Corrigendum: Insulin- like Growth Factor-Binding Protein Action in Bone Tissue: A Key Role for Pregnancy- Associated Plasma Protein-A
- 1Division of Oral Biology, Leeds School of Dentistry, Level 7 Wellcome Trust Brenner Building, University of Leeds, St James University Hospital, Leeds, United Kingdom
- 2Department of Molecular Biology and Genetics, Aarhus University, Aarhus, Denmark
- 3Department of Oral Biology, Dental College, King AbdulAziz University, Jeddah, Saudi Arabia
- 4Department of Oral Pathology, Faculty of Dentistry, Suez Canal University, Ismailia, Egypt
The insulin-like growth factor (IGF) axis is required for the differentiation, development, and maintenance of bone tissue. Accordingly, dysregulation of this axis is associated with various skeletal pathologies including growth abnormalities and compromised bone structure. It is becoming increasingly apparent that the action of the IGF axis must be viewed holistically taking into account not just the actions of the growth factors and receptors, but also the influence of soluble high affinity IGF binding proteins (IGFBPs).There is a recognition that IGFBPs exert IGF-dependent and IGF-independent effects in bone and other tissues and that an understanding of the mechanisms of action of IGFBPs and their regulation in the pericellular environment impact critically on tissue physiology. In this respect, a group of IGFBP proteinases (which may be considered as ancillary members of the IGF axis) play a crucial role in regulating IGFBP function. In this model, cleavage of IGFBPs by specific proteinases into fragments with lower affinity for growth factor(s) regulates the partition of IGFs between IGFBPs and cell surface IGF receptors. In this review, we examine the importance of IGFBP function in bone tissue with special emphasis on the role of pregnancy associated plasma protein-A (PAPP-A). We examine the function of PAPP-A primarily as an IGFBP-4 proteinase and present evidence that PAPP-A induced cleavage of IGFBP-4 is potentially a key regulatory step in bone metabolism. We also highlight some recent findings with regard to IGFBP-2 and IGFBP-5 (also PAPP-A substrates) function in bone tissue and briefly discuss the actions of the other three IGFBPs (-1, -3, and -6) in this tissue. Although our main focus will be in bone we will allude to IGFBP activity in other cells and tissues where appropriate.
Introduction
The insulin-like growth factor (IGF) axis comprises two polypeptide growth factors (IGF1 and IGF2), two cell surface receptors [IGF1 receptor (IGF1R) and IGF2R], and six soluble high-affinity IGF-binding proteins (IGFBP-1–6). Ancillary proteins associated with the IGF axis include various IGFBP proteinases that cleave IGFBPs into fragments with greatly reduced IGF-binding affinity, thus regulating the partition of IGFs between IGFBPs and cell surface receptors (1). IGFs are present at high concentrations in bone matrix (2), and disruption of the IGF1 gene compromises skeletal growth in mice (3) and humans (4). In addition to an anabolic role in mature bone, IGFs also stimulate differentiation of osteoblasts in developing bone tissue and regulate the balance between bone accretion and resorption which occur throughout life (5–7). IGFBP-4 is abundantly expressed in bone tissue (8), and the role of this IGFBP in regulating bone metabolism has been extensively investigated (9–11). In recent years, the activity of a specific IGFBP-4 proteinase, pregnancy-associated plasma protein-A (PAPP-A), has also been investigated in bone and other tissues (12–14). IGFBP-2 and IGFBP-5 are also significantly active in bone tissue demonstrating both IGF-dependent and IGF-independent effects (15–18). Additionally, signaling pathways associated with IGFBP-2 and IGFBP-5 action in osteoblasts and bone tissues have been recently reported (19–22). In this review, we touch on each of these topics and also briefly on the actions of the other IGFBPs (IGFBP-1, -3, and -6) in bone cells and tissues.
Insulin-Like Growth Factor-Binding Protein-4
Insulin-like growth factor-binding protein-4 was first identified as an inhibitory IGFBP in medium conditioned by the TE89 human osteosarcoma cell line (23) and then cloned from cDNA libraries of various tissues in human and rat (24, 25). It is a 237-residue protein sharing the 3-domain structure previously described for other IGFBPs. Early studies showed that IGFBP-4 inhibited IGF2-stimulated thymidine uptake in primary cultures of human osteoblasts (hOB) (26) and in the MC3T3-E1 mouse osteoblast cell line (27) and inhibited IGF1-stimulated aminoisobutyrate uptake in bovine fibroblasts and in the rat neuronal B104 cell line (28, 29). This inhibitory activity in vitro led to the hypothesis that IGFBP-4 generally displayed anti-anabolic and anti-proliferative effects. In confirmation of this, overexpression of IGFBP-4 in a malignant prostate epithelial cell line decreased the proliferative response to IGF1 and delayed tumor development when transfected cells were transplanted into nude mice (30). In vivo data also supported an IGF-inhibitory role for IGFBP-4. Tissue-specific overexpression of IGFBP-4 in smooth muscle cells using an α-actin promoter caused smooth muscle hypoplasia (31) and a similar strategy using a protease resistant form of IGFBP-4 (pr IGFBP-4) (see below) resulted in transgenic mice with decreased internal smooth muscle mass in stomach, bladder, and aorta (32). Importantly, with respect to this review, IGFBP-4 overexpression in osteoblasts decreased bone formation and compromised global skeletal growth (11). Some epidemiological data also supported an inhibitory role for IGFBP-4 with increased levels in a cohort of female patients with age-related osteoporotic fractures of the hip and spine (33). Although this evidence suggested an inhibitory role for IGFBP-4, other reports indicated an anabolic role for IGFBP-4. Therefore, systemic administration of IGFBP-4 to mice increased bone tissue markers (osteocalcin and alkaline phosphatase) in serum and skeletal tissues (10). Additionally, IGFBP-4 knockout (KO) mice exhibited prenatal growth retardation, suggesting that IGFBP-4 may be required for full growth promoting effects of IGF2 in the fetus (34). IGFBP-4 KO mice also showed gender dependent changes in skeletal phenotype with female mice having reduced bone mineral density (BMD) along with other features associated with osteopenia (9). Clearly, further research is required to definitively establish the role of IGFBP-4 in bone tissue physiology. In this respect, the observation of IGFBP-4 proteolysis by fibroblast and bone cell cultures has attracted much interest as a means of regulating the activity of IGFs in bone and other tissues, and we provide a short summary of this area in the following section.
IGFBP-4 Proteolysis
Addition of IGF1 to cultures of human fibroblasts reduced the levels of a 24 kDa IGFBP in conditioned medium and development of specific antibodies confirmed this species as IGFBP4 (35, 36). IGF1-dependent downregulation of IGFBP-4 occurred independently of IGF1R activation and was not associated with changes in IGFBP4 mRNA levels, suggesting a direct post-translational regulation of IGFBP-4. Shortly thereafter, IGF-induced decreases in IGFBP-4 protein levels were shown to be due to the presence of a proteolytic activity in fibroblast-conditioned medium which in cell-free assays was activated by IGF1 or IGF2 (37). IGFBP-4 was cleaved into two discrete fragments by this protease, suggesting a specific cleavage point within the protein (38). The cleavage site was identified at the peptide bond M135-K136 within the central domain of IGFBP-4 producing 14 and 18 kDa protein fragments (29). These data were used to engineer protease-resistant IGFBP-4 mutants that have proven useful in the further study of the biological significance of IGFBP-4 proteolysis (29, 39). This became apparent when intact, but not cleaved IGFBP-4, was shown to inhibit [3H] aminoisobutyric acid uptake into bovine fibroblasts with the inference that cleaved IGFBP-4 fragments did not bind IGF1. Further study indicated that IGF2 was a more potent activator of IGFBP-4 cleavage than IGF1 and IGF2 pre-treatment of human dermal fibroblast cultures increased sensitivity of cell cultures to IGF1. The concept of IGF2-mediated IGFBP4 cleavage as a route for increasing sensitivity to IGF1 (40) may be significant as IGF1 and IGF2 are usually present together in the pericellular environment, suggesting a complex interaction between the growth factors to regulate anabolic responses.
Primary cultures of hOB expressed an IGFBP-4 protease activity identical to that described for fibroblasts (41), and pre-treatment of osteoblast cultures with IGF2 also increased sensitivity to IGF1-stimulated [3H] thymidine incorporation (42). Subsequently, IGFBP-4 protease activity has been reported in human endometrial stromal cells (43) and in porcine aorta-derived smooth muscle cells (44), suggesting that proteolysis of IGFBP-4 may have widespread biological significance. At around this time, a landmark study identified PAPP-A as the enzyme responsible for IGF-dependent cleavage of IGFBP-4 in fibroblast-conditioned medium (34). PAPP-A was also shown to cleave IGFBP-5 in an IGF-independent manner (45). Identification of PAPP-A as the IGF-dependent IGFBP-4 proteinase caused a paradigm shift in this area of IGF research. Whereas previously IGFBP-4 had been viewed mainly as an IGF-inhibitory IGFBP in tissue culture studies, co-expression of PAPP-A in cell culture could negate this inhibitory effect. Furthermore, the “activation” of PAPP-A by IGFs suggested possible positive feedback loop whereby growth factor action could be enhanced. Further aspects of function, structure, and regulation of PAPP-A activity are discussed below.
Pregnancy-Associated Plasma Protein-A
Functional Aspects
Pregnancy-Associated Plasma Protein-A was partially purified from human fibroblast-conditioned medium by Lawrence et al (46), and its identity was confirmed by mass spectroscopy. By using polyclonal anti-PAPP-A antibodies, IGFBP-4 protease activity in fibroblast-conditioned medium could be completely inhibited, suggesting that PAPP-A may be the only IGFBP-4 protease expressed by these cells. PAPP-A isolated from fibroblast cultures was found to be identical to the enzyme described in pregnant serum (46–48), showing both IGF dependency and the same site of proteolytic cleavage in the central domain of IGFBP-4 (see above). Identification of PAPP-A allowed some elegant transgenic studies highlighting the importance of this enzyme. Transgenic mice with a collagen I promoter–PAPP-A construct overexpressed PAPP-A specifically in osteoid tissue causing increased calvarial BMD (14). In double transgenic mice overexpressing PAPP-A and a pr IGFBP-4, bone phenotype was similar to single pr IGFBP-4 transgenics, showing decreased calvarial thickness and BMD compared to WT mice. This provided strong evidence that in vivo anabolic effects of PAPP-A were due to IGFBP-4 proteolysis, most likely resulting in an increase in the local bioavailability of IGF (49). In confirmation of this, PAPP-A KO mice showed reduced femur BMD and blunted responses to the anabolic actions of parathyroid hormone (12). In a clinical context, PAPP-A has been proposed as a target for anti-proliferative therapies in various cancers. Studies in an ovarian cancer tissue model (50) and using xenografts of adenocarcinoma A549 cells (45) showed that antibody-mediated inhibition of PAPP-A activity decreased tumor growth presumably because pericellular IGF remains bound to IGFBPs leading to a reduction in free IGF in the local tumor environment. This may be important as current anti-IGF-based strategies have proved disappointing in clinical trials. Anti-IGF1R strategies are hampered by hyperinsulinemia secondary to elevated GH levels as a result of impaired IGF-1 feedback at the level of the pituitary (51). This may lead to increased mitogenic signaling by elevated insulin levels through the insulin receptor (IR). IGF1R blockade may also result in IGF1 signaling through the IR or through hybrid IGF1R/IR isoforms which are known to exist in many tissues (52) and which may not be blocked by anti-IGF1R-directed monoclonal antibodies. See the study by Yee (53) for an excellent review of the abovementioned arguments. In contrast, the use of anti-PAPP-A-directed antibodies would not be associated with these complications acting only to inhibit IGF1 release from pericellularly proteolysed IGFBP:IGF complexes.
Structural Aspects
Although PAPP-A was isolated over four decades ago from pregnancy serum (54), it was only after the cloning and expression of this large (1,547 residues) protein that detailed work on protein structure began (55). PAPP-A belongs to the metzincin superfamily of metalloendopeptidases containing a Zn-binding motif and a highly structurally conserved Met-turn (56). PAPP-A associates with the cell surface through two of five short consensus repeat modules within the C-terminus of the protein, and membrane-bound PAPP-A remains catalytically active. This may ensure release of IGF in the vicinity of cell surface IGF1R (57). Under reducing conditions, PAPP-A migrates as a 200 kDa protein although in pregnancy serum (and some other biological fluids) it is primarily present as a disulfide-bound dimer associated covalently with another disulfide bound dimer of the proform of eosinophil major basic protein (proMBP) in a 2:2 heterotetrameric complex (58, 59). The structure of the heterotetrameric PAPP-A:proMBP complex identifies a disulfide bridged dimer of PAPP-A covalently bound to a disulfide-bridged dimer of proMBP via two interchain disulfide bridges (60). In this configuration, PAPP-A is inactive with the proMBP dimer binding at or close to the active site of PAPP-A, suggesting that steric inhibition of enzyme activity may result. Both PAPP-A and proMBP are extensively glycosylated, and under non-denaturing gel electrophoresis conditions, the complex runs as a large (>500 kDa) molecular weight species. A mutagenic analysis of the substrate IGFBP-4 suggested that the C-terminal domain of IGFBP-4 conferred the IGF dependence for PAPP-A cleavage of IGFBP-4 (61). In addition, this same study showed that the region between the Zn-binding domain and the Met turn motif of PAPP-A was important for proteolytic activity toward the IGFBP-4:IGF1 complex. Availability of recombinant PAPP-A allowed confirmation that the rate of IGFBP-4 proteolysis is enhanced by binding of IGFs to IGFBP-4 (62), and detailed kinetic analysis confirmed IGF2 as a more potent activator of proteolysis than IGF1. The effect of IGFs on IGFBP4 proteolysis was associated with changes in both affinity (Km) and turnover rate (Kcat). This study also confirmed IGFBP-5 as a PAPP-A substrate although proteolysis of IGFBP5 was not IGF dependent (63). Further mutational analysis suggested that the Lin12-Notch repeat modules present in the C-terminal of PAPP-A are responsible for the differential requirement of IGFBP-4 and IGFBP-5 for IGF during PAPP-A-mediated proteolysis (64, 65).
Regulation of PAPP-A Activity
Relatively few agents have been shown to influence PAPP-A activity. IGFBP-4 proteolysis was inhibited following treatment of fibroblast cultures with phorbol esters. The attenuation of this effect by prior treatment with actinomycin D or cycloheximide suggested PCK-regulated expression of an inhibitor of IGFBP-4 proteolysis (66). Such an inhibitory activity was also reported in SV40-transformed hOB cells, suggesting that the process of cellular transformation may be associated with inhibition of IGFBP-4 proteolysis (67). The finding that phorbol esters and/or SV40-mediated transformation increased the expression of proMBP – a covalent inhibitor of PAPP-A (see above) – suggested at least one route by which these agents may act to inhibit IGFBP-4 cleavage in fibroblast cultures (68).
An early study reported stimulation of IGFBP-4 proteolytic activity in the rat neuronal B104 cell line by glucocorticoids (69) and following identification of PAPP-A as an IGFBP-4 proteinase, the synthetic glucocorticoid dexamethasone was shown to increase enzyme activity in primary cultures of rat vertebral osteoblasts (13). PAPP-A mRNA levels were not altered by dexamethasone treatment, suggesting a post-transcriptional mechanism by which enzyme activity was increased. In contrast to the above, TGFβ increased PAPP-A mRNA levels approximately 12-fold in hOB cultures, and this was associated with increased PAPP-A activity in conditioned medium (70). The demonstration of increased IGF2-mediated IGFBP-4 cleavage following TGFβ treatment of hOB cultures (71) may be of particular significance given the fact that IGF2 and TGFβ are two of the most abundant growth factors present in bone matrix, and a co-ordinated action of TGFβ and IGF2 in bone matrix to increase local availability of IGF may occur. Osteoblasts secrete IGF peptides endogenously (IGF2 > IGF1) and, despite the fact that the IGFBP-4 levels in osteoblast-conditioned media are typically an order of magnitude higher that the IGF2 levels, endogenous IGF2 can stimulate the proteolysis of concurrently expressed IGFBP-4 protein in osteoblast cultures (72, 73).
Recently, two novel protein inhibitors of PAPP-A activity have been described. These are members of the stanniocalcin family (STC1 and STC2) and were first identified as regulators of Ca homeostasis in teleost fish (74, 75). However, in the context of the mammalian IGF axis, their status as PAPP-A inhibitors indicates that these proteins are negative growth regulators. Overexpression of STC1 or STC2 resulted in growth retardation in transgenic mice (76, 77), whereas KO of STC2 causes increased growth (78). Molecular mechanisms of STC1 and STC2 inhibition of PAPP-A differ with STC2 forming a disulfide-bonded covalent complex with PAPP-A and STC1 forming a high-affinity non-covalent complex with the enzyme. Nonetheless, both STC1 and STC2 potently inhibit PAPP-A which may cause an increased concentration of IGF bound in complex with IGFBP-4 (and IGFBP-5, see below) and hence less bioavailable IGF in the pericellular environment. In agreement with this, STC2 inhibited PAPP-A-stimulated IGF1R phosphorylation in transfected cells exposed to IGF1:IGFBP-4 complexes (74). A recent study using whole exome sequencing of a large human cohort reported two separate single amino acid mutations of STC2 leading to compromised inhibition of PAPP-A. The fact that these alleles strongly associated with increased height in the sampled population is of particular interest (79). A diagrammatic representation of the IGFBP-PAPP-A-STC axis is presented in Figure 1.
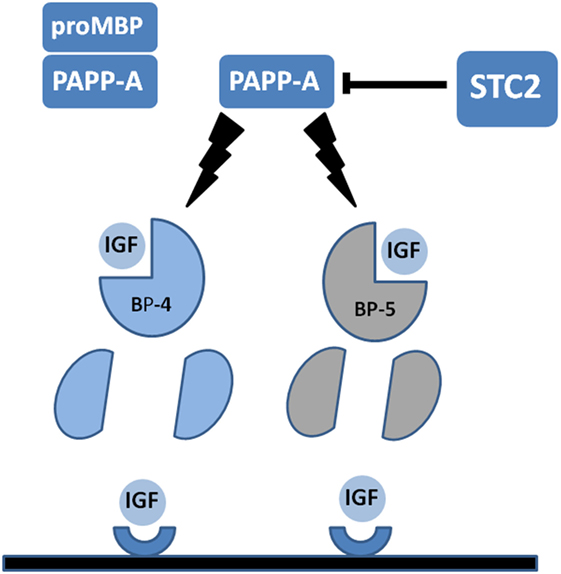
Figure 1. Diagrammatic representation of pregnancy-associated plasma protein-A (PAPP-A) activity in the insulin-like growth factor (IGF) axis. PAPP-A is present in serum and some other biological fluids covalently complexed with the pro-form of eosinophil major basic protein (proMBP). In this form PAPP-A is inactive. Uncomplexed PAPP-A acts to cleave IGF-binding protein-4 (IGFBP-4) and IGFBP-5 into fragments with reduced IGF-binding affinity. IGFBP-4 but not IGFBP-5 requires binding of IGF for PAPP-A cleavage. Proteolysis of IGFBP substrates releases IGFs to allow interaction with cognate cell surface receptors. Recently, discovered stanniocalcins (STC1 and STC2) act to inhibit PAPP-A activity. STC2 is shown, and this inhibitor forms a covalent bond with PAPP-A to inhibit the proteolytic activity. See the text for further details.
PAPP-A in Other Species
Pregnancy-associated plasma protein-A has also been cloned from a mouse cDNA library (80). Although murine (m) PAPP-A shares 91% homology with the human enzyme and cleaves IGFBP-4 in an IGF-dependent manner, mPAPP-A activity is not elevated in pregnant serum or in placenta. In addition, a variant mPAPP-A containing a 29-residue insert (PAPP-Ai) was also isolated. Interestingly, this PAPP-A isoform was a less efficient IGFBP-4 protease than the shorter variant of the enzyme. The significance of these differences between murine and human PAPP-A remains to be resolved, although PAPP-A null mice are 40% smaller than littermates, suggesting a role for PAPP-A during embryogenesis (81). This may be due to diminished IGFBP-4 cleavage and, therefore, reduced IGF availability in the developing fetus. In agreement with this, IGFBP-4 cleavage is absent in fibroblast cultures derived from these null mice. PAPP-A is present in multiple other species, including zebrafish, and interestingly, the absence of PAPP-A in this species causes a developmental delay, which is independent of proteolytic activity (82).
Pregnancy-Associated Plasma Protein-A2
Overgaard et al. described the cloning of a metalloprotease from placental cDNA libraries with homology to PAPP-A. This protein, which cleaves IGFBP-5 (and IGFBP-3), was named PAPP-A2 (83) and is present in human pregnancy serum where it releases IGF1 from the IGF1:IGFBP-5 complexes (84). PAPP-A2 appears as a monomer of 200 kDa in non-reducing gel electrophoresis and in contrast to PAPP-A does not bind to proMBP or associate with cell surfaces. Cleavage of IGFBP-5 by PAPP-A2 is not IGF-dependent, but as IGFBP-5 has been reported to have both IGF-dependent and IGF-independent effects in hOB cultures (see below), PAPP-A2 activity may also have major relevance in bone cell physiology. In agreement with this, homozygous PAPP-A2 KO mice show decreased post-natal growth along with reduced body length (85). Similarly, conditional PAPP-A2 KO in osteoblasts decreased body mass and bone length, although other tissue sources of PAPP-A2 may be involved in appropriate post-natal growth (86). PAPP-A2 may represent a quantitative trait locus regulating body shape in mice (87, 88). Recently, two separate families (of Palestinian and Spanish ancestry) were found to have two different inactivating PAPP-A2 mutations that result in growth retardation in homozygous children (89, 90). Further analysis of affected individuals indicated significant increases in IGF1 in ternary ALS complexes with reduced free serum IGF1. In addition, affected individuals showed moderate microcephaly, mild BMD effects, and thin long bones. This phenotype was presumably associated with the inability of mutant PAPP-A2 to proteolyse IGFBP-3 and IGFBP-5 substrates.
Other IGFBs in Bone Metabolism
Insulin-Like Growth Factor-Binding Protein-5
Insulin-like growth factor-binding protein-5 is also present at high concentrations in bone matrix and has been associated with both inhibitory and stimulatory activities in bone cells and tissues. IGFBP-5 was reported to have IGF-dependent and IGF-independent effects in bone tissue, although the literature is conflicted in this area. IGFBP-5 was shown to enhance IGF-stimulated mitogenesis in hOB cultures (91, 92) and to stimulate the differentiation of two osteoblast cell lines in an IGF-independent fashion (93, 94). In ovariectomized rats, daily subcutaneous injection of IGFBP-5 increased osteoblast proliferation (95) and enhanced the association of IGF1 with bone cells possibly via specific cell-surface binding sites for IGFBP-5 (26, 96) or through a specific IGFBP-5 receptor on osteoblast membranes (97, 98). Disappointingly, however, a specific IGFBP-5 receptor has not been isolated or characterized further. Signaling studies suggest that the actions of IGFBP-5 in osteoblasts involve Ras association family isoform C activation of Erk-1/2 (19). The association of IGFBP-5 with four and a half LIM domain protein within the nucleus of U2 osteosarcoma cells has also been reported although the functional significance of this observation remains unknown (99). Although all the abovementioned findings are consistent with a stimulatory role for IGFBP-5 action in bone tissue (IGF-dependent or independent), some authors have reported contrary findings. For example, IGFBP-5 was reported to inhibit IGF1-stimulated proliferation in the U2 human osteosarcoma cell line (100), and transgenic mice overexpressing IGFBP-5 from the osteocalcin promoter showed decreased trabecular bone formation and reduced rates of mineral deposition during the first few weeks of post-natal life (15). Stromal cells isolated from transgenic animals also showed decreased levels of osteogenic markers. Constitutive overexpression of IGFBP-5 in the mouse osteoblast precursor cell line MC3T3-E1 also decreased osteogenic marker expression and delayed formation of mineralized nodules under osteogenic culture conditions (16). Finally, addition of exogenous wtIGFBP-5 or overexpression of IGFBP-5 from an adenovirus promoter inhibited osteoblast differentiation and growth of mouse metatarsal bones in short-term culture (20).
Although IGFBP-5 is cleaved in an IGF-independent manner by PAPP-A and PAPP-A2 (see above), it is also a substrate for other proteolytic enzymes. Matrix metalloproteinase-1 and -2 (MMP-1 and MMP-2) were shown to degrade IGFBP-5 in a time-dependent fashion in medium conditioned by the mouse MC-3T3-E1 cell line (101), and the complement component C1s was identified as an IGFBP5-specific protease in human dermal fibroblast-conditioned media (102). Following on from this, Mohan et al. described a disintegrin and metalloprotease-9 as an IGFBP-5 protease expressed in the U2 human osteosarcoma cell line (103). Although the importance of IGFBP-5 cleavage may (as for IGFBP4) lie with the regulation of free pericellular IGF concentrations, this is somewhat complicated by the observations of IGF-independent actions of IGFBP-5 described earlier. Clearly, these may also be impacted by IGFBP-5 cleavage. Further work is required to establish the role of IGFBP-5 in osteoblast differentiation and in bone tissue metabolism in general.
Finally, there are reports of broad-spectrum proteolytic enzyme families, which degrade IGFBP-5 (and other IGFBPs). These include plasmin (104), thrombin (105), the serine proteases cathepsin G, and elastase (106), although questions of specificity and biological relevance related to these proteases remain largely unanswered.
Insulin-Like Growth Factor-Binding Protein-2
The literature describes both IGF-dependent and IGF-independent effects of IGFBP-2 in osteoblast cultures and bone tissues. An early study using a unilateral disuse osteoporosis model in the rat showed that osmotic minipump delivery of IGF2/IGFBP-2 complexes prevented the decrease in BMD in affected femurs associated with this model (107). A subsequent report from the same group showed that IGF2/IGFBP-2 complexes bound to heparin-Sepharose and that it was suggested that such complexes may associate with ECM components in bone tissue potentially increasing the local concentration of IGFs (108). In agreement with the abovementioned findings, IGFBP-2 potentiated IGF2-induced increases in ALP activity in cultures of rat tibial osteoblasts (109), and we have demonstrated the same effect of IGFBP-2 on IGF1-stimulated ALP activity in differentiating human dental pulp cells (110). Studies in IGFBP-2 KO mice indicated gender-specific differences in osteogenic phenotype with increased cortical thickness and periosteal circumference in female mice but reduced cortical bone area and trabecular volume in male KOs (111). Although difficult to rationalize, it clearly suggests interplay between the IGF axis and other hormone systems. This same group also reported impaired osteoclastogenesis in bone marrow cells derived from igfbp2−/− mice and a transfection study in these cells and indicated that both the IGF and heparin-binding domain (HBD) of IGFBP-2 were required for osteoclast generation (112). This description of IGF-independent effects of IGFBP-2 in vitro was confirmed in concurrent studies demonstrating restoration of osteogenic phenotype in igfbp2−/− bone marrow cells by addition of a HBD peptide derived from IGFBP-2. In addition, in vivo administration of HBD peptide restored osteoblast number in igfbp2−/− mice (17). Recently, studies in the mouse MC-3T3 pre-osteoblast cell line showed that IGFBP-2 can bind and inhibit the activity of receptor phosphotyrosine phosphatase β causing increased levels of phosphorylated PTEN, activation of Akt, and stimulation of osteogenesis (17, 18). Further reports from this laboratory highlight the importance of the scaffold/adaptor protein IRS-1, PKCζ, and early activation of AMP-dependent protein kinase in the osteoblast differentiation of primary rat calvarial cells and the differentiating MC-3T3 cell line (21, 22). It should be noted that IGFBP-2 is also a PAPP-A substrate, although this IGFBP is cleaved less efficiently than IGFBP-4 and IGFBP-5 (113).
IGFBP-1, -3, and -6
Although IGFBP-1, -3, and -6 have all been reported to be expressed in osteoblasts and to be present in bone tissue (114), there are fewer data describing the functions of these three IGFBPs. IGFBP-1 was expressed at low levels in primary hOB cultures under regulation of glucocorticoid and insulin although the physiological relevance of this effect in bone tissue has not been established (115). A recent prospective study (10-year follow-up) in a cohort of elderly women reported a positive correlation between serum IGFBP-1 and osteoporotic fracture, suggesting an IGF-independent osteopenic effect of IGFBP-1 (116). Further data are required on IGFBP-1 and its effects (if any) on bone physiology.
A very early study reported inhibition of IGF1-stimulated DNA synthesis in two osteoblast cell lines by intact IGFBP-3 (117). This inhibitory effect on both IGF1- and IGF2-stimulated DNA synthesis was confirmed in cultures of rat calvarial cells (118). Although these data suggest an inhibitory role for IGFBP-3 in bone metabolism, other in vivo data (119) and cross-sectional studies in a cohort of female patients with postmenopausal osteoporosis suggest an anabolic role for IGFBP-3 in maintaining bone density (120).
Insulin-like growth factor-binding protein--6 mRNA was expressed in primary osteoblast cultures derived from fetal rat calvaria (121), and the expression of both mRNA and protein was upregulated in a dose-dependent fashion by cortisol or retinoic acid treatment (122, 123) cultures. Conversely, IGFBP-6 expression was negatively regulated by TGFβ1 in the same cell culture system (124). IGFBP-6 shows a higher affinity for IGF2 than IGF1. Accordingly, it was shown to be a more potent inhibitor of IGF2-stimulated DNA and glycogen synthesis in hOB cells than IGF1 (125). This inhibitory effect of IGFBP-6 was confirmed in the SaoS2 human osteosarcoma cell line using a stable antisense transfection strategy to demonstrate that the anti-differentiative activity of all-trans retinoic acid (Vitamin D) was at least partly mediated via IGFBP-6 (126). More recently, IGFBP-6 has been shown to interact with the thyroid hormone receptor alpha1 and to inhibit the tri-iodothyronine-induced increase in osteoblast marker expression in the human U2-OS osteosarcoma cell line (127). In contrast to these reports, the inhibitory effect of IGFBP-6 attenuated by intracellular interaction with the LIM mineralizing protein in both human and mouse osteoblastic cells (128), and one study reported a stimulatory effect of IGFBP-6 on DNA synthesis and mitogenesis in the human osteosarcoma Saos-2/B-10 cell line (129). As for IGFBP-1 and IGFBP-3, the role of IGFBP-6 in osteogenesis and bone tissue physiology has been underreported, and further studies are required to elucidate the role of these 3 IGFBPs in osteogenesis and bone physiology.
Conclusion
Six decades have passed since the initial description of the anabolic role of IGF1 in skeletal tissue (130). In the intervening years, much progress has been made in defining the actions of IGF1 and IGF2 and other members of the IGF axis in bone tissue at all stages of development. This review has focused specifically on the function of IGFBPs in osteogenic tissues – both IGF-dependent and IGF-independent. However, the IGF axis acts in a co-ordinated fashion and is integrated with other hormonal systems and growth factor axes to regulate skeletal tissue development and maintenance. It is anticipated that in this and other aspects of IGF axis physiology, many important observations will be made in the near future.
Author Contributions
All the authors contributed to the writing and editing of the manuscript.
Conflict of Interest Statement
The authors declare that the research was conducted in the absence of any commercial or financial relationships that could be construed as a potential conflict of interest.
Funding
HA-K acknowledges the Higher Education Committee for Education and Development (HCED), Office of Prime Minister, Iraq for financial support. AA and HA acknowledge the Royal Embassy of Saudi Arabia – Cultural Bureau (UK) for financial support. RE-G acknowledges WELMEC, a Centre of Excellence in Medical Engineering funded by the Wellcome Trust and EPSRC, under grant number WT 088908/Z/09/Z for financial support.
Abbreviations
IGF, insulin-like growth factor; IGFBP, IGF-binding proteins; IGF1R, IGF1 receptor; hDPC, human dental pulp cells; PAPP-A, pregnancy-associated plasma protein-A; STC, stanniocalcin; BMD, bone mineral density; hOB, human osteoblasts.
References
1. Clemmons DR. Role of IGF binding proteins in regulating metabolism. Trends Endocrinol Metab (2016) 27(6):375–91. doi:10.1016/j.tem.2016.03.019
2. Xian L, Wu X, Pang L, Lou M, Rosen CJ, Qiu T, et al. Matrix IGF-1 maintains bone mass by activation of mTOR in mesenchymal stem cells. Nat Med (2012) 18(7):1095–101. doi:10.1038/nm.2793
3. Liu JP, Baker J, Perkins AS, Robertson EJ, Efstratiadis A. Mice carrying null mutations of the genes encoding Insulin-like Growth Factor I (Igf-1) and type 1 IGF receptor (Igf1r). Cell (1993) 75(1):59–72. doi:10.1016/S0092-8674(05)80084-4
4. Woods KA, Camacho-Hübner C, Savage MO, Clark AJ. Intrauterine growth retardation and postnatal growth failure associated with deletion of the insulin-like growth factor I gene. N Engl J Med. (1996) 335(18):1363–7. doi:10.1056/NEJM199610313351805
5. Baylink DJ, Finkelman RD, Mohan S. Growth factors to stimulate bone formation. J Bone Miner Res (1993) 8(Suppl 2):S565–72. doi:10.1002/jbmr.5650081326
6. Mohan S, Baylink DJ. Insulin-like growth factor system components and the coupling of bone formation to resorption. Horm Res (1996) 45(Suppl 1):59–62. doi:10.1159/000184833
7. Ohlsson C, Bengtsson BA, Isaksson OG, Andreassen TT, Slootweg MC. Growth hormone and bone. Endocr Rev (1998) 19(1):55–79. doi:10.1210/er.19.1.55
8. Malpe R, Baylink DJ, Linkhart TA, Wergedal JE, Mohan S. Insulin-like Growth Factor (IGF)-I, -II, IGF Binding Proteins (IGFBP)-3, -4, and -5 levels in the conditioned media of normal human bone cells are skeletal site-dependent. J Bone Miner Res (1997) 12(3):423–30. doi:10.1359/jbmr.1997.12.3.423
9. Maridas DE, DeMambro VE, Le PT, Nagano K, Baron R, Mohan S, et al. IGFBP-4 regulates adult skeletal growth in a sex-specific manner. J Endocrinol (2017) 233(1):131–44. doi:10.1530/JOE-16-0673
10. Miyakoshi N, Qin X, Kasukawa Y, Richman C, Srivastava AK, Baylink DJ, et al. Systemic administration of insulin-like growth factor (IGF)-binding protein-4 (IGFBP-4) increases bone formation parameters in mice by increasing IGF bioavailability via an IGFBP-4 protease-dependent mechanism. Endocrinology (2001) 142(6):2641–8. doi:10.1210/endo.142.6.8192
11. Zhang M, Faugere MC, Malluche H, Rosen CJ, Chernausek SD, Clemens TL. Paracrine overexpression of IGFBP-4 in osteoblasts of transgenic mice decreases bone turnover and causes global growth retardation. J Bone Miner Res (2003) 18(5):836–43. doi:10.1359/jbmr.2003.18.5.836
12. Clifton KB, Conover CA. Pregnancy-associated plasma protein-A modulates the anabolic effects of parathyroid hormone in mouse bone. Bone (2015) 81:413–6. doi:10.1016/j.bone.2015.08.015
13. Jia D, Heersche JN. Pregnancy-associated plasma protein-A proteolytic activity in rat vertebral cell cultures: stimulation by dexamethasone – a potential mechanism for glucocorticoid regulation of osteoprogenitor proliferation and differentiation. J Cell Physiol (2005) 204(3):848–58. doi:10.1002/jcp.20344
14. Qin X, Wergedal JE, Rehage M, Tran K, Newton J, Lam P, et al. Pregnancy-associated plasma protein-A increases osteoblast proliferation in vitro and bone formation in vivo. Endocrinology (2006) 147(12):5653–61. doi:10.1210/en.2006-1055
15. Devlin RD, Du Z, Buccilli V, Jorgetti V, Canalis E. Transgenic mice overexpressing insulin-like growth factor binding protein-5 display transiently decreased osteoblastic function and osteopenia. Endocrinology (2002) 143(10):3955–62. doi:10.1210/en.2002-220129
16. Durant D, Pereira RM, Canalis E. Overexpression of insulin-like growth factor binding protein-5 decreases osteoblastic function in vitro. Bone (2004) 35(6):1256–62. doi:10.1016/j.bone.2004.08.011
17. Kawai M, Breggia AC, DeMambro VE, Shen X, Canalis E, Bouxsein ML, et al. The heparin-binding domain of IGFBP-2 has insulin-like growth factor binding-independent biologic activity in the growing skeleton. J Biol Chem (2011) 286(16):14670–80. doi:10.1074/jbc.M110.193334
18. Xi G, Wai C, DeMambro V, Rosen CJ, Clemmons DR. IGFBP-2 directly stimulates osteoblast differentiation. J Bone Miner Res (2014) 29(11):2427–38. doi:10.1002/jbmr.2282
19. Amaar YG, Baylink DJ, Mohan S. Ras-association domain family 1 protein, RASSF1C, is an IGFBP-5 binding partner and a potential regulator of osteoblast cell proliferation. J Bone Miner Res (2005) 20(8):1430–9. doi:10.1359/JBMR.050311
20. Mukherjee A, Rotwein P. Insulin-like growth factor-binding protein-5 inhibits osteoblast differentiation and skeletal growth by blocking insulin-like growth factor actions. Mol Endocrinol (2008) 22(5):1238–50. doi:10.1210/me.2008-0001
21. Xi G, Rosen CJ, Clemmons DR. IGF-I and IGFBP-2 stimulate AMPK activation and autophagy, which are required for osteoblast differentiation. Endocrinology (2016) 157(1):268–81. doi:10.1210/en.2015-1690
22. Xi G, Shen X, Rosen CJ, Clemmons DR. IRS-1 functions as a molecular scaffold to coordinate IGF-I/IGFBP-2 signaling during osteoblast differentiation. J Bone Miner Res (2016) 31(6):1300–14. doi:10.1002/jbmr.2791
23. Mohan S, Bautista CM, Wergedal J, Baylink DJ. Isolation of an inhibitory insulin-like growth factor (IGF) binding protein from bone cell-conditioned medium: a potential local regulator of IGF action. Proc Natl Acad Sci U S A (1989) 86(21):8338–42. doi:10.1073/pnas.86.21.8338
24. LaTour D, Mohan S, Linkhart TA, Baylink DJ, Strong DD. Inhibitory insulin-like growth factor-binding protein: cloning, complete sequence, and physiological regulation. Mol Endocrinol (1990) 4(12):1806–14. doi:10.1210/mend-4-12-1806
25. Shimasaki S, Uchiyama F, Shimonaka M, Ling N, et al. Molecular cloning of the cDNAs encoding a novel insulin-like growth factor-binding protein from rat and human. Mol Endocrinol (1990) 4(10):1451–8. doi:10.1210/mend-4-10-1451
26. Mohan S, Nakao Y, Honda Y, Landale E, Leser U, Dony C, et al. Studies on the mechanisms by which insulin-like growth factor (IGF) binding protein-4 (IGFBP-4) and IGFBP-5 modulate IGF actions in bone cells. J Biol Chem (1995) 270(35):20424–31. doi:10.1074/jbc.270.35.20424
27. Scharla SH, Strong DD, Rosen C, Mohan S, Holick M, Baylink DJ, et al. 1,25-Dihydroxyvitamin D3 increases secretion of insulin-like growth factor binding protein-4 (IGFBP-4) by human osteoblast-like cells in vitro and elevates IGFBP-4 serum levels in vivo. J Clin Endocrinol Metab (1993) 77(5):1190–7. doi:10.1210/jcem.77.5.7521341
28. Cheung PT, Smith EP, Shimasaki S, Ling N, Chernausek SD. Characterization of an insulin-like growth factor binding protein (IGFBP-4) produced by the B104 rat neuronal cell line: chemical and biological properties and differential synthesis by sublines. Endocrinology (1991) 129(2):1006–15. doi:10.1210/endo-129-2-1006
29. Conover CA, Durham SK, Zapf J, Masiarz FR, Kiefer MC. Cleavage analysis of Insulin-like Growth Factor (IGF)-dependent IGF-binding protein-4 proteolysis and expression of protease-resistant IGF-binding protein-4 mutants. J Biol Chem (1995) 270(9):4395–400. doi:10.1074/jbc.270.9.4395
30. Damon SE, Maddison L, Ware JL, Plymate SR. Overexpression of an inhibitory insulin-like growth factor binding protein (IGFBP), IGFBP-4, delays onset of prostate tumor formation. Endocrinology (1998) 139(8):3456–64. doi:10.1210/endo.139.8.6150
31. Wang J, Niu W, Witte DP, Chernausek SD, Nikiforov YE, Clemens TL, et al. Overexpression of insulin-like growth factor-binding protein-4 (IGFBP-4) in smooth muscle cells of transgenic mice through a smooth muscle alpha-actin-IGFBP-4 fusion gene induces smooth muscle hypoplasia. Endocrinology (1998) 139(5):2605–14. doi:10.1210/endo.139.5.5986
32. Zhang M, Smith EP, Kuroda H, Banach W, Chernausek SD, Fagin JA. Targeted expression of a protease-resistant IGFBP-4 mutant in smooth muscle of transgenic mice results in IGFBP-4 stabilization and smooth muscle hypotrophy. J Biol Chem (2002) 277(24):21285–90. doi:10.1074/jbc.M112082200
33. Rosen C, Donahue LR, Hunter S, Holick M, Kavookjian H, Kirschenbaum A, et al. The 24/25-kDa serum insulin-like growth factor-binding protein is increased in elderly women with hip and spine fractures. J Clin Endocrinol Metab (1992) 74(1):24–7. doi:10.1210/jcem.74.1.1370164
34. Ning Y, Schuller AG, Conover CA, Pintar JE. Insulin-like Growth Factor (IGF) binding protein-4 is both a positive and negative regulator of IGF activity in vivo. Mol Endocrinol (2008) 22(5):1213–25. doi:10.1210/me.2007-0536
35. Conover CA. A unique receptor-independent mechanism by which insulin-like growth factor I regulates the availability of insulin-like growth factor binding proteins in normal and transformed human fibroblasts. J Clin Invest (1991) 88(4):1354–61. doi:10.1172/JCI115441
36. Neely EK, Rosenfeld RG. Insulin-like growth factors (IGFs) reduce IGF-binding protein-4 (IGFBP-4) concentration and stimulate IGFBP-3 independently of IGF receptors in human fibroblasts and epidermal cells. Endocrinology (1992) 130(2):985–93. doi:10.1210/en.130.2.985
37. Fowlkes J, Freemark M. Evidence for a novel Insulin-like Growth Factor (IGF)-dependent protease regulating IGF-binding protein-4 in dermal fibroblasts. Endocrinology (1992) 131(5):2071–6. doi:10.1210/endo.131.5.1385096
38. Conover CA, Kiefer MC, Zapf J. Posttranslational regulation of insulin-like growth factor binding protein-4 in normal and transformed human fibroblasts. Insulin-like growth factor dependence and biological studies. J Clin Invest (1993) 91(3):1129–37. doi:10.1172/JCI116272
39. Laursen LS, Overgaard MT, Nielsen CG, Boldt HB, Hopmann KH, Conover CA, et al. Substrate specificity of the metalloproteinase pregnancy-associated plasma protein-A (PAPP-A) assessed by mutagenesis and analysis of synthetic peptides: substrate residues distant from the scissile bond are critical for proteolysis. Biochem J (2002) 367(Pt 1):31–40. doi:10.1042/bj20020831
40. Conover CA, Clarkson JT, Bale LK. Insulin-like growth factor-II enhancement of human fibroblast growth via a nonreceptor-mediated mechanism. Endocrinology (1994) 135(1):76–82. doi:10.1210/endo.135.1.8013394
41. Kanzaki S, Hilliker S, Baylink DJ, Mohan S. Evidence that human bone cells in culture produce insulin-like growth factor-binding protein-4 and -5 proteases. Endocrinology (1994) 134(1):383–92. doi:10.1210/endo.134.1.7506211
42. Durham SK, Kiefer MC, Riggs BL, Conover CA. Regulation of insulin-like growth factor binding protein 4 by a specific insulin-like growth factor binding protein 4 proteinase in normal human osteoblast-like cells: implications in bone cell physiology. J Bone Miner Res (1994) 9(1):111–7. doi:10.1002/jbmr.5650090115
43. Irwin JC, Dsupin BA, Giudice LC. Regulation of insulin-like growth factor-binding protein-4 in human endometrial stromal cell cultures: evidence for ligand-induced proteolysis. J Clin Endocrinol Metab (1995) 80(2):619–26. doi:10.1210/jc.80.2.619
44. Parker A, Gockerman A, Busby WH, Clemmons DR. Properties of an insulin-like growth factor-binding protein-4 protease that is secreted by smooth muscle cells. Endocrinology (1995) 136(6):2470–6. doi:10.1210/endo.136.6.7538463
45. Mikkelsen JH, Resch ZT, Kalra B, Savjani G, Kumar A, Conover CA, et al. Indirect targeting of IGF receptor signaling in vivo by substrate-selective inhibition of PAPP-A proteolytic activity. Oncotarget (2014) 5(4):1014–25. doi:10.18632/oncotarget.1629
46. Lawrence JB, Oxvig C, Overgaard MT, Sottrup-Jensen L, Gleich GJ, Hays LG, et al. The insulin-like growth factor (IGF)-dependent IGF binding protein-4 protease secreted by human fibroblasts is pregnancy-associated plasma protein-A. Proc Natl Acad Sci U S A (1999) 96(6):3149–53. doi:10.1073/pnas.96.6.3149
47. Byun D, Mohan S, Yoo M, Sexton C, Baylink DJ, Qin X. Pregnancy-associated plasma protein-A accounts for the insulin-like growth factor (IGF)-binding protein-4 (IGFBP-4) proteolytic activity in human pregnancy serum and enhances the mitogenic activity of IGF by degrading IGFBP-4 in vitro. J Clin Endocrinol Metab (2001) 86(2):847–54. doi:10.1210/jc.86.2.847
48. Qin X, Byun D, Lau KH, Baylink DJ, Mohan S. Evidence that the interaction between insulin-like growth factor (IGF)-II and IGF binding protein (IGFBP)-4 is essential for the action of the IGF-II-dependent IGFBP-4 protease. Arch Biochem Biophys (2000) 379(2):209–16. doi:10.1006/abbi.2000.1872
49. Phang D, Rehage M, Bonafede B, Hou D, Xing W, Mohan S, et al. Inactivation of insulin-like-growth factors diminished the anabolic effects of pregnancy-associated plasma protein-A (PAPP-A) on bone in mice. Growth Horm IGF Res (2010) 20(3):192–200. doi:10.1016/j.ghir.2010.01.001
50. Becker MA, Haluska P Jr, Bale LK, Oxvig C, Conover CA. A novel neutralizing antibody targeting pregnancy-associated plasma protein-a inhibits ovarian cancer growth and ascites accumulation in patient mouse tumorgrafts. Mol Cancer Ther (2015) 14(4):973–81. doi:10.1158/1535-7163.MCT-14-0880
51. Atzori F, Tabernero J, Cervantes A, Prudkin L, Andreu J, Rodríguez-Braun E, et al. A phase I pharmacokinetic and pharmacodynamic study of dalotuzumab (MK-0646), an anti-insulin-like growth factor-1 receptor monoclonal antibody, in patients with advanced solid tumors. Clin Cancer Res (2011) 17(19):6304–12. doi:10.1158/1078-0432.CCR-10-3336
52. Bailyes EM, Navé BT, Soos MA, Orr SR, Hayward AC, Siddle K. Insulin receptor/IGF-I receptor hybrids are widely distributed in mammalian tissues: quantification of individual receptor species by selective immunoprecipitation and immunoblotting. Biochem J (1997) 327(Pt 1):209–15. doi:10.1042/bj3270209
53. Yee D. Insulin-like growth factor receptor inhibitors: baby or the bathwater? J Natl Cancer Inst (2012) 104(13):975–81. doi:10.1093/jnci/djs258
54. Lin TM, Galbert SP, Kiefer D, Spellacy WN, Gall S. Characterization of four human pregnancy-associated plasma proteins. Am J Obstet Gynecol (1974) 118(2):223–36. doi:10.1016/0002-9378(74)90553-5
55. Kristensen T, Oxvig C, Sand O, Møller NP, Sottrup-Jensen L. Amino acid sequence of human pregnancy-associated plasma protein-A derived from cloned cDNA. Biochemistry (1994) 33(6):1592–8. doi:10.1021/bi00172a040
56. Boldt HB, Overgaard MT, Laursen LS, Weyer K, Sottrup-Jensen L, Oxvig C. Mutational analysis of the proteolytic domain of pregnancy-associated plasma protein-A (PAPP-A): classification as a metzincin. Biochem J (2001) 358(Pt 2):359–67. doi:10.1042/bj3580359
57. Laursen LS, Overgaard MT, Weyer K, Boldt HB, Ebbesen P, Christiansen M, et al. Cell surface targeting of pregnancy-associated plasma protein A proteolytic activity. Reversible adhesion is mediated by two neighboring short consensus repeats. J Biol Chem (2002) 277(49):47225–34. doi:10.1074/jbc.M209155200
58. Oxvig C, Sand O, Kristensen T, Gleich GJ, Sottrup-Jensen L. Circulating human pregnancy-associated plasma protein-A is disulfide-bridged to the proform of eosinophil major basic protein. J Biol Chem (1993) 268(17):12243–6.
59. Oxvig C, Sand O, Kristensen T, Kristensen L, Sottrup-Jensen L. Isolation and characterization of circulating complex between human pregnancy-associated plasma protein-A and proform of eosinophil major basic protein. Biochim Biophys Acta (1994) 1201(3):415–23. doi:10.1016/0304-4165(94)90071-X
60. Overgaard MT, Sorensen ES, Stachowiak D, Boldt HB, Kristensen L, Sottrup-Jensen L, et al. Complex of pregnancy-associated plasma protein-A and the proform of eosinophil major basic protein. Disulfide structure and carbohydrate attachment. J Biol Chem (2003) 278(4):2106–17. doi:10.1074/jbc.M208777200
61. Gaidamauskas E, Gyrup C, Boldt HB, Schack VR, Overgaard MT, Laursen LS, et al. IGF dependent modulation of IGF binding protein (IGFBP) proteolysis by pregnancy-associated plasma protein-A (PAPP-A): multiple PAPP-A-IGFBP interaction sites. Biochim Biophys Acta (2013) 1830(3):2701–9. doi:10.1016/j.bbagen.2012.11.002
62. Laursen LS, Overgaard MT, Søe R, Boldt HB, Sottrup-Jensen L, Giudice LC, et al. Pregnancy-associated plasma protein-A (PAPP-A) cleaves insulin-like growth factor binding protein (IGFBP)-5 independent of IGF: implications for the mechanism of IGFBP-4 proteolysis by PAPP-A. FEBS Lett (2001) 504(1–2):36–40. doi:10.1016/S0014-5793(01)02760-0
63. Gyrup C, Oxvig C. Quantitative analysis of insulin-like growth factor-modulated proteolysis of insulin-like growth factor binding protein-4 and -5 by pregnancy-associated plasma protein-A. Biochemistry (2007) 46(7):1972–80. doi:10.1021/bi062229i
64. Boldt HB, Kjaer-Sorensen K, Overgaard MT, Weyer K, Poulsen CB, Sottrup-Jensen L, et al. The Lin12-notch repeats of pregnancy-associated plasma protein-A bind calcium and determine its proteolytic specificity. J Biol Chem (2004) 279(37):38525–31. doi:10.1074/jbc.M405222200
65. Weyer K, Boldt HB, Poulsen CB, Kjaer-Sorensen K, Gyrup C, Oxvig C. A substrate specificity-determining unit of three Lin12-Notch repeat modules is formed in trans within the pappalysin-1 dimer and requires a sequence stretch C-terminal to the third module. J Biol Chem (2007) 282(15):10988–99. doi:10.1074/jbc.M607903200
66. Conover CA, Clarkson JT, Bale LK. Phorbol ester tumor promoters regulate insulin-like growth factor-binding protein-4 proteolysis. Endocrinology (1993) 133(3):1347–51. doi:10.1210/endo.133.3.7689953
67. Durham SK, Riggs BL, Harris SA, Conover CA. Alterations in insulin-like growth factor (IGF)-dependent IGF-binding protein-4 proteolysis in transformed osteoblastic cells. Endocrinology (1995) 136(4):1374–80. doi:10.1210/endo.136.4.7534697
68. Chen BK, Overgaard MT, Bale LK, Resch ZT, Christiansen M, Oxvig C, et al. Molecular regulation of the IGF-binding protein-4 protease system in human fibroblasts: identification of a novel inducible inhibitor. Endocrinology (2002) 143(4):1199–205. doi:10.1210/endo.143.4.8729
69. Cheung PT, Wu J, Banach W, Chernausek SD. Glucocorticoid regulation of an insulin-like growth factor-binding protein-4 protease produced by a rat neuronal cell line. Endocrinology (1994) 135(4):1328–35. doi:10.1210/endo.135.4.7523095
70. Ortiz CO, Chen BK, Bale LK, Overgaard MT, Oxvig C, Conover CA. Transforming growth factor-beta regulation of the insulin-like growth factor binding protein-4 protease system in cultured human osteoblasts. J Bone Miner Res (2003) 18(6):1066–72. doi:10.1359/jbmr.2003.18.6.1066
71. Durham SK, Riggs BL, Conover CA. The insulin-like growth factor-binding protein-4 (IGFBP-4)-IGFBP-4 protease system in normal human osteoblast-like cells: regulation by transforming growth factor-beta. J Clin Endocrinol Metab (1994) 79(6):1752–8. doi:10.1210/jc.79.6.1752
72. Durham SK, De León DD, Okazaki R, Riggs BL, Conover CA. Regulation of insulin-like growth factor (IGF)-binding protein-4 availability in normal human osteoblast-like cells: role of endogenous IGFs. J Clin Endocrinol Metab (1995) 80(1):104–10. doi:10.1210/jc.80.1.104
73. Qin X, Byun D, Strong DD, Baylink DJ, Mohan S. Studies on the role of human insulin-like growth factor-II (IGF-II)-dependent IGF binding protein (hIGFBP)-4 protease in human osteoblasts using protease-resistant IGFBP-4 analogs. J Bone Miner Res (1999) 14(12):2079–88. doi:10.1359/jbmr.1999.14.12.2079
74. Jepsen MR, Kløverpris S, Mikkelsen JH, Pedersen JH, Füchtbauer EM, Laursen LS, et al. Stanniocalcin-2 inhibits mammalian growth by proteolytic inhibition of the insulin-like growth factor axis. J Biol Chem (2015) 290(6):3430–9. doi:10.1074/jbc.M114.611665
75. Kløverpris S, Mikkelsen JH, Pedersen JH, Jepsen MR, Laursen LS, Petersen SV, et al. Stanniocalcin-1 potently inhibits the proteolytic activity of the metalloproteinase pregnancy-associated plasma protein-A. J Biol Chem (2015) 290(36):21915–24. doi:10.1074/jbc.M115.650143
76. Gagliardi AD, Kuo EY, Raulic S, Wagner GF, DiMattia GE. Human stanniocalcin-2 exhibits potent growth-suppressive properties in transgenic mice independently of growth hormone and IGFs. Am J Physiol Endocrinol Metab (2005) 288(1):E92–105. doi:10.1152/ajpendo.00268.2004
77. Varghese R, Gagliardi AD, Bialek PE, Yee SP, Wagner GF, Dimattia GE. Overexpression of human stanniocalcin affects growth and reproduction in transgenic mice. Endocrinology (2002) 143(3):868–76. doi:10.1210/endo.143.3.8671
78. Chang AC, Hook J, Lemckert FA, McDonald MM, Nguyen MA, Hardeman EC, et al. The murine stanniocalcin 2 gene is a negative regulator of postnatal growth. Endocrinology (2008) 149(5):2403–10. doi:10.1210/en.2007-1219
79. Marouli E, Graff M, Medina-Gomez C, Lo KS, Wood AR, Kjaer TR, et al. Rare and low-frequency coding variants alter human adult height. Nature (2017) 542(7640):186–90. doi:10.1038/nature21039
80. Søe R, Overgaard MT, Thomsen AR, Laursen LS, Olsen IM, Sottrup-Jensen L, et al. Expression of recombinant murine pregnancy-associated plasma protein-A (PAPP-A) and a novel variant (PAPP-Ai) with differential proteolytic activity. Eur J Biochem (2002) 269(8):2247–56. doi:10.1046/j.1432-1033.2002.02883.x
81. Conover CA, Bale LK, Overgaard MT, Johnstone EW, Laursen UH, Füchtbauer EM, et al. Metalloproteinase pregnancy-associated plasma protein A is a critical growth regulatory factor during fetal development. Development (2004) 131(5):1187–94. doi:10.1242/dev.00997
82. Kjaer-Sorensen K, Engholm DH, Kamei H, Morch MG, Kristensen AO, Zhou J, et al. Pregnancy-associated plasma protein A (PAPP-A) modulates the early developmental rate in zebrafish independently of its proteolytic activity. J Biol Chem (2013) 288(14):9982–92. doi:10.1074/jbc.M112.426304
83. Overgaard MT, Boldt HB, Laursen LS, Sottrup-Jensen L, Conover CA, Oxvig C. Pregnancy-associated plasma protein-A2 (PAPP-A2), a novel insulin-like growth factor-binding protein-5 proteinase. J Biol Chem (2001) 276(24):21849–53. doi:10.1074/jbc.M102191200
84. Yan X, Baxter RC, Firth SM. Involvement of pregnancy-associated plasma protein-A2 in insulin-like growth factor (IGF) binding protein-5 proteolysis during pregnancy: a potential mechanism for increasing IGF bioavailability. J Clin Endocrinol Metab (2010) 95(3):1412–20. doi:10.1210/jc.2009-2277
85. Amiri N, Christians JK. PAPP-A2 expression by osteoblasts is required for normal postnatal growth in mice. Growth Horm IGF Res (2015) 25(6):274–80. doi:10.1016/j.ghir.2015.09.003
86. Amiri F, Venema VJ, Wang X, Ju H, Venema RC, Marrero MB. Hyperglycemia enhances angiotensin II-induced Janus-activated kinase/STAT signaling in vascular smooth muscle cells. J Biol Chem (1999) 274(45):32382–6. doi:10.1074/jbc.274.45.32382
87. Christians JK, de Zwaan DR, Fung SH. Pregnancy associated plasma protein A2 (PAPP-A2) affects bone size and shape and contributes to natural variation in postnatal growth in mice. PLoS One (2013) 8(2):e56260. doi:10.1371/journal.pone.0056260
88. Christians JK, Hoeflich A, Keightley PD. PAPPA2, an enzyme that cleaves an insulin-like growth-factor-binding protein, is a candidate gene for a quantitative trait locus affecting body size in mice. Genetics (2006) 173(3):1547–53. doi:10.1534/genetics.106.057513
89. Argente J, Chowen JA, Pérez-Jurado LA, Frystyk J, Oxvig C. One level up: abnormal proteolytic regulation of IGF activity plays a role in human pathophysiology. EMBO Mol Med (2017) 9(10):1338–45. doi:10.15252/emmm.201707950
90. Dauber A, Muñoz-Calvo MT, Barrios V, Domené HM, Kloverpris S, Serra-Juhé C, et al. Mutations in pregnancy-associated plasma protein A2 cause short stature due to low IGF-I availability. EMBO Mol Med (2016) 8(4):363–74. doi:10.15252/emmm.201506106
91. Andress DL. Comparison studies of IGFBP-5 binding to osteoblasts and osteoblast-derived extracellular matrix. Prog Growth Factor Res (1995) 6(2–4):337–44. doi:10.1016/0955-2235(95)00008-9
92. Andress DL, Birnbaum RS. Human osteoblast-derived insulin-like growth factor (IGF) binding protein-5 stimulates osteoblast mitogenesis and potentiates IGF action. J Biol Chem (1992) 267(31):22467–72.
93. Chihara K, Sugimoto T. The action of GH/IGF-I/IGFBP in osteoblasts and osteoclasts. Horm Res (1997) 48(Suppl 5):45–9. doi:10.1159/000191328
94. Nasu M, Sugimoto T, Kaji H, Chihara K. Estrogen modulates osteoblast proliferation and function regulated by parathyroid hormone in osteoblastic SaOS-2 cells: role of insulin-like growth factor (IGF)-I and IGF-binding protein-5. J Endocrinol (2000) 167(2):305–13. doi:10.1677/joe.0.1670305
95. Andress DL. IGF-binding protein-5 stimulates osteoblast activity and bone accretion in ovariectomized mice. Am J Physiol Endocrinol Metab (2001) 281(2):E283–8. doi:10.1152/ajpendo.2001.281.2.E283
96. Schmid C, Schläpfer I, Gosteli-Peter MA, Froesch ER, Zapf J. Expression, effects, and fate of IGFBP-5 are different in normal and malignant osteoblastic cells. Prog Growth Factor Res (1995) 6(2–4):167–73. doi:10.1016/0955-2235(95)00037-2
97. Andress DL. Heparin modulates the binding of insulin-like growth factor (IGF) binding protein-5 to a membrane protein in osteoblastic cells. J Biol Chem (1995) 270(47):28289–96.
98. Andress DL. Insulin-like growth factor-binding protein-5 (IGFBP-5) stimulates phosphorylation of the IGFBP-5 receptor. Am J Physiol (1998) 274(4 Pt 1):E744–50.
99. Amaar YG, Thompson GR, Linkhart TA, Chen ST, Baylink DJ, Mohan S. Insulin-like growth factor-binding protein 5 (IGFBP-5) interacts with a four and a half lim protein 2 (FHL2). J Biol Chem (2002) 277(14):12053–60. doi:10.1074/jbc.M110872200
100. Conover CA, Kiefer MC. Regulation and biological effect of endogenous insulin-like growth factor binding protein-5 in human osteoblastic cells. J Clin Endocrinol Metab (1993) 76(5):1153–9. doi:10.1210/jcem.76.5.7684391
101. Thrailkill KM, Quarles LD, Nagase H, Suzuki K, Serra DM, Fowlkes JL. Characterization of insulin-like growth factor-binding protein 5-degrading proteases produced throughout murine osteoblast differentiation. Endocrinology (1995) 136(8):3527–33. doi:10.1210/endo.136.8.7543045
102. Busby WH Jr, Nam TJ, Moralez A, Smith C, Jennings M, Clemmons DR. The complement component C1s is the protease that accounts for cleavage of insulin-like growth factor-binding protein-5 in fibroblast medium. J Biol Chem (2000) 275(48):37638–44. doi:10.1074/jbc.M006107200
103. Mohan S, Thompson GR, Amaar YG, Hathaway G, Tschesche H, Baylink DJ. ADAM-9 is an insulin-like growth factor binding protein-5 protease produced and secreted by human osteoblasts. Biochemistry (2002) 41(51):15394–403. doi:10.1021/bi026458q
104. Campbell PG, Andress DL. Insulin-like growth factor (IGF)-binding protein-5-(201-218) region regulates hydroxyapatite and IGF-I binding. Am J Physiol (1997) 273(5 Pt 1):E1005–13.
105. Zheng B, Clarke JB, Busby WH, Duan C, Clemmons DR. Insulin-like growth factor-binding protein-5 is cleaved by physiological concentrations of thrombin. Endocrinology (1998) 139(4):1708–14. doi:10.1210/endo.139.4.5945
106. Gibson TL, Cohen P. Inflammation-related neutrophil proteases, cathepsin G and elastase, function as insulin-like growth factor binding protein proteases. Growth Horm IGF Res (1999) 9(4):241–53. doi:10.1054/ghir.1999.0115
107. Conover CA, Johnstone EW, Turner RT, Evans GL, John Ballard FJ, Doran PM, et al. Subcutaneous administration of insulin-like growth factor (IGF)-II/IGF binding protein-2 complex stimulates bone formation and prevents loss of bone mineral density in a rat model of disuse osteoporosis. Growth Horm IGF Res (2002) 12(3):178–83. doi:10.1016/S1096-6374(02)00044-8
108. Conover CA, Khosla S. Role of extracellular matrix in insulin-like growth factor (IGF) binding protein-2 regulation of IGF-II action in normal human osteoblasts. Growth Horm IGF Res (2003) 13(6):328–35. doi:10.1016/S1096-6374(03)00092-3
109. Palermo C, Manduca P, Gazzerro E, Foppiani L, Segat D, Barreca A. Potentiating role of IGFBP-2 on IGF-II-stimulated alkaline phosphatase activity in differentiating osteoblasts. Am J Physiol Endocrinol Metab (2004) 286(4):E648–57. doi:10.1152/ajpendo.00049.2003
110. Alkharobi H, Alhodhodi A, Hawsawi Y, Alkafaji H, Devine D, El-Gendy R, et al. IGFBP-2 and -3 co-ordinately regulate IGF1 induced matrix mineralisation of differentiating human dental pulp cells. Stem Cell Res (2016) 17(3):517–22. doi:10.1016/j.scr.2016.09.026
111. DeMambro VE, Clemmons DR, Horton LG, Bouxsein ML, Wood TL, Beamer WG, et al. Gender-specific changes in bone turnover and skeletal architecture in igfbp-2-null mice. Endocrinology (2008) 149(5):2051–61. doi:10.1210/en.2007-1068
112. DeMambro VE, Maile L, Wai C, Kawai M, Cascella T, Rosen CJ, et al. Insulin-like growth factor-binding protein-2 is required for osteoclast differentiation. J Bone Miner Res (2012) 27(2):390–400. doi:10.1002/jbmr.545
113. Monget P, Mazerbourg S, Delpuech T, Maurel MC, Manière S, Zapf J, et al. Pregnancy-associated plasma protein-A is involved in insulin-like growth factor binding protein-2 (IGFBP-2) proteolytic degradation in bovine and porcine preovulatory follicles: identification of cleavage site and characterization of IGFBP-2 degradation. Biol Reprod (2003) 68(1):77–86. doi:10.1095/biolreprod.102.007609
114. Mohan S, Baylink DJ. IGF-binding proteins are multifunctional and act via IGF-dependent and -independent mechanisms. J Endocrinol (2002) 175(1):19–31. doi:10.1677/joe.0.1750019
115. Conover CA, Lee PD, Riggs BL, Powell DR. Insulin-like growth factor-binding protein-1 expression in cultured human bone cells: regulation by insulin and glucocorticoid. Endocrinology (1996) 137(8):3295–301. doi:10.1210/endo.137.8.8754754
116. Salminen H, Sääf M, Ringertz H, Strender LE. The role of IGF-I and IGFBP-1 status and secondary hyperparathyroidism in relation to osteoporosis in elderly Swedish women. Osteoporos Int (2008) 19(2):201–9. doi:10.1007/s00198-007-0463-4
117. Schmid C, Rutishauser J, Schläpfer I, Froesch ER, Zapf J. Intact but not truncated insulin-like growth factor binding protein-3 (IGFBP-3) blocks IGF I-induced stimulation of osteoblasts: control of IGF signalling to bone cells by IGFBP-3-specific proteolysis? Biochem Biophys Res Commun (1991) 179(1):579–85. doi:10.1016/0006-291X(91)91410-E
118. Schmid C, Schläpfer I, Keller A, Waldvogel M, Froesch ER, Zapf J. Effects of insulin-like growth factor (IGF) binding proteins (BPs) -3 and -6 on DNA synthesis of rat osteoblasts: further evidence for a role of auto-/paracrine IGF I but not IGF II in stimulating osteoblast growth. Biochem Biophys Res Commun (1995) 212(1):242–8. doi:10.1006/bbrc.1995.1962
119. Tanaka H, Moriwake T, Matsuoka Y, Nakamura T, Seino Y. Potential role of rhIGF-I/IGFBP-3 in maintaining skeletal mass in space. Bone (1998) 22(5 Suppl):145S–7S. doi:10.1016/S8756-3282(98)00006-4
120. Ueland T, Brixen K, Mosekilde L, Mosekilde L, Flyvbjerg A, Bollerslev J. Age-related changes in cortical bone content of insulin-like growth factor binding protein (IGFBP)-3, IGFBP-5, osteoprotegerin, and calcium in postmenopausal osteoporosis: a cross-sectional study. J Clin Endocrinol Metab (2003) 88(3):1014–8. doi:10.1210/jc.2002-020977
121. McCarthy TL, Casinghino S, Centrella M, Canalis E. Complex pattern of insulin-like growth factor binding protein expression in primary rat osteoblast enriched cultures: regulation by prostaglandin E2, growth hormone, and the insulin-like growth factors. J Cell Physiol (1994) 160(1):163–75. doi:10.1002/jcp.1041600119
122. Gabbitas B, Canalis E. Retinoic acid stimulates the transcription of insulin-like growth factor binding protein-6 in skeletal cells. J Cell Physiol (1996) 169(1):15–22. doi:10.1002/(SICI)1097-4652(199610)169:1<15::AID-JCP2>3.0.CO;2-H
123. Gabbitas B, Canalis E. Cortisol enhances the transcription of insulin-like growth factor-binding protein-6 in cultured osteoblasts. Endocrinology (1996) 137(5):1687–92. doi:10.1210/endo.137.5.8612502
124. Gabbitas B, Canalis E. Growth factor regulation of insulin-like growth factor binding protein-6 expression in osteoblasts. J Cell Biochem (1997) 66(1):77–86. doi:10.1002/(SICI)1097-4644(19970701)66:1<77::AID-JCB9>3.0.CO;2-V
125. Kiefer MC, Schmid C, Waldvogel M, Schläpfer I, Futo E, Masiarz FR, et al. Recombinant human insulin-like growth factor binding proteins 4, 5, and 6: biological and physiochemical characterization. Growth Regul (1993) 3(1):56–9.
126. Yan T, Wergedal J, Zhou Y, Mohan S, Baylink DJ, Strong DD. Inhibition of human osteoblast marker gene expression by retinoids is mediated in part by insulin-like growth factor binding protein-6. Growth Horm IGF Res (2001) 11(6):368–77. doi:10.1054/ghir.2001.0249
127. Qiu J, Ma XL, Wang X, Chen H, Huang BR. Insulin-like growth factor binding protein-6 interacts with the thyroid hormone receptor alpha1 and modulates the thyroid hormone-response in osteoblastic differentiation. Mol Cell Biochem (2012) 361(1–2):197–208. doi:10.1007/s11010-011-1104-y
128. Strohbach C, Kleinman S, Linkhart T, Amaar Y, Chen ST, Mohan S, et al. Potential involvement of the interaction between insulin-like growth factor binding protein (IGFBP)-6 and lim mineralization protein (LMP)-1 in regulating osteoblast differentiation. J Cell Biochem (2008) 104(5):1890–905. doi:10.1002/jcb.21761
129. Schmid C, Keller C, Gosteli-Peter M, Zapf J. Mitogenic and antiapoptotic effects of insulin-like growth factor binding protein-6 in the human osteoblastic osteosarcoma cell line Saos-2/B-10. Biochem Biophys Res Commun (1999) 263(3):786–9. doi:10.1006/bbrc.1999.1451
Keywords: insulin-like growth factor-binding protein-4, bone, pregnancy-associated plasma protein-A, proteolysis, insulin-like growth factor-binding protein-5
Citation: Beattie J, Al-Khafaji H, Noer PR, Alkharobi HE, Alhodhodi A, Meade J, El-Gendy R and Oxvig C (2018) Insulin- like Growth Factor-Binding Protein Action in Bone Tissue: A Key Role for Pregnancy- Associated Plasma Protein-A. Front. Endocrinol. 9:31. doi: 10.3389/fendo.2018.00031
Received: 24 November 2017; Accepted: 23 January 2018;
Published: 16 February 2018
Edited by:
Andreas Hoeflich, Leibniz-Institut für Nutztierbiologie (FBN), GermanyReviewed by:
Leon Bach, Monash University, AustraliaCunming Duan, University of Michigan, United States
Copyright: © 2018 Beattie, Al-Khafaji, Noer, Alkharobi, Alhodhodi, Meade, El-Gendy and Oxvig. This is an open-access article distributed under the terms of the Creative Commons Attribution License (CC BY). The use, distribution or reproduction in other forums is permitted, provided the original author(s) and the copyright owner are credited and that the original publication in this journal is cited, in accordance with accepted academic practice. No use, distribution or reproduction is permitted which does not comply with these terms.
*Correspondence: James Beattie, ai5iZWF0dGllJiN4MDAwNDA7bGVlZHMuYWMudWs=