- 1Centro de Investigaciones Biomédicas, Universidad Veracruzana, Xalapa, Mexico
- 2Institute of Cellular and Integrative Neurosciences, CNRS UPR-3212, University of Strasbourg, Strasbourg, France
In mammals, the suprachiasmatic nucleus (SCN), the master circadian clock, is mainly synchronized to the environmental light/dark cycle. SCN oscillations are maintained by a molecular clockwork in which certain genes, Period 1–2, Cry1–2, Bmal1, and Clock, are rhythmically expressed. Disruption of these genes leads to a malfunctioning clockwork and behavioral and physiological rhythms are altered. In addition to synchronization of circadian rhythms by light, when subjects are exposed to food for a few hours daily, behavioral and physiological rhythms are entrained to anticipate mealtime, even in the absence of the SCN. The presence of anticipatory rhythms synchronized by food suggests the existence of an SCN-independent circadian pacemaker that might be dependent on clock genes. Interestingly, rabbit pups, unable to perceive light, suckle milk once a day, which entrains behavioral rhythms to anticipate nursing time. Mutations of clock genes, singly or in combination, affect diverse rhythms in brain activity and physiological processes, but anticipatory behavior and physiology to feeding time remains attenuated or unaffected. It had been suggested that compensatory upregulation of paralogs or subtypes genes, or even non-transcriptional mechanisms, are able to maintain circadian oscillations entrained to mealtime. In the present mini-review, we evaluate the current state of the role played by clock genes in meal anticipation and provide evidence for rabbit pups as a natural model of food-anticipatory circadian behavior.
Introduction
The suprachiasmatic nucleus (SCN), located in the ventral forebrain lateral to the third ventricle, is the master circadian pacemaker necessary for the control of endogenous physiological and behavioral rhythms in mammals (1). At the cellular level, a group of genes, known as clock genes, are necessary to generate and sustain circadian rhythms controlled by the SCN. This clock mechanism is a transcription–translation autoregulatory feedback loop with the positive arm comprised of Clock and Bmal1 genes and their proteins. CLOCK and BMAL1 proteins form heterodimers that bind to E-box enhancer elements in the promoter region of the Period (Per1–2) and Cryptochrome (Cry1–2) genes to activate their transcription. In turn, PER and CRY proteins constitute the negative arm of the loop. CLOCK and Bmal1 also activate the transcription of retinoic orphan receptor α, β, and REV-ERBα,β,γ, which form an auxiliary loop driving rhythmic Bmal1 transcription with activating and repressing actions, respectively.
In mammals, light is the main entraining signal for circadian rhythms. However, food also can be a synchronizer. When rats are fed one meal per day, within a few days they display “food-anticipatory activity” [FAA (2–4)], with arousal and an increase in locomotor behavior occurring some hours before mealtime. August Forel was the first to describe this phenomenon around one century ago, showing that bees anticipate the time of morning meals (4). In rats, in addition to increase in locomotor behavior, there is also an increase in serum levels of corticosterone and core body temperature before mealtime (5).
Food-anticipatory activity exhibits circadian properties such as limits of entrainment close to 24 h, transient cycles following phase shifts and persistence during fasting conditions [Rev (3)]. Following the discovery of the SCN as the locus of the master circadian clock, whether the SCN also served as the neural substrate of FAA was explored. Surprisingly, the anticipatory increase in locomotor activity, core body temperature, and corticosterone in food-entrained rats was not abolished by lesions of the SCN (5). This and subsequent experiments led to a search for the existence of a food-entrainable oscillator (3) distinct from the SCN light-entrainable oscillator. Many neural loci and glands were examined as potential sites regulating FAA, including the adrenal gland, several brain regions in the hypothalamus (i.e., ventromedial, dorsomedial and paraventricular nuclei, lateral preoptic area), the parabrachial nuclei, olfactory bulb (OB), hippocampal formation, cerebellum, amygdala and nucleus accumbens, among others [Rev (6, 7)]. In all cases, lesions or excision failed to abolish FAA. These studies suggested that FAA lies in a specific, unknown locus or, perhaps more likely, consists of an organized, distributed system of interacting structures both at the central and peripheral levels (7). In the present overview, we provide evidence for a role of clock genes in FAA, providing a new strategy to explore this phenomenon.
Circadian Genes Relevant to Food Anticipation
Clock genes serve as key elements for the generation of circadian oscillations in the SCN. When one of these elements is rendered non-functional, alterations in behavioral and physiological rhythms appear. Because FAA is under the control of a circadian mechanism, it is logical to hypothesize that clock gene mutations might also affect FAA (Table 1).
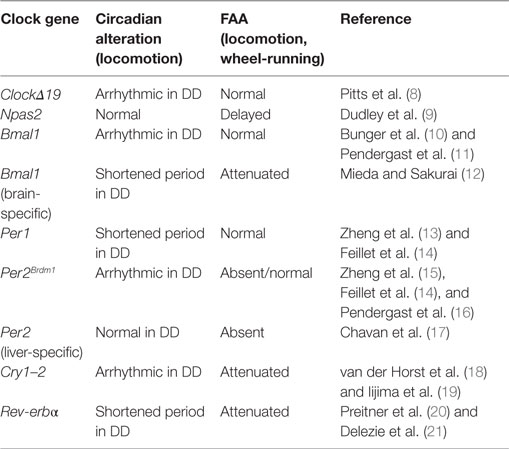
Table 1. Summary of the effects of clock genes mutations in circadian locomotor activity rhythms and food-anticipatory activity (FAA).
One of the first studies pointing to a role for clock genes in FAA comes from studies of ClockΔ19 gene mutant mice (8). The circadian locomotor behavior of these mice is arrhythmic when animals are exposed to constant darkness (DD) conditions (22, 23). When exposed to restricted-feeding schedules, ClockΔ19 mice show strong FAA and its persistence during food deprivation indicates that the Clock gene is not necessary for FAA. While this study suggested that Clock is not essential for FAA, it was later shown that the Npas2 gene can compensate for the loss of Clock, acting as a positive transcription factor in the SCN to maintain circadian oscillations (24). This finding suggested that Npas2 might be implicated in the regulation of FAA. Indeed, Npas2 mutant mice exposed to temporally restricted feeding show altered FAA, requiring two or three more days to develop FAA relative to WT animals (9). Thus, Npas2 appears to be an important gene in the regulation of FAA. However, FAA does not disappear entirely in Npas2 mutant mice, indicating that other genes contribute to the maintenance of FAA.
As mentioned previously, the positive loop of the clockwork also includes Bmal1, a gene that is rhythmically expressed in the SCN and other peripheral oscillators (25). Global mutations of Bmal1 lead to arrhythmic behavior when animals are in DD conditions (10), while FAA is normal in these animals (11). However, in one study, Bmal1 deletions confined to the dorsomedial hypothalamus eliminated FAA (26). The reason for this discrepancy is not readily clear; because methods and protocols to measure locomotor activity differ between studies, this conclusion remains to be confirmed (26, 27). Importantly, in another study using mice with a nervous system-specific deletion of Bmal1, excluding the SCN clock, it was demonstrated that FAA is strongly affected, suggesting the necessity of Bmal1 in an extra-SCN brain locus for FAA (12). Further confirming a role for Bmal1 in FAA, in Rev-erbα-mutant mice exposed to restricted-feeding schedules, FAA was negatively affected (21). Rev-erbα is a transcription factor with a repressor activity on Bmal1 (20).
With regard to the negative arm of the clockwork, double Cry gene mutations (Cry1–2) lead to arrhythmic behavior in mice held under DD (18) and FAA is markedly reduced (19). Per genes (1–2), also important components of the negative loop, are essential in the control of circadian rhythmicity. These genes are expressed rhythmically in diverse brain structures and peripheral organs [liver, heart, and lung (13, 15, 28)]. Whereas Per1−/− mutants show normal FAA, FAA is absent in Per2Brdm1-mutant mice (14). However, in a more recent examination of the same Per2 mutant mice, FAA was not altered (16). Thus, the effects of global mutations of Per2 on FAA remain to be clarified. Interestingly, when Per2 is knock down specifically in the liver, FAA is totally eliminated and can be rescued by viral overexpression of liver Per2 (17). This study indicates that FAA is not only dependent upon the brain but that it also requires normal Per2 expression in the liver for its manifestation, confirming that Per2 is likely an important component of the molecular mechanisms of FAA (Table 1).
Most studies of FAA examine rodents under a schedule of food restriction. However, most animals in their natural environments do no experience food restriction on a circadian schedule. In contrast, rabbit pups are fed for brief periods on a circadian schedule in nature and the lab. Thus, in the present contribution, we present evidence that supports notion that the rabbit pup constitutes a natural model of food entrainment.
FAA in the Rabbit
Rabbit pups are born altricial, they have no fur, their eyelids and outer ears are sealed, and they remain in the maternal burrow in darkness for the first 2 weeks of their life (29). Behavioral studies (29, 30) confirm that shortly after parturition the mother leaves the nest and returns every day with a circadian periodicity to nurse pups whether they are maintained in continuous light or in light–dark conditions (31, 32). Although parturition occurs throughout the day, the time of nursing is rapidly established on lactation day 1 and then nursing occurs every 24 h at around the same hour every night, 03:52 h across lactation days 1–15 (33, 34).
Locomotor Behavior
Although pups are not entrained by the light–dark cycle (their eyes do not open until postnatal day 10) (35), they receive periodic time cues through feeding. Every day at around the same time they ingest up to 35% of their body weight in milk (36) in around 5 min (31, 32). Hence food, in this case milk, seems to be a potent zeitgeber for rabbit pups. To explore in detail behavioral, physiological, and neural consequences of timed feeding, we scheduled nursing at two different hours, at 10:00 a.m. and at 02:00 a.m. (i.e., during the day and during the night, respectively) from postnatal (PD) 1. At PD3 (02:00 a.m. group) and PD4 (10:00 a.m. group), despite their altricial condition, pups show a significant increase in locomotor behavior 2 h before the mother’s arrival. Immediately after suckling, locomotor behavior decreases and pups remain inactive and huddled in the nest. Moreover, this locomotor increase persists for 2 days in nurse-deprived pups at the same hour of the last nursing (37).
Corticosterone
In contrast to neonatal rodents which are in a stress hyporesponsive period (38), we found that 7- to 9-day-old rabbits exhibit rhythmic secretion of corticosterone with higher plasma levels at the time of nursing, reaching a nadir 12 h later and increasing again in advance of the next nursing bout (39). Peak levels of corticosterone shift in parallel with the nursing schedule either during the day or the night and persist during fasting conditions (40, 41), indicating entrainment by time of nursing. In adult rodents this hormone reaches a peak at the time of food presentation (5, 42). See Figure 1.
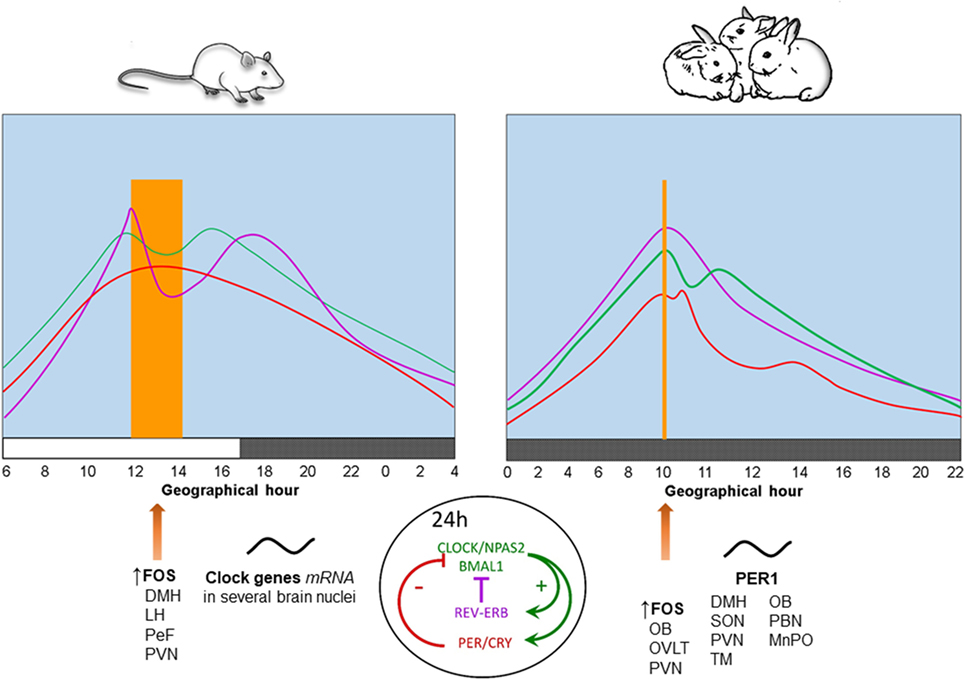
Figure 1. Physiological, behavioral, and neural changes associated with food-anticipatory activity and the molecular clockwork. Daily rhythms of locomotor activity (), corticosterone (
), and body temperature (
) increase in anticipation of the time of feeding in both species. The molecular clock is comprised of two principal feedback loops for the expression of clock genes. In the positive loop (green) the proteins CLOCK/NPAS2 and BMAL1 act on the transcription sites of Per, Cry, and Rev-erbα genes to induce their mRNA expression. Once the final proteins of PER and CRY (negative loop; red) are produced, these have the ability to repress their own transcription via an inhibitory action on the Clock-Npas2/Bmal1 dimer. The REV-ERBα protein is a transcriptional repressor for the Bmal1 gene driving rhythmic Bmal1. FOS protein expression and rhythms of clock genes and proteins in several brain nuclei synchronize to mealtime. DMH, dorsomedial hypothalamic nucleus; LH, lateral hypothalamus; MnPO, median preoptic nucleus; OB, olfactory bulb; OVLT, organum vasculosum of lamina terminalis; PeF, perifornical nucleus; paraventricular nucleus; SON, supraoptic nucleus; TM, tuberomammillary nucleus. Vertical bar and big arrow, feeding time. Figure derived from data previously published by: Angeles-Castellanos et al. (43), Caba et al. (37), Escobar et al. (44), Honma et al. (42), Jilge et al. (45), Mistlberger (7), Morgado et al. (40, 41, 46), and Rovirosa et al. (39).
Core Body Temperature
Rabbit pups maintained in constant dim light exhibit a 24-h rhythm of core body temperature with a significant anticipatory rise of 0.4–0.6°C around 3 h before daily nursing. This increase is followed by a secondary postprandial rise, followed within 1–3 h by a temperature drop. Moreover, during a 48-h fast, the anticipatory rise in temperature persists, while the postprandial increase in temperature does not (45, 47). These results indicate that the anticipatory increase is endogenous and entrained by the timing of nursing, whereas the postprandial increase is induced by food ingestion. In Figure 1, we present a comparison of daily rhythms of locomotor activity, corticosterone release and body temperature in relation to FAA in adult rodents and rabbit pups. In these species, there are changes in FOS protein, clock genes, and PER1 protein in some brain structures described further below.
Clock Genes and FAA in the Rabbit Pup
Olfactory Bulb
Postnatal day 7 pups receive temporal time cues through the brief daily visit by their mother and ingestion of a meal once a day. To successfully ingest milk, pups depend on their OB to detect the emission of a mammary pheromone (48) and to grasp the nipple; anosmic pups are unable to suckle milk and will die of starvation (49). At PD7, rhythms of the clock genes Per1, Bmal1, and Cry1 are already established in the OB, whereas a clear rhythm is not detected until PD45 in the SCN (50). The earlier maturation of the clockwork in the OB is consistent with the dependence on suckling at this age. Analysis of PER1 protein in the OB has been explored to determine the pattern of rhythms in this protein relative to the timing of suckling. At PD7, neonatal rabbits express robust rhythms of PER1 in layers of both main and accessory OBs that shift in parallel to the timing of suckling (i.e., either during the day or during the night). Moreover, PER1 expression persists during fasting conditions. Additionally, significant increases in FOS protein were detected at the time of suckling (i.e., during FAA), suggesting that the OB has a clock mechanism that anticipates nursing (51). This finding is consistent with previous work showing that the OB has an SCN-independent circadian pacemaker (52).
A milk/nipple stimulus appears to be important for OB oscillations. In this regard, the role of a mammary pheromone has been explored as an entraining signal (50); however, its importance remains unclear as the pheromone was applied at concentrations far beyond the effective concentration to elicit the oral nipple grasping response (53). Additionally, food has been explored as the entraining signal. In neonatal rabbits, the intragastric infusion of milk formula at PD7 once during the day or during the night without any maternal contact entrained rhythms of locomotor behavior and CORT, with peak values at the time of FAA. The milk stimulus also entrained rhythms of PER1 in hypothalamic nuclei (see below). These rhythms shift in parallel to the timing of milk formula infusion, demonstrating that food, in this case milk, is sufficient to entrain behavioral, physiological, and neural parameters in the neonatal rabbit (46), similar to what is seen in nursed pups. In contrast, the mammary pheromone is likely necessary for nipple detection, but not FAA.
Suprachiasmatic Nucleus
In the SCN, there is a rhythm of PER1 in nursed and fasted pups fed either during the day or the night from PD1 (37). More importantly, there is a shift in PER1 peak expression of 2.5 h between day and night nursed pups, suggesting an entraining effect of timed nursing on the pup’s SCN. A larger shift of Per1, Per2, and Bmal1 rhythms was demonstrated by shifting the time of nursing from PD4–PD7 (54). However, in this same study, there was a spontaneous advance in Per1 of around 7 h from PD3 to PD9 in pups nursed at the same time since birth. Therefore, it is not clear if the influence of ontogenetic development of the SCN on the shift in clock genes is mediated by the timing of nursing. Although retinal projections are present in the SCN at birth, the nucleus is immature in its response to a light pulse until PD12 (55). Despite methodological differences, it is possible that the pups’ SCN is sensitive to non-photic cues. The effect of food restriction on the SCN has been reported in adult rats and may be involved in the neural mechanism of food entraining (56), although, as already mentioned, this nucleus is not essential for FAA.
Other Brain Structures
In the dorsomedial hypothalamic nucleus (DMH) there is a complete phase shift of PER1 in parallel to a change in the time of nursing that persists in fasted pups (37). These results agree with publications in rodents (57, 58), indicating that the DMH might play an important role in food entrainment, although not as the unique brain structure regulating FAA (17). PER1 has been also analyzed in the median preoptic nucleus (MnPO), organum vasculosum of lamina terminalis, and medial preoptic area (59). However, a robust rhythm of PER1 is only detected in the MnPO at the time of FAA, a rhythm that persists during fasting. To our knowledge, there are no reports regarding a role of the MnPO in FAA in rodents, pointing to a need for further exploration. In the brainstem the dorsal vagal complex (DVC) and parabrachial nucleus (PBN) express PER1 in neonatal rabbits. Whereas the DVC shows rhythms related to food ingestion, the PER1 rhythm was entrained by milk intake in the PBN, a rhythm that persists during fasting (60). It is possible that changes in PER1 are due to food ingestion as the paraventricular, supraoptic, and tuberomammillar nuclei shows PER1 rhythms that shift in parallel to the timing of intragastric milk formula infusion (46).
Metabolic and Hormonal Signals and the Reward System
Metabolic fuels such as glycogen and free fatty acids follow a rhythm associated with the full and empty stomach to maintain stable glucose levels; those levels are maintained even in fasting conditions (40, 41). Interestingly, the orexigenic hormone, ghrelin, which acts on the arcuate nucleus, also follows a rhythm with peak levels 12 h after the last nursing, likely participating in triggering the next FAA episode (40, 46). Indeed, in rats under restricted feeding, plasma ghrelin levels peak before mealtime (61) and, in combination with leptin, modulates the reward circuitry by acting on dopaminergic neurons in the ventral tegmental area to reinforce FAA (62, 63).
Conclusion
Food-anticipatory activity is the expression of a circadian phenomenon in different species, usually studied in adult subjects. Here, we demonstrate that the neonatal rabbit circadian system is an ideal natural model to study the brain and molecular mechanism of FAA. FAA depends, in part, on some clock genes expressed in a circadian network of brain structures, oscillating in synchrony, and coordinated by the SCN. Combining information on brain clock gene expression in rabbit pups with mouse models of clock gene mutations for the study of FAA will help increase understanding of the molecular mechanisms implicated in food anticipation in the wild.
Author Contributions
MC and JM contributed to the writing of the manuscript and approved the final version.
Conflict of Interest Statement
The authors declare that the research was conducted in the absence of any commercial or financial relationships that could be construed as a potential conflict of interest.
The reviewer OV and handling Editor declared their shared affiliation.
Acknowledgments
The authors gratefully acknowledge Manuel Hernandez Pérez for their invaluable help in preparing Figure and table and to Dr. Antonio N Nunez, Dr. Alison Fleming, and Dr. Lance Kriegsfeld for corrections.
Funding
JM is supported by Agence Nationale de la Recherche (grant ANR-14-CE13-0002-01 ADDiCLOCK JCJC) and the Institut Danone France-Fondation pour la Recherche Médicale Consortium.
References
1. Moore RY. The suprachiasmatic nucleus and the circadian timing system. In: Guillette MU editor. Progress in Molecular Biology and Translational Science. (Vol. 119), Elsevier (2013). p. 1–28.
2. Mistlberger RE. Circadian food-anticipatory activity: formal models and physiological mechanisms. Neurosci Biobehav Rev (1994) 18:171–95. doi:10.1016/0149-7634(94)90023-X
3. Stephan FK. The “other” circadian system: food as a zeitgeber. J Biol Rhythms (2002) 17:284–92. doi:10.1177/074873002129002591
4. Antle MC, Silver R. Neural basis of timing and anticipatory behaviors. Eur J Neurosci (2009) 30:1643–9. doi:10.1111/j.1460-9568.2009.06959.x
5. Krieger DT, Hauser LC. Suprachiasmatic nuclear lesions do not abolish food-shifted circadian adrenal and temperature rhythmicity. Science (1977) 197:398–9. doi:10.1126/science.877566
6. Davidson AJ. Lesion studies targeting food-anticipatory activity. Eur J Neurosci (2009) 30:1658–64. doi:10.1111/j.1460-9568.2009.06961.x
7. Mistlberger RE. Neurobiology of food anticipatory circadian rhythms. Physiol Behav (2011) 104:535–45. doi:10.1016/j.physbeh.2011.04.015
8. Pitts S, Perone E, Silver R. Food-entrained circadian rhythms are sustained in arrhythmic Clk/Clk mutant mice. Am J Physiol Regul Integr Comp Physiol (2003) 285:R57–67. doi:10.1152/ajpregu.00023.2003
9. Dudley CA, Erbel-Sieler C, Estill SJ, Reick M, Franken P, Pitts S, et al. Altered patterns of sleep and behavioral adaptability in NPAS2-deficient mice. Science (2003) 301(5631):379–83. doi:10.1126/science.1082795
10. Bunger MK, Wilsbacher LD, Moran SM, Clendenin C, Radcliffe LA, Hogenesch JB, et al. Mop3 is an essential component of the master circadian pacemaker in mammals. Cell (2000) 103(7):1009–17. doi:10.1016/S0092-8674(00)00205-1
11. Pendergast JS, Nakamura W, Friday RC, Hatanaka F, Takumi T, Yamazaki S. Robust food anticipatory activity in BMAL1-deficient mice. PLoS One (2009) 4(3):e4860. doi:10.1371/journal.pone.0004860
12. Mieda M, Sakurai T. Bmal1 in the nervous system is essential for normal adaptation of circadian locomotor activity and food intake to periodic feeding. J Neurosci (2011) 31(43):15391–6. doi:10.1523/JNEUROSCI.2801-11.2011
13. Zheng B, Albrecht U, Kaasik K, Sage M, Lu W, Vaishnav S, et al. Nonredundant roles of the mPer1 and mPer2 genes in the mammalian circadian clock. Cell (2001) 105(5):683–94. doi:10.1016/S0092-8674(01)00380-4
14. Feillet CA, Ripperger J, Magnone MC, Dulloo A, Albrecht U, Challet E. Lack of food anticipation in Per2 mutant mice. Curr Biol (2006) 16:2016–22. doi:10.1016/j.cub.2006.08.053
15. Zheng B, Larkin DW, Albrecht U, Sun ZS, Sage M, Eichele G, et al. The mPer2 gene encodes a functional component of the mammalian circadian clock. Nature (1999) 400(6740):169–73. doi:10.1038/22118
16. Pendergast JS, Wendroth RH, Stenner RC, Keil CD, Yamazaki S. mPeriod2 (Brdm1) and other single Period mutant mice have normal food anticipatory activity. Sci Rep (2017) 7(1):15510. doi:10.1038/s41598-017-15332-6
17. Chavan R, Feillet C, Costa SS, Delorme JE, Okabe T, Ripperger JA, et al. Liver-derived ketone bodies are necessary for food anticipation. Nat Commun (2016) 7:10580. doi:10.1038/ncomms10580
18. van der Horst GT, Muijtjens M, Kobayashi K, Takano R, Kanno S, Takao M, et al. Mammalian Cry1 and Cry2 are essential for maintenance of circadian rhythms. Nature (1999) 398(6728):627–30. doi:10.1038/19323
19. Iijima M, Yamaguchi S, van der Horst GT, Bonnefont X, Okamura H, Shibata S. Altered food-anticipatory activity rhythm in Cryptochrome-deficient mice. Neurosci Res (2005) 52:166–73. doi:10.1016/j.neures.2005.03.003
20. Preitner N, Damiola F, Lopez-Molina L, Zakany J, Duboule D, Albrecht U, et al. The orphan nuclear receptor REV-ERBalpha controls circadian transcription within the positive limb of the mammalian circadian oscillator. Cell (2002) 110(2):251–60. doi:10.1016/S0092-8674(02)00825-5
21. Delezie J, Dumont S, Sandu C, Reibel S, Pevet P, Challet E. Rev-erbalpha in the brain is essential for circadian food entrainment. Sci Rep (2016) 6:29386. doi:10.1038/srep29386
22. Vitaterna MH, King DP, Chang AM, Kornhauser JM, Lowrey PL, McDonald JD, et al. Mutagenesis and mapping of a mouse gene, clock, essential for circadian behavior. Science (1994) 264(5159):719–25. doi:10.1126/science.8171325
23. McClung CA, Sidiropoulou K, Vitaterna M, Takahashi JS, White FJ, Cooper DC, et al. Regulation of dopaminergic transmission and cocaine reward by the Clock gene. Proc Natl Acad Sci U S A (2005) 102(26):9377–81. doi:10.1073/pnas.0503584102
24. Debruyne JP, Noton E, Lambert CM, Maywood ES, Weaver DR, Reppert SM. A clock shock: mouse CLOCK is not required for circadian oscillator function. Neuron (2006) 50(3):465–77. doi:10.1016/j.neuron.2006.03.041
25. Takahashi JS. Molecular components of the circadian clock in mammals. Diabetes Obes Metab (2015) 17(Suppl 1):6–11. doi:10.1111/dom.12514
26. Fuller PM, Lu J, Saper CB. Differential rescue of light- and food-entrainable circadian rhythms. Science (2008) 320(5879):1074–7. doi:10.1126/science.1153277
27. Mistlberger RE, Kent BA, Landry GJ. Phenotyping food entrainment: motion sensors and telemetry are equivalent. J Biol Rhythms (2009) 24(1):95–8. doi:10.1177/0748730408329573
28. Shearman LP, Sriram S, Weaver DR, Maywood ES, Chaves I, Zheng B, et al. Interacting molecular loops in the mammalian circadian clock. Science (2000) 288(5468):1013–9. doi:10.1126/science.288.5468.1013
29. Broekhuizen S, Mulder JL. Differences and similarities in nursing behaviour of hares and rabbits. Acta Zool Fenn (1983) 174:61–3.
30. Caba M, Gonzalez-Mariscal G. The rabbit pup, a natural model of nursing-anticipatory activity. Eur J Neurosci (2009) 30:167–1706. doi:10.1111/j.1460-9568.2009.06964.x
31. Jilge B. The ontogeny of circadian rhythms in the rabbit. J Biol Rhythms (1993) 8:247–60. doi:10.1177/074873049300800307
32. Jilge B. Ontogeny of the rabbit’s circadian rhythms without an external zeitgeber. Physiol Behav (1995) 58:131–40. doi:10.1016/0031-9384(95)00006-5
33. González-Mariscal G, Lemus AC, Vega-Gonzalez A, Aguilar-Roblero R. Litter size determines circadian periodicity of nursing in rabbits. Chronobiol Int (2013) 30:711–8. doi:10.3109/07420528.2013.784769
34. González-Mariscal G, Caba M, Martínez-Gómez M, Bautista A, Hudson R. Mothers and offspring: the rabbit as a model system in the study of mammalian maternal behavior and sibling interactions. Horm Behav (2016) 77:30–41. doi:10.1016/j.yhbeh.2015.05.011
35. Rapisardi SC, Chow KL, Mathers LH. Ontogenesis of receptive field characteristics in the dorsal lateral geniculate nucleus of the rabbit. Exp Brain Res (1975) 22:295–305. doi:10.1007/BF00234771
36. Caba M, Rovirosa MJ, Silver R. Suckling and genital stroking induces Fos expression in hypothalamic oxytocinergic neurons of rabbit pups. Brain Res Dev Brain Res (2003) 143:119–28. doi:10.1016/S0165-3806(03)00064-6
37. Caba M, Tovar A, Silver R, Morgado E, Meza E, Zavaleta Y, et al. Nature’s food anticipatory experiment: entrainment of locomotor behavior, suprachiasmatic and dorsomedial hypothalamic nuclei by suckling in rabbit pups. Eur J Neurosc (2008) 27:432–43. doi:10.1111/j.1460-9568.2008.06017.x
38. Levine S. The ontogeny of the hypothalamic-pituitary-adrenal axis. The influence of maternal factors. Ann NY Acad Sci (1994) 746:275–88. doi:10.1111/j.1749-6632.1994.tb39245.x
39. Rovirosa MJ, Levine S, Gordon MK, Caba M. Circadian rhythm of corticosterone secretion in the neonatal rabbit. Brain Res Dev Brain Res (2005) 8:92–6. doi:10.1016/j.devbrainres.2005.06.007
40. Morgado E, Gordon MK, Miñana-Solis MC, Meza E, Levine S, Escobar C, et al. Hormonal and metabolic rhythms associated with the daily scheduled nursing in rabbit pups. Am J Physiol Regul Integr Comp Physiol (2008) 295(2):R690–5. doi:10.1152/ajpregu.00162.2008
41. Morgado E, Meza E, Gordon MK, Pau FKY, Juárea C, Caba M. Persistence of hormonal and metabolic rhythms during fasting in 7- to 9-day old rabbits entrained by nursing during the night. Horm Behav (2010) 58:465–72. doi:10.1016/j.yhbeh.2010.05.003
42. Honma K-I, Noe Y, Noe Y, Honma S, Katsuno Y, Hiroshige T. Roles of paraventricular catecholamines in feeding-associated corticosterone rhythm in rats. Am J Physiol Endocrinol Metab (1992) 25:E948–55. doi:10.1152/ajpendo.1992.262.6.E948
43. Angeles-Castellanos M, Aguilar-Roblero R, Escobar C. c-Fos expression in hypothalamic nuclei of food-entrained rats. Am J Physiol Regul Integr Comp Physiol (2004) 286:R158–65. doi:10.1152/ajpregu.00216.2003
44. Escobar C, Martínez-Merlos MT, Angeles-Castellanos M, Miñana MC, Buijs R. Unpredictable feeding schedules unmask a system for daily resetting of behavioural and metabolic food entrainment. Eur J Neurosci (2007) 26:2804–14. doi:10.1111/j.1460-9568.2007.05893.x
45. Jilge B, Kuhnt B, Landerer W, Rest S. Circadian thermoregulation in suckling rabbit pups. J Biol Rhythms (2000) 15:329–35. doi:10.1177/074873000129001431
46. Morgado E, Juárez C, Melo AI, Domínguez B, Lehman MN, Escobar C, et al. Artificial feeding synchronizes behavioral, hormonal, metabolic and neural parameters in mother-deprived neonatal rabbit pups. Eur J Neurosci (2011) 34:1807–16. doi:10.1111/j.1460-9568.2011.07898.x
47. Trejo-Muñoz L, Navarrete E, Montúfar-Chaveznava R, Caldelas I. Determining the period, phase and anticipatory component of activity and temperature patterns in newborn rabbits that were maintained under a daily nursing schedule and fasting conditions. Physiol Behav (2012) 106:587–96. doi:10.1016/j.physbeh.2012.04.005
48. Schaal B, Coureaud G, Langlois D, Giniès C, Sémon E, Perrier G. Chemical and behavioural characterization of the rabbit mammary pheromone. Nature (2003) 424:68–72. doi:10.1038/nature01739
49. Distel H, Hudson R. The contribution of the olfactory and tactile modalities to the nipple-search behaviour of newborn rabbits. J Comp Physiol A (1985) 157:599–605. doi:10.1007/BF01351354
50. Montúfar-Chaveznava R, Trejo-Muñoz L, Hernández-Campos O, Navarrete E, Caldelas I. Maternal olfactory cues synchronize the circadian system of artificially raised newborn rabbits. PLoS One (2013) 8(9):e74048. doi:10.1371/journal.pone.0074048
51. Nolasco N, Juárez C, Morgado E, Meza E, Caba M. A circadian clock in the olfactory bulb anticipates feeding during food anticipatory activity. PLoS One (2011) 7(10):e47779. doi:10.1371/journal.pone.0047779
52. Granados-Fuentes D, Prolo LM, Abraham U, Herzog ED. The suprachiasmatic nucleus entrains but does not sustain, circadian rhythmicity in the olfactory bulb. J Neurosci (2004) 24:615–9. doi:10.1523/JNEUROSCI.4002-03.2004
53. Coureaud G, Langlois D, Sicard G, Schaal B. Newborn rabbit responsiveness to the mammary pheromone is concentration-dependent. Chem Senses (2004) 29:341–50. doi:10.1093/chemse/bjh037
54. Caldelas I, González B, Montúfar-Chaveznava R, Hudson R. Endogenous clock gene expression in the suprachiasmatic nuclei of previsual newborn rabbits is entrained by nursing. Dev Neurobiol (2009) 69:47–59. doi:10.1002/dneu.20687
55. Juárez C, Morgado E, Meza E, Waliszewski SM, Aguilar-Rbolero R, Caba M. Development of retinal projections and response to photic input in the suprachiasmatic nucleus of New Zealand white rabbits. Brain Res (2013) 1499:21–8. doi:10.1016/j.brainres.2013.01.010
56. Acosta-Galvan G, CX Y, van der Vliet J, Jhamandas JH, Panula P, Angeles-Castellanos M, et al. Interaction between hypothalamic dorsomedial nucleus and the suprachiasmatic nucleus determines intensity of food anticipatory behavior. Proc Natl Acad Sci U S A (2011) 108:5813–8. doi:10.1073/pnas.1015551108
57. Gooley JJ, Schomer A, Saper CB. The dorsomedial hypothalamic nucleus is critical for the expression of food-entrainable circadian rhythms. Nat Neurosci (2006) 9:398–407. doi:10.1038/nn1651
58. Mieda M, Williams SC, Richardson JA, Tanaka K, Yanagisawa M. The dorsomedial hypothalamic nucleus as a putative food-entrainable circadian pacemaker. Proc Natl Acad Sci U S A (2006) 103:12150–5. doi:10.1073/pnas.0604189103
59. Moreno ML, Meza E, Ortega A, Caba M. The median preoptic nucleus exhibits circadian regulation and is involved in food anticipatory activity in rabbit pups. Chronobiol Int (2014) 31:515–22. doi:10.3109/07420528.2013.874354
60. Juárez C, Morgado E, Waliszewski SM, Martínez AJ, Meza E, Caba M. Synchronization of PER1 protein in parabrachial nucleus in a natural model of food anticipatory activity. Eur J Neurosci (2012) 35:1458–65. doi:10.1111/j.1460-9568.2012.08051.x
61. Patton DF, Katsuyama AM, Pavlovski I, Michalik M, Patterson Z, Parfyonov M, et al. Circadian mechanisms of food anticipatory rhythms in rats fed once or twice daily: clock gene and endocrine correlates. PLoS One (2014) 9(12):e112451. doi:10.1371/journal.pone.0112451
62. van Zessen R, van der Plasse G, Adan RA. Contribution of the mesolimbic dopamine system in mediating the effects of leptin and ghrelin on feeding. Proc Nutr Soc (2012) 71:435–45. doi:10.1017/S0029665112000614
Keywords: circadian rhythms, clock gene mutant, restricted feeding, food entrainment, corticosterone, PER1 protein
Citation: Caba M and Mendoza J (2018) Food-Anticipatory Behavior in Neonatal Rabbits and Rodents: An Update on the Role of Clock Genes. Front. Endocrinol. 9:266. doi: 10.3389/fendo.2018.00266
Received: 29 January 2018; Accepted: 07 May 2018;
Published: 24 May 2018
Edited by:
Jacques Epelbaum, Institut National de la Santé et de la Recherche Médicale (INSERM), FranceReviewed by:
Odile Viltart, INSERM U894 Centre de Psychiatrie et Neurosciences, FranceBen Nephew, Tufts University, United States
Copyright: © 2018 Caba and Mendoza. This is an open-access article distributed under the terms of the Creative Commons Attribution License (CC BY). The use, distribution or reproduction in other forums is permitted, provided the original author(s) and the copyright owner are credited and that the original publication in this journal is cited, in accordance with accepted academic practice. No use, distribution or reproduction is permitted which does not comply with these terms.
*Correspondence: Mario Caba, bWNhYmFAdXYubXg=