- Brain Research Institute, Jeffrey Cheah School of Medicine and Health Sciences, Monash University Malaysia, Bandar Sunway, Malaysia
Kisspeptin is a neuropeptide, encoded by kisspeptin 1 (KISS1)/Kiss1 gene, which primarily acts as the regulator of reproductive functions via its receptor, kisspeptin receptor (KissR) in vertebrates. In the brain, Kiss1 gene is mainly expressed in the hypothalamic region, but KissR gene is widely distributed throughout the brain, suggesting that kisspeptin–KissR system may be involved in not only reproductive, but also non-reproductive functions. In non-mammalian vertebrates, there are two or more kisspeptin and KissR types. The zebrafish (Danio rerio) possess two kisspeptin (Kiss1 and Kiss2) and their respective receptors [Kiss1 receptor (KissR1) and KissR2]. In the brain of zebrafish, while Kiss2 is expressed in the preoptic-hypothalamic area, Kiss1 is predominantly expressed in the habenula, an evolutionarily conserved epithalamic structure. Similarly, KissR1 is expressed only in the habenula, while KissR2 is widely distributed in the brain, suggesting that the two kisspeptin systems play specific roles in the brain. The habenular Kiss1 is involved in the modulation of the raphe nuclei and serotonin-related behaviors such as fear response in the zebrafish. This review summarizes the roles of multiple kisspeptin–KissR systems in reproductive and non-reproductive functions and neuronal mechanism, and debates the biological and evolutional significance of habenular kisspeptin–KissR systems in teleost species.
Introduction
During the past decade, the field of reproductive neuroendocrinology has shifted from its major focus on the hypothalamus–pituitary–gonadal (HPG) axis comprising gonadotropin-releasing hormone (GnRH), gonadotropins [luteinizing hormone (LH) and follicle-stimulating hormone (FSH)], and gonadal steroids to the next level with the discovery of RFamides, which include kisspeptin and gonadotropin-inhibitory hormone (also known as LPXRFamide). Kisspeptin is a neuropeptide, encoded by kisspeptin 1 (KISS1)/Kiss1 gene which was originally identified as a metastasis suppressor gene (1). Kisspeptin binds to its receptor, kisspeptin receptor (KissR), which was originally identified as an orphan G-protein-coupled receptor-54 (GPR54, also known as hOT7T175) (2). Kisspeptin consists of core peptides, including 54-, 14-, and 13-amino acids peptides and its processed mature, biologically active 10-amino acid peptide (Kiss1-10). In 2003, two studies reported consecutively the role of kisspeptin–KissR signaling in reproduction, particularly the control of GnRH-LH secretion during the onset of puberty in mammals (3, 4). Since then, numerous studies have demonstrated the role of kisspeptin–KissR signaling, neuroanatomy, and neuro-molecular mechanisms underlying the control of reproductive physiology in mammalian species (5). We have been interested to understand the importance of kisspeptin–KissR signaling in the vertebrate reproduction from an evolutional perspective using non-mammalian vertebrates. In 2004, we were the first to identify the non-mammalian KissR-like sequence from a cichlid fish, Nile tilapia (Oreochromis niloticus) and also demonstrated their gene expression in GnRH neurons using a laser capture microdissection technique (6). As for kisspeptin in teleosts, fish Kiss1 gene was first reported in zebrafish (Danio rerio) (7, 8). Interestingly, we found another gene encoding kisspeptin-like structure, which is slightly different from Kiss1 in the zebrafish and medaka (Oryzias latipes), we, therefore, named it Kiss2 (9). Similar to kisspeptins in teleosts, there are two or more KissR types, which are distributed in different patterns in the brain (10), suggesting specific role for two kisspeptin types in fish brain. Although in the mammalian species, kisspeptin–KissR system primarily targets GnRH neurons, in fact, kisspeptin neurons actually send their projections to a large number of brain areas and KissR are widely distributed in the brain (11–13). In addition, Kiss1 gene is also expressed in some extra-hypothalamic regions, such as the hippocampal dentate gyrus (14) and the medial amygdala (15). Surprisingly, in the zebrafish and medaka, knockout of two kisspeptins (kiss1 and kiss2) and KissRs [Kiss1 receptor (kissr1) and kissr2] genes had no obvious effect on their reproductive capability (16, 17). These observations indicate that kisspeptin–KissR system may play roles in processing several non-reproductive functions. In fact, a functional MRI study in humans has recently revealed that kisspeptin modulates limbic brain activity in response to sexual and emotional stimuli, and influences mood in healthy men (18). Given that the zebrafish model has clear distinct neuroanatomical patterns of two kisspeptin–KissR systems, the zebrafish is believed to be an ideal model to understand differential role and regulatory mechanism of the two kisspeptin–KissR systems. We have been particularly interested to understand the role of Kiss1 in the habenula. The habenula is an evolutionary conserved epithalamic structure, which is involved in certain forms of emotive decision making in primates. Recent discoveries in primates by Dr. Hikosaka’s group indicate that the habenula plays a prominent role in emotive behavioral choice through neuromodulation of the dopamine and the serotonin systems (19). In addition, the habenula is involved in behavioral responses to pain, stress, anxiety, sleep, and reward. The dysfunction of the habenula is associated with neurological problems, such as depression, schizophrenia, and drug-induced psychosis (20). Therefore, the habenula has been a recent focus as a potential therapeutic target for neuropsychiatric disorders.
The anatomy, molecular biology, functions, and regulatory mechanism of hypothalamic kisspeptin–KissR system have been extensively studied, and summarized in a number of review articles for mammalian species (5, 21–23) and for non-mammalian species (10, 24–29). However, the knowledge on non-hypothalamic kisspeptin–KissR system is still limited (14, 30–33). The role of non-hypothalamic kisspeptin signaling is scarcely examined in non-mammalian vertebrates. In this review, we provide an overview and recent updates of non-hypothalamic kisspeptin–KissR systems in non-mammalian vertebrates, with specific emphasis on the habenular Kiss1-KissR1 system in the zebrafish model.
Two Kisspeptins and KissR Types in Fish
Since their first identification in zebrafish and medaka (9), two kisspeptin types have been identified in several teleost species (24, 25), but some fish species, such as tilapia (34), Astatotilapia burtoni (27), red seabream (35), Atlantic halibut (36), flatfish Senegalese sole (37), black rockfish (38), Japanese flounder (39), and puffer fish (40) are likely to possess only one (Kiss2) type. Similar to multiple kisspeptin forms, multiple KissR (KissR1, KissR2, KissR3, and KissR4) types have also been identified in various fish species (25, 28, 41). In zebrafish, there are two KissR types, KissR2 and KissR3 (also designated as KissR1a and KissR1b, respectively) (41, 42). Pharmacological assays verified the ligand-receptor affinities for two kisspeptins and their receptors (28). In zebrafish, while zebrafish Kiss1 peptide (zfKiss1-10) activates KissR3 more efficiently than zebrafish Kiss2 peptide (zfKiss2-10), KissR2 is activated by both zfKiss1-10 and zfKiss2-10 in zebrafish (42). Distribution of two KissR types in the brain further verified the classification of their relationship with two kisspeptin types. In zebrafish, KissR3 gene is widely expressed in the brain, whereas KissR2 gene and its protein product are mainly expressed in the habenula (43–45), where Kiss1 gene is expressed. Therefore, based on these characteristics, we designate zebrafish KissR2 and KissR3 as KissR1 (kissr1) and KissR2 (kissr2), respectively in our articles (10). However, in some teleost species, relationship between multiple kisspeptins and their receptors has not been clearly characterized because multiple kisspeptins and receptors can cross talk with each other and have different neuroanatomical distributions. Nevertheless, it is very clear that two kisspeptin–KissR types are highly conserved in teleosts species, which are, however, involved in wide range of functions in the brain.
Similar to mammalian species, several functional assays have revealed the major role of Kiss-KissR systems in the control of reproduction in fish. In some fish, including zebrafish, in vivo assays show that Kiss2 (Kiss2–10 or Kiss2–12) rather than Kiss1 (Kiss1–10 or Kiss1–15) mainly exhibits its stimulatory effect on gonadotropin synthesis and release (9, 46–48). In chub mackerel, Kiss2 dodecapeptide (Kiss2–12) but not Kiss1 pentadecapeptide (Kiss1–15) administration alters GnRH1, LHβ, and FSHβ genes expression (47), which is further supported by co-expression or proximity of KissR2 in preoptic-hypothalamic GnRH neurons reported in several fish species (6, 49, 50). On the other hand, in some species, such as medaka, Kiss1 seems to be more potent than Kiss2 in the regulation of gonadotropin stimulation. In chub mackerel, Kiss1–15 is more potent than Kiss2–12 on stimulation of gonadal maturation when it was administered chronically (51, 52). In male yellowtail kingfish, Kiss1–10 and Kiss2–10 administration resulted in different effects depends on duration of treatment and reproductive stages of fish (53). These results indicate that regardless of kisspeptin types, fish kisspeptin can stimulate reproductive functions, which, however, may vary dependent on reproductive stages, gender, fish species, and treatment methods.
Expression of Kiss1–KissR1 in the Teleost Habenula
Expression of Kiss1 gene in the ventral part of the habenula has been shown in the zebrafish (9, 44, 45) as well as in the medaka (9, 54), goldfish (55), European sea bass (56), and the orange spotted grouper (57). However, unlike zebrafish Kiss1 gene expression, in other fish species, Kiss1 gene is also expressed in some brain regions, such as the preoptic-hypothalamic area, suggesting that Kiss1 can act on multiple action sites and have different roles in these species. In contrast to habenular kisspeptin, the expression of habenular KissR1 in the zebrafish, is seen in only a limited fish species. In medaka, KissR1 gene is expressed in the habenula and preoptic nuclei (58). In the European sea bass, not only KissR1 but also KissR2 expression has been reported in the habenula (56). Interestingly, in some species such as the chum mackerel and striped bass, KissR1 gene is expressed in the ventral habenula and preoptic area, in spite of the lack of Kiss1 gene expression in the habenula (50, 59). These results suggest that expression of KissR1 in the habenula is conserved at least among teleosts species that possess two kisspeptin types. In zebrafish, immunohistochemical localization using antibodies specific to zebrafish-Kiss1 and KissR1 reveal that habenular Kiss1/KissR1 cells project to the ventro-anterior corner of the median raphe (vaMR), a subregion of the MR [a division of the superior raphe (SR)] through the fasciculus retroflexus (FR) (44, 45, 60), which has also been confirmed in a kiss1:mCherry transgenic zebrafish (61). However, in the zebrafish brain, the KissR1 antibody also labeled cells in other brain area, such as the telencephalon, diencephalon, and spinal cord regions (60). This is because of the cross-reactivity of the KissR1 antibody against kissr1b-derived protein 2, an alternative splice variant of the KissR1 gene, which shares the epitope of the KissR1 antibody (62). Zebrafish KissR1 gene possess four additional alternative splice variants encoding different protein lengths (KRBDP 1–4), which, however, are functionally incapable of mediating kisspeptin-derived cellular responses (62). In the zebrafish, Kiss1 and its receptor are co-expressed in the same neurons within the habenula (63). Furthermore, central administration of Kiss1 peptides significantly suppresses Kiss1 gene expression, suggesting an autocrine regulation of the Kiss1 gene (63).
Modulation of Serotonin and its Related Behaviors by Habenular Kiss1/KissR1
Habenular Kiss1/KissR1 cells send projections in the vicinity of serotonin [5-hydroxytryptamine (5-HT)]-containing neurons located the median raphe (60). Mammalian habenula consists of two major subnuclei, the medial and lateral habenula. The medial habenula projects to the interpeduncular nucleus (IPN), while the lateral habenula directly projects to the ventral tegmental area and raphe, which are dopaminergic and serotonergic structures, respectively (64). Similar to mammalian habenula, the fish habenula can also be neuroanatomically subdivided into two major nuclei, the dorsal and ventral habenula based on difference in their cytoarchitectual structures (65). In zebrafish, the dorsal habenula project to the IPN via FR, while the ventral habenula project to the vaMR (60, 66). In addition, the dorsal habenula express the POU-domain transcription factor brn3a, a marker for the mice medial habenula (67, 68), while the ventral habenula express protocadherin 10a, a specific marker of the rat lateral habenula (66, 69). Therefore, the fish dorsal and ventral habenula have been elucidated as the homolog of the mammalian medial and lateral habenula, respectively. In mammals, the lateral habenula has been implicated as a pivotal regulator of dopaminergic and serotonergic neurons (19, 70). Furthermore, the lateral habenula is involved in sleep, locomotion, motivation, reward, and behavioral stress responses (19). In the brain of zebrafish treated with zfKiss1–15 peptides, expression of genes associated with serotonin, pet1 and sert (slc6a4a), and c-fos genes are significantly upregulated within the raphe nucleus (63). Central administration of zfKiss1–15 has no effect on anxiety, but shows a trend in anxiolytic effect (increase in number of transition) in zebrafish (45) when observed using a novel-tank diving test (71). Fish administered with zfKiss1–15 peptides failed to exhibit fear (45), characterized by erratic and freezing behaviors in response to an aversive stimulus from skin extract (alarm substance) (72, 73). Such effects were not observed when zfKiss2–10 was administered, suggesting these effects could be mainly modulated by Kiss1–KissR1 pathway. In addition, injections of zfKiss1–15 peptides conjugated with saporin; a ribosome-inactivating cytotoxic protein (74) induced cell death of Kiss1 neurons, the immunoreactivity of KissR1 was significantly reduced in the habenula and median raphe, and in these fish, alarm substance-induced fear response was significantly reduced (45). A recent study using kiss1-mutant fish revealed the potential involvement of habenular Kiss1 neurons to avoid punishment (75). These Kiss1 gene-mutants have a stop codon upstream of the active Kiss1 peptide, which causes deficiency in learning to avoid a shock that is predicted by light. These studies suggest that Kiss1–KissR1 signaling modulates behavioral response to uncontrollable aversive stimuli in the habenula. However, possible involvement of KissR2 or other receptor signaling pathways in this behavioral response should not be ignored, because KissR2 or other GPCRs are also activated by both Kiss1–10 and Kiss2–10 (42, 76).
Mechanism of Serotonergic Modulation by Kiss1–KissR1 Signaling
zfKiss1–15 administration effects serotonin-related genes, although KissR1 is not expressed in serotonergic neurons (60, 63), indicating that Kiss1 neurons act indirectly through interneurons on serotonergic system in zebrafish. Habenular Kiss1 neurons are glutamatergic in nature and their axons form close association with either glutamatergic or GABAergic interneurons in the median raphe region (60) (Figure 1). This suggests that Kiss1 might regulate serotonergic neural activities via the modulation of glutamatergic presynaptic neurotransmission. We speculate KissR1 might function as a presynaptic autoreceptor on habenula Kiss1 nerve terminals to facilitate glutamatergic transmission on serotonergic neurons, which remains to be confirmed.
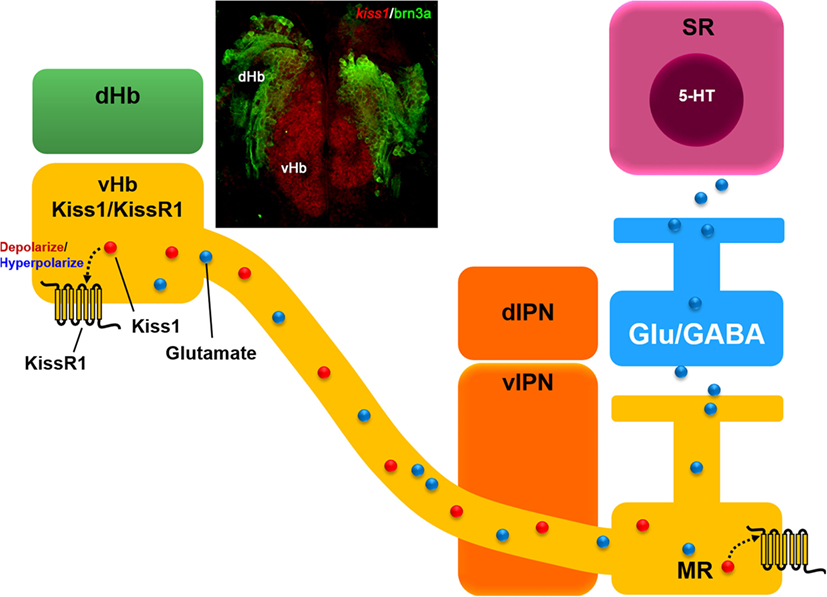
Figure 1. Schematic drawing of hypothetical neural circuit of habenular kisspeptin 1 (Kiss1) neurons. Kiss1 (red dot) modulate ventral habenular (vHb) neuronal activities via concentration-dependent mechanism through co-expressing Kiss1 receptor (KissR1). Photomicrograph shows a coronal brain section image of kiss1 mRNA expression in the vHb (red) but not in the dorsal habenula (dHb, green) expressing brn3a, a marker gene for the dHb in transgenic (brn3a-hsp70:GFP) zebrafish. Habenular Kiss1 neurons send their projections to the median raphe (MR), a division of the superior raphe (SR). Kiss1 cells are glutamatergic in nature and it is hypothesized that the presynaptic action of the Kiss1/KissR1 system causes the release of glutamate (blue dot) in Kiss1 cells from the vHb that potentially regulates the serotonin (5-HT) system in the SR directly or via glutamatergic and GABAergic neurotransmission. Abbreviations: dIPN, dorsal interpeduncular nucleus; vIPN, ventral interpeduncular nucleus modified from Nathan et al. (60).
In the zebrafish, although Kiss1 inhibits alarm substance-induced fear response, but its involvement through the serotonergic system remained unclear. The effect of Kiss1 on alarm substance-induced fear responses was blocked in the presence of serotonin receptor antagonists (77). Interestingly, treatment with different antagonist against two serotonin receptor types (5-HT1A and 5-HT2) results in different behavioral responses. Anxiolytic effect of Kiss1 is modulated via 5-HT1A receptor, while inhibitory effect of Kiss1 on freezing behavior is modulated via 5-HT2 receptor (77). Further, calcium imaging study has shown that Kiss1-gene mutant zebrafish larvae have reduced activation of raphe neurons by aversive stimulus (75). These studies indicate that Kiss1–KissR1 signaling is involved in the modulation of serotonergic neural activity under uncontrollable aversive conditions. Administration of exogenous Kiss1 or ablations of Kiss1 neurons suppresses alarm substance-induced fear response (45). Surprisingly, administration of Kiss1 also elevates c-fos gene expression by cellular excitation in the ventral habenula neurons (63). Optogenetic stimulation of ventral habenula neurons evokes place avoidance behavior (78), which is contradictory to the results of c-fos expression. This issue has been recently resolved by an electrophysiological approach (75), where Kiss1 has been shown to have a concentration-dependent effect on ventral habenula neurons: depolarizing at low concentrations and hyperpolarizing at high concentrations. Furthermore, c-fos expression was induced by a concentration of 10−11 mol/fish of Kiss1 peptides, but not with a higher concentration of 10−9 mol/fish (63). Therefore, suppression of alarm response by exogenous Kiss1 peptides could be due to hyperpolarization of ventral habenula neurons, which, however, remains to be further examined by functional assays.
Although Kiss1–KissR1 signaling modulates serotonin in response to uncontrolled aversive stimulus, but it remains unclear what regulates Kiss1 neurons, including Kiss1 peptide synthesis and secretion and Kiss1 neuronal activities. More importantly, the role of Kiss1–KissR1 signaling within the habenula neurons is still unknown. It is also important to identify the upstream control of the ventral habenula neurons, which could be from several afferent projections from brain regions, such as the entopeduncular nucleus, preoptic area, and hypothalamus (79, 80). Recent studies have revealed a functional connection between a thalamic nucleus and the habenula in zebrafish, and this pathway mediates light-evoked locomotor activity, including circadian behavior (81, 82). In mammals, the habenula (lateral habenula) neurons have been suggested to act as circadian oscillators (83–85). In addition, in the goldfish, Kiss1 and KissR gene expression are influenced by different light spectra (86). Therefore, Kiss1–KissR1 signaling in the habenula could be involved in mediating circadian controlled innate behaviors, such as sexual behavior and sleep–wake cycle, which remain to be studied.
Biological Significance of Habenular Kisspeptin
In a series of our studies, we have provided some evidences for the involvement of kisspeptin–KissR signaling in the zebrafish vertebrate habenula (45, 60, 63, 77). However, the presence of Kiss1 in the fish habenula nuclei has been shown in a limited number of fish species. Some fish species possess only one type of kisspeptin gene (Kiss2), which is expressed in the hypothalamic area (10). Some fish species that possess two kisspeptin types such as the chum mackerel show no expression of Kiss1 in the habenula (59). On the other hand, expression of KissR in the habenula has been identified in several fish species and also in mammals (11, 87) (Table 1) and kisspeptin neurons in the anteroventral periventricular nucleus have been shown to innervate the habenula (88), suggesting that the action of kisspeptin–KissR signaling and its role within the habenula might be evolutionarily conserved regardless of the source of kisspeptin. Interestingly, in the fetal mouse brain, Kiss1R containing cells are seen in a highly restricted population of cells in the habenula as early as embryonic day 17 (89), which is a period when the formation of habenula-IPN pathway is completing (90). Similarly, in embryonic zebrafish, Kiss1-positive cells are first appearing in the ventral habenula by 5-days post fertilization, when the innervation of the ventral habenula axon has reached their target, the medial raphe (91). Furthermore, in a mutant fish that lacks functional Tcf7l2, a downstream modulator of the Wnt signaling cascade, Kiss1 gene expression is lost and the median raphe are not innervated by ventral habenula axons (91). Therefore, the habenular Kiss1–KissR1 signaling may play a role in the habenula axonal formation during brain development. Previous studies have demonstrated the role of the lateral habenula in the hormonal onset of maternal behavior in female rats (92, 93). In both rodent and fish, the habenula is known to be sensitive to steroid hormones and express estrogen receptors (94, 95). In addition, the habenula in the zebrafish produces neurosteroids locally (96, 97). Furthermore, goldfish Kiss1 gene promoter contains putative binding sites for estrogen receptors (55), and in orange-spotted grouper, Kiss1 neurons express estrogen receptors in the habenula (57). Estrogen has effect on mood, mental state, and memory, which are closely related to serotonergic functions (98). Interestingly, in larval zebrafish, treatment with an estrogen receptor β agonist increased c-fos expression in the habenula with anxiolytic effect (increase in exploration behavior) (95). Therefore, kisspeptin–KissR signaling pathway within the habenula could be involved in the neuromodulatory processes of emotional and goal-directed behaviors, which could also be influenced by reproductive conditions.
Author Contributions
Both authors researched and wrote/edited the article and designed the figures.
Conflict of Interest Statement
The authors declare that the research was conducted in the absence of any commercial or financial relationships that could be construed as a potential conflict of interest.
Funding
This work was supported by Monash University Malaysia, and Malaysian Ministry of Higher Education (FRGS/2/2010/ST/MUSM/03/02, FRGS/1/2013/SKK01/MUSM/03/2, and FRGS/1/2014/ST03/MUSM/02/1) and Malaysian Ministry of Science and Technology and Innovation (02-02-10-SF0162).
References
1. Lee J, Miele M, Hicks D, Phillips K, Trent J, Weissman B, et al. KiSS-1, a novel human malignant melanoma metastasis-suppressor gene. J Natl Cancer Inst (1996) 88:1731–7. doi:10.1093/jnci/88.23.1731
2. Ohtaki T, Shintani Y, Honda S, Matsumoto H, Hori A, Kanehashi K, et al. Metastasis suppressor gene KiSS-1 encodes peptide ligand of a G-protein-coupled receptor. Nature (2001) 411:613–7. doi:10.1038/35079135
3. de Roux N, Genin E, Carel J, Matsuda F, Chaussain J, Milgrom E. Hypogonadotropic hypogonadism due to loss of function of the KiSS1-derived peptide receptor GPR54. Proc Natl Acad Sci U S A (2003) 100:10972–6. doi:10.1073/pnas.1834399100
4. Seminara S, Messager S, Chatzidaki E, Thresher R, Acierno J, Shagoury J, et al. The GPR54 gene as a regulator of puberty. N Engl J Med (2003) 349:1614–27. doi:10.1056/NEJMoa035322
5. Smith JT, Clifton DK, Steiner RA. Regulation of the neuroendocrine reproductive axis by kisspeptin-GPR54 signaling. Reproduction (2006) 131:623–30. doi:10.1530/rep.1.00368
6. Parhar IS, Ogawa S, Sakuma Y. Laser-captured single digoxigenin-labeled neurons of gonadotropin-releasing hormone types reveal a novel G protein-coupled receptor (Gpr54) during maturation in cichlid fish. Endocrinology (2004) 145:3613–8. doi:10.1210/en.2004-0395
7. Biran J, Ben-Dor S, Levavi-Sivan B. Molecular identification and functional characterization of the kisspeptin/kisspeptin receptor system in lower vertebrates. Biol Reprod (2008) 79:776–86. doi:10.1095/biolreprod.107.066266
8. van Aerle R, Kille P, Lange A, Tyler CR. Evidence for the existence of a functional Kiss1/Kiss1 receptor pathway in fish. Peptides (2008) 29:57–64. doi:10.1016/j.peptides.2007.10.018
9. Kitahashi T, Ogawa S, Parhar IS. Cloning and expression of kiss2 in the zebrafish and medaka. Endocrinology (2009) 150:821–31. doi:10.1210/en.2008-0940
10. Ogawa S, Parhar IS. Anatomy of the kisspeptin systems in teleosts. Gen Comp Endocrinol (2013) 181:169–74. doi:10.1016/j.ygcen.2012.08.023
11. Lee DK, Nguyen T, O’neill GP, Cheng R, Liu Y, Howard AD, et al. Discovery of a receptor related to the galanin receptors. FEBS Lett (1999) 446:103–7. doi:10.1016/S0014-5793(99)00009-5
12. Desroziers E, Mikkelsen J, Simonneaux V, Keller M, Tillet Y, Caraty A, et al. Mapping of kisspeptin fibres in the brain of the pro-oestrous rat. J Neuroendocrinol (2010) 22:1101–12. doi:10.1111/j.1365-2826.2010.02053.x
13. Herbison AE, De Tassigny Xd, Doran J, Colledge WH. Distribution and postnatal development of Gpr54 gene expression in mouse brain and gonadotropin-releasing hormone neurons. Endocrinology (2010) 151:312–21. doi:10.1210/en.2009-0552
14. Arai AC. The role of kisspeptin and GPR54 in the hippocampus. Peptides (2009) 30:16–25. doi:10.1016/j.peptides.2008.07.023
15. Kim J, Semaan SJ, Clifton DK, Steiner RA, Dhamija S, Kauffman AS. Regulation of Kiss1 expression by sex steroids in the amygdala of the rat and mouse. Endocrinology (2011) 152:2020–30. doi:10.1210/en.2010-1498
16. Tang H, Liu Y, Luo D, Ogawa S, Yin Y, Li S, et al. The kiss/kissr systems are dispensable for zebrafish reproduction: evidence from gene knockout studies. Endocrinology (2015) 156:589–99. doi:10.1210/en.2014-1204
17. Nakajo M, Kanda S, Karigo T, Takahashi A, Akazome Y, Uenoyama Y, et al. Evolutionally conserved function of kisspeptin neuronal system is nonreproductive regulation as revealed by nonmammalian study. Endocrinology (2018) 159:163–83. doi:10.1210/en.2017-00808
18. Comninos AN, Wall MB, Demetriou L, Shah AJ, Clarke SA, Narayanaswamy S, et al. Kisspeptin modulates sexual and emotional brain processing in humans. J Clin Invest (2017) 127:709–19. doi:10.1172/JCI89519
19. Matsumoto M, Hikosaka O. Lateral habenula as a source of negative reward signals in dopamine neurons. Nature (2007) 447:1111–5. doi:10.1038/nature05860
20. Hikosaka O. The habenula: from stress evasion to value-based decision-making. Nat Rev Neurosci (2010) 11:503–13. doi:10.1038/nrn2866
21. Dungan HM, Clifton DK, Steiner RA. Minireview: kisspeptin neurons as central processors in the regulation of gonadotropin-releasing hormone secretion. Endocrinology (2006) 147:1154–8. doi:10.1210/en.2005-1282
22. Oakley AE, Clifton DK, Steiner RA. Kisspeptin signaling in the brain. Endocr Rev (2009) 30:713–43. doi:10.1210/er.2009-0005
23. Lehman MN, Coolen LM, Goodman RL. Minireview: kisspeptin/neurokinin B/dynorphin (KNDy) cells of the arcuate nucleus: a central node in the control of gonadotropin-releasing hormone secretion. Endocrinology (2010) 151:3479–89. doi:10.1210/en.2010-0022
24. Elizur A. The KiSS1/GPR54 system in fish. Peptides (2009) 30:164–70. doi:10.1016/j.peptides.2008.08.018
25. Akazome Y, Kanda S, Okubo K, Oka Y. Functional and evolutionary insights into vertebrate kisspeptin systems from studies of fish brain. J Fish Biol (2010) 76:161–82. doi:10.1111/j.1095-8649.2009.02496.x
26. Parhar I, Ogawa S, Kitahashi T. RFamide peptides as mediators in environmental control of GnRH neurons. Prog Neurobiol (2012) 98:176–96. doi:10.1016/j.pneurobio.2012.05.011
27. Tena-Sempere M, Felip A, Gomez A, Zanuy S, Carrillo M. Comparative insights of the kisspeptin/kisspeptin receptor system: lessons from non-mammalian vertebrates. Gen Comp Endocrinol (2012) 175:234–43. doi:10.1016/j.ygcen.2011.11.015
28. Gopurappilly R, Ogawa S, Parhar IS. Functional significance of GnRH and kisspeptin, and their cognate receptors in teleost reproduction. Front Endocrinol (2013) 4:24. doi:10.3389/fendo.2013.00024
29. Kitahashi T, Parhar IS. Comparative aspects of kisspeptin gene regulation. Gen Comp Endocrinol (2013) 181:197–202. doi:10.1016/j.ygcen.2012.10.003
30. Liu X, Herbison AE. Kisspeptin regulation of neuronal activity throughout the central nervous system. Endocrinol Metab (2016) 31:193–205. doi:10.3803/EnM.2016.31.2.193
31. Uenoyama Y, Pheng V, Tsukamura H, Maeda K-I. The roles of kisspeptin revisited: inside and outside the hypothalamus. J Reprod Dev (2016) 62:537–45. doi:10.1262/jrd.2016-083
32. Stephens SBZ, Kauffman AS. Regulation and possible functions of kisspeptin in the medial amygdala. Front Endocrinol (2017) 8:191. doi:10.3389/fendo.2017.00191
33. Comninos AN, Dhillo WS. Emerging roles of kisspeptin in sexual and emotional brain processing. Neuroendocrinology (2018) 106:195–202. doi:10.1159/000481137
34. Ogawa S, Ng KW, Xue X, Ramadasan PN, Sivalingam M, Li S, et al. Thyroid hormone upregulates hypothalamic kiss2 gene in the male Nile tilapia, Oreochromis niloticus. Front Endocrinol (2013) 4:184. doi:10.3389/fendo.2013.00184
35. Shimizu Y, Tomikawa J, Hirano K, Nanikawa Y, Akazome Y, Kanda S, et al. Central distribution of kiss2 neurons and peri-pubertal changes in their expression in the brain of male and female red seabream Pagrus major. Gen Comp Endocrinol (2012) 175:432–42. doi:10.1016/j.ygcen.2011.11.038
36. Mechaly AS, Vinas J, Murphy C, Reith M, Piferrer F. Gene structure of the Kiss1 receptor-2 (Kiss1r-2) in the Atlantic halibut: insights into the evolution and regulation of Kiss1r genes. Mol Cell Endocrinol (2010) 317:78–89. doi:10.1016/j.mce.2009.11.005
37. Mechaly AS, Vinas J, Piferrer F. Gene structure analysis of kisspeptin-2 (Kiss2) in the Senegalese sole (Solea senegalensis): characterization of two splice variants of Kiss2, and novel evidence for metabolic regulation of kisspeptin signaling in non-mammalian species. Mol Cell Endocrinol (2011) 339:14–24. doi:10.1016/j.mce.2011.03.004
38. Song H, He Y, Ma L, Zhou X, Liu X, Qi J, et al. Characterisation of kisspeptin system genes in an ovoviviparous teleost: Sebastes schlegeli. Gen Comp Endocrinol (2015) 214:114–25. doi:10.1016/j.ygcen.2014.06.010
39. Song H, Wang M, Wang Z, Yu H, Wang Z, Zhang Q. Identification and characterization of kiss2 and kissr2 homologs in Paralichthys olivaceus. Fish Physiol Biochem (2016) 42:1073–92. doi:10.1007/s10695-016-0199-1
40. Shahjahan M, Motohashi E, Doi H, Ando H. Elevation of Kiss2 and its receptor gene expression in the brain and pituitary of grass puffer during the spawning season. Gen Comp Endocrinol (2010) 169:48–57. doi:10.1016/j.ygcen.2010.07.008
41. Pasquier J, Kamech N, Lafont A-G, Vaudry H, Rousseau K, Dufour S. Molecular evolution of GPCRs: kisspeptin/kisspeptin receptors. J Mol Endocrinol (2014) 52:T101–17. doi:10.1530/JME-13-0224
42. Lee YR, Tsunekawa K, Moon MJ, Um HN, Hwang JI, Osugi T, et al. Molecular evolution of multiple forms of kisspeptins and GPR54 receptors in vertebrates. Endocrinology (2009) 150:2837–46. doi:10.1210/en.2008-1679
43. Ogawa S, Kitahashi T, Ng KW, Parhar IS. Autocrine regulation of kiss1 in the habenula of the zebrafish. In: Proceedings of the 7th International Congress of Neuroendocrinology, ICN2010 [Abstract]. Rouen, France (2010). p. 2–20.
44. Servili A, Le Page Y, Leprince J, Caraty A, Escobar S, Parhar IS, et al. Organization of two independent kisspeptin systems derived from evolutionary-ancient kiss genes in the brain of zebrafish. Endocrinology (2011) 152:1527–40. doi:10.1210/en.2010-0948
45. Ogawa S, Nathan FM, Parhar IS. Habenular kisspeptin modulates fear in the zebrafish. Proc Natl Acad Sci U S A (2014) 111:3841–6. doi:10.1073/pnas.1314184111
46. Felip A, Zanuy S, Pineda R, Pinilla L, Carrillo M, Tena-Sempere M, et al. Evidence for two distinct KiSS genes in non-placental vertebrates that encode kisspeptins with different gonadotropin-releasing activities in fish and mammals. Mol Cell Endocrinol (2009) 312:61–71. doi:10.1016/j.mce.2008.11.017
47. Ohga H, Selvaraj S, Adachi H, Imanaga Y, Nyuji M, Yamaguchi A, et al. Functional analysis of kisspeptin peptides in adult immature chub mackerel (Scomber japonicus) using an intracerebroventricular administration method. Neurosci Lett (2014) 561:203–7. doi:10.1016/j.neulet.2013.12.072
48. Espigares F, Carrillo M, Gómez A, Zanuy S. The forebrain-midbrain acts as functional endocrine signaling pathway of Kiss2/Gnrh1 system controlling the gonadotroph activity in the teleost fish European sea bass (Dicentrarchus labrax). Biol Reprod (2015) 92(3):70. doi:10.1095/biolreprod.114.125138
49. Grone BP, Maruska KP, Korzan WJ, Fernald RD. Social status regulates kisspeptin receptor mRNA in the brain of Astatotilapia burtoni. Gen Comp Endocrinol (2010) 169:98–107. doi:10.1016/j.ygcen.2010.07.018
50. Zmora N, Stubblefield J, Zulperi Z, Biran J, Levavi-Sivan B, Muñoz-Cueto J-A, et al. Differential and gonad stage-dependent roles of kisspeptin1 and kisspeptin2 in reproduction in the modern teleosts, Morone species. Biol Reprod (2012) 86:177. doi:10.1095/biolreprod.111.097667
51. Selvaraj S, Ohga H, Kitano H, Nyuji M, Yamaguchi A, Matsuyama M. Peripheral administration of Kiss1 pentadecapeptide induces gonadal development in sexually immature adult scombroid fish. Zoolog Sci (2013) 30:446–54. doi:10.2108/zsj.30.446
52. Selvaraj S, Ohga H, Nyuji M, Kitano H, Nagano N, Yamaguchi A, et al. Subcutaneous administration of Kiss1 pentadecapeptide accelerates spermatogenesis in prepubertal male chub mackerel (Scomber japonicus). Comp Biochem Physiol A Mol Integr Physiol (2013) 166:228–36. doi:10.1016/j.cbpa.2013.06.007
53. Nocillado JN, Zohar Y, Biran J, Levavi-Sivan B, Elizur A. Chronic kisspeptin administration stimulated gonadal development in pre-pubertal male yellowtail kingfish (Seriola lalandi; Perciformes) during the breeding and non-breeding season. Gen Comp Endocrinol (2013) 191:168–76. doi:10.1016/j.ygcen.2013.06.005
54. Mitani Y, Kanda S, Akazome Y, Zempo B, Oka Y. Hypothalamic Kiss1 but not Kiss2 neurons are involved in estrogen feedback in medaka (Oryzias latipes). Endocrinology (2010) 151:1751–9. doi:10.1210/en.2009-1174
55. Wang Q, Sham KWY, Ogawa S, Li S, Parhar IS, Cheng CHK, et al. Regulation of the two kiss promoters in goldfish (Carassius auratus) by estrogen via different ERα pathways. Mol Cell Endocrinol (2013) 375:130–9. doi:10.1016/j.mce.2013.04.023
56. Escobar S, Felip A, Gueguen M-M, Zanuy S, Carrillo M, Kah O, et al. Expression of kisspeptins in the brain and pituitary of the european sea bass (Dicentrarchus labrax). J Comp Neurol (2013) 521:933–48. doi:10.1002/cne.23211
57. Guo Y, Wang Q, Li G, He M, Tang H, Zhang H, et al. Molecular mechanism of feedback regulation of 17β-estradiol on two kiss genes in the protogynous orange-spotted grouper (Epinephelus coioides). Mol Reprod Dev (2017) 84:495–507. doi:10.1002/mrd.22800
58. Kanda S, Akazome Y, Mitani Y, Okubo K, Oka Y. Neuroanatomical evidence that kisspeptin directly regulates isotocin and vasotocin neurons. PLoS One (2013) 8:e62776. doi:10.1371/journal.pone.0062776
59. Ohga H, Adachi H, Kitano H, Yamaguchi A, Matsuyama M. Kiss1 hexadecapeptide directly regulates gonadotropin-releasing hormone 1 in the scombroid fish, chub mackerel. Biol Reprod (2017) 96:376–88. doi:10.1095/biolreprod.116.142083
60. Nathan FM, Ogawa S, Parhar IS. Neuronal connectivity between habenular glutamate-kisspeptin1 co-expressing neurons and the raphe 5-HT system. J Neurochem (2015) 135:814–29. doi:10.1111/jnc.13273
61. Song Y, Duan X, Chen J, Huang W, Zhu Z, Hu W. The distribution of kisspeptin (Kiss)1- and Kiss2-positive neurones and their connections with gonadotrophin-releasing hormone-3 Nneurones in the zebrafish brain. J Neuroendocrinol (2015) 27:198–211. doi:10.1111/jne.12251
62. Onuma TA, Duan C. Duplicated Kiss1 receptor genes in zebrafish: distinct gene expression patterns, different ligand selectivity, and a novel nuclear isoform with transactivating activity. FASEB J (2012) 26:2941–50. doi:10.1096/fj.11-201095
63. Ogawa S, Ng KW, Ramadasan PN, Nathan FM, Parhar IS. Habenular Kiss1 neurons modulate the serotonergic system in the brain of zebrafish. Endocrinology (2012) 153:2398–407. doi:10.1210/en.2012-1062
64. Herkenham M, Nauta WJ. Efferent connections of the habenular nuclei in the rat. J Comp Neurol (1979) 187:19–47. doi:10.1002/cne.901870103
65. Braford MRJ, Northcutt RG. Organization of the diencephalon and pretectum of ray-finned fishes. In: Davis RE, Northcutt RG editors. Fish Neurobiology, Vol 2: Higher Brain Areas and Functions. Ann Arbor: University of Michigan Press (1983). p. 117–63.
66. Amo R, Aizawa H, Takahoko M, Kobayashi M, Takahashi R, Aoki T, et al. Identification of the zebrafish ventral habenula as a homolog of the mammalian lateral habenula. J Neurosci (2010) 30:1566–74. doi:10.1523/JNEUROSCI.3690-09.2010
67. Aizawa H, Bianco IH, Hamaoka T, Miyashita T, Uemura O, Concha ML, et al. Laterotopic representation of left-right information onto the dorso-ventral axis of a zebrafish midbrain target nucleus. Curr Biol (2005) 15:238–43. doi:10.1016/j.cub.2005.01.014
68. Quina LA, Wang S, Ng L, Turner EE. Brn3a and Nurr1 mediate a gene regulatory pathway for habenula development. J Neurosci (2009) 29:14309–22. doi:10.1523/JNEUROSCI.2430-09.2009
69. Aizawa H, Kobayashi M, Tanaka S, Fukai T, Okamoto H. Molecular characterization of the subnuclei in rat habenula. J Comp Neurol (2012) 520:4051–66. doi:10.1002/cne.23167
70. Christoph GR, Leonzio RJ, Wilcox KS. Stimulation of the lateral habenula inhibits dopamine-containing neurons in the substantia nigra and ventral tegmental area of the rat. J Neurosci (1986) 6:613–9. doi:10.1523/JNEUROSCI.06-03-00613.1986
71. Egan RJ, Bergner CL, Hart PC, Cachat JM, Canavello PR, Elegante MF, et al. Understanding behavioral and physiological phenotypes of stress and anxiety in zebrafish. Behav Brain Res (2009) 205:38–44. doi:10.1016/j.bbr.2009.06.022
72. Jesuthasan SJ, Mathuru AS. The alarm response in zebrafish: innate fear in a vertebrate genetic model. J Neurogenet (2008) 22:211–28. doi:10.1080/01677060802298475
73. Speedie N, Gerlai R. Alarm substance induced behavioral responses in zebrafish (Danio rerio). Behav Brain Res (2008) 188:168–77. doi:10.1016/j.bbr.2007.10.031
74. Bergamaschi G, Perfetti V, Tonon L, Novella A, Lucotti C, Danova M, et al. Saporin, a ribosome-inactivating protein used to prepare immunotoxins, induces cell death via apoptosis. Br J Haematol (1996) 93:789–94. doi:10.1046/j.1365-2141.1996.d01-1730.x
75. Lupton C, Sengupta M, Cheng R-K, Chia J, Thirumalai V, Jesuthasan S. Loss of the habenula intrinsic neuromodulator kisspeptin1 affects learning in larval zebrafish. eNeuro (2017) 4. doi:10.1523/ENEURO.0326-16.2017
76. Oishi S, Misu R, Tomita K, Setsuda S, Masuda R, Ohno H, et al. Activation of neuropeptide FF receptors by kisspeptin receptor ligands. ACS Med Chem Lett (2010) 2:53–7. doi:10.1021/ml1002053
77. Nathan FM, Ogawa S, Parhar IS. Kisspeptin1 modulates odorant-evoked fear response via two serotonin receptor subtypes (5-HT1A and 5-HT2) in zebrafish. J Neurochem (2015) 133:870–8. doi:10.1111/jnc.13105
78. Amo R, Fredes F, Kinoshita M, Aoki R, Aizawa H, Agetsuma M, et al. The habenulo-raphe serotonergic circuit encodes an aversive expectation value essential for adaptive active avoidance of danger. Neuron (2014) 84:1034–48. doi:10.1016/j.neuron.2014.10.035
79. Yañez J, Anadón R. Afferent and efferent connections of the habenula in the rainbow trout (Oncorhynchus mykiss): an indocarbocyanine dye (DiI) study. J Comp Neurol (1996) 372:529–43. doi:10.1002/(SICI)1096-9861(19960902)372:4<529::AID-CNE3>3.0.CO;2-6
80. Turner KJ, Hawkins TA, Yáñez J, Anadón R, Wilson SW, Folgueira M. Afferent connectivity of the zebrafish habenulae. Front Neural Circuits (2016) 10:30. doi:10.3389/fncir.2016.00030
81. Cheng R-K, Krishnan S, Lin Q, Kibat C, Jesuthasan S. Characterization of a thalamic nucleus mediating habenula responses to changes in ambient illumination. BMC Biol (2017) 15:104. doi:10.1186/s12915-017-0431-1
82. Lin Q, Jesuthasan S. Masking of a circadian behavior in larval zebrafish involves the thalamo-habenula pathway. Sci Rep (2017) 7:4104. doi:10.1038/s41598-017-04205-7
83. Zhao H, Rusak B. Circadian firing-rate rhythms and light responses of rat habenular nucleus neurons in vivo and in vitro. Neuroscience (2005) 132:519–28. doi:10.1016/j.neuroscience.2005.01.012
84. Tavakoli-Nezhad M, Schwartz WJ. Hamsters running on time: is the lateral habenula a part of the clock? Chronobiol Int (2006) 23:217–24. doi:10.1080/07420520500521947
85. Guilding C, Hughes A, Piggins H. Circadian oscillators in the epithalamus. Neuroscience (2010) 169:1630–9. doi:10.1016/j.neuroscience.2010.06.015
86. Choi CY, Shin HS, Kim NN, Yang S-G, Kim B-S, Yu YM. Time-related effects of various LED light spectra on reproductive hormones in the brain of the goldfish Carassius auratus. Biol Rhythm Res (2015) 46:671–82. doi:10.1080/09291016.2015.1046247
87. Wagner F, French L, Veh RW. Transcriptomic-anatomic analysis of the mouse habenula uncovers a high molecular heterogeneity among neurons in the lateral complex, while gene expression in the medial complex largely obeys subnuclear boundaries. Brain Struct Func (2016) 221:39–58. doi:10.1007/s00429-014-0891-9
88. Gu G, Simerly R. Projections of the sexually dimorphic anteroventral periventricular nucleus in the female rat. J Comp Neurol (1997) 384:142–64. doi:10.1002/(SICI)1096-9861(19970721)384:1<142::AID-CNE9>3.0.CO;2-1
89. Knoll JG, Clay C, Bouma G, Henion T, Schwarting G, Millar R, et al. Developmental profile and sexually dimorphic expression of kiss1 and kiss1r in the fetal mouse brain. Front Endocrinol (2013) 4:140. doi:10.3389/fendo.2013.00140
90. Lenn NJ, Bayer SA. Neurogenesis in subnuclei of the rat interpeduncular nucleus and medial habenula. Brain Res Bull (1986) 16:219–24. doi:10.1016/0361-9230(86)90036-5
91. Beretta CA, Dross N, Bankhead P, Carl M. The ventral habenulae of zebrafish develop in prosomere 2 dependent on Tcf7l2 function. Neural Dev (2013) 8:19. doi:10.1186/1749-8104-8-19
92. Matthews-Felton T, Corodimas KP, Rosenblatt JS, Morrell JI. Lateral habenula neurons are necessary for the hormonal onset of maternal behavior and for the display of postpartum estrus in naturally parturient female rats. Behav Neurosci (1995) 109:1172–88. doi:10.1037/0735-7044.109.6.1172
93. Felton TM, Linton L, Rosenblatt JS, Morrell JI. Intact neurons of the lateral habenular nucleus are necessary for the nonhormonal, pup-mediated display of maternal behavior in sensitized virgin female rats. Behav Neurosci (1998) 112:1458–65. doi:10.1037/0735-7044.112.6.1458
94. Wagner CK, Silverman A-J, Morrell JI. Evidence for estrogen receptor in cell nuclei and axon terminals within the lateral habenula of the rat: regulation during pregnancy. J Comp Neurol (1998) 392:330–42. doi:10.1002/(SICI)1096-9861(19980316)392:3<330::AID-CNE4>3.0.CO;2-2
95. Chen F, Chen S, Liu S, Zhang C, Peng G. Effects of lorazepam and WAY-200070 in larval zebrafish light/dark choice test. Neuropharmacology (2015) 95:226–33. doi:10.1016/j.neuropharm.2015.03.022
96. Goto-Kazeto R, Kight KE, Zohar Y, Place AR, Trant JM. Localization and expression of aromatase mRNA in adult zebrafish. Gen Comp Endocrinol (2004) 139:72–84. doi:10.1016/j.ygcen.2004.07.003
97. Diotel N, Do Rego JL, Anglade I, Vaillant C, Pellegrini E, Gueguen MM, et al. Activity and expression of steroidogenic enzymes in the brain of adult zebrafish. Eur J Neurosci (2011) 34:45–56. doi:10.1111/j.1460-9568.2011.07731.x
98. Fink G, Sumner BE, Rosie R, Grace O, Quinn JP. Estrogen control of central neurotransmission: effect on mood, mental state, and memory. Cell Mol Neurobiol (1996) 16:325–44. doi:10.1007/BF02088099
99. Simonneaux V, Ancel C, Gauer F, Poirel V-J. Kisspeptins and RFRP-3 act in concert to synchronize rodent reproduction with seasons. Front Neurosci (2013) 7:22. doi:10.3389/fnins.2013.00022
100. Imamura S, Hur S-P, Takeuchi Y, Bouchekioua S, Takemura A. Molecular cloning of kisspeptin receptor genes (gpr54-1 and gpr54-2) and their expression profiles in the brain of a tropical damselfish during different gonadal stages. Comp Biochem Physiol A Mol Integr Physiol (2017) 203:9–16. doi:10.1016/j.cbpa.2016.07.015
101. Ando H, Ogawa S, Shahjahan M, Ikegami T, Hattori A, Parhar I. Diurnal and circadian oscillations in expression of kisspeptin, kisspeptin receptor and gonadotrophin-releasing hormone 2 genes in the grass puffer, a semilunar-synchronised spawner. J Neuroendocrinol (2014) 26:459–67. doi:10.1111/jne.12165
Keywords: kisspeptin 1, teleosts fish, zebrafish, non-hypothalamic, Gpr54/Kiss1r
Citation: Ogawa S and Parhar IS (2018) Biological Significance of Kisspeptin–Kiss 1 Receptor Signaling in the Habenula of Teleost Species. Front. Endocrinol. 9:222. doi: 10.3389/fendo.2018.00222
Received: 23 February 2018; Accepted: 19 April 2018;
Published: 07 May 2018
Edited by:
William Henry Colledge, University of Cambridge, United KingdomReviewed by:
Gustavo M. Somoza, Instituto de Investigaciones Biotecnológicas (IIB-INTECH), ArgentinaTsuyoshi Kawada, Suntory Foundation for Life Sciences, Japan
Copyright: © 2018 Ogawa and Parhar. This is an open-access article distributed under the terms of the Creative Commons Attribution License (CC BY). The use, distribution or reproduction in other forums is permitted, provided the original author(s) and the copyright owner are credited and that the original publication in this journal is cited, in accordance with accepted academic practice. No use, distribution or reproduction is permitted which does not comply with these terms.
*Correspondence: Ishwar S. Parhar, aXNod2FyJiN4MDAwNDA7bW9uYXNoLmVkdQ==