent with this observation, FOXO3a gene expression has been reported to be dysregulated in DTC (37).
Next we turned to the in vitro situation and questioned the dependency of protective VD effects on SIRT1-FOXO3a axis. In fact, our investigations indicate anti-proliferative effects of 1,25(OH)2D3 to depend on SIRT1 activity in Thc and cancer cells. Thus, the presence of the SIRT1 inhibitor Ex-527 in concentrations, known to abolish SIRT1 activity by virtually 100% (28), reduced the cell suppressive effect of 1,25(OH)2D3 on BCPAP and Thc. Our results therefore indicate SIRT1 to be a crucial component of VD's anti-proliferative signals in thyroid cancer cells and immune cells. Appropriately, those cell cycle regulative and pro-apoptotic genes that have been attributed as molecular mediators of VD's protective effects, such as Bim or GADD45 (43, 44), are also induced by SIRT1 activated FOXO3a (45, 46). In line with our investigations in DTC and immune cells, Sabir et al. only recently reported attenuation of VD signaling by Ex-527 in two different human non-thyroid cell lines (47). Furthermore An et al. linked VD signaling with enhanced cell cycle control by FOXO3a (23).
Our analyses further revealed a SIRT1 independent interaction of FOXO3a and the VD pathway in Thc. Thus, we observed a stimulative effect of 1,25(OH)2D3 on FOXO3a transcription in Thc, that wasn't affectable by SIRT1 inhibition. In contrast, this effect was not seen in papillary thyroid cancer cells of cell line BCPAP. Considering recent reports of FOXO3a gene expression being dysregulated in DTC (37), separation of FOXO3a transcriptional control from VD signaling should be focused in future studies as a potential oncogenic pathomechanism.
The impact of enzymatic SIRT1 activation or inhibition on thyroid cancer cell growth in vitro is poorly examined. Thus, while Shih et al. analyzed the effects of unspecific SIRT1 activator Resveratrol on proliferation and apoptosis of thyroid cancer cell lines (9), this is the first study to investigate the effect of SIRT1 inhibitor Ex-527. Whilst, Resveratrol induced apoptosis in thyroid cancer cells (9) we did not find effects of SIRT1 inhibition on cell proliferation of Thc and BCPAP cells.
In conclusion, this is the first study that reports FOXO3a to be associated with autoimmune thyroid diseases. Thus, we could identify two SNP's that may constitute genetic risk factors for HT. Moreover, VD-SIRT1 interaction could be confirmed in DTC cells and immune cells, suggesting SIRT1 to be a crucial mediator of immune regulatory and anti-proliferative action and indicating SIRT-FOXO3a as downstream targets of VD effects. So far VD is not a component of DTC therapy or treatment of autoimmune thyroid diseases. A combination of VD with SIRT1 activators, such as resveratrol or SRT1720 (48), could be a promising approach for upcoming anti-proliferative therapies.
All datasets for this study are included in the manuscript and the supplementary files.
The studies subjects were recruited and the patients' blood samples were collected by FG, CV, CD, M-LH, WB, KH, and KB. NR performed the experimental setups. NR and MP-M analyzed and interpreted the patient data. NR was the major contributor in writing this manuscript, which was revised by KB and MP-M. All authors read and approved the final manuscript.
The authors declare that the research was conducted in the absence of any commercial or financial relationships that could be construed as a potential conflict of interest.
The reviewer SF and handling Editor declared their shared affiliation.
The Supplementary Material for this article can be found online at: https://www.frontiersin.org/articles/10.3389/fendo.2018.00527/full#supplementary-material
Ab, Antibody; CI, confidence interval; Co, control; DMSO, dimethylsulfoxide; DTC, differentiated thyroid cancer; FCS, fetal calf serum; GAPDH, Glycerinaldehyd-3-phosphat dehydrogenase; h, hours; HC, healthy controls; LD, linkage disequilibrium; OR, odds ratio; pc, corrected p-values; PHA, phytohemagglutinin; RT-PCR, real time polymerase chain reaction; SIRT1, Sirtuin1; SNP, single nucleotide polymorphism; Thc, T-helper cell; Tg, thyroglobulin; TPO, thyroperoxidase; UTR, untranslated; VD, vitamin D; VDR, vitamin D Receptor.
1. Ferrari SM, Fallahi P, Antonelli A, Benvenga S. Environmental issues in thyroid diseases. Front Endocrinol. (2017) 8:50. doi: 10.3389/fendo.2017.00050
2. Agate L, Lorusso L, Elisei R. New and old knowledge on differentiated thyroid cancer epidemiology and risk factors. J Endocrinol Invest. (2012) 35(6 Suppl.):3–9.
3. Paparodis R, Imam S, Todorova-Koteva K, Staii A, Jaume JC. Hashimoto's thyroiditis pathology and risk for thyroid cancer. Thyroid (2014) 24:1107–14. doi: 10.1089/thy.2013.0588
4. Fallahi P, Ferrari SM, Ruffilli I, Elia G, Biricotti M, Vita R, et al. The association of other autoimmune diseases in patients with autoimmune thyroiditis: review of the literature and report of a large series of patients. Autoimmun Rev. (2016) 15:1125–8. doi: 10.1016/j.autrev.2016.09.009
5. Kivity S, Agmon-Levin N, Zisappl M, Shapira Y, Nagy EV, Dankó K, et al. Vitamin D and autoimmune thyroid diseases. Cell Mol Immunol. (2011) 8:243–7. doi: 10.1038/cmi.2010.73
6. Roskies M, Dolev Y, Caglar D, Hier MP, Mlynarek A, Majdan A. Vitamin D deficiency as a potentially modifiable risk factor for thyroid cancer. J Otolaryngol Head Neck Surg. (2012) 41:160–3.
7. Palacios C, Gonzalez L. Is vitamin D deficiency a major global public health problem? J Steroid Biochem Mol Biol. (2014) 144(Pt A):138–45. doi: 10.1016/j.jsbmb.2013.11.003
8. Sahin M, Uçan B, Giniş Z, Topaloglu O, Güngüneş A, Bozkurt NÇ, et al. Vitamin D3 levels and insulin resistance in papillary thyroid cancer patients. Med Oncol. (2013) 30:589. doi: 10.1007/s12032-013-0589-5
9. Shin DY, Kim KJ, Kim D, Hwang S, Lee EJ. Low serum vitamin D is associated with anti-thyroid peroxidase antibody in autoimmune thyroiditis. Yonsei Med J. (2014) 55:476–81. doi: 10.3349/ymj.2014.55.2.476
10. Trump DL, Muindi J, Fakih M, Yu W-D, Johnson CS. Vitamin D compounds: clinical development as cancer therapy and prevention agents. Anticancer Res. (2006) 26:2551–6.
11. Peng W, Wang K, Zheng R, Derwahl M. 1,25 dihydroxyvitamin D3 inhibits the proliferation of thyroid cancer stem-like cells via cell cycle arrest. Endocr Res. (2016) 41:71–80. doi: 10.3109/07435800.2015.1037048
12. Staeva-Vieira TP, Freedman LP. 1,25-dihydroxyvitamin D3 inhibits IFN-gamma and IL-4 levels during in vitro polarization of primary murine CD4+ T cells. J Immunol. (2002) 168:1181–9.
13. Joshi S, Pantalena L-C, Liu XK, Gaffen SL, Liu H, Rohowsky-Kochan C, et al. 1,25-dihydroxyvitamin D(3) ameliorates Th17 autoimmunity via transcriptional modulation of interleukin-17A. Mol Cell Biol. (2011) 31:3653–69. doi: 10.1128/MCB.05020-11
14. Li D, Cai W, Gu R, Zhang Y, Zhang H, Tang K, et al. Th17 cell plays a role in the pathogenesis of Hashimoto's thyroiditis in patients. Clin Immunol. (2013) 149:411–20. doi: 10.1016/j.clim.2013.10.001
15. Karanikas G, Schuetz M, Wahl K, Paul M, Kontur S, Pietschmann P, et al. Relation of anti-TPO autoantibody titre and T-lymphocyte cytokine production patterns in Hashimoto's thyroiditis. Clin Endocrinol. (2005) 63:191–6. doi: 10.1111/j.1365-2265.2005.02324.x
16. Ucan B, Sahin M, Sayki Arslan M, Paul M, Kontur S, Pietschmann P, et al. Vitamin D treatment in patients with Hashimoto's thyroiditis may decrease the development of hypothyroidism. Int J Vitam Nutr Res. (2017) 86:9–17. doi: 10.1024/0300-9831/a000269
17. Penna-Martinez M, Ramos-Lopez E, Stern J, Kahles H, Hinsch N, Hansmann ML, et al. Impaired vitamin D activation and association with CYP24A1 haplotypes in differentiated thyroid carcinoma. Thyroid (2012) 22:709–16. doi: 10.1089/thy.2011.0330
18. Penna-Martinez M, Ramos-Lopez E, Stern J, Kahles H, Hinsch N, Hansmann ML, et al. Vitamin D receptor polymorphisms in differentiated thyroid carcinoma. Thyroid (2009) 19:623–8. doi: 10.1089/thy.2008.0388
19. Yang J, Xiong F. Relevance of CYP27B1 gene promoter polymorphism to autoimmune thyroid diseases. Nan Fang Yi Ke Da Xue Xue Bao (2008) 28:606–8.
20. Lin W-Y, Wan L, Tsai C-H, Chen R-H, Lee C-C, Tsai F-J. Vitamin D receptor gene polymorphisms are associated with risk of Hashimoto's thyroiditis in Chinese patients in Taiwan. J Clin Lab Anal. (2006) 20:109–12. doi: 10.1002/jcla.20110
21. Chang H-C, Guarente L. SIRT1 and other sirtuins in metabolism. Trends Endocrinol Metab. (2014) 25:138–45. doi: 10.1016/j.tem.2013.12.001
22. Simmons GE, Pruitt WM, Pruitt K. Diverse roles of SIRT1 in cancer biology and lipid metabolism. Int J Mol Sci. (2015) 16:950–65. doi: 10.3390/ijms16010950
23. An B-S, Tavera-Mendoza LE, Dimitrov V, Wang X, Calderon MR, Wang HJ, et al. Stimulation of Sirt1-regulated FoxO protein function by the ligand-bound vitamin D receptor. Mol Cell Biol. (2010) 30:4890–900. doi: 10.1128/MCB.00180-10
24. Penna-Martinez M, Epp F, Kahles H, Ramos-Lopez E, Hinsch N, Hansmann ML, et al. FOXE1 association with differentiated thyroid cancer and its progression. Thyroid (2014) 24:845–51. doi: 10.1089/thy.2013.0274
25. Huang H, Tindall DJ. Dynamic FoxO transcription factors. J Cell Sci. (2007) 120(Pt 15):2479–87. doi: 10.1242/jcs.001222
26. Oh H-M, Yu C-R, Dambuza I, Marrero B, Egwuagu CE. STAT3 protein interacts with Class O Forkhead transcription factors in the cytoplasm and regulates nuclear/cytoplasmic localization of FoxO1 and FoxO3a proteins in CD4(+) T cells. J Biol Chem. (2012) 287:30436–43. doi: 10.1074/jbc.M112.359661
27. Nozhat Z, Hedayati M. PI3K/AKT pathway and its mediators in thyroid carcinomas. Mol Diagn Ther. (2016) 20:13–26. doi: 10.1007/s40291-015-0175-y
28. Solomon JM, Pasupuleti R, Xu L, McDonagh T, Curtis R, DiStefano PS, et al. Inhibition of SIRT1 catalytic activity increases p53 acetylation but does not alter cell survival following DNA damage. Mol Cell Biol. (2006) 26:28–38. doi: 10.1128/MCB.26.1.28-38.2006
29. Prietl B, Treiber G, Pieber TR, Amrein K. Vitamin D and immune function. Nutrients (2013) 5:2502–21. doi: 10.3390/nu5072502
30. Bikle DD. Vitamin D and cancer: the promise not yet fulfilled. Endocrine (2014) 46:29–38. doi: 10.1007/s12020-013-0146-1
31. Ban Y, Taniyama M. Vitamin D receptor gene polymorphisms in Hashimoto's thyroiditis. Thyroid (2001) 11:607–8. doi: 10.1089/105072501750302967
32. Ban Y, Taniyama M, Katagiri T. Vitamin D receptor initiation codon polymorphism in Japanese patients with Graves' disease. Thyroid (2000) 10:475–80. doi: 10.1089/thy.2000.10.475
33. Ban Y, Taniyama M. Vitamin D receptor gene polymorphism is associated with Graves' disease in the Japanese population. J Clin Endocrinol Metab. (2000) 85:4639–43. doi: 10.1210/jcem.85.12.7038
34. Yi J, Luo J. SIRT1 and p53, effect on cancer, senescence and beyond. Biochim Biophys Acta (2010) 1804:1684–9. doi: 10.1016/j.bbapap.2010.05.002
35. Yang H, Bi Y, Xue L, Wang J, Lu Y, Zhang Z, et al. Multifaceted modulation of SIRT1 in cancer and inflammation. Crit Rev Oncog. (2015) 20:49–64.
36. Kong S, McBurney MW, Fang D. Sirtuin 1 in immune regulation and autoimmunity. Immunol Cell Biol. (2012) 90:6–13. doi: 10.1038/icb.2011.102
37. Karger S, Weidinger C, Krause K, Sheu SY, Aigner T, Gimm O, et al. FOXO3a: a novel player in thyroid carcinogenesis? Endocr Relat Cancer (2009) 16:189–99. doi: 10.1677/ERC-07-0283
38. Ozel Turkcu U, Solak Tekin N, Gokdogan Edgunlu T, Karakas Celik S, Oner S. The association of FOXO3A gene polymorphisms with serum FOXO3A levels and oxidative stress markers in vitiligo patients. Gene (2014) 536:129–34. doi: 10.1016/j.gene.2013.11.055
39. Campa D, Hüsing A, Dostal L, Stein A, Drogan D, Boeing H, et al. Genetic variability of the forkhead box O3 and prostate cancer risk in the European Prospective Investigation on Cancer. Oncol Rep. (2011) 26:979–86. doi: 10.3892/or.2011.1359
40. Eelen G, Verlinden L, Meyer MB, Gijsbers R, Pike JW, Bouillon R, et al. 1,25-Dihydroxyvitamin D3 and the aging-related forkhead box O and sestrin proteins in osteoblasts. J Steroid Biochem Mol Biol. (2013) 136:112–9. doi: 10.1016/j.jsbmb.2012.09.011
41. Tan C, Liu S, Tan S, Zeng X, Yu H, Li A, et al. Polymorphisms in microRNA target sites of forkhead box O genes are associated with hepatocellular carcinoma. PLoS ONE (2015) 10:e0119210. doi: 10.1371/journal.pone.0119210
42. Wang Y, Zhou L, Chen J, Li J, He L, Wu P, et al. Association of the 3'UTR FOXO3a polymorphism rs4946936 with an increased risk of childhood acute lymphoblastic leukemia in a Chinese population. Cell Physiol Biochem. (2014) 34:325–32. doi: 10.1159/000363002
43. Suares A, Russo de Boland A, Verstuyf A, Boland R, González-Pardo V. The proapoptotic protein Bim is up regulated by 1α,25-dihydroxyvitamin D3 and its receptor agonist in endothelial cells and transformed by viral GPCR associated to Kaposi sarcoma. Steroids (2015) 102:85–91. doi: 10.1016/j.steroids.2015.08.005
44. Jiang F, Li P, Fornace AJ, Nicosia SV, Bai W. G2/M arrest by 1,25-dihydroxyvitamin D3 in ovarian cancer cells mediated through the induction of GADD45 via an exonic enhancer. J Biol Chem. (2003) 278:48030–40. doi: 10.1074/jbc.M308430200
45. Jeung Y-J, Kim H-G, Ahn J, Lee HJ, Lee SB, Won M, et al. Shikonin induces apoptosis of lung cancer cells via activation of FOXO3a/EGR1/SIRT1 signaling antagonized by p300. Biochim Biophys Acta (2016) 1863:2584–93. doi: 10.1016/j.bbamcr.2016.07.005
46. Kobayashi Y, Furukawa-Hibi Y, Chen C, Horio Y, Isobe K, Ikeda K, et al. SIRT1 is critical regulator of FOXO-mediated transcription in response to oxidative stress. Int J Mol Med. (2005) 16:237–43.
47. Sabir MS, Khan Z, Hu C, Galligan MA, Dussik CM, Mallick S, et al. SIRT1 enzymatically potentiates 1,25-dihydroxyvitamin D3 signaling via vitamin D receptor deacetylation. J Steroid Biochem Mol Biol. (2017) 172:117–29. doi: 10.1016/j.jsbmb.2017.06.010
Keywords: sirtuin1, FOXO3a, vitamin D, vitamin D receptor, differentiated thyroid carcinoma, Hashimoto's thyroiditis
Citation: Roehlen N, Doering C, Hansmann M-L, Gruenwald F, Vorlaender C, Bechstein WO, Holzer K, Badenhoop K and Penna-Martinez M (2018) Vitamin D, FOXO3a, and Sirtuin1 in Hashimoto's Thyroiditis and Differentiated Thyroid Cancer. Front. Endocrinol. 9:527. doi: 10.3389/fendo.2018.00527
Received: 18 May 2018; Accepted: 21 August 2018;
Published: 11 September 2018.
Edited by:
Alessandro Antonelli, Università degli Studi di Pisa, ItalyReviewed by:
Silvia Martina Ferrari, Università degli Studi di Pisa, ItalyCopyright © 2018 Roehlen, Doering, Hansmann, Gruenwald, Vorlaender, Bechstein, Holzer, Badenhoop and Penna-Martinez. This is an open-access article distributed under the terms of the Creative Commons Attribution License (CC BY). The use, distribution or reproduction in other forums is permitted, provided the original author(s) and the copyright owner(s) are credited and that the original publication in this journal is cited, in accordance with accepted academic practice. No use, distribution or reproduction is permitted which does not comply with these terms.
*Correspondence: Natascha Roehlen, bmF0YXNjaGEucm9laGxlbkBnbXguZGU=
†These authors share last authorship
Disclaimer: All claims expressed in this article are solely those of the authors and do not necessarily represent those of their affiliated organizations, or those of the publisher, the editors and the reviewers. Any product that may be evaluated in this article or claim that may be made by its manufacturer is not guaranteed or endorsed by the publisher.
Research integrity at Frontiers
Learn more about the work of our research integrity team to safeguard the quality of each article we publish.