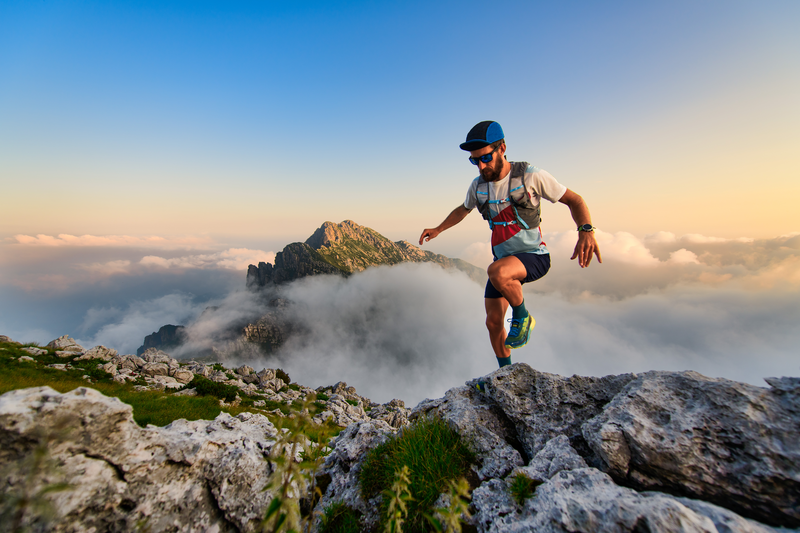
95% of researchers rate our articles as excellent or good
Learn more about the work of our research integrity team to safeguard the quality of each article we publish.
Find out more
ORIGINAL RESEARCH article
Front. Endocrinol. , 13 February 2018
Sec. Experimental Endocrinology
Volume 9 - 2018 | https://doi.org/10.3389/fendo.2018.00036
This article is part of the Research Topic Current Perspectives on Insulin-like Growth Factor Binding Protein (IGFBP) Research View all 16 articles
In previous work using market-weight pigs, we had demonstrated that insulin-like growth factors (IGFs) and insulin-like growth factor binding proteins (IGFBPs) are regulated during shipment characterized by changing conditions of stress due to loading or unloading, transportation, lairage, and slaughter. In addition, we found in a previous study that IGFBP-2 concentrations were lower in pigs transported for longer periods of time. Therefore, we performed a more detailed study on the effects of transport duration and season on the plasma concentrations of IGFs and IGFBPs in adult pigs. For the study, exsanguination blood was collected from 240 market-weight barrows that were transported for 6, 12, or 18 h in January or July. IGF-I and -II were detected using commercial ELISAs whereas IGFBPs were quantified by quantitative Western ligand blotting. In addition, established markers of stress and metabolism were studied in the animals. The results show that plasma concentrations of IGFBP-3 were significantly reduced after 18 h of transport compared to shorter transport durations (6 and 12 h; p < 0.05). The concentrations of IGF-I in plasma were higher (p < 0.001) in pigs transported 12 h compared to shorter or longer durations. Season influenced plasma concentrations of IGFBP-3 and IGF-II (p < 0.05 and p < 0.01, respectively). Neither transport duration nor differential environmental conditions of winter or summer had an effect on glucocorticoids, albumin, triglycerides, or glucose concentrations (p > 0.05). However, low-density lipoprotein concentrations decreased after 18 h compared to 6 h of transport (p < 0.05), whereas high-density lipoprotein concentrations were higher (p < 0.05) in pigs transported for 12 or 18 h compared to those transported for only 6 h. Our findings indicate differential regulation of IGF-compounds in response to longer transport duration or seasonal changes and support current evidence of IGFs and IGFBPs as innovative animal-based indicators of psycho-social or metabolic stress in pigs.
Due to concentration of the slaughter process, more pigs are being transported to less and bigger slaughter plants resulting in increasingly longer transport distances and lengths (1). Longer transport distance or duration is associated with increased loss of live-weight (2), mortality (3), serum concentrations of acute phase proteins (4), creatine kinase (CK) (5, 6), glucose, lactate, and hematocrit levels (7, 8). The collection of exsanguination blood directly after the slaughtering of pigs is a suitable, non-invasive technique for the evaluation of the physiological response to different transport durations and preslaughter conditions. As described in preliminary studies (9), cortisol concentrations, measured in exsanguination blood, were not affected after prolonged duration of shipment. Though, insulin-like growth factor-binding protein (IGFBP)-2 concentrations decreased over time due to the length of shipment indicating biomarker potential for components of insulin-like growth factor (IGF) system. Furthermore, IGFBP-3/-2 ratio was increased in pigs, which were repeatedly stressed in the period of 24 h before slaughter. In humans, parameters of the growth hormone (GH) axis may have biomarker potential for acute or prolonged illness (10, 11). In mammals, it is assumed that acute physical stress, energy restriction, or acute phase of severe illness induce an amplification of GH secretion and increased levels of GH (10, 12, 13). GH affects body growth and metabolism directly and indirectly via control of IGF-I production in the liver or in other tissues (14). In blood, IGF is bound to IGFBPs that control the availability of IGF, but also have IGF-independent functions (15). As IGFBPs are sensitive markers to detect changes of the GH-dependent growth (16, 17), they play a central role in linking nutritional intake with somatic growth (18–20). Furthermore, it is known that glucocorticoids influence the levels of IGF-I and IGFBPs (21–23) suggesting an interference of acute or prolonged stress with the IGF system. Accordingly, in the present study, effects of transport duration under different seasonal conditions on the IGF-system were discussed in conjunction with established stress markers.
All experimental procedures were approved by the University of Saskatchewan’s Animal Research Ethics Board and adhered to the current guidelines of the Canadian Council on Animal Care (CCAC, 2009).
Details about the experimental conditions applied in this experiment were previously described by Goumon et al. (24). Briefly, this experiment was part of a larger study involving 5,040 crossbred pigs (Sus scrofa, Landrace × Large White; mean body weight = 120.8 ± 0.4 kg) transported over 6, 12, or 18 h (average loading density of 0.37–0.38 m2/pig) to a slaughterhouse located in western Canada. This study was conducted with subset of 240 male pigs (barrows, 120.8 ± 0.4 kg) at the age about 24 weeks and includes two trials. One trial was conducted in January to February with a temperature range between −28.8 and 1.9°C during the transport. The second trial was conducted in July and the temperature ranged between 12.5 and 40.1°C. Food was removed from pigs transported for 6 and 12 or 18 h for 20 and 24 h, respectively. As previously described, fasting did not affect the circulating levels of IGF-1 and IGFBPs within the time frame of this experiment (9). The pigs had no access to water on the truck, but water was available in lairage. After unloading, pigs were held in a lairage for approximately 150 min.
At exsanguination, 2 ml of blood were collected from a subsample of 240 barrows (40 barrows/transport duration/season) and EDTA-plasma was extracted (centrifugation at 1,400 × g at 4°C for 12 min) (24). Plasma samples were stored at −80°C before shipment to the laboratory of the Institute of Genome Biology at the Leibniz Institute for Farm Animal Biology (FBN) in Dummerstorf (Germany) for further analyses.
Quantitative Western ligand blotting was applied for the assessment of IGFBP-3, -2 and -5 concentrations as described previously (25). Briefly, plasma samples were denatured for 5 min in sample buffer (312.5 mM Tris (pH 6.8), 50% (w/v) glycerol, 5 mM EDTA, 1% (w/v) SDS, and 0.02% bromophenol blue). After separation by 12% SDS-PAGE, proteins were transferred to a polyvinylidene fluoride membrane (Millipore, Bedford, MA, USA), blocked and incubated with biotin labeled human IGF-II (1:500; BioIGF2-10; ibt-systems, Binzwangen, Germany). IGFBPs were detected by enhanced chemiluminescence using LuminataTM Forte (Millipore, Bedford, MA, USA). The signal intensities were corrected for background using the Gelanalyzer2010a software. On each blot, serial dilutions of recombinant human IGFBP-2 to -5 standards (R & D Systems, Wiesbaden, Germany) in artificial serum matrix (Biopanda, County Down, UK) were used as calibrators enabling signal quantification. Each signal was corrected for unspecific background Curve fitting was achieved by non-linear regression of each separate IGFBP. Due to low abundance, IGFBP-4 was detected but not quantified in the porcine plasma. The analytical range for each plasma IGFBP was 150–15,000 ng/ml. Inter-assay coefficients of variation (CV) were determined by in study validation according to recommendations of EMA guideline (26) using a random selected pig plasma sample of the investigated study samples. The inter-assay CVs (n = 10) for IGFBP-3 (mean: 4850 ng/ml), -2 (mean: 2324 ng/ml), and -5 (679 ng/ml) were 12.8, 15.1, and 20.1%, respectively.
Plasma concentrations of IGF-I and IGF-II (n = 90) were analyzed by Ligandis GbR using commercially available ELISA Kits E20 and E30 according to manufacturer’s instruction (Mediagnost, Reutlingen, Germany). For IGF-I, the analytical range was 21–1,050 ng/ml and the inter- and intra-assay CVs were less than 6.8 and 6.7%, respectively. For IGF-II, the analytical range was from 120 to 2,400 ng/ml.
Plasma concentrations of corticosterone (n = 90) were analyzed using LC-MS technique as already described (27). In brief, after protein precipitation, the supernatant was dried and stored at −20°C. For LC–MS/MS analysis, samples were dissolved in MeOH/H2O (50/50) adding DXM (100 ng/ml), vortexed for 30 s, sonicated for 2 min, and centrifuged at 4°C for 2 min at 14,000 rpm. Subsequently, samples were transferred to mass spectrometry analysis using an Accela HPLC/autosampler system (Thermo Fisher Scientific) coupled to the LTQ Orbitrap high-resolution hybrid mass spectrometer (Thermo Fisher Scientific, Dreieich, Germany). At various concentrations between 5 and 500 ng/ml, the intra-assay CVs were 13.05–4.64%. The inter-assay CV for 100 ng/ml (n = 20) was 5.57%.
Triglycerides (TG), cholesterol, glucose, high-density lipoprotein cholesterol (HDL-C), and albumin (n = 90) were analyzed in plasma using commercial enzymatic colored kits according to the manufacturer’s instructions (TG: No. LT-TR 1002, total cholesterol: No. LT-CH 0503, glucose: LT-GL 0251, HDL: LT-HD 0053, albumin LT-AB 0103; Labor & Technik Eberhard Lehmann Berlin, Germany, respectively) as previously described (28). The intra-assay CV for TG, total cholesterol, glucose, HDL and albumin were 1.60, 1.32, 1.80, 2.44, and 1.66%, respectively. The inter-assay CV% for TG, total cholesterol, glucose, HDL, and albumin were 4.30, 1.98, 2.40, 2.50, and 1.11%, respectively. Low-density lipoprotein cholesterol (LDL-C) was calculated according to the Friedewald Formula = [total cholesterol] − [HDL-C] − ([TG]/5) (29, 30).
Statistical analyses were performed using SAS software version 9.3 (SAS, Cary, NC, USA). The data of all blood parameters were evaluated by ANOVA using the MIXED procedure. The ANOVA model included transport duration (6, 12, and 18 h), environment (January/February versus July), week within environment (subset of weeks 1, 2, 3, and 4 in January/February, subset of weeks 1, 2, 3, and 4 in July) and the two-way interaction duration × environment as fixed effects. For all data, the least squares means and their SE were calculated and tested for each fixed effect in the model using the Tukey–Kramer procedure for all pair-wise multiple comparisons. Effects and differences were considered significant if p < 0.05.
An overview of all calculated F-values, degrees of freedom, and probability F-values of each fixed effect and their interaction is provided by Table 1. Quantitative Western ligand blot analyses of IGFBPs revealed IGFBP-3 as being the most abundant plasma IGFBP in pigs followed by IGFBP-2 and IGFBP-5 (Figures 1A–D) and having similar molecular weights as human recombinant reference standards. Plasma concentrations of IGFBP-3 and IGFBP-2 were lower in pigs transported for 18 h compared to pigs transported for 6 h (p < 0.001 and p < 0.001, respectively; Figures 1A,C). However, the concentrations of both IGFBP did not differ in pigs transported for 6 and 12 h (p > 0.05). There was no significant effect of transport duration on plasma concentrations of IGFBP-5 either (p > 0.05; Figure 1D). Concentrations of IGF-I were higher (p < 0.001) in pigs transported for 12 h than in those transported for 6 or 18 h (Figure 1E). By contrast, plasma concentrations of IGF-II were not significantly affected by transport duration (p > 0.05; Figure 1F). The total amount of quantified IGFBPs, which is an indicator for IGF-binding capacity, was lower in pigs transported for 18 h compared to pigs transported for 6 and 12 h (p < 0.001 and p < 0.05, respectively; Figure 2A). The ratio of IGF-I to total IGFBPs, calculated as an indicator for IGF-I bioavailability in circulation, was greater (p < 0.001) in pigs transported for 12 h compared to those transported for 6 h (Figure 2B). As shown in Figure 2C, the ratio of IGFBP-3 to -2, used as a marker for somatic growth and metabolic homeostasis, was greater in pigs transported for 18 h compared to pigs transported for 6 or 12 h (p < 0.001 and p < 0.05, respectively).
Table 1. F-test for the fixed effects season, week within season, duration, and the interaction of environment × duration.
Figure 1. Effect of transport duration on insulin-like growth factor (IGF)-axis: insulin-like growth factor-binding protein (IGFBP)-2 (A), with kind permission from Nature Publishing Group as previously published in Ref. (9), IGFBP-profile (B), IGFBP-3 (C), IGFBP-5 (D), IGF-I (E), and IGF-II (F). Quantitative data are presented as LS-Means + SE and include the summarized data of the trials in January/February and July due to the absence of the significant interaction duration × environment (*p < 0.05, **p < 0.01, ***p < 0.001, #p < 0.1, rh: recombinant human, quality control: pig plasma sample). IGFBP-2, -3, -5: n = 80 per duration; IGF-I and IGF-II: n = 30 per duration.
Figure 2. Effect of transport duration on total amount of total insulin-like growth factor-binding proteins [IGFBPs; (A)] as a marker for IGF-binding capacity and on the ratio of IGF-I/total IGFBPs (B) as indicator for IGF-I bioavailability. IGFBP-3/-2 ratio (C) was detected as a marker of growth hormone action. Data are presented as LS-Means + SE. Total (A,B): n = 30 per duration, (C) n = 80 per duration. *p < 0.05, ***p < 0.001, #p < 0.1.
Plasma concentrations of corticosterone, glucose, and albumin were not significantly affected by transport duration (p > 0.05; Figures 3A–C). Plasma concentrations of total cholesterol were lower (p < 0.01) in pigs transported for 18 h compared to those transported for 12 h (Figure 3D). When compared to 6 h transports, HDL-cholesterol levels were greater after 12 h (p < 0.01) and 18 h (p < 0.001) transports (Figure 3E). By contrast, LDL-cholesterol concentrations were lower in pigs transported for 18 h compared to pigs transported for 6 or 12 h (p < 0.05 and p < 0.01, respectively; Figure 3F). An effect of transport duration on plasma TG was only detected in summer. Thereby, the concentrations of TG were increased after 12 and 18 h transport compared to 6 h (p < 0.001 and p < 0.05, respectively, Figure 3G).
Figure 3. Effects of transport duration on plasma levels of corticosterone (A), glucose (B), albumin (C), cholesterol (D), HDL (E), LDL (F), and triglycerides (G) (n = 28–30 per duration for all parameters). Data except triglycerides include the summarized data of the trials in January/February and July due to the absence of the significant interaction duration × environment. A significant interaction of duration × environment (p < 0.05) was calculated for TG. All data are presented as LS-Means + SE *p < 0.05, **p < 0.01, ***p < 0.001; LDL = low-density lipoprotein, HDL = high-density lipoprotein.
The environmental conditions had an effect on some plasma parameters in this study, with concentrations of IGFBP-3 being higher (p < 0.05) in January/February than in July and IGF-II concentrations being lower (p < 0.01) in January/February than in July (Table 2). Plasma IGFBP-2, IGFBP-5, IGF-I, cortisol, corticosterone, albumin, and glucose concentrations were not affected by the differential environmental conditions in this study (p > 0.05).
Table 2. Effect of different environmental conditions in January/February and July on plasma parameters.
The results of this study clearly demonstrate that transport duration and differential environmental conditions in winter or summer contribute to the variation of IGFs and IGFBPs in blood collected from pigs at slaughter. Indeed, the concentrations of IGFBP-3 were lower after 18 h transport, contributing also to reduction of total IGFBPs levels in the circulation. Due to a stronger reduction of IGFBP-2, the ratio of IGFBP-3/IGFBP-2 increased after 18 h of transportation. An increase of IGFBP-3/IGFBP-2 ratio was also reported in plasma of pigs subjected to repeated restraint stress in the preslaughter period (9) indicating that this ratio might reflect increased stress conditions before slaughtering. In fact, in a previous study (24), 18 h of transportation were considered as a more stressful condition because the animals were characterized by higher body temperatures or greater drinking and differential resting behavior, compared to 6 or 12 h transports. In the present study, pigs were fasted either 20 h (6 and 12 h transport) or 24 h (18 h transport). Because it has been shown, that fasting of 70 h reduced serum levels of IGF-I and IGFBP-3 (31) in sheep, it might be that the IGF-compounds, at least in theory, were coregulated by metabolic stress in pigs. However, preliminary studies revealed that 19 h of fasting were not sufficient to affect IGFBP-2, -3, or -5 concentrations also in market-weight pigs (9). Furthermore, circadian effects as described for IGFBP-3 (9) are no explanation for the decrease of IGFBP-3 due to the same time of arrival of animals at the slaughterhouse. Therefore, together with the findings from preliminary studies, the reduction of plasma IGFBP-2 and IGFBP-3 levels in two independent conditions of physiological and psycho-social stress in pigs, such as restraint and long transport, provides consistent evidence for the potential of both IGFBPs as stress biomarker in pigs.
Interestingly, in the present study, IGF-I concentrations and IGF-I bioavailability were increased after 12-h transport followed by a decrease after 18 h to levels similar to those after 6 h. Since the amount of IGFBPs present in the circulation was not significantly different after 6 and 12 h of transport, it is difficult to conclude whether the IGFBPs are responsible for the increased IGF-I plasma concentrations after 12 h transport. Nevertheless, altered biosynthesis of IGF-I might be assessed by follow-up studies in hepatic and non-hepatic tissues. Without excluding a possibly reduced expression of IGF-I during 18 h transport, the reduced levels of IGFBPs may explain the reduction of IGF-I concentrations in pig plasma after long-term transport due to reduced half-life of free IGF-I.
In the present study, IGFBP-3 concentrations were higher in pigs transported in January/February, while IGF-II concentrations were higher in pigs transported in July. This might be an effect of environmental conditions such as different temperatures during the transport or husbandry. To our knowledge, there is little evidence regarding the effect of ambient conditions on the IGF-system in farm animals and, before this study, there was none for pigs. In fish, it has been shown that plasma and mRNA levels of IGF-I increase with ambient water temperature, while IGF-II concentrations decrease (32). In the Gabillard et al. (2003) study, ambient temperature variation appears to promote fish growth through IGF-I secretion by the liver following GH stimulation. However, this effect was biased by the fish nutritional condition. By contrast, plasma IGF-II was not affected by the growth-promoting effect of temperature, but appeared to be more related to the metabolic status of the fish. A positive correlation of circulating levels of IGF-1 with increased water temperatures was also described in other fish species (33–36). Differently from the findings in fish, no significant changes in IGF-I levels were found in this study. A possible explanation for this lack of effect may be that IGF-I action was modulated by the increased levels of IGFBP-3, which was by the way the most abundant IGFBP in pig serum in this study, at the conditions of lower temperatures. In addition, regarding the thermoregulation, most fish species are poikilothermic ectotherms. This means, the body temperature is not constant but varies with water temperature which may have direct effects on metabolic and endocrine parameters in contrast to endothermic mammals. Contrary to the results of the fish study (32), IGF-II level variation was not inversely proportional to that in ambient temperature in the present study, probably due to the potential negative effect of heat stress on the expression of growth-related genes (37). It has been also suggested that potential environmental effects on IGF-system in market pigs are strongly dependent on the farm management system. This hypothesis also needs to be assessed in future studies.
Longer transport duration and/or extreme ambient temperatures are known to decrease the welfare of pigs on the truck (3, 24, 38–40). However, the transport times either applied during differential conditions in winter or summer in this study did not result in any variation in the concentrations of corticosterone, glucose, and albumin in exsanguination blood. This is in accordance to previous findings that cortisol was affected by the transport duration (6, 9). The lack of variation in these blood metabolites at slaughter may indicate that lairage conditions and time applied in this study were sufficient to help pigs recover from transport stress, regardless of travel time. A number of studies (41–43) actually reported that during two or 3 h in lairage, levels of blood cortisol are normalizing.
Higher plasma total protein and albumin concentrations in blood of pigs at exsanguination are associated with the dehydration rate at the time of slaughter (44, 45). It is sensible that pigs are getting dehydrated during extended periods of shipment, which is in line with greater drinking behavior in pigs transported longer versus shorter periods of time (41, 46). However, in the present study, no effects of transport duration or environment were observed on blood albumin levels at slaughter. The increased drinking behavior observed in lairage in pigs after a transport period of 18 h in a previous study (24) may have helped circulating levels of albumin to return to the rest ones.
Differently from glucocorticoids and albumin, the indicators of lipid metabolism in the present study were affected by transport duration and/or environment. Plasma lipid levels may reflect constitutional or nutritional status of an animal, but there is also some evidence that plasma lipid concentrations can also be affected by short-term emotional arousal (47), acute stress (48), or activation of inflammatory pathways (49). In the present study, total cholesterol was reduced after 18 h of transport and this reduction was due to the simultaneous reduction of LDL-C concentrations. Conversely, HDL-C concentrations increased in pigs transported for 12 and 18 h. Transport longer than 6 h also increased blood TG levels, but only in summer. These results may be explained by the concurrent effects of longer feed deprivation (24 h) and transport (18 h) on increased lipolysis or even protein degradation for maintenance of metabolic homeostasis as showed by the reduced levels of cholesterol and LDL-C in this study, and the variation of body temperature and post-transport resting behavior reported in a companion study (24). However, as in 24 h fasted pigs, live-weight reductions are possibly related to excretion (50, 51) and considering that the preslaughter fasting intervals between transport groups were almost identical (i.e., 20 h fasting in the 6 and 12 h groups, and 24 h of fasting in the 18 h group), preslaughter lipid metabolism may have been affected by the length of transport rather than fasting time in this study. Warriss, in fact, considered the possibility that shipment might have a stronger effect on reductions of live-weight than fasting alone (52).
To summarize and conclude, in this study the IGF-system was regulated under conditions of different transport durations. With prolonged transport, plasma IGFBP-2 and IGFBP-3 levels were reduced, whereas IGF-I concentrations were dynamically regulated with increased concentrations after intermediate transport duration (12 h) compared to shorter or extended periods of time (6 or 18 h). By contrast, based on the results of this study, glucocorticoids, glucose, or albumin cannot be considered as useful indicators of transport stress in pigs. The metabolic stress condition of pigs being transported for 18 h was also indicated by the reduced levels of cholesterol and LDL-C and was associated with a decline of plasma IGFBPs concentrations. Further studies on the differential effects of psycho-social or metabolic stress on IGFs, IGFBPs, and complex traits of IGF-related signatures in pigs might identify specific biomarker potential for compounds from the IGF-system for animal welfare.
All experimental procedures were approved by the University of Saskatchewan’s Animal Research Ethics Board and adhered to the current guidelines of the Canadian Council on Animal Care (CCAC, 2009).
All authors designed the experiments and wrote the manuscript. SG, JB, and LF performed the animal experiment. EW, MK, CW, MS, and CH carried out wet lab experiments. EW, MK, CW, MS, AT, CH, and AH analyzed data.
CH and AH are related to Ligandis UG. The other authors do not have any potential conflicts of interest to declare.
The authors thank Luong Chau, Sabine Hinrichs, Patrick Hoeflich, Zianka Meyer, Fiona Lang, Sophie Horth, and Meghan Bouvier for excellent technical assistance. The publication of this article was funded by the Open Access Fund of the Leibniz Association and the Open Access Fund of the Leibniz Institute for Farm Animal Biology (FBN).
This study was supported by a grant from the Bundesministerium für Forschung und Technologie (BMBF, Innovationsforum Tierwohl; 01HI1402) and by The Natural Sciences and Engineering Research Council of Canada, Alberta Pork, SaskPork, Manitoba Pork, and Ontario Pork.
1. Marchant-Forde JN, Marchant-Forde RM. Welfare of pigs during transport and slaughter. In: Marchant-Forde JN, editor. The Welfare of Pigs. Dordrecht: Springer (2009). p. 301–30.
2. DeSilva PHGJ, Kalubowila A. Relationship of transport distance, sex on live weight loss of pigs during transit to slaughter house. Vet World (2012) 5(3):150–4. doi:10.5455/vetworld.2012.150-154
3. Vecerek V, Malena M, Malena M, Voslarova E, Chloupek P. The impact of the transport distance and season on losses of fattened pigs during transport to the slaughterhouse in the Czech Republic in the period from 1997 to 2004. Vet Med Praha (2006) 51(1):21.
4. Pineiro M, Pineiro C, Carpintero R, Morales J, Campbell FM, Eckersall PD, et al. Characterisation of the pig acute phase protein response to road transport. Vet J (2007) 173(3):669–74. doi:10.1016/j.tvjl.2006.02.006
5. Averos X, Herranz A, Sanchez R, Comella J, Gosalvez L. Serum stress parameters in pigs transported to slaughter under commercial conditions in different seasons. Vet Med Praha (2007) 52(8):333.
6. Sommavilla R, Faucitano L, Gonyou H, Seddon Y, Bergeron R, Widowski T, et al. Season, transport duration and trailer compartment effects on blood stress indicators in pigs: relationship to environmental, behavioral and other physiological factors, and pork quality traits. Animals (Basel) (2017) 7(2):8. doi:10.3390/ani7020008
7. Mota-Rojas D, Becerril M, Lemus C, Sanchez P, Gonzalez M, Olmos SA, et al. Effects of mid-summer transport duration on pre- and post-slaughter performance and pork quality in Mexico. Meat Sci (2006) 73(3):404–12. doi:10.1016/j.meatsci.2005.11.012
8. Becerril-Herrera M, Alonso-Spilsbury M, Ortega ME, Guerrero-Legarreta I, Ramirez-Necoechea R, Roldan-Santiago P, et al. Changes in blood constituents of swine transported for 8 or 16 h to an Abattoir. Meat Sci (2010) 86(4):945–8. doi:10.1016/j.meatsci.2010.07.021
9. Wirthgen E, Kunze M, Goumon S, Walz C, Hoflich C, Spitschak M, et al. Interference of stress with the somatotropic axis in pigs – lights on new biomarkers. Sci Rep (2017) 7(1):12055. doi:10.1038/s41598-017-11521-5
10. Møller N, Jørgensen JOL. Effects of growth hormone on glucose, lipid, and protein metabolism in human subjects. Endocr Rev (2009) 30(2):152–77. doi:10.1210/er.2008-0027
11. Mesotten D, Van den Berghe G. Changes within the GH/IGF-I/IGFBP axis in critical illness. Crit Care Clin (2006) 22(1):17–28,v. doi:10.1016/j.ccc.2005.09.002
12. Ranabir S, Reetu K. Stress and hormones. Ind J Endocrinol Metab (2011) 15(1):18. doi:10.4103/2230-8210.77573
13. Lawrence LJ, Fowler VR, Novakofski JE. Growth of Farm Animals. 3rd ed. Wallingford; Cambridge, MA: CABI (2012). XV, 352 p.
14. Jones JI, Clemmons DR. Insulin-like growth factors and their binding proteins: biological actions. Endocr Rev (1995) 16(1):3–34. doi:10.1210/edrv-16-1-3
15. Hwa V, Oh Y, Rosenfeld RG. The insulin-like growth factor-binding protein (IGFBP) superfamily 1. Endocr Rev (1999) 20(6):761–87. doi:10.1210/er.20.6.761
16. Bielohuby M, Zarkesh-Esfahani SH, Manolopoulou J, Wirthgen E, Walpurgis K, Toghiany Khorasgani M, et al. Validation of serum IGF-I as a biomarker to monitor the bioactivity of exogenous growth hormone agonists and antagonists in rabbits. Dis Model Mech (2014) 7(11):1263–73. doi:10.1242/dmm.016519
17. Krieger F, Elflein N, Saenger S, Wirthgen E, Rak K, Frantz S, et al. Polyethylene glycol-coupled IGF1 delays motor function defects in a mouse model of spinal muscular atrophy with respiratory distress type 1. Brain (2014) 137(5):1374–93. doi:10.1093/brain/awu059
18. McCusker R, Wangsness PJ, Griel L, Kavanaugh J. Effects of feeding, fasting and refeeding on growth hormone and insulin in obese pigs. Physiol Behav (1985) 35(3):383–8. doi:10.1016/0031-9384(85)90313-0
19. McCusker RH, Cohick WS, Busby WH, Clemmons DR. Evaluation of the developmental and nutritional changes in porcine insulin-like growth factor-binding protein-1 and -2 serum levels by immunoassay. Endocrinology (1991) 129(5):2631–8. doi:10.1210/endo-129-5-2631
20. Frystyk J, Delhanty P, Skjaerbaek C, Baxter R. Changes in the circulating IGF system during short-term fasting and refeeding in rats. Am J Physiol (1999) 277(2):E245–52. doi:10.1152/ajpendo.1999.277.2.E245
21. Unterman TG, Jentel JJ, Oehler DT, Lacson RG, Hofert JF. Effects of glucocorticoids on circulating levels and hepatic expression of insulin-like growth factor (IGF)-binding proteins and IGF-I in the adrenalectomized streptozotocin-diabetic rat. Endocrinology (1993) 133(6):2531–9. doi:10.1210/endo.133.6.7694841
22. Davis KB, Peterson BC. The effect of temperature, stress, and cortisol on plasma IGF-I and IGFBPs in sunshine bass. Gen Comp Endocrinol (2006) 149(3):219–25. doi:10.1016/j.ygcen.2006.05.009
23. Kajimura S, Hirano T, Visitacion N, Moriyama S, Aida K, Grau E. Dual mode of cortisol action on GH/IGF-I/IGF binding proteins in the tilapia, Oreochromis mossambicus. J Endocrinol (2003) 178(1):91–9. doi:10.1677/joe.0.1780091
24. Goumon S, Brown JA, Faucitano L, Bergeron R, Widowski TM, Crowe T, et al. Effects of transport duration on maintenance behavior, heart rate and gastrointestinal tract temperature of market-weight pigs in 2 seasons. J Anim Sci (2013) 91(10):4925–35. doi:10.2527/jas.2012-6081
25. Wirthgen E, HÖflich C, Spitschak M, Helmer C, Brand B, Langbein J, et al. Quantitative Western ligand blotting reveals common patterns and differential features of IGFBP-fingerprints in domestic ruminant breeds and species. Growth Horm IGF Res (2015) 26:42–9. doi:10.1016/j.ghir.2015.11.001
26. EMA. Guideline on bioanalytical method validation. Committee for Medicinal Products for Human Use (CHMP). London (2011).
27. Kunze M, Wirthgen E, Walz C, Spitschak M, Brenmoehl J, Vanselow J, et al. Bioanalytical validation for simultaneous quantification of non-aromatic steroids in follicular fluid from cattle via ESI-LC–MS/MS. J Chromatogr B Analyt Technol Biomed Life Sci (2015) 1007:132–9. doi:10.1016/j.jchromb.2015.10.010
28. Brenmoehl J, Walz C, Renne U, Ponsuksili S, Wolf C, Langhammer M, et al. Metabolic adaptations in the liver of born long-distance running mice. Med Sci Sports Exerc (2013) 45(5):841–50. doi:10.1249/MSS.0b013e31827e0fca
29. Friedewald WT, Levy RI, Fredrickson DS. Estimation of the concentration of low-density lipoprotein cholesterol in plasma, without use of the preparative ultracentrifuge. Clin Chem (1972) 18(6):499–502.
30. Tremblay AJ, Morrissette H, Gagne JM, Bergeron J, Gagne C, Couture P. Validation of the Friedewald formula for the determination of low-density lipoprotein cholesterol compared with beta-quantification in a large population. Clin Biochem (2004) 37(9):785–90. doi:10.1016/j.clinbiochem.2004.03.008
31. Ogawa E, Breier BH, Bauer MK, Gallaher BW, Grant PA, Walton PE, et al. Pretreatment with bovine growth hormone is as effective as treatment during metabolic stress to reduce catabolism in fasted lambs. Endocrinology (1996) 137(4):1242–8. doi:10.1210/endo.137.4.8625895
32. Gabillard JC, Weil C, Rescan PY, Navarro I, Gutierrez J, Le Bail PY. Effects of environmental temperature on IGF1, IGF2, and IGF type I receptor expression in rainbow trout (Oncorhynchus mykiss). Gen Comp Endocrinol (2003) 133(2):233–42. doi:10.1016/S0016-6480(03)00167-9
33. Beckman BR, Larsen DA, Moriyama S, Lee-Pawlak B, Dickhoff WW. Insulin-like growth factor-I and environmental modulation of growth during smoltification of spring chinook salmon (Oncorhynchus tshawytscha). Gen Comp Endocrinol (1998) 109(3):325–35. doi:10.1006/gcen.1997.7036
34. McCormick SD, Moriyama S, Björnsson BT. Low temperature limits photoperiod control of smolting in Atlantic salmon through endocrine mechanisms. Am J Physiol Regul Integr Comp Physiol (2000) 278(5):R1352–61. doi:10.1152/ajpregu.2000.278.5.R1352
35. Larsen DA, Beckman BR, Dickhoff WW. The effect of low temperature and fasting during the winter on metabolic stores and endocrine physiology (insulin, insulin-like growth factor-I, and thyroxine) of coho salmon, Oncorhynchus kisutch. Gen Comp Endocrinol (2001) 123(3):308–23. doi:10.1006/gcen.2001.7677
36. Silverstein JT, Wolters WR, Shimizu M, Dickhoff WW. Bovine growth hormone treatment of channel catfish: strain and temperature effects on growth, plasma IGF-I levels, feed intake and efficiency and body composition. Aquaculture (2000) 190(1):77–88. doi:10.1016/S0044-8486(00)00387-2
37. Del Vesco AP, Gasparino E, Zancanela V, Grieser DO, Guimaraes SE, Nascimento CS, et al. Acute heat stress and dietary methionine effects on IGF-I, GHR, and UCP mRNA expression in liver and muscle of quails. Genet Mol Res (2014) 13(3):7294–303. doi:10.4238/2014.February.13.12
38. Perremans S, Geers R. Effect of transport on some welfare characteristics of slaughter pigs. Vlaams Diergeneeskd Tijdschr (1996) 65(6):310–7.
39. Warriss P. Marketing losses caused by fasting and transport during the preslaughter handling of pigs. Pig News Inform (1985) 6:155–7.
40. Newsholme E, Blomstrand E, Ekblom B. Physical and mental fatigue: metabolic mechanisms and importance of plasma amino acids. Br Med Bull (1992) 48(3):477–95. doi:10.1093/oxfordjournals.bmb.a072558
41. Brown SN, Knowles TG, Edwards JE, Warriss PD. Relationship between food deprivation before transport and aggression in pigs held in lairage before slaughter. Vet Rec (1999) 145(22):630–4. doi:10.1136/vr.145.22.630
42. Perez MP, Palacio J, Santolaria MP, del Acena MC, Chacon G, Verde MT, et al. Influence of lairage time on some welfare and meat quality parameters in pigs. Vet Res (2002) 33(3):239–50. doi:10.1051/vetres:2002012
43. Warriss PD, Brown SN, Edwards JE, Anil MH, Fordham DP. Time in lairage needed by pigs to recover from the stress of transport. Vet Rec (1992) 131(9):194–6. doi:10.1136/vr.131.9.194
45. Sutherland MA, Backus BL, McGlone JJ. Effects of transport at weaning on the behavior, physiology and performance of pigs. Animals (2014) 4(4):657–69. doi:10.3390/ani4040657
46. Warriss PD, Dudley CP, Brown SN. Reduction of carcass yield in transported pigs. J Sci Food Agric (1983) 34(4):351–6. doi:10.1002/jsfa.2740340406
47. Dimsdale JE, Herd JA. Variability of plasma lipids in response to emotional arousal. Psychosom Med (1982) 44(5):413–30. doi:10.1097/00006842-198211000-00004
48. Stoney CM, West SG, Hughes JW, Lentino LM, Finney ML, Falko J, et al. Acute psychological stress reduces plasma triglyceride clearance. Psychophysiology (2002) 39(1):80–5. doi:10.1111/1469-8986.3910080
49. Preiser J-C. The Stress Response of Critical Illness: Metabolic and Hormonal Aspects. Cham: Springer (2016).
50. Beattie VE, Burrows MS, Moss BW, Weatherup RN. The effect of food deprivation prior to slaughter on performance, behaviour and meat quality. Meat Sci (2002) 62(4):413–8. doi:10.1016/S0309-1740(02)00031-1
51. Faucitano L, Chevillon P, Ellis M. Effects of feed withdrawal prior to slaughter and nutrition on stomach weight, and carcass and meat quality in pigs. Livest Sci (2010) 127:110–4. doi:10.1016/j.livsci.2009.10.002
Keywords: animal welfare, stress hormones, insulin-like growth factor, pig shipment, metabolism, biomarker
Citation: Wirthgen E, Goumon S, Kunze M, Walz C, Spitschak M, Tuchscherer A, Brown J, Höflich C, Faucitano L and Hoeflich A (2018) Effects of Transport Duration and Environmental Conditions in Winter or Summer on the Concentrations of Insulin-Like Growth Factors and Insulin-Like Growth Factor-Binding Proteins in the Plasma of Market-Weight Pigs. Front. Endocrinol. 9:36. doi: 10.3389/fendo.2018.00036
Received: 23 November 2017; Accepted: 29 January 2018;
Published: 13 February 2018
Edited by:
John Cockrem, Massey University, New ZealandReviewed by:
Tom Ole Nilsen, University of Bergen, NorwayCopyright: © 2018 Wirthgen, Goumon, Kunze, Walz, Spitschak, Tuchscherer, Brown, Höflich, Faucitano and Hoeflich. This is an open-access article distributed under the terms of the Creative Commons Attribution License (CC BY). The use, distribution or reproduction in other forums is permitted, provided the original author(s) and the copyright owner are credited and that the original publication in this journal is cited, in accordance with accepted academic practice. No use, distribution or reproduction is permitted which does not comply with these terms.
*Correspondence: Luigi Faucitano, bHVpZ2kuZmF1Y2l0YW5vQGFnci5nYy5jYQ==;
Andreas Hoeflich, aG9lZmxpY2hAZmJuLWR1bW1lcnN0b3JmLmRl
†These authors have contributed equally to this work.
Disclaimer: All claims expressed in this article are solely those of the authors and do not necessarily represent those of their affiliated organizations, or those of the publisher, the editors and the reviewers. Any product that may be evaluated in this article or claim that may be made by its manufacturer is not guaranteed or endorsed by the publisher.
Research integrity at Frontiers
Learn more about the work of our research integrity team to safeguard the quality of each article we publish.