- 1Department of Medicine, Division of Clinical Pharmacology, Vanderbilt Center for Bone Biology, Vanderbilt University Medical Center, Nashville, TN, United States
- 2Bone Biology and Disease Unit, St. Vincent’s Institute of Medical Research, Fitzroy, VIC, Australia
- 3Department of Medicine at St. Vincent’s Hospital, University of Melbourne, Melbourne, VIC, Australia
- 4Cellular Orthopaedic Laboratory, School of Biomedical Sciences, The University of Western Australia, Crawley, WA, Australia
Parathyroid hormone-related protein (PTHrP) expression in breast cancer is enriched in bone metastases compared to primary tumors. Human MCF7 breast cancer cells “home” to the bones of immune deficient mice following intracardiac inoculation, but do not grow well and stain negatively for Ki67, thus serving as a model of breast cancer dormancy in vivo. We have previously shown that PTHrP overexpression in MCF7 cells overcomes this dormant phenotype, causing them to grow as osteolytic deposits, and that PTHrP-overexpressing MCF7 cells showed significantly lower expression of genes associated with dormancy compared to vector controls. Since early work showed a lack of cyclic AMP (cAMP) response to parathyroid hormone (PTH) in MCF7 cells, and cAMP is activated by PTH/PTHrP receptor (PTHR1) signaling, we hypothesized that the effects of PTHrP on dormancy in MCF7 cells occur through non-canonical (i.e., PTHR1/cAMP-independent) signaling. The data presented here demonstrate the lack of cAMP response in MCF7 cells to full length PTHrP(1–141) and PTH(1–34) in a wide range of doses, while maintaining a response to three known activators of adenylyl cyclase: calcitonin, prostaglandin E2 (PGE2), and forskolin. PTHR1 mRNA was detectable in MCF7 cells and was found in eight other human breast and murine mammary carcinoma cell lines. Although PTHrP overexpression in MCF7 cells changed expression levels of many genes, RNAseq analysis revealed that PTHR1 was unaltered, and only 2/32 previous PTHR1/cAMP responsive genes were significantly upregulated. Instead, PTHrP overexpression in MCF7 cells resulted in significant enrichment of the calcium signaling pathway. We conclude that PTHR1 in MCF7 breast cancer cells is not functionally linked to activation of the cAMP pathway. Gene expression responses to PTHrP overexpression must, therefore, result from autocrine or intracrine actions of PTHrP independent of PTHR1, through signals emanating from other domains within the PTHrP molecule.
Introduction
Parathyroid hormone-related protein (PTHrP, gene name PTHLH/Pthlh) is a cytokine with functions in both pathology and physiology (1, 2). Although it was identified as the circulating factor responsible for humoral hypercalcemia of malignancy (3), it more commonly acts in a paracrine manner: in breast cancer cells it promotes their metastasis (4, 5), in bone (osteoblasts and osteocytes) it stimulates bone formation (6, 7), and in cartilage cells (chondrocytes) it controls proliferation and hypertrophy (8).
In breast cancer cells that lay dormant in bone (9) we have previously shown that overexpression of PTHrP enables otherwise dormant human MCF7 breast cancer cells to aggressively colonize the bone marrow and induce osteolysis (5). Consistent with enhanced bone colonization, we recently reported that such overexpression of PTHrP in MCF7 cells results in the downregulation of several pro-dormancy genes (9).
The best understood actions of PTHrP are those that are mediated by its binding to the G protein-coupled receptor that it shares with parathyroid hormone (PTH) (PTHR1). Upon ligand binding to the receptor, cyclic AMP (cAMP) is activated, followed by protein kinase A (PKA) activation, cAMP responsive element binding protein (CREB) phosphorylation, and transcription of CREB target genes (10–13). This PTHR1-dependent signaling pathway is shared between PTH and PTHrP due to high sequence homology in their amino-terminal domains; the portion of the molecule that interacts with the receptor (14). Early work showed that MCF7 cells failed to respond to PTH treatment with any increase in cAMP or activation of cAMP-dependent protein kinase, suggesting that PTHR1 in those cells is not functionally linked to adenylyl cyclase (15). In contrast, MCF7 cells possess specific, high affinity receptors for calcitonin linked to adenylyl cyclase activation, and activate cAMP in response to prostaglandin E2 (15, 16). These data suggest that the effect of PTHrP overexpression on tumor dormancy in MCF7 cells may occur through PTHR1-independent actions of the PTHrP molecule.
Using multiple assays, we report here that MCF7 cells, and many other breast cancer cell lines, express PTHR1 mRNA but do not bind PTH, nor do they activate cAMP formation or subsequent cAMP signaling events in response to PTH or PTHrP. Our RNAseq analyses identify many genes induced by PTHrP overexpression in MCF7 cells, and several potential alternative pathways, notably those related to calcium signaling.
Materials and Methods
Cell Culture
Human MCF7 cells were obtained from ATCC and grown in DMEM supplemented with 10% FBS and penicillin/streptomycin (P/S). MCF7pcDNA and MCF7 PTHrP-overexpressing cells were generated as described previously (5) and grown in the same conditions as MCF7 cells; we utilized strains grown and maintained at two separate institutions to validate findings. All breast cancer and mouse mammary carcinoma cell lines were obtained and grown as previously described (9). The rat osteosarcoma (UMR106-01) cell line was maintained in DMEM supplemented with 10% FBS and P/S as described in Ref. (17). MC3T3-E1 cells were maintained in α-MEM supplemented with 10% FBS as described in Ref. (18).
cAMP Response Assay
Briefly, MCF7 cells were cultured in 12-well plate in cell culture media containing 1 mM isobutylmethylxanthine. Cells were then treated for 12 min with either PTH (100 nM) (sourced from Bachem, Bubendorf, Switzerland), PTHrP(1–141) (100 nM) [expressed in Escherichia coli and purified in house (7)], or the known agonists forskolin (10 µM) (sourced from Sigma), prostaglandin E2 (1 µM) (sourced from Sigma), or salmon calcitonin (sCT) (1 µM) (kindly gifted by the late Dr. M Azria, Novartis AG, Basel, Switzerland). The cells were washed, acidified ethanol was added, and after air drying was reconstituted in assay buffer and cAMP formation assayed as previously (19).
CRE-Luciferase Assay
MCF7 cells were transiently transfected with cAMP response element (pCRE)-luciferase (Clontech), a vector containing multiple copies of CRE binding sequences. Fugene (Promega) was used to transfect cells. Four hours after agonist stimulation, cells were lysed, substrate (Promega) was added, and signal was measured using a Polarstar Optima.
Real-Time Quantitative PCR
Cell lines were harvested in TRIzol (Life Technologies) or TriSure (Bioline) for phenol/chloroform extraction of RNA, DNAse digested (TURBO DNA-free kit, Life Technologies), and cDNA was synthesized from 200 ng–1 µg RNA (iScript cDNA synthesis kit, Bio-Rad or Tetro cDNA synthesis kit, Bioline) per the manufacturer’s instructions as previously described (9). Real-time PCR was performed on either a Quantstudio5 384-well plate format (Thermo Fisher) or Stratagene MX3000P (Agilent) with the following cycling conditions: 2 min at 50°C, 10 min at 95°C, (15 s at 95°C, 1 min at 60°C) × 40 cycles, and dissociation curve (15 s at 95°C, 1 min at 60°C, 15 s at 95°C) or 10 min at 95°C, (30 s at 95°C, 1 min at 60°C) × 40 cycles, and dissociation curve (1 min at 95°C, 30 s at 55°C, 30 s at 95°C). Primers for mouse PTHR1 were previously published (20) and human PTHR1 primer sequences were sourced from MGH Primerbank (F: CTGGGCATGATTTACACCGTG, R: CAGTGCAGCCGCCTAAAGTA). Human PTHLH primers were previously published (21) and human HPRT1, RGS2, CREB, PRKAR1, AREG, and NR4A1 primers were previously published (22). Primer sequences for human BDKRB1 and CALML3 were designed using PrimerBLAST (BDKRB1 F: AATGCTACGGCCTGTGACAA, R: TCCCTAGGAGGCCGAAGAAA; CALML3 F: TGGTTGATTCAGCCCACCTC, R: TCCGTGTCATTCAGACGAGC). Gene expression between samples was normalized to B2M expression or B2M: HPRT1 geometric mean. Relative expression was quantified using the comparative CT method [2−(Gene Ct–Normalizer Ct)].
Confocal Microscopy
Antibodies and Reagents
Tetramethylrhodamine (TMR)-labeled PTH(1–34) (PTH-TMR) was synthesized as previously described (23). Anti-VPS35 mouse monoclonal was purchased from Santa Cruz Biotechnology Inc., USA. Alexa Fluor 488 anti-mouse secondary antibody was purchased from Molecular Probes®, Invitrogen, USA.
Imaging
MCF7 and UMR106-01 cells were cultured as described above, and seeded on poly-l-lysine-coated glass coverslips at 1 × 104 cells/well (96-well plate) for 24–48 h prior to agonist stimulation. Cells were then serum starved for 1 h prior to the addition of PTH-TMR (100 nM) for 15 min at 37°C. Cells were then washed in ice-cold 1× PBS and fixed in 4% PFA at room temperature, permeabilized with 0.1% Triton X-100 for 5 min, washed in 0.2% BSA-PBS, and blocked in 3% BSA-PBS for 30 min. Cells were then incubated with anti-VPS35 antibody (Santa Cruz Biotechnology Inc.) for 1 h at room temperature, and washed in 0.2% BSA-PBS and 1× PBS prior to incubation with Alexa Fluor 488 anti-mouse secondary antibody (Molecular Probes®, Invitrogen), for 45 min at room temperature. Cells were then stained with DAPI stain and mounted in ProLong® Diamond Antifade (Molecular Probes®, Invitrogen). Detection of immunofluorescence was performed using a Nikon A1Si confocal microscope running NIS-C Elements Software (Nikon Corp., Japan). A 40× oil immersion objective lens (Nikon, Japan) was used, where serial optical sections (z-stack) of 0.5–1 µm were used to reconstruct 2D projections in FIJI (NIH, USA).
RNA Sequencing and Bioinformatics
RNA samples of MCF7pcDNA control and MCF7 PTHrP-overexpressing cells (n = 3 independent replicates/group) were submitted to the Stanford Functional Genomics Facility and analyzed for RNA integrity using a Bioanalyzer (Eukaryote Total RNA Nano, Agilent) and all samples had a RNA integrity number of 9.50–10 (10 is highest quality possible). RNA samples were sequenced on an Illumina NextSeq with coverage of approximately 40 million reads per sample. Sequence alignment and RNAseq bioinformatics analysis was performed by the Vanderbilt Technologies for Advanced Genomics Analysis and Research Design (VANGARD) core at Vanderbilt University Medical Center. RNAseq files are available in the GEO repository (GEO accession number GSE110713).
Statistics
All data are presented as the mean of n = 3 biological replicates obtained from three independent experiments (one biological replicate, with three technical replicates per experiment). For all graphs error bars indicate the SEM. Statistical tests used are indicated in the figure legends, and p-values were considered significant at p < 0.05.
Results
PTHR1 mRNA Is Detected in Breast Cancer Cells
PTHR1 mRNA levels varied but were detectable across all human breast cancer and mouse mammary carcinoma cell lines tested (Figure 1). The panel included cell lines termed “high metastatic potential” [that aggressively colonize the bone after intracardiac inoculation or lung after tail vein inoculation (9)], and cell lines termed “Low metastatic potential” (9) [that do not colonize, or proliferate very slowly after inoculation (9)]. PTHR1 mRNA levels did not correspond to the metastatic potential of the cell lines. 4T1 and D2A1 cells had the lowest expression of PTHR1, which was nearly undetectable (4T1: Ct values = 33–39; D2A1: Ct values = 33–34). All breast cancer cell lines had at least 10-fold lower PTHR1 mRNA levels than MC3T3-E1 cells, which have a robust cAMP response to exogenous PTH and PTHrP treatment (24).
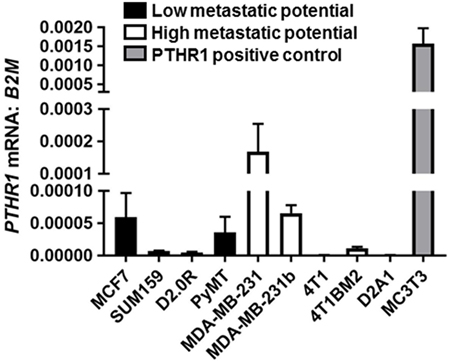
Figure 1. PTHR1 is expressed by breast cancer cells. PTHR1 mRNA levels in human breast cancer cell lines {MCF7, SUM159, MDA-MB-231, MDA-MB-231b [bone metastatic clone (25, 26)]}, mouse mammary carcinoma cell lines {D2.0R, PyMT, 4T1, 4T1BM2 [bone metastatic clone (27)], D2A1}, classified according to metastatic potential, and PTHR1/cyclic AMP responsive MC3T3-E1 cells. mRNA levels were normalized to β-2-microglobulin (B2M) housekeeping gene. Graphs = mean + SE. n = 3 replicates from independent experiments.
Neither PTH nor PTHrP Stimulates cAMP in Breast Cancer Cells
MCF7 cells robustly induced cAMP formation in response to forskolin, PGE2, and sCT, but treatment with high dose PTH(1–34) or PTHrP(1–141) elicited no cAMP response (Figure 2A). This confirmed the lack of a cAMP response to PTH in MCF7 cells as reported at the time of discovery of the functional calcitonin receptor (15). In order to investigate later cellular responses, MCF7 cells were transiently transfected with a cAMP response element (CRE)-luciferase construct (CRE-Luc). Treatment with either sCT or PGE2 resulted in substantial activation of the CRE-Luc reporter, with no detectable effect of PTH(1–34). All were used at multiple doses in repeated experiments, with no measureable effects detected (Figure 2B).
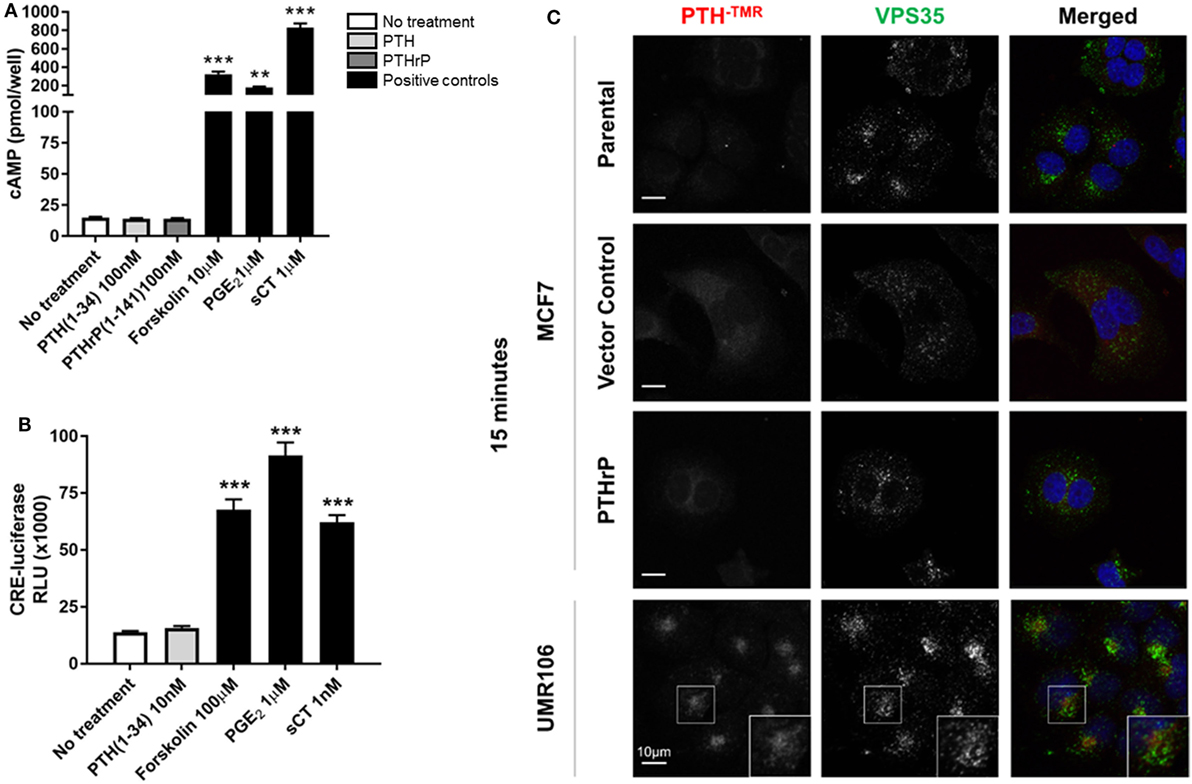
Figure 2. Neither parathyroid hormone (PTH) nor parathyroid hormone-related protein (PTHrP) bind to/activate cyclic AMP (cAMP) in MCF7 cells. (A) cAMP production in MCF7 cells following 12 min stimulation with PTH(1–34) or PTHrP(1–141), or positive controls forskolin, prostaglandin E2 (PGE2), or salmon calcitonin (sCT). Graphs = mean + SE. n = 3 replicates from independent experiments. **p < 0.01, ***p < 0.001 vs no treatment by one-way ANOVA with multiple comparisons. (B) cAMP response element (CRE)-luciferase signal following 4 h stimulation with PTH or positive controls forskolin, prostaglandin E2 (PGE2), or sCT. Graphs = mean + SE. n = 3 replicates from independent experiments. ***p < 0.001 vs no treatment by one-way ANOVA with multiple comparisons. (C) Confocal images of stable MCF7 and UMR106-01 cells cultured on poly-l-lysine-coated glass coverslips and serum starved for 1 h prior to the addition of tetramethylrhodamine-labeled PTH(1–34) (PTH-TMR, 100 nM) for 15 min at 37°C. Cells were fixed in 4% PFA and immunostained for the endogenous retromer subunit, vacuolar protein sorting 35 (VPS35). Scale bar, 10 µm. Representative of n = 3 independent experiments.
Tetramethylrhodamine-labeled PTH (PTH-TMR) has proven useful for monitoring the surface binding and internalization of amino-terminal PTH upon its target cells through the PTHR1 (23). Vacuolar protein sorting 35 (VPS35) is an essential subunit of the mammalian retromer trafficking complex, where retromer coordinates both retrograde (endosome-to-Golgi) and recycling (endosome-to-plasma membrane) of many cell surface receptors (28), including PTHR1 (23, 29) along the endocytic pathway. VPS35, therefore, serves as a marker of internalized PTH-TMR–PTHR1 ligand-receptor complexes following their sequestration into early endosomes (23). Accordingly, the addition of PTH-TMR at saturating conditions (100 nM) for 15 min to UMR106-01 cells, was sufficient to visualize encapsulated ligand–receptor complexes in early endosomes, as determined by its co-localization with VPS35 (Figure 2C). This event coincides with the generation of cAMP following stimulation with either PTH and PTHrP peptides with identical dose responses (19). In contrast, neither PTH-TMR internalization nor co-localization with VPS35 was detected in MCF7 parental, vector-transfected, or PTHrP-transfected cells (Figure 2C).
Lack of cAMP Gene Response in MCF7 Cells
In order to identify novel dormancy genes regulated by PTHrP, we used RNAseq to analyze which pathways are activated in response to PTHrP overexpression in MCF7 cells. We identified >2,500 genes differentially regulated with a log2 fold change >1 and p < 0.05 in MCF7 PTHrP-overexpressing vs MCF7 control cells (Figure 3A). Consistent with our finding that neither PTH nor PTHrP induce cAMP formation or early post-receptor activation events in MCF7 cells, RNAseq analysis confirmed that only 2 of a previously described panel of 32 CREB-responsive genes (22) were significantly upregulated in MCF7 PTHrP-overexpressing cells (Table 1). Three CREB-responsive genes were significantly downregulated, and the remaining 27 were not altered by PTHrP over-expression, confirming that even long term overexpression of PTHrP does not induce genes that result from cAMP signaling in MCF7 cells.
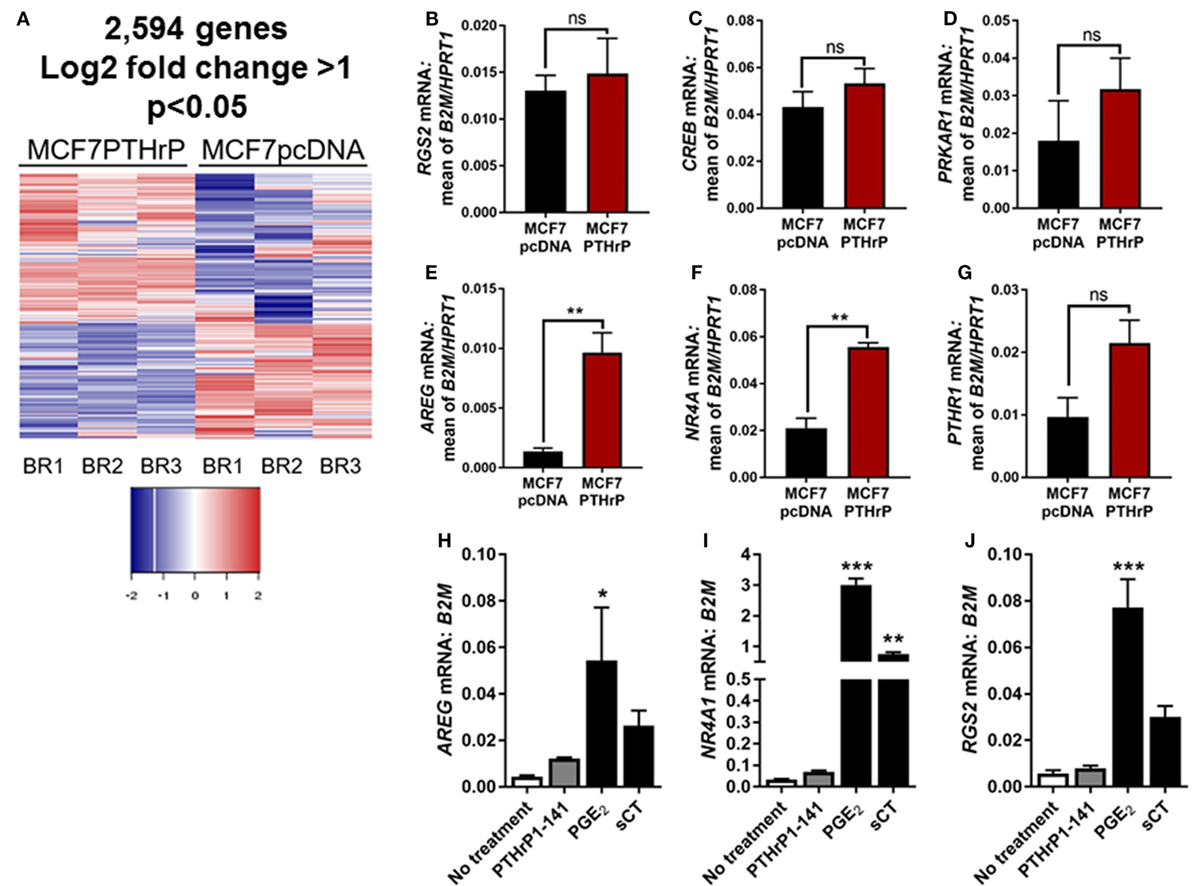
Figure 3. Parathyroid hormone-related protein (PTHrP) overexpression does not induce cyclic AMP (cAMP) target genes. (A) Heat map of gene expression with 95% confidence intervals in MCF7pcDNA (empty vector control) or MCF7 PTHrP-overexpressing cells. BR1 = biological replicate 1, BR2 = biological replicate 2, BR3 = biological replicate 3. (B–G) qPCR for cAMP target genes in MCF7pcDNA or MCF7 PTHrP-overexpressing cells. mRNA levels were normalized to the geometric mean of B2M and HPRT1 housekeeping genes. Graphs = mean + SE. **p < 0.01 by unpaired Student’s T-test. (H–J) qPCR for cAMP target genes in MCF7 cells following stimulation with PTHrP(1–141) or positive controls prostaglandin E2 (PGE2) or salmon calcitonin (sCT). Graphs = mean + SE. n = 3 replicates from independent experiments. *p < 0.05, **p < 0.01, ***p < 0.001 vs no treatment by one-way ANOVA with multiple comparisons.
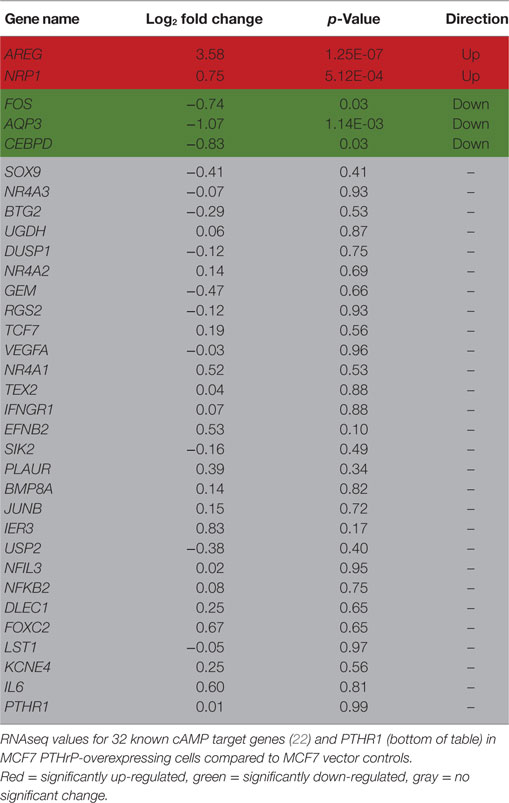
Table 1. Cyclic AMP (cAMP) signaling is not induced by parathyroid hormone-related protein (PTHrP) in MCF7 cells.
Validation of several candidate CREB-responsive genes in MCF7 PTHrP-overexpressing cell lines maintained at a separate institution was consistent with our RNAseq findings (Figures 3B–E). The one exception was NR4A1, which was found to be unaltered by RNAseq, but was significantly upregulated in PTHrP-overexpressing cells by real-time PCR (Figure 3F). We also confirmed that PTHR1 is not downregulated with PTHrP overexpression (Figure 3G). In addition, treatment with positive controls PGE2 and sCT induced significantly greater mRNA levels of CREB-responsive genes AREG, NR4A1, or RGS2, but exogenous treatment with PTHrP(1–141) had no significant effect (Figures 3H–J).
RNAseq Confirms PTHrP Overexpression Reduces Pro-Dormancy Genes
We previously reported that PTHrP overexpression in MCF7 cells significantly reduced the pro-dormancy genes LIFR, SOCS3, TPM1, AMOT, P4HA1, HIST1H2BK, SELENBP1, and QSOX1 (9). RNAseq analysis confirmed that 6/8 of these genes were downregulated in MCF7 PTHrP-overexpressing cells (Table 2).
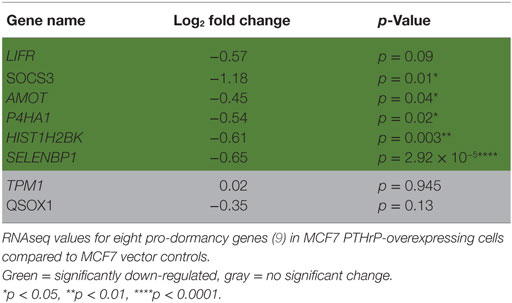
Table 2. Dormancy genes are downregulated by parathyroid hormone-related protein (PTHrP) in MCF7 cells.
Calcium-Related Pathways Are Activated in Response to PTHrP Overexpression in MCF7 Cells
We next performed STRING analysis on the RNAseq data to identify significantly enriched pathways. We separately analyzed the 250 upregulated and downregulated genes (all p < 0.05) with the largest log2 fold change, a total of 500 genes analyzed (Figures 4A,B). STRING pathway analysis of this RNAseq data revealed that the most significantly enriched pathways (false discovery rate = 0.0081–0.0324) in MCF7 cells overexpressing PTHrP in comparison to parental MCF7 cells and across all 500 genes, were the calcium signaling pathway, cytokine–cytokine receptor interaction, chemokine signaling pathway, and inflammatory mediator regulation of transient receptor potential (TRP) channels (Figure 4C).
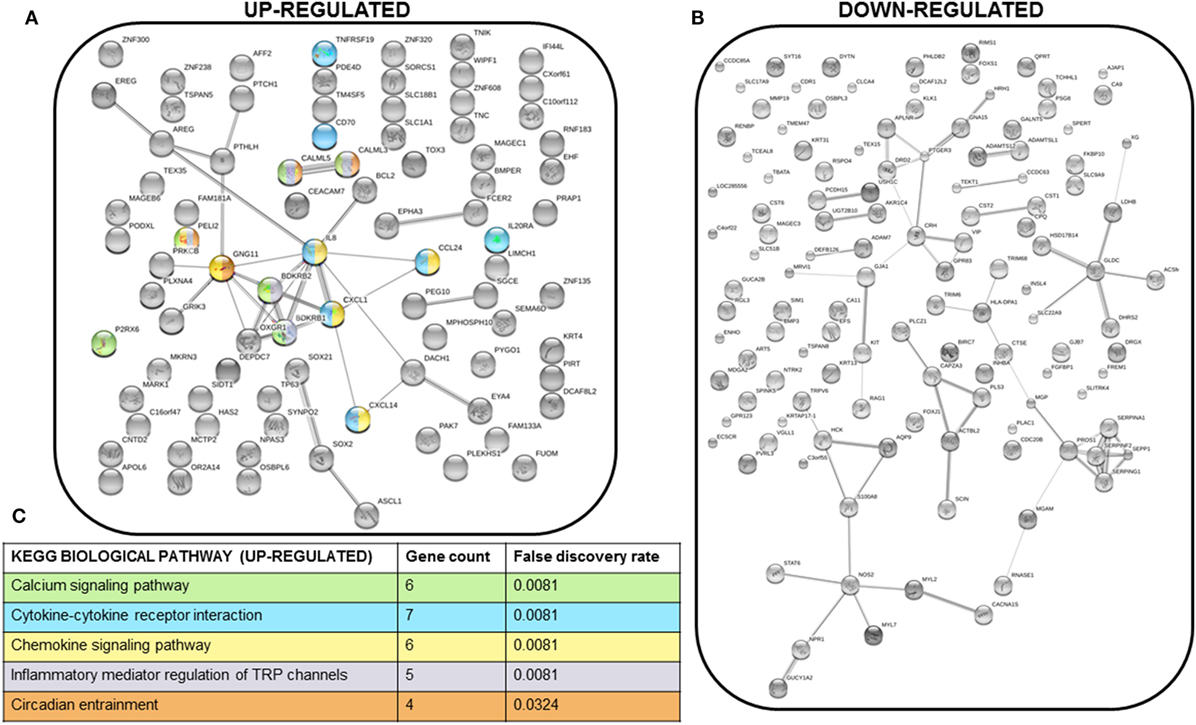
Figure 4. Multiple signaling pathways are upregulated in MCF7 parathyroid hormone-related protein-overexpressing cells. (A) STRING network analysis of the top 250 upregulated genes (with log2 fold change >1 and p < 0.05). Colors of each node correspond to the KEGG pathway indicated in (C). (B) STRING network analysis of the top 250 downregulated genes (with log2 fold change <−1 and p < 0.05). (C) KEGG biological pathways significantly enriched for in the STRING analysis of the top 250 upregulated genes. There were no significantly enriched KEGG biological pathways for the top 250 downregulated genes. Colors correspond to the nodes in (A).
The calcium signaling pathway and TRP channels are ion channels with high selectivity for Ca2+ (30), indicating calcium signaling is dramatically altered with PTHrP overexpression. There was overlap of 5/6 regulated genes in the “calcium signaling pathway” and “regulation of TRP channel pathway” from STRING analysis (P2RX6 was specific for the calcium signaling pathway) (Figure 5A); there were no unique TRP channel pathway genes that were regulated. mRNA levels for PTHLH (control), BDKRB1, and CALML3 (Figures 5B–D) confirmed the RNAseq findings in MCF7 PTHrP-overexpressing cells.
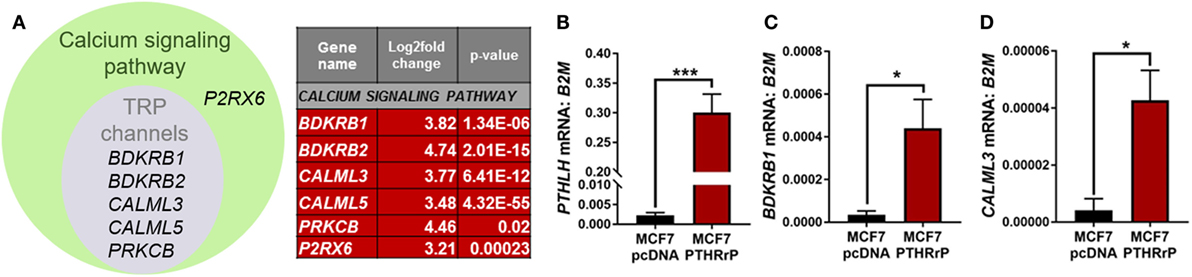
Figure 5. The calcium signaling pathway is significantly enriched downstream of parathyroid hormone-related protein (PTHrP) in MCF7 cells. (A) Venn diagram indicating the calcium signaling pathway and transient receptor potential (TRP) channel genes that were significantly upregulated in PTHrP-overexpressing cells (gray circle). There was also one significantly enriched gene that was unique to the calcium signaling pathway, P2RX6 (green circle). (B) PTHLH mRNA levels, shown as a control for PTHrP overexpression, in MCF7pcDNA control or MCF7 PTHrP-overexpressing cells (C,D). qPCR for mRNA levels of calcium signaling pathway genes in MCF7pcDNA or MCF7 PTHrP-overexpressing cells. Graphs = mean + SE. n = 3 replicates from independent experiments. *p < 0.05, ***p < 0.001 by Unpaired Student’s T-test.
Discussion
This work provides extensive evidence that PTHrP, although it is capable of inducing substantial changes in gene expression and behavior in MCF7 cells, does not signal through the PTHR1 to activate the cAMP pathway in these cells. Although PTHR1 is detected by qPCR, no cAMP response was detected, and no activity was observed in a CREB reporter assay. Furthermore, out of all the known cAMP responsive genes, only 2 of 32 were regulated in a positive direction by RNAseq analysis. In contrast, PTHrP overexpression in these cells upregulated genes associated with the calcium signaling pathway.
When human breast cancer cells were found to express functional receptors for calcitonin and PGE2 linked to adenylyl cyclase activation, no such activation could be detected in response to PTH(1–34) (15). We confirm this observation in the present experiments and show that PTHrP(1–141) also lacks this activity. In addition, we report that PTH(1–34) has no effect on activation of a CREB reporter construct that is readily activated by either sCT or PGE2. The latter two agonists, unlike PTH and PTHrP, also promoted expression of genes known to be regulated by the PKA–CREB pathway. There were only two cAMP responsive genes that were significantly upregulated with PTHrP overexpression by RNAseq: AREG and NRP1. Both of these genes have been implicated in cancer. AREG is essential for estrogen receptor-targeted therapeutic response (31). NRP1 has been previously shown to promote tumorigenesis by enhancing angiogenesis (32) and NRP1-positive cells have been reported to have tumor-initiating properties (33). Thus the upregulation of these genes may result from indirect effects independent of cAMP, a possibility we will investigate. It is also worth noting that the PTHrP induction of AREG mRNA, and the CREB-responsive gene NR4A1, in MCF7s is much lower than its induction with the positive controls prostaglandin E2 (PGE2) and sCT. In a separate study, we have tested the same secreted form of PTHrP, and the same preparation of recombinant PTHrP(1–141) in Ocy454 cells, an osteocyte cell line that expresses the PTHR1 (7). Overexpression and exogenous treatment both induced a significant increase in cAMP in these cells, and overexpression increased the CREB responsive genes, Nr4a1 and Rgs2 (7) confirming that these forms of PTHrP are capable of inducing a CREB response, but not in MCF7 cells.
Our data also indicate that PTH, which shares with PTHrP the same ability to bind to the PTHR1, does not bind to MCF7 cells in any detectable manner. This is illustrated by use of the PTH-TMR reagent, which requires functional PTHR1 for CREB activation and internalization into early endosomes. This suggests that the action of overexpressed PTHrP that suppresses dormancy and results in major changes in gene expression and osteolytic destruction of bone, is not only not cAMP-mediated, but is also not elicited through the PTHR1. However, we have not excluded the possibility that PTHrP binds to PTHR1 at levels below our detection limits, and initiates cAMP-independent signaling.
Parathyroid hormone and PTHrP have identical amino acids in 8 of their first 13 residues, but other similarities within the sequences are no more than would be expected by chance (1, 3). In PTHR1-bearing target cells, recombinant PTHrP(1–141) and synthetic shorter amino-terminal forms were equipotent on a molar basis with each other and with PTH(1–34) in their ability to promote cAMP activity (19). In exerting this function, PTHrP and PTH were shown to share actions upon a common receptor, PTHR1 (14). These functions are absent in MCF7 cells. Instead, our findings suggest that the major changes in gene expression in MCF7 cells in response to PTHrP must occur through PTHrP actions mediated by domains of PTHrP distinct from the (1–34) region known to act on the cAMP–PKA pathway through PTHR1.
A number of biological activities have been ascribed to domains of PTHrP beyond the amino-terminal region, but although these domains have been defined on the basis of the primary amino acid sequence, no receptors for these responses have yet been identified. For example, (i) the mid-molecule portion, between residues 35 and 84, is responsible for placental calcium transport, (ii) many pharmacologic studies have shown biological effects of the C-terminal domain, beginning at residue 107, and (iii) a nuclear localizing sequence mediates transport of PTHrP to the nucleus in many cell types [reviewed in Ref. (1)]. These many biological activities within the PTHrP molecule led to it being regarded as a multifunctional cytokine (34, 35) but the specific intracellular pathways that mediate these non-PTHR1-mediated actions remain unknown. These possibilities have been raised recently with respect to the role of PTHrP in bone remodeling (7), since mice lacking PTHrP in osteocytes exhibit a bone phenotype that is markedly different from mice lacking the PTHR1 (36).
Thus in this work, where substantial effects of PTHrP overexpression on gene expression in MCF7 cells seem to be unrelated to PTHR1-mediated actions though cAMP/PKA/CREB activation, these other domains of PTHrP need to be considered. This question begins to be addressed with the findings from the RNAseq data, which identified the calcium signaling pathway as significantly upregulated by PTHrP overexpression. In cancer, upregulation of Ca2+ channels and pumps promotes tumor proliferation and drives tumorigenesis. Several of these signaling pathway components have been reported as overexpressed in breast, prostate, colon, pancreas, and lung tumors (37–39). It has also been shown that PTHrP nuclear action downstream of the calcium-sensing receptor (CaSR) promotes proliferation and reduces p27Kip1 levels in breast cancer cells, ultimately preventing nuclear accumulation of apoptosis-inducing factor and the cell death that normally occurs when Ca2+ levels are in excess (40). While these actions have never been directly linked to PTHrP-induced bone destruction, our findings are consistent with the known roles for the calcium signaling pathway in cancer. These data suggest that CaSR acts upstream of PTHrP, and our data raise the possibility that PTHrP further promotes calcium signaling, possibly in a feed-forward loop.
We previously reported that PTHrP overexpression in MCF7 cells downregulates eight pro-dormancy genes (9) and our RNAseq analysis now provides a potential pathway through which PTHrP may function to downregulate these genes. Experiments to determine the functional significance of the calcium signaling pathway in tumor dormancy in vivo will be necessary to determine whether this is the pathway through which PTHrP enables dormant tumor cells to aggressively colonize the bone.
Author Contributions
RJ, YS, PH, AC, and JJ performed experiments and analyzed data. RJ, NP, NS, and TM interpreted the data. RJ, NS, and TM wrote the manuscript. YS, PH, AC, JJ, and NP edited the manuscript.
Conflict of Interest Statement
The authors declare that the research was conducted in the absence of any commercial or financial relationships that could be construed as a potential conflict of interest.
Acknowledgments
A portion of these data were previously published as a conference paper supplement (41). The authors wish to acknowledge the expert technical support of the VANGARD core facilities. RJ is supported in part by NIH award R00CA194198 (RJ). Experiments performed at Vanderbilt were supported in part by scholarship funds from NIH award P30CA068485 Vanderbilt-Ingram Cancer Center Support Grant. Experiments performed at SVI were supported in part by NHMRC grant 1081242 to NS and TM, and SVI receives support from the Victorian Government OIS Program. AC was supported in part by an Australian and New Zealand Bone and Mineral Society Christine & T. Jack Martin Research Travel Grant and NHMRC grant 1078280 to NP.
References
1. Martin TJ. Parathyroid hormone-related protein, its regulation of cartilage and bone development, and role in treating bone diseases. Physiol Rev (2016) 96(3):831–71. doi:10.1152/physrev.00031.2015
2. McCauley LK, Martin TJ. Twenty-five years of PTHrP progress: from cancer hormone to multifunctional cytokine. J Bone Miner Res (2012) 27(6):1231–9. doi:10.1002/jbmr.1617
3. Suva LJ, Winslow GA, Wettenhall RE, Hammonds RG, Moseley JM, Diefenbach-Jagger H, et al. A parathyroid hormone-related protein implicated in malignant hypercalcemia: cloning and expression. Science (1987) 237(4817):893–6. doi:10.1126/science.3616618
4. Powell GJ, Southby J, Danks JA, Stillwell RG, Hayman JA, Henderson MA, et al. Localization of parathyroid hormone-related protein in breast cancer metastases: increased incidence in bone compared with other sites. Cancer Res (1991) 51(11):3059–61.
5. Thomas RJ, Guise TA, Yin JJ, Elliott J, Horwood NJ, Martin TJ, et al. Breast cancer cells interact with osteoblasts to support osteoclast formation. Endocrinology (1999) 140(10):4451–8. doi:10.1210/endo.140.10.7037
6. Martin TJ. Osteoblast-derived PTHrP is a physiological regulator of bone formation. J Clin Invest (2005) 115(9):2322–4. doi:10.1172/JCI26239
7. Ansari N, Ho PW, Crimeen-Irwin B, Poulton IJ, Brunt AR, Forwood MR, et al. Autocrine and paracrine regulation of the murine skeleton by osteocyte-derived parathyroid hormone-related protein. J Bone Miner Res (2018) 33(1):137–53. doi:10.1002/jbmr.3291
8. Kobayashi T, Chung UI, Schipani E, Starbuck M, Karsenty G, Katagiri T, et al. PTHrP and Indian hedgehog control differentiation of growth plate chondrocytes at multiple steps. Development (2002) 129(12):2977–86.
9. Johnson RW, Finger EC, Olcina MM, Vilalta M, Aguilera T, Miao Y, et al. Induction of LIFR confers a dormancy phenotype in breast cancer cells disseminated to the bone marrow. Nat Cell Biol (2016) 18(10):1078–89. doi:10.1038/ncb3408
10. Kemp BE, Moseley JM, Rodda CP, Ebeling PR, Wettenhall RE, Stapleton D, et al. Parathyroid hormone-related protein of malignancy: active synthetic fragments. Science (1987) 238(4833):1568–70. doi:10.1126/science.3685995
11. Pizurki L, Rizzoli R, Moseley J, Martin TJ, Caverzasio J, Bonjour JP. Effect of synthetic tumoral PTH-related peptide on cAMP production and Na-dependent Pi transport. Am J Physiol (1988) 255(5 Pt 2):F957–61. doi:10.1152/ajprenal.1988.255.5.F957
12. Fukayama S, Bosma TJ, Goad DL, Voelkel EF, Tashjian AH Jr. Human parathyroid hormone (PTH)-related protein and human PTH: comparative biological activities on human bone cells and bone resorption. Endocrinology (1988) 123(6):2841–8. doi:10.1210/endo-123-6-2841
13. Ebeling PR, Adam WR, Moseley JM, Martin TJ. Actions of synthetic parathyroid hormone-related protein(1-34) on the isolated rat kidney. J Endocrinol (1989) 120(1):45–50. doi:10.1677/joe.0.1200045
14. Juppner H, Abou-Samra AB, Freeman M, Kong XF, Schipani E, Richards J, et al. A G protein-linked receptor for parathyroid hormone and parathyroid hormone-related peptide. Science (1991) 254(5034):1024–6. doi:10.1126/science.1658941
15. Martin TJ, Findlay DM, MacIntyre I, Eisman JA, Michelangeli VP, Moseley JM, et al. Calcitonin receptors in a cloned human breast cancer cell line (MCF 7). Biochem Biophys Res Commun (1980) 96(1):150–6. doi:10.1016/0006-291X(80)91193-6
16. Findlay DM, deLuise M, Michelangeli VP, Ellison M, Martin TJ. Properties of a calcitonin receptor and adenylate cyclase in BEN cells, a human cancer cell line. Cancer Res (1980) 40(4):1311–7.
17. Martin TJ, Ng KW, Partridge NC, Livesey SA. Hormonal influences on bone cells. Methods Enzymol (1987) 145:324–36. doi:10.1016/0076-6879(87)45019-2
18. Mulcrone PL, Campbell JP, Clement-Demange L, Anbinder AL, Merkel AR, Brekken RA, et al. Skeletal colonization by breast cancer cells is stimulated by an osteoblast and beta2AR-dependent neo-angiogenic switch. J Bone Miner Res (2017) 32(7):1442–54. doi:10.1002/jbmr.3133
19. Hammonds RG Jr, McKay P, Winslow GA, Diefenbach-Jagger H, Grill V, Glatz J, et al. Purification and characterization of recombinant human parathyroid hormone-related protein. J Biol Chem (1989) 264(25):14806–11.
20. Allan EH, Hausler KD, Wei T, Gooi JH, Quinn JM, Crimeen-Irwin B, et al. EphrinB2 regulation by PTH and PTHrP revealed by molecular profiling in differentiating osteoblasts. J Bone Miner Res (2008) 23(8):1170–81. doi:10.1359/jbmr.080324
21. Richard V, Luchin A, Brena RM, Plass C, Rosol TJ. Quantitative evaluation of alternative promoter usage and 3’ splice variants for parathyroid hormone-related protein by real-time reverse transcription-PCR. Clin Chem (2003) 49(8):1398–402. doi:10.1373/49.8.1398
22. Walia MK, Ho PM, Taylor S, Ng AJ, Gupte A, Chalk AM, et al. Activation of PTHrP-cAMP-CREB1 signaling following p53 loss is essential for osteosarcoma initiation and maintenance. Elife (2016) 5:e13446. doi:10.7554/eLife.13446
23. Chan AS, Clairfeuille T, Landao-Bassonga E, Kinna G, Ng PY, Loo LS, et al. Sorting nexin 27 couples PTHR trafficking to retromer for signal regulation in osteoblasts during bone growth. Mol Biol Cell (2016) 27(8):1367–82. doi:10.1091/mbc.E15-12-0851
24. Yamada H, Tsutsumi M, Fukase M, Fujimori A, Yamamoto Y, Miyauchi A, et al. Effects of human PTH-related peptide and human PTH on cyclic AMP production and cytosolic free calcium in an osteoblastic cell clone. Bone Miner (1989) 6(1):45–54. doi:10.1016/0169-6009(89)90022-6
25. Guise TA, Yin JJ, Taylor SD, Kumagai Y, Dallas M, Boyce BF, et al. Evidence for a causal role of parathyroid hormone-related protein in the pathogenesis of human breast cancer-mediated osteolysis. J Clin Invest (1996) 98(7):1544–9. doi:10.1172/JCI118947
26. Johnson RW, Nguyen MP, Padalecki SS, Grubbs BG, Merkel AR, Oyajobi BO, et al. TGF-beta promotion of Gli2-induced expression of parathyroid hormone-related protein, an important osteolytic factor in bone metastasis, is independent of canonical Hedgehog signaling. Cancer Res (2011) 71(3):822–31. doi:10.1158/0008-5472.CAN-10-2993
27. Kusuma N, Denoyer D, Eble JA, Redvers RP, Parker BS, Pelzer R, et al. Integrin-dependent response to laminin-511 regulates breast tumor cell invasion and metastasis. Int J Cancer (2012) 130(3):555–66. doi:10.1002/ijc.26018
28. Gallon M, Cullen PJ. Retromer and sorting nexins in endosomal sorting. Biochem Soc Trans (2015) 43(1):33–47. doi:10.1042/BST20140290
29. Feinstein TN, Wehbi VL, Ardura JA, Wheeler DS, Ferrandon S, Gardella TJ, et al. Retromer terminates the generation of cAMP by internalized PTH receptors. Nat Chem Biol (2011) 7(5):278–84. doi:10.1038/nchembio.545
30. Ramsey IS, Delling M, Clapham DE. An introduction to TRP channels. Annu Rev Physiol (2006) 68:619–47. doi:10.1146/annurev.physiol.68.040204.100431
31. Peterson EA, Jenkins EC, Lofgren KA, Chandiramani N, Liu H, Aranda E, et al. Amphiregulin is a critical downstream effector of estrogen signaling in ERalpha-positive breast cancer. Cancer Res (2015) 75(22):4830–8. doi:10.1158/0008-5472.CAN-15-0709
32. Miao HQ, Lee P, Lin H, Soker S, Klagsbrun M. Neuropilin-1 expression by tumor cells promotes tumor angiogenesis and progression. FASEB J (2000) 14(15):2532–9. doi:10.1096/fj.00-0250com
33. Jimenez-Hernandez LE, Vazquez-Santillan K, Castro-Oropeza R, Martinez-Ruiz G, Munoz-Galindo L, Gonzalez-Torres C, et al. NRP1-positive lung cancer cells possess tumor-initiating properties. Oncol Rep (2018) 39(1):349–57. doi:10.3892/or.2017.6089
34. Philbrick WM, Wysolmerski JJ, Galbraith S, Holt E, Orloff JJ, Yang KH, et al. Defining the roles of parathyroid hormone-related protein in normal physiology. Physiol Rev (1996) 76(1):127–73. doi:10.1152/physrev.1996.76.1.127
35. Martin TJ, Moseley JM, Williams ED. Parathyroid hormone-related protein: hormone and cytokine. J Endocrinol (1997) 154(Suppl):S23–37.
36. Saini V, Marengi DA, Barry KJ, Fulzele KS, Heiden E, Liu X, et al. Parathyroid hormone (PTH)/PTH-related peptide type 1 receptor (PPR) signaling in osteocytes regulates anabolic and catabolic skeletal responses to PTH. J Biol Chem (2013) 288(28):20122–34. doi:10.1074/jbc.M112.441360
37. El Hiani Y, Lehen’kyi V, Ouadid-Ahidouch H, Ahidouch A. Activation of the calcium-sensing receptor by high calcium induced breast cancer cell proliferation and TRPC1 cation channel over-expression potentially through EGFR pathways. Arch Biochem Biophys (2009) 486(1):58–63. doi:10.1016/j.abb.2009.03.010
38. Tsavaler L, Shapero MH, Morkowski S, Laus R. Trp-p8, a novel prostate-specific gene, is up-regulated in prostate cancer and other malignancies and shares high homology with transient receptor potential calcium channel proteins. Cancer Res (2001) 61(9):3760–9.
39. Dhennin-Duthille I, Gautier M, Faouzi M, Guilbert A, Brevet M, Vaudry D, et al. High expression of transient receptor potential channels in human breast cancer epithelial cells and tissues: correlation with pathological parameters. Cell Physiol Biochem (2011) 28(5):813–22. doi:10.1159/000335795
40. Kim W, Takyar FM, Swan K, Jeong J, VanHouten J, Sullivan C, et al. Calcium-sensing receptor promotes breast cancer by stimulating intracrine actions of parathyroid hormone-related protein. Cancer Res (2016) 76(18):5348–60. doi:10.1158/0008-5472.CAN-15-2614
Keywords: parathyroid hormone-related protein, cyclic AMP, MCF7, breast cancer, calcium signaling
Citation: Johnson RW, Sun Y, Ho PWM, Chan ASM, Johnson JA, Pavlos NJ, Sims NA and Martin TJ (2018) Parathyroid Hormone-Related Protein Negatively Regulates Tumor Cell Dormancy Genes in a PTHR1/Cyclic AMP-Independent Manner. Front. Endocrinol. 9:241. doi: 10.3389/fendo.2018.00241
Received: 27 January 2018; Accepted: 26 April 2018;
Published: 16 May 2018
Edited by:
Chandi C. Mandal, Central University of Rajasthan, IndiaReviewed by:
Han Qiao, Shanghai Jiao-Tong University School of Medicine, ChinaPetra Simic, Massachusetts Institute of Technology, United States
Copyright: © 2018 Johnson, Sun, Ho, Chan, Johnson, Pavlos, Sims and Martin. This is an open-access article distributed under the terms of the Creative Commons Attribution License (CC BY). The use, distribution or reproduction in other forums is permitted, provided the original author(s) and the copyright owner are credited and that the original publication in this journal is cited, in accordance with accepted academic practice. No use, distribution or reproduction is permitted which does not comply with these terms.
*Correspondence: Rachelle W. Johnson, rachelle.johnson@vanderbilt.edu