- 1Molecular Endocrinology Laboratory (KMEB), Odense University Hospital, University of Southern Denmark, Odense, Denmark
- 2Department of Endocrinology, Odense University Hospital, University of Southern Denmark, Odense, Denmark
- 3Laboratory of Molecular Physiology, Department of Pathology, Odense University Hospital, University of Southern Denmark, Odense, Denmark
Aims: Delta like 1/fetal antigen 1 (Dlk1/FA1) is a protein secreted by hormone producing cells in adult human and mice that is known to inhibit adipogenesis. Recent studies demonstrated the role of Dlk1/FA1 in inducing insulin resistance in mice. To investigate the involvement of circulating Dlk1/FA1 in insulin resistance and type 2 diabetes in human subjects, we studied the effects of chronic FA1 on the intermediary metabolism in myotubes established from lean, obese, and type 2 diabetic (T2D) subjects.
Methods: Myotube cultures were established from lean and obese control subjects, and obese T2D subjects and treated with soluble FA1 for 4 days supplemented with/without palmitate (PA). Lipid- and glucose metabolism were studied with labeled precursors while quantitative expression of genes was analyzed using real-time PCR.
Results: Diabetic myotubes express significantly reduced insulin stimulated glucose metabolism compared to lean myotubes and a significantly decreased basal PA oxidation. Chronic FA1 exposure did not affect the intermediary metabolism in myotubes. Insulin sensitivity of glucose and lipid metabolism was not affected by chronic FA1 exposure in myotubes established from lean, obese, and T2D subjects. Instead, chronic FA1 exposure induced pro-inflammatory cytokines expression (IL-6 and CCL2) in association with reducing adipogenic markers (ADD1, AP2, CD36, and PPARg2) in myotubes. Consistent with this observation, addition of FA1 to cultured myotubes was show to significantly inhibit their differentiation into adipocyte.
Conclusion: Our results exclude direct effects of FA1 on glucose and lipid metabolism in cultured myotubes established from lean, obese, and T2D subjects. Therefore, the pathogenesis of FA1-induced IR might mainly be mediated via the FA1-induced stimulation of pro-inflammatory cytokines, which on turn inhibit adipogenesis in human myotubes.
Introduction
Delta like 1/Pre-adipocyte factor-1 (Dlk1/Pref-1) is a transmembrane protein that contains six cysteine-rich EGF-like repeats in its extracellular domain, similar to those found in the Delta/Notch/Serrate family of signaling molecules (Laborda et al., 1993; Fleming, 1998). Dlk1 is a broadly expressed protein by most embryonic and fetal tissues in human and mice and its expression is dramatically down-regulated to become localized only to the hormone secreting cells in the pituitary gland, pancreatic islets, adrenal glands, and testis (Floridon et al., 2000; Yevtodiyenko and Schmidt, 2006). Dlk1 plays a critical role in modulating cell fate decisions throughout development (Laborda, 2000) and has been identified as a negative regulator of adipocyte differentiation (Smas and Sul, 1993). Mice deficient in Dlk1 displayed growth retardation, obesity, skeletal malformations, and abnormalities of hematopoiesis (Moon et al., 2002; Sakajiri et al., 2005). Also, in the human syndrome of maternal uniparental disomy (UPD)14 (where Dlk1 is silent), patients exhibit obesity, hypotonia, premature puberty, macrocephaly, short stature, and small hands (Berends et al., 1999).
The extracellular domain of the Dlk1 is proteolitically cleaved by tumor necrosis factor alpha converting enzyme (TACE) (Wang and Sul, 2006) and shed into the circulation as a soluble active form named fetal antigen 1 (FA1) (Jensen et al., 1994). Therefore, Dlk1/FA1 can function in a paracrine/endocrine fashion (Jensen et al., 1993). Circulating Dlk1/FA1 is present at very high levels in serum/amniotic fluid of pregnant human and mice (Bachmann et al., 1996; Jensen et al., 1997). In addition, the serum level of FA1 is elevated in some human pathological conditions including neurofibromatosis (Jensen et al., 1999), renal failure (10 times higher) (Jensen et al., 1997), and small cell lung cancer patients (10–1000 times higher) (Harken et al., 1999).
Consistent with to its role in adipogenesis, we have shown that systemic administration of FA1 in adult mice led to reduce both fat and bone mass in a dose-dependent manner (Abdallah et al., 2007b).
Recently, transgenic mice with high circulating level of FA1 were shown to display a lipodystrophic phenotype in association with increased whole body insulin resistance suggesting an endocrine function for FA1 in insulin resistance and T2D (Villena et al., 2008).
In our attempt to investigate the potential role of FA1 in insulin sensitivity and T2D of human subjects, we studied the chronic effect of dlk1/FA1 on insulin sensitivity, and lipid metabolism in myotube cultures established from lean, obese, and type 2 diabetic (T2D) (Gaster et al., 2002) subjects. Our detailed biochemical and molecular analysis revealed an immune-modulatory effect of FA1 on increasing the production of pro-inflammatory cytokines in cultured myotubes without affecting their insulin sensitivity.
Materials and Methods
Materials
Dulbecco’s modified Eagle’s medium, fetal calf serum (FCS), penicillin-streptomycin-amphotericin B, and trypsin-EDTA were obtained from Invitrogen (Invitrogen, Scotland, UK). Ultroser G was purchased from Pall Biosepra (Cergy-Saint-Christophe, France). Protein assay kit was purchased from Bio-Rad (Copenhagen, Denmark). Palmitic acid, L-carnitine, and ECM-gel were purchased from Sigma Chemical Co. (St. Louis, MO, USA). Bovine serum albumin (BSA; essentially fatty acid free) was from Calbiochem (VWR, Roskilde, Denmark). Insulin Actrapid was from Novo Nordisk (Bagsvaerd, Denmark).
Fetal Antigen 1
The full soluble ectodomain, active form of Dlk1 protein named FA1, was purified by immuno-specific affinity chromatography from human amniotic fluid and kindly provided by Dr. Bøger Teisner (Immunology Dept., OUH, Denmark) as described previously (Jensen et al., 1993).
Human Study Subjects
Eight lean, eight obese control subjects, and eight obese T2D patients participated in the study (Table 1), and their clinical characteristics have previously been published (Ortenblad et al., 2005; Gaster and Beck-Nielsen, 2006). All subjects gave written, informed consent, and the study was approved by the local ethics committee of Funen and Vejle County. Muscle biopsies were obtained from the vastus lateralis muscle by needle biopsy under local anesthesia. Diabetic patients were treated with either diet alone or in combination with sulfonylurea, metformin, or insulin withdrawn 1 week before the study. The patients suffered from no diabetic complications except for simplex retinopathy. The control subjects had normal glucose tolerance and no family history of diabetes.
Cell Culture
Cell cultures were established as previously described (Gaster et al., 2001a,b,c). In brief, muscle tissue was minced, washed, and dissociated for 60 min in 0.05% trypsin-EDTA. The cells obtained were seeded for up-scaling on ECM-gel coated dishes after 30 min of pre-plating. Growth medium contains DMEM supplemented with 2% FCS, 2% Ultroser G, 50 U/ml penicillin, 50 μg/ml streptomycin, and 1.25 μg/ml amphotericin B. Cells were sub-cultured twice before final seeding. At 75% confluence, the growth medium was replaced by basal medium (DMEM supplemented with 2% FCS, 50 U/ml penicillin, 50 μg/ml streptomycin, 1.25 μg/ml amphotericin B, and 25 pmol/l insulin) in order to induce differentiation. The cells were cultured in humidified 5% 2CO2 atmosphere at 37°C, and medium was changed every 2–3 days.
Experimental Design
Human myotubes established from lean, obese, and T2D subjects were allowed to differentiate under physiological conditions of insulin (25 pmol/l) and glucose (5.5 mmol/l). All myotube cultures were used for analysis day eight after onset of differentiation. Myotubes were exposed to three different protocols: (1) the last 4 days myotubes established from all three groups were exposed with/without 3.0 μg/ml FA1 followed by subsequent determination of glucose uptake, oxidation and storage, lipid uptake, and oxidation at baseline and during acute insulin stimulation and protein content. RNA were isolated from sister cultures. (2) The last 4 days myotubes established from lean subjects were exposed with/without 3.0 μg/ml FA1 supplemented with 0.4 mmol/l PA followed by subsequent determination of glucose uptake, oxidation and storage, lipid uptake, and oxidation at baseline and during acute insulin stimulation and protein content. RNA were isolated from sister cultures. (3) lean myotubes were exposed for 0.4 mmol/l PA with/without 3.0 μg/ml FA1 day 4 and PA incorporation into the myotubes at various time points determined by scintillation proximity assay (SPA) method (Gaster, 2009b) for 7 days (168 h). PA: BSA molar ratio was 2.5:1.
Substrate Oxidation
Glucose and palmitate (PA) oxidation was determined by a 96 multi-well tracer technique as previous described (Gaster, 2009a). Substrate oxidation was monitored by incubating myotubes with [1-14C]-PA (2.0 μCi/ml) in a final concentration of 0.4 mmol/l PA and [14C(U)]-glucose (2.0 μCi/ml) in a final concentration of 5.0 mmol/l glucose with subsequent capture of liberated 14CO2 for 4 h at 37°. Trapped radioactivity was determined on a Microbeta counter (PerkinElmer, Finland).
Adipocyte Differentiation
AIM Cells were seeded at 3 × 104 cells/cm2 in 60 cm2 petri-dishes (for RNA isolation) or in six-well plates (for histochemical staining and flow cytometry studies) and cultured in a standard growth medium. At 90–100% cell confluence, the medium was replaced by high-glucose Dulbecco’s modified MEM (DMEM; Gibco Invitrogen) containing 10% FCS and supplemented with adipogenic-induction mixture (AIM) [containing 10−7 M dexamethasone (dex), 0.45 mM isobutyl methyl xanthine (IBMX), 2 × 10−6 M insulin (all from, Sigma-Aldrich, Vallensbaek strand, Denmark), and 1 μM Rosiglitazone [(BRL49653; Novo Nordisk, Bagsvaerd, Denmark)]. The adipogenic medium was replaced every 3 days. Cells differentiated into adipocytes were washed twice in PBS and lipid staining was performed using Oil Red O stain.
Glucose and Lipid Uptake
Glucose uptake was measured by capturing 2-[1-14C]-deoxy-glucose and lipid uptake was measured as the incorporation of [1-14C]-PA (2.0 μCi/ml) as previously described (Gaster and Beck-Nielsen, 2004, 2006). Radioactivity was determined on a Microbeta counter (PerkinElmer, Finland).
Glycogen Synthesis
Glycogen synthesis was measured as previous described (Gaster and Beck-Nielsen, 2004) in 96 well plates. Radioactivity was measured with a Microbeta counter (PerkinElmer, Finland).
PA Incorporation in Intracellular Lipids
Incorporation of PA over time into human myotubes was estimated by an SPA (Gaster, 2009b) taking the advantage of the fact that the accumulated radioactivity provides signal stronger than the signal coming from the media. Differentiated myotubes were exposed to increasing C-14 labeled PA concentrations (0.4 mmol/l) with/without 3.0 μg/ml FA1 for 7 days. PA incorporated into the myotubes was monitored regularly by measuring accumulation of radioactivity (Microbeta, PerkinElmer, Finland).
RNA Isolation and Real-Time
Total RNA was isolated from human myotubes using a single step method with TRIzol (Invitrogen A/S, Tastrup, Denmark) according to the manufacturer’s instructions. The integrity and purity of total RNA was verified spectrophotometrically and by gel-electrophoresis on 0.8% SeaKem agarose (BMA, Hellerup, Denmark). cDNA was synthesized from 5 μg of total RNA using a commercial revertAid H minus first strand cDNA synthesis kit (Fermentas, Copenhagen, Denmark) according to manual instructions. Real-time PCR (RT)-PCR was performed in iCycler IQ detection system (Bio-Rad, Herlev, Denmark) by using SYBR® Green I as a double-strand DNA-specific binding dye. Thermocycling was performed in a final volume of 20 μl containing 3 μl of cDNA sample (diluted 1:20), 20 pmole of each primer, and 2× iQ™ SYBR® Green Supermix (Bio-Rad). The quantification of each target gene and β-actin mRNA using primers in Table 2 was performed in separate tubes. Gene expression levels for each target gene were calculated using the comparative Ct method [(1/(2ΔCt) formula, where ΔCt is the difference between Ct target and Ct-reference] after normalization to β-actin mRNA (PerkinElmer’s User Bulletin No. 2). Data were analyzed using optical system software version 3.1 (Bio-Rad) and Microsoft Excel 2000 to generate relative expression values (Frederiksen et al., 2008).
Statistical Analysis
The data in the text, tables, and figures are presented as mean ± SE. The statistical analyses were performed with INSTAT 2.01 (GraphPad, USA). P ≤ 0.05 was considered to be significant.
Results
Chronic FA1 Exposure Did not Affect the Intermediary Metabolism of Lean Myotubes
Increasing serum levels of FA1 was shown to be associated with impairment of insulin sensitivity (Villena et al., 2008). In order to investigate the direct involvement of FA1 in stimulating insulin resistance in myotubes, we studied the effect of soluble FA1 on different parameters of insulin sensitivity in cultured myotubes established from lean subjects. As shown in Table 3, lean myotubes exposed to chronic (4 days; 3.0 μg/ml) FA1 did not express significantly different rates of neither glucose and lipid uptake nor glucose oxidation and glycogen synthesis rates as compared to non-treated control cultures (Table 3).
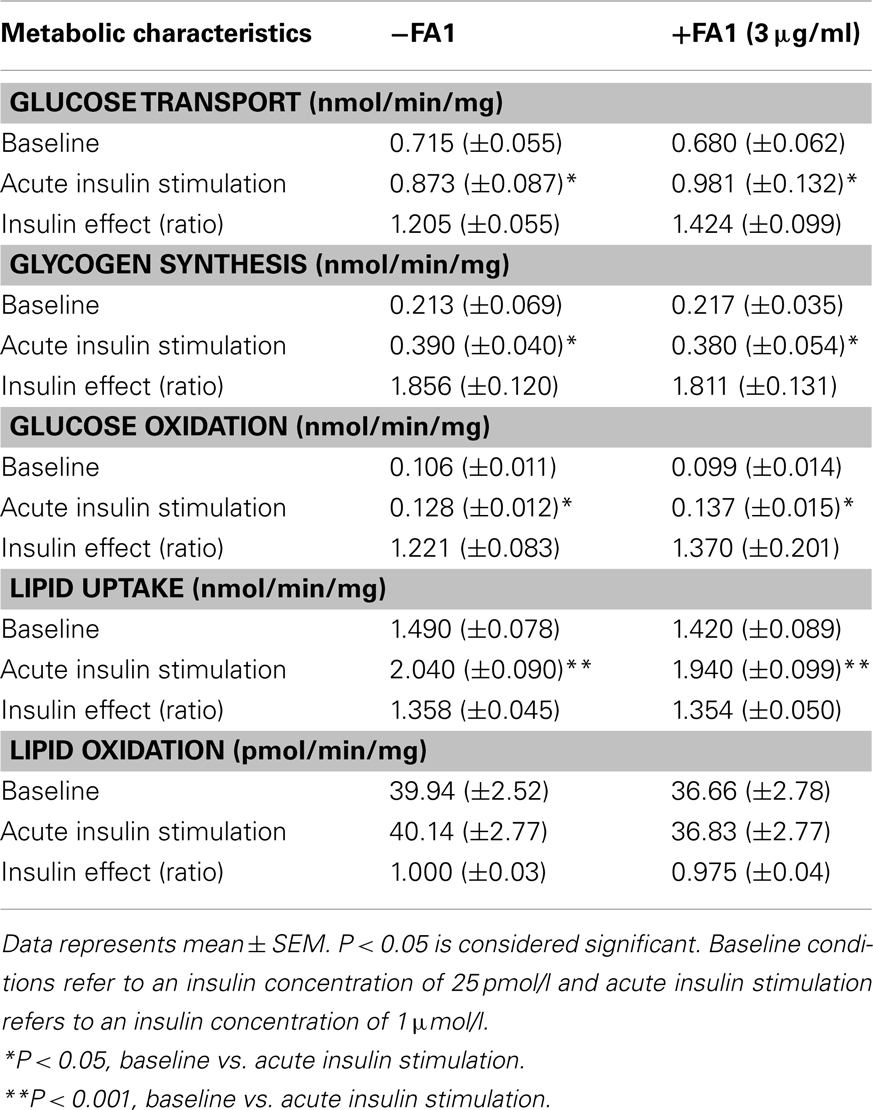
Table 3. Metabolic characteristics of lean myotubes (n = 8) with/without exposure to FA1 (3 μg/ml) for 4 days.
Chronic FA1 Treatment Does not Reduce the Insulin Sensitivity of the Glucose and Lipid Metabolisms in Myotubes Established from Lean, Obese, and T2D Subjects
We also investigated the direct effect of FA1 on insulin and lipid metabolisms in both obese and T2D myotubes. As shown in Figure 1, treatment of obese and diabetic cultured myotubes for 4 days with purified hFA1 did not significantly reduce any of the insulin sensitivity parameters including glucose uptake and oxidation, glycogen synthesis, PA uptake, and oxidation as compared to non-treated control cultures (Figure 1).
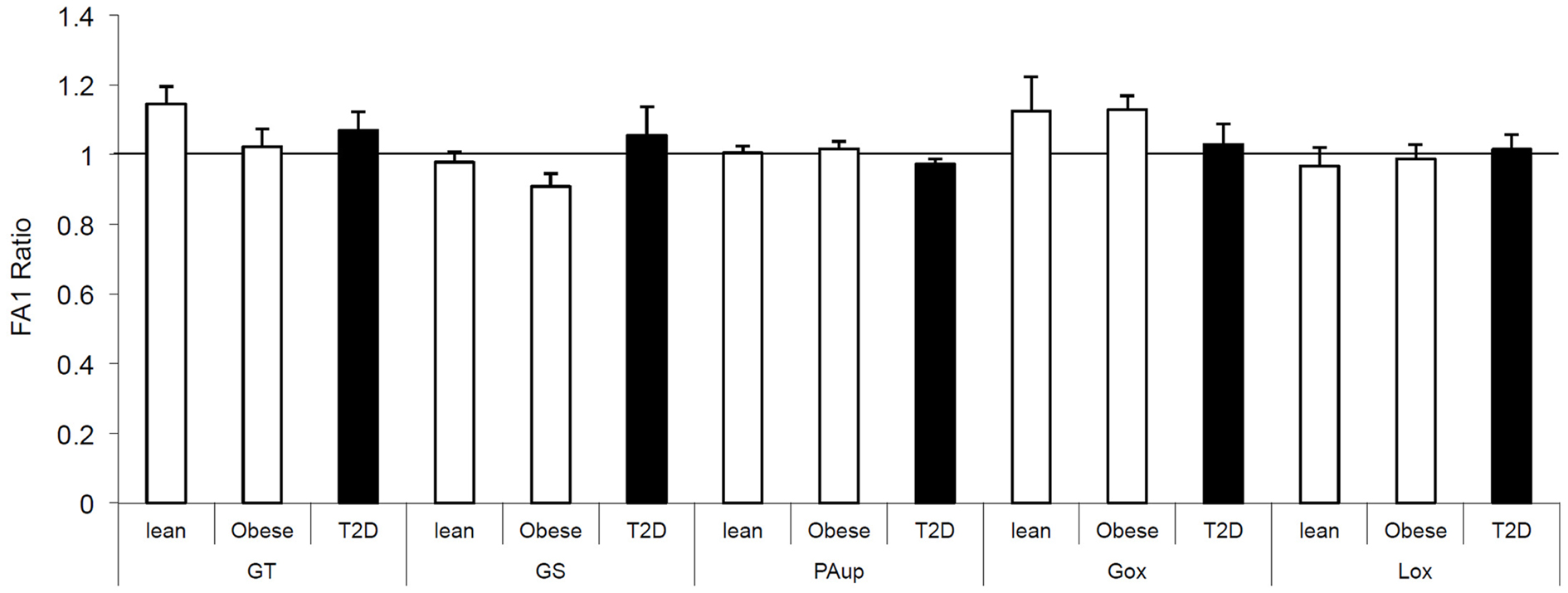
Figure 1. Effect of FA1 treatment on the insulin sensitivity of cultured myotubes from lean, obese, and T2D subjects. Human myotubes established from lean, obese, and T2D subjects were differentiated for 4 days under physiological conditions and subsequently treated without (control) or with 3.0 μg/ml FA1 for further 4 days, as described in Section “Materials and Methods.” Glucose uptake (GT), glucose oxidation (Gox), glycogen synthesis (GS), lipid uptake (PAup), and lipid oxidation (Lox) were determined at baseline and during acute insulin stimulation. Data represented as ratio of (insulin stimulation/basal) with/without FA1. Data are shown as mean ± SEM. N = 8 in each group.
Chronic FA1 Exposure Induces Pro-Inflammatory Gene Expression in Human Myotubes and Inhibits Adipogenic Markers
We further studied the effect of FA1 treatment on gene expression profile of inflammatory, adipogenic, and glucose transport genes in cultured myotube established from lean, obese, and T2D subjects (Figure 2). As shown in Figure 2, FA1 treatment for 4 days significantly reduce the mRNA expression of adipogenic differentiation markers ADD1, AP2, CD36, and PPARΥ2 in association with increasing the expression of the pro-inflammatory markers IL-6 and CCL2 (Figure 2). Consistent with our biochemical assays, FA1 treatment did not change the mRNA expression of GLUT1 and GLUT4 that are important for basal and insulin stimulated glucose uptake respectively.
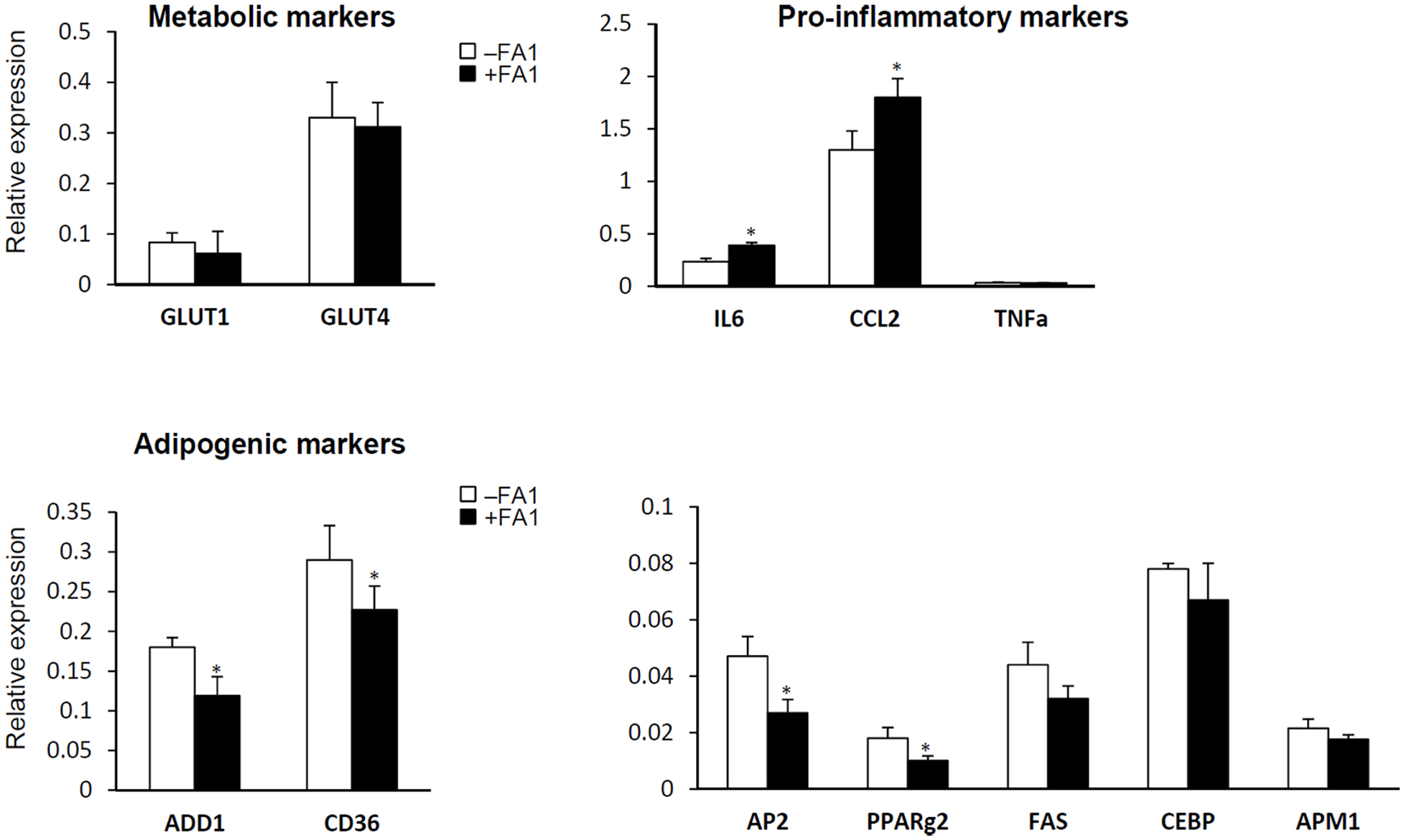
Figure 2. Effect of FA1 treatment on gene expression of metabolic, pro-inflammatory, and adipogenic markers in cultured myotubes from lean, obese, and T2D subjects. Cells were differentiated for 4 days under physiological conditions and subsequently treated without (control) or with 3.0 μg/ml FA1 for further 4 days, as described in Section “Materials and Methods.” Gene expression analysis was measured by real-time PCR and the expression analysis of each target gene was represented as relative expression to β-actin. Data from the three groups were pooled as there were no differences between their expression levels of several genes important for various aspects of the intermediary metabolism. Data represented as mean ± SEM (N = 8 per each group); *P < 0.05.
FA1 Inhibits Adipocyte Differentiation of Human Myotubes
Delta like 1/FA1 has been established as an inhibitor of adipocyte differentiation and fat mass in vitro and in vivo (Abdallah et al., 2004, 2011b). Thus, we studied the inhibitory effect of FA1 on adipogenic differentiation of myotubes as a consequence of FA1-induced down-regulation of the above indicated adipogenic markers. As shown in Figure 3, the addition of soluble FA1 to the myotube cultures inhibited their adipocyte differentiation as assessed by significant reduction in Oil red O staining for fatty acid accumulation (Figure 3A) and marked down-regulation of the expression of adipogenic markers PPARg2, LPL, and aP2 (Figure 3B). These data showed that Dlk1/FA1 exerts an anti-adipogenic effect on human myotubes.
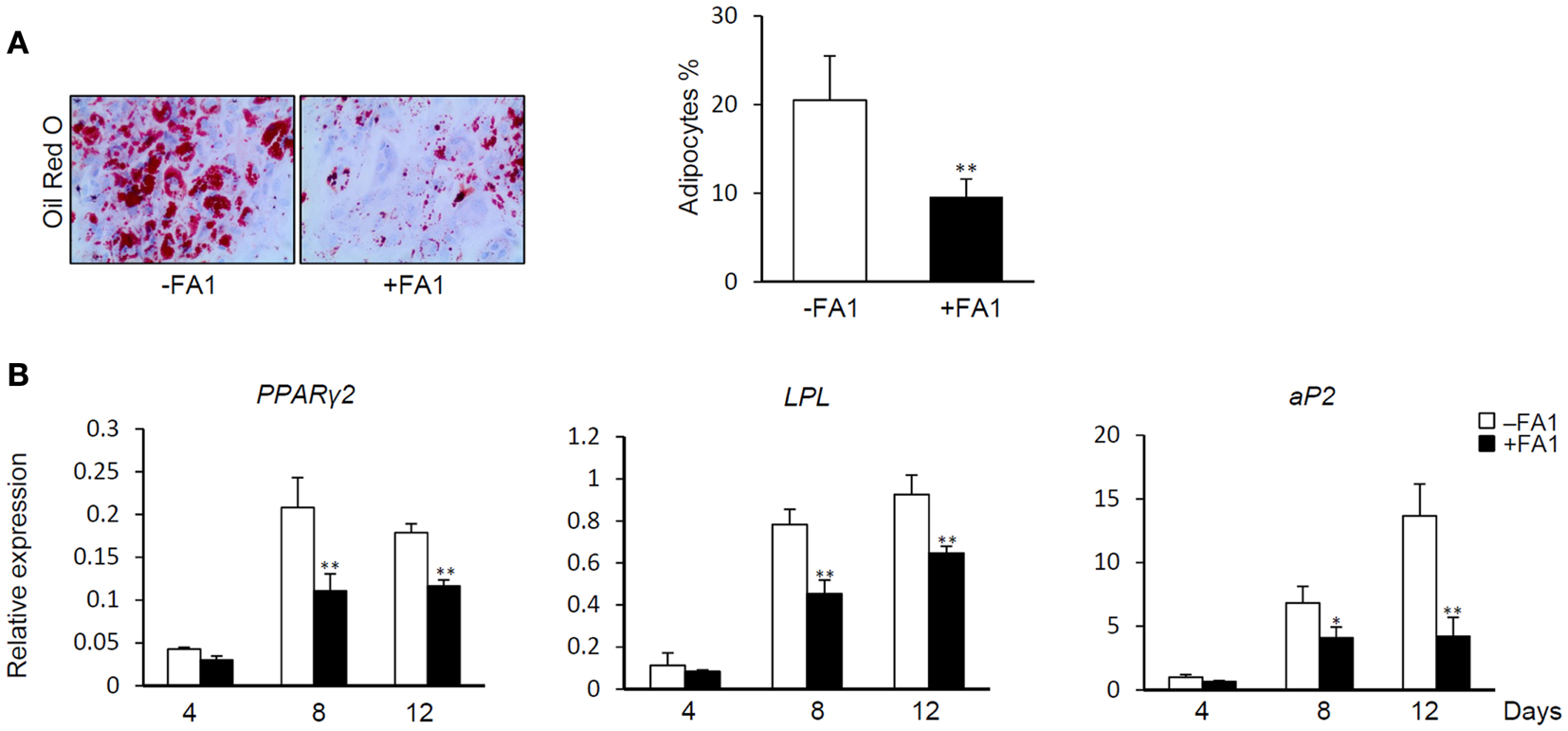
Figure 3. Effect of FA1 on adipocyte differentiation of myotubes. (A) Soluble active form of Dlk1 (FA1) inhibits the adipocyte differentiation of myotubes. Cultured myotubes established from lean subjects were induced to differentiate into adipocyte without (control) or with 3 μg/ml of purified FA1. Adipocytes were stained at day 12 with Oil red O and quantified by microscopic investigation. (B) Expression of adipogenic markers during the time course of differentiation in the absence and the presence of FA1. Gene expression analysis was quantified by real-time PCR and the expression of each target gene was represented as relative expression to β-actin. Data are shown as mean ± SEM of at least three independent experiments; *P < 0.05 and **P < 0.005.
FA1 Did not Change the Effect of PA on the Intermediary Metabolism
In order to clarify whether the inhibitory effect of FA1 on adipogenesis in myotubes could improve their sensitivity for PA-induced insulin resistance, we treated the established cultured myotubes from lean subjects with PA in the presence or the absence of FA1 for 4 days. The incubation of lean myotubes with PA with/without FA1 for 4 days did not show any significant changes in insulin sensitivity parameters for glucose uptake, oxidation, glycogen synthesis, PA uptake, and oxidation (Figure 4).
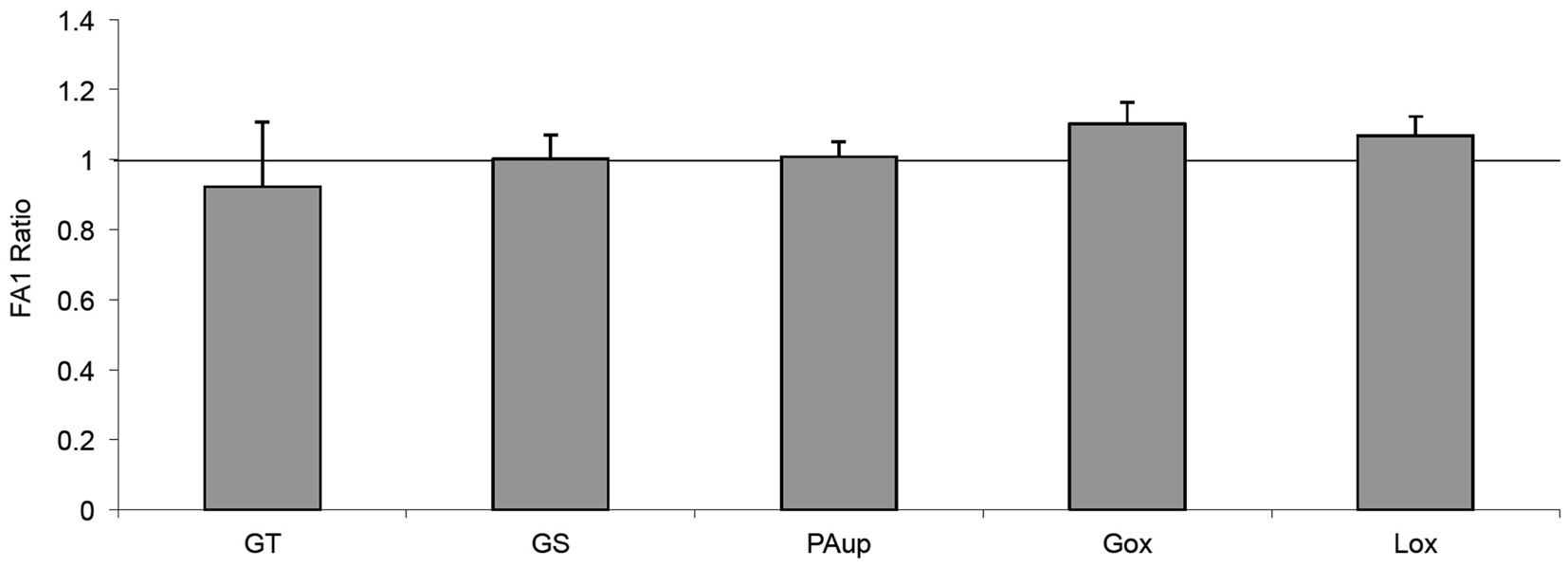
Figure 4. Effect of FA1 on insulin sensitivity of cultured myotubes exposed to PA. Cultured myotubes established from lean subjects were differentiated for 4 days under physiological conditions and subsequently exposed to 0.4 mmol/l PA with or without 3.0 μg/ml FA1 for further 4 days, as described in Section “Materials and Methods.” Glucose uptake (GT), glucose oxidation (Gox), glycogen synthesis (GS), lipid uptake (PAup), and lipid oxidation (Lox) were determined at baseline and during acute insulin stimulation. Data represented as ratio of (insulin stimulation/basal) with/without FA1. Data are shown as mean ± SEM. N = 8.
Chronic FA1 Did Not Reduce/Impair the Incorporation of PA into TAG in Human Myotubes
The incorporation and accumulation of fatty acid in lean myotubes were studied by SPA methodology allowing us to measure the continuous PA incorporation into myotubes at 0.4 mmol/l PA with or without FA1 for 7 days. The accumulation of PA was independent of the presence of FA1 (Figure 5).
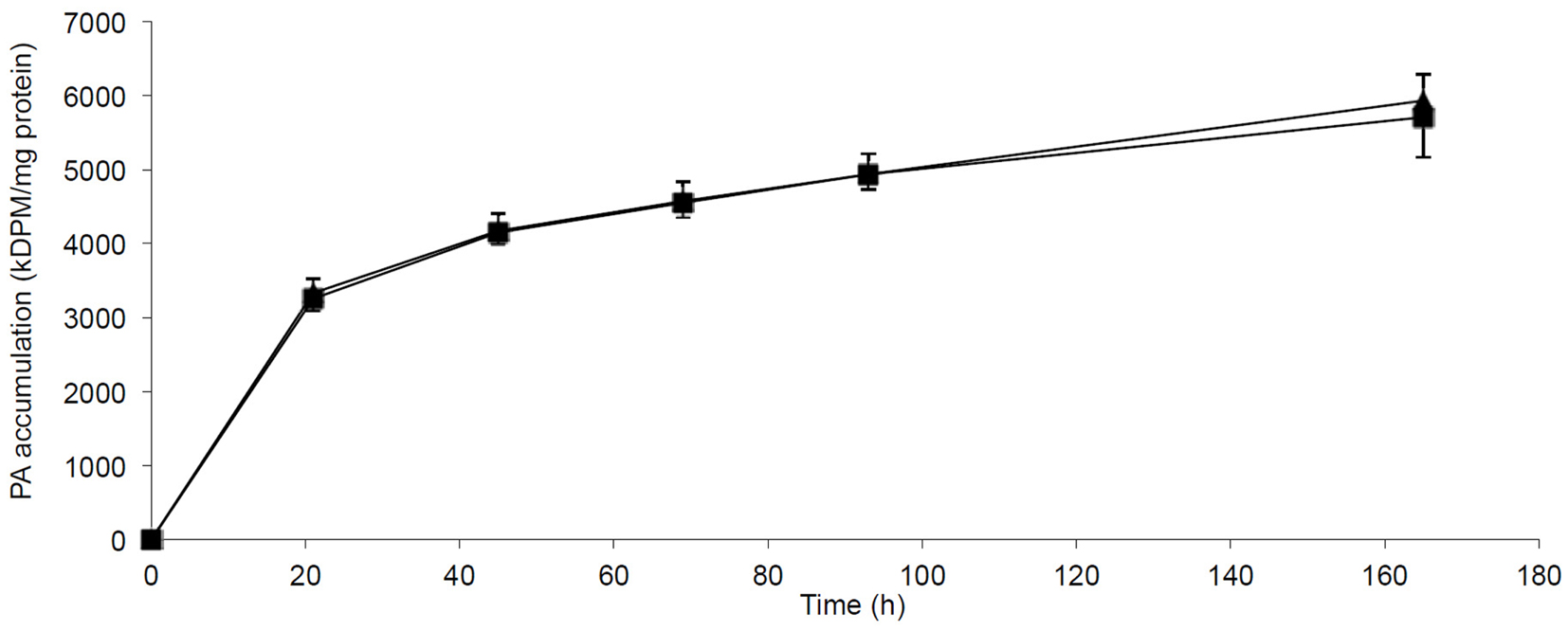
Figure 5. Accumulation of PA in myotubes induced with/without FA1. Cultured myotubes from lean subjects were exposed to 0.4 mmol/l PA with/without 3.0 μg/ml FA1 for 7 days and the intracellular accumulation of PA was regularly monitored. The figure illustrates the time course of PA incorporation into myotubes in the presence (◼) and the absence (▲) of FA1. Data are shown as mean ± SEM. N = 6.
Discussion
We have studied the in vitro effect of circulating FA1 on the insulin sensitivity of human myotubes established from lean, obese, and T2D subjects. Our metabolic studies clearly showed that FA1 does not exert a direct effect on insulin sensitivity of glucose and lipid metabolism in myotubes. Instead, chronic exposure of FA1 stimulates the production of pro-inflammatory cytokines by myotubes in association with inhibiting their adipogenic markers.
Cultured human myotubes are the most similar cell system to intact skeletal muscle that can be modulated ex vivo. Compared to rodent models, our cultured myotubes expresses the right genetic background as well as the specific skeletal muscle phenotype. The extracellular environment can be controlled precisely and kept relatively constant over time, without interference from systemic homeostatic compensatory mechanisms. We and others have reported several potential intrinsic deficiencies in myotubes from individuals with T2D, i.e., reduced capacity for complete oxidation of labeled PA to CO2 compared to control myotubes or reduced insulin mediated increase in glycogen synthesis, and these dysfunctions was reproduced in our present study (Gaster and Beck-Nielsen, 2004; Gaster et al., 2004; Gaster, 2009a).
We and others have shown that the increased serum levels of FA1 was associated with human pathological conditions including neurofibromatosis (5 times higher than the normal range) (Jensen et al., 1999), renal failure (10 times higher) (Jensen et al., 1997), small cell lung cancer patients (10–1000 times higher) (Harken et al., 1999), and estrogen deficiency-induced osteoporosis in post-menopausal women (Abdallah et al., 2011a).
With regard to IR, increasing serum levels of FA1 in mice was shown to be associated with IR through an indirect mechanism involving lipodystrophy-induced IR in peripheral fat (Villena et al., 2008). In accordance, our finding that both insulin sensitivity and lipid metabolism were not per se affected by chronic FA1 exposure in human myotubes suggests that myotube is not the primary targeted tissue by FA1-induced IR.
Our data showed that Dlk1/FA1 inhibits the adipocyte differentiation of cultured human myotubes via a mechanism involved the down-regulation of the adipogenic regulatory genes. This finding is consistent with the established inhibitory effect of Dlk1/FA1 on the adipocyte differentiation of 3T3-L1 and mesenchymal stem cells as well as on reducing fat mass in vivo where, the increased serum levels of FA1 showed to be negatively correlated with fat mass in mice (Lee et al., 2003; Abdallah et al., 2007b). Moreover, in other species including sheep and pigs, the high circulating level of FA1 due to genetic mutation of dlk1 region was shown to be associated with reducing fat mass (Freking et al., 2002; Kim et al., 2004).
Several studies suggested a strong link between inflammation and the development of insulin resistance. Activation of inflammatory pathways in fat and skeletal muscles showed to induce local and systemic insulin resistance (Cai et al., 2005; Plomgaard et al., 2005; Bastard et al., 2006). In this study, the stimulatory effect of Dlk1/FA1 on increasing the production of IL-6 and monocyte chemotactic protein (MCP)-1 by myotubes did not seem to be involved in pathogenesis of insulin resistance in T2D patients (Kalupahana et al., 2012) due to the fact that we could not detect any differences in the FA1-induced pro-inflammatory cytokines in myotubes between T2D and lean and obese controls. Thus, our data could not identify a link between the FA1-induced inflammation in myotubes and insulin resistance. On the other hand, we have recently identified the Dlk1/FA1-induced inflammation as a potential mechanism that mediates the inhibitory effect of Dlk1/FA1 on osteoblast and adipocyte differentiation of human mesenchymal stem cells (Abdallah et al., 2007a) as well as its stimulatory effect on osteoclast differentiation from hematopoietic stem cells in bone marrow (Abdallah et al., 2011b). Thus, it is plausible that the anti-adipogenic effect of Dlk1/FA1 on myotubes is mediated though a mechanism involved increasing the production of cytokines by Dlk1/FA1.
The association between Dlk1/FA1 expression and inflammation has also been demonstrated in a recent report that demonstrated the stimulatory effect of Dlk1/FA1 protein on pro-inflammatory cytokines by immune and fat cells (Chacon et al., 2008). Furthermore, several studies support the involvement of Dlk1/FA1 in general biological processes associated with stimulating inflammatory responses including detection of high Dlk1/FA1 expression levels during ear wound healing (Samulewicz et al., 2002), during liver regeneration in liver injured mice (Jensen et al., 2004), and by satellite cells during muscle regeneration (Crameri et al., 2004). In conclusion, FA1 per se seems to not induce insulin resistance or worsen pre-existing insulin resistance in human myotubes, but shows its effect at the gene level by down-regulating the expression of adipogenic differentiation markers and up-regulating pro-inflammatory cytokines.
Conflict of Interest Statement
The authors declare that the research was conducted in the absence of any commercial or financial relationships that could be construed as a potential conflict of interest.
Acknowledgments
Basem M. Abdallah is also affiliated to Faculty of Science, Helwan University, Cairo, Egypt. Prof. Bøger Teisner is thanked for providing the human purified FA1 for this study. Prof. Moustapha Kassem is thanked for providing lab facilities for some experiments of this study. Irene Lynfort, Bianca Jørgensen, and Karin Dyrgaard provided excellent technical assistance. Kurt Højlund and Klaus Levin are thanked for muscle biopsies.
Abbreviations
FFA, free fatty acids.
References
Abdallah, B. M., Bay-Jensen, A. C., Srinivasan, B., Tabassi, N. C., Garnero, P., Delaisse, J. M., et al. (2011a). Estrogen inhibits Dlk1/FA1 production: a potential mechanism for estrogen effects on bone turnover. J. Bone Miner. Res. 26, 2548–2551.
Abdallah, B. M., Ditzel, N., Mahmood, A., Isa, A., Traustadottir, G. A., Schilling, A. F., et al. (2011b). DLK1 is a novel regulator of bone mass that mediates estrogen deficiency-induced bone loss in mice. J. Bone Miner. Res. 26, 1457–1471.
Abdallah, B. M., Boissy, P., Tan, Q., Dahlgaard, J., Traustadottir, G. A., Kupisiewicz, K., et al. (2007a). dlk1/FA1 regulates the function of human bone marrow mesenchymal stem cells by modulating gene expression of pro-inflammatory cytokines and immune response-related factors. J. Biol. Chem. 282, 7339–7351.
Abdallah, B. M., Ding, M., Jensen, C. H., Ditzel, N., Flyvbjerg, A., Jensen, T. G., et al. (2007b). Dlk1/FA1 is a novel endocrine regulator of bone and fat mass and its serum level is modulated by growth hormone. Endocrinology 148, 3111–3121.
Abdallah, B. M., Jensen, C. H., Gutierrez, G., Leslie, R. G., Jensen, T. G., and Kassem, M. (2004). Regulation of human skeletal stem cells differentiation by Dlk1/Pref-1. J. Bone Miner. Res. 19, 841–852.
Bachmann, E., Krogh, T. N., Hojrup, P., Skjodt, K., and Teisner, B. (1996). Mouse fetal antigen 1 (mFA1), the circulating gene product of mdlk, pref-1 and SCP-1: isolation, characterization and biology. J. Reprod. Fertil. 107, 279–285.
Bastard, J. P., Maachi, M., Lagathu, C., Kim, M. J., Caron, M., Vidal, H., et al. (2006). Recent advances in the relationship between obesity, inflammation, and insulin resistance. Eur. Cytokine Netw. 17, 4–12.
Berends, M. J., Hordijk, R., Scheffer, H., Oosterwijk, J. C., Halley, D. J., and Sorgedrager, N. (1999). Two cases of maternal uniparental disomy 14 with a phenotype overlapping with the Prader-Willi phenotype. Am. J. Med. Genet. 84, 76–79.
Cai, D., Yuan, M., Frantz, D. F., Melendez, P. A., Hansen, L., Lee, J., et al. (2005). Local and systemic insulin resistance resulting from hepatic activation of IKK-beta and NF-kappaB. Nat. Med. 11, 183–190.
Chacon, M. R., Miranda, M., Jensen, C. H., Fernandez-Real, J. M., Vilarrasa, N., Gutierrez, C., et al. (2008). Human serum levels of fetal antigen 1 (FA1/Dlk1) increase with obesity, are negatively associated with insulin sensitivity and modulate inflammation in vitro. Int. J. Obes. (Lond.) 32, 1122–1129.
Crameri, R. M., Langberg, H., Magnusson, P., Jensen, C. H., Schroder, H. D., Olesen, J. L., et al. (2004). Changes in satellite cells in human skeletal muscle after a single bout of high intensity exercise. J. Physiol. (Lond.) 558, 333–340.
Fleming, R. J. (1998). Structural conservation of Notch receptors and ligands. Semin. Cell Dev. Biol. 9, 599–607.
Floridon, C., Jensen, C. H., Thorsen, P., Nielsen, O., Sunde, L., Westergaard, J. G., et al. (2000). Does fetal antigen 1 (FA1) identify cells with regenerative, endocrine and neuroendocrine potentials? A study of FA1 in embryonic, fetal, and placental tissue and in maternal circulation 7. Differentiation 66, 49–59.
Frederiksen, C. M., Hojlund, K., Hansen, L., Oakeley, E. J., Hemmings, B., Abdallah, B. M., et al. (2008). Transcriptional profiling of myotubes from patients with type 2 diabetes: no evidence for a primary defect in oxidative phosphorylation genes. Diabetologia. 51, 2068–2077.
Freking, B. A., Murphy, S. K., Wylie, A. A., Rhodes, S. J., Keele, J. W., Leymaster, K. A., et al. (2002). Identification of the single base change causing the callipyge muscle hypertrophy phenotype, the only known example of polar overdominance in mammals. Genome Res. 12, 1496–1506.
Gaster, M. (2009a). Reduced lipid oxidation in myotubes established from obese and type 2 diabetic subjects. Biochem. Biophys. Res. Commun. 382, 766–770.
Gaster, M. (2009b). The dynamic of lipid oxidation in human myotubes. Biochim. Biophys. Acta 1791, 17–24.
Gaster, M., and Beck-Nielsen, H. (2004). The reduced insulin-mediated glucose oxidation in skeletal muscle from type 2 diabetic subjects may be of genetic origin – evidence from cultured myotubes. Biochim. Biophys. Acta 1690, 85–91.
Gaster, M., and Beck-Nielsen, H. (2006). Triacylglycerol accumulation is not primarily affected in myotubes established from type 2 diabetic subjects. Biochim. Biophys. Acta 1761, 100–110.
Gaster, M., Beck-Nielsen, H., and Schroder, H. D. (2001a). Proliferation conditions for human satellite cells. The fractional content of satellite cells. APMIS 109, 726–734.
Gaster, M., Kristensen, S. R., Beck-Nielsen, H., and Schroder, H. D. (2001b). A cellular model system of differentiated human myotubes. APMIS 109, 735–744.
Gaster, M., Schroder, H. D., Handberg, A., and Beck-Nielsen, H. (2001c). The basal kinetic parameters of glycogen synthase in human myotube cultures are not affected by chronic high insulin exposure. Biochim. Biophys. Acta 1537, 211–221.
Gaster, M., Petersen, I., Hojlund, K., Poulsen, P., and Beck-Nielsen, H. (2002). The diabetic phenotype is conserved in myotubes established from diabetic subjects: evidence for primary defects in glucose transport and glycogen synthase activity 4. Diabetes 51, 921–927.
Gaster, M., Rustan, A. C., Aas, V., and Beck-Nielsen, H. (2004). Reduced lipid oxidation in skeletal muscle from type 2 diabetic subjects may be of genetic origin: evidence from cultured myotubes. Diabetes 53, 542–548.
Harken, J. C., Drivsholm, L., Laursen, I., and Teisner, B. (1999). Elevated serum levels of fetal antigen 1,a member of the epidermal growth factor superfamily, in patients with small cell lung cancer. Tumour Biol. 20, 256–262.
Jensen, C. H., Jauho, E. I., Santoni-Rugiu, E., Holmskov, U., Teisner, B., Tygstrup, N., et al. (2004). Transit-amplifying ductular (oval) cells and their hepatocytic progeny are characterized by a novel and distinctive expression of delta-like protein/preadipocyte factor 1/fetal antigen 1. Am. J. Pathol. 164, 1347–1359.
Jensen, C. H., Krogh, T. N., Hojrup, P., Clausen, P. P., Skjodt, K., Larsson, L. I., et al. (1994). Protein structure of fetal antigen 1 (FA1). A novel circulating human epidermal-growth-factor-like protein expressed in neuroendocrine tumors and its relation to the gene products of dlk and pG2. Eur. J. Biochem. 225, 83–92.
Jensen, C. H., Krogh, T. N., Stoving, R. K., Holmskov, U., and Teisner, B. (1997). Fetal antigen 1 (FA1), a circulating member of the epidermal growth factor (EGF) superfamily: ELISA development, physiology and metabolism in relation to renal function. Clin. Chim. Acta 268, 1–20.
Jensen, C. H., Schroder, H. D., Teisner, B., Laursen, I., Brandrup, F., and Rasmussen, H. B. (1999). Fetal antigen 1, a member of the epidermal growth factor superfamily, in neurofibromas and serum from patients with neurofibromatosis type 1. Br. J. Dermatol. 140, 1054–1059.
Jensen, C. H., Teisner, B., Hojrup, P., Rasmussen, H. B., Madsen, O. D., Nielsen, B., et al. (1993). Studies on the isolation, structural analysis and tissue localization of fetal antigen 1 and its relation to a human adrenal-specific cDNA, pG2. Hum. Reprod. 8, 635–641.
Kalupahana, N. S., Moustaid-Moussa, N., and Claycombe, K. J. (2012). Immunity as a link between obesity and insulin resistance. Mol. Aspects Med. 33, 26–34.
Kim, K. S., Kim, J. J., Dekkers, J. C., and Rothschild, M. F. (2004). Polar overdominant inheritance of a DLK1 polymorphism is associated with growth and fatness in pigs. Mamm. Genome 15, 552–559.
Laborda, J. (2000). The role of the epidermal growth factor-like protein dlk in cell differentiation 3. Histol. Histopathol. 15, 119–129.
Laborda, J., Sausville, E. A., Hoffman, T., and Notario, V. (1993). dlk, a putative mammalian homeotic gene differentially expressed in small cell lung carcinoma and neuroendocrine tumor cell line. J. Biol. Chem. 268, 3817–3820.
Lee, K., Villena, J. A., Moon, Y. S., Kim, K. H., Lee, S., Kang, C., et al. (2003). Inhibition of adipogenesis and development of glucose intolerance by soluble preadipocyte factor-1 (Pref-1). J. Clin. Invest. 111, 453–461.
Moon, Y. S., Smas, C. M., Lee, K., Villena, J. A., Kim, K. H., Yun, E. J., et al. (2002). Mice lacking paternally expressed Pref-1/Dlk1 display growth retardation and accelerated adiposity. Mol. Cell. Biol. 22, 5585–5592.
Ortenblad, N., Mogensen, M., Petersen, I., Hojlund, K., Levin, K., Sahlin, K., et al. (2005). Reduced insulin-mediated citrate synthase activity in cultured skeletal muscle cells from patients with type 2 diabetes: evidence for an intrinsic oxidative enzyme defect. Biochim. Biophys. Acta 1741, 206–214.
Plomgaard, P., Bouzakri, K., Krogh-Madsen, R., Mittendorfer, B., Zierath, J. R., and Pedersen, B. K. (2005). Tumor necrosis factor-alpha induces skeletal muscle insulin resistance in healthy human subjects via inhibition of Akt substrate 160 phosphorylation. Diabetes 54, 2939–2945.
Sakajiri, S., O’Kelly, J., Yin, D., Miller, C. W., Hofmann, W. K., Oshimi, K., et al. (2005). Dlk1 in normal and abnormal hematopoiesis. Leukemia 19, 1404–1410.
Samulewicz, S. J., Seitz, A., Clark, L., and Heber-Katz, E. (2002). Expression of preadipocyte factor-1(Pref-1), a delta-like protein, in healing mouse ears. Wound Repair Regen. 10, 215–221.
Smas, C. M., and Sul, H. S. (1993). Pref-1, a protein containing EGF-like repeats, inhibits adipocyte differentiation. Cell 73, 725–734.
Villena, J. A., Choi, C. S., Wang, Y., Kim, S., Hwang, Y. J., Kim, Y. B., et al. (2008). Resistance to high-fat diet-induced obesity but exacerbated insulin resistance in mice overexpressing preadipocyte factor-1 (Pref-1): a new model of partial lipodystrophy. Diabetes 57, 3258–3266.
Wang, Y., and Sul, H. S. (2006). Ectodomain shedding of preadipocyte factor 1 (Pref-1) by tumor necrosis factor alpha converting enzyme (TACE) and inhibition of adipocyte differentiation. Mol. Cell. Biol. 26, 5421–5435.
Keywords: Dlk1, FA1, Pref-1, insulin resistance, human myotubes, obesity, skeletal muscle, type 2 diabetes
Citation: Abdallah BM, Beck-Nielsen H and Gaster M (2013) FA1 induces pro-inflammatory and anti-adipogenic pathways/markers in human myotubes established from lean, obese, and type 2 diabetic subjects but not insulin resistance. Front. Endocrinol. 4:45. doi: 10.3389/fendo.2013.00045
Received: 19 November 2012; Accepted: 22 March 2013;
Published online: 05 April 2013.
Edited by:
Rhonda C. Bell, University of Alberta, CanadaReviewed by:
Greisa Vila, Medical University of Vienna, AustriaR. Ravi Shankar, Merck Sharpe and Dohme, USA
Copyright: © 2013 Abdallah, Beck-Nielsen and Gaster. This is an open-access article distributed under the terms of the Creative Commons Attribution License, which permits use, distribution and reproduction in other forums, provided the original authors and source are credited and subject to any copyright notices concerning any third-party graphics etc.
*Correspondence: Basem M. Abdallah, Molecular Endocrinology Laboratory (KMEB), Department of Endocrinology, Odense University Hospital, Winslows Vej, 25, 1st floor, DK-5000 Odense, Denmark. e-mail: babdallah@health.sdu.dk