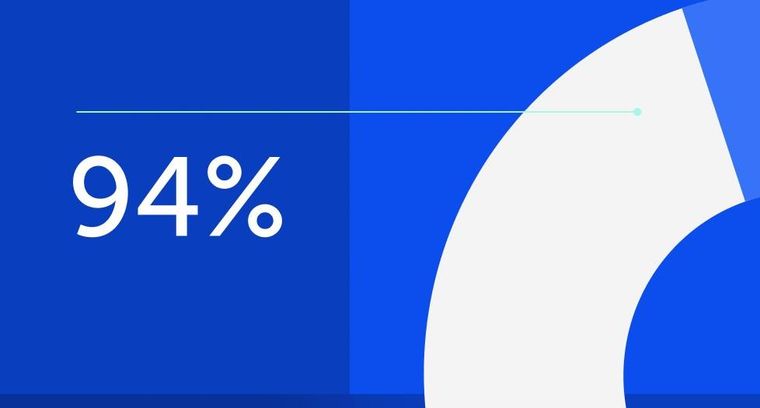
94% of researchers rate our articles as excellent or good
Learn more about the work of our research integrity team to safeguard the quality of each article we publish.
Find out more
REVIEW article
Front. Endocrinol., 22 February 2012
Sec. Systems Endocrinology
Volume 3 - 2012 | https://doi.org/10.3389/fendo.2012.00028
This article is part of the Research TopicEstrogenic control of hypothalamic GnRH neuronsView all 11 articles
Kisspeptin was originally found as a peptide product of Kiss1 gene and is now supposed to be an essential central regulator of reproduction in mammals. However, there is now a growing body of evidence to suggest that kiss2, the paralogous gene for kiss1, evolved in parallel during vertebrate lineage, and the kiss2 product also activates the GPR54 (kisspeptin receptor) signaling pathways. Therefore, it is now widely accepted that both kiss1 and kiss2 are the kisspeptin genes. Interestingly, either kiss1 or kiss2 or both have been lost during evolution in many vertebrate species, and the functional significance of kiss1 or kiss2 for the central regulation of reproduction is suggested to vary according to the species. Here, we argue that the steroid sensitivity of the kiss1 or kiss2 neurons has been well conserved during evolution among tetrapods and teleosts, and thus it may be the key to understanding the functional homologies of certain populations of kisspeptin (kiss1 or kiss2) neurons among different species of vertebrates. In the present review, we will first introduce recent advances in the study of steroid sensitive kiss1 and kiss2 systems in vertebrates and effects of peptide administrations in vivo. By comparing the similarities and differences between kiss1 and kiss2 of neuronal localization and sensitivity to gonadal steroids in various tetrapods and teleosts, we discuss the evolution of kisspeptin neuronal systems after gene duplication of ancestral kisspeptin genes to give rise to kiss1 and kiss2.
Recent studies have shown that kisspeptin plays an essential role in reproductive functions in mammals. Kisspeptin attracts particular attention, since previous reports have shown that the lack of kisspeptin receptors gene, GPR54, in both mice and humans, or of the ligand gene (Kiss1) in mice results in reproductive dysfunctions. More interestingly, the kisspeptin neurons have been shown to express estrogen receptor alpha (ERα), while the GnRH neurons express kisspeptin receptors, and kisspeptin depolarizes GnRH1 neurons in mice. These results suggest that they are not only involved in the sex steroid feedback but also are possible candidate for the “missing link” in the gonadotropin feedback control (Tena-Sempere, 2005; Smith, 2008).
On the other hand, fewer studies exist on the non-mammalian kisspeptin, and somewhat contradictory results among different species appear to confuse general conclusions about the functions of kisspeptin, especially in teleosts. This may be because of the fact that not a few studies in teleost kisspeptin have used pharmacological methods such as intracerebroventricular (ICV) or peripheral administration of kisspeptins. We should consider that the physiological functions of each kisspeptin neuron population may not be assessed only by such analyses, because the pharmacological administration may activate unexpected signaling pathways apart from the actual kisspeptin neuron networks. Therefore, the detailed information on the anatomy of the axonal projections and on the physiology and distribution of receptors are necessary before the administration studies. On the other hand, the kisspeptin neurons in certain brain nuclei show steroid sensitivity in all the animal species thus far examined across teleosts and tetrapods. Therefore, we may be able to correlate the functional properties of the different populations of kisspeptin neurons in various species by using the sex steroid sensitivity as more physiological criteria.
On the other hand, it has been generally accepted in evolutionary biology that genes duplicated from a single gene in the ancestral vertebrate undergo sub-functionalization, neo-functionalization, or non-functionalization (Ohno, 1970). Recent literature on the kisspeptin of non-mammalian species suggests that the kiss1 and kiss2 systems may have undergone such evolutionary processes. To understand the parallel evolution of these genes in the kisspeptin neuronal systems, we here propose that the steroid sensitivity helps to identify the functionally equivalent neuronal populations among different species, because the steroid sensitivity appears to be the evolutionarily well conserved feature of certain populations of the kisspeptin neurons. As will be argued below, the non-mammalian kisspeptin systems show a wealth of diversity of gene expression (kiss1 and/or kiss2) pattern in the brain and dynamic changes in expression according to the sex steroid milieu. Therefore, in spite of some kind of confusion in the kisspeptin studies of non-mammalian species, the biological study of kiss1 and kiss2, and of neurons that express these genes will provide us interesting insights into the general features of the kisspeptin systems in vertebrates. In addition to be an interesting model to understand the general evolutionary mechanisms of paralogous genes, the study of kisspeptin systems in non-mammalian vertebrates may lead us to find novel functions of kisspeptins, which may have been overlooked in previous studies using limited groups of mammalian species, which have globally lost Kiss2.
In the present review, we will introduce recent findings about kiss1 and kiss2 in vertebrates, mainly mammals and teleosts, and discuss their functions from various aspects, including cellular localizations, steroid sensitivity, and receptor distributions. Although there are a small number of studies that have been performed in non-mammalian tetrapods and vertebrates that emerged prior to the divergence of tetrapod from teleosts, we propose some general hypotheses about the evolution of kiss1 and kiss2 in the vertebrate lineage by comparing the two distinct groups, teleosts and tetrapods (see Table 1 for nomenclature of kisspeptin genes and peptides)1.
In 2008, kiss1 gene was isolated in non-mammalian species (Kanda et al., 2008; van Aerle et al., 2008). Then in 2009, kiss2 was cloned from teleosts (Kitahashi et al., 2009) and amphibians (Lee et al., 2009) as the gene responsible for the peptide that showed a similar amino acid sequence to Kiss1. Because some ligand–receptor interaction studies showed that both Kiss2 and Kiss1 activate the kisspeptin receptor signaling pathways in Xenopus tropicalis, zebrafish (Lee et al., 2009), bullfrog (Moon et al., 2009), and goldfish (Li et al., 2009), Kiss2 has been recognized as one of the “kisspeptin” peptides.
Felip et al. (2009) performed a sophisticated synteny analysis of kiss1 and kiss2 genes in vertebrate species. In their report, it was strongly suggested that kiss1 and kiss2 are duplicated together with some surrounding genes such as golt1a/b, plekha5/6, pik3c2b/cg, and etnk1/2. They also discovered that only one co-ortholog for each pair was found in a chordate (Ciona), suggesting that these genes including kiss1/kiss2 resulted from a gene duplication event that occurred at least “after” the divergence of urochordates and vertebrates.
Concerning the duplication of genes in general, it is strongly suggested that the common ancestors of the present vertebrates underwent whole genome duplication (WGD) twice (2R hypothesis; Ohno, 1970) that is evidenced by results of recent genome sequencing in vertebrate and urochordate species (Putnam et al., 2008). In addition, the ancestors of teleosts are suggested to have undergone one additional WGD [third-round (3R)-WGD; reviewed in Sato and Nishida, 2010]. Here, because amphibians and lampreys have both kiss1 and kiss2, these homologs are considered to have duplicated in the ancestral vertebrate before the emergence of lamprey, as suggested by Felip et al. (2009). During evolution, some species such as the puffer fish, stickleback (Felip et al., 2009; Kitahashi et al., 2009; Li et al., 2009; Shahjahan et al., 2010; Yang et al., 2010), and some perciform fish (Felip et al., 2009; Mechaly et al., 2011) seem to have lost the kiss1 gene. On the other hand, in tetrapods, most mammals have lost Kiss2 during evolution. Because the platypus possesses both Kiss1 and Kiss2, the loss of Kiss2 must have occurred at least after the divergence of monotreme and other mammals. On the other hand, because the opossum is reported to lack Kiss2 in its genome database (Felip et al., 2009), we may predict that the loss of Kiss2 in the mammalian lineage occurred before the divergence of marsupials and placentarians (Figure 1).
Figure 1. Summary of the subtypes of ligand and receptor genes for kisspeptin systems in the genome along the vertebrate lineage. kiss1 and kiss2 are supposed to be duplicated before the emergence of lamprey, and probably due to the whole genome duplication of the ancestral vertebrate. It is supposed that kiss2 and gpr54-2 were lost in marsupial and placental mammals after the divergence from the monotreme during mammalian evolution. It should be noted that some teleost species have lost gpr54-1, but no teleosts have lost gpr54-2, suggesting the significance of gpr54-2 in teleosts, which is opposite to the case in the mammalian lineage. It is also consistent with the higher level of expression of gpr54-2 compared to that of gpr54-1 in teleost brains. The loss of kiss1 (blue) or kiss2 (red) is indicated by asterisks.
Recent studies that are based on the genome sequence data suggested that most of the duplicated genes are subsequently lost rapidly after duplication (Brunet et al., 2006; Sato et al., 2009). Thus, for the teleost specific 3R-WGD, it is suggested that both kiss1 and kiss2 duplicated once again to give rise to four genes, and two of them were likely lost immediately in the early teleost lineage.
Taken together, this conservative organization of loci that contain kiss1/kiss2 observed widely in vertebrates strongly suggest that kiss1 and kiss2 genes were duplicated at the locus level, and as Um et al. (2010) suggested, this duplication probably occurred in two rounds of WGD (1R-WGD and 2R-WGD; reviewed in Sato and Nishida, 2010) event (Figure 1).
After the identification of Kiss1 and Kiss2, several ligand–receptor interaction studies have shown that both Kiss1 and Kiss2 activate the kisspeptin receptor signaling pathways in goldfish (Li et al., 2009), zebrafish, Xenopus (Lee et al., 2009), orange spotted grouper (Shi et al., 2010), and bullfrog (Moon et al., 2009), suggesting that Kiss1 and Kiss2 bind to the same kisspeptin receptor in vertebrates. Although the activation of the PKC or the cAMP pathway by Kiss1 and Kiss2 is slightly different in each species, it is generally accepted that both Kiss1 and Kiss2 are ligands for GPR54 in vertebrates. Thus, both Kiss1 and Kiss2, the peptide products of sister genes, can function as kisspeptins.
The kisspeptin receptor in mammals has been referred to either as GPR54 (Seminara et al., 2003) or Kiss1r (Gottsch et al., 2009). In many species studied so far, it has often been shown that more than one ligand and more than one receptor for kisspeptin bind to one another promiscuously (Lee et al., 2009; Li et al., 2009). Since recent studies also show the promiscuity of kisspeptin and other RF amide peptides (Lyubimov et al., 2010), it may be also possible that certain peptides other than Kiss1 and Kiss2 activate the kisspeptin receptor signaling pathways. Thus, although we once proposed a systematic nomenclature for kisspeptin receptor (Akazome et al., 2010), we refer to the kisspeptin receptors as GPR54-1 and 2 as proposed in Lee et al. (2009) in the present review.
In mammals, many studies have shown that peripheral or ICV injection of kisspeptin evokes LH secretion in rodents (Gottsch et al., 2004; Irwig et al., 2004; Matsui et al., 2004; Navarro et al., 2004, 2005a,b), sheep (Messager et al., 2005), monkey (Shahab et al., 2005; Plant et al., 2006; Seminara et al., 2006), and human (Dhillo et al., 2005). Moreover, it has been reported that the kisspeptin stimulates GnRH neurons both directly and indirectly via interneurons (Han et al., 2005; Dumalska et al., 2008; Liu et al., 2008; Pielecka-Fortuna et al., 2008; Zhang et al., 2008; Pielecka-Fortuna and Moenter, 2010). However, the number of studies in animals that possess both Kiss1 and Kiss2 is limited. In such animals, there is no evidence for direct kisspeptin GnRH regulation on GnRH release, and the results of peripheral administration of Kiss1 and Kiss2 decapeptides are not consistent with one another. Administration of the core decapeptide of Kiss2, but not of Kiss1, significantly increased LHβ and FSHβ subunit mRNA expression in the zebrafish pituitary (Kitahashi et al., 2009). Kiss2 also induced LH and FSH secretion in sea bass with higher potency than Kiss1 decapeptide (Felip et al., 2009). In contrast, intraperitoneal administration of Kiss2 peptide in goldfish did not increase serum LH levels, although the administration of Kiss1 peptide did (Li et al., 2009). Thus, there appears to be a difference amongst species in the relative potencies of Kiss1 and Kiss2 for facilitating LH and FSH secretion and in the time of LH/FSH rise after administration of kisspeptins. Therefore, the induction of LH/FSH secretion by kisspeptins (Kiss1 or Kiss2) in non-mammalian vertebrates needs more experimental evidence to be accepted as a general notion. Moreover, because peripheral administration does not reflect the actual axonal projection and the receptor distribution of kisspeptin neurons in the brain, cellular-level studies, such as electrophysiology, are necessary to determine the action sites of kisspeptins and the possible interactions between kisspeptin and GnRH neurons. Therefore, electrophysiological examination of effects of either Kiss1 or Kiss2 on GnRH neurons in species that possess both genes is important for the understanding of such peptidergic systems that arose from the gene duplication.
In the rodent anteroventral periventricular nucleus (AVPV) and sheep preoptic area (POA), kiss1 mRNA expression in Kiss1 neurons are upregulated, whereas those in the arcuate nucleus (ARC) are downregulated, by gonadal steroids (Irwig et al., 2004;Smith et al., 2005a,b, 2007, 2008; Revel et al., 2006; Adachi et al., 2007; Ansel et al., 2010). Although there is a discrepancy that ewe ARC Kiss1 show higher expression in the breeding season in spite of the negative regulation of gonadal steroids (Estrada et al., 2006; Smith, 2009), it is generally accepted that AVPV/POA Kiss1 neurons are positively regulated, and ARC Kiss1 neurons are negatively regulated by the gonadal steroids. These properties are widely recognized in mammalian species.
In mice, it has been shown that GnRH neurons do not express ERα (reviewed in Herbison and Pape, 2001), which are essential for normal reproductive functions (Couse et al., 2003; Dorling et al., 2003; Wintermantel et al., 2006). Thus, Herbison concludes that estrogen acts indirectly on GnRH neurons to bring about their activation (Herbison, 2008). This missing link in mice was found to be explained by steroid sensitive kisspeptin neurons, because kisspeptin directly depolarizes GnRH neurons in mice (Han et al., 2005; Dumalska et al., 2008; Liu et al., 2008; Pielecka-Fortuna et al., 2008; Zhang et al., 2008; Pielecka-Fortuna and Moenter, 2010), which is expected to facilitate firing activities and then GnRH release. Thus, the role of kisspeptin neurons in steroid feedback is one of the most interesting topics in the study of kisspeptin neuron systems.
In non-mammalian vertebrates, the localization of kiss1/kiss2 neurons is reported in medaka (Kanda et al., 2008; Mitani et al., 2010), zebrafish (Kitahashi et al., 2009), Xenopus (Lee et al., 2009), seabream (Shimizu et al., 2012), and puffer fish (Kanda et al., 2010). Among them, medaka and goldfish are the only species whose steroid sensitivity has been examined experimentally. Therefore, recent data shown by us in medaka will be described below. In addition, we will also describe results in the goldfish, which gives us insights into the evolutionary aspects of kiss1/kiss2 genes.
In situ hybridization studies have shown the localization of kiss1 and kiss2 neurons in the medaka brain (Kanda et al., 2008; Kitahashi et al., 2009; Mitani et al., 2010). The kiss1 neurons are localized in the hypothalamic nuclei, nucleus ventralis tuberis (NVT) and nucleus posterioris periventricularis (NPPv), as well as in an extrahypothalamic nucleus, habenula. On the other hand, the kiss2 neurons are localized in nucleus recessus lateralis (NRL). Among these kiss1 and kiss2 neurons, only the kiss1 neurons in NVT show prominent steroid sensitivity in their kisspeptin gene expression. Ovariectomy (OVX) dramatically reduced kiss1 expression in NVT neurons, which were recovered by subsequent estrogen replacement. Because our unpublished data showed that 11 keto-testosterone, a non-aromatizable androgen, did not recover this decrease at all, the steroid feedback activity seems to be mediated by estrogen receptor. It was also shown that NVT kiss1 neurons are the only kisspeptin neuron population that shows expressional variations according to the breeding states. Moreover, double insitu hybridization analysis has shown that kiss1 neurons in NVT express ERα. In addition to ERα, NVT kiss1 neurons in medaka express ERβ as well (Mitani et al., 2010), which is similar to kiss1 neurons in mice (Smith et al., 2005a).
Interestingly, in addition to steroid sensitivity, NVT kiss1 neurons also show sexual dimorphism in number (Kanda et al., 2008). In contrast to the sexual dimorphism found in rodents, in which females have more AVPV Kiss1 neurons than males (Clarkson and Herbison, 2006; Smith et al., 2006; Adachi et al., 2007; Kauffman et al., 2007), male medaka show significantly more NVT kiss1 neurons than females. Comparative analyses in much more vertebrate species should be necessary for understanding the organization of sexual dimorphism and the functional significance of male- or female-predominant expressions.
In zebrafish, localization of kiss1 and kiss2 neurons was analyzed by in situ hybridization (Kitahashi et al., 2009; Servili et al., 2011). The kiss1 neurons in the zebrafish brain are distributed in the habenula and periventricular hypothalamus, while the kiss2 neurons are distributed in the posterior tuberal nucleus, the periventricular hypothalamic nucleus, and parvocellular preoptic nucleus (Kitahashi et al., 2009; Servili et al., 2011). In the juvenile zebrafish, it was demonstrated that estradiol administration increases the mRNA expression of kiss1, kiss2, and gpr54-2 in the brain (Servili et al., 2011). Among them, Servili et al. focused on kiss2 neurons in the dorsal hypothalamus (Hd), caudal hypothalamus (Hc), and anterior tuberal nucleus (ATN), and demonstrated that all of them showed higher kiss2 expression after estradiol administration in juvenile fish. Because it may not be physiological to administrate estrogen to juveniles, it may be rather difficult to interpret these results. In spite of this, it is intriguing to investigate the homologous relationships of these neurons to the medaka steroid sensitive kiss1 neurons in NVT and steroid insensitive kiss2 neurons in NRL. However, as the hypothalamic structures vary even among teleosts, and no clear experimental evidence for the nucleus-specific steroid sensitivity in adults has been shown in any fish except medaka, further examination of the effects of gonadal steroids on the teleost kisspeptin neurons using ovariectomy should be necessary.
Therefore, we recently performed kiss1 and kiss2 in situ hybridization in the goldfish, because the goldfish is rather easily amenable to ovariectomy, and it belongs to the same Cypriniformes as the zebrafish. We found a prominent expression of kiss2 in POA, unlike results in zebrafish, and found that the POA kiss2 neurons show clear steroid sensitivity (Kanda et al., 2012). In the adult goldfish in the breeding condition, the kiss1 neurons are localized in the habenula, whereas the kiss2 neurons are located in nucleus lateralis tuberis (NLT), NRL, and POA. Among these neurons, the POA kiss2 neurons decreased in number after OVX, and the reduction was recovered by estrogen implant. It strongly suggests that POA kiss2 neurons are upregulated by ovarian estrogen, which is similar to the AVPV/POA kiss1 neurons in mammals. The discussion on the homology and the evolutionary hypothesis derived there from will be described in detail later in this review.
From the genome database analysis, puffer fish are supposed to possess only kiss2 expressing neurons, because they have lost kiss1 at the genome level. Shahjahan et al. (2010) took advantage of the seasonally breeding grass puffer and showed changes in the expression levels of kiss2/gpr54-2 genes together with the gonadosomatic index (GSI) during the spawning period. Here, because of the absence of kiss1 neurons, the kiss2 neurons in certain brain area are supposed to subserve the kisspeptin functions in this species.
Recently, we analyzed the localization of kiss2 neurons in juvenile green puffer fish by in situ hybridization and found that they are expressed in the hypothalamic nucleus NRL and the POA (Kanda et al., 2010), which is similar to the results in the zebrafish (Kitahashi et al., 2009; Servili et al., 2011). Unfortunately, it is technically difficult to raise green puffer to breeding conditions, and future studies using puffer fish that are capable of breeding to full maturity in aquarium tanks will be interesting.
In addition to this observation, it was recently shown that kiss2 expression is increased during the pre-spawning season (late spermatogenesis stage in male, and early vitellogenesis stage in female) in club mackerel (Selvaraj et al., 2010). Further expression analyses in some teleost species may lead us to find some general expressional variations of kisspeptin genes in the seasonal breeders.
There are many species differences in the functions of kiss1 and kiss2 neurons in vertebrates. The most extreme example lies between kiss1-lacking puffer fish and KISS2-lacking human. Even within the teleost species that express both kiss1 and kiss2, there are obvious species differences. For example, in medaka, only the NVT kiss1 neurons show steroid sensitivity, whereas there is no such kiss1 neuron in the hypothalamus of zebrafish, and, instead, many kiss2 neurons are localized in the hypothalamus.
Medaka kiss2 neurons are localized in NRL, where kiss2 neurons are also localized in zebrafish. Although Servili et al. (2011) proposed a possibility of functional similarity between the steroid sensitive medaka NVT kiss1 neurons and some of the zebrafish kiss2 neurons in the ventral hypothalamus, the zebrafish kiss2 neurons appear to contain neurons equivalent to the medaka kiss2 neurons and some other populations of neurons. It should be interesting to search for experimental evidence for such homologies. In situ hybridization and immunohistochemistry using some other fish species may give us clues to further understanding of the functional homology and evolution of these sister gene-expressing neuron systems. Experimental analysis on the effects of gonadal steroids by gonadectomy in various non-mammalian species should be very helpful to discuss true functional or morphological homologies, because the steroid sensitivity well characterizes the property of each nucleus, but such studies have been performed only in a small number of species such as medaka and goldfish.
As described above, kiss1 and kiss2 in the present vertebrate species are suggested to be the sister genes, which originate from the gene duplication event in the ancestral vertebrate. Furthermore, it is highly possible that they were duplicated during the genome-wide duplication events. Because these sister genes possess family genes in their loci, they are considered to have duplicated at least at the locus level, regardless of whether the WGD event made these sister genes or not. Thus, just after the duplication, kiss1 and kiss2 must have had completely the same sequence in their open reading frame as well as the regulatory sequence, and they must have been co-expressed in the same location at first. During evolution, one or even both of them was silenced in some species, and their location of expression and function diverged. Moreover, as seen between medaka and zebrafish/goldfish, the general functions or relative functional importance of kiss1 and kiss2 for the central regulation of reproduction are different among species; their functions are considered to have diverged among species during evolution (Kanda et al., 2012).
Interestingly, the inversion of the importance of kiss1 and kiss2 for the central regulation of reproduction occur rather commonly among different species (see the previous section). On the other hand, this phenomenon never occurred during the evolution of the hypophysiotropic GnRH system; the Cyprinids and Salmonids have lost gnrh1, but this lack seems to have been functionally compensated by the remaining genes (Okubo and Nagahama, 2008). We suppose that the functional conservativeness of the GnRH systems may be due to the fact that loss of the gnrh function, especially the hypophysiotropic one, would lead to severe reproductive dysfunctions (Cattanach et al., 1977) or to a failure of normal sexual maturation (Wu et al., 2006).
We have been routinely performing in situ hybridization of kiss1 and kiss2 genes (Kanda et al., 2008; Mitani et al., 2010) and find it more difficult to detect them compared to gnrh2 or gnrh3 (Gopinath et al., 2004; Okubo et al., 2006; Palevitch et al., 2007), which empirically suggests the lower level of expression of kiss1/kiss2, especially during the developmental stages. Moreover, the reported lack of gpr54 expression in the hypophysiotropic GnRH1 neurons in some teleosts (Grone et al., 2010; and our unpublished observation) suggests that the physiological functions and their mechanisms are somewhat more diverged in the vertebrate kiss1/kiss2 systems, compared to the rather conservative GnRH systems. Thus, unlike the GnRH systems, in which the inversion of physiological functions between the hypothalamic hypophysiotropic (GnRH1) and extrahypothalamic neuromodulatory GnRH systems (GnRH2/GnRH3) has never been reported, the kiss1 and kiss2 systems are the ones having rather promiscuous ligand–receptor relationships and are supposed to be more adaptive during evolution; they appear to have avoided extreme selection pressure. Thus, the understanding of the evolution of kiss1 and kiss2 neuron systems may lead to a model for the study of general evolutionary mechanism of peptidergic neurons in the absence of strong selection pressure.
The distribution of kiss1 and kiss2 neurons in representative animal species and in the presumptive common ancestral animals are shown in Figure 2. It has been already suggested that POA Kiss1 neurons in sheep, AVPV Kiss1 neurons in rodents, and PeN Kiss1 neurons in pig are homologous in mammals (Smith, 2009; Tomikawa et al., 2010). These forebrain kisspeptin neurons in mammals, which show steroid sensitivity, are Kiss1 neurons, although they have not been examined in monotreme, which have both Kiss1 and Kiss2 genes. On the other hand, the goldfish steroid sensitive POA kisspeptin neurons express kiss2 mRNA (Kanda et al., 2012). This may sound a little bit strange at first sight. However, the results in Xenopus laevis may give us a hint for the evolutionary mechanism. The Xenopus POA kisspeptin neurons express kiss2 mRNA (Lee et al., 2009). Thus, all the vertebrate forebrain populations of kisspeptin neurons studied so far, except for mammals, are Kiss2 neurons. We therefore hypothesize that both ancestral teleosts and ancestral tetrapods expressed kiss2 in POA. We further hypothesize that in mammals Kiss1 is expressed in the neurons that are homologous to Xenopus POA kiss2 neurons; this conversion of Kiss2 to Kiss1 may have occurred because of the loss of Kiss2 after the divergence of the ancestor of the present mammals from the monotreme. Thus, we assume that, during mammalian evolution, the loss of Kiss2 gene triggered the expression of Kiss1 in mammalian POA. An alternative possibility is that Kiss1 neurons emerged in the POA before they lost Kiss2.
Figure 2. Schematic illustrations for the distribution of kiss1 and kiss2 neurons in vertebrate brains, including some hypotheses. Open circles indicate kiss1, and filled triangles indicate kiss2 neurons. Circles/triangles in red are kiss1/kiss2 neurons that are steroid sensitive. Because kiss1 and kiss2 are duplicated sister genes, they are considered to have been co-expressed in the same neurons in the common ancestor of teleosts and tetrapods. Considering the fact that both amphibians and teleosts express kiss2 in POA, the ancestral teleosts and ancestral tetrapods are supposed to have expressed kiss2. Because Kiss2 was lost in the mammalian lineage, we hypothesize that Kiss1 began to be expressed where Kiss2 used to be expressed, to compensate for the loss of Kiss2 during mammalian evolution.
However, the overlapped functions tend to be lost rapidly during evolution in general. We suppose that the loss of Kiss2 triggered the expression of Kiss1 in the same neurons because of the similar regulatory sequence between Kiss1 and Kiss2. In other words, the loss of Kiss2 might have canceled the expressional inhibition of Kiss1 in POA kisspeptin neurons. Such a phenomenon is called the “genetic robustness” and has been only examined in the duplicated genes of C. elegans and yeast (Gu et al., 2003; Conant and Wagner, 2004), or has been observed in human genetic disease (Hsiao and Vitkup, 2008). In these studies, it was shown that the duplicated genes, which have a similar copy as the sister genes, tend to cause genetic disease less frequently compared to the singleton genes. Thus, it is supposed that closely related genes, such as sister genes, often compensate for the gene loss, and we suppose that Kiss2 gene loss and emergence of Kiss1 neurons in mammalian lineage coincided with each other. To our knowledge, there has been no report on the occurrence of such “genetic robustness” in the highly complicated neuronal systems of vertebrates. Although it is difficult to use monotreme for experimental use, it is intriguing to study the localization of monotreme Kiss1 and Kiss2 neurons. Thus, the evolutionary studies of kiss1 and kiss2 genes should serve as a good model system for the study of evolution of sub-functionalized sister genes in the central nervous system in general.
In mammals, the hypothalamic Kiss1 neurons in ARC are steroid sensitive as described above. Because OVX increases the Kiss1 expression, and estrogen or testosterone nullifies this increase in ARC, the ARC Kiss1 neurons are considered to be negatively regulated by ovarian steroids.
In species other than mammals, the only report about the gonadal steroid regulation on the hypothalamic kisspeptin neurons is on medaka Kiss1 neurons (Kanda et al., 2008; Mitani et al., 2010). Here, unlike the mammalian ARC Kiss1 neurons, their expression is enhanced, instead of inhibited, by ovarian steroids. Thus, one may argue against the homology of these nuclei in teleosts and mammals. We should note, however, that positive or negative regulation can be rather easily inverted by the composition of co-expressed transcription factors, such as Sp1 and Sp3 (Li et al., 2007). Thus, the difference in positive/negative regulation itself should not disprove the homology. Moreover, NVT also contains some other types of neurons involved in the homeostatic regulation like the mammalian ARC, supporting the evidence for the possible homology of these nuclei. However, because the teleosts lack the median eminence, the characteristic projection of ARC Kiss1 neurons to the median eminence, which is usually observed in mammals, cannot be observed. Instead, the medaka NVT Kiss1 neurons are shown to project to the POA. Experimental analysis of steroid sensitivity and the axonal projections of the hypothalamic Kiss1 neurons in other animals are necessary for the understanding of the evolutionary origin and possible homology of the hypothalamic Kiss1 neurons in other animals are necessary for the understanding of the evolutionary origin and possible homology of the hypothalamic Kiss1 neurons.
In medaka, gene expression of the NRL kiss2 neurons was shown to be independent of breeding conditions), and these kiss2 neurons were not shown to express ERα (Mitani et al., 2010). Thus, the neurons that are homologous to the steroid insensitive teleost hypothalamic (NRL) kiss2 neurons may be absent in mammals. Further comparative studies among various species of teleosts and amphibians may solve the problem of whether steroid insensitive hypothalamic kiss2 neurons are specific to the teleosts or just lost in mammals.
The kisspeptin neurons in the habenula have been reported only in teleosts (Kitahashi et al., 2009; Mitani et al., 2010; Servili et al., 2011), and they express kiss1 in all those species. The projection of habenular neurons have been well studied by classical neuroanatomical experiments as well as by using recent molecular genetic techniques (Aizawa et al., 2005; Gamse et al., 2005). Consistent with results of these studies of habenular projections, the habenular Kiss1 neurons in zebrafish (Servili et al., 2011) and medaka (Kanda et al., unpublished data) appears to project to the interpeduncular nucleus (IPN) via fasciculus retroflexus. As gpr54-2, one subtype of gpr54 genes in teleosts, is reported to be expressed in IPN, and gpr54-1, another subtype, is co-expressed in habenula in zebrafish, Servili et al. (2011) suggested the existence of the autocrine regulation of habenula (via gpr54-1) and the target neurons in IPN (via gpr54-2). Because there is no other report on the existence of kiss1 neurons in the tetrapod habenula, it remains to be known whether the habenular kiss1 was originally expressed in the ancestral vertebrate and subsequently lost in the tetrapod lineage, or solely began to be expressed in the teleost lineage. In order to answer this question, expression analysis of kiss1 in phylogenetically significant species such as lungfish and polypterus are ongoing in our laboratory.
In this review article, we reviewed recent findings of kisspeptin neurons in vertebrates, by comparing studies in mammalian species and non-mammalian species, mainly teleosts. To date, only a handful of studies have shown kisspeptin neurons’ functional significance in the central regulation of reproduction in species other than mammals. Because kisspeptin receptor gpr54-2 as well as gpr54-1 are expressed in POA and hypothalamus, but not in GnRH1 neurons in some species, kisspeptin’s novel function other than the central regulation of reproduction (Kadokawa et al., 2008; Szawka et al., 2010; Yang et al., 2010; Luque et al., 2011) will also be an interesting topic in the future studies.
As reviewed above, the steroid sensitivity of kisspeptin neurons has been reported in a wide variety of species including non-mammalian vertebrates. Although the mechanisms are not well studied in the non-mammalian species, the steroid sensing feature and the related functions are highly conserved throughout vertebrates. Although the natural selection of functions of paralogous kiss1 and kiss2 genes are complicated, the study of evolutionary process of these sister genes may give clues to understand the evolution of the central nervous system after genome duplication in general. Here, the kisspeptin neuron system may be not only the regulator of reproductive/homeostatic functions in vertebrates but also the pioneer toward further understanding of the evolution of the central nervous system functions.
The authors declare that the research was conducted in the absence of any commercial or financial relationships that could be construed as a potential conflict of interest.
We thank Drs. Kataaki Okubo and Yasuhisa Akazome of the University at Tokyo for critical reading of and helpful advice for this manuscript. We also thank Ms. Tiffany Pang at Yale University for critically reading the draft, Drs. Yukuto Sato at National Institute of Genetics, and Tomohiro Osugi of Waseda University for insightful discussion. This work was supported by Grants-in-Aid from the JSPS (20-10112) to Shinji Kanda, and JSPS (20247005) and PROBRAIN of Japan to Yoshitaka Oka.
Adachi, S., Yamada, S., Takatsu, Y., Matsui, H., Kinoshita, M., Takase, K., Sugiura, H., Ohtaki, T., Matsumoto, H., Uenoyama, Y., Tsukamura, H., Inoue, K., and Maeda, K. (2007). Involvement of anteroventral periventricular metastin/kisspeptin neurons in estrogen positive feedback action on luteinizing hormone release in female rats. J. Reprod. Dev. 53, 367–378.
Aizawa, H., Bianco, I. H., Hamaoka, T., Miyashita, T., Uemura, O., Concha, M. L., Russell, C., Wilson, S. W., and Okamoto, H. (2005). Laterotopic representation of left-right information onto the dorso-ventral axis of a zebrafish midbrain target nucleus. Curr. Biol. 15, 238–243.
Akazome, Y., Kanda, S., Okubo, K., and Oka, Y. (2010). Functional and evolutionary insights into vertebrate kisspeptin systems from studies of fish brain. J. Fish Biol. 76, 161–182.
Ansel, L., Bolborea, M., Bentsen, A. H., Klosen, P., Mikkelsen, J. D., and Simonneaux, V. (2010). Differential regulation of kiss1 expression by melatonin and gonadal hormones in male and female Syrian hamsters. J. Biol. Rhythms 25, 81–91.
Brunet, F. G., Roest Crollius, H., Paris, M., Aury, J. M., Gibert, P., Jaillon, O., Laudet, V., and Robinson-Rechavi, M. (2006). Gene loss and evolutionary rates following whole-genome duplication in teleost fishes. Mol. Biol. Evol. 23, 1808–1816.
Cattanach, B. M., Iddon, C. A., Charlton, H. M., Chiappa, S. A., and Fink, G. (1977). Gonadotropin-releasing hormone deficiency in a mutant mouse with hypogonadism. Nature 269, 338–340.
Clarkson, J., and Herbison, A. E. (2006). Postnatal development of kisspeptin neurons in mouse hypothalamus; sexual dimorphism and projections to gonadotropin-releasing hormone neurons. Endocrinology 147, 5817–5825.
Conant, G. C., and Wagner, A. (2004). Duplicate genes and robustness to transient gene knock-downs in Caenorhabditis elegans. Proc. Biol. Sci. 271, 89–96.
Couse, J. F., Yates, M. M., Walker, V. R., and Korach, K. S. (2003). Characterization of the hypothalamic-pituitary-gonadal axis in estrogen receptor (ER) null mice reveals hypergonadism and endocrine sex reversal in females lacking ERalpha but not ERbeta. Mol. Endocrinol. 17, 1039–1053.
Dhillo, W. S., Chaudhri, O. B., Patterson, M., Thompson, E. L., Murphy, K. G., Badman, M. K., Mcgowan, B. M., Amber, V., Patel, S., Ghatei, M. A., and Bloom, S. R. (2005). Kisspeptin-54 stimulates the hypothalamic-pituitary gonadal axis in human males. J. Clin. Endocrinol. Metab. 90, 6609–6615.
Dorling, A. A., Todman, M. G., Korach, K. S., and Herbison, A. E. (2003). Critical role for estrogen receptor alpha in negative feedback regulation of gonadotropin-releasing hormone mRNA expression in the female mouse. Neuroendocrinology 78, 204–209.
Dumalska, I., Wu, M., Morozova, E., Liu, R., Van Den Pol, A., and Alreja, M. (2008). Excitatory effects of the puberty-initiating peptide kisspeptin and group I metabotropic glutamate receptor agonists differentiate two distinct subpopulations of gonadotropin-releasing hormone neurons. J. Neurosci. 28, 8003–8013.
Estrada, K. M., Clay, C. M., Pompolo, S., Smith, J. T., and Clarke, I. J. (2006). Elevated KiSS-1 expression in the arcuate nucleus prior to the cyclic preovulatory gonadotropin-releasing hormone/lutenising hormone surge in the ewe suggests a stimulatory role for kisspeptin in oestrogen-positive feedback. J. Neuroendocrinol. 18, 806–809.
Felip, A., Zanuy, S., Pineda, R., Pinilla, L., Carrillo, M., Tena-Sempere, M., and Gomez, A. (2009). Evidence for two distinct KiSS genes in non-placental vertebrates that encode kisspeptins with different gonadotropin-releasing activities in fish and mammals. Mol. Cell. Endocrinol. 312, 61–71.
Gamse, J. T., Kuan, Y. S., Macurak, M., Brosamle, C., Thisse, B., Thisse, C., and Halpern, M. E. (2005). Directional asymmetry of the zebrafish epithalamus guides dorsoventral innervation of the midbrain target. Development 132, 4869–4881.
Gopinath, A., Andrew Tseng, L., and Whitlock, K. E. (2004). Temporal and spatial expression of gonadotropin releasing hormone (GnRH) in the brain of developing zebrafish (Danio rerio). Gene Expr. Patterns 4, 65–70.
Gottsch, M. L., Clifton, D. K., and Steiner, R. A. (2009). From KISS1 to kisspeptins: an historical perspective and suggested nomenclature. Peptides 30, 4–9.
Gottsch, M. L., Cunningham, M. J., Smith, J. T., Popa, S. M., Acohido, B. V., Crowley, W. F., Seminara, S., Clifton, D. K., and Steiner, R. A. (2004). A role for kisspeptins in the regulation of gonadotropin secretion in the mouse. Endocrinology 145, 4073–4077.
Grone, B. P., Maruska, K. P., Korzan, W. J., and Fernald, R. D. (2010). Social status regulates kisspeptin receptor mRNA in the brain of Astatotilapia burtoni. Gen. Comp. Endocrinol. 169, 98–107.
Gu, Z., Steinmetz, L. M., Gu, X., Scharfe, C., Davis, R. W., and Li, W. H. (2003). Role of duplicate genes in genetic robustness against null mutations. Nature 421, 63–66.
Han, S. K., Gottsch, M. L., Lee, K. J., Popa, S. M., Smith, J. T., Jakawich, S. K., Clifton, D. K., Steiner, R. A., and Herbison, A. E. (2005). Activation of gonadotropin-releasing hormone neurons by kisspeptin as a neuroendocrine switch for the onset of puberty. J. Neurosci. 25, 11349–11356.
Herbison, A. E. (2008). Estrogen positive feedback to gonadotropin-releasing hormone (GnRH) neurons in the rodent: the case for the rostral periventricular area of the third ventricle (RP3V). Brain Res. Rev. 57, 277–287.
Herbison, A. E., and Pape, J. R. (2001). New evidence for estrogen receptors in gonadotropin-releasing hormone neurons. Front. Neuroendocrinol. 22, 292–308.
Hsiao, T. L., and Vitkup, D. (2008). Role of duplicate genes in robustness against deleterious human mutations. PLoS Genet. 4, e1000014. doi:10.1371/journal.pgen.1000014
Irwig, M. S., Fraley, G. S., Smith, J. T., Acohido, B. V., Popa, S. M., Cunningham, M. J., Gottsch, M. L., Clifton, D. K., and Steiner, R. A. (2004). Kisspeptin activation of gonadotropin releasing hormone neurons and regulation of KiSS-1 mRNA in the male rat. Neuroendocrinology 80, 264–272.
Kadokawa, H., Suzuki, S., and Hashizume, T. (2008). Kisspeptin-10 stimulates the secretion of growth hormone and prolactin directly from cultured bovine anterior pituitary cells. Anim. Reprod. Sci. 105, 404–408.
Kanda, S., Akazome, Y., Matsunaga, T., Yamamoto, N., Yamada, S., Tsukamura, H., Maeda, K., and Oka, Y. (2008). Identification of KiSS-1 product kisspeptin and steroid-sensitive sexually dimorphic kisspeptin neurons in medaka (Oryzias latipes). Endocrinology 149, 2467–2476.
Kanda, S., Akazome, Y., Mitani, Y., Zempo, B., Yamamoto, E., and Oka, Y. (2010). Analysis of paralogous kisspeptin (kiss1/kiss2) systems in vertebrates using teleosts lacking kiss1. Soc. Neurosci. abstr. 594.7.
Kanda, S., Karigo, T., and Oka, Y. (2012). Steroid sensitive kiss2 neurons in the goldfish: evolutionary insights into the duplicate kisspeptin gene-expressing neurons. J. Neuroendocrinol. (in press).
Kauffman, A. S., Gottsch, M. L., Roa, J., Byquist, A. C., Crown, A., Clifton, D. K., Hoffman, G. E., Steiner, R. A., and Tena-Sempere, M. (2007). Sexual differentiation of Kiss1 gene expression in the brain of the rat. Endocrinology 148, 1774–1783.
Kitahashi, T., Ogawa, S., and Parhar, I. S. (2009). Cloning and expression of kiss2 in the zebrafish and medaka. Endocrinology 150, 821–831.
Lee, Y. R., Tsunekawa, K., Moon, M. J., Um, H. N., Hwang, J. I., Osugi, T., Otaki, N., Sunakawa, Y., Kim, K., Vaudry, H., Kwon, H. B., Seong, J. Y., and Tsutsui, K. (2009). Molecular evolution of multiple forms of kisspeptins and GPR54 receptors in vertebrates. Endocrinology 150, 2837–2846.
Li, D., Mitchell, D., Luo, J., Yi, Z., Cho, S. G., Guo, J., Li, X., Ning, G., Wu, X., and Liu, M. (2007). Estrogen regulates KiSS1 gene expression through estrogen receptor alpha and SP protein complexes. Endocrinology 148, 4821–4828.
Li, S., Zhang, Y., Liu, Y., Huang, X., Huang, W., Lu, D., Zhu, P., Shi, Y., Cheng, C. H., Liu, X., and Lin, H. (2009). Structural and functional multiplicity of the kisspeptin/GPR54 system in goldfish (Carassius auratus). J. Endocrinol. 201, 407–418.
Liu, X., Lee, K., and Herbison, A. E. (2008). Kisspeptin excites gonadotropin-releasing hormone neurons through a phospholipase C/calcium-dependent pathway regulating multiple ion channels. Endocrinology 149, 4605–4614.
Luque, R. M., Cordoba-Chacon, J., Gahete, M. D., Navarro, V. M., Tena-Sempere, M., Kineman, R. D., and Castano, J. P. (2011). Kisspeptin regulates gonadotroph and somatotroph function in nonhuman primate pituitary via common and distinct signaling mechanisms. Endocrinology 152, 957–966.
Lyubimov, Y., Engstrom, M., Wurster, S., Savola, J. M., Korpi, E. R., and Panula, P. (2010). Human kisspeptins activate neuropeptide FF2 receptor. Neuroscience 170, 117–122.
Matsui, H., Takatsu, Y., Kumano, S., Matsumoto, H., and Ohtaki, T. (2004). Peripheral administration of metastin induces marked gonadotropin release and ovulation in the rat. Biochem. Biophys. Res. Commun. 320, 383–388.
Mechaly, A. S., Vinas, J., and Piferrer, F. (2011). Gene structure analysis of kisspeptin-2 (Kiss2) in the Senegalese sole (Solea senegalensis): characterization of two splice variants of Kiss2, and novel evidence for metabolic regulation of kisspeptin signaling in non-mammalian species. Mol. Cell. Endocrinol. 339, 14–24.
Messager, S., Chatzidaki, E. E., Ma, D., Hendrick, A. G., Zahn, D., Dixon, J., Thresher, R. R., Malinge, I., Lomet, D., Carlton, M. B., Colledge, W. H., Caraty, A., and Aparicio, S. A. (2005). Kisspeptin directly stimulates gonadotropin-releasing hormone release via G protein-coupled receptor 54. Proc. Natl. Acad. Sci. U.S.A. 102, 1761–1766.
Mitani, Y., Kanda, S., Akazome, Y., Zempo, B., and Oka, Y. (2010). Hypothalamic Kiss1 but not Kiss2 neurons are involved in estrogen feedback in medaka (Oryzias latipes). Endocrinology 151, 1751–1759.
Moon, J. S., Lee, Y. R., Oh, D. Y., Hwang, J. I., Lee, J. Y., Kim, J. I., Vaudry, H., Kwon, H. B., and Seong, J. Y. (2009). Molecular cloning of the bullfrog kisspeptin receptor GPR54 with high sensitivity to Xenopus kisspeptin. Peptides 30, 171–179.
Navarro, V. M., Castellano, J. M., Fernandez-Fernandez, R., Barreiro, M. L., Roa, J., Sanchez-Criado, J. E., Aguilar, E., Dieguez, C., Pinilla, L., and Tena-Sempere, M. (2004). Developmental and hormonally regulated messenger ribonucleic acid expression of KiSS-1 and its putative receptor, GPR54, in rat hypothalamus and potent luteinizing hormone-releasing activity of KiSS-1 peptide. Endocrinology 145, 4565–4574.
Navarro, V. M., Castellano, J. M., Fernandez-Fernandez, R., Tovar, S., Roa, J., Mayen, A., Barreiro, M. L., Casanueva, F. F., Aguilar, E., Dieguez, C., Pinilla, L., and Tena-Sempere, M. (2005a). Effects of KiSS-1 peptide, the natural ligand of GPR54, on follicle-stimulating hormone secretion in the rat. Endocrinology 146, 1689–1697.
Navarro, V. M., Castellano, J. M., Fernandez-Fernandez, R., Tovar, S., Roa, J., Mayen, A., Nogueiras, R., Vazquez, M. J., Barreiro, M. L., Magni, P., Aguilar, E., Dieguez, C., Pinilla, L., and Tena-Sempere, M. (2005b). Characterization of the potent luteinizing hormone-releasing activity of KiSS-1 peptide, the natural ligand of GPR54. Endocrinology 146, 156–163.
Okubo, K., and Nagahama, Y. (2008). Structural and functional evolution of gonadotropin-releasing hormone in vertebrates. Acta Physiol. (Oxf.) 193, 3–15.
Okubo, K., Sakai, F., Lau, E. L., Yoshizaki, G., Takeuchi, Y., Naruse, K., Aida, K., and Nagahama, Y. (2006). Forebrain gonadotropin-releasing hormone neuronal development: insights from transgenic medaka and the relevance to X-linked Kallmann syndrome. Endocrinology 147, 1076–1084.
Palevitch, O., Kight, K., Abraham, E., Wray, S., Zohar, Y., and Gothilf, Y. (2007). Ontogeny of the GnRH systems in zebrafish brain: in situ hybridization and promoter-reporter expression analyses in intact animals. Cell Tissue Res. 327, 313–322.
Pielecka-Fortuna, J., Chu, Z., and Moenter, S. M. (2008). Kisspeptin acts directly and indirectly to increase gonadotropin-releasing hormone neuron activity and its effects are modulated by estradiol. Endocrinology 149, 1979–1986.
Pielecka-Fortuna, J., and Moenter, S. M. (2010). Kisspeptin increases gamma-aminobutyric acidergic and glutamatergic transmission directly to gonadotropin-releasing hormone neurons in an estradiol-dependent manner. Endocrinology 151, 291–300.
Plant, T. M., Ramaswamy, S., and Dipietro, M. J. (2006). Repetitive activation of hypothalamic G protein-coupled receptor 54 with intravenous pulses of kisspeptin in the juvenile monkey (Macaca mulatta) elicits a sustained train of gonadotropin-releasing hormone discharges. Endocrinology 147, 1007–1013.
Putnam, N. H., Butts, T., Ferrier, D. E., Furlong, R. F., Hellsten, U., Kawashima, T., Robinson-Rechavi, M., Shoguchi, E., Terry, A., Yu, J. K., Benito-Gutierrez, E. L., Dubchak, I., Garcia-Fernandez, J., Gibson-Brown, J. J., Grigoriev, I. V., Horton, A. C., De Jong, P. J., Jurka, J., Kapitonov, V. V., Kohara, Y., Kuroki, Y., Lindquist, E., Lucas, S., Osoegawa, K., Pennacchio, L. A., Salamov, A. A., Satou, Y., Sauka-Spengler, T., Schmutz, J., Shin, I. T., Toyoda, A., Bronner-Fraser, M., Fujiyama, A., Holland, L. Z., Holland, P. W., Satoh, N., and Rokhsar, D. S. (2008). The amphioxus genome and the evolution of the chordate karyotype. Nature 453, 1064–1071.
Revel, F. G., Saboureau, M., Masson-Pevet, M., Pevet, P., Mikkelsen, J. D., and Simonneaux, V. (2006). Kisspeptin mediates the photoperiodic control of reproduction in hamsters. Curr. Biol. 16, 1730–1735.
Sato, Y., Hashiguchi, Y., and Nishida, M. (2009). Temporal pattern of loss/persistence of duplicate genes involved in signal transduction and metabolic pathways after teleost-specific genome duplication. BMC Evol. Biol. 9, 127. doi:10.1186/1471-2148-9-127
Sato, Y., and Nishida, M. (2010). Teleost fish with specific genome duplication as unique models of vertebrate evolution. Environ. Biol. Fishes 88, 169–188.
Selvaraj, S., Kitano, H., Fujinaga, Y., Ohga, H., Yoneda, M., Yamaguchi, A., Shimizu, A., and Matsuyama, M. (2010). Molecular characterization, tissue distribution, and mRNA expression profiles of two Kiss genes in the adult male and female chub mackerel (Scomber japonicus) during different gonadal stages. Gen. Comp. Endocrinol. 169, 28–38.
Seminara, S. B., Dipietro, M. J., Ramaswamy, S., Crowley, W. F. Jr., and Plant, T. M. (2006). Continuous human metastin 45-54 infusion desensitizes G protein-coupled receptor 54-induced gonadotropin-releasing hormone release monitored indirectly in the juvenile male Rhesus monkey (Macaca mulatta): a finding with therapeutic implications. Endocrinology 147, 2122–2126.
Seminara, S. B., Messager, S., Chatzidaki, E. E., Thresher, R. R., Acierno, J. S. Jr., Shagoury, J. K., Bo-Abbas, Y., Kuohung, W., Schwinof, K. M., Hendrick, A. G., Zahn, D., Dixon, J., Kaiser, U. B., Slaugenhaupt, S. A., Gusella, J. F., O’Rahilly, S., Carlton, M. B., Crowley, W. F. Jr., Aparicio, S. A., and Colledge, W. H. (2003). The GPR54 gene as a regulator of puberty. N. Engl. J. Med. 349, 1614–1627.
Servili, A., Le Page, Y., Leprince, J., Caraty, A., Escobar, S., Parhar, I. S., Seong, J. Y., Vaudry, H., and Kah, O. (2011). Organization of two independent kisspeptin systems derived from evolutionary-ancient kiss genes in the brain of zebrafish. Endocrinology 152, 1527–1540.
Shahab, M., Mastronardi, C., Seminara, S. B., Crowley, W. F., Ojeda, S. R., and Plant, T. M. (2005). Increased hypothalamic GPR54 signaling: a potential mechanism for initiation of puberty in primates. Proc. Natl. Acad. Sci. U.S.A. 102, 2129–2134.
Shahjahan, M., Motohashi, E., Doi, H., and Ando, H. (2010). Elevation of Kiss2 and its receptor gene expression in the brain and pituitary of grass puffer during the spawning season. Gen. Comp. Endocrinol. 169, 48–57.
Shi, Y., Zhang, Y., Li, S., Liu, Q., Lu, D., Liu, M., Meng, Z., Cheng, C. H., Liu, X., and Lin, H. (2010). Molecular identification of the Kiss2/Kiss1ra system and its potential function during 17alpha-methyltestosterone-induced sex reversal in the orange-spotted grouper, Epinephelus coioides. Biol. Reprod. 83, 63–74.
Shimizu, Y., Tomikawa, J., Hirano, K., Nanikawa, Y., Akazome, Y., Kanda, S., Kazeto, Y., Okuzawa, K., Uenoyama, Y., Ohkura, S., Tsukamura, H., Maeda, K., Gen, K., Oka, Y., and Yamamoto, N. (2012). Central distribution of kiss2 neurons and peri-pubertal changes in their expression in the brain of male and female red seabream Pagrus major. Gen. Comp. Endocrinol. 175, 432–442.
Smith, J. T. (2008). Kisspeptin signalling in the brain: steroid regulation in the rodent and ewe. Brain Res. Rev. 57, 288–298.
Smith, J. T. (2009). Sex steroid control of hypothalamic Kiss1 expression in sheep and rodents: comparative aspects. Peptides 30, 94–102.
Smith, J. T., Clay, C. M., Caraty, A., and Clarke, I. J. (2007). KiSS-1 messenger ribonucleic acid expression in the hypothalamus of the ewe is regulated by sex steroids and season. Endocrinology 148, 1150–1157.
Smith, J. T., Clifton, D. K., and Steiner, R. A. (2006). Regulation of the neuroendocrine reproductive axis by kisspeptin-GPR54 signaling. Reproduction 131, 623–630.
Smith, J. T., Coolen, L. M., Kriegsfeld, L. J., Sari, I. P., Jaafarzadehshirazi, M. R., Maltby, M., Bateman, K., Goodman, R. L., Tilbrook, A. J., Ubuka, T., Bentley, G. E., Clarke, I. J., and Lehman, M. N. (2008). Variation in kisspeptin and RFamide-related peptide (RFRP) expression and terminal connections to gonadotropin-releasing hormone neurons in the brain: a novel medium for seasonal breeding in the sheep. Endocrinology 149, 5770–5782.
Smith, J. T., Cunningham, M. J., Rissman, E. F., Clifton, D. K., and Steiner, R. A. (2005a). Regulation of Kiss1 gene expression in the brain of the female mouse. Endocrinology 146, 3686–3692.
Smith, J. T., Dungan, H. M., Stoll, E. A., Gottsch, M. L., Braun, R. E., Eacker, S. M., Clifton, D. K., and Steiner, R. A. (2005b). Differential regulation of KiSS-1 mRNA expression by sex steroids in the brain of the male mouse. Endocrinology 146, 2976–2984.
Szawka, R. E., Ribeiro, A. B., Leite, C. M., Helena, C. V., Franci, C. R., Anderson, G. M., Hoffman, G. E., and Anselmo-Franci, J. A. (2010). Kisspeptin regulates prolactin release through hypothalamic dopaminergic neurons. Endocrinology 151, 3247–3257.
Tena-Sempere, M. (2005). Hypothalamic KiSS-1: the missing link in gonadotropin feedback control? Endocrinology 146, 3683–3685.
Tomikawa, J., Homma, T., Tajima, S., Shibata, T., Inamoto, Y., Takase, K., Inoue, N., Ohkura, S., Uenoyama, Y., Maeda, K., and Tsukamura, H. (2010). Molecular characterization and estrogen regulation of hypothalamic KISS1 gene in the pig. Biol. Reprod. 82, 313–319.
Um, H. N., Han, J. M., Hwang, J. I., Hong, S. I., Vaudry, H., and Seong, J. Y. (2010). Molecular coevolution of kisspeptins and their receptors from fish to mammals. Ann. N. Y. Acad. Sci. 1200, 67–74.
van Aerle, R., Kille, P., Lange, A., and Tyler, C. R. (2008). Evidence for the existence of a functional Kiss1/Kiss1 receptor pathway in fish. Peptides 29, 57–64.
Wintermantel, T. M., Campbell, R. E., Porteous, R., Bock, D., Grone, H. J., Todman, M. G., Korach, K. S., Greiner, E., Perez, C. A., Schutz, G., and Herbison, A. E. (2006). Definition of estrogen receptor pathway critical for estrogen positive feedback to gonadotropin-releasing hormone neurons and fertility. Neuron 52, 271–280.
Wu, S., Page, L., and Sherwood, N. M. (2006). A role for GnRH in early brain regionalization and eye development in zebrafish. Mol. Cell. Endocrinol. 257–258, 47–64.
Yang, B., Jiang, Q., Chan, T., Ko, W. K., and Wong, A. O. (2010). Goldfish kisspeptin: molecular cloning, tissue distribution of transcript expression, and stimulatory effects on prolactin, growth hormone and luteinizing hormone secretion and gene expression via direct actions at the pituitary level. Gen. Comp. Endocrinol. 165, 60–71.
Keywords: kisspeptin, kiss1, kiss2, evolution, estrogen, steroid feedback, GnRH
Citation: Kanda S and Oka Y (2012) Evolutionary insights into the steroid sensitive kiss1 and kiss2 neurons in the vertebrate brain. Front. Endocrin. 3:28. doi: 10.3389/fendo.2012.00028
Received: 08 November 2011; Accepted: 06 February 2012;
Published online: 22 February 2012.
Edited by:
Henryk Urbanski, Oregon National Primate Research Center, USAReviewed by:
Andre Scherag, University of Duisburg–Essen, GermanyCopyright: © 2012 Kanda and Oka. This is an open-access article distributed under the terms of the Creative Commons Attribution Non Commercial License, which permits non-commercial use, distribution, and reproduction in other forums, provided the original authors and source are credited.
*Correspondence: Yoshitaka Oka, Department of Biological Sciences, Graduate School of Science, The University of Tokyo, 7-3-1 Hongo, Bunkyo, Tokyo 113-0033, Japan. e-mail:b2theUBiaW9sLnMudS10b2t5by5hYy5qcA==
Disclaimer: All claims expressed in this article are solely those of the authors and do not necessarily represent those of their affiliated organizations, or those of the publisher, the editors and the reviewers. Any product that may be evaluated in this article or claim that may be made by its manufacturer is not guaranteed or endorsed by the publisher.
Research integrity at Frontiers
Learn more about the work of our research integrity team to safeguard the quality of each article we publish.