- Kinsey Institute and Department of Biology, Indiana University, Bloomington, IN, United States
Vasopressin (VP) and oxytocin (OT) are distinct molecules; these peptides and their receptors [OT receptor (OTR) and V1a receptor (V1aR)] also are evolved components of an integrated and adaptive system, here described as the OT–VP pathway. The more ancient peptide, VP, and the V1aRs support individual survival and play a role in defensive behaviors, including mobilization and aggression. OT and OTRs have been associated with positive social behaviors and may function as a biological metaphor for social attachment or “love.” However, complex behavioral functions, including selective sexual behaviors, social bonds, and parenting require combined activities of OT and VP. The behavioral effects of OT and VP vary depending on perceived emotional context and the history of the individual. Paradoxical or contextual actions of OT also may reflect differential interactions with the OTR and V1aR. Adding to the complexity of this pathway is the fact that OT and VP receptors are variable, across species, individuals, and brain region, and these receptors are capable of being epigenetically tuned. This variation may help to explain experience-related individual and sex differences in behaviors that are regulated by these peptides, including the capacity to form social attachments and the emotional consequences of these attachments.
There is no fear in love: but perfect love casteth out fear. (1 John 4: 18)
Introduction
Oxytocin (OT) and vasopressin (VP) are ancient peptide molecules with many behavioral and physiological functions. These pleotropic peptides evolved from a single genetic source (1). OT and VP, with their receptors, function as an integrated, adaptive system, allowing the mammalian body to survive, maintain homeostasis, and reproduce in an ever-changing world. However, OT- and VP-like molecules were co-opted for other functions many times over the course of evolution (2).
Vasopressin is considered the more ancient molecule, with a central role in defense. OT, especially in a context of safety, may override the defensive functions of VP helping to facilitate the evolution of the complex cognition and selective sociality associated with human behavior, including social attachment and love (3, 4) (Figure 1).
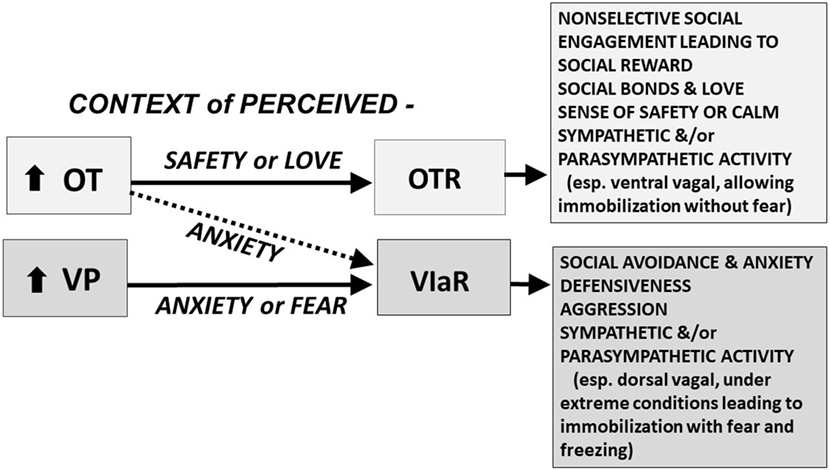
Figure 1. The oxytocin (OT) and vasopressin (VP) pathway includes the OT receptor (OTR) and the V1a receptor (V1aR). We hypothesize that in a context of perceived safety, OT predominately acts on the OTR, facilitating “immobility without fear,” including high levels of social engagement, social bonds, and social reward; these behaviors are at the heart of mammalian reproduction and “love.” VP and the V1aR are more ancient and probably become dominant under conditions of anxiety or trauma. In a context of anxiety or fear, OT may function primarily through effects on the V1aR; under these conditions both OT and VP may act, via the V1aR, to induce additional anxiety, social avoidance, defensiveness, aggression, and fear. We hypothesize that under extreme conditions, fear and the V1aR may dominate leaving the individual vulnerable to “immobility with fear,” which may lead to freezing and cognitive and emotional dissociation. These responses are mediated in part by interactive effects of OT and VP on the sympathetic nervous system and the parasympathetic nervous system, including the ventral vagal complex (necessary for social engagement) and the dorsal vagal complex (functioning to conserve energy and protect against shutting down in the face of trauma) (5). Other components of this adaptive system including the V1bR, and many other molecules or receptors, including those regulated by CRH, dopamine, opioids, GABA, and serotonin, play a role in the expression of social and defensive behaviors. The differential actions of OT and VP are dose, time, and brain-region dependent. The OT and V1a receptors are affected by genetics and epigenetic tuning, especially in early life.
Sources of individual differences in OT and VP and the sensitivity of their receptors include gender and basic genetic differences (6, 7). For example, some species, including humans and other socially monogamous mammals, such as prairie voles and dogs, have high levels of OT (8, 9) and an apparent dependence on OT to allow the expression of high sociality and attention to positive social cues. The OT receptor (OTR) and V1a receptor (V1aR) also can be epigenetically tuned by experience (10–14), increasing the capacity of OT and VP to have complex adaptive functions.
Behavioral work in this field has focused on the neurobiology of OT in social behavior and the management of stressful experiences (3, 4, 15, 16). The systems necessary for actions of OT involve extensive neural networks through the brain and autonomic nervous system. Many recent reviews describe the neural and behavioral roles of these peptides (4, 17–25). Furthermore, these networks are capable of dynamically changing (20, 26, 27), especially in early life (26, 27). Those reviews will not be duplicated here, but in conjunction with primary sources are used as background for a discussion of functional interactions between OT and VP and their receptors in the context of evolution and mammalian social behavior.
The OT and VP Pathway
Current knowledge concerning OT and VP and their receptors indicate that these are interactive components of an evolved and integrated system—here termed the OT–VP pathway (Figure 2). It has long been known that both peptides can bind to both the OT and VP receptors in vitro (28–32). Accumulating evidence dealing with diverse outcomes and from various species supports the hypothesis that when looking at the whole organism OT and VP tend to affect more than one receptor and several types of behavioral functions (7, 20, 33–35). In general in the behavioral literature, OT has received more attention than VP.
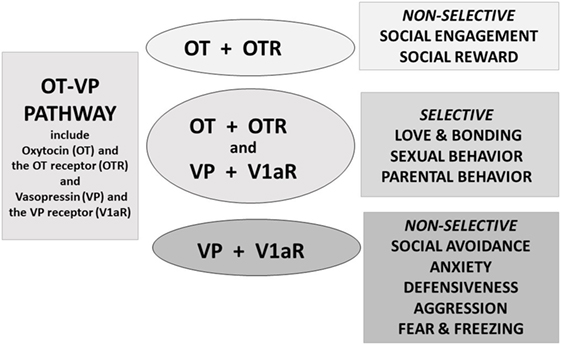
Figure 2. Oxytocin (OT) and vasopressin (VP) are components of an integrated pathway. OT and VP interact dynamically with receptors [including the OT receptor (OTR) or V1a receptor (V1aR)] to influence social engagement and defensive behaviors. In many cases, OT acts in conjunction with VP, via the V1aR or through effects on both the OTR and V1aR, thus regulating the capacity to form selective social behaviors. OT rarely acts alone but, especially under nonthreatening or “safe” conditions, may facilitate features of “love,” including social engagement, and social reward, and “immobility without fear” (36).
The OT–VP pathway allows the body to adapt to highly emotional situations and develop selective attachments. Such experiences require the presence of both peptides (37), as well as molecules associated with reinforcement, such as dopamine (38–40). Conditions under which both OT and VP are necessary for normal behavior include selective social behaviors and emotionally intense experiences, such as sexual behavior, parental behavior, and pair bond formation, as well as regulation of the autonomic nervous system (18, 41, 42).
Until recently OT and VP, and their receptors, were typically treated as independent systems. This is especially true in human studies of the effects of exogenous hormones (43–45). For a notable exception see studies by Rilling and associates, in which both peptides are being studied (46, 47).
Properties of OT and VP
Oxytocin and VP are small peptides that are similar in structure. Both consist of nine amino acids in a six amino acid ring, formed by cysteine bonds, and a three amino acid tail with a terminal amine group. The precursors for OT and VP consist of 12 amino acids and are synthesized and released in conjunction with carrier proteins (neurophysin 1 and 2, respectively). The precursors are later cleaved into the “mature” forms of these peptides. It is also possible that precursors and fragments of OT and VP have unidentified functions (29, 48); although not well studied, it is likely that these forms and the binding of OT and VP in blood and other tissues play a role in the functional interactions of OT and VP (49).
Oxytocin and AVP are primarily synthesized in brain regions that are critical to behavioral and physiological homeostasis. Different cells in specific brain regions produce these two peptides, including the supraoptic nucleus (SON) and paraventricular nucleus (PVN) of the hypothalamus (20). Anatomical studies in rodents indicate that OT and VP are synthesized in discrete areas and in separate cells within the PVN and SON; these cells also produce a network of neural projections reaching throughout the brain and spinal cord (50). For additional details of specific neural targets for OT and VP, see reviews such as those from Wang and his associates (39, 51).
Research using brain slices (25) indicates that in other brain regions, including the amygdala and the bed nucleus of the stria terminalis, both OT and VP containing cells and projections lie adjacent to each other. These OT–VP associations form local functional units, capable of rapid and often opposite interactions—for example, in brain regions associated with fear versus fear reduction. Fear responses are mediated by V1aRs in the amygdala, while OT may act to inhibit fear, depending on context (Figure 3) and gender (52).
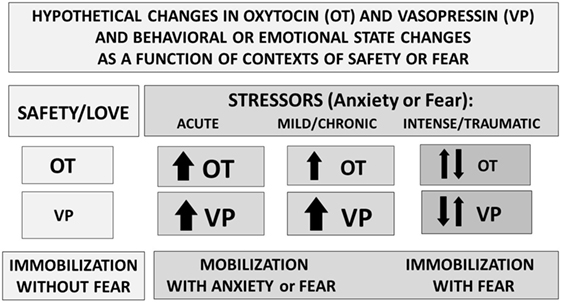
Figure 3. Perceived context and the intensity of challenge can regulate the release or effects of oxytocin (OT) and vasopressin (VP). Under conditions of safety, the actions of OT may dominate, supporting high levels of sociality. In response to an acute stressor, both OT and VP increase, supporting mobilization and escape, followed in some cases by increases in social behavior especially toward “safe” conspecifics. Following intense or traumatic stressors, initial responses would include mobilization and anxiety. However, following a traumatic experience, individuals may vacillate between mobilization and immobilization with fear or revert to the more primitive response of shutting down. These patterns differ between males and females and as a function of individual life histories.
Oxytocin and VP are synthesized and stored in the pituitary gland, where these peptides are thought to remain in vesicles until released as the nine amino acid forms. However, these molecules also may be released from axons within the CNS (20), as well as from the neuronal soma and dendrites or by diffusion within the brain (50). In addition, OT and VP are made throughout the body with local effects on diverse tissues, including the uterus, testes, digestive system, kidney, and thymus (53, 54). The dynamic nature of the OT–VP pathway not only makes this system exciting but also limits research in this field.
Sex differences are adaptive and commonly seen in studies of actions of OT, and especially VP. Sex differences are not always explored, but when they are, males and females frequently differ (52); this is especially true with reference to reactions to treatments involving stressors (3). Most of these studies suggest that males have either more VP (55) or are more sensitive to the effects of VP (56). For example, in a quantitative study of 22 subregions in the forebrain “social behavioral neural network” in rats, VP immunoreactivity show marked regional variation between males and females and as a function of age (57). These differences were particularly apparent in the medial amygdala, bed nucleus of the stria terminalis, and lateral septum—brain regions previously implicated in androgen-dependent sex differences and in defensive aggression. OT immunoreactivity did not show this pattern of variation in rats.
Receptors for OT and VP
The gene (OXTR) for the OTR is found on human chromosome 3. The same OTR located in breast, uterus, and neural tissue also is present in many other bodily tissues. Three VP receptor subtypes are expressed in different tissues, and their genes are located on separate chromosomes. The V1aR is found in nervous system and throughout the cardiovascular system with a broad set of behavioral functions. The VP V1b receptor is not only found in the pituitary but also in brain areas with a role in the management of stress and aggression (58, 59). The VP V2 receptor is localized primarily to the kidney with a classical role in fluid balance.
The V1aR evolved from and is homologous with the vasotocin receptor (2). There is a high level of homology among the OTR and the three VP receptors, especially in the extracellular binding domain which allows OT and VP to bind to each other’s receptors (30, 32, 60). The pharmacological tools available for identifying, stimulating, or blocking receptors for OT and VP often have not been sufficiently selective to allow easy identification or manipulations of these receptors (61).
In mammals, receptors for OT and/or VP are typically abundant in areas of the nervous system that regulate social, emotional, and adaptive behaviors and reward (17). Among the regions with high levels of OTR or V1aR are various parts of the amygdala, the bed nucleus of the stria terminalis, the nucleus accumbens, brainstem source nuclei for the autonomic nervous system (25, 39, 62), and systems that regulate the hypothalamic–pituitary–adrenal (HPA) axis. OTRs also are found in cortex and hippocampus; these are highly variable among species and individuals, with possible consequences for neural and behavioral plasticity (63–66). In the cortex and in the spinal cord, both the V1aR (67) and OTR are present, allowing the possibility for interactions in processes such as social cognition and pain (68).
Expressions of the OTR and V1aR in the nervous system are highly variable even within species; for example, brain regions and individual differences are related to functional and adaptive patterns of sociality and aggression (6, 12, 24, 62, 69, 70). VP and OT and in some cases their receptors may differ between males and females, and across the lifespan (7, 39, 51, 52, 71, 72). The receptor variation characteristic of this system, and especially of VP has been associated with species and individual variation in behavior and brain function. Although sometimes overlooked in behavioral research, both peptides can have regulatory effects throughout the entire body, including the autonomic nervous system (42) and the immune system (53, 73–75) with effects on inflammation and healing.
Initially, it was assumed that only one primary receptor existed for OT (76). Genes for the OXTR and the three VP receptors code for separate G-protein coupled receptors, each with a seven transmembrane domain. Peptides binding to these receptors trigger subcellular cascades. The subcellular signaling pathways are not identical for these different receptors. In addition, the capacity of OT or VP to activate a given receptor subcellular signaling pathway may differ according to the concentrations of the peptides and the regional location of receptors in the nervous system (20, 25, 61). These subcellular differences may help to explain the capacity of OT and VP to have different functions in various processes, such as birth (77), social behavior (17, 34), and reactivity to stressors (13, 16, 25). Further adding to the complexity of this system is the possibility that receptors for OT and VP can form heterodimers with unknown consequences for peptide binding (20).
Evolution and Sociality
Oxytocin and VP are genetic and biochemical siblings. Both originated from a single ancestral gene that produced vasotocin (1, 2). Vasotocin is found in reptiles and other vertebrates and can be measured in the mammalian fetus. OT and VP differ from vasotocin by one amino acid and from each other by two amino acids. It is estimated that the ancestral peptides arose over 500 million years ago, that VP evolved approximately 200 million years ago and OT approximately 100 million years ago, originally through gene duplication (1). The genes for OT and VP reside near each other on human chromosome 20, lying in opposite transcriptional orientations (48).
Compared with OT, VP is the more primitive molecule and closer in function to vasotocin (1, 2, 23). However, other OT-like peptides, including mesotocin and isotocin, have functions that resemble those of OT. Animals that evolved from reptiles, including mammals and birds may be particularly dependent on selective social behaviors and OT-like peptides for reproduction and survival.
Vasopressin-like molecules are critical to adaptation and water balance under difficult environmental conditions. VP can support sympathetic arousal, mobilization (flight–flight responses) or in more extreme cases a metabolically conservative, shutdown response (5). Thus, under conditions of extreme stress or trauma, VP may take precedent over OT and over survival strategies that are more prosocial or mobilized (Figure 3). However, the benefits of either sociality or OT also may be most easily detected in the presence of a stressor or of VP (3, 37).
Combined Actions of OT and VP
At the core of positive social behaviors are neurobiological systems that regulate fear and threats versus safety (Figures 1–3). OT typically supports immobilization without fear, necessary in interactions with family and friendly associates (36). VP supports mobilization, and in some cases defensive aggression and protection of social boundaries. By contrast, VP, in conjunction with CRH, dopamine and many other molecules, may support active and mobilized coping strategies (3, 71, 78). However, as with many features of the OT–VP pathway, exceptions exist—possibly because of the capacity of OT and VP to interact with each other’s receptors (Figure 1).
Increasing evidence suggests that the actions of OT on the V1aR, versus the OTR, vary depending on the behavior and context being examined (7, 17) (Figure 1). In hamsters, fear-based or aggressive effects of OT rely on the V1aR and social reward on the OTR (34, 79, 80). The capacity of OT and VP to bind to each other’s receptors adds complexity to attempts to understand both peptides. However, OT–VP interactions also are adaptive, increasing the capacity for a small number of peptides and receptors to regulate various processes across different tissues.
Both OT and VP are responsive to environmental and social demands, although in somewhat different ways (3, 16). These peptides—presumably via interactions with their receptors—may have diverse physiological and behavioral properties. Regional effects of OT and VP are expected and need to be investigated to fully understand the functional consequences for these peptides. Dynamic interactions either on specific receptors, due to brain region-specific actions, or due to relative availability of the peptides to a receptor (20) could help to explain the behavioral properties of these two molecules. In addition, refined behavioral studies are necessary, since the effects of OT and VP on various behaviors change across time and as behavioral context changes.
As detailed below, a number of studies have attempted to separate the effects of OT versus VP on reproduction, social behavior, and aggression (7, 17). In general, it appears that OT plus VP may be especially critical to allow selective social experiences that involve awareness the individual identity of a partner and the experience of a social reward (18, 37). These behaviors also may require alternating between behavioral mobilization and immobilization, which is seen after trauma (36) (Figure 3).
Methodological Limitations and “Cross Talk” Between OT and VP
The evolved properties of OT and VP permit “cross talk” between these peptides and their receptors (7, 17, 20, 31, 33, 77) (Figure 1). Unexpected outcomes are sometimes reported when exogenous OT or VP is given or when a peptide or specific receptor is inactivated. This work initially depended on pharmacological agonists or antagonists, often using drugs that were relatively non-specific. More recently research, primarily in rodents, has used genetic manipulations including rodents with mutations (81) or optogenetic methods for silencing or activating genes for peptides or receptors (40, 82).
Early evidence for cross talk between VP and the OTR came from research in OT knockout (OTKO) mice (31). For example, single-unit recording from tissue slices from the ventromedial hypothalamus from OTKO mice revealed that VP was capable of stimulating this brain region. Moreover, whether findings from mutant mice can be generalized to wild-type animals remains unclear. For example, OTKO mice showed increased sensitivity to VP. This important finding suggested that the absence of OT across the lifespan could sensitize animals to later VP exposure. The molecular basis of this process remains to be discovered. Components of some functions, such as birth and maternal behavior, continue to be observed in OTKO mice (83, 84). However, upon careful examination, these behaviors often lack the full range of behavioral expression typical of wild-type animals (81).
Similar methodology also has been used to study the OTR. Reductions in social behavior and cognitive flexibility and increases in aggression and seizure susceptibility are seen in OTR-null mice (85). These behaviors in OTR-deficient mice can be rescued not only by OT but also by VP treatments. This may be another expression of the capacity of the nervous system to adapt to changes in the peptidergic systems. However, studies of animals that are missing only one allele for the gene regulating the OTR show selective deficiencies in social behaviors, but not aggression (85). This study further supports the hypothesis that positive social behaviors may be especially sensitive to the reductions in OTR activity, while more defensive and perhaps more primitive processes are preserved.
Interactions among OT and VP and their receptors allow adaptive functions in time frames that are both short term and long term. Studies comparing the short-term versus long-term interactions between OT and VP are rare. However, those studies that do exist suggest that acute versus chronic actions of OT and VP can be very different (Figure 3), and sometimes opposite in function (86). Based on the behavioral patterns that are seen following acute versus chronic exposure to exogenous OT, we can hypothesize that the long-term effects of OT, and possibly the effects of very high levels of OT may involve stimulation of the V1aR (Figure 1).
Behavioral studies dealing with OT’s capacity to affect VP receptors have focused on OT’s effects on the V1aR or combined effects of OT and VP on the OTR and/or the V1aR (Figure 2). In general, the combined effects of OT plus VP are associated with highly rewarding experiences including some components of sexual behavior, parental behavior, and pair bond formation. At present, only a very limited number of studies seem to support the notion that OT functions primarily at the OTR without the participation of the V1aR (7, 82). Among the functions that seem especially dependent on OT are comparatively “modern” mammalian functions including lactation, reversal learning, and behavioral plasticity (33, 34, 61). Whether VP can stimulate the OTR in vivo has received less attention (80).
Interactive Functions of OT and VP
Caveats
Examples of specific studies of functional interactions within the OT–VP pathway are described below. In some cases, only a portion of the possible interactions only a portion has been tested. In most, but not all cases, OT has been shown to have the capacity to affect the V1aR. In cases deliberately involving a stressor, effects of OT or VP that were not otherwise detected may emerge. A possible role for the VP V1bR is beyond the scope of this review, but effects of stress and OT on the VP V1bR also are possible (81). Among the many other molecules of importance to the regulation of OT and VP are CRH (87), GABA (88), dopamine (38), and serotonin (89, 90); these molecules also play roles in the modulation of stress and coping. Brain region- and cell type-specific changes are another source of variation that is relevant to understanding how OT and VP interact. New technologies, such as optogenetics, are allowing more specificity in neural circuitry but are currently limited to comparatively simple behaviors or components of behavioral patterns. There is increasing evidence that the OT–VP receptor pathway is epigenetically tuned by experience, including gonadal hormones, stressors, and probably peptides as well (10, 11, 13, 14, 75). Although not reviewed here, processes such as methylation may be of particular relevance to explaining the role of context and experience in the regulation of social behavior.
Lactation
Lactation is a defining feature of mammals, and contraction of breast tissue and milk ejection requires stimulation of the OTR. Lactation arose in conjunction with the evolution of mammals and is one of the comparatively few reproductive functions that do not continue in the absence of OT or the OTR (81, 83, 84). Immature mammalian offspring depend for varying periods of time after birth on their mother’s milk. Conservation of fluids is necessary for lactation and effects of VP on the kidney and blood pressure probably support normal milk production, but this is presumably under separate control from milk ejection.
Birth and Uterine Contractions
Observations at the beginning of the twentieth century offered early evidence that OT and VP interactions are components of the normal functions of these peptides. Research conducted by Sir Henry Dale in 1906 showed that an extract from the human posterior pituitary gland was capable of producing contractions in the uterus of a pregnant cat. The pituitary gland contains both OT and VP and the effects of pituitary extracts probably reflected the effects of both peptides and possibly other hormones (91).
In his Nobel Lecture, describing the functions of the first “polypeptide,” Vincent du Vigneaud mentions two assays used to test the biological activity of OT. In that research, du Vigneaud (Nobel Lectures, 1955, p. 461) used rat uterine strips, but also noted that he used the “chicken vasopressor method of Coon, which utilizes the property of OT to lower the blood pressure of the fowl and has been adopted by the United States Pharmacopeia as the method for assay for OT.” The use of a vasopressor response to assay OT, indirectly acknowledged the capacity of OT to stimulate the VP system. This was one of the first of what would eventually be many lines of research documenting interactions between OT and VP.
In large mammals, OT adopts a central role in reproduction by helping, in some cases, to expel the big-brained baby from the uterus (4, 18). However, in mice and presumably other mammals, birth can occur without OT (81, 83, 84). Egg laying, which is the precursor to birth, appeared long before the evolution of mammals, and thus may rely on more ancient hormones, including VP or vasotocin.
Although OT has been assumed to play a fundamental role in birth, current evidence suggests that OT alone acting on the OTR is NOT capable of inducing normal labor and blocking only the OTR does NOT prevent premature birth. Rather both OT and VP and both the OTR and V1aR regulate uterine contractions (60, 92). Thus, it is not surprising that female mice made mutant for OT or the OTR remain capable of giving birth (83, 84). In fact, especially under conditions of stress, VP is likely to have a much greater role in birth than has been acknowledged. VP’s effects on the uterus, although functionally different from OT, may help to explain premature labor and preeclampsia, which are associated with adversity or stress across the life span (77, 91).
Parental Behavior
Early research on OT revealed consequences for maternal behavior (93) and filial bonding (94). Although, a role for OT in maternal behavior is now widely accepted, this work was initially controversial (95). Apparent discrepancies regarding the necessity of OT to maternal behavior may have been due to experimental differences related to the role of stress in mothering. Effects of acute OT seem to be most apparent in the face of novelty, acute stressors or against a background of elevated HPA axis activity (96, 97). In the presence of OT, avoidance or fear of the infant may be replaced by approach and positive emotional states (3). Whether this is due to competitive inhibition of VP or more specific actions of OT on the HPA axis deserves additional study.
A functional role for VP in maternal behavior cannot be excluded. Pedersen and colleagues found that centrally administered VP increased maternal behavior in rats, although the effects of VP took longer to appear than those seen after OT. OTKO mice remain maternal to some extent, but their behavioral patterns are not identical to those in wild-type mice (81). The role of OT in maternal behavior may depend in part on the capacity of OT to directly or indirectly override the defensive effects of VP and reduce fear in the presence of young animals. VP, in conjunction with OT, also supports the capacity to protect offspring, in the form of postpartum maternal or paternal aggression in rodents (98, 99).
Threatening Environments and Aggression
Vasopressin and the V1aR may be of critical importance in the capacity for physical and emotional adaption in the presence of stressful experiences (3, 16, 24, 25). VP is involved, synergizing with CRH (78), in hypothalamic regulation of the pituitary, supporting the release of glucocorticoids and mobilized defense strategies against various physical and emotional stressors or threats (25). OT also can be released during stressful experiences and is sometimes considered a “stress-coping” molecule.
Vasopressin also plays a protective role in the behavioral defense of self and the family (3, 100). Various forms of aggression and territoriality have been related to stimulation of the V1aR in both males and females (7, 101, 102). However, at least in golden hamsters the mediation of dominance and aggression was associated with increases in hypothalamic VP in males (but not in females). By contrast, serotonin, acting in the dorsal raphe, was associated with increased aggression in females, and decreased aggression in males (90).
Avoidance of Danger and Anxiety
A growing literature associates increased central VP in the development of memory necessary for the avoidance of danger or survival (24). Psychological processes associated with anxiety and obsessions also may rely on VP (7, 103, 104). VP, in the context of other centrally active molecules, such as CRH, dopamine, and serotonin, regulates emotional states, including anxiety (Figure 3). Anxiety in turn can reduce the capacity to use cognitive or “top down” strategies to manage stressful experiences. VP and CRH can amplify the effects of each other on aggression and anxiety, especially during circumstances involving intense challenges (101, 103).
Increased activity in the central VP system may lower thresholds to impulsive forms of aggression, possibly by reducing cortical inhibition (105). The actions of VP also help to explain the association of anxiety and ruminations with cardiovascular risk (106). VP plays a central role in circadian rhythms and is likely to be important in sleep disturbances or elevations in blood pressure, which are also common following stress and considered defining features of posttraumatic stress (PTS) disorders. In human males, high blood levels of VP have been correlated with emotional dysregulation and aggression (107).
Vasopressin is associated with physical and emotional mobilization and helps support vigilance and behaviors needed for guarding a partner or territory (3), as well as other forms of adaptive self-defense (103). Prairie voles have provided a useful model for examining the importance of peptides in selective aggression (108). In this species, immediately after mating males became lethally aggressive toward strangers, but not familiar partners or family members; this response was blocked by antagonists for the V1aR (109). The formation of partner preferences and pair bonds requires access not only to the V1aR but also the OTR (37). Mate guarding and parental aggression offer examples, among several, suggesting the importance of both OT and VP and their receptors in behaviors that are socially selective (Figure 2).
Non-Selective versus Selective Social Behaviors
Oxytocin’s role in social behaviors has been documented in many species, including humans (4, 18, 110, 111). Based primarily on work in nonhuman animals, in many, but not all cases, the effects of OT are mediated via VP receptors. This seems to be the case in behaviors that are non-selective, such as a general tendency toward sociality or gregarious behavior (Figure 2). This may include behavioral patterns involving social recognition (56, 112). Among other examples, in which both OT and VP receptors were examined, are social contact, including lying adjacent to another member of the same species (113) and huddling with conspecifics in the presence of the odor of a predator (114); these were facilitated by OT but only when the V1aR was accessible. In another example, in golden hamsters the effects of OT on social reward required access to the OTR (80). By contrast, in hamsters for OT to affect aggression, activation of the V1aR was necessary (79).
Research, initially conducted in prairie voles, demonstrated the capacity of OT to increase social contact between adults (115). This work led to studies showing a role for OT and the OTR in the formation of selective social bonds (116). However, in studies in which either the OTR or VP V1a were blocked, both OT and VP receptors were necessary for pair bond formation (37). When both OTR and the V1aR were blocked animals showed very low levels of contact behavior. In pair bond formation, OT and VP interact with motivational and reward systems and may enhance or otherwise amplify the effects of other molecules including dopamine and opioids in specific brain regions, including those that have been implicated in both maternal behavior and social bonding (38, 81, 117).
Social Learning and Conditioning
Research on the behavioral effects of the OT–AVP system began with studies of memory, including avoidance learning (118) and social recognition (56, 112). These continue to be major topics in studies of the functions of the OT–VP pathway (24, 33). Learning of context and cues, as well social salience, may be affected by access to the OTR. There is an increasing tendency to direct attention to specific brain regions. In rodents, brain systems involved in reinforcement and reward, including the nucleus accumbens and ventral tegmental area, have high levels of both OTR and dopamine. OT-related sociality, probably in conjunction with the actions of dopamine, is reinforcing (40, 82). Only a few studies have suggested functions in which OT acts solely via the OTR, without access to the V1aR. For example, in mice, exogenous OT is capable of modulating fear conditioning following treatments directed at the lateral septum (119). OT in the lateral septum reduced fear following a positive social encounter but facilitated fear conditioning after a prior negative social encounter (120). In rats, fear conditioning also was enhanced by OT administered in the bed nucleus of the stria terminalis; blocking access to the OTR eliminated conditioned fear responses, while non-conditioned fear responses were not affected (121). In addition, in rats, effects of peripherally administered OT on neural activation in the central amygdala (indexed by cFos expression) continued to be present even following treatment with a V1aR antagonist. OT may act to increase sociality in the face of fear or challenge, including effects of exogenous OT measured by regional change in cFos in other brain areas. As one example, neural activation by OT in the hypothalamus and brain stem did require V1aR stimulation; among the other brain regions in which cFos was increased by OT and blocked by a V1aR antagonist were the SON, PVN, locus coeruleus, and nucleus tractus solitarius (35). The latter brain areas have many functions, including regulation of the autonomic nervous system and HPA axis, which are necessary for the optimal expression of social behavior (5).
Dose-Dependent Effects of OT: More Is Rarely Better
When infant prairie voles received a low dose of exogenous OT immediately following birth, they showed as adults increased OT in the CNS and an increased tendency to form a pair bond. However, when higher doses of OT were administered, a single exposure to OT in early life disrupted the later capacity to pair bond. Females exposed neonatally to a high dose of OT later preferred a stranger. Stranger preference in prairie voles is very atypical (122, 123) and, especially in males, is most commonly associated with stressful experiences or stress hormones including CRH and cortisol (87, 124).
These and many other experiments suggest that the effects of OT are dose dependent. Low doses may appear to be beneficial, while higher doses of OT can have detrimental behavioral consequences and in some cases may stimulate the VP receptor. Low to moderate doses of OT, especially as acute treatments may reduce anxiety in the face of a challenge or stressor. By contrast, larger amounts of OT, especially if given as a chronical treatment may no longer be anxiolytic, and can have the opposite effect. Chronic or very high levels of OT can reduce the capacity to respond to OT possibly by reducing OTR or binding to the OTR, while also allowing OT to activate VP receptors (86). In another example, when male mice were tested in a social stress paradigm, chronic and high levels of OT (given centrally) were associated with an increase in anxiety-like behaviors; in that study OTR binding was also reduced in the amygdala and septum (125). Perhaps in individuals primed by negative experience, small amounts of OT are capable of activating VP receptors, further supporting mobilization and potentially defensive emotional or behavioral responses. Based on data from OTKO mice, in which the VP system was sensitized (31), we also can hypothesize that individuals (including humans) with low levels of endogenous OT might be more likely to experience increased VP-like activities even when given OT.
Studies of OT, and less commonly VP, using intranasal infusions have generated an increased interest in the behavioral effects of these peptides. The intent is to non-invasively deliver peptides to the brain and there is increasing evidence that this is possible (126, 127). However, it is useful to note, based on imaging studies in rodents, that the tissues activated by exogenous peripheral versus central applications of OT are not identical (128). Furthermore, the concentrations chosen for most human studies are generally arbitrary and based on doses of OT medically available as an intranasal “lactational aid.” Studies using different amounts of OT are needed to examine possible threshold differences among individuals as a function of gender, experience, and emotional lability (129). Different doses of a given peptide can produce different effects, and dose–response curves are only just appearing in this literature (130).
Are There Unique Functions for OT?
In mammals, we have argued that under optimal conditions OT appears to serve as a physiological metaphor for “safety” (18). OT is of special relevance to physical and mental protective adaptations that involve high levels of sociality, a sense of psychological safety within a family or familiar social group, as well as emotional regulation that is necessary for mental health and higher levels of rational cognition (4). At least in rodents, OT seems to play an important role in cortical functions necessary for social cognition (24) and social reward (79).
Oxytocin also promotes autonomic flexibility in the face of threats (42). Parental care and social support in a safe context are particularly important in species of mammals adapted to live in extended families or groups, including humans and prairie voles (131). Social contact between adults or adults and offspring is a defining feature of most families. However, social contact, necessary for mating, parental behavior and nursing, can be dangerous and requires a physiological and autonomic state that permits “immobilization without fear” (36). This behavioral response may be especially adaptive in females but also may leave females more sensitive than males to the consequences of traumatic experiences and symptoms of PTS.
Mammals, with their comparatively large brains, are particularly vulnerable to the need for oxygen, and under extreme conditions the functions of OT may shift from social behavior to survival and protection of the cortex, including dissociation or even loss of consciousness (4) (Figure 3). In mice, exogenous OT elicited a transient activation of cortical regions and a sustained activation of hippocampal and forebrain regions. It is interesting to note that in mice intranasal VP produced a sustain deactivation of pathways associated with cortical function. Many effects of VP still existed when OTRs were genetically deleted, presumably reflecting the capacity of VP to activate cortico-parietal, thalamic, and mesolimbic regions via VP V1aRs (105). Whether V1aRs, possibly responding to OT, can assume such roles in primates needs additional study (62).
Does OT Act Alone?
Many important functions including birth and selective social behaviors, another form of learned behavior, appear to rely on both OT and VP and their receptors. It is uncommon to find evidence that OT functions solely via the OTR. Lactation is one comparatively “modern” function of OT (81). Social reward may be another OT–OTR based function (79, 80), perhaps requiring a co-activation of localized dopaminergic systems (17).
Under circumstances of acute stress or prolonged isolation OT (in females) can be released (132) (Figure 3). If acting on the OTR or the V1aR this OT could allow stress coping (16). However, especially after early-life adversity, epigenetic sensitization or upregulation of VP (133) and V1aR (11) can occur. Under these conditions, OT may no longer be sufficient to be protective. Furthermore, OT may stimulate VP receptors. Thus, although OT is normally protective against stress, if it acts on the VP receptor system the effects may be seen as exacerbation of stress reactivity or anxiety. This may be a particular problem in individuals with a history of trauma and neglect, for whom the effects of exogenous OT have been reported as socially negative or “antisocial” (110, 134, 135).
Mechanisms for OT–VP Interactions
The mechanisms underlying OT–VP interactions in vivo remain largely to be understood. In the face of a challenge, the interactive effects of OT and VP appear to be hierarchical. The more modern peptide, OT, may act via the presumably older V1aR either as an agonist or perhaps as a competitive antagonist (Figure 1). Furthermore, the functions of OT and VP may be regulated by various other processes, including differential availability of OT or VP (or their receptors) which may be regulated locally in the nervous system (20).
The Paradox—Why Are the Social Effects of OT Unpredictable?
As data have accumulated, apparent inconsistencies or “paradoxical” effects of OT have emerged (44, 45). For example, a tendency toward parochial behavior and “outgroup” rejection was described in some human studies after intranasal OT (45, 134, 135). Treatment with OT also has been implicated in increased aggressive tendencies in certain kinds of computer games, an effect that was attributed to an OT-induced increase in social salience (136). These responses may be adaptive but also could reflect the kind of receptor “cross talk” described in studies in nonhuman animals.
In mice (86, 137) and voles (138), chronic OT exposure has been either relatively ineffective or even had negative effects on social behavior. When OT levels are high or chronically elevated their effects may be primarily due to stimulation of VP receptors with a concomitant downregulation of the OTR. This pattern of exposure to either exogenous or endogenous OT might support mobilization and potentially defensive responses, rather than positive sociality and a reduction in anxiety (Figure 3).
The history of the individual, including prior exposure to early-life stress, also can influence the response to OT. Early maltreatment also has been associated with an increase in endogenous OT (27, 139). In another example, individuals who described themselves are relatively lonely were less likely to show an OT-associated increase in parasympathetic activity (140). Perceived loneliness, isolation in early life or maltreatment might alter thresholds for physiological consequences of exogenous OT, also possibly by upregulating VP receptors and/or downregulating the OTR.
In the presence of a challenge or a negative environment (141), OT of either endogenous or exogenous origins, perhaps acting on VP receptors, could support arousal, including activation of CRH, the sympathetic nervous system and other components of the HPA and autonomic nervous system (5, 140). The interactive effects of OT and VP, including actions on the V1aR may help to explain the observation that treatment with OT has frequently been associated with antisocial behaviors, especially in a context of fear or danger.
Summary
Across the lifespan, the effects of OT and VP dynamically interact to adjust to and influence the perception of fear and safety. VP is the evolutionarily older molecule with presumably the older receptors. VP is implicated in mobilized behaviors including defense of self and the family. Among the patterns of behavior for which both OT and VP may be necessary are sexual behavior (142), paternal behavior, and pair bonding (18). OT is of special relevance to adaptations that involve high levels of sociality, a sense of psychological safety within a family or familiar social group, as well as emotional regulation and higher levels of rational cognition (4). Furthermore, working together OT and VP, and their receptors, create a biological and genetic pathway that regulates attachment and bonding, which in turn may be protective against threats or other forms of challenge.
The nature of interactions of OT and VP at their receptors needs further study, especially in vivo and the epigenetic context of development (26). There is considerable interest in using OT-like molecules as therapeutics. However, the evolved and dynamic features of the OT–VP pathway create difficulties for attempts to study OT and VP independently. These also pose challenges for the usefulness of drugs based on this system, including those commonly used around the time of birth, such as synthetic forms of OT, which may affect both the OT and VP receptors.
Author Contributions
CSC conceived and wrote this review.
Conflict of Interest Statement
The author declares that the research was conducted in the absence of any commercial or financial relationships that could be construed as a potential conflict of interest.
Acknowledgments
My thanks to Heather Caldwell for the invitation to submit this review. I am grateful to Stephen W. Porges whose intellectual challenge and emotional input has consistently supported my desire to understand oxytocin. I also wish to especially thank Will Kenkel, who helped gather the bibliography in this paper, and to Will, Allison Perkeybile, Jason Yee, Craig Ferris, Hossein Nazarloo, Jessica Connelly, Eric Porges, and Seth Porges, whose discussions and data have helped me untangle this complex literature. Research described from our laboratory was primarily supported by NIH, most recently by P01 HD 075750.
Funding
Research contributed by Dr. Carter’s laboratory was funded by Eunice Kennedy Shriver National Institute of Child Health and Human Development, P01 HD 07575.
References
1. Acher R, Chauvet J, Chauvet MT. Man and the chimaera. Selective versus neutral oxytocin evolution. Adv Exp Med Biol (1995) 395:615–27.
2. Goodson JL, Kingsbury MA. What’s in a name? Considerations of homologies and nomenclature for vertebrate social behavior networks. Horm Behav (2013) 64:103–12. doi:10.1016/j.yhbeh.2013.05.006
3. Carter CS. Neuroendocrine perspectives on social attachment and love. Psychoneuroendocrinology (1998) 23:779–818. doi:10.1016/S0306-4530(98)00055-9
4. Carter CS. Oxytocin pathways and the evolution of human behavior. Annu Rev Psychol (2014) 65:17–39. doi:10.1146/annurev-psych-010213-115110
5. Porges SW. The Polyvagal Theory: Neurophysiological Foundations of Emotions, Attachment, Communication and Self-Regulation. New York, NY: WW Norton and Co (2011).
6. Okhovat M, Chen IC, Dehghani Z, Zheng DJ, Ikpatt JE, Momoh H, et al. Genetic variation in the developmental regulation of cortical avpr1a among prairie voles. Genes Brain Behav (2017). doi:10.1111/gbb.12396
7. Albers HE. Species, sex and individual differences in the vasotocin/vasopressin system: relationship to neurochemical signaling in the social behavior neural network. Front Neuroendocrinol (2015) 36:49–71. doi:10.1016/j.yfrne.2014.07.001
8. Kramer KM, Cushing BS, Carter CS, Wu J, Ottinger MA. Sex and species differences in plasma oxytocin using an enzyme immunoassay. Can J Zool (2004) 82:1194–200. doi:10.1139/z04-098
9. Rubin LH, Wehring HJ, Demyanovich H, Carter CS, Pournajafi-Nazarloo H, Feldman SM, et al. Peripheral oxytocin and vasopressin are associated with clinical symptom severity and cognitive functioning in midlife women with chronic schizophrenia. Schizophr Res (2017). doi:10.1016/j.schres.2017.09.041
10. Gregory SG, Connelly JJ, Towers AJ, Johnson J, Biscocho D, Markunas CA, et al. Genomic and epigenetic evidence for oxytocin receptor deficiency in autism. BMC Med (2009) 7:62. doi:10.1186/1741-7015-7-62
11. Murgatroyd CA, Patchev AV, Wu Y, Micale V, Bockmuhl Y, Fischer D, et al. Dynamic DNA methylation programs persistent adverse effects of early-life stress. Nat Neurosci (2009) 12:1559–66. doi:10.1038/nn.2436
12. Okhovat M, Maguire SM, Phelps SM. Methylation of avpr1a in the cortex of wild prairie voles: effects of CpG position and polymorphism. R Soc Open Sci (2017) 4:160646. doi:10.1098/rsos.160646
13. Murgatroyd CA, Nephew BC. Effects of early life social stress on maternal behavior and neuroendocrinology. Psychoneuroendocrinology (2013) 38:219–28. doi:10.1016/j.psyneuen.2012.05.020
14. Puglia MH, Lillard TS, Morris JP, Connelly JJ. Epigenetic modification of the oxytocin receptor gene influences the perception of anger and fear in the human brain. Proc Natl Acad Sci U S A (2015) 112:3308–13. doi:10.1073/pnas.1422096112
15. Neumann ID, Landgraf R. Balance of brain oxytocin and vasopressin: implications for anxiety, depression, and social behaviors. Trends Neurosci (2012) 35:649–59. doi:10.1016/j.tins.2012.08.004
16. Neumann ID, Slattery DA. Oxytocin in general anxiety and social fear: a translational approach. Biol Psychiatry (2016) 79:213–21. doi:10.1016/j.biopsych.2015.06.004
17. Caldwell HK, Albers HE. Oxytocin, vasopressin, and the motivational forces that drive social behaviors. Curr Top Behav Neurosci (2016) 27:51–103. doi:10.1007/7854_2015_390
18. Carter CS. Oxytocin and human evolution. Curr Top Behav Neurosci (2017). doi:10.1007/7854_2017_18
19. Carter CS, Keverne EB. The neurobiology of social affiliation and pair bonding. 3rd ed. In: Pfaff DW, Joels M, editors. Hormones, Brain and Behavior. Oxford: Academic Press (2017). p. 117–43.
20. Chini B, Verhage M, Grinevich V. The action radius of oxytocin release in the mammalian CNS: from single vesicles to behavior. Trends Pharmacol Sci (2017) 38(11):982–91. doi:10.1016/j.tips.2017.08.005
21. Ebstein RP, Knafo A, Mankuta D, Chew SH, Lai PS. The contributions of oxytocin and vasopressin pathway genes to human behavior. Horm Behav (2012) 61:359–79. doi:10.1016/j.yhbeh.2011.12.014
22. Feldman R, Monakhov M, Pratt M, Ebstein RP. Oxytocin pathway genes: evolutionary ancient system impacting on human affiliation, sociality, and psychopathology. Biol Psychiatry (2016) 79:174–84. doi:10.1016/j.biopsych.2015.08.008
23. Kelly AM, Goodson JL. Social functions of individual vasopressin-oxytocin cell groups in vertebrates: what do we really know? Front Neuroendocrinol (2014) 35:512–29. doi:10.1016/j.yfrne.2014.04.005
24. Ophir AG. Navigating monogamy: nonapeptide sensitivity in a memory neural circuit may shape social behavior and mating decisions. Front Neurosci (2017) 11:397. doi:10.3389/fnins.2017.00397
25. Stoop R, Hegoburu C, Van Den Burg E. New opportunities in vasopressin and oxytocin research: a perspective from the amygdala. Annu Rev Neurosci (2015) 38:369–88. doi:10.1146/annurev-neuro-071714-033904
26. Hammock EA. Developmental perspectives on oxytocin and vasopressin. Neuropsychopharmacology (2015) 40:24–42. doi:10.1038/npp.2014.120
27. Toepfer P, Heim C, Entringer S, Binder E, Wadhwa P, Buss C. Oxytocin pathways in the intergenerational transmission of maternal early life stress. Neurosci Biobehav Rev (2017) 73:293–308. doi:10.1016/j.neubiorev.2016.12.026
28. Du Vigneaud V. Oxytocin, the principal oxytocic hormone of the posterior pituitary gland: its isolation, structure, and synthesis. Experientia (1955) (Suppl 2):9–26.
29. de Wied D, Elands J, Kovacs G. Interactive effects of neurohypophyseal neuropeptides with receptor antagonists on passive avoidance behavior: mediation by a cerebral neurohypophyseal hormone receptor? Proc Natl Acad Sci U S A (1991) 88:1494–8. doi:10.1073/pnas.88.4.1494
30. Burbach JP, Adan RA, Cox JJ, Da Silva SL. Transactivation of the rat oxytocin and vasopressin promoters by nuclear hormone receptors. Regul Pept (1993) 45:31–5. doi:10.1016/0167-0115(93)90178-B
31. Ragnauth AK, Goodwillie A, Brewer C, Muglia LJ, Pfaff DW, Kow LM. Vasopressin stimulates ventromedial hypothalamic neurons via oxytocin receptors in oxytocin gene knockout male and female mice. Neuroendocrinology (2004) 80:92–9. doi:10.1159/000081844
32. Manning M, Stoev S, Chini B, Durroux T, Mouillac B, Guillon G. Peptide and non-peptide agonists and antagonists for the vasopressin and oxytocin V1a, V1b, V2 and OT receptors: research tools and potential therapeutic agents. Prog Brain Res (2008) 170:473–512. doi:10.1016/S0079-6123(08)00437-8
33. Chini B, Leonzino M, Braida D, Sala M. Learning about oxytocin: pharmacologic and behavioral issues. Biol Psychiatry (2014) 76:360–6. doi:10.1016/j.biopsych.2013.08.029
34. Song Z, Larkin TE, Malley MO, Albers HE. Oxytocin (OT) and arginine-vasopressin (Avp) act on OT receptors and not Avp V1a receptors to enhance social recognition in adult Syrian hamsters (Mesocricetus auratus). Horm Behav (2016) 81:20–7. doi:10.1016/j.yhbeh.2016.02.004
35. Hicks C, Ramos L, Dampney B, Baracz SJ, Mcgregor IS, Hunt GE. Regional c-Fos expression induced by peripheral oxytocin administration is prevented by the vasopressin 1A receptor antagonist SR49059. Brain Res Bull (2016) 127:208–18. doi:10.1016/j.brainresbull.2016.10.005
36. Porges SW. Love: an emergent property of the mammalian autonomic nervous system. Psychoneuroendocrinology (1998) 23:837–61. doi:10.1016/S0306-4530(98)00057-2
37. Cho MM, Devries AC, Williams JR, Carter CS. The effects of oxytocin and vasopressin on partner preferences in male and female prairie voles (Microtus ochrogaster). Behav Neurosci (1999) 113:1071–9. doi:10.1037/0735-7044.113.5.1071
38. Aragona BJ, Liu Y, Yu YJ, Curtis JT, Detwiler JM, Insel TR, et al. Nucleus accumbens dopamine differentially mediates the formation and maintenance of monogamous pair bonds. Nat Neurosci (2005) 9:133–9. doi:10.1038/nn1613
39. Gobrogge KL, Jia X, Liu Y, Wang Z. Neurochemical mediation of affiliation and aggression associated with pair-bonding. Biol Psychiatry (2017) 81:231–42. doi:10.1016/j.biopsych.2016.02.013
40. Hung LW, Neuner S, Polepalli JS, Beier KT, Wright M, Walsh JJ, et al. Gating of social reward by oxytocin in the ventral tegmental area. Science (2017) 357:1406–11. doi:10.1126/science.aan4994
41. Kenkel WM, Paredes J, Lewis GF, Yee JR, Pournajafi-Nazarloo H, Grippo AJ, et al. Autonomic substrates of the response to pups in male prairie voles. PLoS One (2013) 8:e69965. doi:10.1371/journal.pone.0069965
42. Yee JR, Kenkel WM, Frijling JL, Dodhia S, Onishi KG, Tovar S, et al. Oxytocin promotes functional coupling between paraventricular nucleus and both sympathetic and parasympathetic cardioregulatory nuclei. Horm Behav (2016) 80:82–91. doi:10.1016/j.yhbeh.2016.01.010
43. Bernaerts S, Prinsen J, Berra E, Bosmans G, Steyaert J, Alaerts K. Long-term oxytocin administration enhances the experience of attachment. Psychoneuroendocrinology (2017) 78:1–9. doi:10.1016/j.psyneuen.2017.01.010
44. Bethlehem RA, Baron-Cohen S, Van Honk J, Auyeung B, Bos PA. The oxytocin paradox. Front Behav Neurosci (2014) 8:48. doi:10.3389/fnbeh.2014.00048
45. De Dreu CKW. Oxytocin modulates cooperation within and competition between groups: an integrative review and research agenda. Horm Behav (2012) 61:419–28. doi:10.1016/j.yhbeh.2011.12.009
46. Feng C, Hackett PD, Demarco AC, Chen X, Stair S, Haroon E, et al. Oxytocin and vasopressin effects on the neural response to social cooperation are modulated by sex in humans. Brain Imaging Behav (2015) 9:754–64. doi:10.1007/s11682-014-9333-9
47. Li T, Chen X, Mascaro J, Haroon E, Rilling JK. Intranasal oxytocin, but not vasopressin, augments neural responses to toddlers in human fathers. Horm Behav (2017) 93:193–202. doi:10.1016/j.yhbeh.2017.01.006
48. Gainer H. Cell-type specific expression of oxytocin and vasopressin genes: an experimental odyssey. J Neuroendocrinol (2012) 24:528–38. doi:10.1111/j.1365-2826.2011.02236.x
49. MacLean EL, Gesquiere LR, Gee N, Levy K, Martin WL, Carter CS. Validation of salivary oxytocin and vasopressin as biomarkers in domestic dogs. J Neurosci Methods (2017) 293:67–76. doi:10.1016/j.jneumeth.2017.08.033
50. Grinevich V, Knobloch-Bollmann HS, Eliava M, Busnelli M, Chini B. Assembling the puzzle: pathways of oxytocin signaling in the brain. Biol Psychiatry (2016) 79:155–64. doi:10.1016/j.biopsych.2015.04.013
51. Tabbaa M, Paedae B, Liu Y, Wang Z. Neuropeptide regulation of social attachment: the prairie vole model. Compr Physiol (2016) 7:81–104. doi:10.1002/cphy.c150055
52. Dumais KM, Veenema AH. Vasopressin and oxytocin receptor systems in the brain: sex differences and sex-specific regulation of social behavior. Front Neuroendocrinol (2016) 40:1–23. doi:10.1016/j.yfrne.2015.04.003
53. Geenen V. Thymus-dependent T cell tolerance of neuroendocrine functions: principles, reflections, and implications for tolerogenic/negative self-vaccination. Ann N Y Acad Sci (2006) 1088:284–96. doi:10.1196/annals.1366.009
54. Zingg HH, Laporte SA. The oxytocin receptor. Trends Endocrinol Metab (2003) 14:222–7. doi:10.1016/S1043-2760(03)00080-8
55. de Vries GJ. Sex differences in vasopressin and oxytocin innervation of the brain. Prog Brain Res (2008) 170:17–27. doi:10.1016/S0079-6123(08)00402-0
56. Dantzer R. Vasopressin, gonadal steroids and social recognition. Prog Brain Res (1998) 119:409–14. doi:10.1016/S0079-6123(08)61584-8
57. DiBenedictis BT, Nussbaum ER, Cheung HK, Veenema AH. Quantitative mapping reveals age and sex differences in vasopressin, but not oxytocin, immunoreactivity in the rat social behavior neural network. J Comp Neurol (2017) 525:2549–70. doi:10.1002/cne.24216
58. Vaccari C, Lolait SJ, Ostrowski NL. Comparative distribution of vasopressin V1b and oxytocin receptor messenger ribonucleic acids in brain. Endocrinology (1998) 139:5015–33. doi:10.1210/endo.139.12.6382
59. Stevenson EL, Caldwell HK. The vasopressin 1b receptor and the neural regulation of social behavior. Horm Behav (2012) 61:277–82. doi:10.1016/j.yhbeh.2011.11.009
60. Akerlund M. Targeting the oxytocin receptor to relax the myometrium. Expert Opin Ther Targets (2006) 10:423–7. doi:10.1517/14728222.10.3.423
61. Busnelli M, Chini B. Molecular basis of oxytocin receptor signalling in the brain: what we know and what we need to know. Curr Top Behav Neurosci (2017). doi:10.1007/7854_2017_6
62. Freeman SM, Young LJ. Comparative perspectives on oxytocin and vasopressin receptor research in rodents and primates: translational implications. J Neuroendocrinol (2016) 28. doi:10.1111/jne.12382
63. Seelke AM, Perkeybile AM, Grunewald R, Bales KL, Krubitzer LA. Individual differences in cortical connections of somatosensory cortex are associated with parental rearing style in prairie voles (Microtus ochrogaster). J Comp Neurol (2016) 524:564–77. doi:10.1002/cne.23837
64. Seelke AM, Yuan SM, Perkeybile AM, Krubitzer LA, Bales KL. Early experiences can alter the size of cortical fields in prairie voles (Microtus ochrogaster). Environ Epigenet (2016) 2(3):ii:dvw019.
65. Duchemin A, Seelke AM, Simmons TC, Freeman SM, Bales KL. Localization of oxytocin receptors in the prairie vole (Microtus ochrogaster) neocortex. Neuroscience (2017) 348:201–11. doi:10.1016/j.neuroscience.2017.02.017
66. Grunewald R, Seelke AM, Perkeybile AM, Cooke DF, Bales KL, Krubitzer L. Effects of early parenting experience on cortical connections in prairie voles (Microtus ochrogaster). Soc Neurosci (2012).
67. Tribollet E, Barberis C, Arsenijevic Y. Distribution of vasopressin and oxytocin receptors in the rat spinal cord: sex-related differences and effect of castration in pudendal motor nuclei. Neuroscience (1997) 78(2):499–509. doi:10.1016/S0306-4522(96)00591-X
68. Poisbeau P, Grinevich V, Charlet A. Oxytocin signaling in pain: cellular, circuit, system, and behavioral levels. Curr Top Behav Neurosci (2017). doi:10.1007/7854_2017_14
69. Witt DM, Carter CS, Insel TR. Oxytocin receptor binding in female prairie voles: endogenous and exogenous oestradiol stimulation. J Neuroendocrinol (1991) 3:155–61. doi:10.1111/j.1365-2826.1991.tb00258.x
70. Hammock EA, Young LJ. Microsatellite instability generates diversity in brain and sociobehavioral traits. Science (2005) 308:1630–4. doi:10.1126/science.1111427
71. Carter CS. Sex differences in oxytocin and vasopressin: implications for autism spectrum disorders? Behav Brain Res (2007) 176:170–86. doi:10.1016/j.bbr.2006.08.025
72. Johnson ZV, Young LJ. Oxytocin and vasopressin neural networks: implications for social behavioral diversity and translational neuroscience. Neurosci Biobehav Rev (2017) 76:87–98. doi:10.1016/j.neubiorev.2017.01.034
73. Li T, Wang P, Wang SC, Wang YF. Approaches mediating oxytocin regulation of the immune system. Front Immunol (2016) 7:693. doi:10.3389/fimmu.2016.00693
74. Rotondo F, Butz H, Syro LV, Yousef GM, Di Ieva A, Restrepo LM, et al. Arginine vasopressin (AVP): a review of its historical perspectives, current research and multifunctional role in the hypothalamo-hypophysial system. Pituitary (2016) 19:345–55. doi:10.1007/s11102-015-0703-0
75. Murgatroyd CA, Hicks-Nelson A, Fink A, Beamer G, Gurel K, Elnady F, et al. Effects of chronic social stress and maternal intranasal oxytocin and vasopressin on offspring interferon-gamma and behavior. Front Endocrinol (2016) 7:155. doi:10.3389/fendo.2016.00155
76. Gimpl G, Fahrenholz F. The oxytocin receptor system: structure, function, and regulation. Physiol Rev (2001) 81:629–83.
77. Arrowsmith S, Wray S. Oxytocin: its mechanism of action and receptor signalling in the myometrium. J Neuroendocrinol (2014) 26:356–69. doi:10.1111/jne.12154
78. Herman JP, Tasker JG. Paraventricular hypothalamic mechanisms of chronic stress adaptation. Front Endocrinol (2016) 7:137. doi:10.3389/fendo.2016.00137
79. Song Z, Mccann KE, Mcneill JKT, Larkin TE II, Huhman KL, Albers HE. Oxytocin induces social communication by activating arginine-vasopressin V1a receptors and not oxytocin receptors. Psychoneuroendocrinology (2014) 50:14–9. doi:10.1016/j.psyneuen.2014.08.005
80. Song Z, Borland JM, Larkin TE, O’Malley M, Albers HE. Activation of oxytocin receptors, but not arginine-vasopressin V1a receptors, in the ventral tegmental area of male Syrian hamsters is essential for the reward-like properties of social interactions. Psychoneuroendocrinology (2016) 74:164–72. doi:10.1016/j.psyneuen.2016.09.001
81. Caldwell HK, Aulino EA, Freeman AR, Miller TV, Witchey SK. Oxytocin and behavior: lessons from knockout mice. Dev Neurobiol (2017) 77:190–201. doi:10.1002/dneu.22431
82. Xiao L, Priest MF, Nasenbeny J, Lu T, Kozorovitskiy Y. Biased oxytocinergic modulation of midbrain dopamine systems. Neuron (2017) 95:368–84.e5. doi:10.1016/j.neuron.2017.06.003
83. Nishimori K, Young LJ, Guo Q, Wang Z, Insel TR, Matzuk MM. Oxytocin is required for nursing but is not essential for parturition or reproductive behavior. Proc Natl Acad Sci U S A (1996) 93:11699–704. doi:10.1073/pnas.93.21.11699
84. Young WS III, Shepard E, Amico J, Hennighausen L, Wagner KU, Lamarca ME, et al. Deficiency in mouse oxytocin prevents milk ejection, but not fertility or parturition. J Neuroendocrinol (1996) 8:847–53. doi:10.1046/j.1365-2826.1996.05266.x
85. Sala M, Braida D, Lentini D, Busnelli M, Bulgheroni E, Capurro V, et al. Pharmacologic rescue of impaired cognitive flexibility, social deficits, increased aggression, and seizure susceptibility in oxytocin receptor null mice: a neurobehavioral model of autism. Biol Psychiatry (2011) 69:875–82. doi:10.1016/j.biopsych.2010.12.022
86. Huang H, Michetti C, Busnelli M, Manago F, Sannino S, Scheggia D, et al. Chronic and acute intranasal oxytocin produce divergent social effects in mice. Neuropsychopharmacology (2014) 39:1102–14. doi:10.1038/npp.2013.310
87. DeVries AC, Guptaa T, Cardillo S, Cho M, Carter CS. Corticotropin-releasing factor induces social preferences in male prairie voles. Psychoneuroendocrinology (2002) 27:705–14. doi:10.1016/S0306-4530(01)00073-7
88. Ben-Ari Y. Is birth a critical period in the pathogenesis of autism spectrum disorders? Nat Rev Neurosci (2015) 16:498–505. doi:10.1038/nrn3956
89. Arai A, Hirota Y, Miyase N, Miyata S, Young LJ, Osako Y, et al. A single prolonged stress paradigm produces enduring impairments in social bonding in monogamous prairie voles. Behav Brain Res (2016) 315:83–93. doi:10.1016/j.bbr.2016.08.022
90. Terranova JI, Song Z, Larkin TE II, Hardcastle N, Norvelle A, Riaz A, et al. Serotonin and arginine-vasopressin mediate sex differences in the regulation of dominance and aggression by the social brain. Proc Natl Acad Sci U S A (2016) 113:13233–8. doi:10.1073/pnas.1610446113
91. Kenkel WM, Yee JR, Carter CS. Is oxytocin a maternal-foetal signalling molecule at birth? Implications for development. J Neuroendocrinol (2014) 26:739–49. doi:10.1111/jne.12186
92. Lee HJ, Caldwell HK, Macbeth AH, Young WS III. Behavioural studies using temporal and spatial inactivation of the oxytocin receptor. Prog Brain Res (2008) 170:73–7. doi:10.1016/S0079-6123(08)00407-X
93. Pedersen CA, Prange AJ Jr. Induction of maternal behavior in virgin rats after intracerebroventricular administration of oxytocin. Proc Natl Acad Sci U S A (1979) 76:6661–5. doi:10.1073/pnas.76.12.6661
94. Keverne EB, Kendrick KM. Oxytocin facilitation of maternal behavior in sheep. Ann N Y Acad Sci (1992) 652:83–101. doi:10.1111/j.1749-6632.1992.tb34348.x
95. Bridges RS. Neuroendocrine regulation of maternal behavior. Front Neuroendocrinol (2015) 36:178–96. doi:10.1016/j.yfrne.2014.11.007
96. Fahrbach SE, Morrell JI, Pfaff DW. Effect of varying the duration of pre-test cage habituation on oxytocin induction of short-latency maternal behavior. Physiol Behav (1986) 37:135–9. doi:10.1016/0031-9384(86)90396-3
97. Fahrbach SE, Morrell JI, Pfaff DW. Possible role for endogenous oxytocin in estrogen-facilitated maternal behavior in rats. Neuroendocrinology (1985) 40:526–32. doi:10.1159/000124125
98. Bosch OJ, Neumann ID. Both oxytocin and vasopressin are mediators of maternal care and aggression in rodents: from central release to sites of action. Horm Behav (2012) 61:293–303. doi:10.1016/j.yhbeh.2011.11.002
99. Bayerl DS, Klampfl SM, Bosch OJ. Central V1b receptor antagonism in lactating rats: impairment of maternal care but not of maternal aggression. J Neuroendocrinol (2014) 26:918–26. doi:10.1111/jne.12226
100. Kenkel WM, Paredes J, Yee JR, Pournajafi-Nazarloo H, Bales KL, Carter CS. Neuroendocrine and behavioural responses to exposure to an infant in male prairie voles. J Neuroendocrinol (2012) 24:874–86. doi:10.1111/j.1365-2826.2012.02301.x
101. Albers HE. The regulation of social recognition, social communication and aggression: vasopressin in the social behavior neural network. Horm Behav (2012) 61:283–92. doi:10.1016/j.yhbeh.2011.10.007
102. Ferris CF, Potegal M. Vasopressin receptor blockade in the anterior hypothalamus suppresses aggression in hamsters. Physiol Behav (1988) 44:235–9. doi:10.1016/0031-9384(88)90144-8
103. Ferris CF. Functional magnetic resonance imaging and the neurobiology of vasopressin and oxytocin. Prog Brain Res (2008) 170:305–20. doi:10.1016/S0079-6123(08)00425-1
104. Frank E, Landgraf R. The vasopressin system – from antidiuresis to psychopathology. Eur J Pharmacol (2008) 583:226–42. doi:10.1016/j.ejphar.2007.11.063
105. Galbusera A, De Felice A, Girardi S, Bassetto G, Maschietto M, Nishimori K, et al. Intranasal oxytocin and vasopressin modulate divergent brainwide functional substrates. Neuropsychopharmacology (2017) 42:1420–34. doi:10.1038/npp.2016.283
106. Wentworth BA, Stein MB, Redwine LS, Xue Y, Taub PR, Clopton P, et al. Post-traumatic stress disorder: a fast track to premature cardiovascular disease? Cardiol Rev (2013) 21:16–22. doi:10.1097/CRD.0b013e318265343b
107. Lee R, Ferris C, Van De Kar LD, Coccaro EF. Cerebrospinal fluid oxytocin, life history of aggression, and personality disorder. Psychoneuroendocrinology (2009) 34:1567–73. doi:10.1016/j.psyneuen.2009.06.002
108. Carter CS, Devries AC, Getz LL. Physiological substrates of mammalian monogamy: the prairie vole model. Neurosci Biobehav Rev (1995) 19:303–14. doi:10.1016/0149-7634(94)00070-H
109. Winslow JT, Hastings N, Carter CS, Harbaugh CR, Insel TR. A role for central vasopressin in pair bonding in monogamous prairie voles. Nature (1993) 365:545–8. doi:10.1038/365545a0
110. Beery AK, Kaufer D. Stress, social behavior, and resilience: Insights from rodents. Neurobiol Stress (2015) 1:116–27. doi:10.1016/j.ynstr.2014.10.004
111. Feldman R. The neurobiology of human attachments. Trends Cogn Sci (2017) 21:80–99. doi:10.1016/j.tics.2016.11.007
112. Dantzer R, Bluthe RM, Koob GF, Le Moal M. Modulation of social memory in male rats by neurohypophyseal peptides. Psychopharmacology (Berl) (1987) 91:363–8. doi:10.1007/BF00518192
113. Ramos L, Hicks C, Kevin R, Caminer A, Narlawar R, Kassiou M, et al. Acute prosocial effects of oxytocin and vasopressin when given alone or in combination with 3,4-methylenedioxymethamphetamine in rats: involvement of the V1A receptor. Neuropsychopharmacology (2013) 38:2249–59. doi:10.1038/npp.2013.125
114. Bowen MT, McGregor IS. Oxytocin and vasopressin modulate the social response to threat: a preclinical study. Int J Neuropsychopharmacol (2014) 17:1621–33. doi:10.1017/S1461145714000388
115. Witt DM, Carter CS, Walton DM. Central and peripheral effects of oxytocin administration in prairie voles (Microtus ochrogaster). Pharmacol Biochem Behav (1990) 37:63–9. doi:10.1016/0091-3057(90)90042-G
116. Williams JR, Insel TR, Harbaugh CR, Carter CS. Oxytocin administered centrally facilitates formation of a partner preference in female prairie voles (Microtus ochrogaster). J Neuroendocrinol (1994) 6:247–50. doi:10.1111/j.1365-2826.1994.tb00579.x
117. Burkett JP, Spiegel LL, Inoue K, Murphy AZ, Young LJ. Activation of μ-opioid receptors in the dorsal striatum is necessary for adult social attachment in monogamous prairie voles. Neuropsychopharmacology (2011) 36(11):2200–10. doi:10.1038/npp.2011.117
118. De Wied D. Long term effect of vasopressin on the maintenance of a conditioned avoidance response in rats. Nature (1971) 232:58–60. doi:10.1038/232058a0
119. Guzman YF, Tronson NC, Jovasevic V, Sato K, Guedea AL, Mizukami H, et al. Fear-enhancing effects of septal oxytocin receptors. Nat Neurosci (2013) 16:1185–7. doi:10.1038/nn.3465
120. Guzman YF, Tronson NC, Sato K, Mesic I, Guedea AL, Nishimori K, et al. Role of oxytocin receptors in modulation of fear by social memory. Psychopharmacology (Berl) (2014) 231:2097–105. doi:10.1007/s00213-013-3356-6
121. Moaddab M, Dabrowska J. Oxytocin receptor neurotransmission in the dorsolateral bed nucleus of the stria terminalis facilitates the acquisition of cued fear in the fear-potentiated startle paradigm in rats. Neuropharmacology (2017) 121:130–9. doi:10.1016/j.neuropharm.2017.04.039
122. Bales KL, Lewis-Reese AD, Pfeifer LA, Kramer KM, Carter CS. Early experience affects the traits of monogamy in a sexually dimorphic manner. Dev Psychobiol (2007) 49:335–42. doi:10.1002/dev.20216
123. Carter CS, Boone EM, Pournajafi-Nazarloo H, Bales KL. Consequences of early experiences and exposure to oxytocin and vasopressin are sexually dimorphic. Dev Neurosci (2009) 31:332–41. doi:10.1159/000216544
124. DeVries AC, Devries MB, Taymans SE, Carter CS. The effects of stress on social preferences are sexually dimorphic in prairie voles. Proc Natl Acad Sci U S A (1996) 93:11980–4. doi:10.1073/pnas.93.21.11980
125. Peters S, Slattery DA, Uschold-Schmidt N, Reber SO, Neumann ID. Dose-dependent effects of chronic central infusion of oxytocin on anxiety, oxytocin receptor binding and stress-related parameters in mice. Psychoneuroendocrinology (2014) 42:225–36. doi:10.1016/j.psyneuen.2014.01.021
126. Guastella AJ, MacLeod C. A critical review of the influence of oxytocin nasal spray on social cognition in humans: evidence and future directions. Horm Behav (2012) 61:410–8. doi:10.1016/j.yhbeh.2012.01.002
127. Neumann ID, Maloumby R, Beiderbeck DI, Lukas M, Landgraf R. Increased brain and plasma oxytocin after nasal and peripheral administration in rats and mice. Psychoneuroendocrinology (2013) 38:1985–93. doi:10.1016/j.psyneuen.2013.03.003
128. Ferris CF, Yee JR, Kenkel WM, Dumais KM, Moore K, Veenema AH, et al. Distinct BOLD activation profiles following central and peripheral oxytocin administration in awake rats. Front Behav Neurosci (2015) 9:245. doi:10.3389/fnbeh.2015.00245
129. Goldman MB, Gomes AM, Carter CS, Lee R. Divergent effects of two different doses of intranasal oxytocin on facial affect discrimination in schizophrenic patients with and without polydipsia. Psychopharmacology (Berl) (2011) 216:101–10. doi:10.1007/s00213-011-2193-8
130. Price D, Burris D, Cloutier A, Thompson CB, Rilling JK, Thompson RR. Dose-dependent and lasting influences of intranasal vasopressin on face processing in men. Front Endocrinol (2017) 8:220. doi:10.3389/fendo.2017.00220
131. Carter CS, DeVries AC, Getz LL. Physiological substrates of mammalian monogamy: the prairie vole model. Neurosci Biobehav Rev (1995) 19(2):303–14.
132. Grippo AJ, Gerena D, Huang J, Kumar N, Shah M, Ughreja R, et al. Social isolation induces behavioral and neuroendocrine disturbances relevant to depression in female and male prairie voles. Psychoneuroendocrinology (2007) 32:966–80. doi:10.1016/j.psyneuen.2007.07.004
133. Hernandez VS, Hernandez OR, De La Mora M, Gomora MJ, Fuxe K, Eiden LE, et al. Hypothalamic vasopressinergic projections innervate central amygdala GABAergic neurons: implications for anxiety and stress coping. Front Neural Circuits (2016) 10:92. doi:10.3389/fncir.2016.00092
134. Bartz J, Simeon D, Hamilton H, Kim S, Crystal S, Braun A, et al. Oxytocin can hinder trust and cooperation in borderline personality disorder. Soc Cogn Affect Neurosci (2011) 6:556–63. doi:10.1093/scan/nsq085
135. Bartz JA, Zaki J, Bolger N, Ochsner KN. Social effects of oxytocin in humans: context and person matter. Trends Cogn Sci (2011) 15:301–9. doi:10.1016/j.tics.2011.05.002
136. Ne’eman R, Perach-Barzilay N, Fischer-Shofty M, Atias A, Shamay-Tsoory SG. Intranasal administration of oxytocin increases human aggressive behavior. Horm Behav (2016) 80:125–31. doi:10.1016/j.yhbeh.2016.01.015
137. Bales KL, Solomon M, Jacob S, Crawley JN, Silverman JL, Larke RH, et al. Long-term exposure to intranasal oxytocin in a mouse autism model. Transl Psychiatry (2014) 4:e480. doi:10.1038/tp.2014.117
138. Bales KL, Perkeybile AM, Conley OG, Lee MH, Guoynes CD, Downing GM, et al. Chronic intranasal oxytocin causes long-term impairments in partner preference formation in male prairie voles. Biol Psychiatry (2013) 74:180–8. doi:10.1016/j.biopsych.2012.08.025
139. Seltzer LJ, Ziegler T, Connolly MJ, Prososki AR, Pollak SD. Stress-induced elevation of oxytocin in maltreated children: evolution, neurodevelopment, and social behavior. Child Dev (2014) 85:501–12. doi:10.1111/cdev.12136
140. Norman GJ, Cacioppo JT, Morris JS, Malarkey WB, Berntson GG, Devries AC. Oxytocin increases autonomic cardiac control: moderation by loneliness. Biol Psychol (2011) 86:174–80. doi:10.1016/j.biopsycho.2010.11.006
141. Guzmán YF, Tronson NC, Sato K, Mesic I, Guedea AL, Nishimori K, et al. Role of oxytocin receptors in modulation of fear by social memory. Psychopharmacology (Berl) (2014) 231(10):2097–105. doi:10.1007/s00213-013-3356-6
Keywords: oxytocin, vasopressin, oxytocin receptor, vasopressin receptor subtype 1a, love, attachment, prairie voles, aggression
Citation: Carter CS (2017) The Oxytocin–Vasopressin Pathway in the Context of Love and Fear. Front. Endocrinol. 8:356. doi: 10.3389/fendo.2017.00356
Received: 18 October 2017; Accepted: 07 December 2017;
Published: 22 December 2017
Edited by:
Heather K. Caldwell, Kent State University, United StatesReviewed by:
Benedetta Leuner, The Ohio State University, United StatesOliver J. Bosch, University of Regensburg, Germany
Ben Nephew, Tufts University Cummings School of Veterinary Medicine, United States
Copyright: © 2017 Carter. This is an open-access article distributed under the terms of the Creative Commons Attribution License (CC BY). The use, distribution or reproduction in other forums is permitted, provided the original author(s) or licensor are credited and that the original publication in this journal is cited, in accordance with accepted academic practice. No use, distribution or reproduction is permitted which does not comply with these terms.
*Correspondence: C. Sue Carter, cscarter@indiana.edu