- 1Department of Physical Medicine and Rehabilitation, Baylor Institute for Rehabilitation, Dallas, TX, United States
- 2Department of Neurology and Neurotherapeutics, University of Texas Southwestern Medical Center, Dallas, TX, United States
- 3Center for Neuro Skills, Bakersfield, CA, United States
- 4Department of Internal Medicine, University of Michigan, Ann Arbor, MI, United States
- 5Department of Neurology, University of Pennsylvania, Philadelphia, PA, United States
Traumatic brain injury (TBI) is a major cause of death and disability, but there are currently no therapies with proven efficacy for optimizing regeneration of repair during rehabilitation. Using standard stimulation tests, as many as 40–50% of survivors of severe TBI have deficiency of one or more pituitary hormones. Of these, the somatotropic axis is the most commonly affected, with Growth Hormone (GH) deficiency affecting ~20% of persons with severe TBI. Treatment with recombinant human Growth Hormone (rhGH) is generally effective in reversing the effects of acquired GH deficiency, but there is no evidence documenting functional or neurocognitive improvement after GH replacement in TBI patients. As a consequence, screening for GH deficiency and GH replacement when deficiency is found is not routinely performed as part of the rehabilitation of TBI survivors. Given that most of the recovery after TBI occurs within the first 6–12 months after injury and IGF-1 and GH are part of a coordinated restorative neurotrophic system, we hypothesized that patients will optimally benefit from GH therapy during the window of maximal neuroregenerative activity. We performed a Phase IIa, randomized, double-blind, placebo-controlled feasibility trial of recombinant human Growth Hormone (rhGH), starting at discharge from an inpatient rehabilitation unit, with follow up at 6 and 12 months. Our primary hypothesis was that treatment with rhGH in the subacute period would result in improved functional outcomes 6 months after injury. Our secondary hypothesis proposed that treatment with rhGH would increase IGF-1 levels and be well tolerated. Sixty-three subjects were randomized, and 40 completed the trial. At baseline, there was no correlation between IGF-1 levels and peak GH levels after L-arginine stimulation. IGF-1 levels increased after rhGH treatment, but it took longer than 1 month for levels to be higher than for placebo-treated patients. rhGH therapy was well-tolerated. The rhGH group was no different from placebo in the Disability Rating Scale, Glasgow Outcome Scale-Extended, or neuropsychological function. However, a trend toward greater improvement from baseline in Functional Independence Measure (FIM) was noted in the rhGH treated group. Future studies should include longer treatment periods, faster titration of rhGH, and larger sample sizes.
Introduction
Traumatic brain injury (TBI) is a major cause of death and disability, with an estimated cost of 45 billion dollars a year in the United States alone. Every year, ~2.5 million people in the United States sustain a TBI, of which 53,000 people die, and another 284,000 are hospitalized and survive the injury (1). Currently, more than 5.3 million Americans (or 3% of the population) live with disabilities resulting from TBI and virtually no injury is without consequence (1). The magnitude of this problem has led to numerous clinical trials aimed at improving survival and/or functional outcome, yet no effective therapies have been identified. In particular, no therapies aimed at optimizing regeneration and repair during the rehabilitation period have been demonstrated to be effective.
TBI has long been recognized as a chronic cause of neuroendocrine dysfunction. While overt deficiency of the somatotropic, gonadotropic, thyroid, or adrenal axis have been recognized for many decades, subtler deficiencies have come to light only more recently. Using standard stimulation tests, as many as 40–50% of survivors of severe TBI have deficiency of one or more pituitary hormones (2). Of these, the somatotropic axis is the most vulnerable, with estimates of Growth Hormone (GH) deficiency affecting ~20% of persons with severe TBI (3). In contrast, thyroid hormone and adrenocorticotrophic hormone deficiency are relatively uncommon, with rates averaging 4–6%, while the gonadotrophic axis shows intermediate rates of dysfunction, between 8 and 12% (4–6). The clinical consequences of GH deficiency include decrease in muscle strength, decrease in lean body mass, and increase in abdominal fat, and reduced basal metabolic rate. However, the most common symptoms are neuropsychiatric, particularly fatigue, asthenia, depression, sleep disturbances, and memory problems (2). In a recently published study, GH deficiency, or insufficiency was associated with neurobehavioral deficits, decreased concentration, depression, and reduced quality of life (7) which underscores why GH deficiency after TBI is underdiagnosed as the symptoms and signs are non-specific and are often mistaken for or masked by the underlying traumatic injury.
Treatment with recombinant human Growth Hormone (rhGH) is generally effective in reversing the effects of acquired GH deficiency (8). In randomized controlled trials of adult patients with acquired GH deficiency (resulting primarily from pituitary tumors), rhGH therapy resulted in improved cognitive function, well-being, and quality of life (8, 9), in addition to improvements in metabolic factors such as bone mineral density and lean body mass. While studies have demonstrated the presence of GH deficiency in patients surviving TBI, there has been a paucity of evidence documenting functional or neurocognitive improvement after GH replacement therapy in TBI patients (10–13). A consequence of such lack of evidence is that screening for GH deficiency (and GH replacement when deficiency is found) is not routinely performed as part of the rehabilitation of TBI survivors.
Given that most of the recovery after TBI occurs within the first 6–12 months after injury and IGF-1 and GH are part of a coordinated restorative neurotrophic system, it is likely that they exert their effects in synergy with a host of other trophic and restorative factors that are maximally functioning within the first few months after injury. While treatment >1 year after injury in patients who remain GH deficient holds promise, we hypothesized that many patients will optimally benefit from GH therapy (and resulting elevations in IGF-1 levels) during the window of maximal neuroregenerative activity.
Treating only patients who have GH deficiency defined using stimulation tests (either glucagon or GHRH + L-arginine, which were developed for non-trauma populations), as was done in a past clinical trial, may be too conservative (14), as it may deny therapy to many patients with hypothalamic dysfunction and serum IGF-1 concentrations suboptimal to maximize recovery. It remains unclear what the optimal IGF-1 level is in patients recovering from TBI, and patients with serum IGF-1 levels in the lower end of the normal distribution may benefit from augmenting IGF-1 production with GH treatment, to maintain concentrations of this neurotrophic factor near the upper third of the normal range during the time window of maximal repair and regeneration.
This study is a Phase IIa, randomized, double-blind, placebo-controlled feasibility trial of rhGH, starting at discharge from an inpatient rehabilitation unit or while in a transitional rehabilitation setting, with follow up at 6 and 12 months. Our primary hypothesis was that treatment with recombinant human Growth Hormone (rhGH) in the subacute period after TBI would result in improved functional outcomes 6 months after injury as compared to placebo. Our secondary hypothesis proposed that treatment with rhGH would result in increased IGF-1 levels, that rhGH therapy would be well tolerated, and would not result in an increased rate of diabetes mellitus, arthralgias, or peripheral edema.
Methods
Participants who suffered acute TBI were recruited into the study from an acute inpatient rehabilitation or patients receiving care at a transitional post-acute rehabilitation setting. Our goal was to enroll participants who suffered a sufficiently severe injury to require inpatient rehabilitation or transitional rehab care and were at a timeframe with the most potential for neuroregeneration. Table 1 details eligibility criteria used for screening. This study was approved by the Institutional Review Boards at Baylor University Medical Center and the University of Texas Southwestern Medical Center, both in Dallas, Texas. Written consent was obtained from all participants or their legally authorized representatives, when the participant did not have capacity to provide informed consent. In these latter cases assent was obtained from the participant. The trial was registered with ClinicalTrials.gov (NCT 00766038) prior to the first enrollment.
Subjects
Figure 1 shows a CONSORT diagram which shows the flow of patient activity from screening through completion of the project. Informed consent was obtained from all participants before any study activities were conducted.
A total of 63 subjects out of 126 subjects who consented were eligible for randomization into the study with 31 randomized to treatment with rhGH and 32 randomized to placebo. IGF-1 levels were measured on all patients with some patients undergoing dynamic GH testing. Initial inclusion criteria required low initial IGF-1 (<1 standard deviation below mean) and/or a low Peak GH upon stimulation (</= 1.4 ng/mL). Age and gender-specific reference ranges (mean and SDs) were taken from published tables (15). Due to a national unavailability of intravenous L-arginine which developed during the study, dynamic stimulation testing was not available for all participants. Eligible patients were randomized in a double-blind fashion to (Group 1) rhGH subcutaneously or (Group 2) placebo. The vehicle for placebo treatment was normal saline, packaged in multi-dose injectors identical to the rhGH given to the treatment group. The GH treatment arm received a starting dose of 400 μg/day, with increases (or decreases) in dose by 100–200 μg/day based on IGF-1 labs results obtained at 1 and 3 months, until target IGF-1 (in the upper quintile of the range for age and body weight) was reached up to maximum dose of 1,000 μg/day. Doses for participants receiving placebo were also adjusted monthly to maintain the blinding. Treatment continued for a period of 6 months.
Treatment was overseen by a board certified endocrinologist (RA) according to practice guidelines released by the Endocrine Society “Clinical Guidelines for Evaluation and Treatment of Adult Growth Hormone Deficiency” (16, 17). In this study, to accommodate both GH-deficient and GH-sufficient strata, the treatment goal was serum IGF-1 as close as possible to the upper limit for the age-adjusted reference range without exceeding the normal range. The dose of GH (or placebo) was adjusted by the study physician depending on the occurrence and severity of adverse effects.
Safety Assessments
Safety was assessed by clinical evaluations at 1, 3, and 6 and 12 months after initiating therapy. At these evaluations participants and their caregivers were asked about possible adverse effects of GH treatment, including fluid retention, paresthesias, joint stiffness, peripheral edema, arthralgias, and myalgias. Additionally, blood was obtained for assessment of glucose, free T4, lipid profile, and insulin level.
Study visits and procedures at each visit are depicted in Table 2.
Functional Outcomes and Neuropsychological Testing
An abridged neuropsychological battery was conducted at baseline, with additional tests at 6 and 12 months (Table 2). These measures are designed to capture a broad perspective of functional and cognitive parameters that are important following TBI, and utilizes scales that have a long history in TBI research. Further, there is convincing evidence that they can be reliably administered 6–12 month after injury. Functional Independence Measure (FIM) was tracked at baseline, 3, 6, and 12 months as a measure of the severity of functional impairment, during the course of study enrollment. FIM consists of 18 items, with each item rated on a 7-point scale, ranging from 1 (total assistance) to 7 (complete independence). Global function was measured by the Disability Rating Scale (DRS) and Glasgow Outcome Scale—Extended (GOSE). Cognitive executive function was measured with the Galveston Orientation and Amnesia Test (GOAT), Digit Span and Processing Speed Index from the WAIS-III, the DKEFS Stroop test, Trail Making Test—Part A and B, California Verbal Learning Test-2 (CVLT-2), and Controlled Word Association (COWA). Emotional behavior function was measured with the Brief Symptom Inventory (BSI), Fatigue Severity Scale (FSS), and the Rivermead Post Concussion Symptom Questionnaire. Finally, quality of life was assessed using the Satisfaction with Life Scale (SWLS) and the Short-Form 36 (SF-36).
Biochemical Tests
IGF-1 levels were measured by Quest Laboratories using a liquid chromatrography/mass spectrometry assay. This laboratory also carried out the safety lab assessments (glucose, insulin, lipid profile). See legend at bottom of Table 7 for normative lab values.
Statistical Analysis
In this Phase II study, for the primary hypothesis, the design of our study was that of a non-futility study, powered to not reject a potentially useful therapy, rather than prove efficacy. All participants in the GH treatment arm did not achieve goal serum IGF-1 values in the first month, data was analyzed in an intention-to-treat manner.
Final analysis included 40 subjects who completed 6 month follow up measures. Baseline characteristics for the treatment and placebo groups were compared using t-tests or Mann–Whitney-U-test for numerical variables and chi-square or Fisher's exact tests for categorical variables. To compare IGF-1 levels between groups at each visit, Mann–Whitney U-tests were used. Other functional and neuropsychological outcomes listed in Table 2 were compared at both the 6-month visit and the 12 month visit. T-tests, Mann–Whitney U-tests or chi-square tests were performed, as appropriate, to determine if there were significant differences in each outcome. Comparison of adverse events between treatment groups were performed using Fisher's exact tests due to low counts. To determine if participants who withdrew or were lost to follow-up were different than those who completed the 6 month visit, baseline characteristics were compared using the same tests mentioned above. All analyses were performed using SAS 9.4 with a 5% significance level of 5%.
Results
Sixty-three subjects were randomized into the study, 31 in the rhGH intervention group and 32 in the placebo group. Of those randomized, 40 subjects (20 in each group) completed treatment through the 6 month follow up visit. Table 3 shows the baseline characteristics of those participants who completed 6 months of treatment, stratified by treatment and placebo groups. On average, subjects were 31.1 ± 14.3 years old, male (85%), and White (92.5%). There were no significant differences between the intervention and placebo group except in cause of injury and FIM admission scores.
Only 24 participants were underwent dynamic GH testing (Table 3), due to the unforeseen unavailability of L-arginine mid-way through the study. Figure 2 shows a scatterplot that depicts there was no correlation between IGF-1 levels and peak GH levels after L-arginine stimulation.
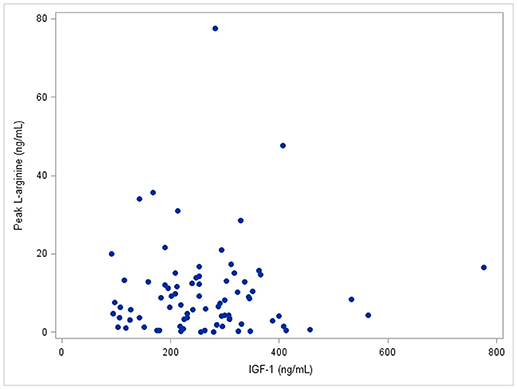
Figure 2. IGF-1 levels vs. peak L-arginine level. The Pearson correlation between IGF-1 and peak L-arginine is 0.03, p > 0.2.
Table 4 shows a crude analysis of IGF-1 levels between the treatment and placebo groups. There was a significant difference between the two groups at 3 and 6 months (p < 0.05), but not after 1 month of treatment. The lack of difference between the 2 groups at 1 month was expected, as the initial dose of rhGH chosen was relative low and time was required to adjust the dose of rhGH properly to therapeutic levels based on measured drug levels. As expected, there were no longer differences in IGF-1 levels at 12 months since treatment with either drug or placebo ended 6 months.
Tables 5, 6 show the primary outcomes, including neuropsychological testing and functional outcomes, stratified by the treatment and placebo groups. There were no significant differences seen between the two groups for any of the neuropsychological measures or DRS. While neuropsychological testing was conducted at 3 months, the results are not shown in table form as they are consistent with the results at 6 and 12 months, showing no statistically significant differences. However, there was variation in FIM scores, with the rhGH treatment group showing significantly greater change for FIM motor, FIM cognitive, and FIM total from baseline to 6 month follow up (p = 0.02 for all strata). At 12 month follow up FIM motor change and FIM total change were significantly greater in the rhGH treatment group compared to the placebo group (p = 0.02 and p = 0.01, respectively). There was no correction for multiple comparisons.
There was no difference among safety labs between rhGH and placebo groups as outlined in Table 7. Finally, adverse events between the rhGH and placebo groups for all 63 participants are described in Table 8. Two participants in the rhGH group and 3 participants in the placebo group discontinued the study drug and participation in the project due to adverse events. There was no statistically significant difference in the reporting of undesirable side effects in the treatment group compared to the placebo group.
Discussion
There is extensive data from experimental models that GH and IGF-1 are part of the neuro-restorative response after TBI and other acquired brain injuries, such as ischemic stroke, hypoxia-ischemia, and excitotoxins. Treatment with rhGH (19–24) or IGF-1 (19, 25–27) results in improved neurological and cognitive outcome in multiple experimental models. This is particularly the case when therapy is administered early after injury (21, 23). In humans, higher levels of IGF-1 are associated with greater white matter recovery after TBI (16), and improved functional outcome after ischemic stroke (28, 29). These well-established observations formed the scientific rationale for this study.
While GH deficiency is a well-recognized consequence of TBI, there is little Class I evidence that rhGH replacement produces clinical benefits. A consequence of such lack of evidence is that screening for GH deficiency (and GH replacement when deficiency is found) is not routinely performed as part of the rehabilitation of TBI survivors. Several small clinical trials provide useful information on the issue of whether replacement therapy with rhGH is clinically beneficial in chronic post-TBI patients (>1 year after injury). High et al. (11) randomized 23 subjects to either GH replacement or placebo. Despite the small sample size, this study clearly showed that GH replacement therapy is effective in increasing serum IGF-1 levels in GH deficient and insufficient subjects, defined as a GH peak under 3 or 8 ng/ml (respectively) upon glucagon stimulation. There was also an indication that GH replacement was associated with improvement in several neuropsychological measures. Moreau et al. (12) conducted an open-label study on 23 TBI patients with GH deficiency (average 7.8 years after injury), compared to 27 controls (not treated with rhGH because they had normal or near-normal somatotropic function (n = 24) or who had contra-indications or refused treatment with rhGH. There were benefits in cognitive function, particularly in attention, memory, and visuospatial abilities. Reimunde et al. (13) treated 11 patients (average 3.7 years after injury) with GH deficiency with rhGH, and compared them with 8 controls without GH deficiency, and found evidence of greater improvement in several cognitive tests in the rhGH treated group. Devesa et al. (10) reported treated 13 TBI patients (2.5 months to 11 years after injury) with rhGH; 5 patients had GH deficiency and 8 did not. In this open label study inconsistent cognitive improvements were noted.
For the primary hypothesis, the treatment group had significantly greater FIM motor change and FIM total change from baseline that the placebo group at 6 and 12 months. This must be interpreted with caution given that the treatment group had a significant lower FIM motor and total FIM score at baseline as compared to the placebo group. However, the treatment group did achieve a statistically significant improvement in the FIM cognitive change at 6 months despite the two groups having similar FIM cog scores at baseline. However, there was no significance seen in the FIM cognitive change at 12 months that was seen at 6 months testing. The most likely reason for FIM change significance is the imbalance of the functional measures at entry to the study and the possible statistical artifact with regression of the treatment group toward the mean. Additionally, there was no statistically significant difference in the FIM motor, FIM cognitive or FIM total scores between the two groups at either time period.
Our key secondary analyses did show increased IGF-1 levels in those treated with rhGH, however the first measure where IGF-1 levels statistically differed from the placebo group was not seen until month 3. The fact that this effect took at least 3 months to manifest was likely due to the design of the study in which subjects were started at lower rhGH doses which were likely to be well-tolerated. It is possible that more aggressive initial dosing, faster titration of dosing, or longer period of treatment may be necessary to see an observable impact on functional outcomes.
Patients treated with rhGH did achieve higher IGF-1 levels that those receiving placebo, with significant increases seen at 3 and 6 months with IGF-1 levels returning to pre-treatment levels by 12 months. At the 12-month timeframe, both groups had similar IGF-1 levels, indicating that IGF-1 does not stay elevated after cessation of treatment. Clinically this could hold promise since IGF-1 is a putative neuroregenerative factor after TBI. Recent preclinical and clinical data suggests that maintenance of high IGF-1 levels would be beneficial after TBI. IGF-1 is a neurotrophic factor (30), both in experimental models (27) as well as in human diseases such as Alzheimer's disease and vascular dementia (31). It appears that IGF-1 is part of a neurotrophic response to multiple types of injury to the CNS, including TBI (32). In rodents, hypoxic-ischemic injury is ameliorated by recombinant IGF-1 administration (25), regardless of whether the drug is administered 2 h after or 1 h before injury. In the cortical stab injury model, blockade of IGF-1 by an anti-IGF-1 antibody reduces neovascularization at the wound site (30). In the same model, administration of IGF-1 4 and 12 h post-injury resulted in improved motor activity and decrease in apoptotic cells in the peritraumatic area (26). In the fluid percussion model in rats, administration of IGF-1 starting 15 min postinjury and continuing for 14 days resulted improved neuromotor function and enhanced learning ability, which was evident 14 days after injury but not at 2 or 7 days (27).
There have been studies that found greater incidence of depression and decreased quality of life in GH-deficient subjects (7). While no significance was reached in clinical outcomes at 6 and 12 months, there was a trend in the rhGH treated group to have higher SF 36 Physical health verse the placebo group at 6 months and another trend was seen in the rhGH treated group to having higher scores on the SF 36 mental health at 12 months. Additionally, it is important to note that the majority of those in the study (both drug and placebo groups) achieved relatively good functional outcomes, with high moderate disability to good recovery noted on the Glasgow Outcome Scale-Extended (GOSE) by 12 months. It is possible that rhGH would have a more favorable effect in more severely injured subjects, destined for less favorable recovery.
We were able to show that treatment with rhGH was overall well-tolerated in subjects with no significant side effects compared to placebo in developing rates of undesirable side effects that can occur with rhGH replacement. In fact, comparison of adverse event data revealed that there were only 2 events in the treatment group requiring temporary cessation or discontinuation of the study drug compared to 3 such events in the placebo group.
There are limitations that need mentioning. Due to low power based on smaller than projected enrollment and a moderate number of dropouts, we were not able to show that IGF-1 is a prognostic biomarker (meaning that those that have a higher baseline IGF-1 have improved outcomes). Additionally, analysis of the data in only male subjects in order to address sex differences in regards to treatment effect was not able to be performed due to lack of sufficient power. Another limitation of our study was the lack of all patients having dynamic testing of GH. While there are studies that report IGF-1 levels to be highly predictive of GH deficiency (5, 33), GH levels are most routinely assessed following stimulation with arginine, glucagon, or an insulin tolerance test. Due to national shortage of L-arginine during the study, not all patients were assessed with dynamic testing methods.
Conclusions
This phase IIa, randomized, double-blind, placebo-controlled trial showed that it is feasible to achieve IGF-1 levels in the upper quartile in patients following severe TBI in the subacute period, and that treatment with rhGH is well-tolerated. While the rhGH treatment group did achieve statistically greater FIM total and FIM motor change than the placebo group, it is impossible to ascribe such change to rhGH treatment, since the relatively low sample size made it impossible to adjust for multiple comparisons and possible confounders. Future studies should look at longer treatment periods, consider faster titration of rhGH during the treatment period as well as seek further evidence in supporting rhGH treatment effect on clinical outcomes.
Author Contributions
RD, LC,CD, CH, LK, MB, and RD-A contributed to the design of the study, participated in patient enrollment and followup, and was involved in data analysis and preparation or manuscript for publication. RA participated in study design and adjusted medication doses in a blinded fashion.
Funding
National Institute of Disability, Independent Living, and Rehabilitation Research (Award H133A020526). Pfizer contributed rhGH and placebo preparations (Award GA62816O).
Conflict of Interest Statement
The authors declare that the research was conducted in the absence of any commercial or financial relationships that could be construed as a potential conflict of interest.
References
1. Centers for Disease Control and Prevention. Report to Congress on Traumatic Brain Injury in the United States: Epidemiology and Rehabilitation. Atlanta (2015).
2. Schneider M, Schneider HJ, Stalla GK. Anterior pituitary hormone abnormalities following traumatic brain injury. J Neurotrauma (2005) 22:937–46. doi: 10.1089/neu.2005.22.937
3. Agha A, Walker D, Perry L, Drake WM, Chew SL, Jenkins PJ, et al. Unmasking of central hypothyroidism following growth hormone replacement in adult hypopituitary patients. Clin Endocrinol. (2007) 66:72–7. doi: 10.1111/j.1365-2265.2006.02688.x
4. Agha A, Rogers B, Sherlock M, O'Kelly P, Tormey W, Phillips J, et al. Anterior pituitary dysfunction in survivors of traumatic brain injury. J Clin Endocrinol Metab. (2004) 89:4929–36. doi: 10.1210/jc.2004-0511
5. Aimaretti G, Ambrosio MR, Di Somma C, Fusco A, Cannavo S, Gasperi M, et al. Traumatic brain injury and subarachnoid haemorrhage are conditions at high risk for hypopituitarism: screening study at 3 months after the brain injury. Clin Endocrinol. (2004) 61:320–6. doi: 10.1111/j.1365-2265.2004.02094.x
6. Bondanelli M, De Marinis L, Ambrosio MR, Monesi M, Valle D, Zatelli MC, et al. Occurrence of pituitary dysfunction following traumatic brain injury. J Neurotrauma (2004) 21:685–96. doi: 10.1089/0897715041269713
7. Kelly DF, McArthur DL, Levin HS, Swimmer S, Dusik JR, Cohan P, et al. Neurobehavioral and quality of life changes associated with Growth Hormone insufficiency after complicated mild, moderate, and severe traumatic brain injury. J Neurotrauma (2006) 23:928–42. doi: 10.1089/neu.2006.23.928
8. Carroll PV, Christ ER, Bengtsson BA, Carlsson L, Christiansen JS, Clemmons D, et al. Growth hormone deficiency in adulthood and the effects of growth hormone replacement: a review. Growth Hormone Research Society Scientific Committee. J Clin Endocrinol Metab. (1998) 83:382–95.
9. Burman P, Broman JE, Hetta J, Wiklund I, Erfurth EM, Hagg E, et al. Quality of life in adults with growth hormone (GH) deficiency: response to treatment with recombinant human GH in a placebo-controlled 21-month trial. J Clin Endocrinol Metab. (1995) 80:3585–90.
10. Devesa J, Reimunde P, Devesa P, Barbera M, Arce V. Growth hormone (GH) and brain trauma. Horm Behav. (2013) 63:331–44. doi: 10.1016/j.yhbeh.2012.02.022
11. High WM Jr, Briones-Galang M, Clark JA, Gilkison C, Mossberg KA, Zgaljardic DJ, et al. Effect of growth hormone replacement therapy on cognition after traumatic brain injury. J Neurotrauma (2010) 27:1565–75. doi: 10.1089/neu.2009.125
12. Moreau OK, Cortet-Rudelli C, Yollin E, Merlen E, Daveluy W, Rousseaux M. Growth hormone replacement therapy in patients with traumatic brain injury. J Neurotrauma (2013) 30:998–1006. doi: 10.1089/neu.2012.2705
13. Reimunde P, Quintana A, Castanon B, Casteleiro N, Vilarnovo Z, Otero A, et al. Effects of growth hormone (GH) replacement and cognitive rehabilitation in patients with cognitive disorders after traumatic brain injury. Brain Inj. (2011) 25:65–73. doi: 10.3109/02699052.2010.536196
14. Leal-Cerro A, Flores JM, Rincon M, Murillo F, Pujol M, Garcia-Pesquera F, et al. Prevalence of hypopituitarism and growth hormone deficiency in adults long-term after severe traumatic brain injury. Clin Endocrinol. (2005) 62:525–32. doi: 10.1111/j.1365-2265.2005.02250.x
15. Brabant G, von zur MA, Wuster C, Ranke MB, Kratzsch J, Kiess W, et al. Serum insulin-like growth factor I reference values for an automated chemiluminescence immunoassay system: results from a multicenter study. Horm Res. (2003) 60:53–60. doi: 10.1159/000071871
16. Feeney C, Sharp DJ, Hellyer PJ, Jolly AE, Cole JH, Scott G, et al. Serum insulin-like growth factor-I levels are associated with improved white matter recovery after traumatic brain injury. Ann Neurol. (2017) 82:30–43. doi: 10.1002/ana.24971
17. Molitch ME, Clemmons DR, Malozowski S, Merriam GR, Shalet SM, Vance ML, et al. Evaluation and treatment of adult growth hormone deficiency: an endocrine society clinical practice guideline. J Clin Endocrinol Metab. (2006) 91:1621–34. doi: 10.1210/jc.2011-0179
18. Malec JF, Brown AW, Leibson CL, Flaada JT, Mandrekar JN, Diehl NN, et al. The Mayo classification system for traumatic brain injury severity. J Neurotrauma (2007) 24:1417–24. doi: 10.1089/neu.2006.0245
19. Bianchi VE, Locatelli V, Rizzi L. Neurotrophic and neuroregenerative effects of GH/IGF1. Int J Mol Sci. (2017) 18:E2441. doi: 10.3390/ijms18112441
20. Devesa P, Reimunde P, Gallego R, Devesa J, Arce VM. Growth hormone (GH) treatment may cooperate with locally-produced GH in increasing the proliferative response of hippocampal progenitors to kainate-induced injury. Brain Inj. (2011) 25:503–10. doi: 10.3109/02699052.2011.559611
21. Heredia M, Fuente A, Criado J, Yajeya J, Devesa J, Riolobos AS. Early growth hormone (GH) treatment promotes relevant motor functional improvement after severe frontal cortex lesion in adult rats. Behav Brain Res. (2013) 247:48–58. doi: 10.1016/j.bbr.2013.03.012
22. Ong LK, Chow WZ, TeBay C, Kluge M, Pietrogrande G, Zalewska K, et al. Growth hormone improves cognitive function after experimental stroke. Stroke (2018) 49:1257–66. doi: 10.1161/STROKEAHA.117.020557
23. Scheepens A, Sirimanne ES, Breier BH, Clark RG, Gluckman PD, Williams CE. Growth hormone as a neuronal rescue factor during recovery from CNS injury. Neuroscience (2001) 104:677–87.
24. Zhang H, Han M, Zhang X, Sun X, Ling F. The effect and mechanism of growth hormone replacement on cognitive function in rats with traumatic brain injury. PLoS ONE (2014) 9:e108518. doi: 10.1371/journal.pone.0108518
25. Guan J, Williams C, Gunning M, Mallard C, Gluckman P. The effects of IGF-1 treatment after hypoxic-ischemic brain injury in adult rats. J Cereb Blood Flow Metab. (1993) 13:609–16.
26. Kazanis I, Bozas E, Philippidis H, Stylianopoulou F. Neuroprotective effects of insulin-like growth factor-I (IGF-I) following penetrating brain injury in rats. Brain Res. (2003) 991:34–45.
27. Saatman KE, Contreras PC, Smith DH, Raghupathi R, McDermott KL, Fernandez SC, et al. Insulin-like Growth Factor-1 (IGF-1) improves both neurologic motor and cognitive outcome following experimental brain injury. Exp Neurol. (1997) 147:418–27.
28. Aberg D, Jood K, Blomstrand C, Jern C, Nilsson M, Isgaard J, et al. Serum IGF-I levels correlate to improvement of functional outcome after ischemic stroke. J Clin Endocrinol Metab. (2011) 96:E1055–64. doi: 10.1210/jc.2010-2802
29. Bondanelli M, Ambrosio MR, Onofri A, Bergonzoni A, Lavezzi S, Zatelli MC, et al. Predictive value of circulating insulin-like growth factor I levels in ischemic stroke outcome. J Clin Endocrinol Metab. (2006) 91:3928–34. doi: 10.1210/jc.2006-1040
30. Lopez-Lopez C, LeRoith D, Torres-Aleman I. Insulin-like growth factor I is required for vessel remodeling in the adult brain. Proc Natl Acad Sci USA. (2004) 101:9833–8. doi: 10.1073/pnas.0400337101
31. Watanabe T, Miyazaki A, Katagiri T, Yamamoto H, Idei T, Iguchi T. Relationship between serum insulin-like growth factor-I levels and Alzheimer's disease and vascular dementia. J Am Geriatr Soc. (2005) 53:1748–53. doi: 10.1111/j.1532-5415.2005.53524.x
32. Walter HJ, Berry M, Hill DJ, Logan A. Spatial and temporal changes in the insulin-like growth factor (IGF) axis indicate autocrine/paracrine actions of IGF-1 within wounds of the rat brain. Endocrinology (1997) 138:3024–34.
Keywords: insulin-like growth factor I, glasgow outcome scale, disability rating scale, functional independence measure, short form 36
Citation: Dubiel R, Callender L, Dunklin C, Harper C, Bennett M, Kreber L, Auchus R and Diaz-Arrastia R (2018) Phase 2 Randomized, Placebo-Controlled Clinical Trial of Recombinant Human Growth Hormone (rhGH) During Rehabilitation From Traumatic Brain Injury. Front. Endocrinol. 9:520. doi: 10.3389/fendo.2018.00520
Received: 06 December 2017; Accepted: 20 August 2018;
Published: 10 September 2018.
Edited by:
Hubert Vaudry, Université de Rouen, FranceReviewed by:
Virginie Tolle, Institut National de la Santé et de la Recherche Médicale (INSERM), FranceLin Kooi Ong, University of Newcastle, Australia
Copyright © 2018 Dubiel, Callender, Dunklin, Harper, Bennett, Kreber, Auchus and Diaz-Arrastia. This is an open-access article distributed under the terms of the Creative Commons Attribution License (CC BY). The use, distribution or reproduction in other forums is permitted, provided the original author(s) and the copyright owner(s) are credited and that the original publication in this journal is cited, in accordance with accepted academic practice. No use, distribution or reproduction is permitted which does not comply with these terms.
*Correspondence: Ramon Diaz-Arrastia, ramon.diaz-arrastia@uphs.upenn.edu