- 1Department of Endocrinology, Hospital Infantil Universitario Niño Jesús, Madrid, Spain
- 2Instituto de Investigación La Princesa, Madrid, Spain
- 3CIBER Fisiopatología de la Obesidad y Nutrición, Instituto de Salud Carlos III, Madrid, Spain
- 4Department of Pediatrics, Universidad Autónoma de Madrid, Madrid, Spain
- 5Department of Cell Biology, Physiology and Immunology, Instituto Maimónides de Investigacion Biomédicas de Córdoba (IMIBIC), Hospital Universitario Reina Sofia, University of Córdoba, Córdoba, Spain
- 6IMDEA Food Institute, CEI UAM-CSIC, Madrid, Spain
Proper nutrition is important for growth and development. Maturation of the reproductive axis and the timing of pubertal onset can be delayed when insufficient nutrition is available, or possibly advanced with nutritional abundance. The childhood obesity epidemic has been linked to a secular trend in advanced puberty in some populations. The increase in circulating leptin that occurs in association with obesity has been suggested to act as a signal that an adequate nutritional status exists for puberty to occur, allowing activation of central mechanisms. However, obesity-associated hyperleptinemia is linked to decreased leptin sensitivity, at least in adults. Here, we analyzed whether neonatal overnutrition modifies the response to an increase in leptin in peripubertal male rats, as previously demonstrated in females. Wistar rats were raised in litters of 4 (neonatal overnutrition) or 12 pups (controls) per dam. Leptin was administered sc (3 µg/g body weight) at postnatal day 35 and the rats killed 45 min or 2 h later. Postnatal overfeeding resulted in increased body weight and circulating leptin levels; however, we found no overweight-related changes in the mRNA levels of neuropeptides involved in metabolism or reproduction. In contrast, pituitary expression of luteinizing hormone (LH) beta-subunit was increased in overweight rats, as was testicular weight. There were no basal differences between L4 and L12 males or in their response to leptin administration in pSTAT3 levels in the hypothalamus at either 45 min or 2 h. In contrast, pJAK2 was found to be higher at 45 min in L4 compared to L12 males regardless of leptin treatment, while at 2 h it was higher in L4 leptin-treated males compared to L12 leptin-treated males, as well as L4 vehicle-treated rats. There were no changes in response to leptin administration in the expression of the neuropeptides analyzed. However, serum LH levels rose only in L4 males in response to leptin, but with no change in testosterone levels. In conclusion, the advancement in pubertal onset in males with neonatal overnutrition does not appear to be related to overt modifications in the central response to exogenous leptin during the peripubertal period.
Introduction
Pubertal onset is the culmination of maturational processes that result in an increase in the activation of gonadotropin-releasing hormone (GnRH) neurons, which then activate the gonadotropin axis to induce maturation of the gonads and reproductive function (1–4). The timing of this phenomenon is determined by the interaction between an individual’s genetic makeup and environmental influences (5). For example, a suboptimal nutritional status, such as in anorexia nervosa or extreme physical exercise, has been shown to delay pubertal maturation in children (6, 7), and this has been interpreted as indicating that sufficient energy stores are required for reproductive functions to be capacitated, at least in females. In turn, it is very likely that the inverse is also true; that an abundance of available energy advances pubertal development. Indeed, studies suggest that the mean age of pubertal onset is advanced in the growing population of obese/overweight children in developed countries compared to their lean peers, with both boys and girls being affected (1, 2, 8–11). Although this trend in overall earlier pubertal development should not be confused with pathological precocious puberty, it could result in an increased risk for these individuals to develop hypertension or metabolic diseases in later life (12), emphasizing the importance in understanding the mechanisms underlying this phenomenon.
Circulating leptin levels are increased in obese children (13, 14), with the levels of this hormone reported to be inversely related to the age at menarche (15). Indeed, leptin is not only an important anorexigenic signal, modulating proopiomelanocortin (POMC) and neuropeptide Y/Agouti-related peptide (NPY/AgRP) neurons (16, 17), but it is also considered to be a permissive signal for pubertal onset (18–20) and an important link between metabolism and puberty (21). Patients or rodents that are genetically lacking leptin or that harbor LepR mutations lack pubertal development and are infertile (22–25), with leptin replacement normalizing the reproductive axis in leptin-deficient subjects (22, 23, 25). Although this hormone does not trigger pubertal onset, a minimum level of leptin levels/activity appears to be required for pubertal development and maintenance of the reproductive axis. Indeed, leptin treatment of normal mice has been shown to advance pubertal onset indicating that the levels of this hormone are important for pubertal timing (26).
The experimental model of neonatal overnutrition induced by a reduction in litter size results in advancement of the mean age of pubertal onset in both male and female rodents (27–29), which is most likely due, at least in part, to the rise in circulating leptin levels associated with their increased bodyweight/fat mass (28, 30). Moreover, we previously reported that during the peripubertal period, the central response to leptin is increased in neonatally overnourished female rats (31), suggesting that this increased leptin sensitivity could also participate in advancing the activation of the reproductive axis. However, it is unknown whether a similar phenomenon occurs in peripubertal male rats. Hence, the aim of the study presented here was to analyze whether early overnutrition affects hypothalamic leptin sensitivity in peripubertal male rats.
Materials and Methods
Animals
Wistar rats bred in the animal facilities of the University of Córdoba were employed. After mating and confirmation of pregnancy, dams were housed individually given free access to standard laboratory chow and tap water. On the day of birth [postnatal day (PND) 0], pups were arranged into two different litter sizes: 4 pups/litter (overnutrition; ON) and 12 pups/litter (control; Ct) with equal number of males and females in each. Cross-fostering was used. Only male offspring were analyzed in the studies reported here. Rats were kept under constant conditions of light (12 h of light, from 0730 h) and temperature (22–24°C). The experimental procedures were approved by the respective University Ethical Committee for Animal Experimentation (Royal Decree 53/2013) and conducted in accordance with the European Union guidelines for use of experimental animals (2010/63/EU).
Experimental Design
On PND 22, the pups were weaned and housed in groups of four rats of the same experimental group per cage. All rats had free access to normal rat chow and water. Body weight (BW) gain and food intake were evaluated at PNDs 25, 30, and 35. As an external sign of puberty, balano-preputial separation was monitored between PNDs 30 and 35 (end of the study).
On PND 35 rats (n = 24/group) were injected sc with either rat leptin (3 µg/g BW; National Hormone and Pituitary Program, Torrance, CA, USA) or vehicle (saline + 0.1% BSA, 10 ml/kg), after a fasting period of 12 h. This dose of recombinant leptin was chosen as it activates leptin signaling cascades and modulates hypothalamic neuropeptide expression (31–33). Rats were injected with leptin and killed 45 min later for the analysis of intracellular leptin-signaling pathways in the hypothalamus and 2 h after hormone injection to study changes in gene expression in the hypothalamus and pituitary, in addition to intracellular leptin-signaling pathways. All rats were killed between 0900 and 1100.
Trunk blood was collected in tubes containing EDTA. Samples were centrifuged at 3,000 rpm (20 min) and plasma stored at −80°C until processed. After rapidly removing the brain, the hypothalamus was isolated on ice by making an anterior cut at the level of the optic chiasm, a posterior coronal section anterior to the mammillary bodies, two sagittal cuts parallel to the lateral ventricles and a dorsal horizontal cut at the level of the anterior commissure. The block was then immediately stored at −80°C until processed. The testes were removed and the surrounding fat removed before weighing and freezing. The pituitary gland and visceral (perigonadal) and subcutaneous (inguinal) fat pads were also removed, weighed, and stored at −80°C.
Hormone Measurements
Plasma leptin and insulin levels were measured by multiplexed immunoassay following the manufacturer’s instructions (Millipore, Billerica, MA, USA). The sensitivity of the assay was 21.5 and 51 pg/ml for leptin and insulin, respectively. The intra-assay coefficient of variation (CV) was 8.2 and 7.3% and the inter-assay CV was 8.5 and 9.8% for leptin and insulin, respectively.
A radioimmunoassay supplied by the National Institutes of Health (National Hormone and Peptide Program, Torrance, CA, USA) was used to measure circulating luteinizing hormone (LH) concentrations. Rat LH-I-10 was labeled with 125I using iodo-gen tubes (Pierce Chemical Co., Rockford, IL, USA). Hormone concentrations are expressed using reference preparation LH-RP-3 as standard. The sensitivity of the assay was 3.75 ng/tube and intra-assay CV was less than 8%.
Protein Extraction and Western Blotting
Hypothalami from rats sacrificed 45 min after acute leptin treatment were homogenized in lysis buffer [0.1 M NaH2PO4 (pH 7.4), 20% Triton X-100, 10% SDS, 0.5% NaN3, 0.5% sodium deoxycholate, 100 mM phenylmethylsulfonyl fluoride, and a cocktail of EDTA-free protease inhibitors (Roche Diagnostics, Mannhein, Germany)]. The lysates were centrifuged at 14,000 g for 10 min at 4°C, and the supernatants stored at −80°C until assayed.
Hypothalami from rats sacrificed 2 h after acute leptin treatment were processed according to RNeasy Plus Mini Kit protocol (QIAGEN Iberia), obtaining a protein fraction that was precipitated with acetone. The pellets were reconstituted in 3-[(3-cholamidopropyl) dimethylammonio] propanesulfonate CHAPS buffer (7 mM urea, 2 M thiourea, CHAPS 4% w/v, 0.5% v/v 1 M Tris pH 8.8). The protein concentration in each sample was determined by the method of Bradford (Bio-Rad Laboratories, Hercules, CA, USA) and 40 µg of protein were resolved on a 10% SDS-polyacrylamide gel under denaturing conditions, electro-transferred to polyvinyl difluoride membranes (Bio-Rad Laboratories), and the transfer efficiency determined by Ponceau red dyeing. Membranes were blocked in Tris-buffered saline (20 mM) containing 5% non-fat dried milk or BSA and 0.1% Tween 20 for 2 h, and incubated overnight at 4°C under agitation with the primary antibody at a concentration of 1:1,000. Primary antibodies toward the following proteins were used: anti-STAT3, phospho-STAT3 (Tyr705), phospho-Akt (Ser 473), phospho-ERK1/2, and phospho-JAK2 (Tyr1007/1008) from Cell Signaling (Boston, MA, USA), Akt, ERK1/2, and phospho-PTEN (Ser380/Thr383) from Santa Cruz Biotechnology (Santa Cruz, CA, USA), SOCS3 from Proteintech (Chicago, IL, USA), PTEN from Sigma (St. Louis, MO, USA), PTP1B from Novus Biologicals (Atlanta, GA, USA), and GAPDH from Anaspec (San Jose, CA, USA). Secondary antibodies conjugated with peroxidase were from Pierce Biotechnology (Rockford, IL, USA). Bound peroxidase activity was visualized by chemiluminescence (Bio-Rad Laboratories) and quantified by densitometry using an ImageQuant LAS 4000 mini system (GE Healthcare Little Chalfont, UK). All blots were normalized with Ponceau, GAPDH, or total protein to control for loading variability and all values were normalized to control values on each gel.
RNA Preparation and Quantitative Real-Time PCR
Total RNA was extracted from hypothalami and pituitary glands by using RNeasy Plus Mini columns (QIAGEN Iberia). cDNA was synthesized from 2 µg of total RNA by using a high-capacity cDNA reverse transcription kit (Applied BioSystems). Relative mRNA levels in hypothalamic samples were assessed by quantitative real-time PCR and predesigned primers (Applied BioSystems) for AgRP (Rn01431703), GHRH (Rn00580832), GnRH (Rn00562754), Kiss1 (Rn00710914), Kiss1R (Rn00576940), LepR (Rn01433205), POMC (Rn00595020), somatostatin (Rn00561967), and NPY (Rn01410145). In the pituitary, the mRNA levels of the beta-subunit of LH (Rn00563443) and FSH (Rn01484594) were measured. Quantitative real-time PCR was performed by using assay on-demand kits (Applied Biosystems) and TaqMan universal PCR master mix (Applied Biosystems) according to the manufacturer’s protocol in an ABI PRISM 7000 sequence detection system (Applied Biosystems). Values were normalized to the housekeeping genes GAPDH (Rn99999916) and phosphoglycerate kinase 1 (Rn01474008). The ΔΔCT method was used to determine relative expression levels, according to manufacturer’s guidelines. Statistics were performed using ΔΔCT values (31).
Statistical Analysis
ANOVA with repeated measures were used to analyze changes in weight and food intake over time. Results were analyzed using unpaired Student’s t-tests when only two groups were involved or two-way ANOVA for more than two groups. When indicated, a one-way ANOVA followed by a Scheffé F-test was then used (SPSS v.15.0, IBM Corp., Armonk, NY, USA). P < 0.05 was considered significant. Data are presented as the mean ± SEM.
Results
Body Weight, Length, Food Intake, and Fat Mass
At the onset of the study (birth), there were no differences between the two litter sizes in body weight (L4: 6.3 ± 0.1, L12: 6.1 ± 0.3 g) or length (L4: 5.0 ± 0.1, L12: 5.0 ± 0.1 cm).
When weaned at PND21, rats from the small litters weighed more than those from litters of 12 (L12) pups (p < 0.0001; Figure 1A). They were also longer (p < 0.0001; Figure 1B). These rats from small litters continued to weigh more and be longer than those from L12 pups throughout the study (Figures 1A,B, respectively), with the exception of body length at PND30 due to an unexplained variation in the weights of L4 pups. At the end of the study, L4 rats remained longer (p < 0.01) and weighed more (p < 0.01) than L12 rats, even after fasting before sacrifice.
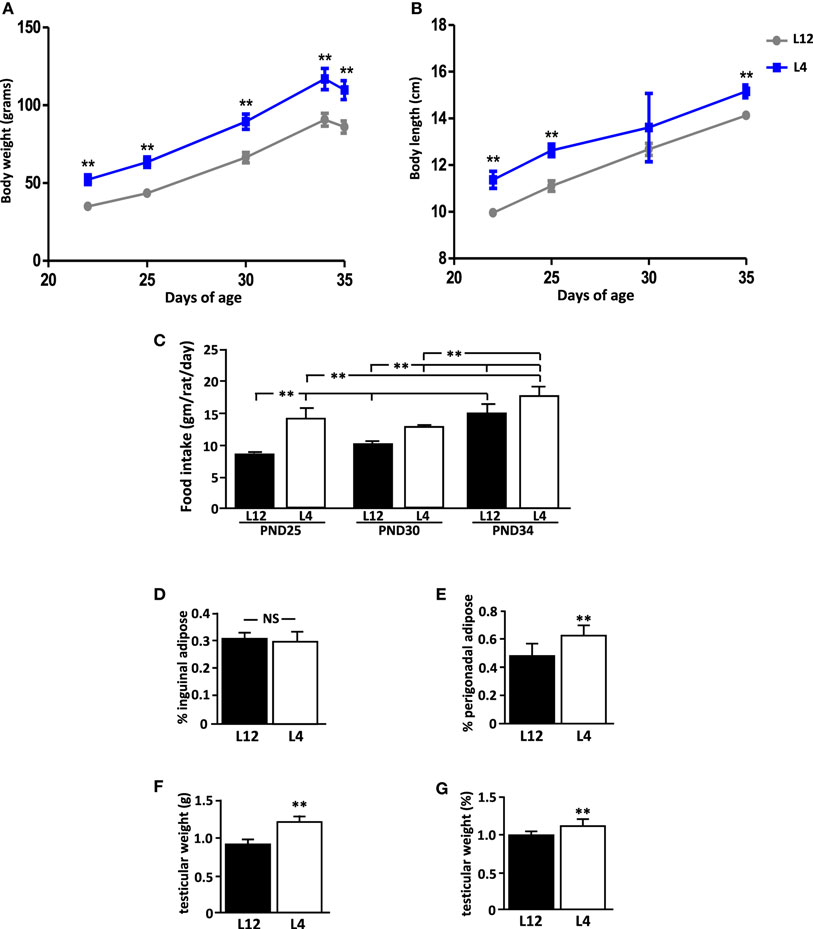
Figure 1. Effect of neonatal overnutrition (ON) on (A) body weight (BW), (B) body length (BL), (C) food intake, (D) inguinal adipose tissue, (E) perigonadal adipose tissue, (F) testicular weight, and (G) normalized testicular weight in peripubertal male rats raised in litters of 12 (L12) or litters of 4 pups (n = 12). PND, postnatal day. **p < 0.01. NS, non-significant. Results are presented as mean ± SEM.
Rats from small litters had a higher food intake (p < 0.01; Figure 1C) during the first part of the study (PND25 and PND30), but this difference was not significant at the end of the study (PND34). Food intake increased throughout the experiment in both groups.
At PND35, there was no difference between the experimental groups in the relative amount of inguinal adipose tissue (Figure 1D), while L4 rats had more perigonadal adipose tissue than L12 rats (p < 0.001; Figure 1E).
External Signs of Puberty and Testicular Weight
No rat showed any signs of pubertal onset at any time throughout the study. At PND35, both the testicular weight (p < 0.01; Figure 1F) and relative testicular weight compared to BW (p < 0.01; Figure 1G) were greater in L4 rats than in L12 rats.
Circulating Hormones during the Peripubertal Period in Response to Leptin
Baseline serum leptin levels were higher in L4 rats compared to L12 rats (p < 0.001; Figure 2A) at both time-points. As expected, circulating leptin levels increased significantly (p < 0.001) after leptin treatment, with no difference between L4 and L12 rats in the levels of serum leptin after leptin injection (Figure 2A).
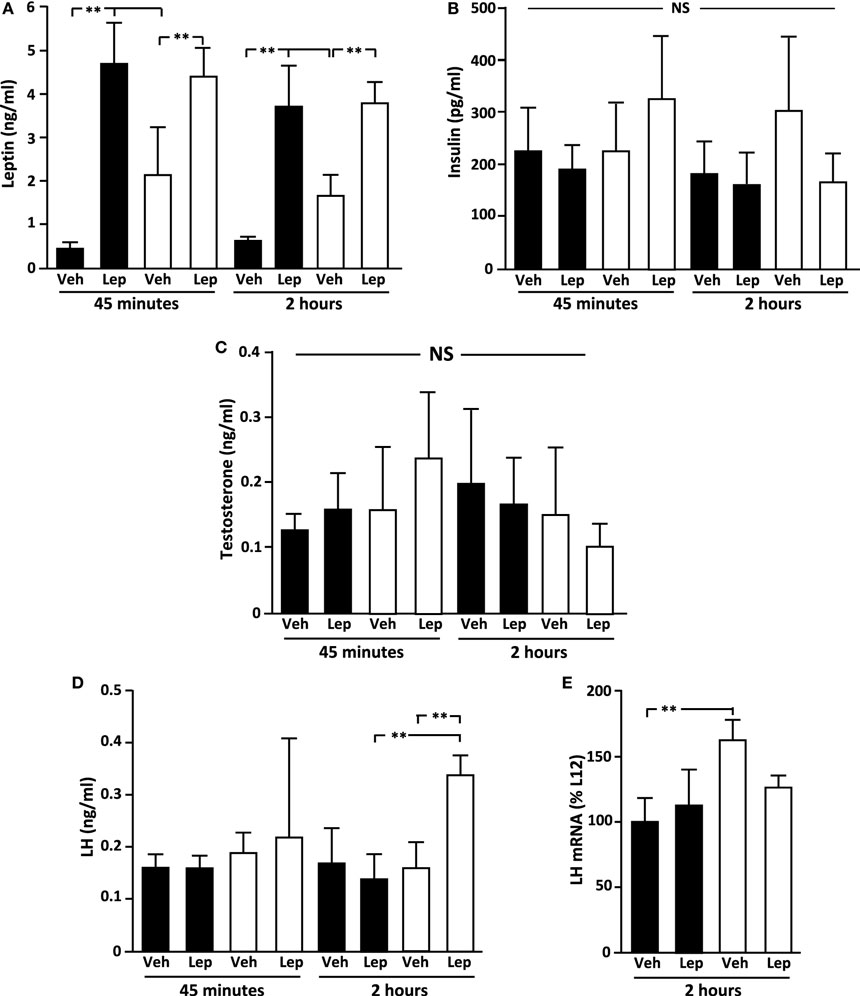
Figure 2. Serum (A) leptin, (B) insulin, (C) testosterone, and (D) luteinizing hormone (LH) levels in peripubertal male rats treated with vehicle (Veh) or leptin (Lep; 3 µg/g body weight) at 45 min and 2 h after administration and (E) LH mRNA levels in the pituitary 2 h after administration. Black bars: males raised in litters of 12 pups. White bars: rats raised in litters of 4 pups. **p < 0.01. NS, non-significant. n = 6/group. Results are presented as mean ± SEM.
There was no effect of either litter size or leptin treatment on circulating insulin (Figure 2B) or testosterone (Figure 2C) levels. The basal concentration of LH was not different between groups. However, 2 h after leptin treatment, LH levels increased significantly in the overweight L4 rats resulting in higher levels compared to both L12 leptin-treated and L4 vehicle-treated rats (p < 0.01; Figure 2D).
Pituitary Hormone Expression
The baseline levels of LH beta-subunit (LHβ) mRNA were higher in L4 rats compared to L12 rats (Figure 2E), with no significant effect of leptin, although there was no difference between the groups after leptin treatment. There were no differences between experimental groups in the mRNA levels of FSHβ, GH, ACTH (POMC), PRL, or TSHβ (Table 1).

Table 1. Relative levels of mRNA of selected genes in the pituitary of male peripubertal rats from litters of 12 (L12) or litters of 4 (L4) pups that were treated with vehicle (control, CT) or leptin (Lep) and sacrificed 2 h later.
Expression of Neuropeptides and Receptors in the Hypothalamus
There was no effect of litter size or leptin treatment on the hypothalamic RNA levels of GnRH, Kiss, or KissR (Table 2). Likewise, there were no differences between experimental groups in the expression of the metabolic neuropeptides, NPY, AgRP, POMC, or orexin, as well as no difference in LepR (Table 2). The mRNA levels of the neuropeptides GHRH and somatostatin, involved in control of systemic growth and also metabolism (34), were unaffected by litter size or leptin treatment (Table 2).
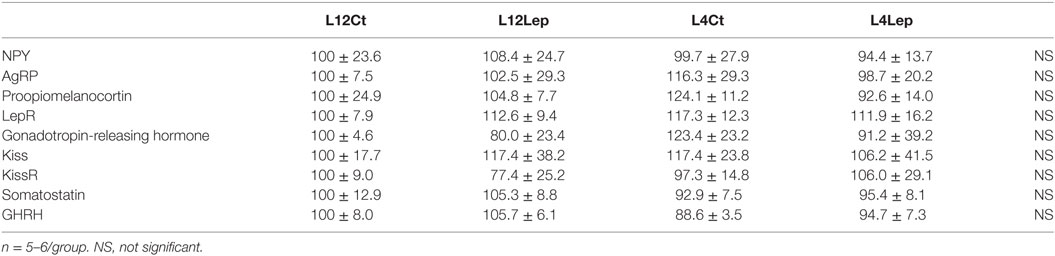
Table 2. Relative levels of mRNA of selected genes in the hypothalamus of male peripubertal rats from litters of 12 (L12) or litters of 4 (L4) pups that were treated with vehicle (control, CT) or leptin (Lep) and sacrificed 2 h later.
Intracellular Signaling
Basal levels of hypothalamic pSTAT3Tyr705 (Figures 3A,B) and pSTAT3Ser727 (Figures 3C,D) were not different between L12 and L4 rats at PND35. Leptin administration increased pSTAT3Tyr705 levels at both 45 min (p < 0.0001) and 2 h (p < 0.001). There was no difference in the response of L12 and L4 rats. In contrast, there was no effect of leptin treatment on pSTAT3Ser727 levels at either time-point. At 45 min, pJAK2 levels were higher in L4 compared to L12 rats in vehicle-treated rats (p < 0.05), with this difference not reaching significance in leptin-treated rats (Figure 3E). At 2 h, pJAK2 levels were higher in L4 leptin treated rats compared to L12 leptin-treated rats and L4 vehicle-treated rats (p < 0.05; Figure 3F).
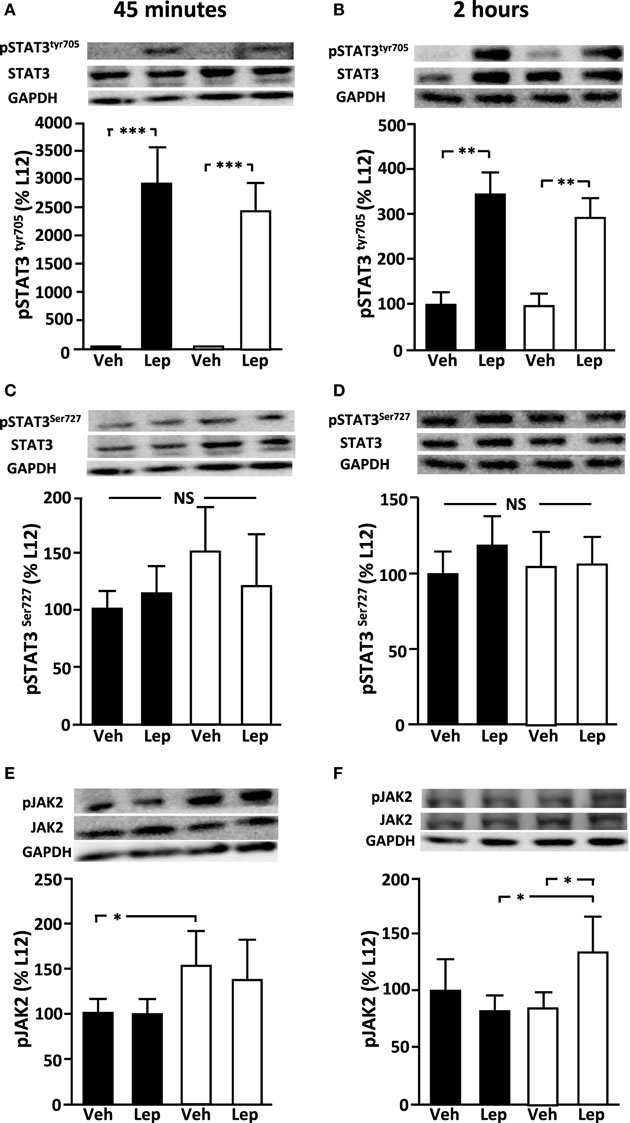
Figure 3. Relative levels of (A,B) pSTAT3tyr705, (C,D) pSTAT3ser727, and (E,F) pJAK2 in the hypothalamus of peripubertal male rats at 45 min (left panel) and 2 h (right panel) after either vehicle (Veh) or leptin (Lep; 3 µg/g body weight) administration. Representative western blots are shown for each protein. Black bars: males raised in litters of 12 pups. White bars: rats raised in litters of 4 pups. *p < 0.05; **p < 0.01. NS, non-significant (n = 6/group). Results are presented as mean ± SEM.
There was no effect of litter size or leptin treatment on relative pAKT, pERK, SOCS3, pPTEN, or PTP1B levels at either 45 min or 2 h (Table 3).
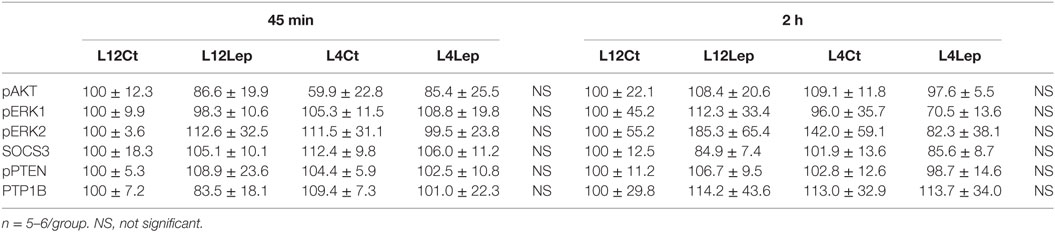
Table 3. Relative protein levels of intracellular signaling molecules in the hypothalamus of male peripubertal rats from litters of 12 (L12) or litters of 4 (L4) pups that were treated with vehicle (control, CT) or leptin (Lep) and sacrificed 45 min or 2 h later.
Discussion
Both over- and undernutrition during prepubertal life can affect the timing of pubertal onset in humans and rodents (1, 2, 5, 6, 8–10, 28), with changes in circulating leptin levels being one of the possible signals involved (15, 21). The experimental model used here to induce overnutrition from the time of birth, a reduction in litter size, has been repeatedly shown to increase body weight, fat mass, and length in male rats (28, 30–33, 35, 36) and has also been shown to advance the mean age of pubertal onset in both sexes (27–29).
Circulating leptin levels are increased as early as 10 days of life in both males and females with neonatal overnutrition (30) and here we found that they continued to be higher at PND35 in males with neonatal overnutrition, as previously reported for peripubertal (PND30) females (31). As leptin is an important permissive signal involved in the timing of pubertal onset (19, 23, 24, 37), the increase in this hormone during prepubertal life could be involved in the advancement of puberty in these animals. Obesity can be associated with leptin resistance (38, 39); however, we previously demonstrated that in female rats overweight due to neonatal overnutrition hypothalamic sensitivity to leptin is accentuated during the peripubertal period (31). In overweight peripubertal females (PND30), activation of hypothalamic pSTAT3 signaling in response to leptin, as well as the expression of LepR, were increased in females from small litters compared to those from large litters (31). In contrast, the increase in hypothalamic pSTAT in response to exogenous leptin did not differ between peripubertal male rats raised in small litters and controls, nor did the mRNA levels of LepR. There were no differences in the activation of MAP kinases, PTP1B, PTEN, or SOCS3 in response to neonatal overnutrition or leptin treatment in male rats, which is also in contrast to that found in females. These differences between males and females in the response to exogenous leptin could be due to innate differences between the sexes, but could also be in part due to the disparity in the levels of leptin reached after exogenous administration. Indeed, the levels obtained in females were much higher (31) than those observed in males. We are unable to explain this difference, as these treatments were performed by the same investigators during the same period of time as those of the female rats in our previous published studies, as were the determinations of leptin levels. It is possible that leptin is cleared from the circulation more rapidly in males compared to females, possibly by binding to specific receptors and being taken-up by target tissues or through renal clearance, but this remains to be demonstrated.
At 45 min, baseline hypothalamic pJAK2 levels were higher in L4 males compared to controls regardless of leptin treatment, which could reflect the higher baseline leptin levels. However, at 2 h after treatment pJAK2 levels were only higher in L4 males treated with leptin, as a result of the normalization of the levels in the L4 vehicle treated males. Thus, an increase in leptin sensitivity may not be involved in pubertal onset in male rats. However, it is also possible that modifications in leptin sensitivity have not yet occurred at PND35 and at an age closer to physiological pubertal onset differential leptin sensitivities might be found. What is clear is that the response of the intracellular pathways activated by leptin are not reduced in these overweight peripubertal males, suggesting that leptin resistance has not yet developed.
In peripubertal males, the baseline expression of metabolic neuropeptides was not affected by neonatal overnutrition or by leptin treatment. This is also in contrast to that previously reported in females (31). In peripubertal females, NPY mRNA levels were decreased and POMC mRNA levels increased in response to leptin in L4 overnourished rats, suggesting a possible increase in the central sensitivity to the anorexic actions of leptin. These changes could be related to the fact that by PND30 neonatally overnourished females have begun to normalize their body composition compared to controls (31) and at later ages females exposed to neonatal overnutrition are less apt to exhibit significant increases in body weight (30). In contrast, males are reported to be less capable of normalizing their food intake/body weight after neonatal overnutrition compared to females (30, 36).
Early overnutrition resulted in increased testicular mass, which might be associated with the advancement of pubertal onset previously reported in this experimental model (28). We might expect testosterone levels to increase with increasing testicular size, and although mean levels were not significantly different, it is possible that the secretory pattern of this steroid was affected. Indeed, testosterone values varied widely within each experimental group and this could be due to the occurrence of secretory bursts and troughs, possibly indicating pulsatile awakening and the nearing of pubertal onset. However, in rats, changes in testicular volume are not necessarily correlated with changes in serum testosterone levels in rodents (40, 41), as reported to occur in adult humans (42). In rodents, testicular volume increase from birth into early adulthood (43) and neonatal overnutrition appears to advance this age-related increase.
Basal circulating LH levels were not altered in these overnourished males, but basal LH β-subunit mRNA levels were increased in the anterior pituitary. Similar to the results regarding testosterone, single sampling of a hormone with pulsatile secretion could underlie the large standard errors in LH values. The higher levels of basal LH β-subunit expression in L4 rats could indicate greater synthesis of this gonadotrophin, with this gonadotrophin being stored in preparation for its release in response to stimulation. Indeed, LH secretion was significantly greater in response to leptin treatment in these overweight peripubertal pups, which could reflect higher LH stores available for release. It is also possible that in overweight L4 rats there is increased sensitivity to leptin to induce LH release, which could occur at the hypothalamic level (21, 44). However, at 2 h the mRNA levels of LH in L4 rats treated with leptin were no longer higher than those found in L12 rats. Leptin could possibly be directly inhibiting LH expression in these rats at the pituitary level, although recent studies suggest that this is not the case (45). It is also plausible that the increased release of LH itself could feedback to inhibit its own expression. In contrast, no effect of overnutrition or leptin was found on GnRH or Kiss1 gene expression levels, which might indicate that a direct effect of leptin on the pituitary underlies these results.
As stated above, the peripubertal response of male rats to overnutrition and leptin administration was different from that reported previously in females (31). This could reflect the innate differences between the sexes in the neuroendocrine control of puberty and reproductive functions. Indeed, the GnRH/Kiss1 system is sexually dimorphic (46–48). Moreover, the interplay of neurons involved in metabolism and the interaction between nutrition and reproductive function also appears to be different between sexes. For example, the lack of leptin signaling in AgRP neurons was found to delay puberty in female mice, but with no significant effect in males (19). It is possible that the requirement of an adequate body weight for reproductive capacity is greater in females than in males, rendering them more sensitive to modifications in body weight during the peripubertal period. Indeed, we found few changes in the response of peripubertal males to leptin in the paradigm used here. It is possible that modifications in central leptin sensitivity are not involved in the advancement in pubertal onset of overnourished/overweight male rats or that central changes occur later than the age used here to analyze the response to leptin, i.e., PND35. However, the increase in testicular size and LH levels suggests that there are peripubertal differences in the reproductive system between control and overnourished male rats.
In conclusion, although pubertal onset can be modified by nutritional signals in both males and females, the sensitivity to these signals, as well as the mechanisms involved, may differ between the sexes. The advancement in the mean age at pubertal onset of both males and females as a result of early overnutrition/obesity is of concern considering the continuing rise in the prevalence of obesity in the pediatric population in developed countries and the possible long-term effects of this pubertal advance. Our results suggest that studies aimed at understanding the underlying mechanisms involved in this obesity-associated pubertal advancement should include both males and females.
Ethics Statement
The authors have nothing to disclose.
Author Contributions
PA-A: performed animal experiments, performed assays, and wrote manuscript; DC-G: performed animal experiments and performed assays; FD: performed assays; MF-G: performed assays; MS-G: performed animal experiments; MT-S: designed experiment and interpreted results; JA: designed experiment and interpreted results; JC: designed experiment, interpreted results, and wrote manuscript.
Conflict of Interest Statement
The authors declare that the research was conducted in the absence of any commercial or financial relationships that could be construed as a potential conflict of interest.
Acknowledgments
We thank Sandra Canelles for technical assistance. The authors are funded by Fondos de Investigación Sanitaria (PI1600485 to JA), Ministerio de Ciencia e Innovación (BFU2014-51836-C2-2-R and BFU2017-82565-C2-1-R to JC) and fondos FEDER, Centro de Investigación Biomédica en Red Fisiopatología de Obesidad y Nutrición (CIBEROBN), Instituto de Salud Carlos III (JA), and Fundación Endocrinología y Nutrición.
References
1. Cousminer DL, Berry DJ, Timpson NJ, Ang W, Thiering E, Byrne EM, et al. Genome-wide association and longitudinal analyses reveal genetic loci linking pubertal height growth, pubertal timing and childhood adiposity. Hum Mol Genet (2013) 22(13):2735–47. doi:10.1093/hmg/ddt104
2. Cousminer DL, Stergiakouli E, Berry DJ, Ang W, Groen-Blokhuis MM, Korner A, et al. Genome-wide association study of sexual maturation in males and females highlights a role for body mass and menarche loci in male puberty. Hum Mol Genet (2014) 23(16):4452–64. doi:10.1093/hmg/ddu150
3. Pinilla L, Aguilar E, Dieguez C, Millar RP, Tena-Sempere M. Kisspeptins and reproduction: physiological roles and regulatory mechanisms. Physiol Rev (2012) 92(3):1235–316. doi:10.1152/physrev.00037.2010
4. Sisk CL, Foster DL. The neural basis of puberty and adolescence. Nat Neurosci (2004) 7(10):1040–7. doi:10.1038/nn1326
5. Roth CL, DiVall S. Consequences of early life programing by genetic and environmental influences: a synthesis regarding pubertal timing. Endocr Dev (2016) 29:134–52. doi:10.1159/000438883
6. Munoz-Calvo MT, Argente J. Nutritional and pubertal disorders. Endocr Dev (2016) 29:153–73. doi:10.1159/000438884
7. Warren MP. The effects of exercise on pubertal progression and reproductive function in girls. J Clin Endocrinol Metab (1980) 51(5):1150–7. doi:10.1210/jcem-51-5-1150
8. Aksglaede L, Juul A, Olsen LW, Sorensen TI. Age at puberty and the emerging obesity epidemic. PLoS One (2009) 4(12):e8450. doi:10.1371/journal.pone.0008450
9. Aksglaede L, Sorensen K, Petersen JH, Skakkebaek NE, Juul A. Recent decline in age at breast development: the Copenhagen Puberty Study. Pediatrics (2009) 123(5):e932–9. doi:10.1542/peds.2008-2491
10. De Leonibus C, Marcovecchio ML, Chiavaroli V, de Giorgis T, Chiarelli F, Mohn A. Timing of puberty and physical growth in obese children: a longitudinal study in boys and girls. Pediatr Obes (2014) 9(4):292–9. doi:10.1111/j.2047-6310.2013.00176.x
11. Kaplowitz PB, Slora EJ, Wasserman RC, Pedlow SE, Herman-Giddens ME. Earlier onset of puberty in girls: relation to increased body mass index and race. Pediatrics (2001) 108(2):347–53. doi:10.1542/peds.108.2.347
12. Day FR, Elks CE, Murray A, Ong KK, Perry JR. Puberty timing associated with diabetes, cardiovascular disease and also diverse health outcomes in men and women: the UK Biobank study. Sci Rep (2015) 5:11208. doi:10.1038/srep11208
13. Argente J, Barrios V, Chowen JA, Sinha MK, Considine RV. Leptin plasma levels in healthy Spanish children and adolescents, children with obesity, and adolescents with anorexia nervosa and bulimia nervosa. J Pediatr (1997) 131(6):833–8. doi:10.1016/S0022-3476(97)70029-5
14. Maffei M, Halaas J, Ravussin E, Pratley RE, Lee GH, Zhang Y, et al. Leptin levels in human and rodent: measurement of plasma leptin and ob RNA in obese and weight-reduced subjects. Nat Med (1995) 1(11):1155–61. doi:10.1038/nm1195-1155
15. Matkovic V, Ilich JZ, Skugor M, Badenhop NE, Goel P, Clairmont A, et al. Leptin is inversely related to age at menarche in human females. J Clin Endocrinol Metab (1997) 82(10):3239–45. doi:10.1210/jcem.82.10.4280
16. Elias CF, Aschkenasi C, Lee C, Kelly J, Ahima RS, Bjorbaek C, et al. Leptin differentially regulates NPY and POMC neurons projecting to the lateral hypothalamic area. Neuron (1999) 23(4):775–86. doi:10.1016/S0896-6273(01)80035-0
17. Korner J, Savontaus E, Chua SC Jr, Leibel RL, Wardlaw SL. Leptin regulation of Agrp and Npy mRNA in the rat hypothalamus. J Neuroendocrinol (2001) 13(11):959–66. doi:10.1046/j.1365-2826.2001.00716.x
18. Donato J Jr, Cravo RM, Frazao R, Gautron L, Scott MM, Lachey J, et al. Leptin’s effect on puberty in mice is relayed by the ventral premammillary nucleus and does not require signaling in Kiss1 neurons. J Clin Invest (2011) 121(1):355–68. doi:10.1172/JCI45106
19. Egan OK, Inglis MA, Anderson GM. Leptin signaling in AgRP neurons modulates puberty onset and adult fertility in mice. J Neurosci (2017) 37(14):3875–86. doi:10.1523/JNEUROSCI.3138-16.2017
20. Sanchez-Garrido MA, Tena-Sempere M. Metabolic control of puberty: roles of leptin and kisspeptins. Horm Behav (2013) 64(2):187–94. doi:10.1016/j.yhbeh.2013.01.014
21. Manfredi-Lozano M, Roa J, Ruiz-Pino F, Piet R, Garcia-Galiano D, Pineda R, et al. Defining a novel leptin-melanocortin-kisspeptin pathway involved in the metabolic control of puberty. Mol Metab (2016) 5(10):844–57. doi:10.1016/j.molmet.2016.08.003
22. Chehab FF, Lim ME, Lu R. Correction of the sterility defect in homozygous obese female mice by treatment with the human recombinant leptin. Nat Genet (1996) 12(3):318–20. doi:10.1038/ng0396-318
23. Farooqi IS, Matarese G, Lord GM, Keogh JM, Lawrence E, Agwu C, et al. Beneficial effects of leptin on obesity, T cell hyporesponsiveness, and neuroendocrine/metabolic dysfunction of human congenital leptin deficiency. J Clin Invest (2002) 110(8):1093–103. doi:10.1172/JCI15693
24. Farooqi IS, Wangensteen T, Collins S, Kimber W, Matarese G, Keogh JM, et al. Clinical and molecular genetic spectrum of congenital deficiency of the leptin receptor. N Engl J Med (2007) 356(3):237–47. doi:10.1056/NEJMoa063988
25. Mounzih K, Lu R, Chehab FF. Leptin treatment rescues the sterility of genetically obese ob/ob males. Endocrinology (1997) 138(3):1190–3. doi:10.1210/endo.138.3.5024
26. Chehab FF, Mounzih K, Lu R, Lim ME. Early onset of reproductive function in normal female mice treated with leptin. Science (1997) 275(5296):88–90. doi:10.1126/science.275.5296.88
27. Bohlen TM, Silveira MA, Zampieri TT, Frazao R, Donato J Jr. Fatness rather than leptin sensitivity determines the timing of puberty in female mice. Mol Cell Endocrinol (2016) 423:11–21. doi:10.1016/j.mce.2015.12.022
28. Sanchez-Garrido MA, Castellano JM, Ruiz-Pino F, Garcia-Galiano D, Manfredi-Lozano M, Leon S, et al. Metabolic programming of puberty: sexually dimorphic responses to early nutritional challenges. Endocrinology (2013) 154(9):3387–400. doi:10.1210/en.2012-2157
29. Wu XQ, Li XF, Xia WT, Ye B, O’Byrne KT. The effects of small litter rearing on ovarian function at puberty and adulthood in the rat. Reprod Biol (2016) 16(2):130–7. doi:10.1016/j.repbio.2016.02.001
30. Argente-Arizon P, Ros P, Diaz F, Fuente-Martin E, Castro-Gonzalez D, Sanchez-Garrido MA, et al. Age and sex dependent effects of early overnutrition on metabolic parameters and the role of neonatal androgens. Biol Sex Differ (2016) 7:26. doi:10.1186/s13293-016-0079-5
31. Castro-Gonzalez D, Fuente-Martin E, Sanchez-Garrido MA, Argente-Arizon P, Tena-Sempere M, Barrios V, et al. Increased prepubertal body weight enhances leptin sensitivity in proopiomelanocortin and neuropeptide y neurons before puberty onset in female rats. Endocrinology (2015) 156(4):1272–82. doi:10.1210/en.2014-1759
32. Fuente-Martin E, Garcia-Caceres C, Granado M, de Ceballos ML, Sanchez-Garrido MA, Sarman B, et al. Leptin regulates glutamate and glucose transporters in hypothalamic astrocytes. J Clin Invest (2012) 122(11):3900–13. doi:10.1172/JCI64102
33. Glavas MM, Kirigiti MA, Xiao XQ, Enriori PJ, Fisher SK, Evans AE, et al. Early overnutrition results in early-onset arcuate leptin resistance and increased sensitivity to high-fat diet. Endocrinology (2010) 151(4):1598–610. doi:10.1210/en.2009-1295
34. Stanley TL, Grinspoon SK. Effects of growth hormone-releasing hormone on visceral fat, metabolic, and cardiovascular indices in human studies. Growth Horm IGF Res (2015) 25(2):59–65. doi:10.1016/j.ghir.2014.12.005
35. Fuente-Martin E, Garcia-Caceres C, Granado M, Sanchez-Garrido MA, Tena-Sempere M, Frago LM, et al. Early postnatal overnutrition increases adipose tissue accrual in response to a sucrose-enriched diet. Am J Physiol Endocrinol Metab (2012) 302(12):E1586–98. doi:10.1152/ajpendo.00618.2011
36. Stefanidis A, Spencer SJ. Effects of neonatal overfeeding on juvenile and adult feeding and energy expenditure in the rat. PLoS One (2012) 7(12):e52130. doi:10.1371/journal.pone.0052130
37. Yura S, Ogawa Y, Sagawa N, Masuzaki H, Itoh H, Ebihara K, et al. Accelerated puberty and late-onset hypothalamic hypogonadism in female transgenic skinny mice overexpressing leptin. J Clin Invest (2000) 105(6):749–55. doi:10.1172/JCI8353
38. Donoso MA, Munoz-Calvo MT, Barrios V, Martinez G, Hawkins F, Argente J. Increased leptin/adiponectin ratio and free leptin index are markers of insulin resistance in obese girls during pubertal development. Horm Res Paediatr (2013) 80(5):363–70. doi:10.1159/000356046
39. Flier JS, Maratos-Flier E. Leptin’s physiologic role: does the emperor of energy balance have no clothes? Cell Metab (2017) 26(1):24–6. doi:10.1016/j.cmet.2017.05.013
40. Chen H, Hardy MP, Huhtaniemi I, Zirkin BR. Age-related decreased Leydig cell testosterone production in the brown Norway rat. J Androl (1994) 15(6):551–7.
41. Kim IS, Ariyaratne HB, Mendis-Handagama SM. Changes in the testis interstitium of Brown Norway rats with aging and effects of luteinizing and thyroid hormones on the aged testes in enhancing the steroidogenic potential. Biol Reprod (2002) 66(5):1359–66. doi:10.1095/biolreprod66.5.1359
42. Ruiz-Olvera SF, Rajmil O, Sanchez-Curbelo JR, Vinay J, Rodriguez-Espinosa J, Ruiz-Castane E. Association of serum testosterone levels and testicular volume in adult patients. Andrologia (2018) 50(3):e12933. doi:10.1111/and.12933
43. Ariyaratne HB, Chamindrani Mendis-Handagama S. Changes in the testis interstitium of Sprague Dawley rats from birth to sexual maturity. Biol Reprod (2000) 62(3):680–90. doi:10.1095/biolreprod62.3.680
44. Quennell JH, Mulligan AC, Tups A, Liu X, Phipps SJ, Kemp CJ, et al. Leptin indirectly regulates gonadotropin-releasing hormone neuronal function. Endocrinology (2009) 150(6):2805–12. doi:10.1210/en.2008-1693
45. Sarmento-Cabral A, Peinado JR, Halliday LC, Malagon MM, Castano JP, Kineman RD, et al. Adipokines (leptin, adiponectin, resistin) differentially regulate all hormonal cell types in primary anterior pituitary cell cultures from two primate species. Sci Rep (2017) 7:43537. doi:10.1038/srep43537
46. de Croft S, Piet R, Mayer C, Mai O, Boehm U, Herbison AE. Spontaneous kisspeptin neuron firing in the adult mouse reveals marked sex and brain region differences but no support for a direct role in negative feedback. Endocrinology (2012) 153(11):5384–93. doi:10.1210/en.2012-1616
47. Ruiz-Pino F, Navarro VM, Bentsen AH, Garcia-Galiano D, Sanchez-Garrido MA, Ciofi P, et al. Neurokinin B and the control of the gonadotropic axis in the rat: developmental changes, sexual dimorphism, and regulation by gonadal steroids. Endocrinology (2012) 153(10):4818–29. doi:10.1210/en.2012-1287
Keywords: neonatal, overnutrition, leptin, hypothalamus, puberty
Citation: Argente-Arizón P, Castro-González D, Díaz F, Fernández-Gómez MJ, Sánchez-Garrido MA, Tena-Sempere M, Argente J and Chowen JA (2018) Neonatal Overnutrition Increases Testicular Size and Expression of Luteinizing Hormone β-Subunit in Peripubertal Male Rats. Front. Endocrinol. 9:168. doi: 10.3389/fendo.2018.00168
Received: 05 December 2017; Accepted: 28 March 2018;
Published: 13 April 2018
Edited by:
Sebastien G. Bouret, University of Southern California, United StatesReviewed by:
Andras Garami, University of Pécs, HungarySoyoung Park, Children’s Hospital of Los Angeles, United States
Copyright: © 2018 Argente-Arizón, Castro-González, Díaz, Fernández-Gómez, Sánchez-Garrido, Tena-Sempere, Argente and Chowen. This is an open-access article distributed under the terms of the Creative Commons Attribution License (CC BY). The use, distribution or reproduction in other forums is permitted, provided the original author(s) and the copyright owner are credited and that the original publication in this journal is cited, in accordance with accepted academic practice. No use, distribution or reproduction is permitted which does not comply with these terms.
*Correspondence: Jesús Argente, amVzdXMuYXJnZW50ZSYjeDAwMDQwO3VhbS5lcw==;
Julie A. Chowen, anVsaWVhbm4uY2hvd2VuJiN4MDAwNDA7c2FsdWQubWFkcmlkLm9yZw==