- Department of Endocrinology and Metabolism, WHO Collaborating Center for the Study and Treatment of Thyroid Diseases and Other Endocrine and Metabolic Disorders, University of Pisa, Pisa, Italy
Thyroid carcinoma is the most frequent endocrine cancer accounting for 5–10% of thyroid nodules. Papillary histotype (PTC) is the most prevalent form accounting for 80% of all thyroid carcinoma. Although much is known about its epidemiology, pathogenesis, clinical, and biological behavior, the only documented risk factor for PTC is the ionizing radiation exposure. Rearrangements of the Rearranged during Transfection (RET) proto-oncogene are found in PTC and have been shown to play a pathogenic role. The first RET rearrangement, named RET/PTC, was discovered in 1987. This rearrangement constitutively activates the transcription of the RET tyrosine-kinase domain in follicular cell, thus triggering the signaling along the MAPK pathway and an uncontrolled proliferation. Up to now, 13 different types of RET/PTC rearrangements have been reported but the two most common are RET/PTC1 and RET/PTC3. Ionizing radiations are responsible for the generation of RET/PTC rearrangements, as supported by in vitro studies and by the evidence that RET/PTC, and particularly RET/PTC3, are highly prevalent in radiation induced PTC. However, many thyroid tumors without any history of radiation exposure harbor similar RET rearrangements. The overall prevalence of RET/PTC rearrangements varies from 20 to 70% of PTCs and they are more frequent in childhood than in adulthood thyroid cancer. Controversial data have been reported on the relationship between RET/PTC rearrangements and the PTC prognosis. RET/PTC3 is usually associated with a more aggressive phenotype and in particular with a greater tumor size, the solid variant, and a more advanced stage at diagnosis which are all poor prognostic factors. In contrast, RET/PTC1 rearrangement does not correlate with any clinical–pathological characteristics of PTC. Moreover, the RET protein and mRNA expression level did not show any correlation with the outcome of patients with PTC and no correlation between RET/PTC rearrangements and the expression level of the thyroid differentiation genes was observed. Recently, a diagnostic role of RET/PTC rearrangements has been proposed. It can be searched for in the mRNA extracted from cytological sample especially in case with indeterminate cytology. However, both the fact that it can be present in a not negligible percentage of benign cases and the technical challenge in extracting mRNA from cytological material makes this procedure not applicable at routine level, at least for the moment.
Introduction
Thyroid nodules are a very common clinical finding; the prevalence of palpable nodules ranges from 4 to 7% in general population (Mazzaferri, 1992; Gharib, 2004). Although only less than 5% of palpable nodules are malignant lesions, thyroid cancers are the most frequent endocrine malignancy accounting for about 5–10% of thyroid nodules (Braverman and Utiger, 2005). Epidemiological studies in USA and in Europe demonstrated a relevant increased incidence of thyroid cancer and, in particular, thyroid cancer rate of incidence has been the highest among all human tumors during the last decades (Farahati et al., 2004; Davies and Welch, 2006; Kent et al., 2007; Enewold et al., 2009).
About 95% of malignant lesions are derived from thyroid follicular cells and are distinguished into well differentiated, either papillary (PTC) or follicular (FTC) histotype, and anaplastic thyroid carcinoma (ATC; Cardis et al., 2006). As shown in Figure 1, PTC is the most prevalent form accounting for about 80% of cases, while FTC represent only 15%. PTC and, to a lesser extent, FTC have a good prognosis if adequately treated (Elisei et al., 2010). Very rare (5%), but also very aggressive and almost invariably lethal, is ATC. Another relatively small percentage of thyroid carcinomas (7.5–10%; Schlumberger and Pacini, 1999) are derived from parafollicular C cells and are called medullary thyroid carcinoma (MTC; De Lellis et al., 2003; Nikiforov, 2009).
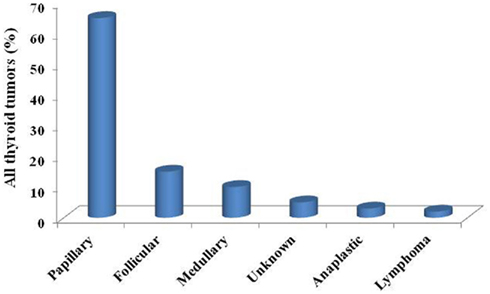
Figure 1. The prevalence of different histotypes among all thyroid tumors: a clear higher prevalence of papillary thyroid cancer (about 80%) is usually reported in all series.
In the last 25 years, many studies have been conducted to identify the genetic alterations related to the pathogenesis of these tumors. Several oncogenes have been analyzed for mutations and some of them have been found to be strictly correlated with a specific thyroid carcinoma histotype (Table 1).
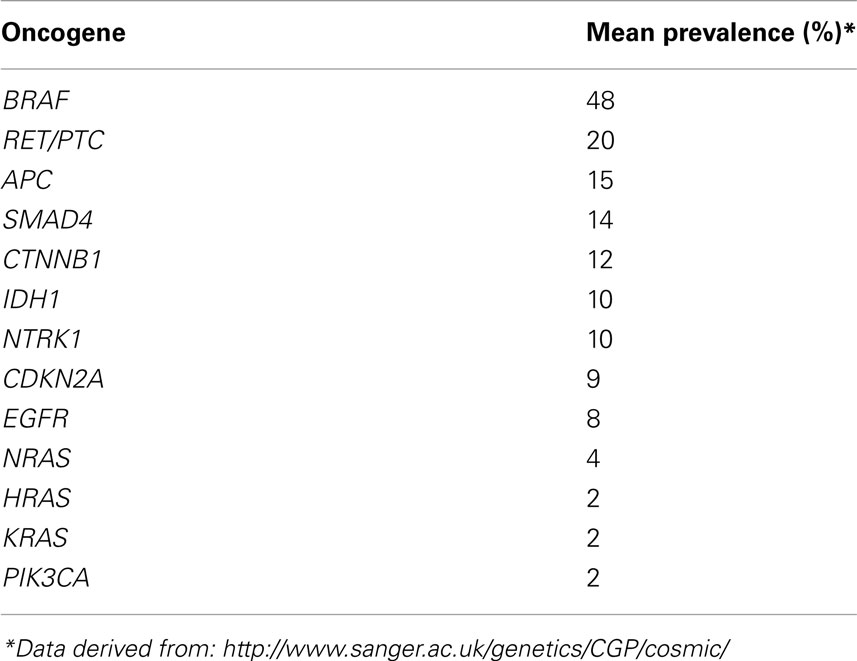
Table 1. Different prevalences of different oncogenes reported to be involved in thyroid carcinogenesis.
In this review we will focus on PTC and in particular on the relationship between the presence of RET/PTC rearrangements, that have been defined to be specific for PTC development and the clinical and pathological features of PTC. The putative role of RET/PTC rearrangements in the diagnosis and in the prediction of prognosis will be discussed.
Papillary Thyroid Cancer
The annual incidence of thyroid cancer per 105 individuals ranges from 1.2 to 2.6 in men and from 2.0 to 3.8 in women and tumor mortality is very low (Franceschi et al., 1993). Thyroid cancer is two to four times more frequent in females than in males. It is rare in children below 16 years of age while in adults the incidence increases with age and the median age at diagnosis is between 45 and 50 years. Differences in the incidence of thyroid cancer according to ethnic origin have been also reported. These differences may be due to environmental factors and dietary habits (Spitz et al., 1988).
Although the etiology of PTC is not well understood, there are some risk factors which are known to put an individual at higher risk of developing the disease. Radiation exposure significantly increases the risk for thyroid malignancies, particularly PTC. This finding was observed in children exposed to radiation after the nuclear bombings in Hiroshima and Nagasaki during the Second World War (Nagataki et al., 1994). Additional evidence was gathered after atomic bombs were tested in the Marshall Islands (Cronkite et al., 1995), after the accident at the Chernobyl nuclear power plant (Williams, 2002; Nikiforov, 2006), and in patients who received low-dose radiation therapy for benign disorders of the head, neck, and thorax (Duffy and Fitzgerald, 1950; Winship and Rosvoll, 1970).
Among other risk factors female gender play an important role in fact it has been shown (Ron et al., 1987) that the sex ratio approaches 1 before puberty and after menopause while during the fertile woman age it is between two and four times higher than in the correspondent men age period. A heavier body weight have been shown also to increase the risk of thyroid cancer (Kitahara et al., 2011).
An important role is also played by the iodine exposure. Areas with low iodine intake usually show higher prevalence of FTC than area with sufficient iodine intake where, conversely, the PTC histotype is more represented (Belfiore et al., 1987). Furthermore the high prevalence of thyroid cancer in regions where the iodine intake is high suggests that iodine intake may play role in thyroid tumorigenesis (Vejbjerg et al., 2007; Guan et al., 2009) as well as other factors not related to iodine like volcanic activity (Pellegriti et al., 2009).
RET/PTC Rearrangements
The RET proto-oncogene is located on chromosome 10q11.2 and encodes for a transmembrane tyrosine-kinase receptor involved in the control of cell differentiation and proliferation. Four different ligands have been reported up to now: glial derived neurotrophic (GDN) factors, Neurturin (NRTN), Artimin (ARTN), and Persepin (PSPN), respectively (Arighi et al., 2005). All these ligands induce RET activation through the binding to specific coreceptors. The RET gene is expressed in tissues deriving from the neural crest including thyroid C cells and adrenal medulla but it is not expressed in normal thyroid follicular cells (Santoro et al., 1990).
In 1987 the first finding of a new oncogene activated in human PTC was reported (Fusco et al., 1987). Interestingly, the tumoral tissue where the oncogene was firstly described was derived from an irradiated PTC. After 3 years, this oncogene was molecularly cloned: it was a chimeric gene generated by the fusion of the RET tyrosine-kinase domain (Wirtschafter et al., 1997) with the 5′ terminal region of a new gene denominated CCD6 (formerly called H4; Figure 2; Grieco et al., 1990). This oncogene was named RET/PTC.
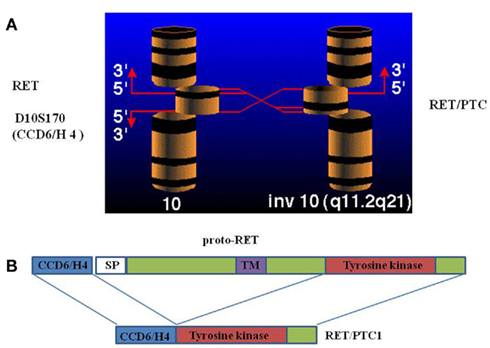
Figure 2. Schematic representation of RET/PTC1 rearrangement: a centromeric inversion of chromosome 10 (A) generates a chimeric gene through the fusion of the RET tyrosine-kinase domain with the 5′ terminal region of a new gene denominated CCD6, formerly called H4 (B).
After the first identification, several types of RET/PTC rearrangements have been described (Greco et al., 2009; Table 2). At the present 13 different types of RET/PTC rearrangements have been reported and all of them are the result of the fusion of the RET tyrosine-kinase (TK) domain with different genes, which are characterized by the presence of nucleotide sequences coding for proteins with an extremely high probability of forming coiled-coil domains, thus allowing constitutive dimerization of the RET-TK domain. This constitutive dimerization determines an uncontrolled proliferation of the follicular cells harboring the RET/PTC rearrangement and the development of malignancy. The presence of RET/PTC rearrangement in microcarcinoma strongly support the hypothesis of a driving role of this oncogene in the tumoral transformation (Viglietto et al., 1995).
So far, RET/PTC rearrangements have been identified almost exclusively in thyroid lesions, and in particular in PTC (Santoro et al., 1993; Tallini and Asa, 2001; Nikiforov, 2002). To our knowledge, the only other human tumor harboring RET/PTC rearrangements is primary peritoneal carcinoma (Flavin et al., 2009). However these rearrangements are supposed to be “passenger” mutations reflecting RET instability in tumor subclones more than true pathogenetic events. RET/PTC rearrangements more frequently found in PTC are RET/PTC1, given by the fusion with the CCDC6 (formerly H4) gene and RET/PTC3, given by the fusion with the NCOA4 (formerly ELE1) gene (Santoro et al., 1994).
The reported RET/PTC prevalence in thyroid tumors varies greatly in different series (Zou et al., 1994; Lam et al., 1998; Mayr et al., 1998; Chua et al., 2000; Cinti et al., 2000; Fenton et al., 2000; Sheils et al., 2000; Puxeddu et al., 2003; Rhoden et al., 2004, 2006; Table 3). This difference can be attributed to ethnical and geographic variations as well as to the different sensitivities of detection methods (Zhu et al., 2006). It has been carefully demonstrated that the method used has an important effect on the efficacy of RET/PTC rearrangement determination and thus on the reported prevalence (Marotta et al., 2011). Tumor heterogeneity is another factor that can affect the evaluation of RET/PTC prevalence. The distribution of RET/PTC rearrangement within the same tumor may be heterogeneous, varying from the involvement of most neoplastic cells (i.e., clonal RET/PTC) to the presence of a small fraction of tumor cells (i.e., non-clonal RET/PTC) in the sample (Unger et al., 2004; Zhu et al., 2006).
Clonal RET/PTC rearrangements occur in about 20% of PTC and are specific for this tumor (Nikiforov, 2002, 2011; Zhu et al., 2006). Non-clonal RET/PTC rearrangements have been found not only in PTC but also in 10–45% of thyroid adenomas and other non-neoplastic thyroid lesions (Ishizaka et al., 1991; Wirtschafter et al., 1997; Sheils et al., 2000; Elisei et al., 2001; Chiappetta et al., 2002; Sapio et al., 2011) and Hashimoto’s thyroiditis (Rhoden et al., 2006).
As shown in Table 3, RET/PTC rearrangements are more frequently found in thyroid cancers following radiation exposure (50–80%; Fugazzola et al., 1995; Nikiforov et al., 1997; Thomas et al., 1999; Di Cristofaro et al., 2005; Unger et al., 2006; Hamatani et al., 2008; Hieber et al., 2011). In particular RET/PTC3 has been found to be more frequent than RET/PTC1 in post-Chernobyl radiation exposed thyroid cancer especially in those with a short latency period (Williams, 2008). RET/PTC rearrangements have been also found to be more prevalent in children than in adults both in irradiated and non-irradiated PTC (Nikiforov et al., 1997; Elisei et al., 2001).
Over the time, the proportion of post-Chernobyl thyroid tumors with a RET/PTC rearrangement has declined, and in the RET/PTC-positive tumors the percentage of those with RET/PTC1 has increased while the proportion of those with RET/PTC3 has in parallel decreased (Unger et al., 2006). The hypothesis to explain this variation is that with the increase of latency period the effect of radiation is becoming less significant and most recently developed PTC around Chernobyl are more similar to non-irradiated cases. This hypothesis is at least in part supported by the evidence that also the histological features are changing according to the latency period (Williams, 2008).
The high prevalence of RET/PTC rearrangements found in post-Chernobyl thyroid tumors and in atomic bomb survivors exposed to high-dose radiation (Hamatani et al., 2008) strongly supports a direct role of radiation exposure in RET/PTC generation (Fugazzola et al., 1995; Klugbauer et al., 1995; Bounacer et al., 1997; Nikiforov et al., 1997). RET/PTC rearrangements were also found after high-dose radiation to human undifferentiated thyroid carcinoma cells (Ito et al., 1993) and to fetal human thyroid tissue transplanted into SCID mice (Mizuno et al., 2000). The generation of RET/PTC rearrangements has been also demonstrated in normal thyroid cultured cells after in vitro exposure to radiation (Gandhi et al., 2010a,b). Furthermore, the strong relationship between ionizing radiation exposure and RET/PTC rearrangements have been shown by a COMET assay that demonstrated that RET oncogene is very susceptible to ionizing radiation (Volpato et al., 2008). Moreover, the spatial contiguity of RET and H4 genes within the chromatin may provide a structural basis for generation of RET/PTC1 rearrangement by allowing a single radiation track to produce a double-strand break in each gene (Nikiforova et al., 2000; Gandhi et al., 2006, 2010a).
Over the last few decades, significant progress has been achieved in the understanding of the biological mechanisms of radiation carcinogenesis. It has been shown that damage to cellular DNA is responsible for mutagenesis and carcinogenesis and that double-strand breaks is the most important event for the direct generation of gene translocations and rearrangements. In particular there are several in vitro evidences demonstrating that thyroid cells exposed to X-Ray develop RET/PTC rearrangements (Ito et al., 1993; Goodhead, 1994; Ward, 1995; Mizuno et al., 2000; Caudill et al., 2005). Other than ionizing radiations, other putative carcinogens, like caffeine, ethanol, hypoxia, and others, are able to induce DNA double-strand breaks and generate RET/PTC rearrangements (Gandhi et al., 2010a,b). Recently, a direct relationship between ionizing radiation exposure, intracellular hydrogen peroxidase (H2O2) generation, double-strand induction, and RET/PTC1 rearrangements development was also demonstrated (Ameziane-El-Hassani et al., 2010). After this observation, it can be hypothesize that other agents able to initiate biological production of superoxidase anions and H2O2 (Narayanan et al., 1997) can induce RET/PTC rearrangements.
RET/PTC Rearrangements and Clinico-Pathological Features of PTC
A correlation with a more aggressive phenotype and a more advanced stage has been reported for RET/PTC rearrangements, especially RET/PTC3 (Nikiforov et al., 1997; Powell et al., 1998). In particular, in post-Chernobyl childhood thyroid cancer the RET/PTC3 rearrangement was more frequently associated with the solid variant of PTC which is considered a more aggressive variant and the most prevalent among these irradiated tumors. As matter of fact, with the increase of the latency period both the solid variant and the RET/PTC rearrangements prevalence have declined suggesting a strong relationship between radiation exposure, solid variant, RET/PTC3 rearrangements, and a more rapid development of the tumor as indicated by the short latency period (Williams, 2008).
In a previous study on sporadic PTC we also demonstrated a positive correlation between the presence of RET/PTC3 rearrangement, but not of RET/PTC1, with a bigger size of the tumor and a more advanced stage at diagnosis. However, in the same study the levels of expression of thyroid differentiation genes (i.e., thyrotropin stimulating hormone receptor, thyroglobulin, sodium-iodide symporter etc.) was shown to be not significantly different in PTC with or without RET/PTC rearrangements, thus suggesting that these genetic alterations should not play a major role in the de-differentiation process (Romei et al., 2008).
Controversial data have been also reported about the correlation of the presence of RET/PTC rearrangements and clinical and epidemiological features of patients with PTC. Although there is a general agreement that RET/PTC rearrangements, particularly RET/PTC1, are more frequent in young patients, also in non-irradiated cases (Elisei et al., 2001) there are other studies that did not show any correlation between RET/PTC rearrangements and age, sex, tumor size, staging, number of neoplastic foci, and histological subtype (Puxeddu et al., 2004).
So far no consensus concerning the clinical prognostic value of the presence of a RET/PTC rearrangement, either RET/PTC3 or RET/PTC1, has been reached. Some evidences exists suggesting that PTC with RET/PTC1 rearrangement are associated with a more favorable behavior (Saad et al., 2004) while those harboring RET/PTC3 rearrangement may be more prone to de-differentiation and a more aggressive behavior (Sugg et al., 1999; Mochizuki et al., 2010). Initial reports on this specific issue claimed a role in metastatic spread (Jhiang and Mazzaferri, 1994; Sugg et al., 1999) and more recently, it has been suggested that RET/PTC-positive cases show higher rates of local extension and lymph node involvement than RET/PTC negative cases (Adeniran et al., 2006). At variance, several other studies were unable to find any relationship between RET/PTC rearrangements and classical prognostic factors (Tallini et al., 1998; Musholt et al., 2000; Basolo et al., 2001; Romei et al., 2008).
In our experience, RET/PTC rearrangements do not seem to be correlated with any clinical and pathological feature of aggressiveness either by studying the expression of the RET/PTC protein by immunohistochemistry (Basolo et al., 2001) or by studying the mRNA expression of the RET/PTC rearrangements by real time RT-PCR (Romei et al., 2008).
Diagnostic Role of RET/PTC Rearrangements
Fine needle aspiration cytology (FNAC) is the first choice method to distinguish between benign and malignant thyroid nodules (Pacini et al., 2006; Baloch and LiVolsi, 2008; Cooper et al., 2009). However, about 30% of FNAC yields uncertain results because of inadequate sampling (i.e., only few cells or great blood contamination) or because cytological features do not clearly indicate the nature of the lesion (i.e., follicular neoplasia; Gharib et al., 1984; Mazzaferri, 1993). Whenever a follicular neoplasia is diagnosed, surgical treatment, and histological examination are required to differentiate the malignant from the benign nature of the nodule. Since only 20–30% of these nodules turn out to be malignant (Rago et al., 2007), about 80% of patients with a follicular neoplasia undergo an unnecessary thyroidectomy.
The rapidly expanding knowledge of molecular genetics of thyroid cancer has started to translate into clinical practice, offering significant improvement in accuracy of the preoperative diagnosis of thyroid cancer. Several studies have been already published on the possibility to improve the diagnostic power of FNAC by looking for thyroid cancer specific oncogenic alterations (Nikiforova and Nikiforov, 2009). Most studies have explored the diagnostic role of BRAF mutation (Jin et al., 2006; Xing et al., 2009; Adeniran et al., 2011; Marchetti et al., 2012). In a large prospective study recently published, the detection of BRAF mutations in FNA showed a very high positive predictive value (PPV). However, the biggest diagnostic impact can be achieved only by testing FNA samples for a panel of mutations (i.e., BRAF, RET/PTC, RAS, TRK) rather than for a single mutation (Cantara et al., 2010; Guerra et al., 2011). Indeed, RET/PTC detection can improve the preoperative diagnosis of thyroid nodules, particularly in samples that are indeterminate by cytology or have an insufficient amount of cells for cytologic evaluation (Cheung et al., 2001; Salvatore et al., 2004; Pizzolanti et al., 2007). Nevertheless the PPV of the detection of RET/PTC rearrangements is not as high as the detection of BRAF mutations because a low, but not negligible, percentage of nodules positive for RET/PTC rearrangements on FNA which turned out to be benign at histology (Cantara et al., 2010; Guerra et al., 2011). This is not unexpected since, have previously said, there are several reports showing that 5% of benign thyroid nodules and several cases of Hashimoto’s thyroiditis are positive for RET/PTC rearrangements. Last but not least, the search for RET/PTC rearrangements requires the mRNA extraction form the cytological smears that is a much more challenging techniques than the extraction of DNA that is required for the search of BRAF. As matter of fact, at the present the search for RET/PTC rearrangements in cytologically indeterminate thyroid nodule is still not considered a diagnostic routine tool.
Conclusion
In the last years important achievements have been reached in the understanding of the molecular basis of PTC. Although different oncogenes have been found to be altered in PTC, BRAF mutations, and RET/PTC rearrangements are the most frequently involved. A lot has been discovered between the relationship of RET/PTC rearrangements and PTC clinical, pathological, and epidemiological features. Although present also in non-irradiated cases, RET/PTC rearrangements are related to radiation exposure and are more frequent in patients with radio induced PTC. Among all RET/PTC rearrangements, RET/PTC1, and RET/PTC3 are in general the most frequent. RET/PTC3 is much more prevalent in irradiated PTC especially in those with solid variants. Both of them are more prevalent in children than in adults. Despite all these observations there are not yet clear data showing a definitive prognostic role of neither RET/PTC1 or RET/PTC3. The presence of a RET/PTC rearrangement in the RNAs extracted from cytological material aspirated from a thyroid nodule is strongly predictive of malignancy but some cases of benign nodules can also result to be positive.
Conflict of Interest Statement
The authors declare that the research was conducted in the absence of any commercial or financial relationships that could be construed as a potential conflict of interest.
References
Adeniran, A. J., Hui, P., Chhieng, D. C., Prasad, M. L., Schofield, K., and Theoharis, C. (2011). BRAF mutation testing of thyroid fine-needle aspiration specimens enhances the predictability of malignancy in thyroid follicular lesions of undetermined significance. Acta Cytol. 55, 570–575.
Adeniran, A. J., Zhu, Z., Gandhi, M., Steward, D. L., Fidler, J. P., Giordano, T. J., Biddinger, P. W., and Nikiforov, Y. E. (2006). Correlation between genetic alterations and microscopic features, clinical manifestations, and prognostic characteristics of thyroid papillary carcinomas. Am. J. Surg. Pathol. 30, 216–222.
Ameziane-El-Hassani, R., Boufraqech, M., Lagente-Chevallier, O., Weyemi, U., Talbot, M., Metivier, D., Courtin, F., Bidart, J. M., El Mzibri, M., Schlumberger, M., and Dupuy, C. (2010). Role of H2O2 in RET/PTC1 chromosomal rearrangement produced by ionizing radiation in human thyroid cells. Cancer Res. 70, 4123–4132.
Arighi, E., Borrello, M. G., and Sariola, H. (2005). RET tyrosine kinase signaling in development and cancer. Cytokine Growth Factor Rev. 16, 441–467.
Baloch, Z. W., and LiVolsi, V. A. (2008). Fine-needle aspiration of the thyroid: today and tomorrow. Best Pract. Res. Clin. Endocrinol. Metab. 22, 929–939.
Basolo, F., Molinaro, E., Agate, L., Pinchera, A., Pollina, L., Chiappetta, G., Monaco, C., Santoro, M., Fusco, A., Miccoli, P., Elisei, R., Capezzone, M., and Pacini, F. (2001). RET protein expression has no prognostic impact on the long-term outcome of papillary thyroid carcinoma. Eur. J. Endocrinol. 145, 599–604.
Belfiore, A., La Rosa, G. L., Padova, G., Sava, L., Ippolito, O., and Vigneri, R. (1987). The frequency of cold thyroid nodules and thyroid malignancies in patients from an iodine-deficient area. Cancer 60, 3096–3102.
Bounacer, A., Wicker, R., Caillou, B., Cailleux, A. F., Sarasin, A., Schlumberger, M., and Suarez, H. G. (1997). High prevalence of activating ret proto-oncogene rearrangements, in thyroid tumors from patients who had received external radiation. Oncogene 15, 1263–1273.
Braverman, L. E., and Utiger, R. D. (2005). Werner and Ingbar’s the Thyroid: A Fundamental and Clinical Text, 9th Edn. Philadelphia: Williams and Wilkins.
Cantara, S., Capezzone, M., Marchisotta, S., Capuano, S., Busonero, G., Toti, P., Di Santo, A., Caruso, G., Carli, A. F., Brilli, L., Montanaro, A., and Pacini, F. (2010). Impact of proto-oncogene mutation detection in cytological specimens from thyroid nodules improves the diagnostic accuracy of cytology. J. Clin. Endocrinol. Metab. 95, 1365–1369.
Cardis, E., Howe, G., Ron, E., Bebeshko, V., Bogdanova, T., Bouville, A., Carr, Z., Chumak, V., Davis, S., Demidchik, Y., Drozdovitch, V., Gentner, N., Gudzenko, N., Hatch, M., Ivanov, V., Jacob, P., Kapitonova, E., Kenigsberg, Y., Kesminiene, A., Kopecky, K. J., Kryuchkov, V., Loos, A., Pinchera, A., Reiners, C., Repacholi, M., Shibata, Y., Shore, R. E., Thomas, G., Tirmarche, M., Yamashita, S., and Zvonova, I. (2006). Cancer consequences of the Chernobyl accident: 20 years on. J. Radiol. Prot. 26, 127–140.
Caudill, C. M., Zhu, Z., Ciampi, R., Stringer, J. R., and Nikiforov, Y. E. (2005). Dose-dependent generation of RET/PTC in human thyroid cells after in vitro exposure to gamma-radiation: a model of carcinogenic chromosomal rearrangement induced by ionizing radiation. J. Clin. Endocrinol. Metab. 90, 2364–2369.
Cheung, C. C., Carydis, B., Ezzat, S., Bedard, Y. C., and Asa, S. L. (2001). Analysis of ret/PTC gene rearrangements refines the fine needle aspiration diagnosis of thyroid cancer. J. Clin. Endocrinol. Metab. 86, 2187–2190.
Chiappetta, G., Toti, P., Cetta, F., Giuliano, A., Pentimalli, F., Amendola, I., Lazzi, S., Monaco, M., Mazzuchelli, L., Tosi, P., Santoro, M., and Fusco, A. (2002). The RET/PTC oncogene is frequently activated in oncocytic thyroid tumors (Hurthle cell adenomas and carcinomas), but not in oncocytic hyperplastic lesions. J. Clin. Endocrinol. Metab. 87, 364–369.
Chua, E. L., Wu, W. M., Tran, K. T., Mccarthy, S. W., Lauer, C. S., Dubourdieu, D., Packham, N., O’Brien, C. J., Turtle, J. R., and Dong, Q. (2000). Prevalence and distribution of ret/ptc 1, 2, and 3 in papillary thyroid carcinoma in New Caledonia and Australia. J. Clin. Endocrinol. Metab. 85, 2733–2739.
Cinti, R., Yin, L., Ilc, K., Berger, N., Basolo, F., Cuccato, S., Giannini, R., Torre, G., Miccoli, P., Amati, P., Romeo, G., and Corvi, R. (2000). RET rearrangements in papillary thyroid carcinomas and adenomas detected by interphase FISH. Cytogenet. Cell Genet. 88, 56–61.
Cooper, D. S., Doherty, G. M., Haugen, B. R., Kloos, R. T., Lee, S. L., Mandel, S. J., Mazzaferri, E. L., Mciver, B., Pacini, F., Schlumberger, M., Sherman, S. I., Steward, D. L., and Tuttle, R. M. (2009). Revised American Thyroid Association management guidelines for patients with thyroid nodules and differentiated thyroid cancer. Thyroid 19, 1167–1214.
Cronkite, E. P., Bond, V. P., and Conard, R. A. (1995). Medical effects of exposure of human beings to fallout radiation from a thermonuclear explosion. Stem Cells 13(Suppl. 1), 49–57.
Davies, L., and Welch, H. G. (2006). Increasing incidence of thyroid cancer in the United States, 1973–2002. JAMA 295, 2164–2167.
De Lellis, R., Lloyd, R., Heitz, P., and Eng, C. (2003). The International Agency for Research on Cancer Pathology and Genetics of Tumours of Endocrine Organs (IARC WHO Classification of Tumours), Lyon.
Di Cristofaro, J., Vasko, V., Savchenko, V., Cherenko, S., Larin, A., Ringel, M. D., Saji, M., Marcy, M., Henry, J. F., Carayon, P., and De Micco, C. (2005). ret/PTC1 and ret/PTC3 in thyroid tumors from Chernobyl liquidators: comparison with sporadic tumors from Ukrainian and French patients. Endocr. Relat. Cancer 12, 173–183.
Duffy, B. J. Jr., and Fitzgerald, P. J. (1950). Cancer of the thyroid in children: a report of 28 cases. J. Clin. Endocrinol. Metab. 10, 1296–1308.
Elisei, R., Molinaro, E., Agate, L., Bottici, V., Masserini, L., Ceccarelli, C., Lippi, F., Grasso, L., Basolo, F., Bevilacqua, G., Miccoli, P., Di Coscio, G., Vitti, P., Pacini, F., and Pinchera, A. (2010). Are the clinical and pathological features of differentiated thyroid carcinoma really changed over the last 35 years? Study on 4187 patients from a single Italian institution to answer this question. J. Clin. Endocrinol. Metab. 95, 1516–1527.
Elisei, R., Romei, C., Vorontsova, T., Cosci, B., Veremeychik, V., Kuchinskaya, E., Basolo, F., Demidchik, E. P., Miccoli, P., Pinchera, A., and Pacini, F. (2001). RET/PTC rearrangements in thyroid nodules: studies in irradiated and not irradiated, malignant and benign thyroid lesions in children and adults. J. Clin. Endocrinol. Metab. 86, 3211–3216.
Enewold, L., Zhu, K., Ron, E., Marrogi, A. J., Stojadinovic, A., Peoples, G. E., and Devesa, S. S. (2009). Rising thyroid cancer incidence in the United States by demographic and tumor characteristics, 1980–2005. Cancer Epidemiol. Biomarkers Prev. 18, 784–791.
Farahati, J., Geling, M., Mader, U., Mortl, M., Luster, M., Muller, J. G., Flentje, M., and Reiners, C. (2004). Changing trends of incidence and prognosis of thyroid carcinoma in lower Franconia, Germany, from 1981–1995. Thyroid 14, 141–147.
Fenton, C. L., Lukes, Y., Nicholson, D., Dinauer, C. A., Francis, G. L., and Tuttle, R. M. (2000). The ret/PTC mutations are common in sporadic papillary thyroid carcinoma of children and young adults. J. Clin. Endocrinol. Metab. 85, 1170–1175.
Flavin, R., Jackl, G., Finn, S., Smyth, P., Ring, M., O’Regan, E., Cahill, S., Unger, K., Denning, K., Jinghuan, L., Aherne, S., Tallini, G., Gaffney, E., O’Leary, J. J., Zitzelsberger, H., and Sheils, O. (2009). RET/PTC rearrangement occurring in primary peritoneal carcinoma. Int. J. Surg. Pathol. 17, 187–197.
Franceschi, S., Boyle, P., Maisonneuve, P., La Vecchia, C., Burt, A. D., Kerr, D. J., and Macfarlane, G. J. (1993). The epidemiology of thyroid carcinoma. Crit. Rev. Oncog. 4, 25–52.
Fugazzola, L., Pilotti, S., Pinchera, A., Vorontsova, T.V., Mondellini, P., Bongarzone, I., Greco, A., Astakhova, L., Butti, M. G., Demidchik, E. P., Pacini, F., and Pierotti, M. A. (1995). Oncogenic rearrangements of the RET proto-oncogene in papillary thyroid carcinomas from children exposed to the Chernobyl nuclear accident. Cancer Res. 55, 5617–5620.
Fusco, A., Grieco, M., Santoro, M., Berlingieri, M. T., Pilotti, S., Pierotti, M. A., Della Porta, G., and Vecchio, G. (1987). A new oncogene in human thyroid papillary carcinomas and their lymph-nodal metastases. Nature 328, 170–172.
Gandhi, M., Dillon, L. W., Pramanik, S., Nikiforov, Y. E., and Wang, Y. H. (2010a). DNA breaks at fragile sites generate oncogenic RET/PTC rearrangements in human thyroid cells. Oncogene 29, 2272–2280.
Gandhi, M., Evdokimova, V., and Nikiforov, Y. E. (2010b). Mechanisms of chromosomal rearrangements in solid tumors: the model of papillary thyroid carcinoma. Mol. Cell. Endocrinol. 321, 36–43.
Gandhi, M., Medvedovic, M., Stringer, J. R., and Nikiforov, Y. E. (2006). Interphase chromosome folding determines spatial proximity of genes participating in carcinogenic RET/PTC rearrangements. Oncogene 25, 2360–2366.
Gharib, H. (2004). Changing trends in thyroid practice: understanding nodular thyroid disease. Endocr. Pract. 10, 31–39.
Gharib, H., Goellner, J. R., Zinsmeister, A. R., Grant, C. S., and Van Heerden, J. A. (1984). Fine-needle aspiration biopsy of the thyroid. The problem of suspicious cytologic findings. Ann. Intern. Med. 101, 25–28.
Goodhead, D. T. (1994). Initial events in the cellular effects of ionizing radiations: clustered damage in DNA. Int. J. Radiat. Biol. 65, 7–17.
Greco, A., Borrello, M. G., Miranda, C., Degl’Innocenti, D., and Pierotti, M. A. (2009). Molecular pathology of differentiated thyroid cancer. Q. J. Nucl. Med. Mol. Imaging. 53, 440–453.
Grieco, M., Santoro, M., Berlingieri, M. T., Melillo, R. M., Donghi, R., Bongarzone, I., Pierotti, M. A., Della Porta, G., Fusco, A., and Vecchio, G. (1990). PTC is a novel rearranged form of the ret proto-oncogene and is frequently detected in vivo in human thyroid papillary carcinomas. Cell 60, 557–563.
Guan, H., Ji, M., Bao, R., Yu, H., Wang, Y., Hou, P., Zhang, Y., Shan, Z., Teng, W., and Xing, M. (2009). Association of high iodine intake with the T1799A BRAF mutation in papillary thyroid cancer. J. Clin. Endocrinol. Metab. 94, 1612–1617.
Guerra, A., Sapio, M. R., Marotta, V., Campanile, E., Moretti, M. I., Deandrea, M., Motta, M., Limone, P. P., Fenzi, G., Rossi, G., and Vitale, M. (2011). Prevalence of RET/PTC rearrangement in benign and malignant thyroid nodules and its clinical application. Endocr. J. 58, 31–38.
Hamatani, K., Eguchi, H., Ito, R., Mukai, M., Takahashi, K., Taga, M., Imai, K., Cologne, J., Soda, M., Arihiro, K., Fujihara, M., Abe, K., Hayashi, T., Nakashima, M., Sekine, I., Yasui, W., Hayashi, Y., and Nakachi, K. (2008). RET/PTC rearrangements preferentially occurred in papillary thyroid cancer among atomic bomb survivors exposed to high radiation dose. Cancer Res. 68, 7176–7182.
Hieber, L., Huber, R., Bauer, V., Schaffner, Q., Braselmann, H., Thomas, G., Bogdanova, T., and Zitzelsberger, H. (2011). Chromosomal rearrangements in post-Chernobyl papillary thyroid carcinomas: evaluation by spectral karyotyping and automated interphase FISH. J. Biomed. Biotechnol. 2011, 693691.
Ishizaka, Y., Kobayashi, S., Ushijima, T., Hirohashi, S., Sugimura, T., and Nagao, M. (1991). Detection of retTPC/PTC transcripts in thyroid adenomas and adenomatous goiter by an RT-PCR method. Oncogene 6, 1667–1672.
Ito, T., Seyama, T., Iwamoto, K. S., Hayashi, T., Mizuno, T., Tsuyama, N., Dohi, K., Nakamura, N., and Akiyama, M. (1993). In vitro irradiation is able to cause RET oncogene rearrangement. Cancer Res. 53, 2940–2943.
Jhiang, S. M., and Mazzaferri, E. L. (1994). The ret/PTC oncogene in papillary thyroid carcinoma. J. Lab. Clin. Med. 123, 331–337.
Jin, L., Sebo, T. J., Nakamura, N., Qian, X., Oliveira, A., Majerus, J. A., Johnson, M. R., and Lloyd, R. V. (2006). BRAF mutation analysis in fine needle aspiration (FNA) cytology of the thyroid. Diagn. Mol. Pathol. 15, 136–143.
Kent, W. D., Hall, S. F., Isotalo, P. A., Houlden, R. L., George, R. L., and Groome, P. A. (2007). Increased incidence of differentiated thyroid carcinoma and detection of subclinical disease. CMAJ 177, 1357–1361.
Kitahara, C. M., Platz, E. A., Freeman, L. E., Hsing, A. W., Linet, M. S., Park, Y., Schairer, C., Schatzkin, A., Shikany, J. M., and Berrington De Gonzalez, A. (2011). Obesity and thyroid cancer risk among U.S. men and women: a pooled analysis of five prospective studies. Cancer Epidemiol. Biomarkers Prev. 20, 464–472.
Klugbauer, S., Lengfelder, E., Demidchik, E. P., and Rabes, H. M. (1995). High prevalence of RET rearrangement in thyroid tumors of children from Belarus after the Chernobyl reactor accident. Oncogene 11, 2459–2467.
Lam, A. K., Montone, K. T., Nolan, K. A., and Livolsi, V. A. (1998). Ret oncogene activation in papillary thyroid carcinoma: prevalence and implication on the histological parameters. Hum. Pathol. 29, 565–568.
Marchetti, I., Iervasi, G., Mazzanti, C. M., Lessi, F., Tomei, S., Naccarato, A. G., Aretini, P., Alberti, B., Di Coscio, G., and Bevilacqua, G. (2012). Detection of the BRAF(V600E) mutation in fine needle aspiration cytology of thyroid papillary microcarcinoma cells selected by manual macrodissection: an easy tool to improve the preoperative diagnosis. Thyroid 22, 292–298.
Marotta, V., Guerra, A., Sapio, M. R., and Vitale, M. (2011). RET/PTC rearrangement in benign and malignant thyroid diseases: a clinical standpoint. Eur. J. Endocrinol. 165, 499–507.
Mayr, B., Potter, E., Goretzki, P., Ruschoff, J., Dietmaier, W., Hoang-Vu, C., Dralle, H., and Brabant, G. (1998). Expression of Ret/PTC1, -2, -3, -delta3 and -4 in German papillary thyroid carcinoma. Br. J. Cancer 77, 903–906.
Mazzaferri, E. L. (1992). Thyroid cancer in thyroid nodules: finding a needle in the haystack. Am. J. Med. 93, 359–362.
Mizuno, T., Iwamoto, K. S., Kyoizumi, S., Nagamura, H., Shinohara, T., Koyama, K., Seyama, T., and Hamatani, K. (2000). Preferential induction of RET/PTC1 rearrangement by X-ray irradiation. Oncogene 19, 438–443.
Mochizuki, K., Kondo, T., Nakazawa, T., Iwashina, M., Kawasaki, T., Nakamura, N., Yamane, T., Murata, S., Ito, K., Kameyama, K., Kobayashi, M., and Katoh, R. (2010). RET rearrangements and BRAF mutation in undifferentiated thyroid carcinomas having papillary carcinoma components. Histopathology 57, 444–450.
Musholt, T. J., Musholt, P. B., Khaladj, N., Schulz, D., Scheumann, G. F., and Klempnauer, J. (2000). Prognostic significance of RET and NTRK1 rearrangements in sporadic papillary thyroid carcinoma. Surgery 128, 984–993.
Nagataki, S., Shibata, Y., Inoue, S., Yokoyama, N., Izumi, M., and Shimaoka, K. (1994). Thyroid diseases among atomic bomb survivors in Nagasaki. JAMA 272, 364–370.
Narayanan, P. K., Goodwin, E. H., and Lehnert, B. E. (1997). Alpha particles initiate biological production of superoxide anions and hydrogen peroxide in human cells. Cancer Res. 57, 3963–3971.
Nikiforov, Y. E. (2006). Radiation-induced thyroid cancer: what we have learned from Chernobyl. Endocr. Pathol. 17, 307–317.
Nikiforov, Y. E. (2009). “Thyroid tumors: classification and general consideration,” in Molecular Genetics of the Thyroid, eds P. W. Biddinger, Y. E. Nikiforov, and L. D. R. Thompson (Baltimore, MD: Lippincott Williams and Wilkins), 94–102.
Nikiforov, Y. E. (2011). Molecular diagnostics of thyroid tumors. Arch. Pathol. Lab. Med. 135, 569–577.
Nikiforov, Y. E., Rowland, J. M., Bove, K. E., Monforte-Munoz, H., and Fagin, J. A. (1997). Distinct pattern of ret oncogene rearrangements in morphological variants of radiation-induced and sporadic thyroid papillary carcinomas in children. Cancer Res. 57, 1690–1694.
Nikiforova, M. N., and Nikiforov, Y. E. (2009). Molecular diagnostics and predictors in thyroid cancer. Thyroid 19, 1351–1361.
Nikiforova, M. N., Stringer, J. R., Blough, R., Medvedovic, M., Fagin, J. A., and Nikiforov, Y. E. (2000). Proximity of chromosomal loci that participate in radiation-induced rearrangements in human cells. Science 290, 138–141.
Pacini, F., Schlumberger, M., Dralle, H., Elisei, R., Smit, J. W., and Wiersinga, W. (2006). European consensus for the management of patients with differentiated thyroid carcinoma of the follicular epithelium. Eur. J. Endocrinol. 154, 787–803.
Pellegriti, G., De Vathaire, F., Scollo, C., Attard, M., Giordano, C., Arena, S., Dardanoni, G., Frasca, F., Malandrino, P., Vermiglio, F., Previtera, D. M., D’Azzo, G., Trimarchi, F., and Vigneri, R. (2009). Papillary thyroid cancer incidence in the volcanic area of Sicily. J. Natl. Cancer Inst. 101, 1575–1583.
Pizzolanti, G., Russo, L., Richiusa, P., Bronte, V., Nuara, R. B., Rodolico, V., Amato, M. C., Smeraldi, L., Sisto, P. S., Nucera, M., Bommarito, A., Citarrella, R., Lo Coco, R., Cabibi, D., Lo Coco, A., Frasca, F., Gulotta, G., Latteri, M. A., Modica, G., Galluzzo, A., and Giordano, C. (2007). Fine-needle aspiration molecular analysis for the diagnosis of papillary thyroid carcinoma through BRAF V600E mutation and RET/PTC rearrangement. Thyroid 17, 1109–1115.
Powell, D. J. Jr., Russell, J., Nibu, K., Li, G., Rhee, E., Liao, M., Goldstein, M., Keane, W. M., Santoro, M., Fusco, A., and Rothstein, J. L. (1998). The RET/PTC3 oncogene: metastatic solid-type papillary carcinomas in murine thyroids. Cancer Res. 58, 5523–5528.
Puxeddu, E., Moretti, S., Elisei, R., Romei, C., Pascucci, R., Martinelli, M., Marino, C., Avenia, N., Rossi, E. D., Fadda, G., Cavaliere, A., Ribacchi, R., Falorni, A., Pontecorvi, A., Pacini, F., Pinchera, A., and Santeusanio, F. (2004). BRAF(V599E) mutation is the leading genetic event in adult sporadic papillary thyroid carcinomas. J. Clin. Endocrinol. Metab. 89, 2414–2420.
Puxeddu, E., Moretti, S., Giannico, A., Martinelli, M., Marino, C., Avenia, N., Cristofani, R., Farabi, R., Reboldi, G., Ribacchi, R., Pontecorvi, A., and Santeusanio, F. (2003). Ret/PTC activation does not influence clinical and pathological features of adult papillary thyroid carcinomas. Eur. J. Endocrinol. 148, 505–513.
Rago, T., Di Coscio, G., Basolo, F., Scutari, M., Elisei, R., Berti, P., Miccoli, P., Romani, R., Faviana, P., Pinchera, A., and Vitti, P. (2007). Combined clinical, thyroid ultrasound and cytological features help to predict thyroid malignancy in follicular and Hupsilonrthle cell thyroid lesions: results from a series of 505 consecutive patients. Clin. Endocrinol. (Oxf.) 66, 13–20.
Rhoden, K. J., Johnson, C., Brandao, G., Howe, J. G., Smith, B. R., and Tallini, G. (2004). Real-time quantitative RT-PCR identifies distinct c-RET, RET/PTC1 and RET/PTC3 expression patterns in papillary thyroid carcinoma. Lab. Invest. 84, 1557–1570.
Rhoden, K. J., Unger, K., Salvatore, G., Yilmaz, Y., Vovk, V., Chiappetta, G., Qumsiyeh, M. B., Rothstein, J. L., Fusco, A., Santoro, M., Zitzelsberger, H., and Tallini, G. (2006). RET/papillary thyroid cancer rearrangement in nonneoplastic thyrocytes: follicular cells of Hashimoto’s thyroiditis share low-level recombination events with a subset of papillary carcinoma. J. Clin. Endocrinol. Metab. 91, 2414–2423.
Romei, C., Ciampi, R., Faviana, P., Agate, L., Molinaro, E., Bottici, V., Basolo, F., Miccoli, P., Pacini, F., Pinchera, A., and Elisei, R. (2008). BRAFV600E mutation, but not RET/PTC rearrangements, is correlated with a lower expression of both thyroperoxidase and sodium iodide symporter genes in papillary thyroid cancer. Endocr. Relat. Cancer 15, 511–520.
Ron, E., Lunenfeld, B., Menczer, J., Blumstein, T., Katz, L., Oelsner, G., and Serr, D. (1987). Cancer incidence in a cohort of infertile women. Am. J. Epidemiol. 125, 780–790.
Saad, A., Falciglia, M., Steward, D. L., and Nikiforov, Y. E. (2004). Amiodarone-induced thyrotoxicosis and thyroid cancer: clinical, immunohistochemical, and molecular genetic studies of a case and review of the literature. Arch. Pathol. Lab. Med. 128, 807–810.
Salvatore, G., Giannini, R., Faviana, P., Caleo, A., Migliaccio, I., Fagin, J. A., Nikiforov, Y. E., Troncone, G., Palombini, L., Basolo, F., and Santoro, M. (2004). Analysis of BRAF point mutation and RET/PTC rearrangement refines the fine-needle aspiration diagnosis of papillary thyroid carcinoma. J. Clin. Endocrinol. Metab. 89, 5175–5180.
Santoro, M., Dathan, N. A., Berlingieri, M. T., Bongarzone, I., Paulin, C., Grieco, M., Pierotti, M. A., Vecchio, G., and Fusco, A. (1994). Molecular characterization of RET/PTC3; a novel rearranged version of the RETproto-oncogene in a human thyroid papillary carcinoma. Oncogene 9, 509–516.
Santoro, M., Rosati, R., Grieco, M., Berlingieri, M. T., D’Amato, G. L., De Franciscis, V., and Fusco, A. (1990). The ret proto-oncogene is consistently expressed in human pheochromocytomas and thyroid medullary carcinomas. Oncogene 5, 1595–1598.
Santoro, M., Sabino, N., Ishizaka, Y., Ushijima, T., Carlomagno, F., Cerrato, A., Grieco, M., Battaglia, C., Martelli, M. L., Paulin, C., Fabien, N., Sugimura, T., Fusco, A., and Nagao, M. (1993). Involvement of RET oncogene in human tumours: specificity of RET activation to thyroid tumours. Br. J. Cancer 68, 460–464.
Sapio, M. R., Guerra, A., Marotta, V., Campanile, E., Formisano, R., Deandrea, M., Motta, M., Limone, P. P., Fenzi, G., Rossi, G., and Vitale, M. (2011). High growth rate of benign thyroid nodules bearing RET/PTC rearrangements. J. Clin. Endocrinol. Metab. 96, E916–E919.
Schlumberger, M., and Pacini, F. (1999). “Medullary thyroid carcinoma,” in Thyroid Tumors (Paris: Nucleon), 267–269.
Sheils, O. M., O’Eary J, J., Uhlmann, V., Lattich, K., and Sweeney, E. C. (2000). ret/PTC-1 activation in Hashimoto thyroiditis. Int. J. Surg. Pathol. 8, 185–189.
Smida, J., Salassidis, K., Hieber, L., Zitzelsberger, H., Kellerer, A. M., Demidchik, E. P., Negele, T., Spelsberg, F., Lengfelder, E., Werner, M., and Bauchinger, M. (1999). Distinct frequency of ret rearrangements in papillary thyroid carcinomas of children and adults from Belarus. Int. J. Cancer 80, 32–38.
Spitz, M. R., Sider, J. G., Katz, R. L., Pollack, E. S., and Newell, G. R. (1988). Ethnic patterns of thyroid cancer incidence in the United States, 1973–1981. Int. J. Cancer 42, 549–553.
Sugg, S. L., Ezzat, S., Zheng, L., Freeman, J. L., Rosen, I. B., and Asa, S. L. (1999). Oncogene profile of papillary thyroid carcinoma. Surgery 125, 46–52.
Tallini, G., and Asa, S. L. (2001). RET oncogene activation in papillary thyroid carcinoma. Adv. Anat. Pathol. 8, 345–354.
Tallini, G., Santoro, M., Helie, M., Carlomagno, F., Salvatore, G., Chiappetta, G., Carcangiu, M. L., and Fusco, A. (1998). RET/PTC oncogene activation defines a subset of papillary thyroid carcinomas lacking evidence of progression to poorly differentiated or undifferentiated tumor phenotypes. Clin. Cancer Res. 4, 287–294.
Thomas, G. A., Bunnell, H., Cook, H. A., Williams, E. D., Nerovnya, A., Cherstvoy, E. D., Tronko, N. D., Bogdanova, T. I., Chiappetta, G., Viglietto, G., Pentimalli, F., Salvatore, G., Fusco, A., Santoro, M., and Vecchio, G. (1999). High prevalence of RET/PTC rearrangements in Ukrainian and Belarusian post-Chernobyl thyroid papillary carcinomas: a strong correlation between RET/PTC3 and the solid-follicular variant. J. Clin. Endocrinol. Metab. 84, 4232–4238.
Unger, K., Zitzelsberger, H., Salvatore, G., Santoro, M., Bogdanova, T., Braselmann, H., Kastner, P., Zurnadzhy, L., Tronko, N., Hutzler, P., and Thomas, G. (2004). Heterogeneity in the distribution of RET/PTC rearrangements within individual post-Chernobyl papillary thyroid carcinomas. J. Clin. Endocrinol. Metab. 89, 4272–4279.
Unger, K., Zurnadzhy, L., Walch, A., Mall, M., Bogdanova, T., Braselmann, H., Hieber, L., Tronko, N., Hutzler, P., Jeremiah, S., Thomas, G., and Zitzelsberger, H. (2006). RET rearrangements in post-Chernobyl papillary thyroid carcinomas with a short latency analysed by interphase FISH. Br. J. Cancer 94, 1472–1477.
Vejbjerg, P., Knudsen, N., Perrild, H., Carle, A., Laurberg, P., Pedersen, I. B., Rasmussen, L. B., Ovesen, L., and Jorgensen, T. (2007). Effect of a mandatory iodization program on thyroid gland volume based on individuals’ age, gender, and preceding severity of dietary iodine deficiency: a prospective, population-based study. J. Clin. Endocrinol. Metab. 92, 1397–1401.
Viglietto, G., Chiappetta, G., Martinez-Tello, F. J., Fukunaga, F. H., Tallini, G., Rigopoulou, D., Visconti, R., Mastro, A., Santoro, M., and Fusco, A. (1995). RET/PTC oncogene activation is an early event in thyroid carcinogenesis. Oncogene 11, 1207–1210.
Volpato, C. B., Martinez-Alfaro, M., Corvi, R., Gabus, C., Sauvaigo, S., Ferrari, P., Bonora, E., De Grandi, A., and Romeo, G. (2008). Enhanced sensitivity of the RET proto-oncogene to ionizing radiation in vitro. Cancer Res. 68, 8986–8992.
Ward, J. F. (1995). Radiation mutagenesis: the initial DNA lesions responsible. Radiat. Res. 142, 362–368.
Williams, D. (2002). Cancer after nuclear fallout: lessons from the Chernobyl accident. Nat. Rev. Cancer 2, 543–549.
Williams, D. (2008). Twenty years’ experience with post-Chernobyl thyroid cancer. Best Pract. Res. Clin. Endocrinol. Metab. 22, 1061–1073.
Winship, T., and Rosvoll, R. V. (1970). Cancer of the thyroid in children. Proc. Natl. Cancer Conf. 6, 677–681.
Wirtschafter, A., Schmidt, R., Rosen, D., Kundu, N., Santoro, M., Fusco, A., Multhaupt, H., Atkins, J. P., Rosen, M. R., Keane, W. M., and Rothstein, J. L. (1997). Expression of the RET/PTC fusion gene as a marker for papillary carcinoma in Hashimoto’s thyroiditis. Laryngoscope 107, 95–100.
Xing, M., Clark, D., Guan, H., Ji, M., Dackiw, A., Carson, K. A., Kim, M., Tufaro, A., Ladenson, P., Zeiger, M., and Tufano, R. (2009). BRAF mutation testing of thyroid fine-needle aspiration biopsy specimens for preoperative risk stratification in papillary thyroid cancer. J. Clin. Oncol. 27, 2977–2982.
Zhu, Z., Ciampi, R., Nikiforova, M. N., Gandhi, M., and Nikiforov, Y. E. (2006). Prevalence of RET/PTC rearrangements in thyroid papillary carcinomas: effects of the detection methods and genetic heterogeneity. J. Clin. Endocrinol. Metab. 91, 3603–3610.
Keywords: RET, RET/PTC, papillary thyroid cancer, oncogene
Citation: Romei C and Elisei R (2012) RET/PTC translocations and clinico-pathological features in human papillary thyroid carcinoma. Front. Endocrin. 3:54. doi: 10.3389/fendo.2012.00054
Received: 03 February 2012; Paper pending published: 03 March 2012;
Accepted: 26 March 2012; Published online: 11 April 2012.
Edited by:
Carmelo Nucera, Beth Israel Deaconess Medical Center/Harvard Medical School, USAReviewed by:
Mario Vitale, University of Salerno, ItalyOrla Sheils, University of Dublin, Trinity College, Ireland
Copyright: © 2012 Romei and Elisei. This is an open-access article distributed under the terms of the Creative Commons Attribution Non Commercial License, which permits non-commercial use, distribution, and reproduction in other forums, provided the original authors and source are credited.
*Correspondence: Rossella Elisei, Department of Endocrinology, University of Pisa, Via Paradisa 2, 56124 Pisa, Italy. e-mail:cmVsaXNlaUBlbmRvYy5tZWQudW5pcGkuaXQ=