- Section of Pharmacology and Toxicology, Department of Psychology, Neurology, Drug Sciences, Health of the Child, Pharmacology, University of Florence, Florence, Italy
A commentary on
3-Iodothyronamine reduces insulin secretion in vitro via a mitochondrial mechanism
by Lehmphul I, Hoefig CS, Köhrle J. J Mol Cell Endocrinol (2017) 460:219–228. doi: 10.1016/j.mce.2017.07.026
Lehmphul et al. report the effect of 3-iodothyronamine in reducing insulin release in a model of immortalized pancreatic β-cells. Notwithstanding the simplified β-cell model used, this article offers an opportunity to reconsider, possibly under a new light, an old issue of research, which excited people working on amine oxidases (AOs) in the last 20 years. Toward this aim, we would like to propose some points of reflection to the scientific community working on 3-iodothyronamine and thyroid hormone metabolites:
1. the paper indicates that 3-iodothyronamine reduces insulin release with a mechanism mediated, at least in part, by its oxidative metabolite, the 3-iodothyroacetic acid, produced by mitochondrial monoamine oxidase (MAOs), type B (MAO-B) activity. This finding, confirming our observations and hypothesis on the role of 3-iodothyronamine as a source of active metabolites (1, 2), demonstrates for the first time that 3-iodothyronamine is a substrate for MAO-B, the MAO isoform in search of substrates and of functions;
2. the degradation of 3-iodothyronamine by MAO-B, with production of the corresponding aldehyde and hydrogen peroxide (H2O2), potentially represents a self-standing mechanism independently of 3-iodothyronamine receptor activation on pancreatic cells.
Amine oxidases are a heterogeneous class of enzymes, including MAOs (type A and B) and semicarbazide-sensitive amine oxidases (SSAOs). While MAOs are ubiquitous enzymes, being linked to the outer mitochondrial membrane (active site facing the cytoplasm), plasma membrane SSAOs can have selective and species-specific tissue/cell expression. In addition, MAOs and SSAOs are distinguishable by inhibitor sensitivity, substrate selectivity and affinity, and subcellular localization. Noradrenaline and serotonin are among MAO-A substrates, dopamine and other trace amines, including tyramine and β-phenylethylamine, are MAO-A, B, and SSAO substrates. Up to now, direct evidence that 3-iodothyronamine is a substrate for MAO-A is lacking. However, now we know that 3-iodothyroanime is a substrate for MAO-B.
AO Catalysis: A Pro-Oxidant Source for Diabetes Complications
The oxidative deamination carried out by AOs produces substrate-derived aldehydes, H2O2, and ammonia. Aldehydes and H2O2 are well known pro-oxidant compounds scavenged by aldehyde dehydrogenase(s) and catalase activities, respectively, to the corresponding carboxylic acid and water. If produced outside the cell by SSAO activity, H2O2 may have two fates: to enter cells or to remain outside cells. Both conditions can be a trigger for intra- or extracellular milieu oxidation with the latter compartmentalization as a pathogenic mechanistic event generating micro- and macrovascular damage. Aldehydes from SSAO catalysis can generate carbonylation of extracellular proteins as their scavenging to the corresponding carboxylic acid can only occur intracellularly.
If produced, by MAO activities, H2O2 may be scavenged by catalase or freely diffused throughout organelle membranes, generating a potential localized change in the redox state, a condition recognized as one among the main pathogenic events triggering the pancreatic dysfunction, insulin resistance, and long-term deleterious effects in exhausting cell/tissue antioxidant defenses. Furthermore, insulin-target cells were described as a preferential site for SSAO and MAO expression (2, 3), with their activities further increased in hyperglycemia (4) as well as in hypertension, obesity, and in other cardiovascular diseases (2–5), likely as a consequence of increased levels of pro-inflammatory signals (6).
AO Catalysis: The Hypoglycemic and Insulin Mimetic Effects of AO Substrates
Hydrogen peroxide can also have beneficial signaling activities, including its capacity to activate the trafficking of GLUT4 in adipocytes and other insulin-sensitive cells. Several studies have highlighted the use of high concentrations of non-selective SSAO and MAO substrates in stimulating GLUT4 activity, thus reducing hyperglycemia and mimicking insulin effects, including adipocyte differentiation (7–9). On the other hand, SSAO substrate degradation was found to be a trigger for the generation of advanced glycation products (10). Therefore, whether AO inhibitors or substrates should be proposed for controlling diabetes thus remained an open issue (11).
Protective Effects of AO Inhibition: Clinical and Experimental Evidence
Aminoguanidine, an inhibitor of SSAOs, is effective in reducing advanced glycation end products in diabetic patients and in experimental diabetes (12, 13). More interestingly, clinical and experimental evidence indicate that the beneficial effects of drug targeting angiotensin II cascade in preventing diabetes complications might include the control of MAO activities (14, 15), which may play a pathogenic role in different cardiomyopathies (5).
These evidence confirm the pro-oxidant and pro-inflammatory role for AO catalysis and an overall beneficial effect of reducing their activities.
3-Iodothyronamine: What’s New?
The novel fact is that 3-iodothyronamine (i) is a common endogenous substrate for MAO-B and SSAOs, (ii) its plasma levels increased in diabetic patients (16), and (iii) when administered to mice it induces hyperglycemia (central and/or peripheral effects) with a mechanism that remains to be clarified but dependent, at least in part, on MAO activity (17, 18). To note, we have collected evidence demonstrating that MAO activities are involved in the conversion of endogenous but also pharmacological administered 3-iodothyronamine into 3-iodothyroacetic acid (19). This latter result suggests that 3-iodothyroacetic acid/3-iodothyronamine might be homeostatically regulated via AO activities.
Even if it is not demonstrated yet, under conditions of hyperglycemia, the products of oxidative deamination of 3-iodothyronamine are expected to increase. Overall, hyperglycemia might reflect a condition of an unbalanced 3-iodothyronamine rate of synthesis and degradation, making available a great amount of the “pro-diabetic” 3-iodothyroacetic acid and pro-oxidant compounds, which can exacerbate diabetes and its complications.
Since thyroid dysfunctions are a risk factor for diabetes and because 3-iodothyroacetic acid/3-iodothyronamine seems to be homeostatically regulated, the circle around AOs and hyperglycemia might be conclusively closed. Consequently, the measure of 3-iodothyroacetic acid/3-iodothyronamine plasma levels may have diagnostic relevance to predict the risk of hyperglycemia (Figure 1).
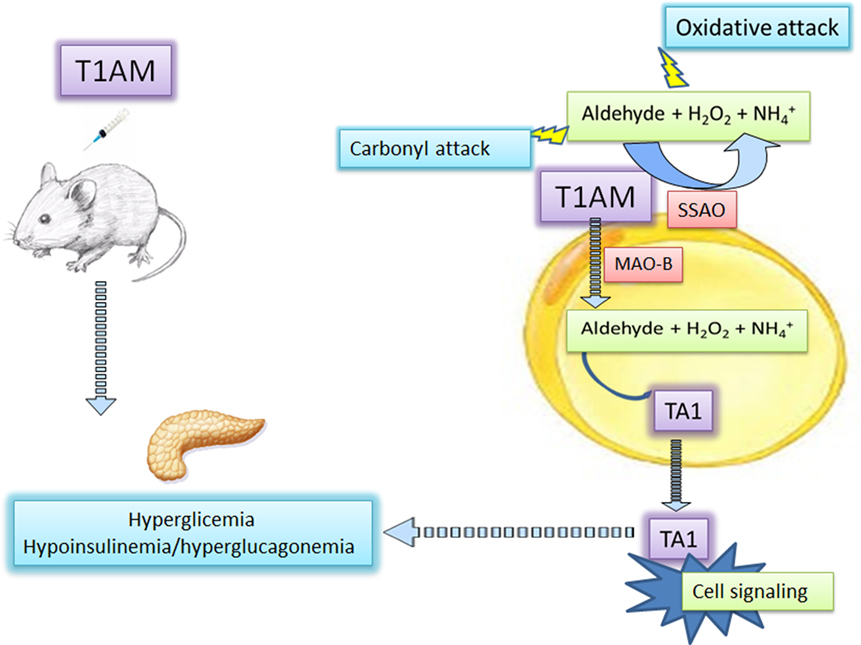
Figure 1. 3-Iodothyronamine and hyperglycemia: the mediation of amine oxidases. 3-Iodothyronamine (T1AM) injected in mice induces hyperglycemia likely interacting at different pancreatic receptors promoting glucagon and reducing insulin release. At insulin-sensitive cells, including the pancreas, T1AM is converted into 3-iodotyroactic acid (TA1), the oxidative metabolite of T1AM by the activities of mitochondrial monoamine oxidase (MAOs), or semicarbazide-sensitive amine oxidases (SSAOs). TA1 can diffuse from cells and induce cell signaling activities and promoting hyperglycemia. The secondary product of amine oxidase activities, i.e., hydrogen peroxide (H2O2) and the aldehyde, may promote oxidative attack to cell components.
Author Contributions
AL and LR wrote the paper and prepared the figure.
Conflict of Interest Statement
The authors declare that the research was conducted in the absence of any commercial or financial relationships that could be construed as a potential conflict of interest.
Acknowledgments
This paper was supported by a grant from the University of Florence and from Ente Cassa di Risparmio di Firenze 2017 to LR.
References
1. Laurino A, Raimondi L. Torpor: the rise and fall of 3-monoiodothyronamine from brain to gut-from gut to brain? Front Endocrinol (2017) 8:206. doi:10.3389/fendo.200206
2. Raimondi L, Pirisino R, Ignesti G, Capecchi S, Banchelli G, Buffoni F. Semicarbazide-sensitive amine oxidase activity (SSAO) of rat epididymal white adipose tissue. Biochem Pharmacol (1991) 41:467–70. doi:10.1016/0006-2952(91)90549-K
3. Carpéné C, Bour S, Visentin V, Pellati F, Benvenuti S, Iglesias-Osma MC, et al. Amine oxidase substrates for impaired glucose tolerance correction. J Physiol Biochem (2005) 61(2):405–19. doi:10.1007/BF03167058
4. Mészáros Z, Szombathy T, Raimondi L, Karádi I, Romics L, Magyar K. Elevated serum semicarbazide-sensitive amine oxidase activity in non-insulin-dependent diabetes mellitus: correlation with body mass index and serum triglyceride. Metabolism (1999) 48:113–7. doi:10.1016/S0026-0495(99)90019-7
5. Manni ME, Rigacci S, Borchi E, Bargelli V, Miceli C, Giordano C, et al. Monoamine oxidase is overactivated in left and right ventricles from ischemic hearts: an intriguing therapeutic target. Oxid Med Cell Longev (2016) 16:4375418. doi:10.1155/2016/4375418
6. Manni ME, Zazzeri M, Musilli C, Bigagli E, Lodovici M, Raimondi L. Exposure of cardiomyocytes to angiotensin II induces over-activation of monoamine oxidase type A: implications in heart failure. Eur J Pharmacol (2013) 718:271–6. doi:10.1016/j.ejphar.2013.08.022
7. Morin N, Visentin V, Calise D, Marti L, Zorzano A, Testar X, et al. Tyramine stimulates glucose uptake in insulin-sensitive tissues in vitro and in vivo via its oxidation by amine oxidases. J Pharmacol Exp Ther (2002) 303:1238–47. doi:10.1124/jpet.102.040592
8. Morin N, Lizcano JM, Fontana E, Marti L, Smih F, Rouet P, et al. Semicarbazide-sensitive amine oxidase substrates stimulate glucose transport and inhibit lipolysis in human adipocytes. J Pharmacol Exp Ther (2001) 297:563–72.
9. Carpéné C, Daviaud D, Boucher J, Bour S, Visentin V, Grès S, et al. Short- and long-term insulin-like effects of monoamine oxidases and semicarbazide-sensitive amine oxidase substrates in cultured adipocytes. Metabolism (2006) 55:1397–405. doi:10.1016/j.metabol.2006.06.011
10. Yu PH, Wright S, Fan EH, Lun ZR, Gubisne-Harberle D. Physiological and pathological implications of semicarbazide-sensitive amine oxidase. Biochim Biophys Acta (2003) 1647:193–9. doi:10.1016/S1570-9639(03)00101-8
11. Stolen CM, Madanat R, Marti L, Kari S, Yegutkin GG, Sariola H, et al. Semicarbazide sensitive amine oxidase overexpression has dual consequences: insulin mimicry and diabetes-like complications. FASEB J (2004) 18:702–4. doi:10.1096/fj.03-0562fje
12. Ronald L, Engerman E, Kern TS. Pharmacological inhibition of diabetic retinopathy aminoguanidine and aspirin. Diabetes (2001) 50:1636–42. doi:10.2337/diabetes.50.7.1636
13. Nenna A, Nappi F, Avtaar Singh SS, Sutherland FW, Di Domenico F, Chello M, et al. Pharmacologic approaches against advanced glycation end products (AGEs) in diabetic cardiovascular disease. Res Cardiovasc Med (2015) 4:e26949. doi:10.5812/cardiovascmed.4(2)2015.26949
14. Raasch W, Bartels T, Gieselberg A, Dendorfer A, Dominiak P. Angiotensin I-converting enzyme inhibition increases cardiac catecholamine content and reduces monoamine oxidase activity via an angiotensin type 1 receptor-mediated mechanism. J Pharmacol Exp Ther (2002) 300:428–34. doi:10.1124/jpet.300.2.428
15. Manni ME, Bigagli E, Lodovici M, Zazzeri M, Raimondi L. The protective effect of losartan in the nephropathy of the diabetic rat includes the control of monoamine oxidase type A activity. Pharmacol Res (2012) 65:465–71. doi:10.1016/j.phrs.2011.11.010
16. Galli E, Marchini M, Saba A, Berti S, Tonacchera M, Vitti P, et al. Detection of 3-iodothyronamine in human patients: a preliminary study. J Clin Endocrinol Metab (2012) 97:E69–74. doi:10.1210/jc.2011-1115
17. Regard JB, Kataoka H, Cano DA, Camerer E, Yin L, Zheng YW, et al. Probing cell type-specific functions of Gi in vivo identifies GPCR regulators of insulin secretion. J Clin Invest (2007) 117:4034–43. doi:10.1172/JCI32994
18. Manni ME, De Siena G, Saba A, Marchini M, Dicembrini I, Bigagli E, et al. 3-Iodothyronamine: a modulator of the hypothalamus-pancreas-thyroid axes in mice. Br J Pharmacol (2012) 166:650–8. doi:10.1111/j.1476-5381.2011.01823.x
19. Laurino A, De Siena G, Saba A, Chiellini G, Landucci E, Zucchi R, et al. In the brain of mice, 3-iodothyronamine (T1AM) is converted into 3-iodothyroacetic acid (TA1) and it is included within the signaling network connecting thyroid hormone metabolites with histamine. Eur J Pharmacol (2015) 15(761):130–4. doi:10.1016/j.ejphar.2015.04.038
Keywords: 3-iodothyronamine, 3-iodothyroacetic acid, hyperglycemia, diabetes, amine oxidases, amine oxidase inhibitors
Citation: Laurino A and Raimondi L (2018) Commentary: 3-Iodothyronamine Reduces Insulin Secretion In Vitro via a Mitochondrial Mechanism. Front. Endocrinol. 9:57. doi: 10.3389/fendo.2018.00057
Received: 12 September 2017; Accepted: 09 February 2018;
Published: 28 February 2018
Edited by:
Alessandro Antonelli, University of Pisa, ItalyReviewed by:
Jean Albert Boutin, Servier, FranceThomas Scanlan, Oregon Health & Science University, United States
Copyright: © 2018 Laurino and Raimondi. This is an open-access article distributed under the terms of the Creative Commons Attribution License (CC BY). The use, distribution or reproduction in other forums is permitted, provided the original author(s) and the copyright owner are credited and that the original publication in this journal is cited, in accordance with accepted academic practice. No use, distribution or reproduction is permitted which does not comply with these terms.
*Correspondence: Laura Raimondi, bGF1cmEucmFpbW9uZGkmI3gwMDA0MDt1bmlmaS5pdA==