- Department of Molecular Pharmacology, National Institute of Neuroscience, Kodaira, Tokyo, Japan
Accumulating evidence shows that H2S has physiological functions in various tissues and organs. It includes regulation of neuronal activity, vascular tension, a release of insulin, and protection of the heart, kidney, and brain from ischemic insult. H2S is produced by enzymes from l-cysteine; cystathionine β-synthase, cystathionine γ-lyase, and 3-mercaptopyruvate sulfurtransferase (3MST) along with cysteine aminotransferase. We recently discovered an additional pathway for the production of H2S from d-cysteine. d-Amino acid oxidase provides 3-mercaptopyruvate for 3MST to produce H2S. d-Cysteine protects cerebellar neurons from oxidative stress and attenuates ischemia-reperfusion injury caused in the kidney more effectively than l-cysteine. This review focuses on a novel pathway for the production of H2S and its therapeutic application especially to the renal diseases.
Introduction
The discovery of endogenous sulfide in the brain urged us to study the function of hydrogen sulfide (H2S) in the brain (1–3). The recent re-evaluation showed that the endogenous levels of H2S are much lower than those initially evaluated, but this finding confirmed the existence of sulfide in tissues (4–6).
H2S facilitates the induction of hippocampal long-term potentiation, a synaptic model of learning and memory, by enhancing the activity of n-methyl-d-aspartate (NMDA) receptors in neurons, and it induces Ca2+ waves in astrocytes (7, 8). It relaxes vascular smooth muscle by activating K+ channels, regulates the release of insulin and induces angiogenesis (9–14). It protects neurons from oxidative stress by enhancing the activity of glutathione synthesis, scavenging reactive oxygen species, and suppressing the excessive increase in the intracellular Ca2+ (15–17). In cardiovascular system, H2S protects cardiomyocytes from ischemia-reperfusion injury by preserving mitochondrial function (18). A similar protective effect was also observed in the kidney (19). H2S is produced from l-cysteine by two pyridoxal 5′-phosphate (PLP)-dependent enzymes, cystathionine β-synthase (CBS), and cystathionine γ-lyase (CSE) and PLP-independent 3-mercaptopyruvate sulfurtransferase (3MST) (Figure 1) (7, 9, 20–23). 3MST produces H2S from 3-mercaptopyruvate (3MP), an achiral α-keto acid, which is generated by PLP-dependent cysteine aminotransferase (CAT) from l-cysteine and α-ketoglutarate (α-KG) (24–26). Thioredoxin (Trx) and dihydrolipoic acid (DHLA) are endogenous reducing cofactors that facilitate H2S release from 3MST (23). We recently discovered a novel pathway with d-cysteine as a substrate (27).
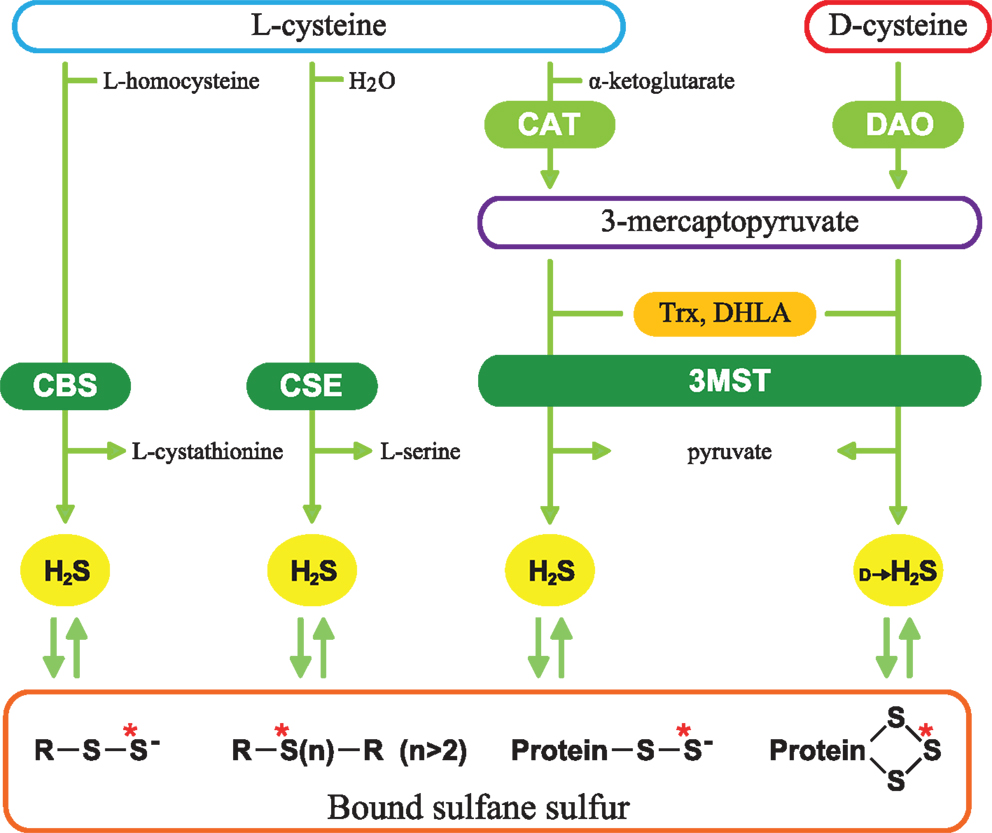
Figure 1. Schematic representation of H2S-producing pathways. Cystathionine β-synthase (CBS) catalyzes β-replacement of l-cysteine to produce H2S and l-cystathionine. Cystathionine γ-lyase (CSE) catalyzes the hydrolysis of l-cysteine. 3-Mercaptopyruvate sulfurtransferase (3MST) produces H2S from 3-mercaptopyruvate (3MP), which is generated by cysteine aminotransferase (CAT) and d-amino acid oxidase (DAO) from l-cysteine and d-cysteine, respectively. Thioredoxin (Trx) and dihydrolipoic acid (DHLA) are endogenous reducing cofactors that facilitate the release of H2S from 3MST. H2S is stored as bound sulfane sulfur, which is divalent sulfur bound only to other sulfur, such as outer sulfur atoms of persulfides and innerchain atoms of polysulfides. Red asterisks show bound sulfane sulfur.
Production of H2S from d-Cysteine
When we examined the production of H2S from brain homogenates, we found that H2S was produced from d-cysteine, originally used as a negative control for l-cysteine (27). H2S-producing pathway from d-cysteine is distinct from the pathways involving l-cysteine. There are critical differences between the two pathways; (i) the optimal pH, (ii) the dependency on PLP, and (iii) the stability against the freeze and thaw procedure. The production of H2S from d-cysteine is optimal at pH 7.4, whereas production from l-cysteine is maximal under the alkaline condition. H2S production from d-cysteine is PLP-independent, while that from l-cysteine is PLP-dependent. A single freeze-thaw cycle greatly increases the H2S production from d-cysteine. d-Amino acid oxidase (DAO) that produces 3MP from d-cysteine is localized to peroxisomes, while 3MST is mainly found in mitochondria (21, 28). Mitochondria and peroxisomes exchange various metabolites via a specific form of vesicular trafficking, and are usually in close proximity to each other or have physical contact (29). 3MST and DAO can produce H2S by the interaction of both organelles.
Localization of H2S-Producing Enzymes
Enzymes producing H2S from l-cysteine are expressed in many tissues (7, 9, 17, 20, 21, 23, 30, 31). 3MST is found in neurons in the cerebral cortex, cerebellum, olfactory bulb, pons, and retina, while CBS is preferentially expressed in cerebellar Bergmann glia and in astrocytes throughout the brain (21, 32). CSE activity in the brain is only 1% of the hepatic activity (33). CBS, CSE and 3MST, and CAT are expressed in the liver and kidney (20). Vascular endothelium co-expresses 3MST and CAT (31). The localization of CSE in vascular endothelium is controversial (31, 34). Unlike the l-cysteine pathways, the d-cysteine pathway operates predominantly in the cerebellum and the kidney (27, 35). In the cerebellum, DAO is expressed in astrocytes, Bergmann glia, and several types of neurons including the Golgi and Purkinje cells (35, 36). In the kidney, DAO and 3MST are expressed in the proximal convoluted tubules of the cortex similarly to CBS and CSE (30, 37–39).
Regulation of H2S-Producing Enzymes by Ca2+
3MST/CAT is regulated by Ca2+; the activity is maximal in the absence of Ca2+ and is completely suppressed at 2.9 μM Ca2+ (17). A similar regulation by Ca2+ is observed in CSE activity (40). H2S is produced by CSE at the steady-state low Ca2+ concentrations and that the production is suppressed by increased Ca2+ (40). Calmodulin is not involved in the regulation of CSE activity. It was previously reported that CSE activity is regulated by Ca2+/calmodulin in the presence of 1–2 mM Ca2+ (34). Because the intracellular Ca2+ concentrations are between 100 nM and 3 μM in endothelium, Ca2+ concentrations used in the previous study are not in the physiological range (41).
Source of d-Cysteine
Relatively large amounts of d-serine are found in mammalian tissues, and the content of d-serine is up to 15 ∼ 30% of the l-form in the brain (42, 43). d-Serine is thought to be produced by PLP-dependent serine racemase, but the Michaelis-constant value of serine racemase is higher than the endogenous levels of l-serine (42, 44–46). Although cysteine is structurally similar to serine with an OH replaced by an SH, serine racemase does not change l-cysteine to d-cysteine (27). Aspartate racemase is homologous to CAT and has an affinity for both aspartate and cysteine (24, 47), but does not produce d-cysteine.
A possible source of d-cysteine is absorption from food. l-Amino acids are non-enzymatically racemized by heat and alkaline treatment applied during food processing. l-Cysteine is one of the fastest racemizing amino acid, and 21–44% of l-cysteine is changed to d-cysteine by alkaline treatment (48, 49). Although d-cysteine is easily absorbed through the gastrointestinal tract and enters the blood stream (50), d-cysteine is not detected either in the cerebellum or the kidney after the oral administration. Considering the fact that the levels of bound sulfane sulfur, a storage form of H2S (Figure 1), are increased after oral administration of d-cysteine (5, 27), d-cysteine may be immediately metabolized to produce bound sulfane sulfur in tissues.
Cytoprotective Effect of d-Cysteine
The most characteristic feature of the d-cysteine pathway is the greater H2S-producing activity in the cerebellum and the kidney compared to the l-cysteine pathway; 7- and 80-fold greater in the cerebellum and the kidney, respectively. Although both d-cysteine and l-cysteine protect cerebellar neurons from hydrogen peroxide-induced oxidative stress (27), d-cysteine protected neurons more greatly than l-cysteine, probably because the transport activity for d-cysteine is greater than that for l-cysteine (51). d-Cysteine may have a potential to improve the developmental neuronal diseases in the cerebellum like autism in which oxidative stress may be involved (52, 53).
Ischemia-reperfusion injury is observed after cardiovascular surgery, transplantation, or septic as well as hemorrhagic shock. Renal ischemia-reperfusion injury reduces the filtering capacity of the glomerulus and causes acute renal failure (54). Endothelin antagonists, atrial natriuretic peptides, prostaglandins, nitric oxide inhibitors, thyroxine, and human insulin-like growth factor 1 have been studied for the prophylaxis and treatment of acute tubular necrosis without clinical benefit (55–58). We found that the oral administration of d-cysteine attenuates renal ischemia-reperfusion injury (27). The structure of glomeruli, which is disintegrated after ischemia-reperfusion, is well preserved by d-cysteine. In contrast, the glomeruli are shrunk and a wide space is observed between glomerulus and the surrounding capsule after ischemia-reperfusion when l-cysteine is applied. d-Cysteine increases the levels of bound sulfane sulfur and protects the renal cortex from the ischemia-reperfusion injury more efficiently than l-cysteine.
d-Cysteine: Its Therapeutic Potential
l-Cysteine is metabolized to produce (i) cysteinyl-tRNA by cysteinyl-tRNA synthetase (59), (ii) γ-glutamylcysteine, a precursor of glutathione, by γ-glutamylcysteine synthetase, (iii) taurine or pyruvate by cysteine dioxygenase (60), and (iv) propionyl CoA by α-keto acid dehydrogenase. Because d-cysteine is not metabolized by these enzymes, d-cysteine must efficiently be utilized to produce H2S in the cerebellum and the kidney.
l-Cysteine is an excitotoxin comparable in potency to other excitatory amino acids and increases the blood pressure and heart rate (61, 62). In contrast, d-cysteine neither causes excitotoxic damage to the brain nor disturbs heart function (63, 64). Therefore, d-cysteine can be systemically and repeatedly applied with less toxicity compared to l-cysteine. The administration of d-cysteine may provide a new therapeutic approach to protect specific tissues from oxidative stress or ischemia-reperfusion injury through its conversion to H2S via a novel pathway with 3MST and DAO.
Conflict of Interest Statement
The authors declare that the research was conducted in the absence of any commercial or financial relationships that could be construed as a potential conflict of interest.
Acknowledgments
This work was supported by a grant from the National Institute of Neuroscience, a KAKENHI (23659089) Grant-in-Aid for Challenging Exploratory Research to Hideo Kimura, a KAKENHI (23700434) Grant-in-Aid for Young Scientists (B), a Health Labour Sciences Research Grant from the Ministry of Health Labour and Welfare to Norihiro Shibuya.
References
1. Goodwin LR, Francom D, Dieken FP, Taylor JD, Warenycia MW, Reiffenstein RJ, et al. Determination of sulfide in brain tissue by gas dialysis/ion chromatography: postmortem studies and two case reports. J Anal Toxicol (1989) 13:105–9.
2. Warenycia MW, Goodwin LR, Benishin CG, Reiffenstein RJ, Francom DM, Taylor JD, et al. Acute hydrogen sulfide poisoning. Demonstration of selective uptake of sulfide by the brainstem by measurement of brain sulfide levels. Biochem Pharmacol (1989) 38:973–81.
3. Savage JC, Gould DH. Determination of sulfide in brain tissue and rumen fluid by ion-interaction reversed-phase high-performance liquid chromatography. J Chromatogr (1990) 526:540–5.
4. Furne J, Saeed A, Levitt MD. Whole tissue hydrogen sulfide concentrations are orders of magnitude lower than presently accepted values. Am J Physiol Regul Integr Comp Physiol (2008) 295:R1479–85.
5. Ishigami M, Hiraki K, Umemura K, Ogasawara Y, Ishii K, Kimura H. A source of hydrogen sulfide and a mechanism of its release in the brain. Antioxid Redox Signal (2009) 11:205–14.
6. Wintner EA, Deckwerth TL, Langston W, Bengtsson A, Leviten D, Hill P, et al. A monobromobimane-based assay to measure the pharmacokinetic profile of reactive sulphide species in blood. Br J Pharmacol (2010) 160:941–57.
7. Abe K, Kimura H. The possible role of hydrogen sulfide as an endogenous neuromodulator. J Neurosci (1996) 16:1066–71.
8. Nagai Y, Tsugane M, Oka J, Kimura H. Hydrogen sulfide induces calcium waves in astrocytes. FASEB J (2004) 18:557–9.
9. Hosoki R, Matsuki N, Kimura H. The possible role of hydrogen sulfide as an endogenous smooth muscle relaxant in synergy with nitric oxide. Biochem Biophys Res Commun (1997) 237:527–31.
10. Zhao W, Zhang J, Lu Y, Wang R. The vasorelaxant effect of H2S as a novel endogenous gaseous KATP channel opener. EMBO J (2001) 20:6008–16.
11. Yang W, Yang G, Jia X, Wu L, Wang R. Activation of KATP channels by H2S in rat insulin-secreting cells and the underlying mechanisms. J Physiol (2005) 569:519–31.
12. Kaneko Y, Kimura Y, Kimura H, Niki I. l-Cysteine inhibits insulin release from the pancreatic beta-cell: possible involvement of metabolic production of hydrogen sulfide, a novel gasotransmitter. Diabetes (2006) 55:1391–7.
13. Papapetropoulos A, Pyriochou A, Altaany Z, Yang G, Marazioti A, Zhou Z, et al. Hydrogen sulfide is an endogenous stimulator of angiogenesis. Proc Natl Acad Sci U S A (2009) 106:21972–7.
14. Mustafa AK, Sikka G, Gazi SK, Steppan J, Jung SM, Bhunia AK, et al. Hydrogen sulfide as endothelium-derived hyperpolarizing factor sulfhydrates potassium channels. Circ Res (2011) 109:1259–68.
15. Kimura Y, Kimura H. Hydrogen sulfide protects neurons from oxidative stress. FASEB J (2004) 18:1165–7.
16. Kimura Y, Goto Y, Kimura H. Hydrogen sulfide increases glutathione production and suppresses oxidative stress in mitochondria. Antioxid Redox Signal (2010) 12:1–13.
17. Mikami Y, Shibuya N, Kimura Y, Nagahara N, Yamada M, Kimura H. Hydrogen sulfide protects the retina from light-induced degeneration by the modulation of Ca2+ influx. J Biol Chem (2011) 286:39379–86.
18. Elrod JW, Calvert JW, Morrison J, Doeller JE, Kraus DW, Tao L, et al. Hydrogen sulfide attenuates myocardial ischemia-reperfusion injury by preservation of mitochondrial function. Proc Natl Acad Sci U S A (2007) 104:15560–5.
19. Tripatara P, Patel NS, Collino M, Gallicchio M, Kieswich J, Castiglia S, et al. Generation of endogenous hydrogen sulfide by cystathionine gamma-lyase limits renal ischemia/reperfusion injury and dysfunction. Lab Invest (2008) 88:1038–48.
20. Stipanuk MH, Beck PW. Characterization of the enzymic capacity for cysteine desulphhydration in liver and kidney of the rat. Biochem J (1982) 206:267–77.
21. Shibuya N, Tanaka M, Yoshida M, Ogasawara Y, Togawa T, Ishii K, et al. 3-Mercaptopyruvate sulfurtransferase produces hydrogen sulfide and bound sulfane sulfur in the brain. Antioxid Redox Signal (2009) 11:703–14.
22. Singh S, Padovani D, Leslie RA, Chiku T, Banerjee R. Relative contributions of cystathionine beta-synthase and gamma-cystathionase to H2S biogenesis via alternative trans-sulfuration reactions. J Biol Chem (2009) 284:22457–66.
23. Mikami Y, Shibuya N, Kimura Y, Nagahara N, Ogasawara Y, Kimura H. Thioredoxin and dihydrolipoic acid are required for 3-mercaptopyruvate sulfurtransferase to produce hydrogen sulfide. Biochem J (2011) 439:479–85.
24. Ubuka T, Umemura S, Yuasa S, Kinuta M, Watanabe K. Purification and characterization of mitochondrial cysteine aminotransferase from rat liver. Physiol Chem Phys (1978) 10:483–500.
25. Akagi R. Purification and characterization of cysteine aminotransferase from rat liver cytosol. Acta Med Okayama (1982) 36:187–97.
27. Shibuya N, Koike S, Tanaka M, Ishigami-Yuasa M, Kimura Y, Ogasawara Y, et al. A novel pathway for the production of hydrogen sulfide from d-cysteine in mammalian cells. Nat Commun (2013) 4::1366.
28. Gould SJ, Keller GA, Subramani S. Identification of peroxisomal targeting signals located at the carboxy terminus of four peroxisomal proteins. J Cell Biol (1988) 107:897–905.
29. Schumann U, Subramani S. Special delivery from mitochondria to peroxisomes. Trends Cell Biol (2008) 18:253–6.
30. Ishii I, Akahoshi N, Yu XN, Kobayashi Y, Namekata K, Komaki G, et al. Murine cystathionine gamma-lyase: complete cDNA and genomic sequences, promoter activity, tissue distribution and developmental expression. Biochem J (2004) 381:113–23.
31. Shibuya N, Mikami Y, Kimura Y, Nagahara N, Kimura H. Vascular endothelium expresses 3-mercaptopyruvate sulfurtransferase and produces hydrogen sulfide. J Biochem (2009) 146:623–6.
32. Enokido Y, Suzuki E, Iwasawa K, Namekata K, Okazawa H, Kimura H. Cystathionine beta-synthase, a key enzyme for homocysteine metabolism, is preferentially expressed in the radial glia/astrocyte lineage of developing mouse CNS. FASEB J (2005) 19:1854–6.
33. Diwakar L, Ravindranath V. Inhibition of cystathionine-gamma-lyase leads to loss of glutathione and aggravation of mitochondrial dysfunction mediated by excitatory amino acid in the CNS. Neurochem Int (2007) 50:418–26.
34. Yang G, Wu L, Jiang B, Yang W, Qi J, Cao K, et al. H2S as a physiologic vasorelaxant: hypertension in mice with deletion of cystathionine gamma-lyase. Science (2008) 322:587–90.
35. Mitchell J, Paul P, Chen HJ, Morris A, Payling M, Falchi M, et al. Familial amyotrophic lateral sclerosis is associated with a mutation in d-amino acid oxidase. Proc Natl Acad Sci U S A (2010) 107:7556–61.
36. Moreno S, Nardacci R, Cimini A, Cerù MP. Immunocytochemical localization of d-amino acid oxidase in rat brain. J Neurocytol (1999) 28:169–85.
37. Perotti ME, Gavazzi E, Trussardo L, Malgaretti N, Curti B. Immunoelectron microscopic localization of d-amino acid oxidase in rat kidney and liver. Histochem J (1987) 19:157–69.
38. House JD, Brosnan ME, Brosnan JT. Characterization of homocysteine metabolism in the rat kidney. Biochem J (1997) 328:287–92.
39. Nagahara N, Ito T, Kitamura H, Nishino T. Tissue and subcellular distribution of mercaptopyruvate sulfurtransferase in the rat: confocal laser fluorescence and immunoelectron microscopic studies combined with biochemical analysis. Histochem Cell Biol (1998) 110:243–50.
40. Mikami Y, Shibuya N, Ogasawara Y, Kimura H. Hydrogen sulfide is produced by cystathionine γ-lyase at the steady-state low intracellular Ca2+ concentrations. Biochem Biophys Res Commun (2013) 431:131–5.
41. Rutter GA, Rizzuto R. Regulation of mitochondrial metabolism by ER Ca2+ release: an intimate connection. Trends Biochem Sci (2000) 25:215–21.
42. Mothet JP, Parent AT, Wolosker H, Brady RO Jr, Linden DJ, Ferris CD, et al. d-serine is an endogenous ligand for the glycine site of the N-methyl-d-aspartate receptor. Proc Natl Acad Sci U S A (2000) 97:4926–31.
43. Hamase K, Konno R, Morikawa A, Zaitsu K. Sensitive determination of d-amino acids in mammals and the effect of d-amino-acid oxidase activity on their amounts. Biol Pharm Bull (2005) 28:1578–84.
44. Wolosker H, Sheth KN, Takahashi M, Mothet JP, Brady RO Jr, Ferris CD, et al. Purification of serine racemase: biosynthesis of the neuromodulator d-serine. Proc Natl Acad Sci U S A (1999) 96:721–5.
45. Strísovský K, Jirásková J, Barinka C, Majer P, Rojas C, Slusher BS, et al. Mouse brain serine racemase catalyzes specific elimination of l-serine to pyruvate. FEBS Lett (2003) 535:44–8.
46. Miyoshi Y, Konno R, Sasabe J, Ueno K, Tojo Y, Mita M, et al. Alteration of intrinsic amounts of d-serine in the mice lacking serine racemase and d-amino acid oxidase. Amino Acids (2012) 43:1919–31.
47. Kim PM, Duan X, Huang AS, Liu CY, Ming GL, Song H, et al. Aspartate racemase, generating neuronal d-aspartate, regulates adult neurogenesis. Proc Natl Acad Sci U S A (2010) 107:3175–9.
48. Liardon R, Ledermann S. Racemization kinetics of free and protein-bound amino acids under moderate alkaline treatment. J Agric Food Chem (1986) 34:557–65.
49. Friedman M. Origin, microbiology, nutrition, and pharmacology of d-amino acids. Chem Biodivers (2010) 7:1491–530.
50. Krijgsheld KR, Glazenburg EJ, Scholtens E, Mulder GJ. The oxidation of l- and d-cysteine to inorganic sulfate and taurine in the rat. Biochim Biophys Acta (1981) 677:7–12.
51. Fukasawa Y, Segawa H, Kim JY, Chairoungdua A, Kim DK, Matsuo H, et al. Identification and characterization of a Na+-independent neutral amino acid transporter that associates with the 4F2 heavy chain and exhibits substrate selectivity for small neutral d- and l-amino acids. J Biol Chem (2000) 275:9690–8.
52. Fonnum F, Lock EA. Cerebellum as a target for toxic substances. Toxicol Lett (2000) 112–113:9–16.
53. Sajdel-Sulkowska EM, Xu M, Koibuchi N. Increase in cerebellar neurotrophin-3 and oxidative stress markers in autism. Cerebellum (2009) 8:366–72.
55. Allgren RL, Marbury TC, Rahman SN, Weisberg LS, Fenves AZ, Lafayette RA, et al. Anaritide in acute tubular necrosis. Auriculin Anaritide Acute Renal Failure Study Group. N Engl J Med (1997) 336:828–34.
56. Hirschberg R, Kopple J, Lipsett P, Benjamin E, Minei J, Albertson T, et al. Multicenter clinical trial of recombinant human insulin-like growth factor I in patients with acute renal failure. Kidney Int (1999) 55:2423–32.
57. Acker CG, Singh AR, Flick RP, Bernardini J, Greenberg A, Johnson JP. A trial of thyroxine in acute renal failure. Kidney Int (2000) 57:293–8.
58. Wang A, Holcslaw T, Bashore TM, Freed MI, Miller D, Rudnick MR, et al. Exacerbation of radiocontrast nephrotoxicity by endothelin receptor antagonism. Kidney Int (2000) 57:1675–80.
60. Yamaguchi K, Hosokawa Y, Kohashi N, Kori Y, Sakakibara S, Ueda I. Rat liver cysteine dioxygenase (cysteine oxidase). Further purification, characterization, and analysis of the activation and inactivation. J Biochem (1978) 83:479–91.
61. Olney JW, Zorumski C, Price MT, Labruyere J. l-cysteine, a bicarbonate-sensitive endogenous excitotoxin. Science (1990) 248:596–9.
62. Janáky R, Varga V, Hermann A, Saransaari P, Oja SS. Mechanisms of l-cysteine neurotoxicity. Neurochem Res (2000) 25:1397–405.
63. Misra CH. Is a certain amount of cysteine prerequisite to produce brain damage in neonatal rats? Neurochem Res (1989) 14:253–7.
Keywords: hydrogen sulfide, bound sulfane sulfur, l-cysteine, d-cysteine, 3MST, DAO, ischemia-reperfusion injury
Citation: Shibuya N and Kimura H (2013) Production of hydrogen sulfide from d-cysteine and its therapeutic potential. Front. Endocrinol. 4:87. doi: 10.3389/fendo.2013.00087
Received: 28 May 2013; Paper pending published: 26 June 2013;
Accepted: 01 July 2013; Published online: 16 July 2013.
Edited by:
Sho Kakizawa, Kyoto University, JapanCopyright: © 2013 Shibuya and Kimura. This is an open-access article distributed under the terms of the Creative Commons Attribution License, which permits use, distribution and reproduction in other forums, provided the original authors and source are credited and subject to any copyright notices concerning any third-party graphics etc.
*Correspondence: Hideo Kimura, Department of Molecular Pharmacology, National Institute of Neuroscience, 4-1-1 Ogawahigashi, Kodaira, Tokyo 187-8502, Japan e-mail:a2ltdXJhQG5jbnAuZ28uanA=